- 1Wuxi School of Medicine, Jiangnan University, Wuxi, China
- 2CAPsoul Medical Biotechnology Company, Ltd., Beijing, China
- 3Department of Gynaecology, Lishui Hospital of Traditional Chinese Medicine, Lishui, China
- 4First School of Clinical Medicine, Zhejiang Chinese Medicine Museum, Zhejiang Chinese Medical University, Hangzhou, China
Exosomes, nano-sized extracellular vesicles for intercellular communications, are gaining rapid momentum as a novel strategy for the diagnosis and therapeutics of a spectrum of diseases including cancers. Secreted by various cell sources, exosomes pertain numerous functionalities from their parental cells and have enhanced stability that enable them with many features favorable for clinical use and commercialization. This paper focuses on the possible roles of exosomes in cancer therapeutics and reviews current exosome-based innovations toward enhanced cancer management and challenges that limit their clinical translation. Importantly, this paper casts insights on how cold atmospheric plasma, an emerging anticancer strategy, may aid in innovations on exosome-based onco-therapeutics toward improved control over cancers.
1 Introduction
Exosomes, firstly discovered in the early 1980s, represent a class of extracellular vehicles (EVs) at a size of 30~150 nm in diameter that are secreted by all types of cells (1, 2). Exosomes were initially considered as a means for maturing reticulocytes to get rid of superfluous proteins and discard garbage (1, 2). Accumulating evidence has suggested their other roles besides eliminating unwanted molecules from parental cells such as intercellular communications, cell content exchange, immune system modulation, antigen presentation, and pathogen propagation (3–5). With our incremental understanding on exosome, its prominent functionalities under both normal and pathophysiological conditions such as lactation (6), immune homeostasis (7), neuronal signaling (8), and disease development (9, 10) have been acknowledged and taken advantages of for the purpose of theranostics.
A rising momentum has been witnessed on exosome recently, in both academia and industry. Exosome has been integrated into the field of precision medicine as it provides an extremely useful source of biomarkers for cancer diagnosis and an excellent tool for immune-therapeutics and drug delivery (7, 11). The diverse range of biofluids capable of producing exosomes makes exosome-based diagnostics minimally invasive, easy to use, and fast in detection (12, 13). By isolating a patient’s exosomes, modifying them with appropriate nucleic acids or proteins, and transfusing them back to the patient (14, 15), exosomes can be administrated in clinical practice in a similar fashion to adoptive cell therapy (ACT) that is characteristic of extreme personalization. At the same time, exosomes offer a cell-free solution. That is, they can be manufactured ex vivo free of cells and thus be exempted from the risks and difficulties of administering cells to patients (16).
For these beneficial traits, the era of exosomes has arrived. In academia, there had been approximately 20,000 publications on exosomes, among which three quarters have been published within the past 5 years. Clinically, at least 204 clinical trials associated with exosomes have been launched, with 114 and 74 trials related to therapeutics and diagnosis, respectively, and two diagnostic tests (i.e., Bio-Techne’s ExoDx Prostate IntelliScore Test for prostate cancers [17), Guardant360 CDx test for non-small cell lung cancers (18)] have been approved by the FDA. Commercially, at least seven partnership deals, eight large venture capital events, and two landmark acquisitions have occurred in the exosome industry during the past 5 years. Given the surging amounts of scientific publications, the rising number of clinical trials, and the swelling appetite among investors for exosome biotechnology, it is imperative to characterize the features of exosomes (derived from various sources) that enable their diversified innovative theranostic applications and, ultimately, our improved power over diseases including cancers.
Among the varied types of disorders and clinical applications where exosomes may intervene (19, 20), this review focuses on cancer therapeutics. We briefly introduce some basic information on exosomes in Section 2, categorize the primary characteristics of exosomes enabling their onco-therapeutic potential (i.e., immune modulation and cargo delivery) in Section 3, cast our insights on current and emerging innovations relevant to exosome-associated onco-therapeutic strategies in Sections 4 and 5, and focus on the status and challenges in the clinical translation of exosomes as an onco-therapeutic tool in Sections 6 and 7. Importantly, we forecast possible synergies cold atmospheric plasma (CAP), an emerging onco-therapeutic, can create with exosomes toward enhanced cancer control.
2 Exosome biogenesis and composition
The initial form of exosome in eukaryotic cells is early endosome which is tube-like and distributed in the peripheral part of the cytoplasm (3). When early endosome develops into late endosome, it becomes spherical, accumulates around the nucleus, and forms intraluminal vesicles inside the lumen (3). Late endosome either fuses with the lysosome toward content degradation or fuses with the cell membrane to release its intraluminal vesicles in the form of exosomes (3) (Supplementary Figure 1). Exosomes can be secreted either constitutively or in an inducible manner (21, 22). Tumor suppressors such as p53 can activate exosome production and secretion in response to external stimuli such as oxidative or toxic stress (22). It has been demonstrated that irradiation of human prostate cancer cells can trigger DNA damage and thus induce a p53-depenent increase in exosome secretion (23). Once exosomes are taken up by other cells, they can either release their inner content without affecting the membrane integrity or be entirely endocytosed and re-legated to clathrin-coated pits (3).
Exosomes contain many diversified cell surface molecules that allow them to participate in intercellular material exchange. The content of exosomes is complex which contains thousands of proteins and nucleic acids, as well as hundreds of lipids (24). Exosomes are enriched with proteins involved in antigen presentation such as CD1 and major histocompatibility molecules I and II (MHCI/II), co-stimulatory molecules such as CD86, adhesion molecules such as CD11b and CD54, heat shock proteins such as HSP70 and HSP90, cytoplasmic proteins such as Annexins and Rab proteins, membrane proteins such as CD55 and CD59, signal transduction proteins such as G-proteins, and protein kinases (3). Exosomes do not contain mitochondrial, nuclear, and endoplasmic proteins (25). Exosomes derived from immune cells display proteins that pertain their roles in immune responses in addition to typical exosome contents; e.g., exosomes secreted by dendritic cells (DCs) contain CD80 and CD86 for naïve CD4+ T-cell activation (26). Exosomes also contain numerous nucleic acids such as let-7, miRNA-1, miRNA-15, and miRNA-16 which play critical roles in angiogenesis, hematopoiesis, exocytosis, and tumorigenesis (25). Recent studies have reported the critical functionalities of exosome-derived long non-coding RNAs (lncRNAs) in cancer initiation and development. For instance, PTENP1 in exosomes derived from normal cells confers tumor-suppressive roles on breast cancer cells both in vitro and in vivo (27). Compared with the source cells, lipids contained in exosomes are enriched with cholesterol, sphingomyelin, glycosphingolipids, hexosylceramide, lactosylceramide, and phosphatidylserine and contain less phosphatidylcholine, phophatidylethanolamine, phosphatidylglycerol, phosphatidylinositol, and cholesteryl ester (28). Exosomes have asymmetric membrane bilayers regarding lipid decomposition (3). While sphigomyelins, sphingolipids, and most phosphatidylcholines are distributed in the outer leaflet of exosomes, all other types of lipids are mainly concentrated in the inner leaflet (28). Exosomal cargos are involved in various signalings in recipient cells and can modulate diverse biological processes such as autophagy (29) and inflammation (30).
3 Exosome for onco-therapeutics
3.1 Immunotherapy
3.1.1 Exosomes of immune origin
Innate and adaptive immune responses are two major immune systems of vertebrates (31). While DCs, natural killer cells (NKs), and macrophages are essential players in the innate immunity, B and T cells are major immune cells in the adaptive immune response (31). In the adaptive immune response that is specific to vertebrates, B cells recognize foreign antigens by themselves, and T cells identify antigens with the aid of antigen-presenting cells (APCs) such as DCs, macrophages, and B cells (31) (Supplementary Figure 2).
Exosomes of immune cell origin have strictly defined molecular profiles that can be used for immune boosting. Exosomes derived from DCs, NK cells, and type I macrophages (M1) are known to promote cancer cell death directly or via presenting tumor antigens to T cells (7). Given the immune-promotive roles of exosomes derived from these immune cells, they have been proposed with profound utilities in immune-therapeutics (Table 1). Specifically, DCs function in immunotherapy by presenting tumor antigens to naïve T cells, and exosomes secreted from DCs contain CD80 and CD86 that are required for naïve CD4+ T-cell activation (26). In addition, DC-derived exosomes can overcome the limitations of DC cells in suffering from a short lifespan once activated (32, 33) by enabling their long-term storage at -80°C (34). Also, exosomes of DC origin are more efficient in antigen presentation than DCs as they have 10~100-fold more abundant MHCII molecules expressed on the surface (35) and can directly kill cancer cells by expressing peptides capable of activating NK cells (36). Besides, DC-derived exosomes are more stable than DCs in therapeutic preparation and have strictly defined molecular profiles for each patient that can be used to determine the molecular parameters for quality control (35). NK cells recognize tumor antigens primarily via surface-activated receptors and secrete cytotoxic molecules such as perforin and granzyme to lyse malignant cells (37). Similarly, NK cell-derived exosomes can kill cancer cells by providing FasL and perforin/granzyme, with the efficacy demonstrated in various tumors such as breast cancer (38) and neuroblastoma (39). NK-derived exosomes may take on the cytolytic activity after a short time interval and/or at low concentrations and are advantageous in being detectable in peripheral blood, diffusible into tissues, and thus having a cytolytic effect at the tumor sites (37). Thus, efforts have been devoted to construct engineered NK exosomes toward synergistic benefits. For instance, light-activatable silencing NK-derived exosomes were generated by engineering NK cells with hydrophilic small interfering RNA (siRNA) and hydrophobic photosensitizer Ce6, which can boost DC maturation and M1 polarization besides eliciting effective NK-cell cytotoxicity against tumor cells and triggering a photodynamic therapeutic effect (57). Compared with DC and NK cell-derived exosomes, relatively little has been reported on M1-derived exosomes. M1 activates the anticancer immune response by functioning as a type of APCs and producing the type I interferon IL12 and nitric oxide (58). M1-derived exosomes were proposed as promising vaccine adjuvant, since they displayed a tropism toward lymph nodes, induced Th1 cytokine release, and stimulated a strong T-cell cytotoxicity, which collectively fostered a pro-inflammatory microenvironment in the lymph nodes (40). Also, M1-derived exosomes can synergize with their encapsulated agents toward enhanced antitumor effects such as the improved efficacy of cisplatin observed in exosome-treated lung cancer cells (41).
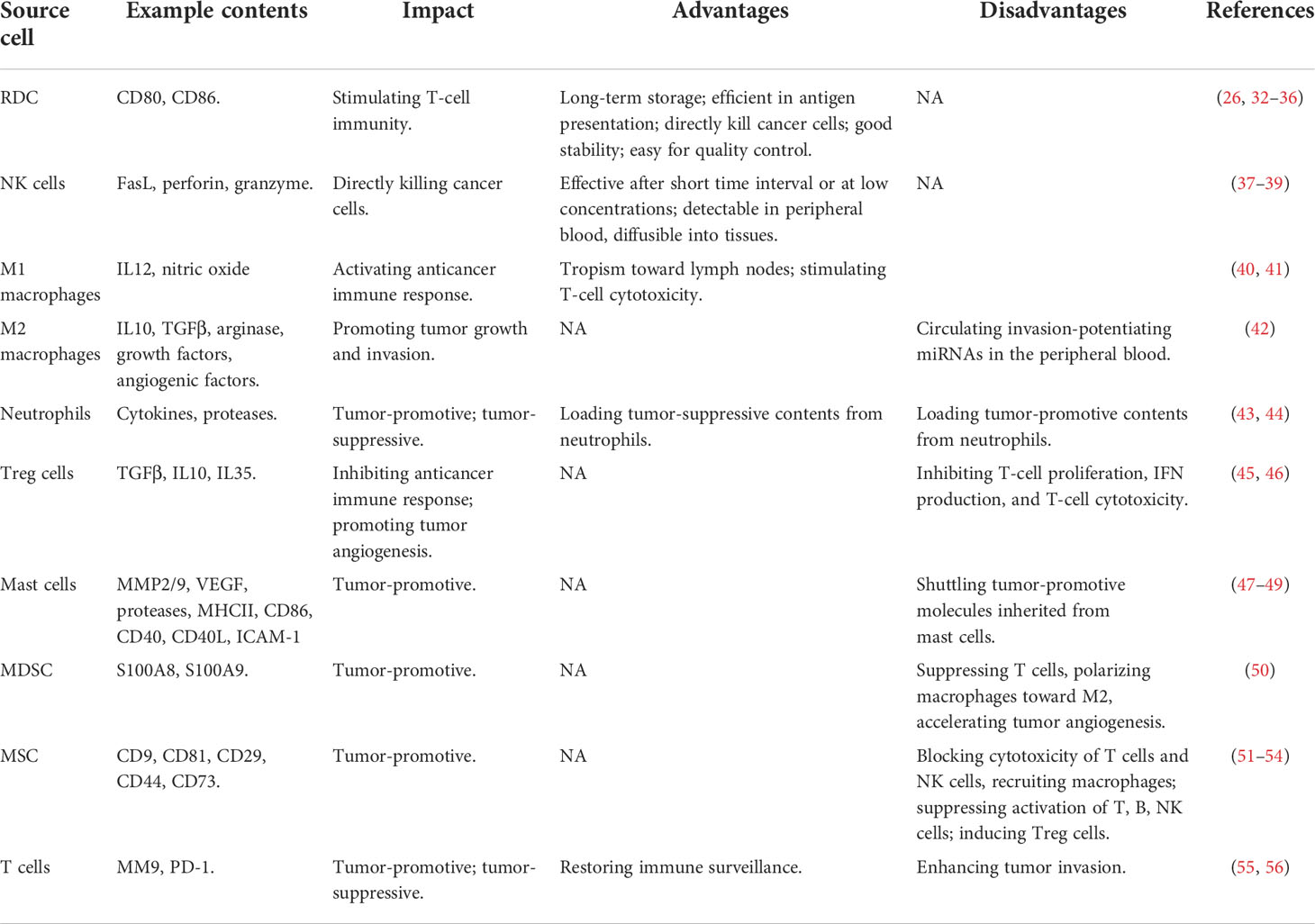
Table 1 Advances and limitations of using exosomes originated from different immune cells for cancer control.
Exosomes derived from immune cells such as type II macrophages (M2), neutrophils, T regulatory (Treg) cells, mast cells, myeloid-derived suppressor cells (MDSCs), and mesenchymal stem cells (MSCs) are tumor promotive (Table 1). Specifically, M2 can form a tumor-promotive immunity and are characterized by suppressed expression of MHCII and IL12 and enhanced expression of IL10, TGFβ, arginase, growth factors, and angiogenic factors (59). M2-derived exosomes can circulate invasion-potentiating miRNAs in the peripheral blood and deliver them to cancer cells for promoted cancer cell growth and invasion, with one example being the delivery of miRNA-223 to breast cancer cells (42). Neutrophils play both tumor-promoting and tumoricidal functions in the innate immune system through the production of, e.g., cytokines and proteases (60). Neutrophil-derived exosomes convey tumor-promotive roles by loading these parental contents (43). It was reported that neutrophil-originated exosomes promoted the proliferation of lung cancer cells via releasing elastases, a neutrophil-derived proteinase that causes complex pathway alterations in malignant cells toward hyperactivated PI3K and AKT signaling (44). Treg cells are tumor-promotive via inhibiting the anticancer immune response and promoting tumor angiogenesis (61). Exosomes of Treg-cell origin can help construct an immune microenvironment favorable for tumor growth. As demonstrated using a melanoma cell model, Treg-derived exosomes inhibited T-cell proliferation, IFN production, and CD8+ T-cell cytotoxicity (45, 46). Mast cells are hematopoietic cells of the immune system with a detrimental impact on allergic reactions and accumulate in the tumor site to form the tumor microenvironment (TME). Factors secreted by mast cells such as matrix-degrading enzymes (MMP2, MMP9), vascular endothelial growth factor (VEGF), proteases, MHCII proteins, co-stimulatory factors (CD86, CD40, CD40L), and adhesion-related molecules (ICAM-1) are tumor-promotive (47, 48) and can be shuttled by mast cell-derived exosomes to promote carcinogenesis. For instance, exosomes of this type promoted the growth of lung adenocarcinoma cells by transferring KIT (a member of the tyrosine kinase family of growth receptors) to cancer cells, where KIT positivity was associated with short-term lung cancer survival (49). MDSCs represent a population of immature myeloid cells capable of suppressing cytotoxic T cells, polarizing macrophages toward the M2 state, and accelerating tumor angiogenesis via producing some soluble immunosuppressive mediators (50). MDSC-derived exosomes transferred these mediating molecules, such as S100A8/S100A9 in breast cancers (50), from sender to receiver cells to trigger M2 polarization. MSCs orchestrate the tumor immune microenvironment together with immune cells and promote tumor growth via blocking cytotoxic responses of T cells and NK cells and recruiting macrophages (62). Similarly, exosomes isolated from human MSCs suppressed the activation and proliferation of T cells, B cells, NK cells, and induced Tregs (51–54).
Exosomes derived from T cells play double-edged roles on cancer (Table 1). It was reported that exosomes of T-cell origin led to an increased invasion of melanoma and lung cancer cells via secreting MM9 (a marker characteristic of tumor migration) (55). However, it was lately demonstrated that T cell-derived exosomes restored immune surveillance against triple-negative breast cancer cells by secreting PD-1 that led to PD-L1 internalization and attenuated suppression on T-cell activity (56).
Besides cancer-associated traits, exosomes may convey other therapeutic efficacies due to their inherited bioactive molecules from donor cells. For instance, exosomes originating from M2 macrophages delivered parental anti-inflammatory cytokines that accelerated wound healing (63); exosomes of MSC origin stimulated angiogenesis-related factors that modulated immunity and promoted tissue regeneration (64).
3.1.2 Exosomes of cancer origin
The roles of exosomes derived from cancer cells in immune response are controversial. For instance, while acute lymphoblastic leukemia-derived exosomes inhibited the cytotoxicity of NK cells by enhancing TGFβ signaling (65), leukemia-derived exosomes activated the anticancer immune response by downregulating TGFβ1 expression (66). Also, exosomes derived from head and neck squamous cell carcinoma patients carried inhibitory factors that are immune suppressive (67). Tumor cell-derived exosomes, once functionalized, can act as an antitumor immunotherapy. For instance, hepatocellular carcinoma cell-derived exosomes painted with the functional domain of HMGN1 boosted the ability of DCs in activating T cells toward long-lasting anticancer immunity both in vitro and in vivo (68).
Exosomes derived from cancer-associated fibroblasts (CAFs) and endothelial cells are mostly tumor-promotive as they typically transfer “signals” to tumor cells and promote tumor progression. For instance, CAF-derived exosomes were obligated to induce metabolic reprogramming toward enhanced tumor growth and metastasis (69). Exosomes derived from brain microvascular endothelial cells were found capable of enhancing the survival of small-cell lung cancer cells metastasized to the brain by delivering and enhancing the expression of S100A16 using an in vitro cell coculture system (70).
3.1.3 Exosomes of food origin
Exosomes derived from food such as milk and edible plants may inherit some features from their parental sources and thus per se be preventive against some undesirable immune responses. For instance, exosomes derived from milk contain immune-related miRNAs that showed excellent therapeutic efficacy against inflammatory bowel diseases in vivo (71); grape-derived exosomes modulated intestinal homeostasis and were protective against inflammatory bowel diseases (72) such as dextran sulfate sodium-induced colitis (73); and ginger-originated exosomes inhibited the activation of the NLRP3 inflammasome, a key innate immune response regulator typically activated in Alzheimer’s diseases and type II diabetes (74).
3.2 Drug delivery
Nano-vehicles for drug delivery can be synthesized or biologically derived at the scale of nanometers that has the physiochemical properties for targeted delivery of agents against cancerous cells. Various nanoparticles have been employed for this purpose including metallic nanoparticles (75), polymeric nanoparticles (76–78), lipid-based carriers such as liposomes and micelles (79), and viral nano-vehicles (80). Metallic nano-carriers suffer from metallic toxicity as a result of particle accumulation in vital organs and difficulty in entire clearance (75). Polymeric nanoparticles are primarily limited by their low yield and biodegradability (78). Lipid-based nano-carriers, although having little concern on toxicity and yield, have biocompatibility issues such as the cause of mucositis (81). Although viral particles can be produced in a large amount at a relatively low cost without biocompatibility issues, they raise safety concerns due to potential spontaneous mutations (82).
Exosomes offer an excellent solution to overcome these aforementioned shortcomings in drug delivery. Compared with artificial or conventional nanoparticles, exosomes are advantageous in easier blood circulation clearance (83), large capacity for ex vivo expansion (11), and biocompatibility due to their endogenous origin (84). Also, exosomes have high cellular uptake due to the existence of membrane proteins such as integrin (85), tetraspanin (CD9, CD63, CD81) (86), and fibronectin (87), are flexible in surface modification (88, 89), and can evade the immune system toward prolonged body circulation time (90). Importantly, exosomes can overcome biological barriers such as the blood–brain barrier (BBB) (88) and lung clearance (91, 92) which make their roles in drug delivery more promising.
3.2.1 Origins of exosomes for drug delivery
Sources of exosomes that can be used as drug carriers include cells (93), body fluids such as blood (94), and food such as milk (95). Exosomes of cell origin can be derived from, e.g., human embryonic kidney (HEK) cells, stem cells, immune cells, and cancer cells (Figure 1).
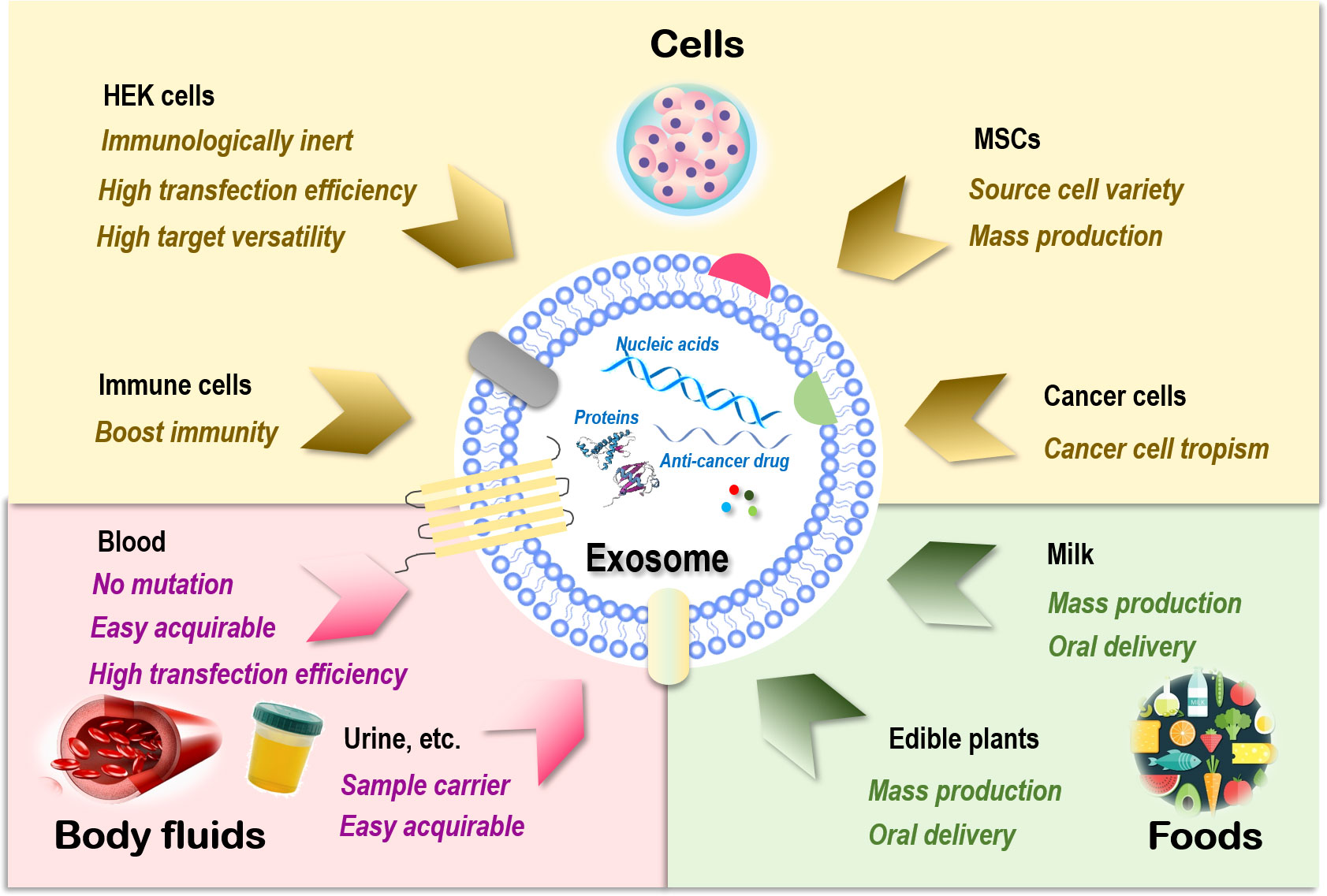
Figure 1 Sources of exosomes and their therapeutic advantages for drug delivery. Exosomes can be generated from almost all types of cells. Those are relevant to therapeutics fall into three main categories, i.e., cells, body fluids, and food. There are four major cell sources for exosome generation, i.e., human embryonic kidney (HEK) cells, mesenchymal stem cells (MSCs), immune cells, and cancer cells. Exosomes of HEK cell origin are immunologically inert without safety concerns, have high transfection efficiency, and can deliver drugs to various target tissues. Exosomes derived from MSCs can be easily obtained from a variety of human tissues and expanded ex vivo in large scale. Exosomes originated from immune cells can enhance the anticancer efficacy of its entrapped drugs by boosting the immunity. Exosomes derived from cancer cells have the tropism toward their parental cells and thus can be used as Trojan horses to target these malignant cells. Exosomes derived from blood have a low risk of unexpected mutations, are easily acquirable, and have higher transfection efficiency. Exosomes isolated from other body fluids such as urine, saliva, and amniotic fluids can be used as parental cell sample carriers for disease diagnosis. Exosomes derived from food such as milk and edible plants are stable under acidic conditions that can be orally delivered and massively produced.
HEK cells, extensively used as an expression tool for recombinant proteins, can also be employed for exosome production, and these exosomes are immunologically inert without safety concerns (96), are highly efficient in transfection, and have membrane resemblances to various human tissues that make it possible to deliver drugs to various target tissues (97, 98). In addition, HEK-derived exosomes can deliver the natural forms of membrane proteins to target cells that are fully functional, resulting in improved tumor penetration and antitumor efficacy. For example, expressing native PH20 hyaluronidase on exosome surface remarkably enhanced the anticancer efficacy of exosomes carrying doxorubicin (Dox) in vivo by degrading hyaluronan in the tumor extracellular matrix (99). Yet, the yield of HEK-derived exosomes was lower than that of exosomes originated from body fluid and food (100).
Exosomes derived from stem cells such as MSCs can be easily obtained and expanded ex vivo (93). MSC represents an ideal source for exosome preparation as cells of this kind can be obtained from a variety of human tissues. Large-scale production of good manufacturing practice (GMP)-grade exosomes for clinical use has been made available from bone marrow-derived MSCs, where the quantities were threefold those of exosomes obtained from human foreskin fibroblasts (101). MSC-derived exosomes delivering siRNAs that target Kras, namely, “MSCs siKras Exo,” effectively resolved tumors and halted tumor metastasis in vivo (102).
Exosomes derived from immune cells can enhance the anticancer efficacy of the entrapped drugs. For instance, the effectiveness of paclitaxel in killing breast cancer cells was substantially enhanced once encapsulated in NK-derived exosomes (103). Another study used macrophage-derived exosomes to load paclitaxel, where an aminoethylanisamide-polyethylene glycol (AA-PEG) vector moiety was incorporated to target the sigma receptor overexpressed in lung cancer cells (104). Similarly, a macrophage-derived exosome-coated poly(lactic-co-glycolic acid) nano-vehicle was established for targeted chemotherapy against triple-negative breast cancers, where the exosome surface was modified with a peptide against c-Met toward enhanced targetability (105).
Exosomes derived from cancer cells have tropism toward their parental cells and thus can be used as Trojan horses to target these malignant cells (105–107). For example, the anti-inflammatory activity of curcumin was improved when encapsulated in breast cancer cell-derived exosomes as a result of the innocent bystander or off-target effect (108). However, in-depth investigations on the metastatic roles of these exosomes are needed before they can be safely used for anticancer drug delivery.
Blood-derived exosomes have a relatively low risk of unexpected in vitro mutations during cell culture due to the large percentage of red blood cells (enucleated) contained in their source materials. In addition, exosomes derived from blood are easily acquirable (i.e., from blood banks or patients) and have higher transfection efficiency. These advantages have made exosomes of this kind ideal delivery vehicles for nucleic acid-based therapeutics (94). Exosomes isolated from other types of body fluids such as blood plasma, urine, saliva, and amniotic fluids can be used as parental cell sample carriers for disease diagnosis (109–112).
Exosomes derived from food are excellent sources for effective delivery of encapsulated therapeutic molecules as, e.g., milk is stable under acidic conditions that can be orally delivered (113, 114) and massively produced (92). Orally administered exosomes loaded with paclitaxel have been shown capable of substantially inhibiting tumor growth in vivo without obvious side effects (115). In addition, milk exosomes can be functionalized toward enhanced stability and biocompatibility. For instance, polyethylene glycol (PEG)-engineered milk exosomes showed around 3.2-fold increased mucus permeability than their unmodified peers (116).
3.2.2 Cargos of exosomes for therapeutics
Cargos imbedded in exosomes as cancer therapies can be in various forms including nucleic acids, proteins, and anticancer agents.
Nucleic acids such as miRNAs, siRNAs, mRNAs, lncRNAs, and circular RNAs can all be delivered by exosomes. Desired RNAs can be loaded into exosomes via overexpressing candidate RNAs in parental cells. For example, exosomes encapsulating miRNA-122 can be obtained by transfecting plasmids expressing miRNA-122 into adipose tissue-derived MSCs (117). Alternatively, nucleic acids can be transferred into exosomes through electroporation such as in the case of loading antisense miRNA-21 into exosomes (118). As endeavors to achieve therapeutic goals, exosome-delivered miRNA-375-3p mimic significantly suppressed the EMT process of colon cancer cells (119), and exosome-mediated delivery of the miRNA-142-3p inhibitor suppressed breast cancer tumorigenicity both in vitro and in vivo (120). Breast cancer cell-derived exosomes were used to deliver S100A4 siRNA that led to significantly reduced postoperative breast cancer metastasis (121), and exosomes loaded with SCD-1 siRNA significantly promoted anaplastic thyroid carcinoma cell apoptosis by enhancing its intracellular ROS level (122). Exosomes loaded with mRNAs encoding SARS-CoV-2 spike and nucleocapsid proteins triggered long-lasting immune response both in vitro and in vivo (123), and exosome-mediated delivery of IL10 mRNA effectively alleviated atherosclerosis (124). Exosome-transmitted lncRNA SENP3-EIF4A1 suppressed the progression of hepatocellular cancer cells (125), and exosome-delivered lncRNA PTENP1 inhibited the development of bladder cancer cells (27). Exosome-carried circular RNA hsa_circ_0051443 suppressed hepatocellular cancer progression (105).
Similar to nucleic acids, proteins can be encapsulated into exosomes via either genetic engineering of donor cells or physical loading such as electroporation and detergent-based approaches. For instance, surviving-T34A was overexpressed in exosomes derived from melanoma cells through plasmid transfection, leading to enhanced gemcitabine sensitivity and significant apoptosis of pancreatic adenocarcinoma cells (126). Tyrosinase-related protein-2 (TRP2) was loaded into exosomes of the serum origin through the use of the detergent saponin or electroporation, which were internalized into macrophages and DCs to effectively stimulate the adaptive immune response (127). Another interesting attempt for exosome protein encapsulation relying on genetic engineering is to load tumor-specific antigens or immune stimulants on exosome surface for vaccination. For instance, by infecting a mouse DC cell line with lentiviruses encoding the α-fetoprotein (AFP) gene, DC-derived exosomes expressing AFP induced a robust immune response in vivo that led to suppressed tumor growth and prolonged animal survival (128). Using a similar strategy, exosomes expressing signal-regulatory protein alpha (SIRPα) on the membrane surface were constructed that can avoid failed immune surveillance via blocking the recognition of CD47 (expressed by tumor cells) by immune cells, leading to enhanced phagocytic ability of macrophages and inhibited growth of cancer cells in vivo (9, 10).
Anticancer drugs can be directly loaded into exosomes as onco-therapeutics. Approaches enabling drug loading primarily include incubation, sonication, and electroporation, with the most commonly reported drugs for exosome encapsulation being Dox and paclitaxel (PTX) (100, 129). Compared with free Dox or Dox loaded by liposomes, Dox delivered by exosomes showed a superior anticancer efficacy against colon cancers in vivo (130); this is attributable to the optimized endocytosis as determined by the cholesterol and the phospholipid composition of the exosome membrane surface (131). MSC exosome-delivered Dox enhanced the cellular uptake and anticancer effect of Dox against osteosarcoma due to the tropism of MSC toward tumor tissues (132). Another advantage of exosome-delivered Dox is its enhanced safety, since exosome can prevent its encapsulated agents from being delivered to myocardial endothelial cells that may lead to cardiotoxicity (133).
4 Exosome innovations for improved onco-therapeutics
4.1 Exosome mimetics
Although red blood cell-derived exosomes are featured with high yield, most studies obtain exosomes from MSC or immortalized cells. Thus, how to effectively enhance exosome yield still imposes one major obstacle limiting the development of exosome-based therapeutics.
One innovation to resolve this issue is the development of nano-sized exosome mimetics (also called “nanovesicles” or “hybrid exosomes”). Exosome mimetics are artificial delivery vehicles mimicking exosomes, which are advantageous in high yield and flexibility in content genetic engineering as compared with exosomes. These features enable them with higher pharmaceutical acceptability due to their more effective and safer delivery manner and well-characterized content (134). However, incorporating multiple proteins on the membranes of exosome mimetics is complex and time-consuming, and the functionalities of the incorporated proteins need to be validated (134).
Technologies enabling the delivery of nucleic acids through exosome mimics have been established. One strategy is to produce size-controllable exosome mimics via serial extrusion of non-tumorigenic MCF10A cells through filters of various pore sizes followed by encapsulating siRNA using electroporation (135). The yield of exosome mimics increased approximately 150-fold as compared with that of exosomes without sacrificing the efficiency and safety (135). The roles of MSC-derived exosome mimetics as alternative vesicles of exosomes for drug delivery have been evaluated, where PTX and breast cancer cells were used as the drug and tumor models, respectively (136). The results showed that MSC-derived exosome mimetics could be easily isolated using simple protocols, and drug-loaded mimetics could effectively kill breast cancer cells both in vitro and in vivo (136). Besides successes reported on MSC-derived exosome mimetics, mimetics of immune cells such as macrophages and NK cells were proven effective for treating various types of cancers (137, 138).
4.2 Exosome surface modification
Surface modification of exosomes can impart additional functionalities to exosomes such as ① sensitizing TME and stimulating immune response, ② improving tumor targetability and retention, and ③ in vivo imaging and trafficking (139).
The purposes of the first two categories are meant for optimized therapeutics and often involve genetic engineering. For instance, by modifying exosomes with folic acids, Feng et al. constructed a novel exosome-based drug delivery system, namely, Exos-PH20-PA, using genetic engineering and self-assembly techniques, and the modified exosomes effectively polarized macrophages from the M2 to M1 phenotype toward an immune-supportive state (140). Activated T cell-derived exosomes were shown to express PD-1 that attenuated PD-L1-triggered immune dysfunction in triple-negative breast cancers, suggestive of the feasibility of attenuating the suppressive TME by modifying the exosome surface with inhibitory immune checkpoint receptors (56). Toward improved tumor targetability, IL12 was displayed on exosome surface via fusion with the exosome surface protein PTGFRN, and the resultant exosome (exoIL12) conveyed tumor-restricted pharmacology that led to prolonged tumor retention and immune memory (141).
The purposes of the last category are designed for the in vitro or in vivo investigation of exosome features such as biodistribution, uptake, and mechanism that typically involve fluorescence labeling. For example, bone marrow MSC-derived exosomes were labeled by DiR dye followed by intraperitoneal administration using pancreatic tumor-bearing C57BL/6 mice to study in vivo exosome biodistribution, which revealed specific exosome retention in tumor cells (142). MDA-MB-231 breast cancer cell-derived exosomes were cultured in the hypoxic environment induced by deferoxamine (DFO) to generate hypoxic exosomes and were subjected to DiO (a fluorescent lipophilic tracer) labeling to study the uptake of hypoxic exosomes; the results showed ~97% vs. ~73.1% uptake of hypoxic vs. normal exosomes by hypoxic cells, suggesting the affinity of hypoxic cells toward hypoxic exosomes (143). Exosomes of bovine milk origin were labeled by PKH67 and incubated with H1299 lung cancer cells together with endocytosis inhibitor cytochalasin D to study the endocytosis pathway, and the results showed that cytochalasin D reduced endocytosis by 21% (144).
4.3 Exosome-based therapeutic synergies
Given that exosomes can function as both immuno-therapeutics and drug carriers, as well as their flexibilities in surface modification that allow for various de novo and improved functionalities, synergies have been sought for through exploring the varied combinatorial possibilities.
Attempt integrating the roles of exosomes in immunotherapies and drug delivery on cancer therapeutics represents an interesting and promising field trend. In other words, exosomes, especially those derived from immune cells, can be considered as both immune-modulators and drug vehicles at the same time, and by modulating exosome content, we can achieve various synergies such as improved immunotherapeutic efficacy and dual targeting. For instance, NK-derived exosomes carrying the tumor-suppressive miRNA-186 exhibited cytotoxicity against MYCN-amplified neuroblastoma cells, where miRNA-186 inhibited MYCN and exempted cancer cells from TGFβ1-dependent immune escape (39).
Lots of successes have been reported taking advantage of exosome surface modification and drug encapsulation. That is, one can achieve synergies by editing the surface of exosomes toward enabled functionalities such as improved tumor targetability and utilizing their drug delivery role. For example, expressing PH20 hyaluronidase on exosomes derived from HEK293PT cells enhanced the anticancer efficacy of Dox by degrading hyaluronan in TME when PH20 and Dox were co-delivered using exosomes in vivo (99).
Conventional dual-targeting strategies have been actualized using exosomes as the effective drug encapsulator and delivery vehicle. This is actually the most straightforward way of creating synergies where multiple drugs can be harmonically mixed and encapsulated into exosomes toward improved therapeutics. For instance, a cocktail therapy was established by combining a natural polymer hyaluronic acid-based hydrogel, engineered endothelial cell-derived exosomes (EC-ExosmiR-26a-5p), and APY29 (an IRE-1α inhibitor), which could simultaneously regulate osteoblast and M1/M2 macrophage balance (145); a combinatorial strategy encapsulating TGFβR1 kinase inhibitor and TLR7/8 agonist in exosomes was shown effective in halting tumor growth in vivo and proposed as a novel therapeutic strategy against melanoma and prostate cancer (146).
5 Aid of CAP in exosome innovations for improved onco-therapeutics
Following successive innovations in creating synergies between exosomes and various drugs and exosome-modulating approaches, relatively little has been focused on the potential aid of emerging technologies in exosome innovations. Here we introduce an emerging anticancer tool, CAP, and its possible synergies with exosomes toward improved onco-therapeutics.
CAP, a fourth state of matter that relies on reactive species toward selective control over malignant cells for death (147–151), has become an emerging onco-therapeutic tool with great translational potential (152, 153). It is a cocktail of multiple reactive oxygen and nitrogen species (RONS) such as short-lived species singlet oxygen (O), hydroxyl radical (OH·), superoxide (O2−), and nitric oxide (NO·), and long-lived species hydrogen peroxide (H2O2), ozone (O3), anionic (OONO−), and protonated (ONOOH) forms of peroxynitrite. These species, by themselves or their interactions, interact with the surface of cancer cells and generate a series of intracellular signalings that selectively arrest cancer cells at various types of death states (such as immunogenic cell death (ICD) (154), apoptosis (150), cell-cycle arrest (147), autophagy (155), ferroptosis (156)) by perturbating their redox homeostasis. Such a selectivity not only attributes to the higher basal level of cells under the malignant state as compared with their healthy peers but also is associated with the membrane features of cancer cells. The latter includes, e.g., a high expression of aquaporins on cell surface that is associated with increased H2O2 uptake (157) and a high local concentration of catalysis on the surface that determines the specific response of tumor cells to self-destruction as a result of secondary singlet oxygen generation (158).
We identify three possible synergies that CAP may create with exosomes. That is, CAP may synergize with exosome toward enhanced immuno-modulation, function as the cargo of exosomes, and boost exosome production.
5.1 CAP for enhanced sensitivity to exosome-triggered immunity
Despite the various reports on the sensitizing role of CAP to chemotherapies (159, 160), little has been examined on the potential synergies between CAP and immunotherapies. Yet, as CAP can trigger ICD (161) that transforms non-immunogenic cells to the immunogenic state via promoting the release of tumor antigens, it is plausible to believe that CAP may enhance the antitumor immunity if applied together with immunotherapies including those based on exosomes (Figure 2). Besides, ROS are known to promote the expression of MHCI for improved macrophage-mediated tumor antigen presentation toward enhanced T-cell adaptive immune response (162) (Figure 2), providing additional support for the theoretical basis of possible synergies between CAP and exosomes that warrants in-depth explorations.
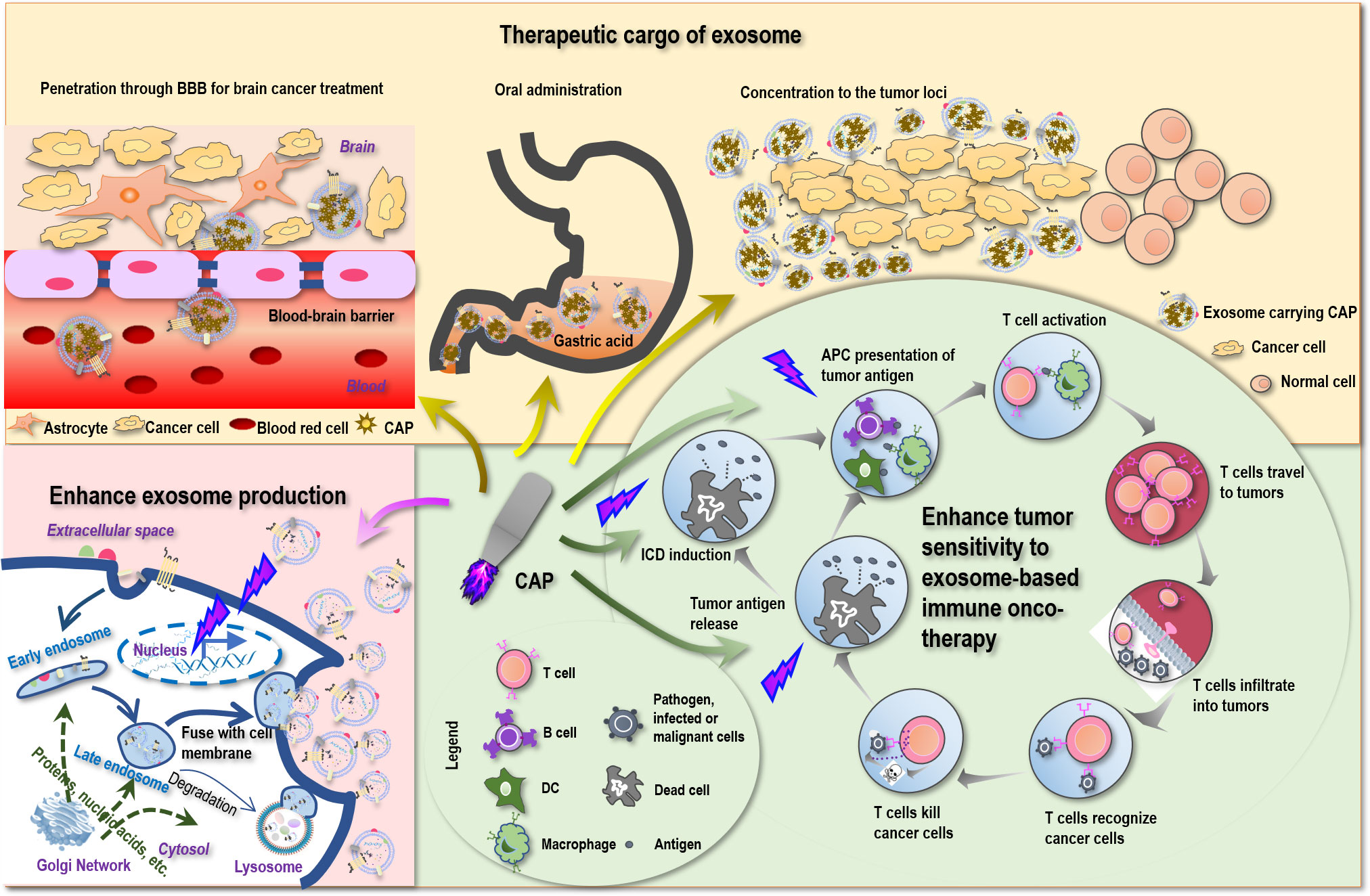
Figure 2 Possible scenarios where CAP creates synergies with exosomes toward conceptual and technological onco-therapeutic innovations. First, CAP can promote tumor antigen release, stimulate immunogenic cell death (ICD), enhance tumor antigen presentation by APC cells, and thus can enhance the sensitivity of tumor cells to exosome-based immune onco-therapies. Second, CAP can function as an onco-therapeutic and the cargo of exosomes to treat brain cancers by breaking the blood–brain barrier (BBB), to enable oral intake as a result of increased tolerance to gastric acidity, and to achieve enhanced drug utility by being concentrated to the tumor loci. Third, CAP can stimulate the expression of p53 that ultimately leads to enhanced exosome production.
5.2 CAP as an emerging therapeutic cargo
Besides the selectivity of CAP against various types of cancer cells demonstrated in vitro and in vivo (147–151, 163, 164), intensive efforts have also been devoted to translating CAP into clinics as an onco-therapeutic modality. The first clinical success was reported in 2018 where the life of a 75-year-old late-stage pancreatic cancer patient was secured (165). The first clinical trial using CAP as a cancer therapy was issued on 30 July 2019 and completed on 14 April 2021 in USA (NCT04267575). In this trial, 20 stage IV solid cancer patients (including breast cancer, prostate cancer, pancreatic cancer, lung cancer, ovarian cancer, fallopian tube cancer, colon cancer, liver cancer, renal cell cancer, rectal cancer, small intestinal cancer, gastric cancer) were recruited, out of whom 17 patients were still alive by the completion of this study (166). These studies suggested CAP as a safe and effective novel modality against malignant cancers. Despite these successful stories, the clinical translation of CAP is hindered by several shortcomings of CAP such as limited penetration in-depth and temporary lifespan of short-lived reactive species.
Being a nano-sized endogenous traveling vehicle with many unique benefits such as BBB transverse ability, biocompatibility, tissue-specific targeting, and cargo stability protection, exosome is ideal for CAP delivery. Since CAP can be prepared in the form of liquid, namely, plasma-activated medium (PAM), it can be made as the cargo of exosomes, alone or mixed with hyaluronic acid (167) for enhanced stability or mixed with hydrogel (168) for extended release. Although CAP is mild and selective against cancer cells without affecting their healthy peers (169), targeted delivery can help concentrate CAP in the tumor loci toward enhanced “drug” utility (Figure 2).
The most promising is that by imbedding PAM within exosomes, it is possible to pass PAM through BBB and treat brain diseases that currently lack an effective treatment approach with little side effect. Indeed, by loading superparamagnetic iron oxide nanoparticles (SPIONs) and curcumin into exosomes and conjugating the membrane of exosomes with neuropilin-1-targeted peptide (RGE), glioma-targeting exosomes were obtained that achieved simultaneous cancer diagnosis and therapeutics (170). MSC-originated exosomes were shown as a promising approach for treating posttraumatic brain injury since, among others, these exosomes were capable of crossing over BBB, feasible for long-term storage, non-tumorigenic, non-immunogenic, and microvascular embolism non-inducive (8). Also promising is the potential to deliver PAM in the form of capsules with the aid of exosomes that can tolerate gastric acidity and enable oral drug intake (Figure 2), which represents an interesting research topic with strong clinical impact and translational potential.
5.3 CAP for inducible exosome secretion
Exosomes can be produced either constitutively or in an inducible fashion on stress (22, 23). It is known that p53, a critical player for genome stability maintenance and DNA damage repair, can be activated on oxidative stress and trigger DNA damage response that ultimately leads to enhanced exosome secretion (23). CAP, a redox modulator, was reported capable of modulating p53 in keratinocytes (171) and activating p53 pathway-related genes in cancer cells (172). These collectively suggested the possible role of CAP in promoting exosome generation (Figure 2), making the massive production and utilization of exosomes of various origins possible and encouraging.
6 Clinical efforts using exosomes as an onco-therapeutic tool
Clinical trials using exosome as an onco-therapeutic approach can be dated back to almost 20 years ago when two phase I clinical trials using immature DC-derived exosomes for treating melanoma and lung cancer were launched (14, 15). In the first trial, exosomes originated from DCs were pulsed with melanoma-associated antigen (MAGE) and inoculated to 15 stage III/IV melanoma patients, where approximately 62% patients exhibited an enhanced NK-cell activity with no major toxicity reported 2 weeks after immunization (14). In the second trial, DC-derived exosomes were loaded with HLA-restricted MAGEs, followed by back infusion into patients carrying HLA A2+ non-small cell lung cancers (NSCLCs), where one-third of the patients showed MAGE-specific T-cell responses and, among the four analyzed patients, half presented an increased NK-cell activity after a 1-month weekly treatment (15). It has been proposed that mature DC-derived exosomes can induce more potent T-cell priming than those derived from immature DCs (173). A non-randomized phase I/II clinical trial achieved antigen-specific T-cell responses among seven esophageal cancer patients using mature DC-derived exosomes pulsed with SART1 (a biomarker of squamous cell esophagus carcinoma) (174). However, the use of mature DC for exosome generation is not the sole golden standard for activating an antigen-specific T-cell response that also relies on many other factors. For instance, a phase II clinical trial documented the use of exosomes derived from mature DCs in treating patients carrying NSCLCs in 2016. In this trial, mature DC exosomes loaded with MHC class I and II-restricted cancer antigens and IFN-γ were administrated to 22 patients. The results showed elevated NK-cell activities without obvious toxicity; yet, unlike what was expected, no cancer-specific T-cell immune response was boosted (175). This may be attributable to the suppressive role of PD-1 on T-cell activity, the expression of which from exosomes was elevated by IFN-γ. Other studies documented the indispensable role of CD4+ T and B cells in activating cytotoxic T cells by DC-derived exosomes (176).
Besides DC-derived exosomes, clinical efforts in cancer treatment have also been devoted to utilize exosomes originating from other cell sources. One phase I clinical trial used ascites-derived exosomes together with granulocyte-macrophage colony-stimulating factor as a combined therapeutic for advanced colorectal cancers. After a 4-week treatment administration, colorectal cancer patients demonstrated a strong cytotoxic T-cell response against cancer cells carrying the carcinoembryonic antigen (a biomarker of colorectal cancers) (177).
Ongoing efforts have been made to translate exosomes into clinical use for drug delivery. For example, Codiak BioSciences, being one of the major biotech start-up companies dedicated for exosome therapeutic development, constructed an engineered exosome for pancreatic cancer treatment by delivering siRNAs targeting the KRAS (G12D) mutation (iExosomes) (142), for which they have obtained the investigational new drug (IND) approval for conducting the phase IA/B clinical trial from US FDA (NCT03608631).
7 Challenges limiting the use of exosomes as therapeutic tools
The many clinically favorable features superimposed on exosomes such as the immune-boosting and molecule-carrying roles have provided us with a unique option to target tumors using cell-free cancer vaccination (7) capable of synergizing with various onco-therapeutic cargoes. This has led to an industrial zest toward its clinical translation that, however, is currently limited by the following therapeutic hurdles (Figure 3).
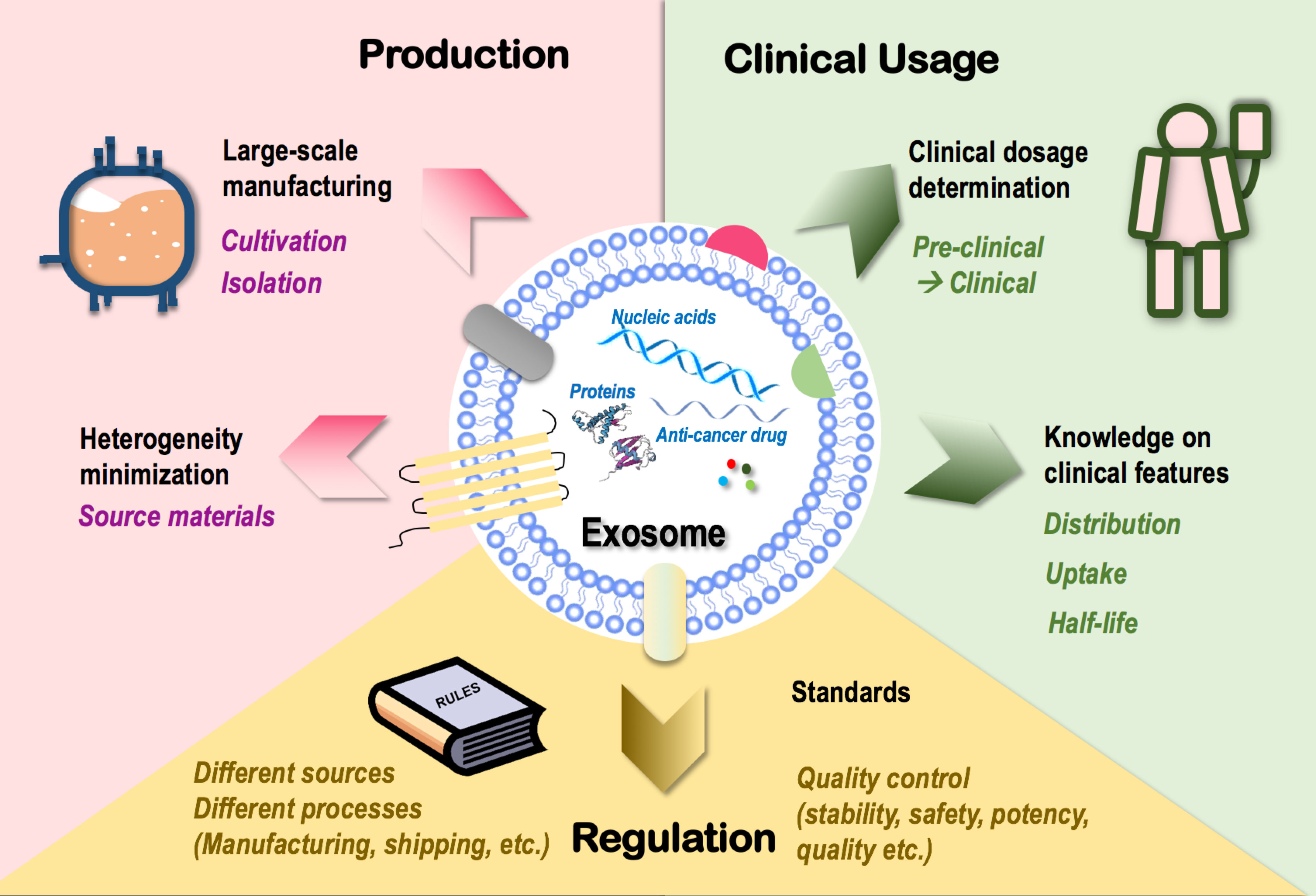
Figure 3 Challenges limiting the clinical translation of exosomes as onco-therapeutics. Challenges limiting the clinical translation of exosomes fall into three categories, i.e., exosome production, clinical usage, and regulation. In “production,” techniques that enable large-scale exosome manufacturing such as source cell cultivation and exosome isolation, and techniques minimizing exosome heterogeneity such as control over the heterogeneity of source materials are limiting factors. In “clinical usage,” knowledge on exosome dosage for clinical use and clinical features of exosomes such as distribution, cell uptake, and half-life are limiting factors. In “regulation,” lack of a set of industrial standards feasible for the manufacturing and shipping processes of exosomes derived from different sources and lack of quality control guidelines over exosome stability, safety, potency, and quality are limiting factors.
Large-scale production is essential for the wide clinical application of novel techniques including exosomes. Cultivation and isolation are critical steps in exosome manufacturing. Conventional cell culture has been traditionally used for cultivating source cells of exosomes. The 3D culturing technique (through the use of bioreactors) has been established to maximize the cell culture surface area toward enhanced exosome yield (178, 179) which, however, is not necessarily cost-effective as more media and more frequent passages are needed for bioreactor-based cell culturing. Ultracentrifugation represents a dominant approach for exosome isolation that suffers from quantity loss due to exosome heterogeneity and from biological contamination (180).
How to minimize the heterogeneity of exosomes represents another important problem challenging large-scale exosome production. Among all possible influential factors, the heterogeneity of parental cells (even of the same cell type) imposes the leading effort that may substantially affect the quality, consistency, and functionality of exosomes produced. For instance, exosomes released from the muscle cells of aged mice are prone to induce inflammation and accelerate aging due to the enrichment of miRNA-34a than those derived from young mice (20, 181). Thus, a careful assessment on potential therapeutic indexes of source materials with critical impacts on the functionalities of derived exosomes such as age should be considered as an important guideline for large-scale exosome manufacturing.
Besides issues relevant to exosome mass production, there also exist unresolved problems on how to translate preclinical experiences into clinics. First and foremost is how to determine exosome dosage under different therapeutic scenarios, where experiences gained using cells or animal models need to be extrapolated into the clinical level. Other clinical features such as exosome distribution, uptake, and half-life should be carefully investigated to avoid off-target tissue accumulation prior to clinical administration (182).
Also worth mentioning is the limitations of exosomes in stimulating the immune system. Similar with canonical agents, exogenous exosomes cannot produce long-term consecutive stimulation to the immune system and may lose the immune-stimulatory efficacy if running exhausted. However, it is not feasible to inject overdosing exosomes to the human body as an overactivated immune system may lead to undesirable clinical outcomes such as cytokine storm that, sometimes, can be life-threatening. Thus, achieving extended exosome release by synergizing with materials such as hydrogel may represent a promising solution and a possible future research direction. Another remaining issue is how to determine the dose and frequency of repeated exosome administration as well as the dosage regime that may differ among patients, without which it may be difficult to achieve an optimal or expected therapeutic response. Thereby, reinforcing our control over the choice of exosome dosage under each treatment scenario represents another major obstacle awaiting to be resolved.
Lastly, lack of industrial standards limits exosome commercialization. Exosomes derived from different sources have different features and thus require different manufacturing protocols and quality control standards. Besides, the stability, safety, potency, and quality of exosomes should all be carefully controlled throughout the manufacturing process and under the shipping conditions. Thus, a set of standards systematically covering all these scenarios is urgently needed for standardized exosome manufacturing that requires collective advances in technologies and government/industrial regulations.
8 Conclusion
Being homogeneous EVs secreted by various types of cells for intercellular communication, exosomes carry information from host cells that can be utilized for diagnosis and, importantly, as immuno-therapeutics due to inherited features from their immune cell origin (for exosomes derived from some immune cells). On the other hand, exosomes are ideal natural nano-carriers for drug delivery due to their small sizes that allow them to penetrate through BBB, flexibilities in surface modulation that allow tissue-specific targeting, endogenous origins that enable their biocompatibilities, natural expression of surface receptors that allow their easy communication with target cells, and bilayer membrane structures that protect their imbedded cargos from, e.g., gastric acidity.
CAP, being an emerging onco-therapeutic approach and redox modulator, can aid in exosome-based onco-therapeutics by sensitizing exosome-based immunotherapies, being the cargo of exosomes for effective malignant cell removal with little side effect, and functioning as a controllable inducer for massive exosome production.
We forecast the emerging and wide application of exosomes in onco-therapeutics, and the prominent role of CAP in availing this process toward, hopefully, eventual malignant tumor eradication. Our insights not only categorize the unique traits of exosomes favorable for disease management (especially for treating brain cancers) but also identify a novel opportunity for cancer therapeutics through a combined use of exosomes and CAP.
Author contributions
XD conceived the study, conducted the literature searching, drafted the manuscript, and prepared the figures. YY contributed in manuscript revision. FH provided the financial support. All authors contributed to the article and approved the submitted version.
Funding
This work was supported by the National Natural Science Foundation of China (Grant No. 81972789), Fundamental Research Funds for the Central Universities (Grant No. JUSRP22011), and Technology Development Funding of Wuxi (Grant No. WX18IVJN017). The funding bodies played no role in the design of the study and collection, analysis, and interpretation of data and in writing the manuscript.
Conflict of interest
Author XD was employed by CAPsoul Medical Biotechnology Company, Ltd.
The remaining authors declare that the research was conducted in the absence of any commercial or financial relationships that could be construed as a potential conflict of interest.
Publisher’s note
All claims expressed in this article are solely those of the authors and do not necessarily represent those of their affiliated organizations, or those of the publisher, the editors and the reviewers. Any product that may be evaluated in this article, or claim that may be made by its manufacturer, is not guaranteed or endorsed by the publisher.
Supplementary material
The Supplementary Material for this article can be found online at: https://www.frontiersin.org/articles/10.3389/fimmu.2022.865245/full#supplementary-material
Supplementary Figure 1 | Biogenesis of exosomes. Exosomes are generated from late endosomes, which are formed by inward budding of the limited multivesicular body (MVB) membrane. Invagination of the late endosomes forms intraluminal vesicles (ILVs) within MVBs, where certain molecules of the parental cells such as proteins and nucleic acids are incorporated into ILVs. While some ILVs are trafficked to lysosomes for degradation, most fuse with cell membrane and are released into the extracellular space, forming ‘exosome’.
Supplementary Figure 2 | Illustrative diagram on human immune system and its key players. Human immune system is composed of ‘innate immunity’ and ‘adaptive immunity’, where the latter is divided into ‘cell-mediated immunity’ and ‘humoral immunity’. Dendritic cells (DCs), macrophages and B cells are professional antigen presentation cells (APCs). During innate immune response, DCs can directly kill pathogens, infected or malignant cells, activate NK cells to aid in the killing process, and present foreign antigens to T cells to stimulate cell-mediated immune response; NK cells, once activated by DCs, can kill pathogens, infected or malignant cells directly, and activate macrophages; macrophages can directly kill pathogens, infected or malignant cells directly, and present foreign antigens to T cells. Innate immune response also involves other participants such as mast cells, basophils, eosinophils, neutrophils and complements. During cell-mediated immune response, T cells, on receival of antigens presented by DCs, macrophages and B cells, are amplified and kill infected or malignant cells by producing perforin (protein that forms pore on targeted cells). During humoral immunity, B cells recognize pathogens, amplify and secrete antibodies to kill them. On recognition of pathogens, infected or malignant cells, innate immune response is activated first (within 12h) followed by adaptive immune response (within days and can last weeks or even longer). Both innate and adaptive immune responses are firstly pro-inflammative (highest at 1-3h in innate immunity and 1-2d in adaptive immunity) and then anti-inflammative (highest at 6-12h in innate immunity and days in adaptive immunity).
References
1. Geminard C, de Gassart A, Vidal M. Reticulocyte maturation: mitoptosis and exosome release. Biocell (2002) 26:205–15. doi: 10.32604/biocell.2002.26.205
2. Johnstone RM, Bianchini A, Teng K. Reticulocyte maturation and exosome release: transferrin receptor containing exosomes shows multiple plasma membrane functions. Blood (1989) 74:1844–51. doi: 10.1182/blood.V74.5.1844.1844
3. Mashouri L, Yousefi H, Aref AR, Ahadi AM, Molaei F, Alahari SK. Exosomes: composition, biogenesis, and mechanisms in cancer metastasis and drug resistance. Mol Cancer (2019) 18:75. doi: 10.1186/s12943-019-0991-5
4. Voichitoiu A-D, Radu BM, Pavelescu L, Cretoiu D, Deftu AT, Suciu N, et al. Extracellular vesicles in cancer, extracellular vesicles and their importance in human health. IntechOpen (2019) 85117:66821. doi: 10.5772/intechopen.85117
5. Jabbari N, Nawaz M, Rezaie J. Bystander effects of ionizing radiation: conditioned media from X-ray irradiated MCF-7 cells increases the angiogenic ability of endothelial cells. Cell Commun Signal (2019) 17:165. doi: 10.1186/s12964-019-0474-8
6. Dai W, White R, Liu J, Liu H. Organelles coordinate milk production and secretion during lactation: Insights into mammary pathologies. Prog Lipid Res (2022) 86:101159. doi: 10.1016/j.plipres.2022.101159
7. Nikfarjam S, Rezaie J, Kashanchi F, Jafari R. Dexosomes as a cell-free vaccine for cancer immunotherapy. J Exp Clin Cancer Res (2020) 39:258. doi: 10.1186/s13046-020-01781-x
8. Ghosh S, Garg S, Ghosh S. Cell-derived exosome therapy: A novel approach to treat post-traumatic brain injury mediated neural injury. ACS Chem Neurosci (2020) 11:2045–7. doi: 10.1021/acschemneuro.0c00368
9. Koh E, Lee EJ, Nam GH, Hong Y, Cho E, Yang Y, et al. Exosome-SIRPalpha, a CD47 blockade increases cancer cell phagocytosis. Biomaterials (2017) 121:121–9. doi: 10.1016/j.biomaterials.2017.01.004
10. Cho E, Nam GH, Hong Y, Kim YK, Kim DH, Yang Y, et al. Comparison of exosomes and ferritin protein nanocages for the delivery of membrane protein therapeutics. J Control Release (2018) 279:326–35. doi: 10.1016/j.jconrel.2018.04.037
11. Yeo RW, Lai RC, Zhang B, Tan SS, Yin Y, Teh BJ, et al. Mesenchymal stem cell: an efficient mass producer of exosomes for drug delivery. Adv Drug Delivery Rev (2013) 65:336–41. doi: 10.1016/j.addr.2012.07.001
12. Chen Y, Sun Y, Luo Z, Lin J, Qi B, Kang X, et al. Potential mechanism underlying exercise upregulated circulating blood exosome miR-215-5p to prevent necroptosis of neuronal cells and a model for early diagnosis of alzheimer's disease. Front Aging Neurosci (2022) 14:860364. doi: 10.3389/fnagi.2022.860364
13. Zhang Y, Li X, Wang D, Jiang X, Zhang M, Lv K. Serum exosome microRNA panel as a noninvasive biomarker for molecular diagnosis of fulminant myocarditis. Mol Ther Methods Clin Dev (2021) 20:142–51. doi: 10.1016/j.omtm.2020.11.006
14. Escudier B, Dorval T, Chaput N, Andre F, Caby MP, Novault S, et al. Vaccination of metastatic melanoma patients with autologous dendritic cell (DC) derived-exosomes: results of thefirst phase I clinical trial. J Transl Med (2005) 3:10. doi: 10.1186/1479-5876-3-10
15. Morse MA, Garst J, Osada T, Khan S, Hobeika A, Clay TM, et al. A phase I study of dexosome immunotherapy in patients with advanced non-small cell lung cancer. J Transl Med (2005) 3:9. doi: 10.1186/1479-5876-3-9
16. Zhang Y, Bi J, Huang J, Tang Y, Du S, Li P. Exosome: A review of its classification, isolation techniques, storage, diagnostic and targeted therapy applications. Int J Nanomedicine (2020) 15:6917–34. doi: 10.2147/IJN.S264498
17. Margolis E, Brown G, Partin A, Carter B, McKiernan J, Tutrone R, et al. Predicting high-grade prostate cancer at initial biopsy: clinical performance of the ExoDx (EPI) prostate intelliscore test in three independent prospective studies. Prostate Cancer Prostatic Dis (2022) 25:296–301. doi: 10.1038/s41391-021-00456-8
18. FDA Approves first liquid biopsy next-generation sequencing companion diagnostic test. In: FDA Newsroom.
19. Babaei M, Rezaie J. Application of stem cell-derived exosomes in ischemic diseases: opportunity and limitations. J Transl Med (2021) 19:196. doi: 10.1186/s12967-021-02863-w
20. Ahmadi M, Rezaie J. Ageing and mesenchymal stem cells derived exosomes: Molecular insight and challenges. Cell Biochem Funct (2021) 39:60–6. doi: 10.1002/cbf.3602
21. Kwon SH, Oh S, Nacke M, Mostov KE, Lipschutz JH. Adaptor protein CD2AP and l-type lectin LMAN2 regulate exosome cargo protein trafficking through the golgi complex. J Biol Chem (2017) 292:16523. doi: 10.1074/jbc.A116.729202
22. Mayo JN, Bearden SE. Driving the hypoxia-inducible pathway in human pericytes promotes vascular density in an exosome-dependent manner. Microcirculation (2015) 22:711–23. doi: 10.1111/micc.12227
23. Lespagnol A, Duflaut D, Beekman C, Blanc L, Fiucci G, Marine JC, et al. Exosome secretion, including the DNA damage-induced p53-dependent secretory pathway, is severely compromised in TSAP6/Steap3-null mice. Cell Death Differ (2008) 15:1723–33. doi: 10.1038/cdd.2008.104
24. Keerthikumar S, Chisanga D, Ariyaratne D, Al Saffar H, Anand S, Zhao K, et al. ExoCarta: A web-based compendium of exosomal cargo. J Mol Biol (2016) 428:688–92. doi: 10.1016/j.jmb.2015.09.019
25. Beach A, Zhang HG, Ratajczak MZ, Kakar SS. Exosomes: an overview of biogenesis, composition and role in ovarian cancer. J Ovarian Res (2014) 7:14. doi: 10.1186/1757-2215-7-14
26. Schorey JS, Bhatnagar S. Exosome function: from tumor immunology to pathogen biology. Traffic (2008) 9:871–81. doi: 10.1111/j.1600-0854.2008.00734.x
27. Zheng R, Du M, Wang X, Xu W, Liang J, Wang W, et al. Exosome-transmitted long non-coding RNA PTENP1 suppresses bladder cancer progression. Mol Cancer (2018) 17:143. doi: 10.1186/s12943-018-0880-3
28. Skotland T, Sandvig K, Llorente A. Lipids in exosomes: Current knowledge and the way forward. Prog Lipid Res (2017) 66:30–41. doi: 10.1016/j.plipres.2017.03.001
29. Hassanpour M, Rezabakhsh A, Rezaie J, Nouri M, Rahbarghazi R. Exosomal cargos modulate autophagy in recipient cells via different signaling pathways. Cell Biosci (2020) 10:92. doi: 10.1186/s13578-020-00455-7
30. Rezaie J, Aslan C, Ahmadi M, Zolbanin NM, Kashanchi F, Jafari R. The versatile role of exosomes in human retroviral infections: from immunopathogenesis to clinical application. Cell Biosci (2021) 11:19. doi: 10.1186/s13578-021-00537-0
31. de Souza AP, Bonorino C. The immune system: endogenous anticancer mechanism. Front Biosci (Elite Ed) (2012) 4:2354–64. doi: 10.2741/e547
32. Yang J, Huck SP, McHugh RS, Hermans IF, Ronchese F. Perforin-dependent elimination of dendritic cells regulates the expansion of antigen-specific CD8+ T cells in vivo. Proc Natl Acad Sci USA (2006) 103:147–52. doi: 10.1073/pnas.0509054103
33. Hermans IF, Ritchie DS, Yang J, Roberts JM, Ronchese F. CD8+ T cell-dependent elimination of dendritic cells in vivo limits the induction of antitumor immunity. J Immunol (2000) 164:3095–101. doi: 10.4049/jimmunol.164.6.3095
34. Andre F, Escudier B, Angevin E, Tursz T, Zitvogel L. Exosomes for cancer immunotherapy. Ann Oncol (2004) 15 Suppl 4:iv141–4. doi: 10.1093/annonc/mdh918
35. Pitt JM, Andre F, Amigorena S, Soria JC, Eggermont A, Kroemer G, et al. Dendritic cell-derived exosomes for cancer therapy. J Clin Invest (2016) 126:1224–32. doi: 10.1172/JCI81137
36. Munich S, Sobo-Vujanovic A, Buchser WJ, Beer-Stolz D, Vujanovic NL. Dendritic cell exosomes direclty kill tumor cells and activate natural killer cells via TNF superfamily ligands. Oncoimmunology (2012) 1:1074–83. doi: 10.4161/onci.20897
37. Di Pace AL, Tumino N, Besi F, Alicata C, Conti LA, Munari E, et al. Characterization of human NK cell-derived exosomes: Role of DNAM1 receptor in exosome-mediated cytotoxicity against tumor. Cancers (Basel) (2020) 12:661. doi: 10.3390/cancers13102397
38. Kaban K, Hinterleitner C, Zhou Y, Salva E, Kantarci AG, Salih HR, et al. Therapeutic silencing of BCL-2 using NK cell-derived exosomes as a novel therapeutic approach in breast cancer. Cancers (Basel) (2021) 13:2397. doi: 10.3390/cancers13102397
39. Neviani P, Wise PM, Murtadha M, Liu CW, Wu CH, Jong AY, et al. Natural killer-derived exosomal miR-186 inhibits neuroblastoma growth and immune escape mechanisms. Cancer Res (2019) 79:1151–64. doi: 10.1158/0008-5472.CAN-18-0779
40. Cheng L, Wang Y, Huang L. Exosomes from M1-polarized macrophages potentiate the cancer vaccine by creating a pro-inflammatory microenvironment in the lymph node. Mol Ther (2017) 25:1665–75. doi: 10.1016/j.ymthe.2017.02.007
41. Li J, Li N, Wang J. M1 macrophage-derived exosome-encapsulated cisplatin can enhance its anti-lung cancer effect. Minerva Med (2020) 20:06564. doi: 10.23736/S0026-4806.20.06564-7
42. Yang M, Chen J, Su F, Yu B, Su F, Lin L, et al. Microvesicles secreted by macrophages shuttle invasion-potentiating microRNAs into breast cancer cells. Mol Cancer (2011) 10:117. doi: 10.1186/1476-4598-10-117
43. Zhang J, Ji C, Zhang H, Shi H, Mao F, Qian H, et al. Engineered neutrophil-derived exosome-like vesicles for targeted cancer therapy. Sci Adv (2022) 8:eabj8207. doi: 10.1126/sciadv.abj8207
44. Houghton AM. The paradox of tumor-associated neutrophils: fueling tumor growth with cytotoxic substances. Cell Cycle (2010) 9:1732–7. doi: 10.4161/cc.9.9.11297
45. Chatila TA, Williams CB. Regulatory T cells: exosomes deliver tolerance. Immunity (2014) 41:3–5. doi: 10.1016/j.immuni.2014.07.001
46. Xie Y, Zhang X, Zhao T, Li W, Xiang J. Natural CD8(+)25(+) regulatory T cell-secreted exosomes capable of suppressing cytotoxic T lymphocyte-mediated immunity against B16 melanoma. Biochem Biophys Res Commun (2013) 438:152–5. doi: 10.1016/j.bbrc.2013.07.044
47. Frossi B, Mion F, Tripodo C, Colombo MP, Pucillo CE. Rheostatic functions of mast cells in the control of innate and adaptive immune responses. Trends Immunol (2017) 38:648–56. doi: 10.1016/j.it.2017.04.001
48. Benito-Martin A, Di Giannatale A, Ceder S, Peinado H. The new deal: a potential role for secreted vesicles in innate immunity and tumor progression. Front Immunol (2015) 6:66. doi: 10.3389/fimmu.2015.00066
49. Xiao H, Lasser C, Shelke GV, Wang J, Radinger M, Lunavat TR, et al. Mast cell exosomes promote lung adenocarcinoma cell proliferation - role of KIT-stem cell factor signaling. Cell Commun Signal (2014) 12:64. doi: 10.1186/s12964-014-0064-8
50. Burke M, Choksawangkarn W, Edwards N, Ostrand-Rosenberg S, Fenselau C. Exosomes from myeloid-derived suppressor cells carry biologically active proteins. J Proteome Res (2014) 13:836–43. doi: 10.1021/pr400879c
51. Conforti A, Scarsella M, Starc N, Giorda E, Biagini S, Proia A, et al. Microvescicles derived from mesenchymal stromal cells are not as effective as their cellular counterpart in the ability to modulate immune responses in vitro. Stem Cells Dev (2014) 23:2591–9. doi: 10.1089/scd.2014.0091
52. Di Trapani M, Bassi G, Midolo M, Gatti A, Kamga PT, Cassaro A, et al. Differential and transferable modulatory effects of mesenchymal stromal cell-derived extracellular vesicles on T, b and NK cell functions. Sci Rep (2016) 6:24120. doi: 10.1038/srep24120
53. Del Fattore A, Luciano R, Pascucci L, Goffredo BM, Giorda E, Scapaticci M, et al. Immunoregulatory effects of mesenchymal stem cell-derived extracellular vesicles on T lymphocytes. Cell Transplant (2015) 24:2615–27. doi: 10.3727/096368915X687543
54. Blazquez R, Sanchez-Margallo FM, de la Rosa O, Dalemans W, Alvarez V, Tarazona R, et al. Immunomodulatory potential of human adipose mesenchymal stem cells derived exosomes on in vitro stimulated T cells. Front Immunol (2014) 5:556. doi: 10.3389/fimmu.2014.00556
55. Cai Z, Yang F, Yu L, Yu Z, Jiang L, Wang Q, et al. Activated T cell exosomes promote tumor invasion via fas signaling pathway. J Immunol (2012) 188:5954–61. doi: 10.4049/jimmunol.1103466
56. Qiu Y, Yang Y, Yang R, Liu C, Hsu JM, Jiang Z, et al. Activated T cell-derived exosomal PD-1 attenuates PD-L1-induced immune dysfunction in triple-negative breast cancer. Oncogene (2021) 40:4992–5001. doi: 10.1038/s41388-021-01896-1
57. Zhang M, Shao W, Yang T, Liu H, Guo S, Zhao D, et al. Conscription of immune cells by light-activatable silencing NK-derived exosome (LASNEO) for synergetic tumor eradication. Adv Sci (Weinh) (2022) 9(22):e2201135. doi: 10.1002/advs.202201135
58. Barone A, d'Avanzo N, Cristiano MC, Paolino D, Fresta M. Macrophage-derived extracellular vesicles: A promising tool for personalized cancer therapy. Biomedicines (2022) 10:1252. doi: 10.3390/biomedicines10061252
59. Grivennikov SI, Greten FR, Karin M. Immunity, inflammation, and cancer. Cell (2010) 140:883–99. doi: 10.1016/j.cell.2010.01.025
60. Buonacera A, Stancanelli B, Colaci M, Malatino L. Neutrophil to lymphocyte ratio: An emerging marker of the relationships between the immune system and diseases. Int J Mol Sci (2022) 23:3636. doi: 10.3390/ijms23073636
61. Facciabene A, Motz GT, Coukos G. T-Regulatory cells: key players in tumor immune escape and angiogenesis. Cancer Res (2012) 72:2162–71. doi: 10.1158/0008-5472.CAN-11-3687
62. Huang Y, Yu P, Li W, Ren G, Roberts AI, Cao W, et al. p53 regulates mesenchymal stem cell-mediated tumor suppression in a tumor microenvironment through immune modulation. Oncogene (2014) 33:3830–8. doi: 10.1038/onc.2013.355
63. Kim H, Wang SY, Kwak G, Yang Y, Kwon IC, Kim SH. Exosome-guided phenotypic switch of M1 to M2 macrophages for cutaneous wound healing. Adv Sci (Weinh) (2019) 6:1900513. doi: 10.1002/advs.201900513
64. Zhu W, Huang L, Li Y, Zhang X, Gu J, Yan Y, et al. Exosomes derived from human bone marrow mesenchymal stem cells promote tumor growth in vivo. Cancer Lett (2012) 315:28–37. doi: 10.1016/j.canlet.2011.10.002
65. Yu H, Huang T, Wang D, Chen L, Lan X, Liu X, et al. Acute lymphoblastic leukemia-derived exosome inhibits cytotoxicity of natural killer cells by TGF-beta signaling pathway. 3 Biotech (2021) 11:313. doi: 10.1007/s13205-021-02817-5
66. Huang F, Wan J, Hu W, Hao S. Enhancement of anti-leukemia immunity by leukemia-derived exosomes Via downregulation of TGF-beta1 expression. Cell Physiol Biochem (2017) 44:240–54. doi: 10.1159/000484677
67. Beccard IJ, Hofmann L, Schroeder JC, Ludwig S, Laban S, Brunner C, et al. Immune suppressive effects of plasma-derived exosome populations in head and neck cancer. Cancers (Basel) (2020) 12:1997. doi: 10.3390/cancers12071997
68. Zuo B, Qi H, Lu Z, Chen L, Sun B, Yang R, et al. Alarmin-painted exosomes elicit persistent antitumor immunity in large established tumors in mice. Nat Commun (2020) 11:1790. doi: 10.1038/s41467-020-15569-2
69. Ilkhani K, Bastami M, Delgir S, Safi A, Talebian S, Alivand MR. The engaged role of tumor microenvironment in cancer metabolism: Focusing on cancer-associated fibroblast and exosome mediators. Anticancer Agents Med Chem (2021) 21:254–66. doi: 10.2174/18715206MTA53ODY5y
70. Xu ZH, Miao ZW, Jiang QZ, Gan DX, Wei XG, Xue XZ, et al. Brain microvascular endothelial cell exosome-mediated S100A16 up-regulation confers small-cell lung cancer cell survival in brain. FASEB J Off Publ Fed Am Societies Exp Biol (2019) 33:1742–57. doi: 10.1096/fj.201800428R
71. Reif S, Elbaum-Shiff Y, Koroukhov N, Shilo I, Musseri M, Golan-Gerstl R. Cow and human milk-derived exosomes ameliorate colitis in DSS murine model. Nutrients (2020) 12:2589. doi: 10.3390/nu12092589
72. Rahimi Ghiasi M, Rahimi E, Amirkhani Z, Salehi R. Leucine-rich repeat-containing G-protein coupled receptor 5 gene overexpression of the rat small intestinal progenitor cells in response to orally administered grape exosome-like nanovesicles. Adv BioMed Res (2018) 7:125. doi: 10.4103/abr.abr_114_18
73. Ju S, Mu J, Dokland T, Zhuang X, Wang Q, Jiang H, et al. Grape exosome-like nanoparticles induce intestinal stem cells and protect mice from DSS-induced colitis. Mol Ther (2013) 21:1345–57. doi: 10.1038/mt.2013.64
74. Chen X, Zhou Y, Yu J. Exosome-like nanoparticles from ginger rhizomes inhibited NLRP3 inflammasome activation. Mol Pharm (2019) 16:2690–9. doi: 10.1021/acs.molpharmaceut.9b00246
75. Gaharwar US, Meena R, Rajamani P. Biodistribution, clearance and morphological alterations of intravenously administered iron oxide nanoparticles in Male wistar rats. Int J Nanomedicine (2019) 14:9677–92. doi: 10.2147/IJN.S223142
76. Martinez-Jothar L, Beztsinna N, van Nostrum CF, Hennink WE, Oliveira S. Selective cytotoxicity to HER2 positive breast cancer cells by saporin-loaded nanobody-targeted polymeric nanoparticles in combination with photochemical internalization. Mol Pharm (2019) 16:1633–47. doi: 10.1021/acs.molpharmaceut.8b01318
77. Ahir M, Upadhyay P, Ghosh A, Sarker S, Bhattacharya S, Gupta P, et al. Delivery of dual miRNA through CD44-targeted mesoporous silica nanoparticles for enhanced and effective triple-negative breast cancer therapy. Biomater Sci (2020) 8:2939–54. doi: 10.1039/D0BM00015A
78. Bhavsar D, Gajjar J, Sawant K. Formulation and development of smart pH responsive mesoporous silica nanoparticles for breast cancer targeted delivery of anastrozole: In vitro and in vivo characterizations. Microporous Mesoporous Materials (2019) 279:107–16. doi: 10.1016/j.micromeso.2018.12.026
79. Zhao Y, Alakhova DY, Zhao X, Band V, Batrakova EV, Kabanov AV. Eradication of cancer stem cells in triple negative breast cancer using doxorubicin/pluronic polymeric micelles. Nanomedicine (2020) 24:102124. doi: 10.1016/j.nano.2019.102124
80. Esfandiari N, Arzanani MK, Soleimani M, Kohi-Habibi M, Svendsen WE. A new application of plant virus nanoparticles as drug delivery in breast cancer. Tumour Biol (2016) 37:1229–36. doi: 10.1007/s13277-015-3867-3
81. Ansari L, Shiehzadeh F, Taherzadeh Z, Nikoofal-Sahlabadi S, Momtazi-Borojeni AA, Sahebkar A, et al. The most prevalent side effects of pegylated liposomal doxorubicin monotherapy in women with metastatic breast cancer: a systematic review of clinical trials. Cancer Gene Ther (2017) 24:189–93. doi: 10.1038/cgt.2017.9
82. Zdanowicz M, Chroboczek J. Virus-like particles as drug delivery vectors. Acta Biochim Pol (2016) 63:469–73. doi: 10.18388/abp.2016_1275
83. Yamashita T, Takahashi Y, Nishikawa M, Takakura Y. Effect of exosome isolation methods on physicochemical properties of exosomes and clearance of exosomes from the blood circulation. Eur J Pharm Biopharm (2016) 98:1–8. doi: 10.1016/j.ejpb.2015.10.017
84. Soltani F, Parhiz H, Mokhtarzadeh A, Ramezani M. Synthetic and biological vesicular nano-carriers designed for gene delivery. Curr Pharm Des (2015) 21:6214–35. doi: 10.2174/1381612821666151027153410
85. Shen AR, Zhong X, Tang TT, Wang C, Jing J, Liu BC, et al. Integrin, exosome and kidney disease. Front Physiol (2020) 11:627800. doi: 10.3389/fphys.2020.627800
86. Ninomiya M, Inoue J, Krueger EW, Chen J, Cao H, Masamune A, et al. The exosome-associated tetraspanin CD63 contributes to the efficient assembly and infectivity of the hepatitis b virus. Hepatol Commun (2021) 5:1238–51. doi: 10.1002/hep4.1709
87. Purushothaman A, Bandari SK, Liu J, Mobley JA, Brown EE, Sanderson RD. Fibronectin on the surface of myeloma cell-derived exosomes mediates exosome-cell interactions. J Biol Chem (2016) 291:1652–63. doi: 10.1074/jbc.M115.686295
88. Khongkow M, Yata T, Boonrungsiman S, Ruktanonchai UR, Graham D, Namdee K. Surface modification of gold nanoparticles with neuron-targeted exosome for enhanced blood-brain barrier penetration. Sci Rep (2019) 9:8278. doi: 10.1038/s41598-019-44569-6
89. Hosseini A, Soleimani S, Pezeshgi Modarres H, Hojjati Emami S, Tondar M, Bahlakeh G, et al. Exosome-inspired targeting of cancer cells with enhanced affinity. J Mater Chem B (2016) 4:768–78. doi: 10.1039/C5TB01741F
90. Liu C, Zhang W, Li Y, Chang J, Tian F, Zhao F, et al. Microfluidic sonication to assemble exosome membrane-coated nanoparticles for immune evasion-mediated targeting. Nano Lett (2019) 19:7836–44. doi: 10.1021/acs.nanolett.9b02841
91. Wang J, Zheng Y, Zhao M. Exosome-based cancer therapy: Implication for targeting cancer stem cells. Front Pharmacol (2016) 7:533. doi: 10.3389/fphar.2016.00533
92. Kawikova I, Askenase PW. Diagnostic and therapeutic potentials of exosomes in CNS diseases. Brain Res (2015) 1617:63–71. doi: 10.1016/j.brainres.2014.09.070
93. Baek G, Choi H, Kim Y, Lee HC, Choi C. Mesenchymal stem cell-derived extracellular vesicles as therapeutics and as a drug delivery platform. Stem Cells Transl Med (2019) 8:880–6. doi: 10.1002/sctm.18-0226
94. Usman WM, Pham TC, Kwok YY, Vu LT, Ma V, Peng B, et al. Efficient RNA drug delivery using red blood cell extracellular vesicles. Nat Commun (2018) 9:2359. doi: 10.1038/s41467-018-04791-8
95. Del Pozo-Acebo L, Hazas MLL, Tome-Carneiro J, Gil-Cabrerizo P, San-Cristobal R, Busto R, et al. Bovine milk-derived exosomes as a drug delivery vehicle for miRNA-based therapy. Int J Mol Sci (2021) 22:1105. doi: 10.3390/ijms22031105
96. Zhu X, Badawi M, Pomeroy S, Sutaria DS, Xie Z, Baek A, et al. Comprehensive toxicity and immunogenicity studies reveal minmal effects in mice following sustained dosing of extracellualr vesicles derived from HEK293PT cells. J Extracell Vesicles (2017) 6:1324730. doi: 10.1080/20013078.2017.1324730
97. Jurgielewicz BJ, Yao Y, Stice SL. Kinetics and specificity of HEK293T extracellular vesicle uptake using imaging flow cytometry. Nanoscale Res Lett (2020) 15:170. doi: 10.1186/s11671-020-03399-6
98. Vilanova-Perez T, Jones C, Balint S, Dragovic R, Dustin LM, Yeste M, et al. Exosomes derived from HEK293T cells interact in an efficient and noninvasive manner with mammalian sperm in vitro. Nanomedicine (Lond) (2020) 15:1965–80. doi: 10.2217/nnm-2020-0056
99. Hong Y, Nam GH, Koh E, Jeon S, Kim GB, Jeong C, et al. Exosome as a vehicle for delivery fo membrane protein therapeutics, PH20, for enhanced tumor penetration and antitumor efficacy. Adv Funct Mater (2018) 28:1703074. doi: 10.1002/adfm.201703074
100. Kim H, Jang H, Cho H, Choi J, Hwang KY, Choi Y, et al. Recent advances in exosome-based drug delivery for cancer therapy. Cancers (Basel) (2021) 13:4435. doi: 10.3390/cancers13174435
101. Chen YS, Lin EY, Chiou TW, Harn HJ. Exosomes in clinical trial and their production in compliance with good manufacturing practice. Ci Ji Yi Xue Za Zhi (2020) 32:113–20. doi: 10.4103/tcmj.tcmj_182_19
102. Mendt M, Kamerkar S, Sugimoto H, McAndrews KM, Wu C-C, Gagea M, et al. Generation and testing of clincial-grade exosomes for pancreatic cancer. JCI Insight (2018) 3:e99263. doi: 10.1172/jci.insight.99263
103. Li S, Wu Y, Ding F, Yang J, Li J, Gao X, et al. Engineering macrophage-derived exosomes for targeted chemotherapy of triple-negative breast cancer. Nanoscale (2020) 12:10854–62. doi: 10.1039/D0NR00523A
104. Kim MS, Haney MJ, Zhao Y, Yuan D, Deygen I, Klyachko NL, et al. Engineering macrophage-derived exosomes for targeted paclitaxel delivery to pulmonary metastases: in vitro and in vivo evaluations. Nanomedicine (2018) 14:195–204. doi: 10.1016/j.nano.2017.09.011
105. Qiao L, Hu S, Huang K, Su T, Li Z, Vandergriff A, et al. Tumor cell-derived exosomes home to their cells of origin and can be used as Trojan horses to deliver cancer drugs. Theranostics (2020) 10:3474–87. doi: 10.7150/thno.39434
106. Shao J, Zaro J, Shen Y. Advances in exosome-based drug delivery and tumor targeting: From tissue distribution to intracellular fate. Int J Nanomedicine (2020) 15:9355–71. doi: 10.2147/IJN.S281890
107. Ghaffari-Makhmalbaf P, Sayyad M, Pakravan K, Razmara E, Bitaraf A, Bakhshinejad B, et al. Docosahexaenoic acid reverses the promoting effects of breast tumor cell-derived exosomes on endothelial cell migration and angiogenesis. Life Sci (2021) 264:118719. doi: 10.1016/j.lfs.2020.118719
108. Sun D, Zhuang X, Xiang X, Liu Y, Zhang S, Liu C, et al. A novel nanoparticle drug delivery system: the anti-inflammatory activity of curcumin is enhanced when encapsulated in exosomes. Mol Ther (2010) 18:1606–14. doi: 10.1038/mt.2010.105
109. Sheller-Miller S, Menon R. Isolation and characterization of human amniotic fluid-derived exosomes. Methods Enzymol (2020) 645:181–94. doi: 10.1016/bs.mie.2020.07.006
110. Cheshmi B, Cheshomi H. Salivary exosomes: properties, medical applications, and isolation methods. Mol Biol Rep (2020) 47:6295–307. doi: 10.1007/s11033-020-05659-1
111. Rani K, Rastogi S, Vishwakarma P, Bharti PS, Sharma V, Renu K, et al. A novel approach to correlate the salivary exosomes and their protein cargo in the progression of cognitive impairment into alzheimer's disease. J Neurosci Methods (2021) 347:108980. doi: 10.1016/j.jneumeth.2020.108980
112. Wu M, Chen Z, Xie Q, Xiao B, Zhou G, Chen G, et al. One-step quantification of salivary exosomes based on combined aptamer recognition and quantum dot signal amplification. Biosens Bioelectron (2021) 171:112733. doi: 10.1016/j.bios.2020.112733
113. Liao Y, Du X, Li J, Lonnderdal B. Human milk exosomes and their microRNAs survive digestion in vitro and are taken up by human intestinal cells. Mol Nutr Food Res (2017) 61:1700082. doi: 10.1002/mnfr.201700082
114. Shandilya S, Rani P, Onteru SK, Singh D. Small interfering RNA in milk exosomes is resistant to digestion and crosses the intestinal barier in vitro. J Agric Food Chem (2017) 65:9506–13. doi: 10.1021/acs.jafc.7b03123
115. Agrawal AK, Aqil F, Jeyabalan J, Spencer WA, Beck J, Gachuki BW, et al. Milk-derived exosomes for oral delivery of paclitaxel. Nanomedicine (2017) 13:1627–36. doi: 10.1016/j.nano.2017.03.001
116. Warren MR, Zhang C, Vedadghavami A, Bokvist K, Dhal PK, Bajpayee AG. Milk exosomes with enhanced mucus penetrability for oral delivery of siRNA. Biomater Sci (2021) 9:4260–77. doi: 10.1039/D0BM01497D
117. Lou G, Song X, Yang F, Wu S, Wang J, Chen Z, et al. Exosomes derived from miR-122-modified adipose tissue-derived MSCs increase chemosensitivity of hepatocellular carcinoma. J Hematol Oncol (2015) 8:122. doi: 10.1186/s13045-015-0220-7
118. Kim G, Kim M, Lee Y, Byun JW, Hwang DW, Lee M. Systemic delivery of microRNA-21 antisense oligonucleotides to the brain using T7-peptide decorated exosomes. J Control Release (2020) 317:273–81. doi: 10.1016/j.jconrel.2019.11.009
119. Rezaei R, Baghaei K, Amani D, Piccin A, Hashemi SM, Asadzadeh Aghdaei H, et al. Exosome-mediated delivery of functionally active miRNA-375-3p mimic regulate epithelial mesenchymal transition (EMT) of colon cancer cells. Life Sci (2021) 269:119035. doi: 10.1016/j.lfs.2021.119035
120. Naseri Z, Oskuee RK, Jaafari MR, Forouzandeh Moghadam M. Exosome-mediated delivery of functionally active miRNA-142-3p inhibitor reduces tumorigenicity of breast cancer in vitro and in vivo. Int J Nanomedicine (2018) 13:7727–47. doi: 10.2147/IJN.S182384
121. Zhao L, Gu C, Gan Y, Shao L, Chen H, Zhu H. Exosome-mediated siRNA delivery to suppress postoperative breast cancer metastasis. J Control Release (2020) 318:1–15. doi: 10.1016/j.jconrel.2019.12.005
122. Chen M, Li S, Liang Y, Zhang Y, Luo D, Wang W. Integrative multi-omics analysis of identified NUF2 as a candidate oncogene correlates with poor prognosis and immune infiltration in non-small cell lung cancer. Front Oncol (2021) 11:656509. doi: 10.3389/fonc.2021.656509
123. Tsai SJ, Atai NA, Cacciottolo M, Nice J, Salehi A, Guo C, et al. Exosome-mediated mRNA delivery in vivo is safe and can be used to induce SARS-CoV-2 immunity. J Biol Chem (2021) 297:101266. doi: 10.1016/j.jbc.2021.101266
124. Bu T, Li Z, Hou Y, Sun W, Zhang R, Zhao L, et al. Exosome-mediated delivery of inflammation-responsive il-10 mRNA for controlled atherosclerosis treatment. Theranostics (2021) 11:9988–10000. doi: 10.7150/thno.64229
125. Wang J, Pu J, Zhang Y, Yao T, Luo Z, Li W, et al. Exosome-transmitted long non-coding RNA SENP3-EIF4A1 suppresses the progression of hepatocellular carcinoma. Aging (Albany NY) (2020) 12:11550–67. doi: 10.18632/aging.103302
126. Aspe JR, Diaz Osterman CJ, Jutzy JM, Deshields S, Whang S, Wall NR. Enhancement of gemcitabine sensitivity in pancreatic adenocarcinoma by novel exosome-mediated delivery of the survivin-T34A mutant. J Extracell Vesicles (2014) 3:3. doi: 10.3402/jev.v3.23244
127. Park O, Choi ES, Yu G, Kim JY, Kang YY, Jung H, et al. Efficient delivery of tyrosinase related protein-2 (TRP2) peptides to lymph nodes using serum-derived exosomes. Macromol Biosci (2018) 18:e1800301. doi: 10.1002/mabi.201800301
128. Lu Z, Zuo B, Jing R, Gao X, Rao Q, Liu Z, et al. Dendritic cell-derived exosomes elicit tumor regression in autochthonous hepatocellular carcinoma mouse models. J Hepatol (2017) 67:739–48. doi: 10.1016/j.jhep.2017.05.019
129. Zhang M, Zang X, Wang M, Li Z, Qiao M, Hu H, et al. Exosome-based nanocarriers as bio-inspired and versatile vehicles for drug delivery: recent advances and challenges. J Mater Chem B (2019) 7:2421–33. doi: 10.1039/C9TB00170K
130. Jang SC, Kim OY, Yoon CM, Choi DS, Roh TY, Park J, et al. Bioinspired exosome-mimetic nanovesicles for targeted delivery of chemotherapeutics to malignant tumors. ACS Nano (2013) 7:7698–710. doi: 10.1021/nn402232g
131. Smyth TJ, Redzic JS, Graner MW, Anchordoquy TJ. Examination of the specificity of tumor cell derived exosomes with tumor cells in vitro. Biochim Biophys Acta (2014) 1838:2954–65. doi: 10.1016/j.bbamem.2014.07.026
132. Wei H, Chen J, Wang S, Fu F, Zhu X, Wu C, et al. A nanodrug consisting of doxorubicin and exosome derived from mesenchymal stem cells for osteosarcoma treatment in vitro. Int J Nanomedicine (2019) 14:8603–10. doi: 10.2147/IJN.S218988
133. Hadla M, Palazzolo S, Corona G, Caligiuri I, Canzonieri V, Toffoli G, et al. Exosomes increase the therapeutic index of doxorubicin in breast and ovarian cancer mouse models. Nanomedicine (Lond) (2016) 11:2431–41. doi: 10.2217/nnm-2016-0154
134. Kooijmans SA, Vader P, van Dommelen SM, van Solinge WW, Schiffelers RM. Exosome mimetics: a novel class of drug delivery systems. Int J Nanomedicine (2012) 7:1525–41. doi: 10.2147/IJN.S29661
135. Yang Z, Xie J, Zhu J, Kang C, Chiang C, Wang X, et al. Functional exosome-mimic for delivery of siRNA to cancer: in vitro and in vivo evaluation. J Control Release (2016) 243:160–71. doi: 10.1016/j.jconrel.2016.10.008
136. Kalimuthu S, Gangadaran P, Rajendran RL, Zhu L, Oh JM, Lee HW, et al. A new approach for loading anticancer drugs into mesenchymal stem cell-derived exosome mimetics for cancer therapy. Front Pharmacol (2018) 9:1116. doi: 10.3389/fphar.2018.01116
137. Pisano S, Pierini I, Gu J, Gazze A, Francis LW, Gonzalez D, et al. Immune (Cell) derived exosome mimetics (IDEM) as a treatment for ovarian cancer. Front Cell Dev Biol (2020) 8:553576. doi: 10.3389/fcell.2020.553576
138. Zhu L, Gangadaran P, Kalimuthu S, Oh JM, Baek SH, Jeong SY, et al. Novel alternatives to extracellular vesicle-based immunotherapy - exosome mimetics derived from natural killer cells. Artif Cells Nanomed Biotechnol (2018) 46:S166–79. doi: 10.1080/21691401.2018.1489824
139. Salunkhe S, Dheeraj, Basak M, Chitkara D, Mittal A. Surface functionalization of exosomes for target-specific delivery and in vivo imaging & tracking: Strategies and significance. J Control Release (2020) 326:599–614. doi: 10.1016/j.jconrel.2020.07.042
140. Feng C, Xiong Z, Wang C, Xiao W, Xiao H, Xie K, et al. Folic acid-modified exosome-PH20 enhances the efficiency of therapy via modulation of the tumor microenvironment and directly inhibits tumor cell metastasis. Bioact Mater (2021) 6:963–74. doi: 10.1016/j.bioactmat.2020.09.014
141. Lewis ND, Sia CL, Kirwin K, Haupt S, Mahimkar G, Zi T, et al. Exosome surface display of IL12 results in tumor-retained pharmacology with superior potency and limited systemic exposure compared with recombinant IL12. Mol Cancer Ther (2021) 20:523–34. doi: 10.1158/1535-7163.MCT-20-0484
142. Mendt M, Kamerkar S, Sugimoto H, McAndrews KM, Wu CC, Gagea M, et al. Generation and testing of clinical-grade exosomes for pancreatic cancer. JCI Insight (2018) 3:e99263. doi: 10.1172/jci.insight.99263
143. Jung KO, Jo H, Yu JH, Gambhir SS, Pratx G. Development and MPI tracking of novel hypoxia-targeted theranostic exosomes. Biomaterials (2018) 177:139–48. doi: 10.1016/j.biomaterials.2018.05.048
144. Aqil F, Munagala R, Jeyabalan J, Agrawal AK, Gupta R. Exosomes for the enhanced tissue bioavailability and efficacy of curcumin. AAPS J (2017) 19:1691–702. doi: 10.1208/s12248-017-0154-9
145. Mi B, Chen L, Xiong Y, Yang Y, Panayi AC, Xue H, et al. Osteoblast/Osteoclast and immune cocktail therapy of an Exosome/Drug delivery multifunctional hydrogel accelerates fracture repair. ACS Nano (2022) 16:771–82. doi: 10.1021/acsnano.1c08284
146. Lee JH, Song J, Kim IG, You G, Kim H, Ahn JH, et al. Exosome-mediated delivery of transforming growth factor-beta receptor 1 kinase inhibitors and toll-like receptor 7/8 agonists for combination therapy of tumors. Acta Biomater (2022) 141:354–63. doi: 10.1016/j.actbio.2022.01.005
147. Hua D, Cai D, Ning M, Yu L, Zhang Z, Han P, et al. Cold atmospheric plasma selectively induces G0/G1 cell cycle arrest and apoptosis in AR-independent prostate cancer cells. J Cancer (2021) 12:5977–86. doi: 10.7150/jca.54528
148. Dai X, Bazaka K, Thompson EW, Ostrikov KK. Cold atmospheric plasma: A promising controller of cancer cell states. Cancers (Basel) (2020) 12:3360. doi: 10.3390/cancers12113360
149. Zhou X, Cai D, Xiao S, Ning M, Zhou R, Zhang S, et al. InvivoPen: A novel plasma source for in vivo cancer treatment. J Cancer (2020) 11:2273–82. doi: 10.7150/jca.38613
150. Xiang L, Xu X, Zhang S, Cai D, Dai X. Cold atmospheric plasma conveys selectivity on triple negative breast cancer cells both in vitro and in vivo. Free Radic Biol Med (2018) 124:205–13. doi: 10.1016/j.freeradbiomed.2018.06.001
151. Wang P, Zhou R, Thomas P, Zhao L, Zhou R, Mandal S, et al. Epithelial-to-Mesenchymal transition enhances cancer cell sensitivity to cytotoxic effects of cold atmospheric plasmas in breast and bladder cancer systems. Cancers (Basel) (2021) 13:2889. doi: 10.3390/cancers13122889
152. Dai X, Bazaka K, Richard DJ, Thompson ERW, Ostrikov KK. The emerging role of gas plasma in oncotherapy. Trends Biotechnol (2018) 36:1183–98. doi: 10.1016/j.tibtech.2018.06.010
153. Dai X, Li H, Ning M. Plasma robot engineering: The next generation of precision disease management. Ann BioMed Eng (2021) 49:1593–7. doi: 10.1007/s10439-021-02799-8
154. Miller V, Lin A, Fridman A. Why target immune cells for plasma treatment of cancer. Plasma Chem Plasma Process (2015) 36:259–68. doi: 10.1007/s11090-015-9676-z
155. Miao Y, Han P, Hua D, Zhou R, Guan Z, Lv Q, et al. Cold atmospheric plasma increases IBRV titer in MDBK cells by orchestrating the host cell network. Virulence (2021) 12:679–89. doi: 10.1080/21505594.2021.1883933
156. Furuta T, Shi L, Toyokuni S. Non-thermal plasma as a simple ferroptosis inducer in cancer cells: A possible role of ferritin. Pathol Int (2018) 68:442–3. doi: 10.1111/pin.12665
157. Yan D, Talbot A, Nourmohammadi N, Sherman JH, Cheng X, Keidar M. Toward understanding the selective anticancer capacity of cold atmospheric plasma–a model based on aquaporins (Review). Biointerphases (2015) 10:040801. doi: 10.1116/1.4938020
158. Bauer G, Sersenova D, Graves DB, Machala Z. Dynamics of singlet oxygen-triggered, RONS-based apoptosis induction after treatment of tumor cells with cold atmospheric plasma or plasma-activated medium. Sci Rep (2019) 9:1393. doi: 10.1038/s41598-019-50329-3
159. Köritzer J, Boxhammer V, Schafer A, Shimizu T, Klampfl TG, Li YF, et al. Restoration of sensitivity in chemo-resistant glioma cells by cold atmospheric plasma. PloS One (2013) 8:e64498. doi: 10.1371/journal.pone.0064498
160. Xu D, Luo X, Xu Y, Cui Q, Yang Y, Liu D, et al. The effects of cold atmospheric plasma on cell adhesion, differentiation, migration, apoptosis and drug sensitivity of multiple myeloma. Biochem Biophys Res Commun (2016) 473:1125–32. doi: 10.1016/j.bbrc.2016.04.027
161. Lin A, Gorbanev Y, De Backer J, Van Loenhout J, Van Boxem W, Lemiere F, et al. Non-thermal plasma as a unique delivery system of short-lived reactive oxygen and nitrogen species for immunogenic cell death in melanoma cells. Adv Sci (Weinh) (2019) 6:1802062. doi: 10.1002/advs.201802062
162. Wang C, Li P, Liu L, Pan H, Li H, Cai L, et al. Self-adjuvanted nanovaccine for cancer immunotherapy: Role of lysosomal rupture-induced ROS in MHC class I antigen presentation. Biomaterials (2016) 79:88–100. doi: 10.1016/j.biomaterials.2015.11.040
163. Schneider C, Gebhardt L, Arndt S, Karrer S, Zimmermann JL, Fischer MJM, et al. Acidification is an essential process of cold atmospheric plasma and promotes the anti-cancer effect on malignant melanoma cells. Cancers (Basel) (2019) 11:1802062. doi: 10.3390/cancers11050671
164. Bekeschus S, Freund E, Spadola C, Privat-Maldonado A, Hackbarth C, Bogaerts A, et al. Risk assessment of kINPen plasma treatment of four human pancreatic cancer cell lines with respect to metastasis. Cancers (Basel) (2019) 11:1237. doi: 10.3390/cancers11091237
165. Canady Helios cold plasma scalpel (2016). Available at: https://www.fox32chicago.com/news/new-procedure-gives-hope-to-those-battling-pancreatic-cancer.
166. FDA. Summary FDA IDE #G190165 clinical trials.gov identifier: NCT04267575 canady Helios cold plasma scalpel treatment at the surgical margin & macroscopic tumor site. (2021).
167. Liu Z, Zheng Y, Dang J, Zhang J, Dong F, Wang K, et al. A novel antifungal plasma-activated hydrogel. ACS Appl Mater Interfaces (2019) 11:22941–9. doi: 10.1021/acsami.9b04700
168. Xu Y, Niu Y, Wu B, Cao X, Gong T, Zhang ZR, et al. Extended-release of therapeutic microRNA via a host-guest supramolecular hydrogel to locally alleviate renal interstitial fibrosis. Biomaterials (2021) 275:120902. doi: 10.1016/j.biomaterials.2021.120902
169. Dai X, Cai D, Wang P, Nan N, Yu L, Zhang Z, et al. Cold atmospheric plasmas target breast cancer stemness via modulating AQP3-19Y mediated AQP3-5K and FOXO1 K48-ubiquitination. Int J Biol Sci (2022) 18:3544–61. doi: 10.7150/ijbs.72296
170. Jia G, Han Y, An Y, Ding Y, He C, Wang X, et al. NRP-1 targeted and cargo-loaded exosomes facilitate simultaneous imaging and therapy of glioma in vitro and in vivo. Biomaterials (2018) 178:302–16. doi: 10.1016/j.biomaterials.2018.06.029
171. Schmidt A, Bekeschus S, Jarick K, Hasse S, von Woedtke T, Wende K. Cold physical plasma modulates p53 and mitogen-activated protein kinase signaling in keratinocytes. Oxid Med Cell Longev (2019) 2019:7017363. doi: 10.1155/2019/7017363
172. Shi L, Yu L, Zou F, Hu H, Liu K, Lin Z. Gene expression profiling and functional analysis reveals that p53 pathway-related gene expression is highly activated in cancer cells treated by cold atmospheric plasma-activated medium. PeerJ (2017) 5:e3751. doi: 10.7717/peerj.3751
173. Fu C, Zhou L, Mi QS, Jiang A. DC-Based vaccines for cancer immunotherapy. Vaccines (2020) 8:706. doi: 10.3390/vaccines8040706
174. Narita M, Kanda T, Abe T, Uchiyama T, Iwafuchi M, Zheng Z, et al. Immune responses in patients with esophageal cancer treated with SART1 peptide-pulsed dendritic cell vaccine. Int J Oncol (2015) 46:1699–709. doi: 10.3892/ijo.2015.2846
175. Besse B, Charrier M, Lapierre V, Dansin E, Lantz O, Planchard D, et al. Dendritic cell-derived exosomes as maintenance immunotherapy after first line chemotherapy in NSCLC. Oncoimmunology (2016) 5:e1071008. doi: 10.1080/2162402X.2015.1071008
176. Naslund TI, Gehrmann U, Qazi KR, Karlsson MC, Gabrielsson S. Dendritic cell-derived exosomes need to activate both T and b cells to induce antitumor immunity. J Immunol (2013) 190:2712–9. doi: 10.4049/jimmunol.1203082
177. Dai S, Wei D, Wu Z, Zhou X, Wei X, Huang H, et al. Phase I clinical trial of autologous ascites-derived exosomes combined with GM-CSF for colorectal cancer. Mol Ther J Am Soc Gene Ther (2008) 16:782–90. doi: 10.1038/mt.2008.1
178. Phan J, Kumar P, Hao D, Gao K, Farmer D, Wang A. Engineering mesenchymal stem cells to improve their exosome efficacy and yield for cell-free therapy. J Extracell Vesicles (2018) 7:1522236. doi: 10.1080/20013078.2018.1522236
179. Watson DC, Bayik D, Srivatsan A, Bergamaschi C, Valentin A, Niu G, et al. Efficient production and enhanced tumor delivery of engineered extracellular vesicles. Biomaterials (2016) 105:195–205. doi: 10.1016/j.biomaterials.2016.07.003
180. Li P, Kaslan M, Lee SH, Yao J, Gao Z. Progress in exosome isolation techniques. Theranostics (2017) 7:789–804. doi: 10.7150/thno.18133
181. Fulzele S, Mendhe B, Khayrullin A, Johnson M, Kaiser H, Liu Y, et al. Muscle-derived miR-34a increases with age in circulating extracellular vesicles and induces senescence of bone marrow stem cells. Aging (Albany NY) (2019) 11:1791–803. doi: 10.18632/aging.101874
Keywords: exosome, cold atmospheric plasma, immunotherapy, cancer, therapeutics
Citation: Dai X, Ye Y and He F (2022) Emerging innovations on exosome-based onco-therapeutics. Front. Immunol. 13:865245. doi: 10.3389/fimmu.2022.865245
Received: 29 January 2022; Accepted: 29 July 2022;
Published: 31 August 2022.
Edited by:
Bertrand Kaeffer, Institut National de recherche pour l’agriculture, l’alimentation et l’environnement (INRAE), FranceReviewed by:
Jafar Rezaie, Urmia University of Medical Sciences, IranAmada Torres, Instituto de Biotecnología - UNAM, Mexico
Copyright © 2022 Dai, Ye and He. This is an open-access article distributed under the terms of the Creative Commons Attribution License (CC BY). The use, distribution or reproduction in other forums is permitted, provided the original author(s) and the copyright owner(s) are credited and that the original publication in this journal is cited, in accordance with accepted academic practice. No use, distribution or reproduction is permitted which does not comply with these terms.
*Correspondence: Fule He, hefule@163.com; Yongju Ye, ls8558@163.com