- 1Laboratoire Inflammation Tissus Epithéliaux et Cytokines LITEC UR 15560, Université de Poitiers, Poitiers, France
- 2ImmunoConcEpT, Centre National de la Recherche Scientifique, Unité Mixte de Recherche 5164, University of Bordeaux, Bordeaux, France
Atopic dermatitis (AD) is a chronic inflammatory skin disease characterized by barrier dysfunction, dysregulated immune response, and dysbiosis with increased Staphylococcus aureus colonization. Infiltration of various T helper cell subsets into lesional skin and subsequent cytokine release are a hallmark of AD. Release of cytokines by both T cells and keratinocytes plays a key role in skin inflammation and drives many AD features. This review aims to discuss cytokine-mediated crosstalk between T cells and keratinocytes in AD pathogenesis and the potential impact of virulence factors produced by Staphylococcus aureus on these interactions.
Introduction
Atopic dermatitis (AD) is one of the most common chronic inflammatory skin diseases, affecting approximately 15% to 25% of children and 3% to 10% of adults worldwide (1, 2). Lesions generally appear during childhood and/or during the third decade of life. AD is characterized by eczematous skin lesions, xerosis, erythematous scaling plaques and intense pruritus during flare-ups (1). The pathogenesis of AD is complex and involves multiple combinatorial factors, including genetic risk factors associated with immune dysregulation, mainly related to type 2 inflammation, and environmental stimuli, including allergens, stress, and microbial dysbiosis with dominant Staphylococcus aureus colonization (3, 4). AD is often associated with elevated serum concentrations of immunoglobulin E and a personal or family history of type I hypersensitivity, with an increased risk of developing food allergy, allergic rhinitis or asthma, called the atopic march, together with other comorbidities such as obesity, cardiovascular diseases, and cancers (5, 6). One of the classical features of AD is a strong type 2 immune response that leads to skin barrier dysfunction, such as inhibition of epidermal differentiation and increased skin permeability (3). Inflammatory cell infiltration found in lesional AD skin is characterized by the presence of type 2 innate lymphoid cells (ILC2) and various T cell subpopulations producing cytokines driving a T helper (Th) type 2-predominant inflammation (7, 8). Keratinocytes, the predominant cell type of the epidermis, are also key players in the AD pro-inflammatory environment (9). These cells constitute a major source of cytokines, including interleukin (IL)-25, IL-33, and Thymic stromal lymphopoietin (TSLP), chemokines, and antimicrobial peptides that orchestrate infiltration of T cells as well as innate immune cells (such as ILCs and mast cells) in lesional skin (9). Moreover, S. aureus-associated dysbiosis contributes to immune dysregulation and barrier dysfunction. This review aims to focus on the role of cytokines in the interplay between skin T cells and keratinocytes in AD physiopathology and to discuss the potential impact of virulence factors produced by S. aureus on this crosstalk.
T Cells in AD
Skin infiltration of various CD4+ Th and CD8+ cytotoxic T cells (Tc) subsets is strongly implicated in AD pathogenesis (8, 10–12). Atopic skin is characterized by a predominant type 2 immune response, with Th2/Tc2 cells being involved in many features of AD. These cells are the main source responsible for increased levels of the type 2 cytokines IL-4, IL-5, IL-13, and IL-31 (12, 13), and infiltration of ILC2 in lesional AD skin also contributes to elevation of IL-13 and IL-5 levels (14). In addition, numerous studies have revealed the heterogeneity of T cell sub-populations infiltrating AD skin lesions, with the presence of Th2/Tc2, Th22/Tc22, Th17, and Th1 cells (10, 12, 15–18). Compared to healthy skin, non-lesional AD skin displays increased T cell infiltration and expression of Th2, Th22, and Th1-related cytokines and shares features with lesional AD skin, suggesting a global dysregulation of the T cell immune response in the skin of AD patients (12, 19–21). Similarly, blood of AD subjects harbors heterogeneous T cell subsets with an increased frequency of Th2/Tc2 and Th22/Tc22 cells compared to healthy donors (22–24).
This excessive effector response could be the result of a tolerance breach with a dysbalance of effector versus regulatory T cells (Tregs) and/or Tregs dysfunction in AD patients. Tregs are critical in the development and maintenance of self-tolerance mediated by immunosuppressive cytokine (25). Many studies have highlighted a dysregulated proportion of Tregs in lesional skin and peripheral blood of AD patients, while upward or downward variations have been reported depending on patient cohorts (22, 25–31). In addition, the deficiency of Tregs function can contribute to the development of AD. Indeed, Tregs dysfunction may be related to their propensity to differentiate into effector-like T cells under the influence of cytokines overexpressed in AD patients, leading to the expansion of pathogenic Tregs such as circulating Th2-like Tregs (31–33).
Importantly, persistence of identical T-cell clones in lesional and non-lesional AD skin suggests the involvement of resident memory T (TRM) cells, which are involved not only in disease progression and flares, but also during relapse of the disease (34), as previously shown in other chronic inflammatory dermatoses, such as psoriasis and vitiligo (35–37). Indeed, mouse models of allergic contact dermatitis have highlighted the presence of CD4+ and CD8+ TRM cells in lesions, expressing inhibitory checkpoint receptors to control their reactivation in order to avoid the development of severe pathology (38, 39). So far, few studies have focused on the phenotype and function of TRM cells in human AD skin and revealed their ability to produce multiple cytokines, including IL-4, IL-17A, IL-22 and IFN-γ (10, 40, 41). Interestingly, a recent study identified an enriched CXCR4+ TRM natural killer T cell population in AD skin that may contribute to AD (42). Although these studies highlight the possible role of TRM cells in AD, a more precise characterization of TRM cell phenotype in AD lesions appears relevant to better decipher their function in disease development.
Recent development of single-cell RNA technology brought new insight of the characterization of T cells in AD skin (10, 18, 43, 44). The lesional AD samples were characterized by expansion of TRM, Th2/Tc2, and Th22 cells. Lesional T cells showed strong expression of type 2 (IL-13), type 17 (IL-26) and partly also of Th22 (IL-22) cytokines in activated T cells, proliferating T cells, and NK T cells (10, 18). Elevated expression of IL-26 was found to be associated with low levels of IL-17A in adult AD lesions, suggesting that Th17 cells are functionally deviant during disease progression. Pathway enrichment analysis revealed enrichment of the immune response, antigen processing and presentation, and regulation of apoptosis in lesional AD T cells. In healed AD lesion of adults, it was suggested that a Th1-skewed immune profile is involved in the clinical remission (44). Interestingly, identification of specific populations of disease-linked immune cells maintaining an inflammatory phenotype in resolved AD, including Th2 and Tc2 cells, suggest that these cells can be crucial for disease recurrence (43).
Cytokines: Key Players in the Crosstalk Between T Cells and Keratinocytes in AD Skin
Through the release of cytokines in response to environmental stimuli such as microbial antigens and allergens, keratinocytes contribute to ILC2 and Th2 cell activation and hyperreactivity of AD skin. The resulting inflammatory environment drives many AD features such as barrier dysfunction, itch, defect in antimicrobial peptide production and infiltration of both innate and adaptive immune cells. Cytokine-mediated crosstalk between T cells and keratinocytes thereby plays a key role in AD onset and progression. Nevertheless, AD is a heterogenous disorder with differences in the immune phenotypes depending on the racial group and age that may impact the predominance of T cell subsets (45, 46).
Impact on Barrier Dysfunction and Pruritus
Proteins expressed during the keratinocyte differentiation process leading to cornified envelope formation are responsible for the stratum corneum barrier function. Epidermal barrier dysfunction in lesional AD skin is partly related to a decreased filaggrin level and tight junction abnormalities. Numerous upregulated cytokines in AD lesions, including IL-4, IL-13, IL-31, IL-22, IL-17A, and oncostatin M, are known to alter barrier function through the inhibition of epidermal barrier protein synthesis (corneodesmosin, filaggrin, involucrin, loricrin, keratin-10), tight junctions (desmocollin, ZO-1, claudin-1, and -4), and/or lipids (fatty acid elongases ELOVL3 and ELOVL6, glucocerebrosidase, EO ceramides) (47–58). Interestingly, IL-24, whose expression is increased in Th2 cytokine-stimulated keratinocytes and epidermis from AD patients, has been suggested as a pivotal mediator for inhibition of keratinocyte differentiation (59–61). Moreover, IL-4 and IL-13 increase the expression in keratinocytes of kallikrein (KLK)5 and KLK7, proteins known to be upregulated in the stratum corneum of AD patients (51, 62). KLKs are key proteases involved in degradation of intercellular adhesion molecules, leading to desquamation, inhibition of barrier integrity and induction of pro-inflammatory cytokine production by keratinocytes, including TSLP (63). In addition, barrier disruption facilitates the entry of microbial products and allergens acting as danger signals that stimulate the release of AD-typical epidermal alarmins (TSLP, IL-25, and IL-33) from keratinocytes (64, 65). These alarmins activate immune cells that populate the skin, including T cells and ILCs, and are major contributors to allergic and type 2 immune response. In addition, they decrease filaggrin, claudin-1 and involucrin expression in keratinocytes (66–70). Through cytokine secretion, both T cells and keratinocytes thereby contribute to the disruption of skin barrier integrity (Figure 1A and Table 1).
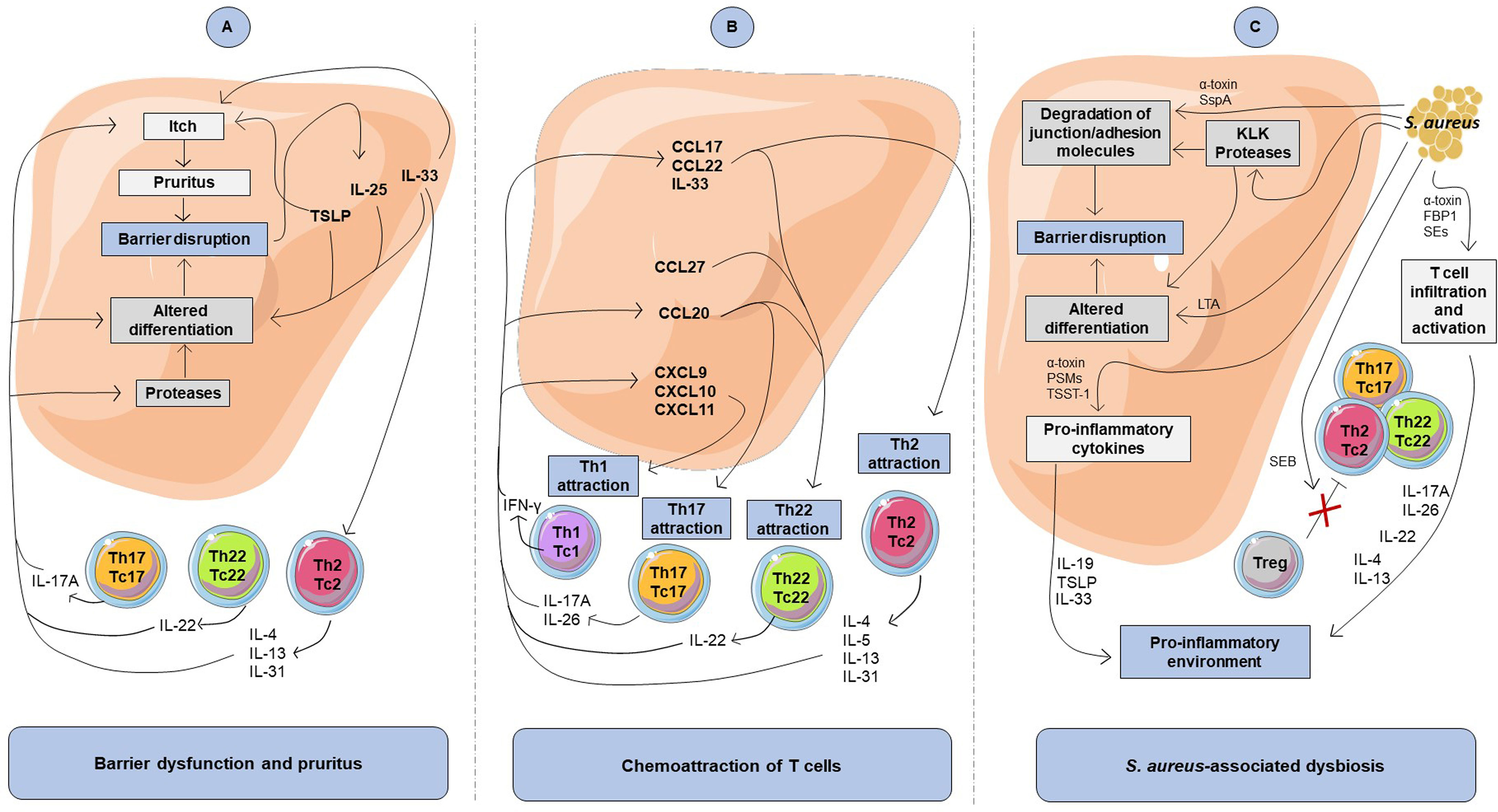
Figure 1 Impact of cytokine-mediated crosstalk between keratinocytes and T cells on atopic dermatitis features. (A) Involvement of the cytokine-mediated crosstalk in barrier dysfunction and pruritus. Th2, Th17, and Th22 cytokines inhibit differentiation markers and favor itch, leading to barrier disruption, which in return induces secretion of pro-inflammatory cytokine including TSLP, IL-33, and IL-25 by keratinocytes. (B) Involvement of the cytokine-mediated crosstalk in chemoattraction of T cells. The keratinocyte-derived inflammatory mediators include chemokines that lead to chemoattraction of T cells. Attraction of T cells and Th2 response promote a pro-inflammatory cytokine production loop in keratinocytes. (C) Impact of S. aureus on keratinocytes and T cells in atopic dermatitis. Virulence factors produced by S. aureus can promote degradation of junctional adhesion molecules and alteration of keratinocyte differentiation that contribute to atopic skin barrier disruption. S. aureus can also favor a pro-inflammatory environment through Th2 cell infiltration into the skin, pro-inflammatory cytokine release by keratinocytes and T cells as well as by inhibiting Treg immunosuppressive activity. FBP1, fibronectin-binding protein-1; LTA, lipoteichoic acid; PSMs, phenol soluble modulins; SEs, staphylococcal enterotoxins; SspA, serine protease A; TSST-1, toxic shock syndrome toxin-1.
Cytokines released by Th2 and Th22 cells, in particular IL-31, IL-4, IL-13 and IL-22, can aggravate barrier alteration through itch-induced scratching. Th2 cells are the major contributor of pruritus via their production of IL-31, known as a pruritogen factor inducing nerve fiber elongation and branching (96). Moreover, IL-4, IL-13, IL-31, IL-33, human β-defensins (hBD)-2 and TSLP are known to directly activate sensory neurons, which cause itching (82, 97, 99, 101, 104). In addition, activation of sensory neurons by IL-4 and IL-13 through IL-4Rα signaling intensifies itch responses to other pruritogens such as IL-31 and histamine (97). Otherwise, IL-22 has been shown to cause chronic pruritus and induces keratinocyte expression of TSLP and IL-33 (90), which in turn contributes to itch response. Consequently, pruritogenic cytokines produced by T cells and keratinocytes promote atopic itch and promote barrier disruption and entry of allergens and dysbiotic bacteria, which may increase skin inflammation (Figure 1A and Table 1).
Impact on Chemoattraction and Polarization of T Cells
Keratinocytes can amplify the attraction and production of cytokines by various subsets of T cells through their release of chemokines, including CCL17, CCL22, CCL20, CCL27, CXCL9, CXCL10 and CXCL11 (73, 75, 105, 106), whose expression is increased in AD skin (15, 19, 107–112). These chemokines influence the skin T cell environment, with recruitment of circulating effector memory Th2, Th22, Th1, and Th17 cells, highlighting the complexity of AD pathogenesis (Figure 1B). In addition, IL-33 secreted by keratinocytes from AD patients exerts a direct chemoattractant activity on Th2 cells (100). Once attracted, T cell subsets will further amplify the inflammatory response through the promotion of chemokines secretion by skin cells, including keratinocytes (50, 72, 75, 77, 84, 95, 98, 113).
In addition to T cell chemoattraction, keratinocyte-derived cytokines may favor exacerbation of a skewed T cell inflammatory response (Figure 1B and Table 1). Indeed, increased secretion of TSLP, IL-33 and IL-25 by epidermal cells can promote secretion of cytokines by Th2 cells (114–116). IL-33 potentiates IL-31 secretion by Th2 cells (96), while TSLP and IL-25 directly promote Th2 cell polarization as well as IL-4, IL-13, and IL-5 secretion (78, 92, 93, 102) which exacerbates barrier disruption through potentiation of the type 2 inflammatory response. In addition, IL-25, produced by both Th2 cells and keratinocytes (117), increases IL-25R receptor expression on Th2 cells, further amplifying IL-25-induced Th2 cytokine secretion (92, 93). Recently, the prominent role of IL-25 was highlighted in a mouse model of AD, which showed that IL-25 is essential for IL-13 production and contributes to epidermal thickening, CD4+ T cell infiltration and the expression of the Th2 cell–attracting chemokines CCL17 and CCL22 (115).
In turn, cytokines released by Th2 and Th22 cells may increase type 2 cytokine secretion by keratinocytes (Table 1). Indeed, IL-4, IL-13, IL-22 and IL-31 induce TSLP, IL-33, CCL17 and CCL22 expression by keratinocytes, both in 2D cultures (77) and 3D models of reconstructed human epidermis (50, 90, 98), a phenomenon that may reinforce skin homing of Th2 and Th22 cells. Moreover, IL-4 increases the expression of the receptor TSLPR on CD4+ T cells, which may promote IL-4 induction by TSLP (78, 79), suggesting a positive regulatory loop between Th2 cells and TSLP. Therefore, the cytokine crosstalk between keratinocytes and T cells reinforces AD as a Th2- and Th22-polarized disease.
Furthermore, IL-18 production by keratinocytes is increased in the epidermis of AD patients (118) and contributes to Th1/Th2 balance regulation that might favor AD physiopathology. IL-18 induces IL-4 and IL-13 production by T cells (87). Moreover, addition of IL-18 on IFN-γ-treated keratinocytes further increases CXCL10 secretion (119). Predominance of Th2 cytokines in AD lesions may regulate Th17 response, IL-25 being known to decrease Th17 cell through inhibition of Th17-inducing cytokine release (94). On the other hand, Th17 cells may also favor a Th2 environment. IL-17A induces IL-19 secretion by keratinocytes (80, 81), a cytokine stimulating the production of Th2 cytokines (88, 120). Moreover, in a mouse model of AD, IL-17A has been shown to mediate Th2 immune response by inducing TSLP and CCL17 expression and IL-4-producing cells (85). In addition, the Th17-derived cytokine IL-26 increases in lesional AD skin and induces CCL20 and IL-33 expression by keratinocytes, suggesting a role of IL-26 for bridging between Th17 and Th2 responses in AD (95). These data suggest that IL-18, IL-19 and IL-26 could be important cytokines of the keratinocyte-T cell crosstalk in AD, which may require further exploration.
Impact on Antimicrobial Peptide Production
The modest increase of antimicrobial peptides (AMPs), such as hBD-2, hBD-3, or LL-37, observed in AD skin as compared to psoriasis lesions, results mainly from the type 2 cytokine microenvironment which limits AMP production by keratinocytes (121, 122). On the one hand, IL-4, IL-13, IL-33, and TSLP inhibit hBD-2, hBD-3, and LL-37 expression (83, 103, 123). On the other hand, other cytokines such as IL-1β, IL-17A, IL-26, IL-22, and IL-31 can stimulate hBD-2, hBD-3, and S100A production by keratinocytes (86, 91, 95, 96, 124). Furthermore, AMPs can initiate negative feedback on their production through Th2 cytokine induction. For example, hBD-2 and hBD-3 induce IL-13 and IL-4 and inhibit IL-17A secretion by T cells (86). Impaired expression of AMPs, key components of keratinocyte defenses against microorganisms, can lead to perturbed innate immunity and favor susceptibility to skin infections and dysbiosis in AD patients.
Role of the Cutaneous Dysbiosis on T Cell and Keratinocyte Crosstalk
AD patients are characterized by skin dysbiosis, with dramatically reduced diversity of cutaneous microbiota and overabundance of staphylococci (mainly S. aureus and S. epidermidis) on lesional AD skin, correlated to disease severity (125–128). A meta-analysis reported that S. aureus colonizes 70% of lesional AD skin and 39% of non-lesional AD skin (129). During lesion recovery or treatment of AD flares, skin bacterial diversity is improved and S. aureus proportion is decreased (128, 130). Some AD features, such as filaggrin deficiency and altered AMP production, associated with elevated skin pH, may favor S. aureus colonization of lesional skin (131–135). S. aureus can counteract cutaneous antibacterial defense through various AMP resistance mechanisms (136–138) and potentiate skin inflammatory response and barrier dysfunction through secretion of various virulence factors, including superantigens, enterotoxins (SEs), fibronectin-binding protein-1 (FBP1), phenol-soluble modulins (PSMs), α-toxin, and proteases (139).
Firstly, S. aureus and S. epidermidis can worsen AD skin barrier disruption by acting directly on keratinocytes. S. aureus-derived compounds, such as lipoteichoic acid (LTA), have been shown to inhibit terminal differentiation of keratinocytes (140, 141). In addition, ɑ-toxin secreted from S. aureus induces skin barrier disruption and cell death on filaggrin-deficient keratinocytes (142, 143), thereby contributing to AD exacerbation. In addition, S. aureus serine protease A (SspA) and S. epidermidis cysteine protease (Ecpa) cleave tight junction proteins and degrade adhesion molecules in the epidermis (125, 144). Finally, S. aureus enhances keratinocyte KLK proteolytic activity, leading to degradation of differentiation proteins such as filaggrin and desmoglein-1 (145). As a result, through various virulence mechanisms, staphylococci-associated dysbiosis promotes disruption of barrier integrity (Figure 1C).
In addition, S. aureus is thought to exacerbate skin inflammation in AD patients through the activity of cytolytic toxins and superantigens. On the one hand, it has been shown that many virulence factors of S. aureus promote keratinocyte pro-inflammatory response. In vitro, PSMs, α-toxin, and TSST-1 induce secretion of pro-inflammatory cytokines and chemokines from keratinocytes (143, 146–148). Interestingly, dysbiosis-driven intracellular IL-1α release from keratinocytes has been shown to trigger chronic skin inflammation in filaggrin-deficient mice (149). Concordantly, studies of epicutaneous S. aureus colonization on mice have revealed that PSMα peptide drives skin inflammation through production of IL-1α and IL-36α by keratinocytes, leading to subsequent IL-17A secretion by T cells (150–152). On the other hand, S. aureus superantigens and cytotoxins enhance cytokine secretion by various effector T cells (153–156). Moreover, staphylococcal superantigens have the ability to bind directly to the major histocompatibility complex class II from antigen-presenting cells and to the T cell receptor without antigen presentation (157), leading to non-specific T cell activation and abundant cytokine secretion (158). For example, the staphylococcal enterotoxins SEE and SEA induce IL-26 secretion by T cells (159), a cytokine promoting AD development (95). At sublytic concentrations, in the absence of antigen-presenting cells α-toxin also activates T cells by upregulating IFN-γ and IL-17A secretion by CD4+ T cells (160, 161).
Furthermore, some data have shown that S. aureus-associated dysbiosis can favor types 2 and 22 immune responses in AD skin. S. aureus components trigger the release of TSLP, IL-33, and IL-19 in keratinocytes (162–164), which could amplify cytokine secretion by Th2 cells. Moreover, cutaneous application of staphylococcal peptidoglycan or δ-toxin on mouse skin has highlighted the involvement of S. aureus in increasing cutaneous infiltration of CCR4+ cells associated with cytokine secretion leading to Th2-dominant inflammation (165, 166). Concordantly, in an epicutaneous sensitization mouse model, topical application of live S. aureus or SEB increase cutaneous accumulation of T cells and type 2 cytokine expression, thereby highlighting a mutually reinforcing role of allergic inflammation and S. aureus colonization in AD skin (167, 168). Moreover, repeated topical applications of S. aureus to mouse skin reproduce AD-like skin inflammation with TRM cells accumulation and IL-4 and IL-17A increased expression (169). In filaggrin-deficient mice, S. aureus entry into the skin is associated with increased expression of inflammatory cytokines, including IL-17A, IL-22 and the type 2 cytokines TSLP, IL-13, and IL-4 (134). Furthermore, SEB, α-toxin, and FBP1 can induce T cell proliferation and production of IL-31, IL-4, IL-13, and IL-22 by T cells (170–172). To summarize, there is ample evidence that virulence factors of S. aureus may promote Th2, Th17 and Th22 cytokine secretion and influence the phenotypic profile of T cells in AD skin, thereby contributing to disease persistence (Figure 1C).
Finally, the intensity of the inflammatory response may be potentiated by S. aureus through several strategies designed to avoid Tregs immunosuppressive activity. Stimulation of Tregs with S. aureus secretome and SEB inhibits their suppressive functions on conventional T cell proliferation, suggesting that S. aureus favors T cell-dependent skin inflammation (29, 173, 174). In addition, reprogramming of Tregs toward a Th2-like phenotype after activation by S. aureus superantigen has been reported (175) and could contribute to the predominance of Th2 effector cells in AD.
Conclusion
Notwithstanding the type 2 response predominance, the AD inflammatory environment is the result of cytokine release from various skin T cell subpopulations, including Th2/Tc2, Th22/Tc22, Th17/Tc17 and Th1/Tc1 subsets, associated with Tregs dysfunction. The crosstalk between T cells and keratinocytes is involved in barrier dysfunction and favors both attraction of T cells and persistence of TRM cells in AD lesions, thereby promoting consistent release of cytokines. The dialogue between T cells and keratinocytes is mediated mostly via a complex dynamic network of cytokines and chemokines. This inflammatory environment resulting from the T cell-keratinocyte interactions is also involved in altered AMP production characteristic of AD, which may favor colonization and persistence of S. aureus on AD lesions. In return, secreted virulence factors by S. aureus act on barrier disruption and exert pro-inflammatory effects on both keratinocytes and T cells, impacting disease-driving mechanisms. Further investigations are needed to better understand the impact of this immune dialog and dysbiosis on the dynamics of skin inflammation in AD patients.
Author Contributions
MH wrote the first draft. KB and CB contributed to the revision of the manuscript. All authors approved the final version of the manuscript.
Conflict of Interest
The authors declare that the research was conducted in the absence of any commercial or financial relationships that could be construed as a potential conflict of interest.
Publisher’s Note
All claims expressed in this article are solely those of the authors and do not necessarily represent those of their affiliated organizations, or those of the publisher, the editors and the reviewers. Any product that may be evaluated in this article, or claim that may be made by its manufacturer, is not guaranteed or endorsed by the publisher.
Acknowledgments
We thank Jeffrey Arsham for English revision of the paper.
Abbreviations
AD, atopic dermatitis; AMP, antimicrobial peptide; CCL, chemokine (C-C motif) ligand; FBP1, fibronectin-binding protein-1; hBD, human beta-defensin; IFN, interferon; IL, interleukin; KLK, kallikrein; LTA, lipoteichoic acid; PSM, phenol-soluble modulin; TARC/CCL17, thymus and activation regulated chemokine; MDC/CCL22, macrophage-derived chemokine; SspA, serine protease A; SE, staphylococcal enterotoxin; Tc, cytotoxic T cells; Th, T helper cells; Treg, regulatory T cells; TRM, resident memory T cells; TSLP, thymic stromal lymphopoietin.
References
1. Peters N, Peters AT. Atopic Dermatitis. Allergy Asthma Proc (2019) 40:433–6. doi: 10.2500/aap.2019.40.4265
2. Chiesa Fuxench ZC, Block JK, Boguniewicz M, Boyle J, Fonacier L, Gelfand JM, et al. Atopic Dermatitis in America Study: A Cross-Sectional Study Examining the Prevalence and Disease Burden of Atopic Dermatitis in the US Adult Population. J Invest Dermatol (2018) 139:583–90. doi: 10.1016/j.jid.2018.08.028
3. Yang G, Seok JK, Kang HC, Cho Y-Y, Lee HS, Lee JY. Skin Barrier Abnormalities and Immune Dysfunction in Atopic Dermatitis. Int J Mol Sci (2020) 21:2867. doi: 10.3390/ijms21082867
4. Williams MR, Gallo RL. The Role of the Skin Microbiome in Atopic Dermatitis. Curr Allergy Asthma Rep (2015) 15:65. doi: 10.1007/s11882-015-0567-4
5. Eichenfield LF, Tom WL, Chamlin SL, Feldman SR, Hanifin JM, Simpson EL, et al. Guidelines of Care for the Management of Atopic Dermatitis. J Am Acad Dermatol (2014) 70:338–51. doi: 10.1016/j.jaad.2013.10.010
6. Paller A, Jaworski JC, Simpson EL, Boguniewicz M, Russell JJ, Block JK, et al. Major Comorbidities of Atopic Dermatitis: Beyond Allergic Disorders. Am J Clin Dermatol (2018) 19:821–38. doi: 10.1007/s40257-018-0383-4
7. Alkon N, Bauer WM, Krausgruber T, Goh I, Griss J, Nguyen V, et al. Single-Cell Analysis Reveals Innate Lymphoid Cell Lineage Infidelity in Atopic Dermatitis. J Allergy Clin Immunol (2021) 149:S0091-6749(21)01198-2. doi: 10.1016/j.jaci.2021.07.025
8. Kader HA, Azeem M, Jwayed SA, Al-Shehhi A, Tabassum A, Ayoub MA, et al. Current Insights Into Immunology and Novel Therapeutics of Atopic Dermatitis. Cells (2021) 10:1392. doi: 10.3390/cells10061392
9. Roan F, Obata-Ninomiya K, Ziegler SF. Epithelial Cell–Derived Cytokines: More Than Just Signaling the Alarm. J Clin Invest (2019) 129:1441–51. doi: 10.1172/JCI124606
10. He H, Suryawanshi H, Morozov P, Gay-Mimbrera J, Del Duca E, Kim HJ, et al. Single-Cell Transcriptome Analysis of Human Skin Identifies Novel Fibroblast Subpopulation and Enrichment of Immune Subsets in Atopic Dermatitis. J Allergy Clin Immunol (2020) 145:1615–28. doi: 10.1016/j.jaci.2020.01.042
11. Hennino A, Vocanson M, Toussaint Y, Rodet K, Benetiere J, Schmitt A-M, et al. Skin-Infiltrating CD8+ T Cells Initiate Atopic Dermatitis Lesions. J Immunol (2007) 178:5571–7. doi: 10.4049/jimmunol.178.9.5571
12. Hijnen D, Knol EF, Gent YY, Giovannone B, Beijn SJP, Kupper TS, et al. CD8(+) T Cells in the Lesional Skin of Atopic Dermatitis and Psoriasis Patients are an Important Source of IFN-γ, IL-13, IL-17, and IL-22. J Invest Dermatol (2013) 133:973–9. doi: 10.1038/jid.2012.456
13. Akdis CA, Akdis M, Simon D, Dibbert B, Weber M, Gratzl S, et al. T Cells and T Cell-Derived Cytokines as Pathogenic Factors in the Nonallergic Form of Atopic Dermatitis. J Invest Dermatol (1999) 113:628–34. doi: 10.1046/j.1523-1747.1999.00720.x
14. Mashiko S, Mehta H, Bissonnette R, Sarfati M. Increased Frequencies of Basophils, Type 2 Innate Lymphoid Cells and Th2 Cells in Skin of Patients With Atopic Dermatitis But Not Psoriasis. J Dermatol Sci (2017) 88:167–74. doi: 10.1016/j.jdermsci.2017.07.003
15. Esaki H, Brunner PM, Renert-Yuval Y, Czarnowicki T, Huynh T, Tran G, et al. Early-Onset Pediatric Atopic Dermatitis Is T H 2 But Also T H 17 Polarized in Skin. J Allergy Clin Immunol (2016) 138:1639–51. doi: 10.1016/j.jaci.2016.07.013
16. Gittler JK, Shemer A, Suárez-Fariñas M, Fuentes-Duculan J, Gulewicz KJ, Wang CQF, et al. Progressive Activation of TH2/TH22 Cytokines and Selective Epidermal Proteins Characterizes Acute and Chronic Atopic Dermatitis. J Allergy Clin Immunol (2012) 130:1344–54. doi: 10.1016/j.jaci.2012.07.012
17. Reynolds G, Vegh P, Fletcher J, Poyner EFM, Stephenson E, Goh I, et al. Developmental Cell Programs Are Co-Opted in Inflammatory Skin Disease. Science (2021) 371:eaba6500. doi: 10.1126/science.aba6500
18. Rojahn TB, Vorstandlechner V, Krausgruber T, Bauer WM, Alkon N, Bangert C, et al. Single-Cell Transcriptomics Combined With Interstitial Fluid Proteomics Defines Cell-Type-Specific Immune Regulation in Atopic Dermatitis. J Allergy Clin Immunol (2020) 146:1056–69. doi: 10.1016/j.jaci.2020.03.041
19. He H, Del Duca E, Diaz A, Kim HJ, Gay-Mimbrera J, Zhang N, et al. Mild Atopic Dermatitis Lacks Systemic Inflammation and Shows Reduced Nonlesional Skin Abnormalities. J Allergy Clin Immunol (2021) 147:1369–80. doi: 10.1016/j.jaci.2020.08.041
20. Suárez-Fariñas M, Tintle SJ, Shemer A, Chiricozzi A, Nograles K, Cardinale I, et al. Nonlesional Atopic Dermatitis Skin is Characterized by Broad Terminal Differentiation Defects and Variable Immune Abnormalities. J Allergy Clin Immunol (2011) 127:954–64. doi: 10.1016/j.jaci.2010.12.1124
21. Guttman-Yassky E, Diaz A, Pavel AB, Fernandes M, Lefferdink R, Erickson T, et al. Use of Tape Strips to Detect Immune and Barrier Abnormalities in the Skin of Children With Early-Onset Atopic Dermatitis. JAMA Dermatol (2019) 155:1333–436. doi: 10.1001/jamadermatol.2019.2983
22. Czarnowicki T, He H, Leonard A, Kim HJ, Kameyama N, Pavel AB, et al. Blood Endotyping Distinguishes the Profile of Vitiligo From That of Other Inflammatory and Autoimmune Skin Diseases. J Allergy Clin Immunol (2019) 143:2095–107. doi: 10.1016/j.jaci.2018.11.031
23. Czarnowicki T, He H, Leonard A, Malik K, Magidi S, Rangel S, et al. The Major Orphan Forms of Ichthyosis Are Characterized by Systemic T-Cell Activation and Th-17/Tc-17/Th-22/Tc-22 Polarization in Blood. J Invest Dermatol (2018) 138:2157–67. doi: 10.1016/j.jid.2018.03.1523
24. Czarnowicki T, He HY, Wen H-C, Hashim PW, Nia JK, Malik K, et al. Alopecia Areata Is Characterized by Expansion of Circulating Th2/Tc2/Th22, Within the Skin-Homing and Systemic T-Cell Populations. Allergy (2018) 73:713–23. doi: 10.1111/all.13346
25. Uttarkar S, Brembilla NC, Boehncke W-H. Regulatory Cells in the Skin: Pathophysiologic Role and Potential Targets for Anti-Inflammatory Therapies. J Allergy Clin Immunol (2019) 143:1302–10. doi: 10.1016/j.jaci.2018.12.1011
26. Antiga E, Quaglino P, Volpi W, Pierini I, Del Bianco E, Bianchi B, et al. Regulatory T Cells in Skin Lesions and Blood of Patients With Bullous Pemphigoid. J Eur Acad Dermatol Venereol (2014) 28:222–30. doi: 10.1111/jdv.12091
27. Verhagen J, Akdis M, Traidlhoffmann C, Schmidgrendelmeier P, Hijnen D, Knol E, et al. Absence of T-Regulatory Cell Expression and Function in Atopic Dermatitis Skin. J Allergy Clin Immunol (2006) 117:176–83. doi: 10.1016/j.jaci.2005.10.040
28. García E-M, Galicia-Carreón J, Novak N. In Vitro Conversion Into CD4+CD25+Foxp3+ Induced Regulatory T Cells Is Reduced in Atopic Dermatitis Patients. Int Arch Allergy Immunol (2020) 181:1–4. doi: 10.1159/000506285
29. Gáspár K, Baráth S, Nagy G, Mócsai G, Gyimesi E, Szodoray P, et al. Regulatory T-Cell Subsets With Acquired Functional Impairment: Important Indicators of Disease Severity in Atopic Dermatitis. Acta Derm Venereol (2015) 95:151–5. doi: 10.2340/00015555-1882
30. Ma L, Xue H-B, Guan X-H, Shu C-M, Wang F, Zhang J-H, et al. The Imbalance of Th17 Cells and CD4 + CD25 High Foxp3 + Treg Cells in Patients With Atopic Dermatitis. J Eur Acad Dermatol Venereol (2014) 28:1079–86. doi: 10.1111/jdv.12288
31. Moosbrugger-Martinz V, Gruber R, Ladstätter K, Bellutti M, Blunder S, Schmuth M, et al. Filaggrin Null Mutations are Associated With Altered Circulating Tregs in Atopic Dermatitis. J Cell Mol Med (2018) 23:1288–99. doi: 10.1111/jcmm.14031
32. Reefer AJ, Satinover SM, Solga MD, Lannigan JA, Nguyen JT, Wilson BB, et al. Analysis of CD25hiCD4+ “Regulatory” T-Cell Subtypes in Atopic Dermatitis Reveals a Novel TH2-Like Population. J Allergy Clin Immunol (2008) 121:415–22.e3. doi: 10.1016/j.jaci.2007.11.003
33. Van Gool F, Nguyen MLT, Mumbach MR, Satpathy AT, Rosenthal WL, Giacometti S, et al. A Mutation in the Transcription Factor Foxp3 Drives T Helper 2 Effector Function in Regulatory T Cells. Immunity (2019) 50:362–77. doi: 10.1016/j.immuni.2018.12.016
34. Brunner PM, Emerson RO, Tipton C, Garcet S, Khattri S, Coats I, et al. Nonlesional Atopic Dermatitis Skin Shares Similar T-Cell Clones With Lesional Tissues. Allergy (2017) 72:2017–25. doi: 10.1111/all.13223
35. Boniface K, Jacquemin C, Darrigade A-S, Dessarthe B, Martins C, Boukhedouni N, et al. Vitiligo Skin Is Imprinted With Resident Memory CD8 T Cells Expressing Cxcr3. J Invest Dermatol (2018) 138:355–64. doi: 10.1016/j.jid.2017.08.038
36. Cheuk S, Schlums H, Gallais Sérézal I, Martini E, Chiang SC, Marquardt N, et al. CD49a Expression Defines Tissue-Resident CD8 + T Cells Poised for Cytotoxic Function in Human Skin. Immunity (2017) 46:287–300. doi: 10.1016/j.immuni.2017.01.009
37. Khalil S, Bardawil T, Kurban M, Abbas O. Tissue-Resident Memory T Cells in the Skin. Inflamm Res (2020) 69:245–54. doi: 10.1007/s00011-020-01320-6
38. Gamradt P, Laoubi L, Nosbaum A, Mutez V, Lenief V, Grande S, et al. Inhibitory Checkpoint Receptors Control CD8+ Resident Memory T Cells to Prevent Skin Allergy. J Allergy Clin Immunol (2019) 143:2147–57.e9. doi: 10.1016/j.jaci.2018.11.048
39. Murata A, Hayashi S-I. CD4+ Resident Memory T Cells Mediate Long-Term Local Skin Immune Memory of Contact Hypersensitivity in BALB/c Mice. Front Immunol (2020) 11:775. doi: 10.3389/fimmu.2020.00775
40. Kim S, Park C, Shin J, Noh J, Kim H, Kim J, et al. 049 Multicytokine-Producing Tissue Resident Memory (T RM) Cells in Atopic Dermatitis Patient. J Invest Dermatol (2016) 136:S9. doi: 10.1016/j.jid.2016.02.074
41. Kim S, Kim J, Park C, Kupper T, Lee K. 022 Distinct Transcriptome Signature of Skin-Resident Memory T Cells and Migratory Memory T Cells in Atopic Dermatitis. J Invest Dermatol (2018) 138:S4. doi: 10.1016/j.jid.2018.03.026
42. Sun Z, Kim JH, Kim SH, Kim HR, Zhang K, Pan Y, et al. Skin-Resident Natural Killer T Cells Participate to Cutaneous Allergic Inflammation in Atopic Dermatitis. J Allergy Clin Immunol (2021) 147:S009167492100097X. doi: 10.1016/j.jaci.2020.11.049
43. Bangert C, Rindler K, Krausgruber T, Alkon N, Thaler FM, Kurz H, et al. Persistence of Mature Dendritic Cells, TH2A, and Tc2 Cells Characterize Clinically Resolved Atopic Dermatitis Under IL-4rα Blockade. Sci Immunol (2021) 6:eabe2749. doi: 10.1126/sciimmunol.abe2749
44. Rindler K, Krausgruber T, Thaler FM, Alkon N, Bangert C, Kurz H, et al. Spontaneously Resolved Atopic Dermatitis Shows Melanocyte and Immune Cell Activation Distinct From Healthy Control Skin. Front Immunol (2021) 12:630892. doi: 10.3389/fimmu.2021.630892
45. Leung DYM. Atopic Dermatitis: Age and Race do Matter! J Allergy Clin Immunol (2015) 136:1265–7. doi: 10.1016/j.jaci.2015.09.011
46. Brunner PM, Guttman-Yassky E. Racial Differences in Atopic Dermatitis. Ann Allergy Asthma Immunol (2019) 122:449–55. doi: 10.1016/j.anai.2018.11.015
47. Berdyshev E, Goleva E, Bronova I, Dyjack N, Rios C, Jung J, et al. Lipid Abnormalities in Atopic Skin are Driven by Type 2 Cytokines. JCI Insight (2018) 3:e98006. doi: 10.1172/jci.insight.98006
48. Boniface K, Diveu C, Morel F, Pedretti N, Froger J, Ravon E, et al. Oncostatin M Secreted by Skin Infiltrating T Lymphocytes Is a Potent Keratinocyte Activator Involved in Skin Inflammation. J Immunol (2007) 178:4615–22. doi: 10.4049/jimmunol.178.7.4615
49. Cornelissen C, Marquardt Y, Czaja K, Wenzel J, Frank J, Lüscher-Firzlaff J, et al. IL-31 Regulates Differentiation and Filaggrin Expression in Human Organotypic Skin Models. J Allergy Clin Immunol (2012) 129:426–33.e8. doi: 10.1016/j.jaci.2011.10.042
50. Danso MO, van Drongelen V, Mulder A, van Esch J, Scott H, van Smeden J, et al. TNF-α and Th2 Cytokines Induce Atopic Dermatitis–Like Features on Epidermal Differentiation Proteins and Stratum Corneum Lipids in Human Skin Equivalents. J Invest Dermatol (2014) 134:1941–50. doi: 10.1038/jid.2014.83
51. Di Z-H, Ma L, Qi R-Q, Sun X-D, Huo W, Zhang L, et al. Gao X-H. T Helper 1 and T Helper 2 Cytokines Differentially Modulate Expression of Filaggrin and its Processing Proteases in Human Keratinocytes. Chin Med J (Engl) (2016) 129:295. doi: 10.4103/0366-6999.174489
52. Dudakov JA, Hanash AM, van den Brink MRM. Interleukin-22: Immunobiology and Pathology. Annu Rev Immunol (2015) 33:747–85. doi: 10.1146/annurev-immunol-032414-112123
53. Furue M. Regulation of Filaggrin, Loricrin, and Involucrin by IL-4, IL-13, IL-17a, IL-22, AHR, and NRF2: Pathogenic Implications in Atopic Dermatitis. Int J Mol Sci (2020) 21:5382. doi: 10.3390/ijms21155382
54. Gruber R, Börnchen C, Rose K, Daubmann A, Volksdorf T, Wladykowski E, et al. Diverse Regulation of Claudin-1 and Claudin-4 in Atopic Dermatitis. Am J Pathol (2015) 185:2777–89. doi: 10.1016/j.ajpath.2015.06.021
55. Hänel KH, Pfaff CM, Cornelissen C, Amann PM, Marquardt Y, Czaja K, et al. Control of the Physical and Antimicrobial Skin Barrier by an IL-31–IL-1 Signaling Network. J Immunol (2016) 196:3233–44. doi: 10.4049/jimmunol.1402943
56. Hatano Y, Terashi H, Arakawa S, Katagiri K. Interleukin-4 Suppresses the Enhancement of Ceramide Synthesis and Cutaneous Permeability Barrier Functions Induced by Tumor Necrosis Factor-α and Interferon-γ in Human Epidermis. J Invest Dermatol (2005) 124:786–92. doi: 10.1111/j.0022-202X.2005.23651.x
57. Kim BE, Leung DYM. Significance of Skin Barrier Dysfunction in Atopic Dermatitis. Allergy Asthma Immunol Res (2018) 10:207. doi: 10.4168/aair.2018.10.3.207
58. Yuki T, Tobiishi M, Kusaka-Kikushima A, Ota Y, Tokura Y. Impaired Tight Junctions in Atopic Dermatitis Skin and in a Skin-Equivalent Model Treated With Interleukin-17. PLoS One (2016) 11:e0161759. doi: 10.1371/journal.pone.0161759
59. Mitamura Y, Nunomura S, Nanri Y, Ogawa M, Yoshihara T, Masuoka M, et al. The IL-13/Periostin/IL-24 Pathway Causes Epidermal Barrier Dysfunction in Allergic Skin Inflammation. Allergy (2018) 73:1881–91. doi: 10.1111/all.13437
60. Mitamura Y, Nunomura S, Furue M, Izuhara K. IL-24: A New Player in the Pathogenesis of Pro-Inflammatory and Allergic Skin Diseases. Allergol Int (2020) 69:S132389301930200X. doi: 10.1016/j.alit.2019.12.003
61. Vu YH, Hashimoto-Hachiya A, Takemura M, Yumine A, Mitamura Y, Nakahara T, et al. IL-24 Negatively Regulates Keratinocyte Differentiation Induced by Tapinarof, an Aryl Hydrocarbon Receptor Modulator: Implication in the Treatment of Atopic Dermatitis. Int J Mol Sci (2020) 21:E9412. doi: 10.3390/ijms21249412
62. Komatsu N, Saijoh K, Kuk C, Liu AC, Khan S, Shirasaki F, et al. Human Tissue Kallikrein Expression in the Stratum Corneum and Serum of Atopic Dermatitis Patients. Exp Dermatol (2007) 16:513–9. doi: 10.1111/j.1600-0625.2007.00562.x
63. Briot A, Deraison C, Lacroix M, Bonnart C, Robin A, Besson C, et al. Kallikrein 5 Induces Atopic Dermatitis–Like Lesions Through PAR2-Mediated Thymic Stromal Lymphopoietin Expression in Netherton Syndrome. J Exp Med (2009) 206:1135–47. doi: 10.1084/jem.20082242
64. Islam SA, Luster AD. T Cell Homing to Epithelial Barriers in Allergic Disease. Nat Med (2012) 18:705–15. doi: 10.1038/nm.2760
65. Uluçkan Ö, Jiménez M, Roediger B, Schnabl J, Díez-Córdova LT, Troulé K, et al. Cutaneous Immune Cell-Microbiota Interactions Are Controlled by Epidermal JunB/AP-1. Cell Rep (2019) 29:844–59.e3. doi: 10.1016/j.celrep.2019.09.042
66. Hvid M, Vestergaard C, Kemp K, Christensen GB, Deleuran B, Deleuran M. IL-25 in Atopic Dermatitis: A Possible Link Between Inflammation and Skin Barrier Dysfunction? J Invest Dermatol (2011) 131:150–7. doi: 10.1038/jid.2010.277
67. Kim BE, Bin L, Ye Y-M, Ramamoorthy P, Leung DYM. IL-25 Enhances HSV-1 Replication by Inhibiting Filaggrin Expression, and Acts Synergistically With Th2 Cytokines to Enhance HSV-1 Replication. J Invest Dermatol (2013) 133:2678–85. doi: 10.1038/jid.2013.223
68. Kim JH, Bae HC, Ko NY, Lee SH, Jeong SH, Lee H, et al. Thymic Stromal Lymphopoietin Downregulates Filaggrin Expression by Signal Transducer and Activator of Transcription 3 (STAT3) and Extracellular Signal-Regulated Kinase (ERK) Phosphorylation in Keratinocytes. J Allergy Clin Immunol (2015) 136:205–8.e9. doi: 10.1016/j.jaci.2015.04.026
69. Nygaard U, van den Bogaard EH, Niehues H, Hvid M, Deleuran M, Johansen C, et al. The “Alarmins” HMBG1 and IL-33 Downregulate Structural Skin Barrier Proteins and Impair Epidermal Growth. Acta Derm Venereol (2017) 97:305–12. doi: 10.2340/00015555-2552
70. Seltmann J, Roesner LM, von Hesler F-W, Wittmann M, Werfel T. IL-33 Impacts on the Skin Barrier by Downregulating the Expression of Filaggrin. J Allergy Clin Immunol (2015) 135:1659–61.e4. doi: 10.1016/j.jaci.2015.01.048
71. Imai T, Nagira M, Takagi S, Kakizaki M, Nishimura M, Wang J, et al. Selective Recruitment of CCR4-Bearing Th2 Cells Toward Antigen-Presenting Cells by the CC Chemokines Thymus and Activation-Regulated Chemokine and Macrophage-Derived Chemokine. Int Immunol (1999) 11:81–8. doi: 10.1093/intimm/11.1.81
72. Harper EG, Guo C, Rizzo H, Lillis JV, Kurtz SE, Skorcheva I, et al. Th17 Cytokines Stimulate CCL20 Expression in Keratinocytes In Vitro and In Vivo: Implications for Psoriasis Pathogenesis. J Invest Dermatol (2009) 129:2175–83. doi: 10.1038/jid.2009.65
73. Xiong N, Fu Y, Hu S, Xia M, Yang J. CCR10 and its Ligands in Regulation of Epithelial Immunity and Diseases. Protein Cell (2012) 3:571–80. doi: 10.1007/s13238-012-2927-3
74. Homey B, Alenius H, Müller A, Soto H, Bowman EP, Yuan W, et al. CCL27–CCR10 Interactions Regulate T Cell–Mediated Skin Inflammation. Nat Med (2002) 8:157–65. doi: 10.1038/nm0202-157
75. Klunker S, Trautmann A, Akdis M, Verhagen J, Schmid-Grendelmeier P, Blaser K. Akdis CA. A Second Step of Chemotaxis After Transendothelial Migration: Keratinocytes Undergoing Apoptosis Release IFN- -Inducible Protein 10, Monokine Induced by IFN-, and IFN- -Inducible -Chemoattractant for T Cell Chemotaxis Toward Epidermis in Atopic Dermatitis. J Immunol (2003) 171:1078–84. doi: 10.4049/jimmunol.171.2.1078
76. Xanthou G, Duchesnes CE, Williams TJ, Pease JE. CCR3 Functional Responses Are Regulated by Both CXCR3 and its Ligands CXCL9, CXCL10 and CXCL11. Eur J Immunol (2003) 33:2241–50. doi: 10.1002/eji.200323787
77. Tsuji G, Hashimoto-Hachiya A, Yen VH, Miake S, Takemura M, Mitamura Y, et al. Aryl Hydrocarbon Receptor Activation Downregulates IL-33 Expression in Keratinocytes via Ovo-Like 1. J Clin Med (2020) 9:891. doi: 10.3390/jcm9030891
78. Tatsuno K, Fujiyama T, Yamaguchi H, Waki M, Tokura Y. TSLP Directly Interacts With Skin-Homing Th2 Cells Highly Expressing its Receptor to Enhance IL-4 Production in Atopic Dermatitis. J Invest Dermatol (2015) 135:3017–24. doi: 10.1038/jid.2015.318
79. Kitajima M, Lee H-C, Nakayama T, Ziegler SF. TSLP Enhances the Function of Helper Type 2 Cells: Cellular Immune Response. Eur J Immunol (2011) 41:1862–71. doi: 10.1002/eji.201041195
80. Oka T, Sugaya M, Takahashi N, Nakajima R, Otobe S, Kabasawa M, et al. Increased Interleukin-19 Expression in Cutaneous T-Cell Lymphoma and Atopic Dermatitis. Acta Derm Venereol (2017) 97:1172–7. doi: 10.2340/00015555-2723
81. Bao L, Alexander JB, Shi VY, Mohan GC, Chan LS. Interleukin-4 Up-Regulation of Epidermal Interleukin-19 Expression in Keratinocytes Involves the Binding of Signal Transducer and Activator of Transcription 6 (Stat6) to the Imperfect Stat6 Sites. Immunology (2014) 143:601–8. doi: 10.1111/imm.12339
82. Campion M, Smith L, Gatault S, Métais C, Buddenkotte J, Steinhoff M. Interleukin-4 and Interleukin-13 Evoke Scratching Behaviour in Mice. Exp Dermatol (2019) 28:1501–4. doi: 10.1111/exd.14034
83. Hata TR, Gallo RL. Antimicrobial Peptides, Skin Infections, and Atopic Dermatitis. Semin Cutan Med Surg (2008) 27:144–50. doi: 10.1016/j.sder.2008.04.002
84. Nograles KE, Zaba LC, Guttman-Yassky E, Fuentes-Duculan J, Suárez-Fariñas M, Cardinale I, et al. Th17 Cytokines Interleukin (IL)-17 and IL-22 Modulate Distinct Inflammatory and Keratinocyte-Response Pathways. Br J Dermatol (2008) 159:1092–102. doi: 10.1111/j.1365-2133.2008.08769.x
85. Nakajima S, Kitoh A, Egawa G, Natsuaki Y, Nakamizo S, Moniaga CS, et al. IL-17A as an Inducer for Th2 Immune Responses in Murine Atopic Dermatitis Models. J Invest Dermatol (2014) 134:2122–30. doi: 10.1038/jid.2014.51
86. Kanda N, Watanabe S. Increased Serum Human β-Defensin-2 Levels in Atopic Dermatitis: Relationship to IL-22 and Oncostatin M. Immunobiology (2012) 217:436–45. doi: 10.1016/j.imbio.2011.10.010
87. Nakanishi K, Yoshimoto T, Tsutsui H, Okamura H. Interleukin-18 Regulates Both Th1 and Th2 Responses. Annu Rev Immunol (2001) 19:423–74. doi: 10.1146/annurev.immunol.19.1.423
88. Liao S-C, Cheng Y-C, Wang Y-C, Wang C-W, Yang S-M, Yu C-K, et al. IL-19 Induced Th2 Cytokines and Was Up-Regulated in Asthma Patients. J Immunol (2004) 173:6712–8. doi: 10.4049/jimmunol.173.11.6712
89. Gallagher G. Interleukin-19: Multiple Roles in Immune Regulation and Disease. Cytokine Growth Factor Rev (2010) 21:345–52. doi: 10.1016/j.cytogfr.2010.08.005
90. Lou H, Lu J, Choi EB, Oh MH, Jeong M, Barmettler S, et al. Expression of IL-22 in the Skin Causes Th2-Biased Immunity, Epidermal Barrier Dysfunction, and Pruritus via Stimulating Epithelial Th2 Cytokines and the GRP Pathway. J Immunol (2017) 198:2543–55. doi: 10.4049/jimmunol.1600126
91. Boniface K, Bernard F-X, Garcia M, Gurney AL, Lecron J-C, Morel F. IL-22 Inhibits Epidermal Differentiation and Induces Proinflammatory Gene Expression and Migration of Human Keratinocytes. J Immunol (2005) 174:3695–702. doi: 10.4049/jimmunol.174.6.3695
92. Angkasekwinai P, Park H, Wang Y-H, Wang Y-H, Chang SH, Corry DB, et al. Interleukin 25 Promotes the Initiation of Proallergic Type 2 Responses. J Exp Med (2007) 204:1509–17. doi: 10.1084/jem.20061675
93. Bredo G, Storie J, Shrestha Palikhe N, Davidson C, Adams A, Vliagoftis H, et al. Interleukin-25 Initiates Th2 Differentiation of Human CD4 + T Cells and Influences Expression of its Own Receptor: IL-25 Influences T Cell Phenotypes. Immun Inflamm Dis (2015) 3:455–68. doi: 10.1002/iid3.87
94. Mantani PT, Vallejo J, Ljungcrantz I, Nilsson J, Björkbacka H, Fredrikson GN. Interleukin-25 Reduces Th17 Cells and Inflammatory Responses in Human Peripheral Blood Mononuclear Cells. Hum Immunol (2018) 79:685–92. doi: 10.1016/j.humimm.2018.06.008
95. Kamijo H, Miyagaki T, Hayashi Y, Akatsuka T, Watanabe-Otobe S, Oka T, et al. Increased Interleukin-26 Expression Promotes Th17 and Th2-Associated Cytokine Production by Keratinocytes in Atopic Dermatitis. J Invest Dermatol (2019) 140:S0022202X19331483. doi: 10.1016/j.jid.2019.07.713
96. Furue M, Yamamura K, Kido-Nakahara M, Nakahara T, Fukui Y. Emerging Role of Interleukin-31 and Interleukin-31 Receptor in Pruritus in Atopic Dermatitis. Allergy (2018) 73:29–36. doi: 10.1111/all.13239
97. Oetjen LK, Mack MR, Feng J, Whelan TM, Niu H, Guo CJ, et al. Sensory Neurons Co-Opt Classical Immune Signaling Pathways to Mediate Chronic Itch. Cell (2017) 171:217–28.e13. doi: 10.1016/j.cell.2017.08.006
98. Dillon SR, Sprecher C, Hammond A, Bilsborough J, Rosenfeld-Franklin M, Presnell SR, et al. Interleukin 31, a Cytokine Produced by Activated T Cells, Induces Dermatitis in Mice. Nat Immunol (2004) 5:752–60. doi: 10.1038/ni1084
99. Liu B, Tai Y, Achanta S, Kaelberer MM, Caceres AI, Shao X, et al. IL-33/ST2 Signaling Excites Sensory Neurons and Mediates Itch Response in a Mouse Model of Poison Ivy Contact Allergy. Proc Natl Acad Sci USA (2016) 113:E7572–9. doi: 10.1073/pnas.1606608113
100. Komai-Koma M, Xu D, Li Y, McKenzie ANJ, McInnes IB, Liew FY. IL-33 is a Chemoattractant for Human Th2 Cells. Eur J Immunol (2007) 37:2779–86. doi: 10.1002/eji.200737547
101. Wilson SR, Thé L, Batia LM, Beattie K, Katibah GE, McClain SP, et al. The Epithelial Cell-Derived Atopic Dermatitis Cytokine TSLP Activates Neurons to Induce Itch. Cell (2013) 155:285–95. doi: 10.1016/j.cell.2013.08.057
102. Rochman Y, Dienger-Stambaugh K, Richgels PK, Lewkowich IP, Kartashov AV, Barski A, et al. TSLP Signaling in CD4 + T Cells Programs a Pathogenic T Helper 2 Cell State. Sci Signal (2018) 11:eaam8858. doi: 10.1126/scisignal.aam8858
103. Lee H, Ryu W-I, Kim HJ, Bae HC, Ryu HJ, Shin JJ, et al. TSLP Down-Regulates S100A7 and ß-Defensin 2 Via the JAK2/STAT3-Dependent Mechanism. J Invest Dermatol (2016) 136:2427–35. doi: 10.1016/j.jid.2016.07.027
104. Tseng P-Y, Hoon MA. Specific β-Defensins Stimulate Pruritus Through Activation of Sensory Neurons. J Invest Dermatol (2021) 142:S0022202X21021692. doi: 10.1016/j.jid.2021.07.178
105. Horikawa T, Nakayama T, Hikita I, Yamada H, Fujisawa R, Bito T, et al. IFN-G-Inducible Expression of Thymus and Activation-Regulated Chemokine/CCL17 and Macrophage-Derived Chemokine/CCL22 in Epidermal Keratinocytes and Their Roles in Atopic Dermatitis. Intern Immunol (2002) 7:767–73. doi: 10.1093/intimm/dxf044
106. Nakayama T, Fujisawa R, Yamada H, Horikawa T, Kawasaki H, Hieshima K, et al. Inducible Expression of a CC Chemokine Liver- and Activation-Regulated Chemokine (LARC)/macrophage Inflammatory Protein (MIP)-3 Alpha/CCL20 by Epidermal Keratinocytes and its Role in Atopic Dermatitis. Int Immunol (2001) 13:95–103. doi: 10.1093/intimm/13.1.95
107. Clausen M-L, Kezic S, Olesen CM, Agner T. Cytokine Concentration Across the Stratum Corneum in Atopic Dermatitis and Healthy Controls. Sci Rep (2020) 10:21895. doi: 10.1038/s41598-020-78943-6
108. Jurakic Toncic R, Jakasa I, Sun Y, Hurault G, Ljubojevic Hadzavdic S, Tanaka RJ, et al. Stratum Corneum Markers of Innate and T Helper Cell-Related Immunity and Their Relation to the Disease Severity in Croatian Patients With Atopic Dermatitis. J Eur Acad Dermatol Venereol JEADV (2021) 35:1186–96. doi: 10.1111/jdv.17132
109. Lyubchenko T, Collins HK, Goleva E, Leung DYM. Skin Tape Sampling Technique Identifies Proinflammatory Cytokines in Atopic Dermatitis Skin. Ann Allergy Asthma Immunol (2021) 126:46–53.e2. doi: 10.1016/j.anai.2020.08.397
110. Möbus L, Rodriguez E, Harder I, Stölzl D, Boraczynski N, Gerdes S, et al. Atopic Dermatitis Displays Stable and Dynamic Skin Transcriptome Signatures. J Allergy Clin Immunol (2021) 147:213–23. doi: 10.1016/j.jaci.2020.06.012
111. Pavel AB, Zhou L, Diaz A, Ungar B, Dan J, He H, et al. The Proteomic Skin Profile of Moderate-to-Severe Atopic Dermatitis Patients Shows an Inflammatory Signature. J Am Acad Dermatol (2020) 82:690–9. doi: 10.1016/j.jaad.2019.10.039
112. Renert-Yuval Y, Del Duca E, Pavel AB, Fang M, Lefferdink R, Wu J, et al. The Molecular Features of Normal and Atopic Dermatitis Skin in Infants, Children, Adolescents, and Adults. J Allergy Clin Immunol (2021) 148:148–63. doi: 10.1016/j.jaci.2021.01.001
113. Purwar R, Werfel T, Wittmann M. IL-13-Stimulated Human Keratinocytes Preferentially Attract CD4+CCR4+ T Cells: Possible Role in Atopic Dermatitis. J Invest Dermatol (2006) 126:1043–51. doi: 10.1038/sj.jid.5700085
114. Borowczyk J, Shutova M, Brembilla NC, Boehncke W-H. IL-25 (IL-17E) in Epithelial Immunology and Pathophysiology. J Allergy Clin Immunol (2021) 148:S0091674920324179. doi: 10.1016/j.jaci.2020.12.628
115. Leyva-Castillo JM, Galand C, Mashiko S, Bissonnette R, McGurk A, Ziegler SF, et al. ILC2 Activation by Keratinocyte-Derived IL-25 Drives IL-13 Production at Sites of Allergic Skin Inflammation. J Allergy Clin Immunol (2020) 145:1606–14.e4. doi: 10.1016/j.jaci.2020.02.026
116. Salimi M, Barlow JL, Saunders SP, Xue L, Gutowska-Owsiak D, Wang X, et al. A Role for IL-25 and IL-33-Driven Type-2 Innate Lymphoid Cells in Atopic Dermatitis. J Exp Med (2013) 210:2939–50. doi: 10.1084/jem.20130351
117. Divekar R, Kita H. Recent Advances in Epithelium-Derived Cytokines (IL-33, IL-25, and Thymic Stromal Lymphopoietin) and Allergic Inflammation. Curr Opin Allergy Clin Immunol (2015) 15:98–103. doi: 10.1097/ACI.0000000000000133
118. Inoue Y, Aihara M, Kirino M, Harada I, Komori-Yamaguchi J, Yamaguchi Y, et al. Interleukin-18 Is Elevated in the Horny Layer in Patients With Atopic Dermatitis and Is Associated With Staphylococcus Aureus Colonization. Br J Dermatol (2011) 164:560–7. doi: 10.1111/j.1365-2133.2010.10145.x
119. Wittmann M, Purwar R, Hartmann C, Gutzmer R, Werfel T. Human Keratinocytes Respond to Interleukin-18: Implication for the Course of Chronic Inflammatory Skin Diseases. J Invest Dermatol (2005) 124:1225–33. doi: 10.1111/j.0022-202X.2005.23715.x
120. Gallagher G, Eskdale J, Jordan W, Peat J, Campbell J, Boniotto M, et al. Human Interleukin-19 and its Receptor: A Potential Role in the Induction of Th2 Responses. Int Immunopharmacol (2004) 4:615–26. doi: 10.1016/j.intimp.2004.01.005
121. Guttman-Yassky E, Lowes MA, Fuentes-Duculan J, Zaba LC, Cardinale I, Nograles KE, et al. Low Expression of the IL-23/Th17 Pathway in Atopic Dermatitis Compared to Psoriasis. J Immunol (2008) 181:7420–7. doi: 10.4049/jimmunol.181.10.7420
122. Ong PY, Ohtake T, Brandt C, Strickland I, Boguniewicz M, Ganz T, et al. Endogenous Antimicrobial Peptides and Skin Infections in Atopic Dermatitis. N Engl J Med (2002) 347:1151–60. doi: 10.1056/NEJMoa021481
123. Alase A, Seltmann J, Werfel T, Wittmann M. Interleukin-33 Modulates the Expression of Human β-Defensin 2 in Human Primary Keratinocytes and may Influence the Susceptibility to Bacterial Superinfection in Acute Atopic Dermatitis. Br J Dermatol (2012) 167:1386–9. doi: 10.1111/j.1365-2133.2012.11140.x
124. Bitschar K, Wolz C, Krismer B, Peschel A, Schittek B. Keratinocytes as Sensors and Central Players in the Immune Defense Against Staphylococcus Aureus in the Skin. J Dermatol Sci (2017) 87:215–20. doi: 10.1016/j.jdermsci.2017.06.003
125. Cau L, Williams MR, Butcher AM, Nakatsuji T, Kavanaugh JS, Cheng JY, et al. Staphylococcus Epidermidis Protease EcpA can be a Deleterious Component of the Skin Microbiome in Atopic Dermatitis. J Allergy Clin Immunol (2020) 147:955–66. doi: 10.1016/j.jaci.2020.06.024
126. Edslev SM, Olesen CM, Nørreslet LB, Ingham AC, Iversen S, Lilje B, et al. Staphylococcal Communities on Skin Are Associated With Atopic Dermatitis and Disease Severity. Microorganisms (2021) 9:432. doi: 10.3390/microorganisms9020432
127. Hülpüsch C, Tremmel K, Hammel G, Bhattacharyya M, de Tomassi A, Nussbaumer T, et al. Skin pH-Dependent Staphylococcus Aureus Abundance as Predictor for Increasing Atopic Dermatitis Severity. Allergy (2020) 75:2888–98. doi: 10.1111/all.14461
128. Kong HH, Oh J, Deming C, Conlan S, Grice EA, Beatson MA, et al. Temporal Shifts in the Skin Microbiome Associated With Disease Flares and Treatment in Children With Atopic Dermatitis. Genome Res (2012) 22:850–9. doi: 10.1101/gr.131029.111
129. Totté JEE, van der Feltz WT, Hennekam M, van Belkum A, van Zuuren EJ, Pasmans SGMA. Prevalence and Odds of Staphylococcus Aureus Carriage in Atopic Dermatitis: A Systematic Review and Meta-Analysis. Br J Dermatol (2016) 175:687–95. doi: 10.1111/bjd.14566
130. Kwon S, Choi JY, Shin J-W, Huh C-H, Park K-C, Du M-H, et al. Changes in Lesional and Non-Lesional Skin Microbiome During Treatment of Atopic Dermatitis. Acta Derm Venereol (2019) 99:284–90. doi: 10.2340/00015555-3089
131. Blicharz L, Rudnicka L, Czuwara J, Waśkiel-Burnat A, Goldust M, Olszewska M, et al. The Influence of Microbiome Dysbiosis and Bacterial Biofilms on Epidermal Barrier Function in Atopic Dermatitis—An Update. Int J Mol Sci (2021) 22:8403. doi: 10.3390/ijms22168403
132. Clausen M-L, Edslev SM, Andersen PS, Clemmensen K, Krogfelt KA, Agner T. Staphylococcus Aureus Colonization in Atopic Eczema and its Association With Filaggrin Gene Mutations. Br J Dermatol (2017) 177:1394–400. doi: 10.1111/bjd.15470
133. van Drongelen V, Haisma EM, Out-Luiting JJ, Nibbering PH, El Ghalbzouri A. Reduced Filaggrin Expression is Accompanied by Increased Staphylococcus Aureus Colonization of Epidermal Skin Models. Clin Exp Allergy J Br Soc Allergy Clin Immunol (2014) 44:1515–24. doi: 10.1111/cea.12443
134. Nakatsuji T, Chen TH, Two AM, Chun KA, Narala S, Geha RS, et al. Staphylococcus Aureus Exploits Epidermal Barrier Defects in Atopic Dermatitis to Trigger Cytokine Expression. J Invest Dermatol (2016) 136:2192–200. doi: 10.1016/j.jid.2016.05.127
135. Danby SG, Cork MJ. pH in Atopic Dermatitis. In: Surber C, Abels C, Maibach H, editors. Current Problems in Dermatology (2018). p. 95–107. S. Karger AG. Basel: Karger. doi: 10.1159/000489523
136. Ryu W-I, Lee H, Bae HC, Jeon J, Ryu HJ, Kim J, et al. IL-33 Down-Regulates CLDN1 Expression Through the ERK/STAT3 Pathway in Keratinocytes. J Dermatol Sci (2018) 90:313–22. doi: 10.1016/j.jdermsci.2018.02.017
137. Scudiero O, Brancaccio M, Mennitti C, Laneri S, Lombardo B, De Biasi MG, et al. Human Defensins: A Novel Approach in the Fight Against Skin Colonizing Staphylococcus Aureus. Antibiotics (2020) 9:198. doi: 10.3390/antibiotics9040198
138. Sieprawska-Lupa M, Mydel P, Krawczyk K, Wójcik K, Puklo M, Lupa B, et al. Degradation of Human Antimicrobial Peptide LL-37 by Staphylococcus Aureus-Derived Proteinases. Antimicrob Agents Chemother (2004) 48:4673–9. doi: 10.1128/AAC.48.12.4673-4679.2004
139. Tam K, Torres VJ. Staphylococcus Aureus Secreted Toxins and Extracellular Enzymes. Microbiol Spectr (2019) 7. doi: 10.1128/microbiolspec.GPP3-0039-2018
140. Brauweiler AM, Goleva E, Leung DYM. Staphylococcus Aureus Lipoteichoic Acid Damages the Skin Barrier Through an IL-1-Mediated Pathway. J Invest Dermatol (2019) 139:1753–61.e4. doi: 10.1016/j.jid.2019.02.006
141. Son ED, Kim H-J, Park T, Shin K, Bae I-H, Lim K-M, et al. Staphylococcus Aureus Inhibits Terminal Differentiation of Normal Human Keratinocytes by Stimulating Interleukin-6 Secretion. J Dermatol Sci (2014) 74:64–71. doi: 10.1016/j.jdermsci.2013.12.004
142. Brauweiler AM, Bin L, Kim BE, Oyoshi MK, Geha RS, Goleva E, et al. Filaggrin-Dependent Secretion of Sphingomyelinase Protects Against Staphylococcal α-Toxin–Induced Keratinocyte Death. J Allergy Clin Immunol (2013) 131:421–7.e2. doi: 10.1016/j.jaci.2012.10.030
143. Hong S-W, Choi E-B, Min T-K, Kim J-H, Kim M-H, Jeon SG, et al. An Important Role of α-Hemolysin in Extracellular Vesicles on the Development of Atopic Dermatitis Induced by Staphylococcus Aureus. PLoS One (2014) 9:e100499. doi: 10.1371/journal.pone.0100499
144. Wang B, McHugh BJ, Qureshi A, Campopiano DJ, Clarke DJ, Fitzgerald JR, et al. IL-1β–Induced Protection of Keratinocytes Against Staphylococcus Aureus -Secreted Proteases Is Mediated by Human β-Defensin 2. J Invest Dermatol (2017) 137:95–105. doi: 10.1016/j.jid.2016.08.025
145. Williams MR, Nakatsuji T, Sanford JA, Vrbanac AF, Gallo RL. Staphylococcus Aureus Induces Increased Serine Protease Activity in Keratinocytes. J Invest Dermatol (2017) 137:377–84. doi: 10.1016/j.jid.2016.10.008
146. Damour A, Robin B, Deroche L, Broutin L, Bellin N, Verdon J, et al. Phenol-Soluble Modulins α are Major Virulence Factors of Staphylococcus Aureus Secretome Promoting Inflammatory Response in Human Epidermis. Virulence (2021) 12:2474–92. doi: 10.1080/21505594.2021.1975909
147. Syed AK, Reed TJ, Clark KL, Boles BR, Kahlenberg JM. Staphlyococcus Aureus Phenol-Soluble Modulins Stimulate the Release of Proinflammatory Cytokines From Keratinocytes and Are Required for Induction of Skin Inflammation. Infect Immun (2015) 83:3428–37. doi: 10.1128/IAI.00401-15
148. Schlievert PM, Roller RJ, Kilgore SH, Villarreal M, Klingelhutz AJ, Leung DYM. Staphylococcal TSST-1 Association With Eczema Herpeticum in Humans. mSphere (2021) 6:e0060821. doi: 10.1128/mSphere.00608-21
149. Archer NK, Jo J-H, Lee SK, Kim D, Smith B, Ortines RV, et al. Injury, Dysbiosis, and Filaggrin Deficiency Drive Skin Inflammation Through Keratinocyte IL-1α Release. J Allergy Clin Immunol (2018) 143:1426–43. doi: 10.1016/j.jaci.2018.08.042
150. Liu H, Archer NK, Dillen CA, Wang Y, Ashbaugh AG, Ortines RV, et al. Staphylococcus Aureus Epicutaneous Exposure Drives Skin Inflammation via IL-36-Mediated T Cell Responses. Cell Host Microbe (2017) 22:653–66.e5. doi: 10.1016/j.chom.2017.10.006
151. Nakagawa S, Matsumoto M, Katayama Y, Oguma R, Wakabayashi S, Nygaard T, et al. Staphylococcus Aureus Virulent Psmα Peptides Induce Keratinocyte Alarmin Release to Orchestrate IL-17-Dependent Skin Inflammation. Cell Host Microbe (2017) 22:667–77.e5. doi: 10.1016/j.chom.2017.10.008
152. Patrick GJ, Liu H, Alphonse MP, Dikeman DA, Youn C, Otterson JC, et al. Epicutaneous Staphylococcus Aureus Induces IL-36 to Enhance IgE Production and Ensuing Allergic Disease. J Clin Invest (2021) 131:143334. doi: 10.1172/JCI143334
153. Wichmann K, Uter W, Weiss J, Breuer K, Heratizadeh A, Mai U, et al. Isolation of α-Toxin-Producing Staphylococcus Aureus From the Skin of Highly Sensitized Adult Patients With Severe Atopic Dermatitis. Br J Dermatol (2009) 161:300–5. doi: 10.1111/j.1365-2133.2009.09229.x
154. Orfali RL, Yoshikawa FSY, Oliveira LM da S, Pereira NZ, de Lima JF, Ramos Y, et al. Staphylococcal Enterotoxins Modulate the Effector CD4+ T Cell Response by Reshaping the Gene Expression Profile in Adults With Atopic Dermatitis. Sci Rep (2019) 9:13082. doi: 10.1038/s41598-019-49421-5
155. Bonifacius A, Goldmann O, Floess S, Holtfreter S, Robert PA, Nordengrün M, et al. Staphylococcus Aureus Alpha-Toxin Limits Type 1 While Fostering Type 3 Immune Responses. Front Immunol (2020) 11:1579. doi: 10.3389/fimmu.2020.01579
156. Ferraro A, Buonocore SM, Auquier P, Nicolas I, Wallemacq H, Boutriau D, et al. Role and Plasticity of Th1 and Th17 Responses in Immunity to Staphylococcus Aureus. Hum Vaccines Immunother (2019) 15:2980–92. doi: 10.1080/21645515.2019.1613126
157. Goldmann O, Medina E. Staphylococcus Aureus Strategies to Evade the Host Acquired Immune Response. Int J Med Microbiol (2018) 308:625–30. doi: 10.1016/j.ijmm.2017.09.013
158. Iwamoto K, Moriwaki M, Miyake R, Hide M. Staphylococcus Aureus in Atopic Dermatitis: Strain-Specific Cell Wall Proteins and Skin Immunity. Allergol Int (2019) 68:309–15. doi: 10.1016/j.alit.2019.02.006
159. Woetmann A, Alhede M, Dabelsteen S, Bjarnsholt T, Rybtke M, Nastasi C, et al. Interleukin-26 (IL-26) is a Novel Anti-Microbial Peptide Produced by T Cells in Response to Staphylococcal Enterotoxin. Oncotarget (2018) 9:19481–9. doi: 10.18632/oncotarget.24603
160. Breuer K, Wittmann M, Kempe K, Kapp A, Mai U, Dittrich-Breiholz O, et al. Alpha-Toxin is Produced by Skin Colonizing Staphylococcus Aureus and Induces a T Helper Type 1 Response in Atopic Dermatitis. Clin Exp Allergy (2005) 35:1088–95. doi: 10.1111/j.1365-2222.2005.02295.x
161. Niebuhr M, Gathmann M, Scharonow H, Mamerow D, Mommert S, Balaji H, et al. Staphylococcal Alpha-Toxin Is a Strong Inducer of Interleukin-17 in Humans. Infect Immun (2011) 79:1615–22. doi: 10.1128/IAI.00958-10
162. Al Kindi A, Williams H, Matsuda K, Alkahtani AM, Saville C, Bennett H, et al. Staphylococcus Aureus Second Immunoglobulin-Binding Protein Drives Atopic Dermatitis via IL-33. J Allergy Clin Immunol (2020) 147:1354–68. doi: 10.1016/j.jaci.2020.09.023
163. Myles IA, Fontecilla NM, Valdez PA, Vithayathil PJ, Naik S, Belkaid Y, et al. Signaling via the IL-20 Receptor Inhibits Cutaneous Production of IL-1β and IL-17A to Promote Infection With Methicillin-Resistant Staphylococcus Aureus. Nat Immunol (2013) 14:804–11. doi: 10.1038/ni.2637
164. Vu AT, Baba T, Chen X, Le TA, Kinoshita H, Xie Y, et al. Staphylococcus Aureus Membrane and Diacylated Lipopeptide Induce Thymic Stromal Lymphopoietin in Keratinocytes Through the Toll-Like Receptor 2-Toll-Like Receptor 6 Pathway. J Allergy Clin Immunol (2010) 126:985–93, 993.e1–3. doi: 10.1016/j.jaci.2010.09.002
165. Matsui K, Nishikawa A. Percutaneous Application of Peptidoglycan From Staphylococcus Aureus Induces Infiltration of CCR4+ Cells Into Mouse Skin. J Investig Allergol Clin Immunol (2011) 21:354–62.
166. Matsuo K, Nagakubo D, Komori Y, Fujisato S, Takeda N, Kitamatsu M, et al. CCR4 Is Critically Involved in Skin Allergic Inflammation of BALB/c Mice. J Invest Dermatol (2018) 138:1764–73. doi: 10.1016/j.jid.2018.02.027
167. Laouini D, Kawamoto S, Yalcindag A, Bryce P, Mizoguchi E, Oettgen H, et al. Epicutaneous Sensitization With Superantigen Induces Allergic Skin Inflammation. J Allergy Clin Immunol (2003) 112:981–7. doi: 10.1016/j.jaci.2003.07.007
168. Leyva-Castillo J-M, McGurk A, Geha MDR. Allergic Skin Inflammation and S. Aureus Skin Colonization are Mutually Reinforcing. Clin Immunol Orlando Fla (2020) 218:108511. doi: 10.1016/j.clim.2020.108511
169. Braun C. ESDR010 - Innate and Adaptive Immunity to Staphylococcus Aureus Contribute to the Development of Atopic Dermatitis-Like Skin Inflammation. J Invest Dermatol (2021) 141:supplement S150. doi: 10.26226/morressier.61081ff8bc981037240fe392
170. Niebuhr M, Scharonow H, Gathmann M, Mamerow D, Werfel T. Staphylococcal Exotoxins are Strong Inducers of IL-22: A Potential Role in Atopic Dermatitis. J Allergy Clin Immunol (2010) 126:1176–83.e4. doi: 10.1016/j.jaci.2010.07.041
171. Niebuhr M, Mamerow D, Heratizadeh A, Satzger I, Werfel T. Staphylococcal α-Toxin Induces a Higher T Cell Proliferation and Interleukin-31 in Atopic Dermatitis. Int Arch Allergy Immunol (2011) 156:412–5. doi: 10.1159/000323905
172. Farag AK, Roesner LM, Wieschowski S, Heratizadeh A, Eiz-Vesper B, Kwok WW, et al. Specific T Cells Targeting Staphylococcus Aureus Fibronectin-Binding Protein 1 Induce a Type 2/Type 1 Inflammatory Response in Sensitized Atopic Dermatitis Patients. Allergy (2021) 0:1–9. doi: 10.1111/all.15120
173. Laborel-Préneron E, Bianchi P, Boralevi F, Lehours P, Fraysse F, Morice-Picard F, et al. Effects of the Staphylococcus Aureus and Staphylococcus Epidermidis Secretomes Isolated From the Skin Microbiota of Atopic Children on CD4+ T Cell Activation. PLoS One (2015) 10:e0141067. doi: 10.1371/journal.pone.0141067
174. Ou L-S, Goleva E, Hall C, Leung DYM. T Regulatory Cells in Atopic Dermatitis and Subversion of Their Activity by Superantigens. J Allergy Clin Immunol (2004) 113:756–63. doi: 10.1016/j.jaci.2004.01.772
175. Lin Y-T, Wang C-T, Chao P-S, Lee J-H, Wang L-C, Yu H-H, et al. Skin-Homing CD4+ Foxp3+ T Cells Exert Th2-Like Function After Staphylococcal Superantigen Stimulation in Atopic Dermatitis Patients: Skin-Homing SsAg Stimulated Foxp3+ T Cells in AD. Clin Exp Allergy (2011) 41:516–25. doi: 10.1111/j.1365-2222.2010.03681.x
Keywords: cytokines, T cells, keratinocytes, atopic dermatitis (AD), staphylococcus aureus
Citation: Humeau M, Boniface K and Bodet C (2022) Cytokine-Mediated Crosstalk Between Keratinocytes and T Cells in Atopic Dermatitis. Front. Immunol. 13:801579. doi: 10.3389/fimmu.2022.801579
Received: 25 October 2021; Accepted: 07 March 2022;
Published: 07 April 2022.
Edited by:
Valerie Julia, Galderma, SwitzerlandReviewed by:
Yasutaka Mitamura, University of Zurich, SwitzerlandJohn Common, Agency for Science, Technology and Research (A*STAR), Singapore
Copyright © 2022 Humeau, Boniface and Bodet. This is an open-access article distributed under the terms of the Creative Commons Attribution License (CC BY). The use, distribution or reproduction in other forums is permitted, provided the original author(s) and the copyright owner(s) are credited and that the original publication in this journal is cited, in accordance with accepted academic practice. No use, distribution or reproduction is permitted which does not comply with these terms.
*Correspondence: Charles Bodet, charles.bodet@univ-poitiers.fr