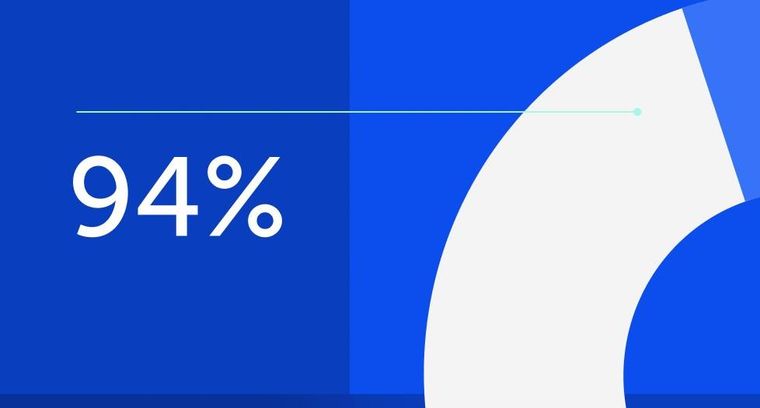
94% of researchers rate our articles as excellent or good
Learn more about the work of our research integrity team to safeguard the quality of each article we publish.
Find out more
REVIEW article
Front. Immunol., 23 August 2022
Sec. Cancer Immunity and Immunotherapy
Volume 13 - 2022 | https://doi.org/10.3389/fimmu.2022.954235
This article is part of the Research TopicThe Mechanism and Novel Strategies of Overcoming Resistance of Hematological Malignancies to CAR T-cell KillingView all 6 articles
Chimeric antigen receptor (CAR) T cell therapy has revolutionized the therapeutic landscape of haematological malignancies. However, resistance and relapse remain prominent limitations, and they are related to the limited persistence and efficacy of CAR T cells, downregulation or loss of tumour antigens, intrinsic resistance of tumours to death signalling, and immune suppressive microenvironment. Rational combined modality treatments are regarded as a promising strategy to further unlock the antitumor potential of CAR T cell therapy, which can be applied before CAR T cell infusion as a conditioning regimen or in ex vivo culture settings as well as concomitant with or after CAR T cell infusion. In this review, we summarize the combinatorial strategies, including chemotherapy, radiotherapy, haematopoietic stem cell transplantation, targeted therapies and other immunotherapies, in an effort to further enhance the effectiveness of this impressive therapy and benefit more patients.
Chimeric antigen receptor (CAR) T cell therapy is one of the major breakthroughs in the field of cancer immunotherapy. Remarkably, it has greatly improved the prognosis of haematological malignancies, and six CAR T cell products have been approved by the U.S. Food and Drug Administration (FDA) for relapsed/refractory B cell non-Hodgkin lymphoma (R/R B-NHL), R/R B cell acute lymphoblastic leukaemia (B-ALL), and R/R multiple myeloma (MM) (Table 1). Although CAR T cell products are commercially available, the issues of primary resistance and relapse remain. In B-ALL, 80% of patients can achieve CR after CD19 CAR T cell therapy, whereas approximately 30-50% of patients relapse, the majority within 1 year after infusion (13). The primary efficacy of CAR T cells in B-NHL and MM is comparatively inferior, with 54% and 44.8% CR, respectively (18, 19). There is still significant room to improve the efficacy of CAR T cell therapy.
Laboratory optimization mainly focuses on CAR engineering and searching for new targets (20). At the same time, increasing efforts have been made to harness other oncological treatments along with CAR T cell therapy in pursuit of synergistic antitumor effects. The modalities and timing of combination therapies are diverse. After apheresis and before CAR T cell infusion, the combination with bridging therapies and lymphodepletion aims at preparing patients with low tumour burden and creating a favourable microenvironment for CAR T cells. Simultaneously, CAR T cells are produced, and during this process pharmacological agents can be added in culture settings to produce potent T cells for adoptive transfer. Infusion of CAR T cells ensues, and this is when the interactions between CAR T cells and combined agents should be deliberately considered if a concurrent or consolidative treatment is going to be conducted to avoid the impaired fitness of CAR T cells. The main combinatorial strategies include traditional haematological cancer treatment, such as chemotherapy, radiotherapy, haematopoietic stem cell transplantation (HSCT) and small molecule-based targeted therapies. In addition, coupling CAR T cell therapy with other immunotherapies is under intense investigation. Herein, we summarize the available evidence and clinical experience to date on different combination strategies, hoping to provide insight to optimize applications of CAR T cell therapy.
The resistance and relapse of CAR T cell therapy are the result of interactions among CAR T cells, tumour cells and the tumour microenvironment (TME) (Figure 1). The poor persistence of CAR T cells is the most important reason for antigen-positive relapse. The fitness of T cells collected from patients is likely to be devastated by tumour and frontline treatments, resulting in insufficient CAR T cell quantity and poor quality (21). In addition, sustained antigenic stimulation in vivo tends to cause exhaustion of CAR T cells by transcriptomic and epigenetic modulation, whose features include impaired persistence and cytotoxicity, upregulation of inhibitory receptors, metabolic changes and increased apoptosis. CAR molecule can also mediate antigen-independent tonic signalling (22). Another mechanism for less persistent CAR T cells in vivo can be immune rejection of the murine-derived single-chain variable fragment (scFv), which is adopted by most clinically available CAR T cell products (23). The activation-induced cell death (AICD) effect also leads to irreversible clearance of CAR T cells (24).
Figure 1 Challenges of efficacy of CAR T cell therapy. Limited efficacy of CAR T cell therapy in haematological malignancies can be attributed to poor persistence of CAR T cells, antigen modulation and intrinsic resistance to death signalling of tumour cells and immunosuppressive microenvironment.
In terms of tumour cells, it is common to modulate antigen expression and escape CAR T cell recognition by various mechanisms. CD19-negative relapse has been studied most widely, and its mechanisms include alternative splicing, immune selection, lineage switching, trogocytosis, epitope masking and methylation silencing (25). MM relapse from B cell maturation antigen (BCMA) CAR T cell therapy occurs through the cleavage of the extracellular domain of BCMA by γ-secretase (GS), a universally expressed intramembrane protease (26). Moreover, biallelic deletion and trogocytosis can also contribute to BCMA antigen escape (27). Sometimes, there is no requirement for complete depletion of antigen expression, and downregulation of the expression of other antigens on haematological malignancies, such as CD22 and CD33, can also achieve resistance (28, 29). On the other hand, tumour cells can resist immune killing via intrinsic antiapoptotic pathways. Tumour cells downregulate the expression of death receptors, such as FasL and TNF-related apoptosis-inducing ligand (TRAIL), to become insensitive to the cytotoxicity of CAR T cells (30). They can also increase the expression of endogenous anti-apoptotic proteins such as B cell lymphoma-2 (Bcl-2) and inhibitor of apoptosis proteins (IAPs), resulting in damaged sensitivity to CAR T cell killing (31).
The immunosuppressive microenvironment also plays a role in attenuating the potency and persistence of CAR T cells. Although there are still few studies in the field of haematological malignancies, the critical effects of some critical immunosuppressive cells, such as myeloid-derived suppressor cells (MDSCs), tumour-associated macrophages (TAMs) and regulatory T cells (Tregs), immune checkpoints and soluble molecules, such as indoleamine 2,3-dioxygenase (IDO) and adenosine and inhibitory cytokines, on the efficacy of CAR T cell therapy have been gradually elucidated (32). Together, it is the complex interactions among these three components that lead to primary resistance and relapse of CAR T cell therapy and provide targets for combination therapies, aiming at directly eradiating tumour cells as well as improving CAR T cell killing effects (Figure 2).
Figure 2 Mechanisms of combination strategies with CAR T cell therapy. The combined agents mainly focus on three aspects to optimize antitumor effects, including tumour cells, CAR T cells and the TME. For CAR T cells, attention is given to targeting different pathways to prevent terminal differentiation and exhaustion. In addition, inhibition of the Fas pathway prevents AICD, the other mechanism to prolong CAR T cell survival. For tumour cells, combined therapies are expected to both directly inhibit tumour survival and boost the cytotoxicity of CAR T cells by sensitizing tumours to apoptotic signalling or upregulating antigen expression. Components of the inhibitory microenvironment are also emerging as promising targets to enhance the effectiveness of CAR T cell therapy. BCR, B cell receptor; sBCMA, soluble B cell maturation antigen; mAb, monoclonal antibody; BiTE, bispecific T cell engager; TCR, T cell receptor; ROS, reactive oxygen species.
Chemotherapy is the mainstay treatment for haematological malignancies and is typically utilized as a preconditioning regimen before CAR T cell infusion. Bridging chemotherapy plays roles in controlling disease progression and reducing tumour burden, and it is strongly associated with improved long-term outcomes and less toxicity (Figure 2) (33). The regimen choices vary depending on patients’ status and prior therapies. On an individual basis, regimens that have been shown to be efficient or have not been used previously can be administered at low intensity to prevent long-term marrow suppression and severe infections, which may delay or exclude patients from CAR T cell infusion. A retrospective study showed that B-ALL patients who received high-intensity myelosuppressive bridging chemotherapy had a higher incidence of severe chemotherapy-related infections than those who received low-intensity myelosuppressive chemotherapy (70% vs. 31%; P<0.001), while there was no difference in overall survival (OS) (33). Shahid et al. gave B-ALL patients with progressive disease (PD) additional cycles of chemotherapy (≥2 cycles) and found that clinical outcomes were not closely associated with tumour burden but rather were associated with the cycles of chemotherapy (34). Those who received more than one cycle of chemotherapy had a higher incidence of severe infections (94% vs. 56%; P=0.019) and lower OS (hazard ratio, HR=3.73, 95% confidence interval, CI, 1.39-9.97; P=0.006) than those who had only one cycle.
Lymphodepletion chemotherapy is generally performed 3-5 days before CAR T cell infusion to create a favourable TME for CAR T cell engraftment, expansion and survival in vivo (Figure 2). The most widely used regimen is cyclophosphamide (Cy) plus fludarabine (Flu). Compared with Cy alone, Cy/Flu significantly improves CAR T cell efficacy (35, 36), possibly because of the properties of Flu in reducing the anti-CAR immune response, increasing the level of homeostatic cytokines and decreasing the expression of the suppressive molecule indoleamine 2,3-dioxygenase (IDO) in the TME (35–37). Generally, Flu is given based on body surface area (BSA), which leads to high interindividual variability of Flu exposure (38, 39). Recent studies indicated that optimizing Flu exposure had a favourable influence on clinical outcomes. In a retrospective analysis, Dekker et al. found that cumulative Flu area under the curve (AUC) T0-∞≥14 mg*h/L was strongly associated with improved leukaemia-free survival (LFS) of CD19 CAR T cell therapy (40). Consistently, Fabrizio et al. used a validated population-pharmacokinetic model and found that an estimated Flu AUC≥13.8 mg×h/L was associated with a lower rate of relapse and B cell recovery (41). Prospective clinical trials are warranted for further validation. Alternative regimens are also being explored. Bendamustine is a safer and more tolerable chemotherapy agent with no cross-resistance to Cy (42). In the JULIET trial, bendamustine was used for B-NHL patients who were resistant to Cy (7). In addition, bendamustine plus Flu was found to be more effective than bendamustine or Cy/Flu alone in CD30 CAR T cell therapy for patients with R/R Hodgkin lymphoma (HL) (43, 44). Currently, many efforts to standardize bridging therapy and lymphodepletion regimens are underway. At the same time, the dose of chemotherapy should be optimized, and multiple alternative regimens should be explored to meet individualized needs by different patients and their disease states.
Radiotherapy is a conventional treatment modality for lymphoma with bulky masses. It can also boost the immune response by promoting the recruitment of cytotoxic T cells, reversing T cell exhaustion and releasing tumour antigens, which can theoretically synergize CAR T cell function (45). Additionally, radiotherapy has been proven to sensitize antigen-negative tumour cells to CAR T cell-mediated apoptosis via TRAIL (Figure 2) (46). As the bridging therapy of CAR T cell therapy for B-NHL, radiotherapy showed safer and more efficient characteristics than other bridging therapies, such as chemotherapy, monoclonal antibodies, Bruton’s tyrosine kinase (BTK) inhibitors and lenalidomide (47, 48). Although the optimal timing for conducting radiotherapy is unclear, most previous clinical trials administered radiotherapy after apheresis, protecting T cells from potential radiation damage (48, 49). For the field size, bridging radiotherapy is usually delivered focally to the primary tumour and/or specific metastatic sites. However, Pinnx et al. reported that focal radiotherapy excluding active disease has a higher risk of relapse, but those encompassing all sites of lymphoma benefited more (48). Of 8 B-NHL patients who received focal radiotherapy, 17% showed 1-year PFS and 28% OS. Six patients progressed or relapsed, among whom 3 occurred outside the radiotherapy field. For 9 patients who received comprehensive radiotherapy for all lymphoma sites, the 1-year PFS and OS were 57% and 71%, respectively. In terms of B-ALL, radiotherapy is not the standard treatment and is rarely coupled with CAR T cell therapy. However, it is considered a promising strategy for removing extramedullary lesions and increasing the sensitivity of CAR T cell killing (50, 51). Together, radiotherapy is a promising combined treatment regimen and has shown preliminary superiorities. More direct comparisons with other combined therapies are required for further validation, and exploration of the optimal dose and field size of radiation is warranted.
HSCT has been regarded as the only potentially curative treatment in haematological malignancies for a long time, and it has been pursued to utilize this potential treatment to optimize CAR T cell therapy (Figure 3). Consolidative allogeneic HSCT (allo-HSCT) after CAR T cell therapy is an area of intense research, and it aims to nonselectively eradicate residual tumour cells via the graft-versus-leukaemia (GVL) effect, preventing antigen-positive and antigen-negative relapse at the same time. For B-ALL patients, conflicting results exist regarding whether patients can benefit from consolidative allo-HSCT (Table 2). Recently, a meta-analysis pooled the studies and reported that the OS (HR=0.34, 95% CI, 0.17~0.68, P=0.003) and LFS (HR=0.15, 95% CI, 0.08~0.28, P<0.001) of the bridging group were markedly improved and the relapse rate (HR=0.16, 95% CI, 0.10~0.25, P<0.001) was decreased compared with the nonbridging group (72). The incidence of transplantation-related toxicities did not increase significantly (72). Large-scale randomized controlled trials are warranted to further validate the efficacy and safety of the combinatorial strategy.
Figure 3 HSCT in combination with CAR T cell therapy to enhance efficacy. (A) Standard-of-care CAR T cell therapy following consolidative allo-HSCT. (B) Concurrent infusion of allo-CAR T cells and allo-HSCT to enhance antitumor effects and prolong the persistence of allo-CAR T cells without additional gene editing against graft rejection. (C) Allo-HSCT following allo-CAR T cell therapy for MRD clearance. (D) High-dose chemotherapy conditioning and autologous stem cell transplantation to remodel the immune environment for subsequent CAR T cell therapy. allo-HSC, allogeneic haemopoietic stem cell.
To date, decisions regarding whether to consolidate allo-HSCT should be made individually, considering the costs and risk of fatal transplantation-associated toxicities. Patients with a high tumour burden (≥5%) before CAR T cell infusion or poor prognostic factors, including high-risk genetic mutations and complex karyotypes, are more likely to benefit from consolidative allo-HSCT because of their high relapse risk (73, 74). Of note, it is believed that MLL-rearranged, BCR/ABL1 and TCF3-ZNF384 are associated with CD19-negative relapse via lineage switching to the myeloid lineage (75, 76), and patients with these markers are more likely to benefit from allo-HSCT to eliminate the origin of CD19-negative relapse. In addition, the detection of leukaemic sequences in bone marrow, CAR T cell loss and B cell recovery can predict a higher probability of relapse, facilitating timely allo-HSCT consolidation (76). In terms of timing for bridging allo-HSCT, early transplantation is likely to be a more effective and safer choice, while a ≥80-day interval may cause decreased OS and increased toxicities (58, 77). With regard to B-NHL and MM, few attempts to administer consolidative allo-HSCT have been made, and the efficacy is unclear.
When combining allo-HSCT with the same donor-derived CAR T cells, which are suitable for heavily treated patients who cannot harvest adequate T cells for autologous CAR T cell manufacturing (78), the haematopoietic remodelling function of allo-HSCT can be utilized to help graft allogeneic CAR T cells to avoid additional gene editing. Seven R/R B-NHL and 2 R/R MM patients who relapsed from autologous CAR T cells received allogeneic CAR T cells in combination with busulfan, a Flu-based conditioning regimen. Seven days later, allo-HSCT was performed in combination with graft-versus-host disease (GVHD) prophylaxis (79). The overall response rate (ORR) and complete remission (CR) were 54.5% and 45.5%, respectively, and the OS was 63.6% in a median follow-up of 171 days. A total of 81.8% of patients had persistent allogeneic CAR T cell survival, and the longest survival lasted up to 239 days after allo-HSCT. Adverse events included controllable cytokine release syndrome (CRS) and mild acute GVHD, preliminarily showing the safety and efficacy of the combined approach. Despite this finding, it is a challenge to manage adverse events caused by donor cell infusion because the application of GVHD prophylaxis is likely to cause compromised CAR T cell persistence (80–82). More clinical data are required to find a balance between safety and long-term effectiveness. Donor-derived CAR T cells can also be used as a consolidative regimen after allo-HSCT, and it is more effective than donor lymphocyte infusion in minimal residual disease (MRD) clearance (59, 60). In addition, the safety of CAR T cell infusion after allo-HSCT is likely to be improved because of the reduced tumour burden by HSCT before CAR T cell infusion (59, 60).
Autologous haematopoietic stem cell transplantation (ASCT) is also a standard of care for haematological tumours and can be utilized to enhance adoptive T cell functions by reducing tumour cells and remodelling the immune environment for T cell expansion (68, 83). The feasibility of CAR T cell therapy shortly following high-dose chemotherapy and ASCT (HDT-ASCT) has been confirmed in several clinical studies (Table 2). Wei et al. compared CAR T cell infusion after ASCT and CAR T cells alone and demonstrated that the best ORR, CR rate and long-term outcomes improved significantly in the combination group (62). In another study comparing CAR T cell infusion after ASCT and ASCT alone, the combination group showed higher CR rate (71% vs. 33%; p=0.003) and 3-year PFS (80% vs. 44%; p=0.036) than the ASCT group, and demonstrated lower 3 year relapse/progression rate (15% vs. 56%; p=0.015) (63). Incidences of adverse events differ from studies and were manageable in most cases (Table 2). However, Sauter et al. highlighted aggravated immune effector cell-associated neurotoxicity syndrome (ICANS) in their study. Sixty-seven percent of poor-risk R/R B-NHL patients who underwent BEAM conditioning (carmustine, etoposide, cytarabine, and melphalan) and ASCT following CD19-CD28 CAR T cell infusion experienced severe ICANS, possibly resulting from wide adoption of pegylated G-CSF post HDT-ASCT (68). Moreover, a study explored prolonging the interval between ASCT and CAR T cell therapy for adequate haematological recovery after ASCT (84). It showed that CAR T cells could further induce CR without aggravated toxicity for those in whom CR was not induced by ASCT. The feasibility of this strategy is further being explored (NCT04923789). In MM treatment, busulfan and Cy (BUCY) conditioning and ASCT followed by infusion of CD19 CAR T cells or their coupling with B cell maturation antigen (BCMA) CAR T cells is also a promising regimen (66, 71).
The various combined modalities between HSCT and CAR T cells provide more treatment options for haematological malignancies, among which whether to consolidate allo-HSCT after CAR T cell therapy is the most researched. High-quality randomized controlled trials to guide clinical decisions are expected. ASCT bridging to CAR T cell therapy is also likely to exert potent anti-tumour efficacy, while further validation and comparisons with other bridging therapies before CAR T cell infusion are warranted.
Ibrutinib, the first BTK inhibitor, regulates B cell receptor signalling and the development of B cells and has been approved for chronic lymphocytic leukaemia (CLL) and mantle cell lymphoma (MCL) (85). It also plays an immunoregulatory role via the inhibition of interleukin-2 inducible T cell kinase (ITK), which may have a positive effect on the expansion and function of CAR T cells (86).
Patients with a history of BTK inhibitor exposure are likely to benefit more from robust CAR T cells (87, 88). Furthermore, pilot studies confirmed the feasibility of BTK inhibitor administration prior to or concurrent with CAR T cell infusion. Nineteen patients with ibrutinib-refractory CLL were scheduled for ibrutinib infusion from 2 weeks before leukapheresis to more than 3 months after CAR T cell infusion. The ORR was 83%, and 61% achieved an MRD-negative marrow response. The estimated 1-year OS and PFS rates were 86% and 59%, respectively. The toxicities included 14 cases of mild CRS and 5 cases of grade 3 ICANS (89). Similarly, in a phase II clinical trial, 19 patients with CLL were infused with CAR T cells along with a continuous infusion of ibrutinib. The three-month CR was 44%, and 72% of patients were MRD-negative at the 1-year follow-up. The estimated 48-month OS and PFS were 84% and 70%, respectively. Eighteen patients developed CRS, including 3 cases of severe CRS, and 5 patients developed ICANS, including 1 case of grade 4 ICANS (90). Apart from in vivo administration of ibrutinib, adding ibrutinib into an ex vivo culture system can also increase the number of CAR T cells and decrease the expression of exhaustion markers (91).
Ibrutinib combination therapy has also been applied in B-NHL. Liu et al. reported that 7 MCL/follicular lymphoma patients who did not achieve CR by CD19 CAR T cell therapy received ibrutinib as salvage treatment for 7-16 months, followed by a second CAR T cell infusion. A significant improvement in the in vivo expansion of CAR T cells was observed (median peak of CAR T cells in CD3+ T cells 32.11% ± 13.28% vs. 20.34% ± 10.70%), and 6 patients achieved CR (92). In ZUMA-2, the analysis of CAR T cells and peripheral blood mononuclear cells (PBMCs) collected from MCL patients 7 days after infusion showed that compared with acalabrutinib-exposed CAR T cells, ibrutinib-exposed CAR T cells had a T helper cell (Th)1 predominant phenotype and were more likely to shift from a central memory phenotype (Tscm) to an effector memory phenotype (Tem) for the enhancement of function, possibly because of additional targeting of ITK by ibrutinib compared with acalabrutinib (93). With regard to the safety of the combination therapy, ITK inhibition by ibrutinib can prohibit cytokine hyperproduction and reduce the incidence of CRS (87, 89). However, ibrutinib may cause cardiac toxicity, and fatal arrhythmia has been reported in combination treatment (89, 94), emphasizing the need for continuous monitoring.
Acalabrutinib is a second-generation BTK inhibitor. Although it does not target ITK, it has higher selectivity and may cause fewer adverse events (95). In a B-ALL mouse model, the combination of acalabrutinib and CD19 CAR T cells prolonged the durability of CAR T cells in vivo and improved the survival of mice (96). Clinical trials of combined acalabrutinib with CAR T cells for B cell lymphoma have been launched (NCT04257578; NCT04484012).
The phosphatidylinositol-3-kinase (PI3K)-Akt-mTOR pathway is a popular therapeutic target for various tumour cells (97). It also promotes T cell proliferation and differentiation by regulating metabolism and transcription (98). Ex vivo PI3K blockade promotes Tscm, central memory T cell (Tcm) and naïve T cell (Tn) development without perturbing expansion; decreases exhaustion marker expression; and increases the ratio of CD8/CD4 T cells, facilitating in vivo expansion and cytotoxicity (98, 99). The CRB-402 (NCT03274219) trial is evaluating BCMA CAR T cells pretreated with the PI3K inhibitor bb007 ex vivo for R/R MM. The updated results showed a prolonged duration of response (DOR), and the median DOR of 72 patients was 17 months (100). Inhibition of the downstream Akt during the CAR T cell manufacturing process can also harvest products with a higher percentage of early memory phenotype (101, 102). In addition, the mTORC1 inhibitor rapamycin could upregulate CXCR4 expression on CAR T cells for bone marrow migration and inhibit terminal effector T cell differentiation but with the risk of attenuating T cell proliferation ex vivo (99, 103).
Dasatinib, a commercial tyrosine kinase inhibitor (TKI), reversibly inhibits phosphorylation of proximal TCR and CAR signalling kinases, preventing activation and differentiation induced by toxic CAR signalling and reversing T cells that have already been exhausted by epigenetic remodelling (104, 105). This mechanism facilitates ex vivo manufacturing to turn off CAR signalling and produce high-quality CAR T cells (104, 105). On the other hand, when introducing dasatinib into in vivo administration, long-term resting CAR T cells may increase the risk of tumour progression. Therefore, an intermittent and pulsed administration regimen is a potential strategy (105). Additionally, the rapid and reversed inhibitory profile of dasatinib indicates that it is an ideal agent for attenuating CRS and making CAR T cell therapy more controllable (106).
GS inhibitor (GSI), clinically used for cancers and Alzheimer’s disease (107), can effectively prohibit the cleavage of BCMA on MM cells by GS (26, 108). MM patients who received GSI showed increases in BCMA density on myeloma cells and decreases in soluble BCMA. As a result, the ORR of 6 patients, including those who had failed prior BCMA targeted therapy, was 100% (5 very good partial remission (VGPR); 1 PR) (108). Long-term follow-up is warranted. Furthermore, epigenetic modulation by all-trans retinoic acid (ATRA) could be synchronized with GSI to further increase BCMA density (109).
Bcl-2 family members are key regulators of the intrinsic apoptosis pathway (110). Venetoclax, an inhibitor of the antiapoptotic protein Bcl-2, has been approved for CLL and acute myeloid leukaemia (AML) and clinically confirmed to be efficient for B-ALL (111, 112). It was demonstrated to promote the release of proapoptotic proteins and increase CD19 expression by priming tumour cells with venetoclax, making tumours more sensitive to CAR T cell killing (113). In contrast, concurrent or post CAR T cell treatment is likely to impair the repetitive killing effect of CAR T cells by directly inducing apoptosis and inhibiting proliferation (113). However, there is an opposite argument that concurrent administration of venetoclax selectively affects CAR T cells with different immunophenotypes, supporting stem-like CD8+ T cell proliferation and decreasing Tregs. This conversion results in synergy with CAR T cell therapy (114). Further investigations on CAR T cell biology under exposure to venetoclax are warranted. Other antiapoptotic Bcl-2 family inhibitors, such as ABT-737 and myeloid leukaemia 1 (Mcl-1) inhibitor S63845, can also presensitize B cell malignancies to CAR T cell therapy (113, 115).
During ex vivo CAR T cell production, cytokines are usually involved in potentiating expansion. IL-2 is the most common cytokine but is likely to induce CAR T cell terminal differentiation and increase the Treg proportion (116, 117). In contrast, other γ-chain cytokines IL-7 and IL-15 can preserve the proliferative capability of CAR T cells without hampering ex vivo expansion, which contributes to better in vivo persistence and antitumor effects (117, 118). In addition, IL-21 can preserve a less differentiated CAR T cell phenotype with other strengths, such as enhancing transfection efficiency and cytolytic activities when combined with other γ-chain cytokines, while IL-21 alone may not be able to support sufficient CAR T cell expansion (117, 119, 120). Other cytokine family members, such as transforming growth factor-β (TGF-β), have also been proven to induce early memory T cell subsets ex vivo and subsequently potentiate the function of BCMA CAR T cells in vivo without a suppressive effect on T cell expansion (121).
After CAR T cell infusion, cytokines can also be administered to assist expansion and persistence. Fourth-generation CAR T cells are genetically engineered to secrete cytokines. By comparison, pharmacological grade cytokines may be more tunable and convenient as adjuvants. Administration of NKTR-255, a polymer-conjugated human IL-15, in combination with CD19 CAR T cells increased CAR T cell numbers, cytotoxicity and survival in a murine lymphoma model (122). R/R B-NHL and MM patients who had progressed or relapsed after CAR T cell therapy were administered with NKTR-255, and re-expansion of CAR T cells and other CD8+ T cells was subsequently observed (123). Clinical trials for consolidative NKTR-255 following CAR T cell therapy are ongoing (NCT03233854; NCT05359211). In addition, NT-I7, a long-acting human IL-7, could be used as a consolidative regimen in vivo to enhance the expansion, persistence and antitumor activities of CAR T cells in lymphoma and leukaemia mouse models (124). A clinical trial is currently ongoing to test the efficacy of NT-I7 administered 21 days after CD19 CAR T cell infusion in lymphoma patients (NCT05075603). Other immune stimulatory cytokines, such as IL-18 and IL-12, can also enhance the killing effects of CAR T cells (125, 126). Their coupling with CAR T cells in haematological malignancies deserves future exploration.
Epigenetic modulators are emerging oncological treatment agents that target aberrant epigenetic regulation and inhibit the occurrence and development of tumour cells (127). Meanwhile, epigenomic modulation results in upregulation of tumour antigen expression and improved survival and cytotoxicity of T cells (128), providing rationales for combination strategies with CAR T cell therapy.
Decitabine (DAC), a DNA methyltransferase inhibitor (DNMTi), is commonly used in myelodysplastic syndromes and AML (129). Combining DAC with CD19 CAR T cell therapy can effectively enhance antitumor effects by upregulating CD19 expression on tumour cells and reversing CAR T cell exhaustion (130, 131). A clinical trial of DAC-primed tandem CD19/CD20 CAR T cells for R/R B-NHL is ongoing (NCT04697940). Azacitidine (AZA), another FDA-approved DNMTi, can increase the antigen density of CD70 or CD123 in AML, potentiating CAR T cell targeting (132, 133). Existing data are mainly based on tumours pretreated with AZA, and no attempt to directly expose CAR T cells to AZA has been made because there is a concern that AZA promotes Tregs (134). However, the influence of AZA on CAR T cells may be positive and worth further investigation.
Histone deacetylase inhibitor (HDACi), another popular type of epigenetic modulator, such as chidamide, has been proven to effectively upregulate CD22 on B cell malignancies (135). HDACi can also upregulate tumour pro-apoptotic genes, sensitizing tumour cells to CAR T cell-mediated killing (136). Moreover, the bromodomain and extraterminal domain (BET) family member BRD4 contributes to upregulating the expression of the transcription factor BATF and promoting Tem differentiation (137). The BRD4 inhibitor JQ1 reversed differentiation and enhanced CAR T cell persistence and antitumor effects in B-ALL mouse models (138). The interactions between c-Myc and the epigenetic regulator canonical BRG1/BRM-associated factor (cBAF) promote the remodelling of the chromosomal landscape and the accessibility of T cell differentiation-related gene regions, thereby driving the differentiation of T cells towards effector T cells. Treatment with BRD-K98645985 (BD98), a probable BAF inhibitor, maintained the memory-like phenotype of T cells in vivo and enhanced the antitumor function and persistence of CAR T cells in osteosarcoma and glioma models (139). In summary, epigenetic modifications are promising strategies to address resistance from the perspectives of both CAR T cells and tumour cells. Despite compelling preclinical evidence, the efficacy of the combination of epigenomic agents and CAR T cell therapy needs to be tested in clinical trials.
With profound mechanisms of resistance and relapse of CAR T cell therapy, more potential targets and corresponding pharmacological treatments are emerging (Table 3). Preventing and reversing CAR T cell exhaustion is one of the most intense study fields. Along with the strategies mentioned above, such as targeting the PI3K-Akt pathway and TKI, another T cell expansion and differentiation-associated kinase glycogen synthase kinase-3β (GSK-3β) was found to be inhibited by TWS119 ex vivo for memory maintenance (165–167). In addition, metabolic changes are a characteristic of CAR T cell exhaustion with impaired antioxidant capacity. Scavenging of reactive oxygen species by the antioxidant N-acetylcysteine can effectively promote Tscm formation and expansion ex vivo for better product manufacturing (168). Ideal phenotypes of cytotoxic T cells in vivo are believed to comprise expansion, differentiation, oxidative and genomic stress, and CRISPR-cas9-based genetic screening of TCR-based kinases identified Mapk14(p38) as a central regulator of those determining factors (141). Targeting it with BIRB796 ex vivo significantly enhanced the antitumor effects of CAR T cells in vivo. On the other hand, overactivation of T cells triggers Fas-mediated AICD, which can be abrogated by asunercept, a FasL inhibitor, subsequently increasing the number of CD19 CAR T cells and enhancing antitumor killing effects (142). However, this strategy may attenuate Fas-mediated cytotoxicity against tumour cells or trigger autoimmune diseases (169).
Table 3 Rationales for the combination of CAR T cell therapy with targeted therapies and other immunotherapies.
From the perspective of tumour cells, antigen loss or downregulation is the trickiest limitation. In addition to epigenetic modulators and GSI, another small molecule drug, protein kinase C inhibitor bryostatin I, can stabilize CD22 in B-ALL and B-NHL and improve CD22 CAR T cell functionality and durability of response (29). Furthermore, there are some intrinsic resistance mechanisms in tumour cells via abnormal interactions between anti-apoptotic and pro-apoptotic molecules. Through high-throughput coculture drug sensitivity screens of more than 500 oncological drugs, the apoptosis-regulating drug SMAC mimic was identified to enhance CD19 CAR T cell toxicity (145). Complementary CRISPR screening identified that SMAC mimics play synergetic roles by inhibiting the IAPs and sensitize leukaemia and lymphoma to death receptor signalling (145). In addition, celecoxib, a well-known cyclooxygenase-2 (COX-2) inhibitor, also contributes to the upregulation of apoptotic proteins, thereby restoring the sensitivity of B-NHL cells to CD19 CAR T cells (136). However, it may unselectively inhibit CAR T cells and increase the risk of CAR T cell apoptosis (79).
Targeting the immunosuppressive TME is another direction for developing combined regimens. Metabolites in the TME, such as IDO and adenosine, are a promising direction. The combination of the IDO inhibitor 1-methyl-tryptophan and CD19 CAR T cells showed improved tumour control in lymphoma models (37). In addition, anti-T cell responses mediated by adenosine can be mitigated by pharmacological blockage of the adenosine 2A receptor (170, 171). Interestingly, GM-CSF inhibitors were initially used to alleviate CRS and ICANS, but they also showed an inhibitory profile towards the proliferation and migration of immunosuppressive cells, consequently enhancing the antitumor effect of CD19 CAR T cells (172).
Lenalidomide, the primary treatment modality for MM, exerts direct antitumor effects as well as immunomodulatory effects. When combined with CAR T cell therapy, it plays a stimulatory role on CAR T cells by enhancing cytotoxicity, maintaining memory phenotype, skewing Th2 towards Th1 and increasing immune synapses (173, 174). In murine models, lenalidomide was further proven to enhance the antitumor effects of CAR T cells and prolong mouse survival significantly (173, 174).
Clinically, 10 newly diagnosed MM patients received sequential CD19 and BCMA CAR T cell treatment after ASCT, followed by lenalidomide maintenance until relapse. Ninety percent of patients achieved stringent CR, and 10% of patients achieved CR. During a median follow-up of 42 months, the median PFS was not reached, and 70% of patients maintained MRD negativity for more than 2 years (148). In addition, R/R MM patients who did not respond to CAR T cell therapy alone are also likely to improve outcomes by CAR T cells in cooperation with lenalidomide (147). A phase I clinical trial is ongoing (NCT03070327).
The combination of lenalidomide and CAR T cells is also viable in B-NHL (146). PiggyBac-produced CD19 CAR T cells induced CR in a triple-hit R/R DLBCL patient with the TP53 mutation. Then, oral lenalidomide was given from the fourth month after CAR T cell infusion, and CAR copies were still detectable 9 months post-infusion. Although the patient stopped lenalidomide after one cycle because of side effects, including rash, itching and joint pain, he maintained CR for more than 2 years, and his OS was over 3 years (146). ZUMA-14 is exploring the clinical efficacy of the combination of axicabtagene ciloleucel and lenalidomide (NCT04002401).
Immune checkpoint inhibitors have been widely used in cancer treatment, among which programmed-cell-death-1 (PD-1)/PD-ligand 1 (PD-L1) are the most significant targets. Combining CAR T cell therapy with PD-1/PD-L1 inhibitors, further unleashing efficacy in haematological tumours, is an attractive strategy.
Pembrolizumab, an anti-PD-1 mAb, was administered in combination with CAR T cells to treat patients who were resistant to or relapsed from previous CAR T cell therapy. Twelve patients with B cell lymphoma with disease progression (n=8) or relapse (n=4) after CD19 CAR T therapy continued to receive pembrolizumab from 3.3 (0.4-42.8) months after CAR T cell infusion, with a 25% optimal ORR (1 CR and 2 PR) (149). The only ≥ grade 3 adverse event was 25% neutropenia. After the first pembrolizumab infusion, CAR T cell re-expansion was detected in 83% of patients. Deep immune profiling demonstrated that, compared with nonresponders, lower-level expression of exhaustion and terminal differentiation markers was found in clinical responders, suggesting that PD-1 blockade is more effective in reversing exhaustion for patients with PD-1 expression under a certain threshold (149). Moreover, the Alexander trial administered CD19/CD22 dual-targeted CAR T cells plus pembrolizumab to 8 diffuse large B cell lymphoma (DLBCL) patients, with 75% ORR and 63% CR. No cases of severe CRS or ICANS were observed (150).
Nivolumab, another anti-PD-1 mAb, was infused into 11 patients with R/R B-NHL three days after CD19 CAR T cell infusion. The ORR and CR rate were 81.81% and 45.45%, respectively (152). Mild CRS was observed in 9 patients, and it could be relieved by glucocorticoids. Although the efficacy of the combination regimen did not appear to be significantly better than that of CD19 CAR T cells alone, it may be associated with a worse baseline of the combination cohort (152). Low-dose nivolumab (1.5 mg/kg) and CD19 CAR T cells were also feasible for follicular lymphoma patients with high PD-1 expression (175).
In ZUMA-6, 4 doses of atezolizumab, an anti-PD-L1 mAb, combined with axicabtagene ciloleucel were administered to 12 patients with R/R DLBCL. CAR T cells were observed to expand robustly, and the ORR of 10 evaluable patients was 90%, including 6 (60%) CR (153). Similarly, another anti-PD-L1 mAb, durvalumab, was infused in 11 patients on Day 29 after CD19 CAR T therapy at a total dose of 1500 mg/4 weeks for 12 months. The optimal ORR was 91% (10/11), and 64% of patients (7/11) achieved CR. Grade 3 or more severe neutropenia and cytopenia occurred in 2 patients, and no CRS was reported (155).
The combination of PD-1/PD-L1 blockade has also been applied in other haematological malignancies. For children with R/R B-ALL, PD-1 inhibition is relatively efficient for those with less persistent CAR T cells and bulk extramedullary disease; however, it is less useful for those with a poor initial response to CAR T cells (151). In terms of MM, BCMA/CS1 bispecific CAR T cells in combination with anti-PD-1 mAb accelerated the clearance of MM cells in mouse models (176). However, prolonged injections of anti-PD-1 mAb after tumour clearance in this study appeared to cause CAR T cell dysfunction, with mice relapsing or failing to prevent tumour outgrowth after tumour rechallenge (176). Further studies are required to determine the optimal duration of PD-1/PD-L1 inhibitor administration to avoid impairing the sustained antitumor potency of CAR T cells.
Together, PD-1/PD-L1 inhibitors appear to be tolerable and can achieve therapeutic improvement of CAR T cell therapy for some patients. However, the overall efficacy is less promising, and for ineffective cases other independent resistance mechanisms deserve further clarification. Other checkpoint inhibitors, such as ipilimumab, a cytotoxic T lymphocyte-associated antigen-4 (CTLA-4) blockade, in combination with CAR T cells are under investigation (NCT00586391). Recently, the utilization of single-cell sequencing facilitated the identification of T cell immunoreceptor with immunoglobulin and immunoreceptor tyrosine-based inhibitory motif domain (TIGIT) as the most notable exhaustion marker of CAR T cells at the transcriptional and protein levels in B-NHL patients. Sequentially, TIGIT blockade was proven to improve the antitumor function of CAR T cells in preclinical studies (156).
T cell costimulatory receptors play crucial roles in the CAR molecule construct, and they have also been explored as targets for cancer immunotherapy. CAR T cells with a CD28 costimulatory domain are believed to lead to robust but transient activation, while the signalling of 4-1BB CAR is mild and persistent (177). The addition of exogenous 4-1BB agonists to CAR T cells with the CD28 costimulatory domain is likely to enhance CAR T cell activation, persistence and memory. 4-1BB agonists can also activate NK cells, DCs and other myeloid lineage cells to further boost the immune response (178). Combining HER2-CD28 CAR T cells with an exogenous agonistic 4-1BB monoclonal antibody (mAb) achieved stronger antitumor efficacy and long-term tumour-free survival in a breast tumour mouse model (179). Utomilumab, a 4-1BB agonist entering clinical trials, is being scheduled to be applied for refractory LBCL in the ZUMA-11 trial 1 day following infusion of axicabtagene ciloleucel, an FDA-approved CAR T cell product with the CD28 costimulatory domain, and every 4 weeks for 6 months or until progressive disease (NCT03704298) (180). Data are still being collected.
Antibodies targeting tumour surface antigens are the most prevalent immunotherapy in the treatment of haematological malignancies and have been widely used to bridge CAR T cell therapy. In B-NHL, the CD20 mAbs rituximab and obinutuzumab are the most popular bridging immunotherapies (47). In addition, researchers are exploring their administration concomitantly with CD19 CAR T cells (NCT04002401, NCT04889716). Daratumumab, a first-in-class anti-CD38-mAb for the treatment of MM, is an attractive candidate for cooperating with CAR T cells as it can eliminate MM cells and simultaneously target CD38-positive immunosuppressive cells such as Tregs, regulatory B cells (Bregs) and MDSCs, reversing the suppressive immune microenvironment for CAR T cells (157, 181). Antibody-drug conjugates (ADCs), including the anti-CD30 ADC brentuximab vedotin and the anti-CD79 ADC polatuzumab vedotin for B-NHL and the anti-CD22 antibody inotuzumab ozogamicin, are also used as bridging therapies (10, 158, 159). Bispecific antibodies used in the bridging period include CD20/CD3-targeting mosunetuzumab and glofitamab for B-NHL and CD19/CD3-targeting blinatumomab for B-ALL (160, 164). They can bind to tumour antigens and recruit T cells for accurate killing. Notably, repeated targeting by CAR T cells and antibodies should be highlighted due to the high risk of antigen escape (162, 164). Therefore, close monitoring of antigen expression is needed to design combinations of these immunotherapy agents.
Targeting multiple antigens is a pivotal strategy for overcoming antigen-negative relapse. Dual or bispecific CAR T cells are being investigated, while a multitargeted single-CAR design in those products is challenging (182). On the other hand, as clinical applications of multiple single-target CAR T cell products are gradually maturing, combined infusion of different CAR T cell products may achieve clinical translation more easily (Table 4).
The most common combination scheme is cocktail infusion of CD19/CD22 CAR T cells in B cell malignancies (Figure 4) (62, 192). Wang et al. administered CD19 CAR T and CD22 CAR T cells at Day 0 and Day 2, respectively, to B-ALL and B-NHL patients. A total of 94.1% (48/51) of B-ALL patients achieved MRD-negative CR or complete remission with incomplete count recovery (CRi), and 1 patient achieved PR. The median PFS was 13.6 months (95% CI, 6.5-not reached), and the median OS was 31 months (95% CI, 10.6-NR). Among the B-NHL cohorts, 68% (26/38) achieved ORR, and 47% reached CR (192). The median PFS was 9.9 months (95% CI, 3.3-NR), and the median OS was 18.0 months (95% CI, 6.1-NR). The cocktail appears to resolve antigen-negative relapse effectively but still faces the issue of inadequate CAR T cell persistence. Only one patient relapsed with CD19-negative CD22-dim, while 23 patients exhibited CD19+CD22+ relapse. CD19/CD22 CAR T cell cocktail therapy is also promising in improving the outcome of B cell malignancies with the TP53 mutation, which confers a higher rate of CD19-negative relapse and poor prognosis (56, 193). The optimal ORR of 32 TP53-mutated lymphoma patients who received cocktail therapy was similar to that of 34 lymphoma patients without the mutation (87.1% vs. 88.2%, P=0.927) (62). In terms of R/R MM, CD19/BCMA CAR T cell cocktail therapy was designed to extend the target range, covering a proportion of CD19-positive myeloma-like stem cells with drug resistance and propagating characteristics (194). Yan et al. enrolled 21 MM patients and administered CD19 CAR T cells and BCMA CAR T cells simultaneously. Twenty (95%) patients had an overall response, including 9 with stringent CR, 3 with CR, and 5 with VGPR (189).
Figure 4 Two combinations of CD19 and CD22 CAR T cell products as an example of combinations among different types of CAR T cells. (A) Cocktail infusion of CD19 and CD22 CAR T cells for addressing antigen-negative relapse. (B) Sequential infusion of CD19 and CD22 CAR T cells according to the in vivo activity of CAR T cells and patient status to overcome both short duration and antigen escape.
Sequential infusion is another form of combination. Patients in CR with undetectable CAR T cells are expected to undergo infusion with another type of CAR T cell as maintenance to prolong CAR T cell persistence and prevent antigen-negative relapse. For patients in PR or SD, the second infusion is considered a consolidative regimen to induce further CR (184, 188). Pan et al. infused CD22 CAR T cells into 20 R/R B-ALL patients in CR when the first infusion of CD19 CAR T cells was undetectable, with a median interval of two CAR T cell infusions of 1.65 months. The 12-month LFS was 79.5%, and the 18-month OS rate was 92.3% (188). Moreover, 23 R/R Burkitt lymphoma patients were administered sequential infusions of murine CD19 (mCD19)-humanized CD22 (hCD22)-hCD20 CAR T cells depending on their disease states and in vivo CAR T cell lifespan (184). The CR rate was 95% at 3 months after the last infusion. The estimated 18-month CR rate and PFS rate were 78% (95% CI, 54%-91%) and 78% (95% CI, 55%-90%), respectively (184). The toxicities of sequential infusions in these trials were moderate and had no influence on the subsequent CAR T cell infusion. The time interval between infusions should be optimized, as reinfusion too early may impair effective CAR T cells in vivo by lymphodepletion, while tumours may recur if there is no timely CAR T cell maintenance. Interestingly, Meng et al. shortened the time interval and did not apply lymphodepletion before the second CAR T cell infusion. They observed re-expansion of the first CAR T cells with unclear mechanisms (195). However, the excessive expansion and activation of CAR T cells raise the concern of aggravated CRS, requiring conscious monitoring in more studies. Other combined CAR T cell products targeting different antigens, such as CD19/CD79b CAR T cells for B-NHL, CD19/CD38, CD19/CD123 CAR T cells for B-ALL, and BCMA/GPRC5D CAR T cells for MM, are being explored (182).
Resistance and relapse are the most prominent challenges of CAR T cell therapy in haematological malignancies, for which searching for combination treatment is a promising strategy for overcoming CAR T cell-centric, tumour-centric and TME-associated hurdles. Chemotherapy and radiotherapy as preconditioning treatments play an important role in subsequent clinical outcomes. In particular, radiotherapy, with its stimulatory function on the immune response, may achieve desired priming of CAR T cell therapy. Head-to-head clinical trials are needed to compare the bridging of radiotherapy with chemotherapy and other emerging bridging therapies. Consolidative allo-HSCT after CAR T cell therapy is a research hotspot among diverse combination modalities of CAR T cell therapy and HSCT. Clarifying indications for consolidative allo-HSCT is crucial to avoiding wasting residual functioning CAR T cells in vivo. Targeted therapy and immunotherapy also provide multiple choices to enhance the efficacy of CAR T cell therapy, among which some agents have already been the standard of care for specific haematological tumours, providing safe and reliable therapeutic effects. Patient selection is quite important. Based on genetic testing, targeted therapy is expected to simultaneously eliminate tumours and enhance CAR T cell function. For instance, BCR-ABL-positive patients are likely to benefit more from CAR T cell infusion concomitant with TKI regardless of the turn-off period of CAR T cells. In terms of immunotherapy, there are large interindividual variations in treatment effects, suggesting urgent needs to identify patients who are sensitive to the treatments along with clarifying interactions of the combined agents in a complicated immune microenvironment.
To date, there have been limited clinical data on combinatorial strategies, and more large-scale trials are needed to directly compare them with monotherapy. Simultaneously, more mechanistic studies are expected to further unveil interactions between adjuvant agents and CAR T cells in different differentiation phases, facilitating rational combinatorial regimen design with optimal timing and dosing. As high-throughput screening technologies such as CRISPR screening and single-cell sequencing are utilized to identify more druggable mechanisms and effects of combined agents on CAR T cells, more safe and efficient combination modalities will be discovered to help elicit more potential of the legendary CAR T cell therapy.
XXiao, ST and YL designed the study. XXiao, YW, ZZ, XW and XXin drafted the manuscript. YW, YY, ZZ and XXiao prepared the tables and figures. All authors participated in the revision of the manuscript. All authors read and approved the final manuscript.
This research was supported by grants from the National Natural Science Foundation of China (U2001224); Guangzhou Regenerative Medicine and Health Guangdong Laboratory (2018GZR110105014); the National Key Research and Development Programme of China (2017YFA0105503); Guangdong Students’ Platform for Innovation and Entrepreneurship Training Program (No. S202112121059) and Special Funds for the Cultivation of Guangdong College Students’ Scientific and Technological Innovation (No. pdjh2022b0096).
XXiao, YW, YY and XW are members of the Small Small bird (SSb) group and acknowledge the strong support from SSb. We would like to acknowledge Xinjie Xu, the founder and leader of SSb, for the constructive suggestions of the manuscript. We also sincerely appreciate continuous support from our best friends Sifei Chen and Shengkang Huang.
The authors declare that the research was conducted in the absence of any commercial or financial relationships that could be construed as a potential conflict of interest.
All claims expressed in this article are solely those of the authors and do not necessarily represent those of their affiliated organizations, or those of the publisher, the editors and the reviewers. Any product that may be evaluated in this article, or claim that may be made by its manufacturer, is not guaranteed or endorsed by the publisher.
1. Neelapu SS, Locke FL, Bartlett NL, Lekakis LJ, Miklos DB, Jacobson CA, et al. Axicabtagene ciloleucel CAR T-cell therapy in refractory Large b-cell lymphoma. N Engl J Med (2017) 377(26):2531–44. doi: 10.1056/NEJMoa1707447
2. Nastoupil LJ, Jain MD, Feng L, Spiegel JY, Ghobadi A, Lin Y, et al. Standard-of-Care axicabtagene ciloleucel for relapsed or refractory Large b-cell lymphoma: Results from the US lymphoma CAR T consortium. J Clin Oncol (2020) 38(27):3119–28. doi: 10.1200/jco.19.02104
3. Pasquini MC, Locke FL, Herrera AF, Siddiqi T, Ghobadi A, Komanduri KV, et al. Post-marketing use outcomes of an anti-CD19 chimeric antigen receptor (CAR) T cell therapy, axicabtagene ciloleucel (Axi-cel), for the treatment of Large b cell lymphoma (LBCL) in the united states (US). Blood (2019) 134(Supplement 1):764. doi: 10.1182/Blood-2019-124750
4. Baird JH, Epstein DJ, Tamaresis JS, Ehlinger Z, Spiegel JY, Craig J, et al. Immune reconstitution and infectious complications following axicabtagene ciloleucel therapy for Large b-cell lymphoma. Blood Adv (2021) 5(1):143–55. doi: 10.1182/Bloodadvances.2020002732
5. Jacobson CA, Hunter BD, Redd R, Rodig SJ, Chen PH, Wright K, et al. Axicabtagene ciloleucel in the non-trial setting: Outcomes and correlates of response, resistance, and toxicity. J Clin Oncol (2020) 38(27):3095–106. doi: 10.1200/jco.19.02103
6. Kuhnl A, Roddie C, Kirkwood AA, Tholouli E, Menne T, Patel A, et al. A national service for delivering CD19 CAR-tin Large b-cell lymphoma - the UK real-world experience. Br J Haematol (2022) 198(3):492–502. doi: 10.1111/bjh.18209
7. Schuster SJ, Bishop MR, Tam CS, Waller EK, Borchmann P, McGuirk JP, et al. Tisagenlecleucel in adult relapsed or refractory diffuse Large b-cell lymphoma. N Engl J Med (2019) 380(1):45–56. doi: 10.1056/NEJMoa1804980
8. Pasquini MC, Hu ZH, Curran K, Laetsch T, Locke F, Rouce R, et al. Real-world evidence of tisagenlecleucel for pediatric acute lymphoblastic leukemia and non-Hodgkin lymphoma. Blood Adv (2020) 4(21):5414–24. doi: 10.1182/Bloodadvances.2020003092
9. Iacoboni G, Villacampa G, Martinez-Cibrian N, Bailén R, Lopez Corral L, Sanchez JM, et al. Real-world evidence of tisagenlecleucel for the treatment of relapsed or refractory Large b-cell lymphoma. Cancer Med (2021) 10(10):3214–23. doi: 10.1002/cam4.3881
10. Abramson JS, Palomba ML, Gordon LI, Lunning MA, Wang M, Arnason J, et al. Lisocabtagene maraleucel for patients with relapsed or refractory Large b-cell lymphomas (Transcend nhl 001): A multicentre seamless design study. Lancet (2020) 396(10254):839–52. doi: 10.1016/s0140-6736(20)31366-0
11. Wang M, Munoz J, Goy A, Locke FL, Jacobson CA, Hill BT, et al. KTE-X19 CAR T-cell therapy in relapsed or refractory mantle-cell lymphoma. N Engl J Med (2020) 382(14):1331–42. doi: 10.1056/NEJMoa1914347
12. Jacobson CA, Chavez JC, Sehgal AR, William BM, Munoz J, Salles G, et al. Axicabtagene ciloleucel in relapsed or refractory indolent non-Hodgkin lymphoma (ZUMA-5): A single-arm, multicentre, phase 2 trial. Lancet Oncol (2022) 23(1):91–103. doi: 10.1016/s1470-2045(21)00591-x
13. Maude SL, Laetsch TW, Buechner J, Rives S, Boyer M, Bittencourt H, et al. Tisagenlecleucel in children and young adults with b-cell lymphoblastic leukemia. N Engl J Med (2018) 378(5):439–48. doi: 10.1056/NEJMoa1709866
14. Shah BD, Ghobadi A, Oluwole OO, Logan AC, Boissel N, Cassaday RD, et al. KTE-X19 for relapsed or refractory adult b-cell acute lymphoblastic leukaemia: Phase 2 results of the single-arm, open-label, multicentre zuma-3 study. Lancet (2021) 398(10299):491–502. doi: 10.1016/s0140-6736(21)01222-8
15. Munshi NC, Anderson LD Jr., Shah N, Madduri D, Berdeja J, Lonial S, et al. Idecabtagene vicleucel in relapsed and refractory multiple myeloma. N Engl J Med (2021) 384(8):705–16. doi: 10.1056/NEJMoa2024850
16. Usmani SZ, Berdeja JG, Madduri D, Jakubowiak AJ, Agha ME, Cohen AD, et al. Ciltacabtagene autoleucel, a b-cell maturation antigen (BCMA)-directed chimeric antigen receptor T-cell (CAR-T) therapy, in Relapsed/Refractory multiple myeloma (R/R MM): Updated results from cartitude-1. J Clin Oncol (2021) 39(15_suppl):8005–05. doi: 10.1200/JCO.2021.39.15_suppl.8005
17. Berdeja JG, Madduri D, Usmani SZ, Jakubowiak A, Agha M, Cohen AD, et al. Ciltacabtagene autoleucel, a b-cell maturation antigen-directed chimeric antigen receptor T-cell therapy in patients with relapsed or refractory multiple myeloma (Cartitude-1): A phase 1b/2 open-label study. Lancet (2021) 398(10297):314–24. doi: 10.1016/s0140-6736(21)00933-8
18. Meng J, Wu X, Sun Z, Xun R, Liu M, Hu R, et al. Efficacy and safety of CAR-T cell products axicabtagene ciloleucel, tisagenlecleucel, and lisocabtagene maraleucel for the treatment of hematologic malignancies: A systematic review and meta-analysis. Front Oncol (2021) 11:698607. doi: 10.3389/fonc.2021.698607
19. Roex G, Timmers M, Wouters K, Campillo-Davo D, Flumens D, Schroyens W, et al. Safety and clinical efficacy of BCMA CAR-t-cell therapy in multiple myeloma. J Hematol Oncol (2020) 13(1):164. doi: 10.1186/s13045-020-01001-1
20. Milone MC, Xu J, Chen SJ, Collins MA, Zhou J, Powell DJ Jr., et al. Engineering enhanced CAR T-cells for improved cancer therapy. Nat Cancer (2021) 2(8):780–93. doi: 10.1038/s43018-021-00241-5
21. Pietrobon V, Todd LA, Goswami A, Stefanson O, Yang Z, Marincola F. Improving CAR T-cell persistence. Int J Mol Sci (2021) 22(19):10828. doi: 10.3390/ijms221910828
22. Ajina A, Maher J. Strategies to address chimeric antigen receptor tonic signaling. Mol Cancer Ther (2018) 17(9):1795–815. doi: 10.1158/1535-7163.MCT-17-1097
23. Ghorashian S, Kramer AM, Onuoha S, Wright G, Bartram J, Richardson R, et al. Enhanced CAR T cell expansion and prolonged persistence in pediatric patients with ALL treated with a low-affinity CD19 CAR. Nat Med (2019) 25(9):1408–14. doi: 10.1038/s41591-019-0549-5
24. Huan T, Chen D, Liu G, Zhang H, Wang X, Wu Z, et al. Activation-induced cell death in CAR-T cell therapy. Hum Cell (2022) 35(2):441–7. doi: 10.1007/s13577-022-00670-z
25. Lemoine J, Ruella M, Houot R. Born to survive: How cancer cells resist CAR T cell therapy. J Hematol Oncol (2021) 14(1):199. doi: 10.1186/s13045-021-01209-9
26. Pont MJ, Hill T, Cole GO, Abbott JJ, Kelliher J, Salter AI, et al. γ-secretase inhibition increases efficacy of BCMA-specific chimeric antigen receptor T cells in multiple myeloma. Blood (2019) 134(19):1585–97. doi: 10.1182/Blood.2019000050
27. Van de Donk NW, Usmani SZ, Yong KJTLH. CAR T-cell therapy for multiple myeloma: State of the art and prospects. Lancet Haematol (2021) 8(6):e446–e61. doi: 10.1016/S2352-3026(21)00057-0
28. Xue T, Del Real M, Marcucci E, Toribio C, Setayesh SM, Forman SJ, et al. Checkpoint blockade in combination with CD33 chimeric antigen receptor T cell therapy and hypomethylating agent against acute myeloid leukemia. Blood (2019) 134:1383. doi: 10.1182/blood-2019-121486
29. Ramakrishna S, Highfill SL, Walsh Z, Nguyen SM, Lei H, Shern JF, et al. Modulation of target antigen density improves CAR T-cell functionality and persistence. Clin Cancer Res (2019) 25(17):5329–41. doi: 10.1158/1078-0432.CCR-18-3784
30. Nakata S, Yoshida T, Horinaka M, Shiraishi T, Wakada M, Sakai T. Histone deacetylase inhibitors upregulate death receptor 5/TRAIL-R2 and sensitize apoptosis induced by TRAIL/APO2-l in human malignant tumor cells. Oncogene (2004) 23(37):6261–71. doi: 10.1038/sj.onc.1207830
31. Lemoine J, Ruella M, Houot R. Overcoming intrinsic resistance of cancer cells to CAR T-cell killing. Clin Cancer Res (2021) 27(23):6298–306. doi: 10.1158/1078-0432.Ccr-21-1559
32. Epperly R, Gottschalk S, Velasquez MP. A bump in the road: How the hostile AML microenvironment affects CAR T cell therapy. Front Oncol (2020) 10:262. doi: 10.3389/fonc.2020.00262
33. Perica K, Flynn J, Curran KJ, Rivere I, Wang X, Senechal B, et al. Impact of bridging chemotherapy on clinical outcome of CD19 CAR T therapy in adult acute lymphoblastic leukemia. Leukemia (2021) 35(11):3268–71. doi: 10.1038/s41375-021-01196-3
34. Shahid S, Ramaswamy K, Flynn J, Mauguen A, Perica K, Park JH, et al. Impact of bridging chemotherapy on clinical outcomes of CD19-specific CAR T cell therapy in Children/Young adults with Relapsed/Refractory b cell acute lymphoblastic leukemia. Transplant Cell Ther (2022) 28(2):72.e1– e8. doi: 10.1016/j.jtct.2021.11.014
35. Turtle CJ, Hanafi LA, Berger C, Gooley TA, Cherian S, Hudecek M, et al. CD19 CAR-T cells of defined CD4+:CD8+ composition in adult b cell ALL patients. J Clin Invest (2016) 126(6):2123–38. doi: 10.1172/JCI85309
36. Turtle CJ, Hanafi LA, Berger C, Hudecek M, Pender B, Robinson E, et al. Immunotherapy of non-hodgkin's lymphoma with a defined ratio of CD8+ and CD4+ CD19-specific chimeric antigen receptor-modified T cells. Sci Transl Med (2016) 8(355):355ra116. doi: 10.1126/scitranslmed.aaf8621
37. Ninomiya S, Narala N, Huye L, Yagyu S, Savoldo B, Dotti G, et al. Tumor indoleamine 2,3-dioxygenase (IDO) inhibits CD19-CAR T cells and is downregulated by lymphodepleting drugs. Blood (2015) 125(25):3905–16. doi: 10.1182/blood-2015-01-621474
38. Langenhorst JB, Dorlo TPC, van Maarseveen EM, Nierkens S, Kuball J, Boelens JJ, et al. Population pharmacokinetics of fludarabine in children and adults during conditioning prior to allogeneic hematopoietic cell transplantation. Clin Pharmacokinet (2019) 58(5):627–37. doi: 10.1007/s40262-018-0715-9
39. Langenhorst JB, van Kesteren C, van Maarseveen EM, Dorlo TPC, Nierkens S, Lindemans CA, et al. Fludarabine exposure in the conditioning prior to allogeneic hematopoietic cell transplantation predicts outcomes. Blood Adv (2019) 3(14):2179–87. doi: 10.1182/Bloodadvances.2018029421
40. Dekker L, Calkoen FG, Jiang Y, Blok H, Veldkamp SR, De Koning C, et al. Fludarabine exposure predicts outcome after CD19 CAR T-cell therapy in children and young adults with acute leukemia. Blood Adv (2022) 6(7):1969–76. doi: 10.1182/Bloodadvances.2021006700
41. Fabrizio VA, Boelens JJ, Mauguen A, Baggott C, Prabhu S, Egeler E, et al. Optimal fludarabine lymphodepletion is associated with improved outcomes after CAR T-cell therapy. Blood Adv (2022) 6(7):1961–68. doi: 10.1182/Bloodadvances.2021006418
42. Figgins B, Primeaux B, Shank BR, Chen SE, Weber DM, Lu H, et al. Cyclophosphamide desensitization in patients with severe hypersensitivity reactions to bendamustine. J Oncol Pharm Pract (2020) 26(4):982–5. doi: 10.1177/1078155219867127
43. Ramos CA, Ballard B, Zhang H, Dakhova O, Gee AP, Mei Z, et al. Clinical and immunological responses after CD30-specific chimeric antigen receptor-redirected lymphocytes. J Clin Invest (2017) 127(9):3462–71. doi: 10.1172/JCI94306
44. Ramos CA, Grover NS, Beaven AW, Lulla PD, Wu MF, Ivanova A, et al. Anti-CD30 CAR-T cell therapy in relapsed and refractory Hodgkin lymphoma. J Clin Oncol (2020) 38(32):3794–804. doi: 10.1200/jco.20.01342
45. Pointer KB, Pitroda SP, Weichselbaum RR. Radiotherapy and immunotherapy: Open questions and future strategies. Trends Cancer (2022) 8(1):9–20. doi: 10.1016/j.trecan.2021.10.003
46. DeSelm C, Palomba ML, Yahalom J, Hamieh M, Eyquem J, Rajasekhar VK, et al. Low-dose radiation conditioning enables CAR T cells to mitigate antigen escape. Mol Ther (2018) 26(11):2542–52. doi: 10.1016/j.ymthe.2018.09.008
47. Nasta SD, Hughes ME, Namoglu EC, Landsburg DJ, Chong EA, Barta SK, et al. A characterization of bridging therapies leading up to commercial CAR T-cell therapy. Blood (2019) 134:4108. doi: 10.1182/Blood-2019-131399
48. Pinnix CC, Gunther JR, Dabaja BS, Strati P, Fang P, Hawkins MC, et al. Bridging therapy prior to axicabtagene ciloleucel for Relapsed/Refractory Large b-cell lymphoma. Blood Adv (2020) 4(13):2871–83. doi: 10.1182/Bloodadvances.2020001837
49. Sim AJ, Jain MD, Figura NB, Chavez JC, Shah BD, Khimani F, et al. Radiation therapy as a bridging strategy for CAR T cell therapy with axicabtagene ciloleucel in diffuse Large b-cell lymphoma. Int J Radiat Oncol Biol Phys (2019) 105(5):1012–21. doi: 10.1016/j.ijrobp.2019.05.065
50. Marquez CP, Montiel-Esparza R, Hui C, Schultz LM, Davis KL, Hoppe RT, et al. Use of cardiac radiation therapy as bridging therapy to CAR-T for relapsed pediatric b-cell acute lymphoblastic leukemia. Pediatr Blood Cancer (2021) 68(3):e28870. doi: 10.1002/pbc.28870
51. Denton CC, Gange WS, Abdel-Azim H, Jodele S, Kapoor N, Oberley MJ, et al. Bilateral retinal detachment after chimeric antigen receptor T-cell therapy. Blood Adv (2020) 4(10):2158–62. doi: 10.1182/Bloodadvances.2020001450
52. Gu B, Shi BY, Zhang X, Zhou SY, Chu JH, Wu XJ, et al. Allogeneic haematopoietic stem cell transplantation improves outcome of adults with Relapsed/Refractory Philadelphia chromosome-positive acute lymphoblastic leukemia entering remission following CD19 chimeric antigen receptor T cells. Bone Marrow Transplant (2021) 56(1):91–100. doi: 10.1038/s41409-020-0982-6
53. Li Z, Zheng Q, Wu F, Zhang C, Xu T, Zhao Y, et al. Long-term follow-up of allogeneic hematopoietic stem cell transplantation after chimeric antigen receptor T-cell therapy in Relapsed/Refractory b-cell acute lymphoblastic leukemia. Blood (2021) 138(Supplement 1):1820–20. doi: 10.1182/Blood-2021-150192
54. Hu GH, Zhao XY, Zuo YX, Chang YJ, Suo P, Wu J, et al. Unmanipulated haploidentical hematopoietic stem cell transplantation is an excellent option for children and young adult Relapsed/Refractory Philadelphia chromosome-negative b-cell acute lymphoblastic leukemia after CAR-T-Cell therapy. Leukemia (2021) 35(11):3092–100. doi: 10.1038/s41375-021-01236-y
55. Zhao H, Wei J, Wei G, Luo Y, Shi J, Cui Q, et al. Pre-transplant mrd negativity predicts favorable outcomes of CAR-T therapy followed by haploidentical HSCT for Relapsed/Refractory acute lymphoblastic leukemia: A multi-center retrospective study. J Hematol Oncol (2020) 13(1):42. doi: 10.1186/s13045-020-00873-7
56. Zhang X, Lu XA, Yang J, Zhang G, Li J, Song L, et al. Efficacy and safety of anti-CD19 CAR T-cell therapy in 110 patients with b-cell acute lymphoblastic leukemia with high-risk features. Blood Adv (2020) 4(10):2325–38. doi: 10.1182/Bloodadvances.2020001466
57. Park JH, Riviere I, Gonen M, Wang X, Senechal B, Curran KJ, et al. Long-term follow-up of CD19 CAR therapy in acute lymphoblastic leukemia. N Engl J Med (2018) 378(5):449–59. doi: 10.1056/NEJMoa1709919
58. Shadman M, Gauthier J, Hay KA, Voutsinas JM, Milano F, Li A, et al. Safety of allogeneic hematopoietic cell transplant in adults after CD19-targeted CAR T-cell therapy. Blood Adv (2019) 3(20):3062–69. doi: 10.1182/Bloodadvances.2019000593
59. Zhao XY, Xu ZL, Mo XD, Chen YH, Lv M, Cheng YF, et al. Preemptive donor-derived anti-CD19 CAR T-cell infusion showed a promising anti-leukemia effect against relapse in MRD-positive b-ALL after allogeneic hematopoietic stem cell transplantation. Leukemia (2022) 36(1):267–70. doi: 10.1038/s41375-021-01351-w
60. Kebriaei P, Singh H, Huls MH, Figliola MJ, Bassett R, Olivares S, et al. Phase I trials using sleeping beauty to generate CD19-specific CAR T cells. J Clin Invest (2016) 126(9):3363–76. doi: 10.1172/jci86721
61. Yang F, Shi H, Lei Y, Li R, Xu T, Deng B, et al. Allogeneic hematopoietic stem cell transplantation with conditioning including donor humanized CAR-T cells for Refractory/Relapsed b-cell non-Hodgkin lymphoma and multiple myeloma. Blood (2021) 138:557. doi: 10.1182/Blood-2021-146767
62. Wei J, Xiao M, Mao Z, Wang N, Cao Y, Xiao Y, et al. Outcome of aggressive b-cell lymphoma with TP53 alterations administered with CAR T-cell cocktail alone or in combination with ASCT. Signal Transduct Target Ther (2022) 7(1):101. doi: 10.1038/s41392-022-00924-0
63. Wang T, Xu L, Gao L, Tang G, Chen L, Chen J, et al. Chimeric antigen receptor T-cell therapy combined with autologous stem cell transplantation improved progression-free survival of relapsed or refractory diffuse Large b-cell lymphoma patients: A single-center, retrospective, cohort study. Hematol Oncol (2022). doi: 10.1002/hon.2975
64. Cao Y, Xiao Y, Wang N, Wang G, Huang L, Hong Z, et al. CD19/CD22 chimeric antigen receptor T cell cocktail therapy following autologous transplantation in patients with Relapsed/Refractory aggressive b cell lymphomas. Transplant Cell Ther (2021) 27(11):910.e1–10.e11. doi: 10.1016/j.jtct.2021.08.012
65. Hu K, Yang F, Liu R, Xu T, Zheng P, Feng S, et al. Auto hematopoietic stem cell transplantation combined with another target humanized CAR-T cells for Refractory/Relapsed b-cell non-Hodgkin lymphoma after failure of murinized CD19-CAR-T therapy. Blood (2021) 138(Supplement 1):657–57. doi: 10.1182/Blood-2021-146801
66. Wu J, Meng F, Cao Y, Zhang Y, Zhu X, Wang N, et al. Sequential CD19/22 CAR T-cell immunotherapy following autologous stem cell transplantation for central nervous system lymphoma. Blood Cancer J (2021) 11(7):131. doi: 10.1038/s41408-021-00523-2
67. Wang T, Gao L, Wang Y, Zhu W, Xu L, Wang Y, et al. Hematopoietic stem cell transplantation and chimeric antigen receptor T cell for relapsed or refractory diffuse Large b-cell lymphoma. Immunotherapy (2020) 12(13):997–1006. doi: 10.2217/imt-2020-0075
68. Sauter CS, Senechal B, Riviere I, Ni A, Bernal Y, Wang X, et al. CD19 CAR T cells following autologous transplantation in poor-risk relapsed and refractory b-cell non-Hodgkin lymphoma. Blood (2019) 134(7):626–35. doi: 10.1182/Blood.2018883421
69. Wang X, Popplewell LL, Wagner JR, Naranjo A, Blanchard MS, Mott MR, et al. Phase 1 studies of central memory-derived CD19 CAR T-cell therapy following autologous HSCT in patients with b-cell NHL. Blood (2016) 127(24):2980–90. doi: 10.1182/Blood-2015-12-686725
70. Garfall AL, Stadtmauer EA, Hwang WT, Lacey SF, Melenhorst JJ, Krevvata M, et al. Anti-CD19 CAR T cells with high-dose melphalan and autologous stem cell transplantation for refractory multiple myeloma. JCI Insight (2018) 3(8):1–14. doi: 10.1172/jci.insight.120505
71. Shi X, Yan L, Shang J, Qu S, Kang L, Zhou J, et al. Tandom autologous transplantation and combined infusion of CD19 and bcma-specific chimeric antigen receptor T cells for high risk MM: Initial safety and efficacy report from a clinical pilot study. Blood (2018) 132:1009. doi: 10.1182/Blood-2018-99-117964
72. Xu X, Chen S, Zhao Z, Xiao X, Huang S, Huo Z, et al. Consolidative hematopoietic stem cell transplantation after CD19 CAR-T cell therapy for acute lymphoblastic leukemia: A systematic review and meta-analysis. Front Oncol (2021) 11:651944. doi: 10.3389/fonc.2021.651944
73. Pan J, Yang JF, Deng BP, Zhao XJ, Zhang X, Lin YH, et al. High efficacy and safety of low-dose CD19-directed CAR-T cell therapy in 51 refractory or relapsed b acute lymphoblastic leukemia patients. Leukemia (2017) 31(12):2587–93. doi: 10.1038/leu.2017.145
74. Jiang H, Li C, Yin P, Guo T, Liu L, Xia L, et al. Anti-CD19 chimeric antigen receptor-modified T-cell therapy bridging to allogeneic hematopoietic stem cell transplantation for Relapsed/Refractory b-cell acute lymphoblastic leukemia: An open-label pragmatic clinical trial. Am J Hematol (2019) 94(10):1113–22. doi: 10.1002/ajh.25582
75. Lamble A, Myers RM, Taraseviciute A, John S, Yates B, Steinberg SM, et al. Preinfusion factors impacting relapse immunophenotype following CD19 CAR T cells. Blood Adv (2022). doi: 10.1182/Bloodadvances.2022007423
76. Jiang H, Hu Y, Mei H. Consolidative allogeneic hematopoietic stem cell transplantation after chimeric antigen receptor T-cell therapy for Relapsed/Refractory b-cell acute lymphoblastic leukemia: Who? when? why? biomark Res (2020) 8(1):66. doi: 10.1186/s40364-020-00247-8
77. Fabrizio VA, Kernan NA, Boulad F, Cancio M, Allen J, Higman M, et al. Low toxicity and favorable overall survival in Relapsed/Refractory b-all following CAR T cells and CD34-selected T-cell depleted allogeneic hematopoietic cell transplant. Bone Marrow Transplant (2020) 55(11):2160–69. doi: 10.1038/s41409-020-0926-1
78. Depil S, Duchateau P, Grupp SA, Mufti G, Poirot L. 'Off-the-Shelf' allogeneic CAR T cells: Development and challenges. Nat Rev Drug Discovery (2020) 19(3):185–99. doi: 10.1038/s41573-019-0051-2
79. Yang M, Wang L, Ni M, Neuber B, Wang S, Gong W, et al. Dual effects of cyclooxygenase inhibitors in combination with CD19. CAR-T cell immunotherapy. Front Immunol (2021) 12:670088. doi: 10.3389/fimmu.2021.670088
80. Yu C, Cai B, Wang Y, Wu Z, Hu K, Sun Q, et al. Co-Infusion of high-dose haploidentical donor cells and CD19-targeted CART cells achieves complete remission, successful donor engraftment and significant CART amplification in advanced all. Ther Adv Med Oncol (2020) 12:1758835920927605. doi: 10.1177/1758835920927605
81. Cai B, Guo M, Wang Y, Zhang Y, Yang J, Guo Y, et al. Co-Infusion of haplo-identical CD19-chimeric antigen receptor T cells and stem cells achieved full donor engraftment in refractory acute lymphoblastic leukemia. J Hematol Oncol (2016) 9(1):131. doi: 10.1186/s13045-016-0357-z
82. Zhang C, Kong PY, Li S, Chen T, Ni X, Li Y, et al. Donor-derived CAR-T cells serve as a reduced-intensity conditioning regimen for haploidentical stem cell transplantation in treatment of Relapsed/Refractory acute lymphoblastic leukemia: Case report and review of the literature. J Immunother (Hagerstown Md 1997) (2018) 41(6):306–11. doi: 10.1097/cji.0000000000000233
83. Bazarbachi AH, Al Hamed R, Malard F, Bazarbachi A, Harousseau JL, Mohty M. Induction therapy prior to autologous stem cell transplantation (ASCT) in newly diagnosed multiple myeloma: An update. Blood Cancer J (2022) 12(3):47. doi: 10.1038/s41408-022-00645-1
84. Galli E, Sorà F, Hohaus S, Bellesi S, Autore F, Metafuni E, et al. Autologous stem cell transplantation as bridging therapy followed by CD19 CAR-T cells in relapsed-refractory Large b cell lymphoma. Bone Marrow Transplant (2022) 57(5):837–9. doi: 10.1038/s41409-022-01632-7
85. Wen T, Wang J, Shi Y, Qian H, Liu P. Inhibitors targeting bruton's tyrosine kinase in cancers: Drug development advances. Leukemia (2021) 35(2):312–32. doi: 10.1038/s41375-020-01072-6
86. Dubovsky JA, Beckwith KA, Natarajan G, Woyach JA, Jaglowski S, Zhong Y, et al. Ibrutinib is an irreversible molecular inhibitor of ITK driving a Th1-selective pressure in T lymphocytes. Blood (2013) 122(15):2539–49. doi: 10.1182/Blood-2013-06-507947
87. Long M, Beckwith K, Do P, Mundy BL, Gordon A, Lehman AM, et al. Ibrutinib treatment improves T cell number and function in CLL patients. J Clin Invest (2017) 127(8):3052–64. doi: 10.1172/jci89756
88. Fraietta JA, Beckwith KA, Patel PR, Ruella M, Zheng Z, Barrett DM, et al. Ibrutinib enhances chimeric antigen receptor T-cell engraftment and efficacy in leukemia. Blood (2016) 127(9):1117–27. doi: 10.1182/Blood-2015-11-679134
89. Gauthier J, Hirayama AV, Purushe J, Hay KA, Lymp J, Li DH, et al. Feasibility and efficacy of CD19-targeted CAR T cells with concurrent ibrutinib for CLL after ibrutinib failure. Blood (2020) 135(19):1650–60. doi: 10.1182/Blood.2019002936
90. Gill SI, Frey NV, Hexner E, Metzger S, O'Brien M, Hwang WT, et al. Anti-CD19 CAR T cells in combination with ibrutinib for the treatment of chronic lymphocytic leukemia. Blood Adv (2022). doi: 10.1182/Bloodadvances.2022007317
91. Fan F, Yoo HJ, Stock S, Wang L, Liu Y, Schubert ML, et al. Ibrutinib for improved chimeric antigen receptor T-cell production for chronic lymphocytic leukemia patients. Int J Cancer (2021) 148(2):419–28. doi: 10.1002/ijc.33212
92. Liu M, Deng H, Mu J, Li Q, Pu Y, Jiang Y, et al. Ibrutinib improves the efficacy of anti-CD19-CAR T-cell therapy in patients with refractory non-Hodgkin lymphoma. Cancer Sci (2021) 112(7):2642–51. doi: 10.1111/cas.14915
93. Scarfò I, Gallagher KM, Leick MB, Kann MC, Budka J, Sowrirajan B, et al. Effects of prior exposure to tec kinase (BTK/ITK) inhibitors on KTE-X19 products. Blood (2021) 138(Supplement 1):3849. doi: 10.1182/Blood-2021-146941
94. Gill SI, Vides V, Frey NV, Metzger S, O'Brien M, Hexner E, et al. Prospective clinical trial of anti-CD19 CAR T cells in combination with ibrutinib for the treatment of chronic lymphocytic leukemia shows a high response rate. Blood (2018) 132(Supplement 1):298. doi: 10.1182/Blood-2018-99-115418
95. Zhu S, Gokhale S, Jung J, Spirollari E, Tsai J, Arceo J, et al. Multifaceted immunomodulatory effects of the BTK inhibitors ibrutinib and acalabrutinib on different immune cell subsets - beyond b lymphocytes. Front Cell Dev Biol (2021) 9:727531. doi: 10.3389/fcell.2021.727531
96. Qin JS, Johnstone TG, Baturevych A, Hause RJ, Ragan SP, Clouser CR, et al. Antitumor potency of an anti-CD19 chimeric antigen receptor T-cell therapy, lisocabtagene maraleucel in combination with ibrutinib or acalabrutinib. J Immunother (2020) 43(4):107–20. doi: 10.1097/cji.0000000000000307
97. Vanhaesebroeck B, Perry MWD, Brown JR, André F, Okkenhaug K. PI3K inhibitors are finally coming of age. Nat Rev Drug Discovery (2021) 20(10):741–69. doi: 10.1038/s41573-021-00209-1
98. Funk CR, Wang S, Chen KZ, Waller A, Sharma A, Edgar CL, et al. PI3Kδ/Γ inhibition promotes human CART cell epigenetic and metabolic reprogramming to enhance antitumor cytotoxicity. Blood (2022) 139(4):523–37. doi: 10.1182/Blood.2021011597
99. Zheng W, O'Hear CE, Alli R, Basham JH, Abdelsamed HA, Palmer LE, et al. PI3K orchestration of the in vivo persistence of chimeric antigen receptor-modified T cells. Leukemia (2018) 32(5):1157–67. doi: 10.1038/s41375-017-0008-6
100. Raje NS, Shah N, Jagannath S, Kaufman JL, Siegel DS, Munshi NC, et al. Updated clinical and correlative results from the phase I CRB-402 study of the BCMA-targeted CAR T cell therapy Bb21217 in patients with relapsed and refractory multiple myeloma. Blood (2021) 138:548. doi: 10.1182/Blood-2021-146518
101. Urak R, Walter M, Lim L, Wong CW, Budde LE, Thomas S, et al. Ex vivo akt inhibition promotes the generation of potent CD19CAR T cells for adoptive immunotherapy. J Immunother Cancer (2017) 5(1):26. doi: 10.1186/s40425-017-0227-4
102. Klebanoff CA, Crompton JG, Leonardi AJ, Yamamoto TN, Chandran SS, Eil RL, et al. Inhibition of akt signaling uncouples T cell differentiation from expansion for receptor-engineered adoptive immunotherapy. JCI Insight (2017) 2(23):e95103. doi: 10.1172/jci.insight.95103
103. Nian Z, Zheng X, Dou Y, Du X, Zhou L, Fu B, et al. Rapamycin pretreatment rescues the bone marrow AML cell elimination capacity of CAR-T cells. Clin Cancer Res (2021) 27(21):6026–38. doi: 10.1158/1078-0432
104. Zhang H, Hu Y, Shao M, Teng X, Jiang P, Wang X, et al. Dasatinib enhances anti-leukemia efficacy of chimeric antigen receptor T cells by inhibiting cell differentiation and exhaustion. J Hematol Oncol (2021) 14(1):113. doi: 10.1186/s13045-021-01117-y
105. Weber EW, Parker KR, Sotillo E, Lynn RC, Anbunathan H, Lattin J, et al. Transient rest restores functionality in exhausted CAR-T cells through epigenetic remodeling. Science (2021) 372(6537):1786. doi: 10.1126/science.aba1786
106. Mestermann K, Giavridis T, Weber J, Rydzek J, Frenz S, Nerreter T, et al. The tyrosine kinase inhibitor dasatinib acts as a pharmacologic on/off switch for CAR T cells. Sci Transl Med (2019) 11(499):5907. doi: 10.1126/scitranslmed.aau5907
107. Wen J, Liu D, Zhao L. Small molecules targeting γ-secretase and their potential biological applications. Eur J Med Chem (2022) 232:114169. doi: 10.1016/j.ejmech.2022.114169
108. Cowan AJ, Pont M, Sather BD, Turtle CJ, Till BG, Nagengast AM, et al. Efficacy and safety of fully human BCMA CAR T cells in combination with a gamma secretase inhibitor to increase BCMA surface expression in patients with relapsed or refractory multiple myeloma. Blood (2019) 134:204. doi: 10.1182/Blood-2019-129405
109. Garcia-Guerrero E, Rodríguez-Lobato LG, Danhof S, Sierro-Martínez B, Goetz R, Sauer M, et al. Atra augments BCMA expression on myeloma cells and enhances recognition by BCMA-CAR T-cells. Blood (2020) 136:13–4. doi: 10.1182/Blood-2020-142572
110. Kale J, Osterlund EJ, Andrews DW. BCL-2 family proteins: Changing partners in the dance towards death. Cell Death Differ (2018) 25(1):65–80. doi: 10.1038/cdd.2017.186
111. Warren CF, Wong-Brown MW, Bowden NA. BCL-2 family isoforms in apoptosis and cancer. Cell Death Dis (2019) 10(3):1–12. doi: 10.1038/s41419-019-1407-6
112. Vogler M, Hamali HA, Sun X-M, Bampton ET, Dinsdale D, Snowden RT, et al. BCL2/BCL-xl inhibition induces apoptosis, disrupts cellular calcium homeostasis, and prevents platelet activation. Blood (2011) 117(26):7145–54. doi: 10.1182/Blood-2011-03-344812
113. Yang M, Wang L, Ni M, Neuber B, Wang S, Gong W, et al. Pre-sensitization of malignant b cells through venetoclax significantly improves the cytotoxic efficacy of CD19.CAR-T cells. Front Immunol (2020) 11:608167. doi: 10.3389/fimmu.2020.608167
114. Mandeville TK, Mavis C, Gu J, Olejniczak S, Paragh G, Dey P, et al. Contribution of BCL-2 inhibitor venetoclax toward anti-CD19 CAR T cell efficacy in Relapsed/Refractory diffuse Large b cell lymphoma. Blood (2021) 138:1719. doi: 10.1182/Blood-2021-154128
115. Karlsson H, Lindqvist AC, Fransson M, Paul-Wetterberg G, Nilsson B, Essand M, et al. Combining CAR T cells and the BCL-2 family apoptosis inhibitor ABT-737 for treating b-cell malignancy. Cancer Gene Ther (2013) 20(7):386–93. doi: 10.1038/cgt.2013.35
116. Zhang X, Lv X, Song YJB. Short-term culture with IL-2 is beneficial for potent memory chimeric antigen receptor T cell production. Biochem Biophys Res Commun (2018) 495(2):1833–38. doi: 10.1016/j.bbrc.2017.12.041
117. Xu XJ, Song DG, Poussin M, Ye Q, Sharma P, Rodríguez-García A, et al. Multiparameter comparative analysis reveals differential impacts of various cytokines on CART cell phenotype and function ex vivo and in vivo. Oncotarget (2016) 7(50):82354–68. doi: 10.18632/oncotarget.10510
118. Xu Y, Zhang M, Ramos CA, Durett A, Liu E, Dakhova O, et al. Closely related T-memory stem cells correlate with in vivo expansion of CAR. CD19-T cells and are preserved by IL-7 and IL-15. Blood (2014) 123(24):3750–59. doi: 10.1182/Blood-2014-01-552174
119. Alvarez-Fernandez C, Escribà-Garcia L, Vidal S, Sierra J, Briones J. A short CD3/CD28 costimulation combined with IL-21 enhance the generation of human memory stem T cells for adoptive immunotherapy. J Transl Med (2016) 14(1):1–10. doi: 10.1186/s12967-016-0973-y
120. Du L, Nai Y, Shen M, Li T, Huang J, Han X, et al. IL-21 optimizes the CAR-T cell preparation through improving lentivirus mediated transfection efficiency of T cells and enhancing CAR-T cell cytotoxic activities. Front Mol Biosci (2021) 8:675179. doi: 10.3389/fmolb.2021.675179
121. Dahmani A, Janelle V, Carli C, Richaud M, Lamarche C, Khalili M, et al. TGFβ programs central memory differentiation in ex vivo-stimulated human T cells. Cancer Immunol Res (2019) 7(9):1426–39. doi: 10.1158/2326-6066.Cir-18-0691
122. Chou C, Fraessle S, Steinmetz R, Hawkins RM, Phi T-D, Busch D, et al. Combination of nktr-255, a polymer conjugated human IL-15, with CD19 CAR T cell immunotherapy in a preclinical lymphoma model. Blood (2019) 134:2866. doi: 10.1182/Blood-2019-125960
123. Ewing MM, Karper JC, Abdul S, de Jong RC, Peters HA, de Vries MR, et al. T-Cell Co-stimulation by CD28-CD80/86 and its negative regulator CTLA-4 strongly influence accelerated atherosclerosis development. Int J Cardiol (2013) 168(3):1965–74. doi: 10.1016/j.ijcard.2012.12.085
124. Kim MY, Jayasinghe R, Devenport JM, Ritchey JK, Rettig MP, O'Neal J, et al. A long-acting interleukin-7, rhIL-7-hyFc, enhances CAR T cell expansion, persistence, and anti-tumor activity. Nat Commun (2022) 13(1):3296. doi: 10.1038/s41467-022-30860-0
125. Agliardi G, Liuzzi AR, Hotblack A, De Feo D, Núñez N, Stowe CL, et al. Intratumoral IL-12 delivery empowers CAR-T cell immunotherapy in a pre-clinical model of glioblastoma. Nat Commun (2021) 12(1):1–11. doi: 10.1038/s41467-020-20599-x
126. Chmielewski M, Abken H. CAR T cells releasing IL-18 convert to T-bethigh Foxo1low effectors that exhibit augmented activity against advanced solid tumors. Cell Rep (2017) 21(11):3205–19. doi: 10.1016/j.celrep.2017.11.063
127. Bates S. Epigenetic therapies for cancer. N Engl J Med (2020) 383(7):650–63. doi: 10.1056/NEJMra1805035
128. Schmidl C, Delacher M, Huehn J, Feuerer M, Immunology C. Epigenetic mechanisms regulating T-cell responses. J Allergy Clin Immunol (2018) 142(3):728–43. doi: 10.1016/j.jaci.2018.07.014
129. Gore SD, Jones C, Kirkpatrick P. Decitabine. Nat Rev Drug Discov (2006) 5(11):891–2. doi: 10.1038/nrd2180
130. Li S, Xue L, Wang M, Qiang P, Xu H, Zhang X, et al. Decitabine enhances cytotoxic effect of T cells with an anti-CD19 chimeric antigen receptor in treatment of lymphoma. Onco Targets Ther (2019) 12:5627. doi: 10.2147/OTT.S198567
131. You L, Han Q, Zhu L, Zhu Y, Bao C, Yang C, et al. Decitabine-mediated epigenetic reprograming enhances anti-leukemia efficacy of CD123-targeted chimeric antigen receptor T-cells. Front Immunol (2020) 11:1787. doi: 10.3389/fimmu.2020.01787
132. Leick MB, Silva H, Scarfò I, Larson R, Choi BD, Bouffard AA, et al. Non-cleavable hinge enhances avidity and expansion of CAR-T cells for acute myeloid leukemia. Cancer Cell (2022) 40(5):494–508. doi: 10.1016/j.ccell.2022.04.001
133. El Khawanky N, Hughes A, Yu W, Myburgh R, Matschulla T, Taromi S, et al. Demethylating therapy increases anti-CD123 CAR T cell cytotoxicity against acute myeloid leukemia. Nat Commun (2021) 12(1):6436. doi: 10.1038/s41467-021-26683-0
134. Stübig T, Badbaran A, Luetkens T, Hildebrandt Y, Atanackovic D, Binder TM, et al. 5-azacytidine promotes an inhibitory T-cell phenotype and impairs immune mediated antileukemic activity. Mediators Inflammation (2014) 2014:418292. doi: 10.1155/2014/418292
135. Yang X, Yu Q, Xu H, Zhou J. Upregulation of CD22 by chidamide promotes CAR T cells functionality. Sci Rep (2021) 11(1):1–9. doi: 10.1038/s41598-021-00227-4
136. Torres-Collado AX, Jazirehi AR. Overcoming resistance of human non-hodgkin’s lymphoma to CD19-CAR ctl therapy by celecoxib and histone deacetylase inhibitors. Cancers (Basel) (2018) 10(6):200. doi: 10.3390/cancers10060200
137. Wang N, Wu R, Tang D, Kang R. The bet family in immunity and disease. Signal Transduct Target Ther (2021) 6(1):1–22. doi: 10.1038/s41392-020-00384-4
138. Kagoya Y, Nakatsugawa M, Yamashita Y, Ochi T, Guo T, Anczurowski M, et al. Bet bromodomain inhibition enhances T cell persistence and function in adoptive immunotherapy models. J Clin Invest (2016) 126(9):3479–94. doi: 10.1172/JCI86437
139. Guo A, Huang H, Zhu Z, Chen MJ, Shi H, Yuan S, et al. cBAF complex components and MYC cooperate early in CD8(+) T cell fate. Nature (2022) 607(7917):135–41. doi: 10.1038/s41586-022-04849-0
140. Petersen CT, Hassan M, Morris AB, Jeffery J, Lee K, Jagirdar N, et al. Improving T-cell expansion and function for adoptive T-cell therapy using ex vivo treatment with PI3Kδ inhibitors and VIP antagonists. Blood Adv (2018) 2(3):210–23. doi: 10.1182/bloodadvances.2017011254
141. Gurusamy D, Henning AN, Yamamoto TN, Yu Z, Zacharakis N, Krishna S, et al. Multi-phenotype crispr-Cas9 screen identifies P38 kinase as a target for adoptive immunotherapies. Cancer Cell (2020) 37(6):818–33.e9. doi: 10.1016/j.ccell.2020.05.004
142. He B, Wang L, Neuber B, Schmitt A, Kneisel N, Hoeger T, et al. Blockade of CD95/CD95L death signaling enhances CAR T cell persistence and antitumor efficacy. Blood (2019) 134:3226. doi: 10.1182/Blood-2019-122833
143. Wang L, Zhang Y, Anderson E, Lamble A, Orentas RJ. Bryostatin activates CAR T-cell antigen-Non-Specific killing (CTAK), and CAR-T nk-like killing for pre-b ALL, while blocking cytolysis of a burkitt lymphoma cell line. Front Immunol (2022) 13:825364. doi: 10.3389/fimmu.2022.825364
144. Pullarkat VA, Lacayo NJ, Jabbour E, Rubnitz JE, Bajel A, Laetsch TW, et al. Venetoclax and navitoclax in combination with chemotherapy in patients with relapsed or refractory acute lymphoblastic leukemia and lymphoblastic lymphoma. Cancer Discovery (2021) 11(6):1440–53. doi: 10.1158/2159-8290.CD-20-1465
145. Dufva O, Koski J, Maliniemi P, Ianevski A, Klievink J, Leitner J, et al. Integrated drug profiling and CRISPR screening identify essential pathways for CAR T-cell cytotoxicity. Blood (2020) 135(9):597–609. doi: 10.1182/blood.2019002121
146. Li C, Sun Y, Wang J, Tang L, Jiang H, Guo T, et al. Piggybac-generated CAR19-T cells plus lenalidomide cause durable complete remission of triple-hit Refractory/Relapsed DLBCL: A case report. Front Immunol (2021) 12:599493. doi: 10.3389/fimmu.2021.599493
147. Zhao G, Wei R, Feng L, Wu Y, He F, Xiao M, et al. Lenalidomide enhances the efficacy of anti-BCMA CAR-T treatment in Relapsed/Refractory multiple myeloma: A case report and revies of the literature. Cancer Immunol Immunother (2022) 71(1):39–44. doi: 10.1007/s00262-021-02959-8
148. Shi X, Yan L, Shang J, Kang L, Yan Z, Jin S, et al. Anti-CD19 and anti-BCMA CAR T cell therapy followed by lenalidomide maintenance after autologous stem-cell transplantation for high-risk newly diagnosed multiple myeloma. Am J Hematol (2022) 97(5):537–47. doi: 10.1002/ajh.26486
149. Chong EA, Alanio C, Svoboda J, Nasta SD, Landsburg DJ, Lacey SF, et al. Pembrolizumab for b-cell lymphomas relapsing after or refractory to CD19-directed CAR T-cell therapy. Blood (2022) 139(7):1026–38. doi: 10.1182/Blood.2021012634
150. CD19/CD22 dual-targeted CAR-T therapy active in Relapsed/Refractory DLBCL. Oncologist (2020) 25 Suppl 1(Suppl 1):S12–3. doi: 10.1634/theoncologist.2020-0560
151. Li AM, Hucks GE, Dinofia AM, Seif AE, Teachey DT, Baniewicz D, et al. Checkpoint inhibitors augment CD19-directed chimeric antigen receptor (CAR) T cell therapy in relapsed b-cell acute lymphoblastic leukemia. Blood (2018) 132:556. doi: 10.1182/Blood-2018-99-112572
152. Cao Y, Lu W, Sun R, Jin X, Cheng L, He X, et al. Anti-CD19 chimeric antigen receptor T cells in combination with nivolumab are safe and effective against Relapsed/Refractory b-cell non-Hodgkin lymphoma. Front Oncol (2019) 9:767. doi: 10.3389/fonc.2019.00767
153. Jacobson CA, Locke FL, Miklos DB, Herrera AF, Westin JR, Lee J, et al. End of phase 1 results from ZUMA-6: Axicabtagene ciloleucel (Axi-cel) in combination with atezolizumab for the treatment of patients with refractory diffuse Large b cell lymphoma. Blood (2018) 132:4192. doi: 10.1182/Blood-2018-99-111523
154. Hirayama AV, Gauthier J, Hay KA, Sheih A, Cherian S, Chen X, et al. Efficacy and toxicity of Jcar014 in combination with durvalumab for the treatment of patients with Relapsed/Refractory aggressive b-cell non-Hodgkin lymphoma. Blood (2018) 132(Supplement 1):1680–80. doi: 10.1182/Blood-2018-99-116745
155. Siddiqi T, Abramson J, Lee H, Schuster S, Hasskarl J, Montheard S, et al. Safety of lisocabtagene maraleucel given with durvalumab in patients with Relapsed/Refractory aggressive b-cell non Hodgkin lymphoma: First results from the platform study. Hematol Oncol (2019) 37:171–72. doi: 10.1002/hon.128_2629
156. Jackson Z, Hong C, Schauner R, Dropulic B, Caimi PF, de Lima M, et al. Sequential single cell transcriptional and protein marker profiling reveals tigit as a marker of CD19 CAR-T cell dysfunction in patients with non-hodgkin's lymphoma. Cancer Discovery (2022) 12:1586. doi: 10.1158/2159-8290.Cd-21-1586
157. Mailankody S, Jakubowiak AJ, Htut M, Costa LJ, Lee K, Ganguly S, et al. Orvacabtagene autoleucel (Orva-cel), a b-cell maturation antigen (BCMA)-directed CAR T cell therapy for patients (Pts) with Relapsed/Refractory multiple myeloma (RRMM): Update of the phase 1/2 evolve study (NCT03430011). J Clin Oncol (2020) 38:8504. doi: 10.1200/JCO.2020.38.15_suppl.8504
158. Liebers N, Duell J, Fitzgerald D, Kerkhoff A, Noerenberg D, Kaebisch E, et al. Polatuzumab vedotin as a salvage and bridging treatment in relapsed or refractory Large b-cell lymphomas. Blood Adv (2021) 5(13):2707–16. doi: 10.1182/Bloodadvances.2020004155
159. Shah NN, Highfill SL, Shalabi H, Yates B, Jin J, Wolters PL, et al. CD4/CD8 T-cell selection affects chimeric antigen receptor (CAR) T-cell potency and toxicity: Updated results from a phase I anti-CD22 CAR T-cell trial. J Clin Oncol (2020) 38(17):1938–50. doi: 10.1200/jco.19.03279
160. Schuster SJ, Bartlett NL, Assouline S, Yoon S-S, Bosch F, Sehn LH, et al. Mosunetuzumab induces complete remissions in poor prognosis non-Hodgkin lymphoma patients, including those who are resistant to or relapsing after chimeric antigen receptor T-cell (CAR-T) therapies, and is active in treatment through multiple lines. J Hematol Oncol (2019) 134:6. doi: 10.1186/s13045-016-0283-0
161. Hutchings M, Morschhauser F, Iacoboni G, Carlo-Stella C, Offner FC, Sureda A, et al. Glofitamab, a novel, bivalent CD20-targeting T-Cell-Engaging bispecific antibody, induces durable complete remissions in relapsed or refractory b-cell lymphoma: A phase I trial. J Clin Oncol (2021) 39(18):1959–70. doi: 10.1200/jco.20.03175
162. Wullenkord R, Reicherts C, Mikesch JH, Marx J, Wethmar K, Albring J, et al. Sequential therapy with inotuzumab ozogamicin, CD19 CAR T cells, and blinatumomab in an elderly patient with relapsed acute lymphoblastic leukemia. Ann Hematol (2021) 100(2):587–89. doi: 10.1007/s00277-020-04227-8
163. Cooper ML, Staser KW, Ritchey J, Niswonger J, Lee BH, Park J, et al. A long-acting pharmacological grade interleukin-7 molecule logarithmically accelerates ucart proliferation, differentiation, and tumor killing. Blood (2018) 132(Supplement 1):2199–99. doi: 10.1182/Blood-2018-99-117265
164. Pillai V, Muralidharan K, Meng W, Bagashev A, Oldridge DA, Rosenthal J, et al. CAR T-cell therapy is effective for CD19-dim b-lymphoblastic leukemia but is impacted by prior blinatumomab therapy. Blood Adv (2019) 3(22):3539–49. doi: 10.1182/Bloodadvances.2019000692
165. Jin J, Li Y, Liu Y, Jordan AA, McIntosh J, Vargas J, et al. Bispecific CD19-CD20 and CD19-CD22 CAR-T cells with glycogen synthase kinase (GSK)-3β inhibitor TWS119 treatment have superior therapeutic effects on mantle cell lymphoma. Blood (2021) 138(Supplement 1):1698. doi: 10.1182/Blood-2021-150000
166. Sabatino M, Hu J, Sommariva M, Gautam S, Fellowes V, Hocker JD, et al. Generation of clinical-grade CD19-specific CAR-modified CD8+ memory stem cells for the treatment of human b-cell malignancies. Blood (2016) 128(4):519–28. doi: 10.1182/Blood-2015-11-683847
167. Sengupta S, Katz SC, Sengupta S, Sampath P. Glycogen synthase kinase 3 inhibition lowers PD-1 expression, promotes long-term survival and memory generation in antigen-specific CAR-T cells. Cancer Lett (2018) 433:131–39. doi: 10.1016/j.canlet.2018.06.035
168. Pilipow K, Scamardella E, Puccio S, Gautam S, De Paoli F, Mazza EM, et al. Antioxidant metabolism regulates CD8+ T memory stem cell formation and antitumor immunity. JCI Insight (2018) 3(18):e122299. doi: 10.1172/jci.insight.122299
169. Risso V, Lafont E, Le Gallo M. Therapeutic approaches targeting CD95L/CD95 signaling in cancer and autoimmune diseases. Cell Death Dis (2022) 13(3):1–32. doi: 10.1038/s41419-022-04688-x
170. Giuffrida L, Sek K, Henderson MA, Lai J, Chen AXY, Meyran D, et al. CRISPR/Cas9 mediated deletion of the adenosine A2A receptor enhances CAR T cell efficacy. Nat Commun (2021) 12(1):3236. doi: 10.1038/s41467-021-23331-5
171. Masoumi E, Jafarzadeh L, Mirzaei HR, Alishah K, Fallah-Mehrjardi K, Rostamian H, et al. Genetic and pharmacological targeting of A2A receptor improves function of anti-mesothelin CAR T cells. J Exp Clin Cancer Res (2020) 39(1):49. doi: 10.1186/s13046-020-01546-6
172. Sterner RM, Sakemura R, Cox MJ, Yang N, Khadka RH, Forsman CL, et al. GM-CSF inhibition reduces cytokine release syndrome and neuroinflammation but enhances CAR-T cell function in xenografts. Blood (2019) 133(7):697–709. doi: 10.1182/Blood-2018-10-881722
173. Works M, Soni N, Hauskins C, Sierra C, Baturevych A, Jones JC, et al. Anti-B-Cell maturation antigen chimeric antigen receptor T cell function against multiple myeloma is enhanced in the presence of lenalidomide. Mol Cancer Ther (2019) 18(12):2246–57. doi: 10.1158/1535-7163.Mct-18-1146
174. Wang X, Walter M, Urak R, Weng L, Huynh C, Lim L, et al. Lenalidomide enhances the function of CS1 chimeric antigen receptor-redirected T cells against multiple myeloma. Clin Cancer Res (2018) 24(1):106–19. doi: 10.1158/1078-0432.Ccr-17-0344
175. Wang J, Deng Q, Jiang YY, Zhang R, Zhu HB, Meng JX, et al. CAR-T 19 combined with reduced-dose PD-1 blockade therapy for treatment of refractory follicular lymphoma: A case report. Oncol Lett (2019) 18(5):4415–20. doi: 10.3892/ol.2019.10783
176. Zah E, Nam E, Bhuvan V, Tran U, Ji BY, Gosliner SB, et al. Systematically optimized BCMA/CS1 bispecific CAR-T cells robustly control heterogeneous multiple myeloma. Nat Commun (2020) 11(1):1–13. doi: 10.1038/s41467-020-16160-5
177. Sun C, Shou P, Du H, Hirabayashi K, Chen Y, Herring LE, et al. THEMIS-SHP1 recruitment by 4-1BB tunes LCK-mediated priming of chimeric antigen receptor-redirected T cells. Cancer Cell (2020) 37(2):216–25.e6. doi: 10.1016/j.ccell.2019.12.014
178. Chester C, Sanmamed MF, Wang J, Melero I. The journal of the American society of hematology. immunotherapy targeting 4-1BB: Mechanistic rationale, clinical results, and future strategies. Blood (2018) 131(1):49–57. doi: 10.1182/Blood-2017-06-741041
179. Mardiana S, John LB, Henderson MA, Slaney CY, von Scheidt B, Giuffrida L, et al. A multifunctional role for adjuvant anti-4-1BB therapy in augmenting antitumor response by chimeric antigen receptor T cells. Cancer Res (2017) 77(6):1296–309. doi: 10.1158/0008-5472.CAN-16-1831
180. Reshef R, Miklos DB, Timmerman JM, Jacobson CA, Bennani NN, Rossi JM, et al. ZUMA-11: A phase 1/2 multicenter study of axicabtagene ciloleucel (Axi-cel)+ utomilumab patients with refractory Large b cell lymphoma. Blood (2019) 134:4084. doi: 10.1182/Blood-2019-123772
181. Krejcik J, Casneuf T, Nijhof IS, Verbist B, Bald J, Plesner T, et al. Daratumumab depletes CD38+ immune regulatory cells, promotes T-cell expansion, and skews T-cell repertoire in multiple myeloma. Blood (2016) 128(3):384–94. doi: 10.1182/Blood-2015-12-687749
182. Guo Z, Tu S, Yu S, Wu L, Pan W, Chang N, et al. Preclinical and clinical advances in dual-target chimeric antigen receptor therapy for hematological malignancies. Cancer Sci (2021) 112(4):1357–68. doi: 10.1111/cas.14799
183. Zeng C, Cheng J, Li T, Huang J, Li C, Jiang L, et al. Efficacy and toxicity for CD22/CD19 chimeric antigen receptor T-cell therapy in patients with Relapsed/Refractory aggressive b-cell lymphoma involving the gastrointestinal tract. Cytotherapy (2020) 22(3):166–71. doi: 10.1016/j.jcyt.2020.01.008
184. Liu Y, Deng B, Hu B, Zhang W, Zhu Q, Liu Y, et al. Sequential different b-cell antigen-targeted CAR T-cell therapy for pediatric Refractory/Relapsed burkitt lymphoma. Blood Adv (2022) 6(3):717–30. doi: 10.1182/bloodadvances.2021004557
185. Wei J, Mao Z, Wang N, Huang L, Cao Y, Sun W, et al. Long-term outcomes of Relapsed/Refractory double-hit lymphoma (R/R DHL) treated with CD19/22 CAR T-cell cocktail therapy. Clin Transl Med (2020) 10(5):e176. doi: 10.1002/ctm2.176
186. Yan N, Wang N, Wang G, Huang L, Li C, Wang D, et al. CAR19/22 T cell cocktail therapy for b-ALL relapsed after allogeneic hematopoietic stem cell transplantation. Cytotherapy (2022) 24(8):841–49. doi: 10.1016/j.jcyt.2022.01.011
187. Liu S, Deng B, Yin Z, Lin Y, An L, Liu D, et al. Combination of CD19 and CD22 CAR-T cell therapy in relapsed b-cell acute lymphoblastic leukemia after allogeneic transplantation. Am J Hematol (2021) 96(6):671–9. doi: 10.1002/ajh.26160
188. Pan J, Zuo S, Deng B, Xu X, Li C, Zheng Q, et al. Sequential CD19-22 CAR T therapy induces sustained remission in children with R/R b-ALL. Blood (2020) 135(5):387–91. doi: 10.1182/blood.2019003293
189. Yan Z, Cao J, Cheng H, Qiao J, Zhang H, Wang Y, et al. A combination of humanised anti-CD19 and anti-BCMA CAR T cells in patients with relapsed or refractory multiple myeloma: A single-arm, phase 2 trial. Lancet Haematol (2019) 6(10):e521–e29. doi: 10.1016/s2352-3026(19)30115-2
190. Wang Y, Cao J, Gu W, Shi M, Lan J, Yan Z, et al. Long-term follow-up of combination of b-cell maturation antigen and CD19 chimeric antigen receptor T cells in multiple myeloma. J Clin Oncol (2022) 40(20):2246–56. doi: 10.1200/JCO.21.01676
191. Yan L, Qu S, Shang J, Shi X, Kang L, Xu N, et al. Sequential CD19 and BCMA-specific CAR T-cell treatment elicits sustained remission of relapsed and/or refractory myeloma. Cancer Med (2021) 10(2):563–74. doi: 10.1002/cam4.3624
192. Wang N, Hu X, Cao W, Li C, Xiao Y, Cao Y, et al. Efficacy and safety of CAR19/22 T-cell cocktail therapy in patients with Refractory/Relapsed b-cell malignancies. Blood (2020) 135(1):17–27. doi: 10.1182/Blood.2019000017
193. Pan J, Tan Y, Deng B, Tong C, Hua L, Ling Z, et al. Frequent occurrence of CD19-negative relapse after CD19 CAR T and consolidation therapy in 14 TP53-mutated R/R b-ALL children. Leukemia (2020) 34(12):3382–87. doi: 10.1038/s41375-020-0831-z
194. Paiva B, Puig N, Cedena M-T, de Jong BG, Ruiz Y, Rapado I, et al. Differentiation stage of myeloma plasma cells: Biological and clinical significance. Leukemia (2017) 31(2):382–92. doi: 10.1038/leu.2016.211
Keywords: CAR T cell, resistance, relapse, combination therapy, chemotherapy, radiotherapy, haematological stem cell transplantation, targeted therapy
Citation: Xiao X, Wang Y, Zou Z, Yang Y, Wang X, Xin X, Tu S and Li Y (2022) Combination strategies to optimize the efficacy of chimeric antigen receptor T cell therapy in haematological malignancies. Front. Immunol. 13:954235. doi: 10.3389/fimmu.2022.954235
Received: 27 May 2022; Accepted: 01 August 2022;
Published: 23 August 2022.
Edited by:
Min Xiao, Huazhong University of Science and Technology, ChinaReviewed by:
Jianmin Yang, Second Military Medical University, ChinaCopyright © 2022 Xiao, Wang, Zou, Yang, Wang, Xin, Tu and Li. This is an open-access article distributed under the terms of the Creative Commons Attribution License (CC BY). The use, distribution or reproduction in other forums is permitted, provided the original author(s) and the copyright owner(s) are credited and that the original publication in this journal is cited, in accordance with accepted academic practice. No use, distribution or reproduction is permitted which does not comply with these terms.
*Correspondence: Sanfang Tu, ZG9jdG9ydHV0dUAxNjMuY29t; Yuhua Li, bGl5dWh1YTIwMTFnekAxNjMuY29t
†These authors have contributed equally to this work
Disclaimer: All claims expressed in this article are solely those of the authors and do not necessarily represent those of their affiliated organizations, or those of the publisher, the editors and the reviewers. Any product that may be evaluated in this article or claim that may be made by its manufacturer is not guaranteed or endorsed by the publisher.
Research integrity at Frontiers
Learn more about the work of our research integrity team to safeguard the quality of each article we publish.