- 1Department of Biochemistry and Biotechnology, Kwame Nkrumah University of Science and Technology (KNUST), Kumasi, Ghana
- 2Kumasi Centre for Collaborative Research in Tropical Medicine (KCCR), Kumasi, Ghana
- 3Department of Theoretical and Applied Biology, Kwame Nkrumah University of Science and Technology (KNUST), Kumasi, Ghana
The T cell immune responses in filarial infections are primarily mediated by CD4+ T cells and type 2-associated cytokines. Emerging evidence indicates that CD8+ T cell responses are important for anti-filarial immunity, however, could be suppressed in co-infections. This review summarizes what we know so far about the activities of CD8+ T cell responses in filarial infections, co-infections, and the associations with the development of filarial pathologies.
Filarial Infections: An Overview
Filarial infections of animals and humans include lymphatic filariasis, onchocerciasis, loiasis, and mansonellosis. Lymphatic filariasis is caused by Wuchereria bancrofti, Brugia malayi, and Brugia timori, with W. bancrofti accounting for over 90% of such infections (1). The Onchocerca volvulus is responsible for onchocerciasis, popularly called river blindness. The other disease-causing species of filarial parasites are Loa loa and Mansonella sp., causing loiasis and mansonellosis, respectively. The life-cycle of filarial parasites is relatively complex with several distinct morphological stages in both vector and mammalian hosts, as shown with W. bancrofti in Figure 1. For lymphatic filariasis (LF), the most prominent pathological manifestations are mediated by immune responses against the adult worms and infective stage larvae (2), leading to lymphedema and hydrocele (Figure 1). LF is the second largest cause of disability globally and approximately 40% of the global disease burden of lymphatic filariasis occurs in Africa (3). Current treatment strategies include mass drug administration (MDA) regimens and vector control measures. The MDA programs involve the yearly distribution of microfilaricidal drugs constituting single doses of 400 mg of albendazole (ALB) plus either 150–200 mg/kg of ivermectin (IVM) or 6 mg/kg of Diethylcarbamazine (DEC) administered together for 4–6 years. Tetracycline-based drugs such as doxycycline are used as macrofilaricidal agents (4, 5). Vector control strategies have been used to effectively interrupt the transmission of LF in certain countries (6).
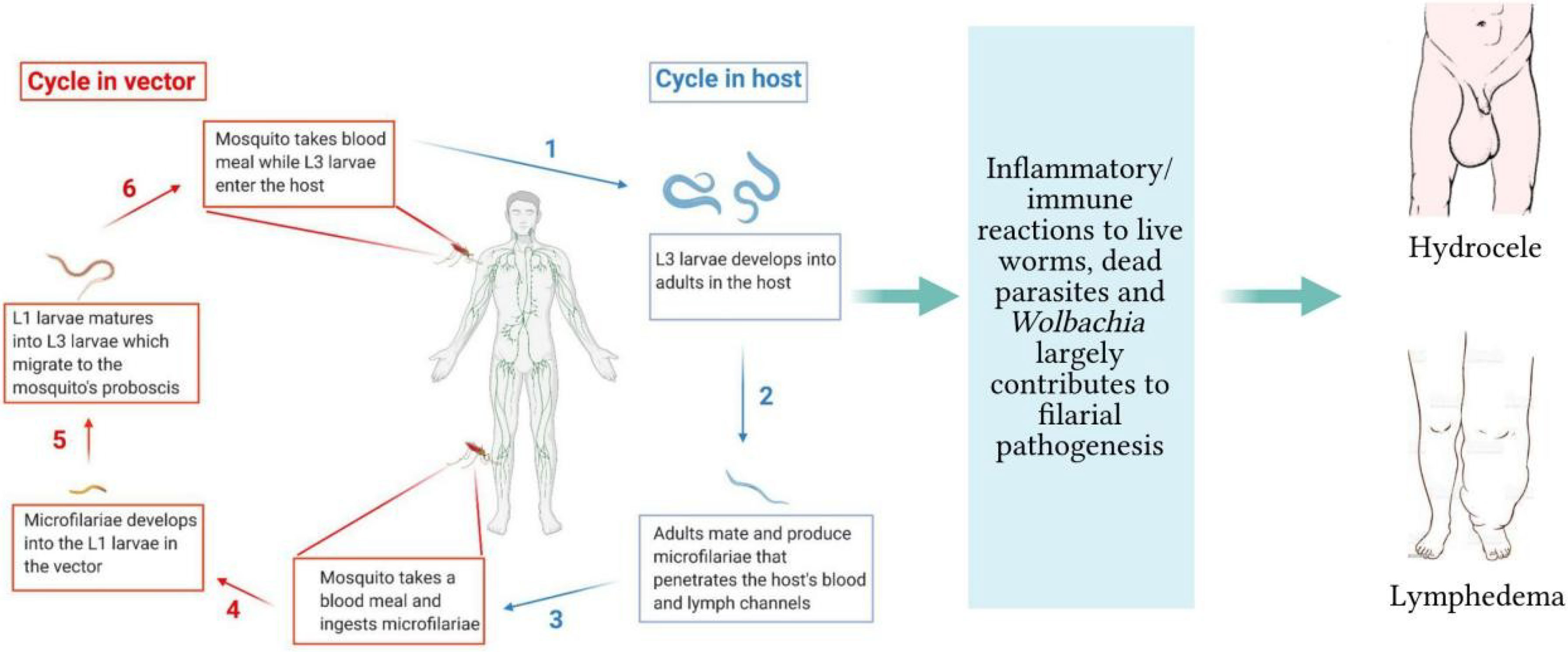
Figure 1 Life-cycle of filarial parasites demonstrated with W. bancrofti and the associated filarial pathologies. (1) The vector transmits the infective-stage larvae into the human when taking a blood meal. (2) The L3 mature into adult worms, which produce microfilariae (3). They migrate to the lymphatics and blood for circulation. (4) The vector again takes up the microfilariae during a blood meal on an infected host. (5) The microfilariae develop into the L1 stage. (6) The L1 larvae mature into L3 larvae, which migrate to the vector’s proboscis via the haemocoel. (1) The infected vector transmits the infective-stage larvae into the human host during a blood meal. While in the host, inflammatory responses to live/dead parasites and immuno-regulatory mechanisms contribute to lymphedema and hydrocele.
Helper T Cells in Anti-Filarial Immunity and Immunoregulation: An Overview
T cell-mediated immune responses are major components of the anti-filarial immunity, as per evidence from experimental animal models and antigen stimulation studies (7–9). This T cell-mediated response has been characterized by activities of both T helper 1 and T helper 2 cytokines, depending on the stage of parasite/infection (10–12). At the interplay of the Th1/Th2 activities are regulatory T cells (Treg) which may be simultaneously active during the filarial infections (13, 14). Thus, several scenarios of helper T cell responses could be observed. First, the responses can be dominated by strong Th1 cytokines with diminished Th2 cytokine activity as a result of poor regulation by Treg. Another scenario could involve a dominant type 2 cytokine response with a marginal activity of Th1, due to immunoregulatory activities. There could also be well-balanced Th1/Th2 responses when Treg activities are sufficient. The activities of primed CD4+ T cells involved in Onchocerca microfilariae clearance in infected mice are dominated by Th2 responses (15). In the early stages of filarial infection, an increased expression of Th1 cytokines, particularly triggered by antigen-presenting cells have been reported (7). This suggests that the initial responses against infective stage larva involve pro-inflammatory responses induced by innate components although the primary responses against the parasites are of Th2 phenotype. A large body of evidence shows that the interactions with host innate cells involve toll-like receptors (16–18). The Wolbachia induces dendritic cell activation and IFN-γ secretion which are correlated with increased TLR2 expression (19). Studies with O. volvulus keratitis-infected mice demonstrated that IFN-γ increased expression of TLR2 on corneal macrophages which triggered the production of TNF-α, interleukin-6 (IL-6), IL-1α, and IL-1β in macrophages (17). Moreover, TLR2 is involved in dendritic cell activation, IFN-γ secretion, and neutrophil recruitment (17, 18). In addition to TLR2, the Wolbachia induces innate immune responses through TLR4 and TLR6 and involves MyD88 (18, 20). The W. bancrofti microfilaria sheath protein directly interacts with TLR4 to mediate macrophage pro-inflammatory responses (IL-6, TNF-α, and IL-1β) via NF-kB activation (20, 21).
Reports by Mukherjee et al. (22) demonstrated that W. bancrofti sheath antigen promotes maturation and activation of dendritic cells by directly interacting with TLR4. In the same study, the matured dendritic cells promoted type-1 cytokines and regulatory T cells (Treg) responses while proportions of Th2, Th17, IL-4, and IL-17A were low (22). While the low Th2 responses could be a characteristic of the sheath antigen, there is a possibility of immunoregulatory activities by Treg. Studies have shown that the Th2 responses to B. malayi adult worm antigen are enhanced upon abrogation of Treg (23). Elsewhere, depletion of Treg promoted Th1 responses without affecting Th2 proportions (24). The Wolbachia surface protein promotes pro-inflammatory responses in mice by increasing the production of Th17 cells while decreasing the levels of Treg (25, 26). In addition, neutralizing CD25 and GITR in B. malayi L3-infected mice elevated Th17 and IFN-γ levels while reducing IL-10 (26). Figure 2 summarizes the mediation of cytokine responses through antigen-presenting cells during filarial infections. This interplay of the type-1 and type-2 CD4+ T cell responses in anti-filarial immunity and the immunomodulatory actions of Treg have been extensively reviewed elsewhere (13, 14).
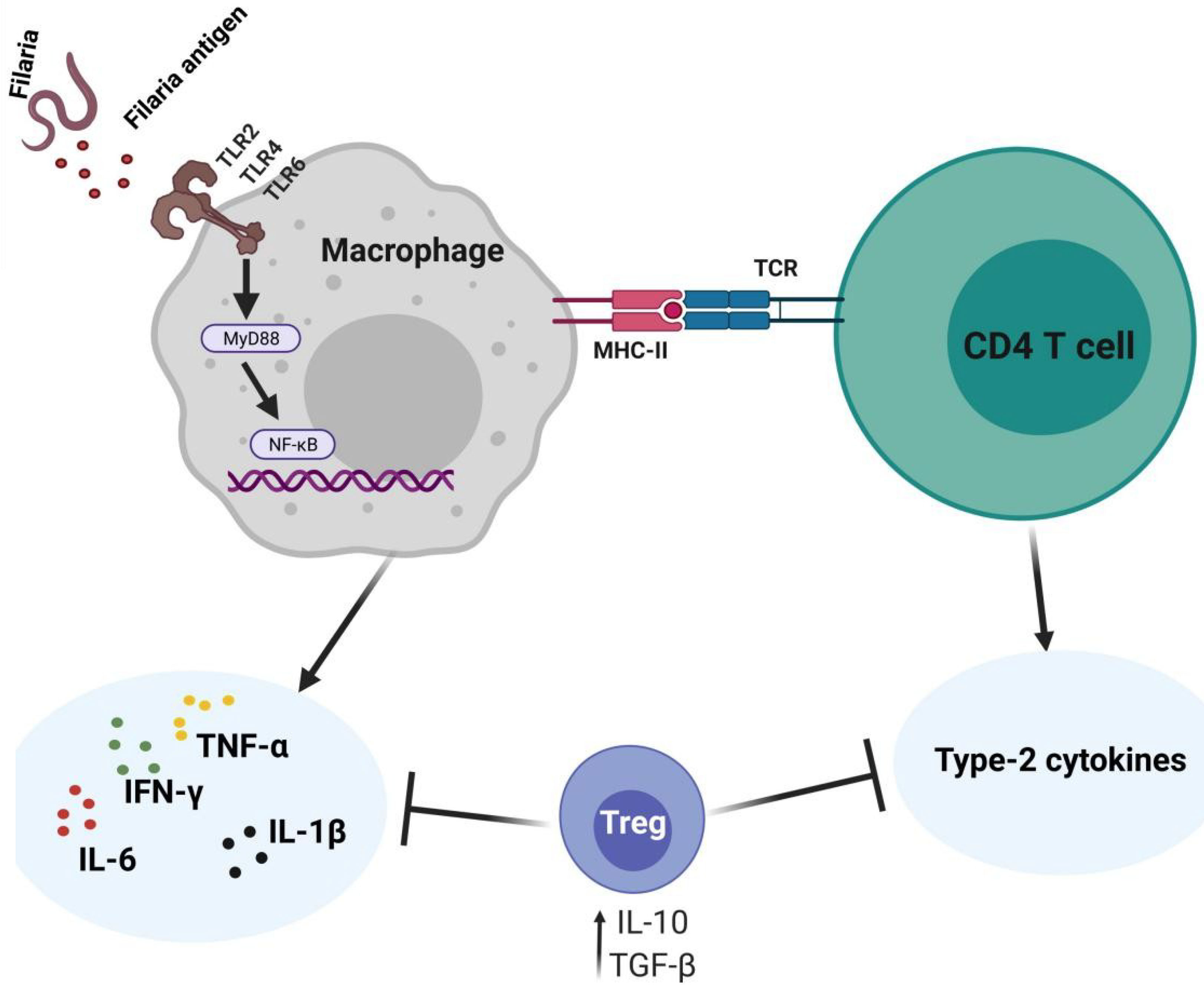
Figure 2 Cytokine expressing profiles against filaria and immunoregulation. The filaria and their endosymbiont interact with TLRs on the APCs to induce pro-inflammatory responses via NF-kB signaling. The CD4+ T cells expressing type-2 cytokines are involved in parasite clearance. Regulatory T cells are simultaneously involved in modulating the Th1/Th2 responses.
Here, we discuss the relevance of CD8+ T cells in the immune responses against filarial parasites, based on documented evidence from animal models and human subjects. We also discuss the roles of CD8+ T cells in the development of filarial pathologies and their possible involvement in immunomodulatory mechanisms during filarial co-infections.
CD8+ T Cells in Anti-Filarial Immunity
Evidence From Studies Involving Humans
There is currently insufficient evidence from human studies which clarify the mechanisms of CD8+ T cell activities in anti-filarial immunity. However, available data from studies in human subjects demonstrate the elevated levels of CD8+ T cells during filarial infections, thus, depicts potential roles in the immune responses against filarial parasites or immunopathology. Kalinkovich and colleagues examined a group of helminth-infected Ethiopian immigrants in Israel and reported elevated CD8+ T cells in this infected group (27). Moreover, W. bancrofti-infected individuals reportedly had increased frequencies of activated CD8+ T cells compared to non-infected subjects (28). From a cohort of 12 African adults with ocular onchocerciasis, Chan et al. found that levels of CD8+ T cells were significantly elevated in the infected group (29). There are additional reports that CD8+ T cells may have regulatory roles during the induction of ocular inflammation by O. volvulus antigen-specific CD4+ T cells (30).
Filarial antigen stimulation studies suggest that type 2 cytokines mediate the cytotoxic activities of CD8+ T cells against filarial parasites. In individuals with patent infections, CD8+ T cells expressing antigen-specific type 1 cytokines (IFN-γ, TNF-α, and IL-22) were diminished, while levels of type 2 cytokine (IL-4, IL-9, IL-13, and IL-21)-expressing CD8+ T cells were elevated (31). This may suggest that CD8+ T cell responses in patent LF infections are mediated by antigen-specific type 2 cytokines (31, 32). Notwithstanding, treatment of the filarial infection changes the CD8+ T cell cytokine secretion pattern, thus, the regulation of these patterns of CD8+ T cell cytokine release is dependent on antigen presence or disease state (31, 32). The activities of CD8+ are modulated by IL-10 depending on the stage of infection (31, 32). CD8+ T cells expressing IL-24 and IL-19 were increased while IL-26 expressing CD4+ T cells were diminished in asymptomatic infected individuals compared to individuals presenting with pathology (32). These regulation of parasite antigen-specific IL-19 and IL-24 expressing CD8+ T cells are more dependent on the stage of the infection than on IL-10, IL-1β, and IL-23 as observed in the regulation of CD4+ T cell response (31–33). Filaria antigen-specific type-1 cytokines (TNF-α, IFN-γ, IL-22) expressing CD8+ T cells were reportedly lower in asymptomatic infected individuals, relative to individuals presenting with pathology (31). This evidence supports the notion that CD8+ T cell responses in filarial infections may be mediated by type 2 cytokines while filarial pathologies are driven by type 2 responses, as a result of continuous antigen presence and immunomodulation (Figure 3).
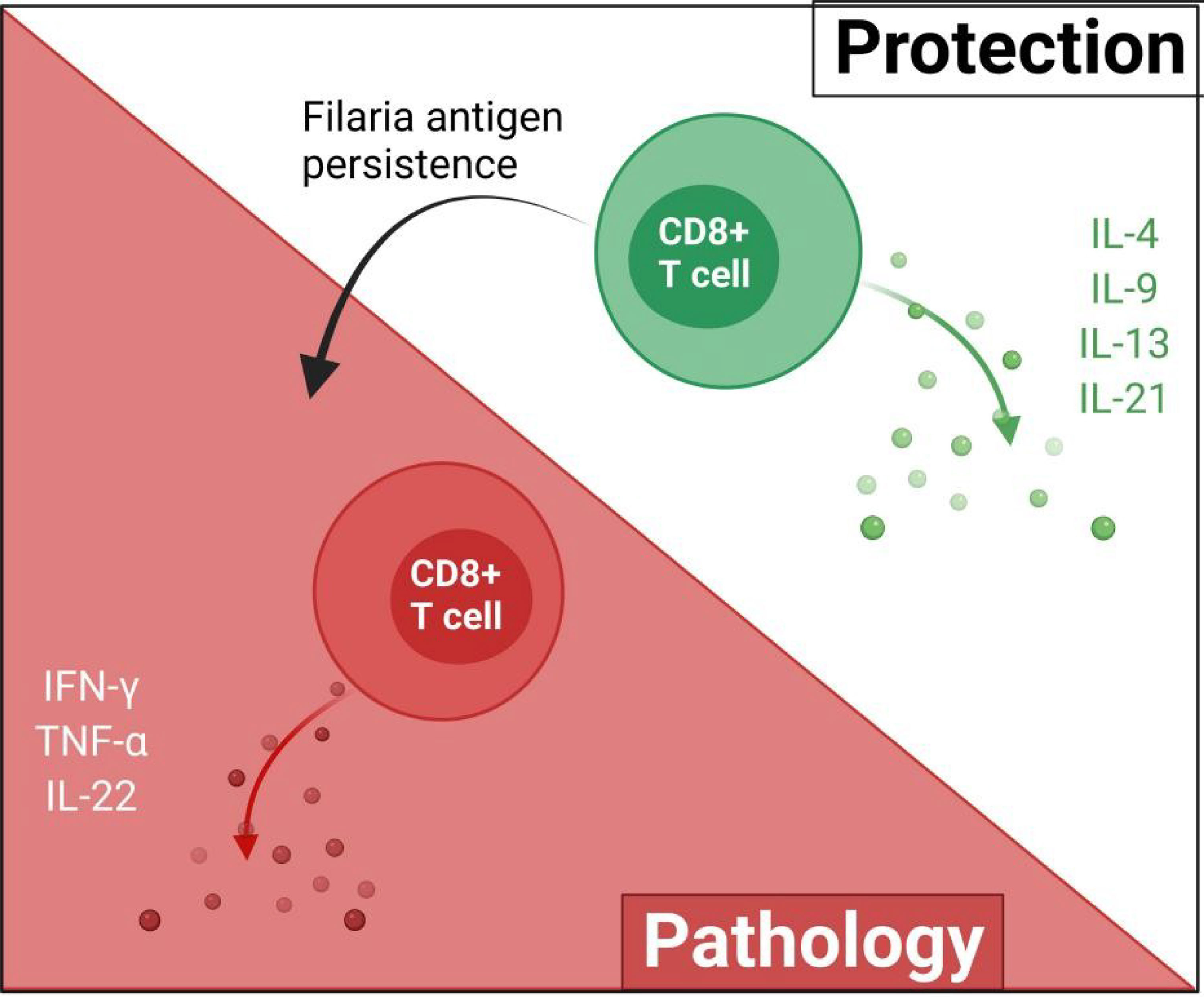
Figure 3 The possible roles of CD8+ in filarial immunity. The protective effects of CD8+ T cells are mediated by type-2 cytokines and upon the persistence of the filaria antigens, switch to pro-inflammatory type-1 responses which ultimately contribute to filarial pathology.
Evidence From Studies With Animal Models
An early description of T cell-mediated immunity in experimental filaria excluded contributions from CD8+ T cells (34). β2-microglobulin deficient mice, which lack MHC class I molecules and therefore do not elicit CD8+ T cell cytotoxicity, were able to establish a resistance to the infective parasites of B. malayi (34). Several in vivo immunization studies, however, also suggested possible CD8+ T cell cytotoxic activities during filarial infections. The B. malayi trehalose-6-phosphate phosphatase antigen induced high levels of CD8+ T cells as part of the host antigen-specific cellular immune responses (35). Immunization of mice with B. malayi Wolbachia recombinase A resulted in a significant expansion of CD8+ T cells in the host spleen (36). Studies in TCR transgenic mice have also shown that the O. volvulus antigens OvALT-2 and OvNLT-1 exert suppressive effects on CD8+ T cell proliferation and cytokine release (37). Recently, heterologous prime-boost vaccination administration of B. malayi heavy chain myosin induced enhanced CD8+ T cell expansion in mouse spleen (38). Although CD8+ T cell levels are elevated during filarial infections, it may be that CD8+ T cell-mediated immunity is most relevant at the initial stages of the infections when the parasite is establishing itself in the host. Babu and Nutman explored the events of host immunity that occur in the early stages following infection with live B. malayi L3 parasites and reported that efficient CD8+ T cell responses are noticed at the very early stages of infection (7). Studies with Onchocerca lienalis-infected mice model demonstrated the contributions of T cell-mediated immunity against microfilariae, which is dominated by CD8+ T cells at the initial stages of the infection (15). The filaria-specific CD8+ T cells expressing cytokines during filarial infections are summarized in Table 1.
CD8+ T Cells and Associations With Filarial Pathology
The degenerating filaria and its endosymbiont promotes pro-inflammatory responses which drive the pathologies associated with the disease. The interactions between the microfilariae (Mf) and host macrophages result in the activation of pro-inflammatory macrophage subtype which induces a pro-inflammatory response (22, 40) aimed at promoting microfilariae clearance (7, 41). However, the macrophage pro-inflammatory responses executed by IL-6, TNF-α, IL-1β, especially to dead parasites contribute to immunopathology (41).
The proportions of CD8+ T cells appear to vary during asymptomatic infections and pathological infections, suggesting that the activities of CD8+ T cells in filarial infections are dependent on the stage of the infection. In terms of cellular subsets, individuals presenting with chronic filarial pathologies have elevated levels of activated CD8+ T lymphocytes when compared to individuals with Mf only (42). Further analysis of patient sera confirmed the elevation of soluble CD8+ T cells in individuals with chronic LF pathology than those at an asymptomatic Mf stage (43). Differential gene expression analysis of blood samples from Loa loa-infected individuals originating from Cameroon, Gabon, and Nigeria have defined the functional annotation of gene expression profiles in CD8+ T cells (44). The responses to microfilaria antigens by CD8+ T cells in endemic individuals were characterized by inflammatory-driven attacks and cell death (44). Furthermore, studies in Loa loa-infected rhesus monkeys suggest that CD8+ T cells may have a role in the hypo-responsiveness to antigens of Loa loa parasites (45). Studies in B. malayi-infected monkeys also showed that levels of CD8+ T cells were increased in symptomatic models than in asymptomatic ones (46).
CD8+ T cells expressing type 1 cytokine responses have been associated with clinical filarial pathologies (2, 31). IFN-γ- and TNF-α-producing CD8+ T cells are known to promote filarial pathology particularly through the stimulation of lymphangiogenic growth factors and inflammatory cell migration (47–49). These observations provide preliminary evidence on the involvement of CD8+ T cells in filarial pathology. The pathophysiological onset of filarial infections is believed to be driven at the cellular level via inappropriate regulation of CD8+ T cell activation in an increased pro-inflammatory-mediated fashion (31, 32), as summarized in Figure 3.
Role of CD8+ T Cells in Filarial Co-Infection
Helminths are known to be master modulators of host immune responses (50). The immunomodulatory benefits of helminths are being exploited for therapeutic purposes against inflammatory bowel diseases and autoimmunity (51). The filarial immuno-regulatory influence on disease progression and control has been demonstrated with several conditions, including filarial-plasmodium co-infection (52), filarial-mycobacterium co-infection (53), filarial-virus co-infections (54) and allergic reactions (55).
Filarial-Virus Co-Infection
Given that both filaria and HIV infections compromise the host immune system, one would anticipate that their co-existence would affect immune responses. It is established that type-1 responses mediate immunity to viruses (56), however, filarial parasites mostly induce a type 2-dependent immunity in their hosts and are capable of regulating these responses to enhance their survival. The suppression of virus-specific CD8+ T cell cytotoxicity in HIV-Schistosoma mansoni co-infected mice models, with a corresponding delay in viral clearance, has been reported previously (57). The Schistosoma-induced reduction in cytotoxic T lymphocyte responsiveness to HIV antigens was markedly due to diminished induction of IFN-γ and IL-2, either through direct IL-10 activities or parasite-induced immunomodulation of innate cells (57). Similar findings in norovirus-Trichinella co-infected mice have been reported, where the modulation of viral-specific CD8+ T cell responses was observed (58). This inhibition of antiviral immunity was associated with innate immunomodulatory mechanisms during virus-helminth co-infection (58). Thus, the efficiency of viral immune responses can be suppressed during helminth-virus co-infections, at least for S. mansoni and Trichinella spiralis.
Although the specific immunomodulatory influence filarial parasites have on HIV co-infections in vivo is not clearly established, several studies have provided insights into the situation. Nielsen et al. (59) found no influence of HIV-W. bancrofti co-infections on the modulation of anti-viral immunity or virus clearance. Notwithstanding, adult B. malayi antigen (BmA) influenced HIV-1 trans-infection of CD4+ T cells in vitro (60). The HIV-1 trans-infection is a common mechanism of virus infection of CD4+ T cells by APCs such as dendritic cells and macrophages. The inhibition of CD4+ T cell trans-infection involves the blocking of HIV-1 capture and transfer through an interaction between the BmA and dendritic cells, thus, preventing the virus from interacting with dendritic cells (60). The BmA, however, does not influence dendritic cell maturation, cytokine production, and HIV-1 replication in CD4+ T-cells (60). Although adult B. malayi antigens can influence HIV-1 trans-infection of CD4+ T cells, they have no deleterious impact on dendritic cell-derived T helper cytokine profiles against the virus (60). On the role of CD8+ T cells in filarial-immunoregulation of viral infections, a considerable gap remains. Dietze et al. (54) studied the immunomodulatory effects of filaria-virus co-infection using an Litosomodes sigmodontis-Friend virus (FV) co-infected mice model. Both filaria-specific and viral-specific humoral responses were diminished, but no effect was found on CD8+ T cell response to FV infections. While the viral infection did not affect the worm burden, the L. sigmodontis infection resulted in increased viral loads due to virus-specific antibody response suppression (54). According to Gopinath et al. (61), the in vivo immunological interactions of filaria-HIV co-infections could be properly modeled if cells of individuals with pre-existing filarial infections are used rather than inducing the filarial-immunity with antigens. By infecting CD8+ T cell-depleted peripheral blood mononuclear cells from filaria-infected individuals with HIV strains, an indication of increased susceptibility to HIV infections were observed (61).
Filaria-Plasmodium Co-Infection
In addition to their co-endemicity in particular regions, malaria parasites and some filarial parasites share similar transmission vectors. Several studies have explored the possible alterations in host immune responses as a result of filaria-plasmodium co-infection (62, 63). Filarial parasites are well known to induce immunosuppressive mechanisms in their human hosts by impairing the production of pro-inflammatory cytokines through the activities of Treg and related cytokines, IL-10 and TGF-β (13, 31, 64). Under the hypothesis that the induced immunosuppression by filarial alters the pro-inflammatory onset of cerebral malaria (CM), Specht et al. (52) studied the development of CM in malaria-infected-murine models with previous filaria (L. sigmodontis) infection. CD8+ T cells are key players in the progression of CM (65). In the co-infected model, CD8+ T cell sequestration into the brain was significantly reduced, which was corroborated with the observed reduction in CM pathology (52). The filaria-induced IL-10 suppresses type-1 cytokines which are known promoters of CM pathologies. In addition, IL-10 mediates the accumulation of CD8+ T cells in the spleen, thus, reducing its sequestration into the brain (52). Pre-existing filarial infections in mice models can suppress the production and functioning of CD8+ T cells induced by anti-Plasmodium vaccination, although the implementation of a heterologous prime/boost immunization regime could stop the observed interference (39).
Filaria-Mycobacteria Co-Infection
Similar to malaria, tuberculosis and filarial infections share a common endemic hotspot. Recent studies have explored the immunoregulatory effect on filaria-mycobacteria co-infections (66, 67), however, the precise influence on TB outcome and progression remains open for further investigations. The influence of filarial infections on Mycobacterium tuberculosis and Plasmodium falciparum infections have been extensively discussed elsewhere (68). Immunity in helminth infections is mostly mediated by Th2 immune responses, whereas immune responses to M. tuberculosis require type-1 cytokines (69). CD8+ T cells are known inducers of Th1 and Th17 associated cytokines, which are critical components of host immunity against TB (70). It is also well established that filarial parasites can modulate CD8+ T cells. The Mycobacterial antigen-specific CD8+ T cell responses were down-modulated by the occurrence of W. bancrofti infection in active pulmonary TB patients (53). Both CD4+ and CD8+ Th1/Th17 cytokines were diminished in the co-infected mice model, as a result of filaria-induced IL-10 modulation of the pro-inflammatory responses against the mycobacterium (53).
Filarial Infections and Sepsis
In the initial phases of septic attacks, microorganisms mount a robust pro-inflammatory immunity, a stage described as systemic inflammatory response syndrome (SIRS) (71, 72). The compensatory anti-inflammatory response syndrome (CARS) phase immediately follows, which involves an increase in anti-inflammatory molecules and apoptosis of activated immune cells with a corresponding decrease in pro-inflammatory agents (73, 74). This dampens the strength of host adaptive immunity, allowing possible infections with opportunistic microbes (74). Moreover, CD8+ T cell cytotoxicity is reduced during the CARS phase (75). Filaria parasites are known to down-modulate CD8+ T cell responses and trigger the production of anti-inflammatory cytokines like IL-10 (50). Thus, the alteration of T-cell responses during the SIRS and CARS phases in Escherichia coli-induced septic mice model with chronic L. sigmodontis infection has been recently explored (76). Interestingly, the weakening of CD8+ T cell cytotoxicity through sepsis attack was not promoted by chronic filarial infection (76).
How Filarial Parasites Could Re-Program CD8+ T Cells
The transcriptional factors that regulate CD8+ T cell phenotypes and functions are important, particularly during chronic infections (44). How filarial parasites influence the differentiation and subsequent proliferation of naive CD8+ T cells is not clear and remain to be established. However, like all other forms of CD8+ T cell activation, the presence of a suitable peptide with an MHC class I molecule drives CD8+ T cell proliferation and differentiation via cross-presentation, given that the filarial parasites are extracellular. An effective CD8+ T cell challenge has been implicated in the clearance against viral infections (77) and helminth infections (78) and similar mechanisms could be applied to filarial parasites. Given that filarial pathogens live long in their host, sustained antigen stimulation may alter the differentiation program of CD8+ T cells and render them exhausted as previously documented in other infection scenarios (77, 78). These exhausted CD8+ T cells are functionally poor since they have reduced lethal impact against their targets. In essence, this could be a possible scenario in filarial infections, where some compromises could be achieved to reduce tissue damage since filarial worms generally survive for at least 10 years in the host. Exhausted CD8+ T cells are predominant in chronic infections (77, 79).
Experimental investigation of the functional relevance of regulatory CD8+ T cells during infection has advanced, following growth in phenotypic characterization of CD8+ T cells subtypes. Activated CD8+ T cells release granzyme B, which plays a seminal role during cytotoxic functions. More recently, granzyme B in the regulatory T cell compartment has been found to mediate the suppression of antigen-specific CD8+ T cells in viral infection (77). In the same study, increased numbers of antigen-specific CD8+ T cells in the lungs of granzyme B-deficient mice were observed, suggesting that granzyme B in Tregs cells regulates either the initiation of antigen-specific CD8+ T cells responses or the down-modulation of terminal effector cells or a combination of both. Further, granzyme B has been implicated in enhancing susceptibility during the filarial nematode, L. sigmodontis infection (78). Additionally, results from field studies also suggest the suppressive role of granzyme B in human onchocerciasis (79). In this study, the authors observed a high expression of granzyme B and Foxp3 Tregs in the generalized hyporeactive than the hyperreactive individuals (79).
Although the underlying mechanisms dictating CD8+ T cell in the presence of filarial antigens is unknown, the fact that increased expression of granzyme B has been associated with immunomodulatory factors such as FoxP3 and TGF-β during filarial infections are good indicators of some immunological cross-talk with CD8+ T cell serving as a key player. Thus, we speculate a possible interplay between CD8+ T cells and granzyme B during filarial infection in a regulatory fashion among individuals with patent infection. Soluble CD8 (sCD8) released upon activation of CD8+ T cells has been documented to reflect filarial disease severity (42, 43).
The Need for CD8+ T Cell Induction by Prophylactic Filarial Vaccines
Currently, the main strategies for controlling filarial infections are through an annual mass drug administration program, management of morbidity, and vector control measures. Despite the progress, millions of individuals are still at risk of filarial infections in over 50 countries worldwide (80). Prophylactic anti-filarial vaccines, replacing or combined with drug therapies, are required to effectively eliminate filarial infections worldwide (81, 82). The search for vaccine candidates for filarial infections has been progressive over the years (reviewed in 81–83), although there are currently no approved consumable vaccines available for filariasis.
Immunization studies in animal models with filarial antigens have provided evidence supporting the efficacy of prophylactic vaccines against filarial infections (84). Several potential vaccine candidates have been identified to offer a wide variety of T cell-mediated responses as part of their protective arsenal against filarial infections in experimental models (85–89). A common observation is that multivalent and cocktail vaccine candidates offer greater protection against filarial infections than single-antigen vaccinations (85–89). This seems plausible, considering the highly complex life-cycle of filarial parasites which involves different stages of development. The majority of immunization trials against filarial infections in experimental models demonstrate induction of IL-2, IL-4, IL-10, IL-17, and IFN-γ, indicating a balanced Th1/Th2 immunity (85–90). The efficacy of these potential vaccine candidates in different animal models ranges from 45% to 94% (83). The vaccine formulations usually include filarial antigens such as heat shock protein 12.6 (86–88), abundant larval transcript-2 (86, 88, 89, 91), tetraspanin large extracellular loop (86–88), vespid allergen homologue (89, 91), thioredoxin peroxide (86), calponin (92), disorganized muscle protein-1 (93), and trehalose-6-phosphate phosphatase (35). In addition to these antigens, adjuvants are added to formulations to improve the vaccine-induced responses. The most used adjuvants for antifilaria immunization formulations are alum, tuftsin and TLR agonists (86, 94–97).
The developmental stages of filarial parasites are complex and are known to regulate several aspects of the cell-mediated immune responses mounted by the host. Emerging evidence shows that CD8+ T cells contribute to immune responses against the filaria parasites at the early stages of infection, but are highly regulated to favor parasite survival (31). Given this, a very plausible therapeutic approach would be to prime potential prophylactic vaccine candidates to induce sufficient CD8+ T cells and associated memory responses which can have detrimental effects on the establishment of the parasites (15). Identifying CD8+ T cell epitopes within filarial antigens could be achieved through immunoinformatics predictions and experimental validations or by screening a library of peptides spanning the complete antigen sequence (83). Recently, our group identified a CD8+ T cell peptide (146KPWENFMRV154) within onchocystatin, as part of a multi-epitope vaccine candidate for onchocerciasis, which demonstrates promising potential based on bioinformatics analysis (98). For most defined T cell antigens, the peptide sequences are usually similar to the corresponding sequences in related filarial parasites which may ensure cross-reaction (98–100).
To accelerate the introduction of filaria vaccines into clinical practice, there is the need to enhance the immunogenicity of potential candidates by boosting their ability to induce CD8+ T cell responses. Vaccine development against filarial infections is focused on multi-antigenic or cocktail vaccine formulations, and their cytotoxic mechanisms can be enhanced by conjugating peptides to adjuvants such as TLR ligands (101).
Concluding Remarks
The current understanding of CD8+ T cell-mediated immunity against filarial parasites suggests a type 2-dependent immunity. The CD8+ T cell cytotoxic activities appear to be effective in the early stages of filarial infections. Available evidence from animal models and human studies show that CD8+ T cells are involved in the immunomodulatory mechanisms that drive the pathogenesis of filarial infections. The mechanistic role of CD8+ T cells in filarial pathology could be associated with its poor regulation as a result of filaria-driven suppression, thus, promoting the secretion of pro-inflammatory agents. In the future, the ability to manipulate the CD8+ T cell response could have major implications, especially towards developing vaccines against filarial infections. Understanding the dynamics of the CD8+ T cells response to infections would help define manipulations that would be optimal for establishing protection against these complex extracellular nematodes. Of note, the recent advances regarding the importance of blocking T cell-derived cytokines or receptors, for instance, provide compelling evidence towards the development of potential therapeutics (102), which could be explored in filarial infections. Here, we have discussed evidence regarding the potential protection of CD8+ T cells against filarial infections as well suppression of same during co-infections; thus, suggesting a need for further studies in the future.
Author Contributions
Conceptualization: AK. Writing - Original Draft: AK, EA, and KK. Writing - Review & Editing: AK, EA, KK, and SA. All authors contributed to the article and approved the submitted version.
Conflict of Interest
The authors declare that the research was conducted in the absence of any commercial or financial relationships that could be construed as a potential conflict of interest.
Publisher’s Note
All claims expressed in this article are solely those of the authors and do not necessarily represent those of their affiliated organizations, or those of the publisher, the editors and the reviewers. Any product that may be evaluated in this article, or claim that may be made by its manufacturer, is not guaranteed or endorsed by the publisher.
Acknowledgments
We are indebted to the staff of the Kwarteng Lab at Kumasi Centre for Collaborative Research in Tropical Medicine (KCCR) for their unflinching support in the preparation of this manuscript.
References
1. Taylor MJ, Hoerauf A, Bockarie M. Lymphatic Filariasis and Onchocerciasis. Lancet (London England) (2010) 376(9747):1175–85. doi: 10.1016/S0140-6736(10)60586-7
2. Subash B, Nutman TB. Immunology of Lymphatic Filariasis. Parasit Immunol (2014) 36(8):142–9. doi: 10.1111/pim.12081
3. Herricks JR, Hotez PJ, Wanga V, Coffeng LE, Buckle G, Budke CM, et al. The Global Burden of Disease Study 2013: What Does it Mean for the NTDs? PloS Neglect Trop Dis (2017) 11:1–21. doi: 10.1371/journal.pntd.0005424
4. Hoerauf A, Specht S, Büttner M, Pfarr K, Mand S, Fimmers R, et al. Wolbachia Endobacteria Depletion by Doxycycline as Antifilarial Therapy has Macrofilaricidal Activity in Onchocerciasis: A Randomized Placebo-Controlled Study. Med Microbiol Immunol (2008) 197(3):295–311. doi: 10.1007/s00430-007-0062-1
5. Batsa Debrah L, Phillips RO, Pfarr K, Klarmann-Schulz U, Opoku VS, Nausch N, et al. The Efficacy of Doxycycline Treatment on Mansonella Perstans Infection: An Open-Label, Randomized Trial in Ghana. Am J Trop Med Hyg (2019) 101(1):84–92. doi: 10.4269/ajtmh.18-0491
6. Reimer LJ, Thomsen EK, Tisch DJ, Henry-Halldin CN, Zimmerman PA, Baea ME, et al. Insecticidal Bed Nets and Filariasis Transmission in Papua New Guinea. New Engl J Med (2013) 369(8):745–53. doi: 10.1056/NEJMoa1207594
7. Babu S, Nutman TB. Pro-Inflammatory Cytokines Dominate the Early Immune Response to Filarial Parasites. J Immunol (Baltimore Md.: 1950) (2003) 171(12):6723–32. doi: 10.4049/jimmunol.171.12.6723
8. Nelson FK, Greiner DL, Shultz LD, Rajan TV. The Immunodeficient Scid Mouse as a Model for Human Lymphatic Filariasis. J Exp Med (1991) 173:659–63. doi: 10.1084/jem.173.3.659
9. Babu S, Shultz LD, Klei TR, Rajan TV. Immunity in Experimental Murine Filariasis: Roles of T and B Cells Revisited. Infect Immun (1999) 67:3166–7. doi: 10.1128/IAI.67.6.3166-3167.1999
10. Nutman TB. Looking Beyond the Induction of Th2 Responses to Explain Immunomodulation by Helminths. Parasit Immunol (2015) 37(6):304–13. doi: 10.1111/pim.12194
11. King CL, Kumaraswami V, Poindexter RW, Kumari S, Jayaraman K, Alling DW, et al. Immunologic Tolerance in Lymphatic Filariasis: Diminished Parasite-Specific T and B Lymphocyte Precursor Frequency in the Microfilaremic State. J Clin Invest (1992) 89:1403. doi: 10.1172/JCI115729
12. Nutman TB, Kumaraswami V. Regulation of the Immune Response in Lymphatic Filariasis: Perspectives on Acute and Chronic Infection With Wuchere- Ria Bancrofti in South India. Parasit Immunol (2001) 23:389. doi: 10.1046/j.1365-3024.2001.00399.x
13. Maizels RM, Yazdanbakhsh M. Immune Regulation by Helminth Parasites: Cellular and Molecular Mechanisms. Nat Rev Immunol (2003) 3(9):733–44. doi: 10.1038/nri1183
14. Metenou S, Nutman TB. Regulatory T Cell Subsets in Filarial Infection and Their Function. Front Immunol (2013) 4:305(SEP). doi: 10.3389/fimmu.2013.00305
15. Folkard SG, Bianco AE. Roles for Both CD4+ and CD8+ T Cells in Protective Immunity Against Onchocerca Lienalis Microfilariae in the Mouse. Parasit Immunol (1995) 17(10):541–53. doi: 10.1111/j.1365-3024.1995.tb00885.x
16. Gentil K, Pearlman E. Gamma Interferon and Interleukin-1 Receptor 1 Regulate Neutrophil Recruitment to the Corneal Stroma in a Murine Model of Onchocerca Volvulus Keratitis. Infect Immun (2009) 77(4):1606–12. doi: 10.1128/IAI.00671-08
17. Gillette-Ferguson I, Daehnel K, Hise AG, Sun Y, Carlson E, Diaconu E, et al. Toll-Like Receptor 2 Regulates CXC Chemokine Production and Neutrophil Recruitment to the Cornea in Onchocerca Volvulus/Wolbachia-Induced Keratitis. Infect Immun (2007) 75(12):5908–15. doi: 10.1128/IAI.00991-07
18. Hise AG, Daehnel K, Gillette-Ferguson I, Cho E, McGarry HF, Taylor MJ, et al. Innate Immune Responses to Endosymbiotic Wolbachia Bacteria in Brugia Malayi and Onchocerca Volvulus Are Dependent on TLR2, TLR6, MyD88, and Mal, But Not TLR4, TRIF, or TRAM. J Immunol (Baltimore Md.: 1950) (2007) 178(2):1068–76. doi: 10.4049/jimmunol.178.2.1068
19. Daehnel K, Gillette-Ferguson I, Hise AG, Diaconu E, Harling MJ, Heinzel FP, et al. Filaria/Wolbachia Activation of Dendritic Cells and Development of Th1-Associated Responses Is Dependent on Toll-Like Receptor 2 in a Mouse Model of Ocular Onchocerciasis (River Blindness). Parasit Immunol (2007) 29:455–65. doi: 10.1111/j.1365-3024.2007.00962.x
20. Gillette-Ferguson I, Hise AG, Sun Y, Diaconu E, McGarry HF, Taylor MJ, et al. Wolbachia- and Onchocerca Volvulus-Induced Keratitis (River Blindness) Is Dependent on Myeloid Differentiation Factor 88. Infect Immun (2006) 74:2442–5. doi: 10.1128/IAI.74.4.2442-2445.2006
21. Mukherjee S, Mukherjee S, Maiti TK, Bhattacharya S, Babu SPS. A Novel Ligand of Toll-Like Receptor 4 From the Sheath of Wuchereria Bancrofti Microfilaria Induces Pro-Inflammatory Response in Macrophages. J Infect Dis (2017) 215(6):954–65. doi: 10.1093/infdis/jix067
22. Mukherjee S, Karnam A, Das M, Babu SPS, Bayry J. Wuchereria Bancrofti Fi Laria Activates Human. Commun Biol (2019) 2:169. doi: 10.1038/s42003-019-0392-8
23. Wammes LJ, Hamid F, Wiria AE, Wibowo H, Sartono E, Maizels RM, et al. Regulatory T Cells in Human Lymphatic Filariasis: Stronger Functional Activity in Microfilaremics. PloS Negl Trop Dis (2012) 6:e1655. doi: 10.1371/journal.pntd.0001655
24. Stephen-Victor E, Karnam A, Fontaine T, Beauvais A, Das M, Hegde P, et al. Aspergillus Fumigatus Cell Wall α-(1,3)-Glucan Stimulates Regulatory T-Cell Polarization by Inducing PD-L1 Expression on Human Dendritic Cells. J Infect Dis (2017) 216:1281–94. doi: 10.1093/infdis/jix469
25. Pathak M, Verma M, Srivastava M, Misra-Bhattacharya S. Wolbachia Endosymbiont of Brugia Malayi Elicits Th-17 Mediated Pro-Inflammatory Immune Response Through Sur- Face Protein (WSP). Immunol (2014) 144:231–44. doi: 10.1111/imm.12364
26. Pathak M, Sharma P, Sharma A, Verma M, Srivastava M, Misra-Bhattacharya S. Regulatory T-Cell Neutralization in Mice During Filariasis Helps in Parasite Clearance by Enhancing T Helper Type 17-Mediated Pro-Inflammatory Response. Immunol (2016) 147(2):190–203. doi: 10.1111/imm.12550
27. Kalinkovich A, Weisman Z, Greenberg Z, Nahmias J, Eitan S, Stein M, et al. Decreased CD4 and Increased CD8 Counts With T Cell Activation Is Associated With Chronic Helminth Infection. Clin Exp Immunol (1998) 114(3):414–21. doi: 10.1046/j.1365-2249.1998.00736.x
28. Kroidl I, Chachage M, Mnkai J, Nsojo A, Berninghoff M, Verweij JJ, et al. Wuchereria Bancrofti Infection Is Linked to Systemic Activation of CD4 and CD8 T Cells. PloS Neglect Trop Dis (2019) 13(8):1–17. doi: 10.1371/journal.pntd.0007623
29. Chan CC, Ottesen EA, Awadzi K, Badu R, Nussenblatt RB. Immunopathology of Ocular Onchocerciasis. I. Inflammatory Cells Infiltrating the Anterior Segment. Clin Exp Immunol (1989) 77(3):367–72.
30. McKechnie NM, Gürr W, Yamada H, Copland D, Braun G. Antigenic Mimicry: Onchocerca Volvulus Antigen-Specific T Cells and Ocular Inflammation. Invest Ophthalmol Visual Sci (2002) 43(2):411–8.
31. Anuradha R, George PJ, Kumaran P, Nutman TB, Babu S. Interleukin-10- and Transforming Growth Factor β-Independent Regulation of CD8+ T Cells Expressing Type 1 and Type 2 Cytokines in Human Lymphatic Filariasis. Clin and Vaccine Immunol: CVI (2014) 21(12):1620–7. doi: 10.1128/CVI.00598-14
32. Anuradha R, George PJ, Hanna LE, Kumaran P, Chandrasekaran V, Nutman TB, et al. Expansion of Parasite-Specific CD4+ and CD8+ T Cells Expressing IL-10 Superfamily Cytokine Members and Their Regulation in Human Lymphatic Filariasis. PloS Neglect Trop Dis (2014) 8(4):e2762. doi: 10.1371/journal.pntd.0002762
33. King CL, Mahanty S, Kumaraswami V, Abrams JS, Regunathan J, Jayaraman K, et al. Cytokine Control of Par- Asite-Specific Anergy in Human Lymphatic Filariasis: Preferential Induction Ofa Regulatory T Helper Type 2 Lymphocyte Subset. J Clin Invest (1993) 92:1667– 1673. doi: 10.1172/JCI116752
34. Rajan ATV, Nelson FK, Shultz LD, Koller BH, Dale L, Journal T. CD8 + T Lymphocytes Are Not Required for Murine Resistance to Human Filarial Parasites Published by: Allen Press on Behalf of American Society of Parasitologists Stable (1992). Available at: http://www.jstor.org/stable/3283557 (Accessed Accessed: 02-03-2016 09).
35. Kushwaha S, Singh PK, Gupta J, Soni VK, Misra-Bhattacharya S. Recombinant Trehalose-6-Phosphate Phosphatase of Brugia Malayi Cross-Reacts With Human Wuchereria Bancrofti Immune Sera and Engenders a Robust Protective Outcome in Mice. Microbes Infect (2012) 14(14):1330–9. doi: 10.1016/j.micinf.2012.08.006
36. Gangwar M, Jha R, Goyal M, Srivastava M. Immunogenicity and Protective Efficacy of Recombinase A From Wolbachia Endosymbiont of Filarial Nematode Brugia Malayi (Wbmreca). Vaccine (2019) 37(4):571–80. doi: 10.1016/j.vaccine.2018.12.015
37. Hartmann W, Brenz Y, Kingsley MT, Ajonina-Ekoti I, Brattig NW, Liebau E, et al. Nematode-Derived Proteins Suppress Proliferation and Cytokine Production of Antigen-Specific T Cells via Induction of Cell Death. PloS One (2013) 8(6):e68380. doi: 10.1371/journal.pone.0068380
38. Gupta J, Pathak M, Misra S, Misra-Bhattacharya S. Immunogenicity and Protective Efficacy of Brugia Malayi Heavy Chain Myosin as Homologous DNA, Protein and Heterologous DNA/Protein Prime Boost Vaccine in Rodent Model. PloS One (2015) 10(11):e0142548. doi: 10.1371/journal.pone.0142548
39. Kolbaum J, Tartz S, Hartmann W, Helm S, Nagel A, Heussler V, et al. Nematode-Induced Interference With the Anti-Plasmodium CD8+ T-Cell Response Can Be Overcome by Optimizing Antigen Administration. Eur J Immunol (2012) 42(4):890–900. doi: 10.1002/eji.201141955
40. Mukhopadhyay S, Mohanty M, Mangla A, George A, Bal V, Rath S, et al. Macrophage Effector Func- Tions Controlled by Bruton’s Tyrosine Kinase Are More Crucial Than the Cyto- Kine Balance of T Cell Responses for Microfilarial Clearance. J Immunol (2002) 168:2914–21. doi: 10.4049/jimmunol.168.6.2914
41. Allen JE, Loke P. Divergent Roles for Macrophages in Lymphatic Filariasis. Parasit Immunol (2001) 23:345–52. doi: 10.1046/j.1365-3024.2001.00394.x
42. Lal RB, Kumaraswami V, Krishnan N, Nutman TB, Ottesen EA. Lymphocyte Subpopulations in Bancroftian Filariasis: Activated (DR+) CD8+ T Cells in Patients With Chronic Lymphatic Obstruction. Clin Exp Immunol (1989) 77(1):77–82.
43. Lal RB, Ramzy RM, Gad AA. Elevated Levels of Soluble CD8 Molecule in Patients With Lymphatic Filariasis. Immunol Lett (1990) 26(1):85–8. doi: 10.1016/0165-2478(90)90180-x
44. Steel C, Varma S, Nutman TB. Regulation of Global Gene Expression in Human Loa Loa Infection Is a Function of Chronicity. PloS Neglect Trop Dis (2012) 6(2):e1527. doi: 10.1371/journal.pntd.0001527
45. Dennis VA, Osae-Addo G, Lowrie RCJ. Blastogenic Responses, Interleukin-2 Production and Interleukin-2 Receptor Expression on CD4+ and CD8+ Lymphocytes in Rhesus Monkeys Experimentally Inoculated With Loa Loa. Parasit Immunol (1997) 19(7):301–8. doi: 10.1046/j.1365-3024.1997.d01-212.x
46. Ghosh RP, Murthy PK, Tyagi K, Murthy PS, Chatterjee RK. Longitudinal Cellular Immune Responses in Asymptomatic and Symptomatic Brugia Malayi-Infected Indian Leaf Monkey Presbytis Entellus. J Parasitol (1999) 85(5):861–6. doi: 10.2307/3285822
47. MacMicking J, Xie QW, Nathan C. Nitric Oxide and Macrophage Function. Annu Rev Immunol (1997) 15:323–50. doi: 10.1146/annurev.immunol.15.1.323
48. Numasaki M, Lotze MT, Sasaki H. Interleukin-17 Augments Tumor Necrosis Factor-␣-Induced Elaboration of Proangiogenic Factors From Fibro- Blasts. Immunol Lett (2004) 93:39–43. doi: 10.1016/j.imlet.2004.01.014
49. Wynn TA. Common and Unique Mechanisms Regulate Fibrosis in Various Fibroproliferative Diseases. J Clin Invest (2007) 117:524–9. doi: 10.1172/JCI31487
50. Maizels RM, Balic A, Gomez-Escobar N, Nair M, Taylor MD, Allen JE. Helminth Parasites - Masters of Regulation. Immunol Rev (2004) 201:89–116. doi: 10.1111/j.0105-2896.2004.00191.x
51. Zaccone P, Cooke A. Vaccine Against Autoimmune Disease: Can Helminths or Their Products Provide a Therapy? Curr Opin Immunol (2013) 25(3):418–23. doi: 10.1016/j.coi.2013.02.006
52. Specht S, Ruiz DF, Dubben B, Deininger S, Hoerauf A. Filaria-Induced IL-10 Suppresses Murine Cerebral Malaria. Microbes Infect (2010) 12(8–9):635–42. doi: 10.1016/j.micinf.2010.04.006
53. George PJ, Anuradha R, Kumar NP, Sridhar R, Banurekha VV, Nutman TB, et al. Helminth Infections Coincident With Active Pulmonary Tuberculosis Inhibit Mono- and Multifunctional CD4+ and CD8+ T Cell Responses in a Process Dependent on IL-10. PloS Pathog (2014) 10(9):e1004375. doi: 10.1371/journal.ppat.1004375
54. Dietze KK, Dittmer U, Koudaimi DK, Schimmer S, Reitz M, Breloer M, et al. Filariae-Retrovirus Co-Infection in Mice Is Associated With Suppressed Virus-Specific IgG Immune Response and Higher Viral Loads. PloS Neglect Trop Dis (2016) 10(12):e0005170. doi: 10.1371/journal.pntd.0005170
55. Dittrich AM, Erbacher A, Specht S, Diesner F, Krokowski M, Avagyan A, et al. Helminth Infection With Litomosoides Sigmodontis Induces Regulatory T Cells and Inhibits Allergic Sensitization, Airway Inflammation, and Hyperreactivity in a Murine Asthma Model. J Immunol (2008) 180(3):1792–9. doi: 10.4049/jimmunol.180.3.1792
56. Mueller SN, Rouse BT. Immune Responses to Viruses. In Clinical Immunology (Third Edition) eds. Rich RR, et al, Moby (2008). pp. 421–31. doi: 10.1016/B978-0-323-04404-2.10027-2
57. Actor JK, Shirai M, Kullberg MC, Buller RML, Sher A, Berzofsky JA. Helminth Infection Results in Decreased Virus-Specific CD8+ Cytotoxic T- Cell and Th1 Cytokine Responses as Well as Delayed Virus Clearance. Proc Natl Acad Sci USA (1993) 90(3):948–52. doi: 10.1073/pnas.90.3.948
58. Osborne LC, Monticelli LA, Nice TJ, Sutherland TE, Siracusa MC, Hepworth MR, et al. Virus-Helminthcoinfection Reveals a Microbiota-Independent Mechanism of Immunomodulation. Science (2014) 345(6196):578–82. doi: 10.1126/science.1256942
59. Nielsen NO, Friis H, Magnussen P, Krarup H, Magesa S, Simonsen PE. Co-Infection With Subclinical HIV and Wuchereria Bancrofti, and the Role of Malaria and Hookworms, in Adult Tanzanians: Infection Intensities, CD4/CD8 Counts and Cytokine Responses. Trans R Soc Trop Med Hyg (2007) 101(6):602–12. doi: 10.1016/j.trstmh.2007.02.009
60. Mouser EEIM, Pollakis G, Yazdanbakhsh M, Harnett W, de Jong EC, Paxton WA. Brugia Malayi Antigen (BmA) Inhibits HIV-1 Trans-Infection But Neither BmA Nor ES-62 Alter HIV-1 Infectivity of DC Induced CD4+ Th-Cells. PloS One (2016) 11(1):e0146527. doi: 10.1371/journal.pone.0146527
61. Gopinath R, Ostrowski M, Justement SJ, Fauci AS, Nutman TB. Filarial Infections Increase Susceptibility to Human Immunodeficiency Virus Infection in Peripheral Blood Mononuclear Cells In Vitro. J Infect Dis (2000) 182(6):1804–8. doi: 10.1086/317623
62. Aliota MT, Chen C-C, Dagoro H, Fuchs JF, Christensen BM. Filarial Worms Reduce Plasmodium Infectivity in Mosquitoes. PloS Neglect Trop Dis (2011) 5(2):e963. doi: 10.1371/journal.pntd.0000963
63. Graham AL, Lamb TJ, Read AF, Allen JE. Malaria-Filaria Coinfection in Mice Makes Malarial Disease More Severe Unless Filarial Infection Achieves Patency. J Infect Dis (2005) 191(3):410–21. doi: 10.1086/426871
64. Specht S, Volkmann L, Wynn T, Hoerauf A. Interleukin-10 (IL-10) Counterregulates IL-4-Dependent Effector Mechanisms in Murine Filariasis. Infect Immun (2004) 72(11):6287–93. doi: 10.1128/IAI.72.11.6287-6293.2004
65. Belnoue E, Kayibanda M, Vigario AM, Deschemin J-C, Rooijen N, Viguier M, et al. On the Pathogenic Role of Brain-Sequestered αβ CD8 + T Cells in Experimental Cerebral Malaria. J Immunol (2002) 169(11):6369–75. doi: 10.4049/jimmunol.169.11.6369
66. Chatterjee S, Kolappan C, Subramani R, Gopi PG, Chandrasekaran V, Fay MP, et al. Incidence of Active Pulmonary Tuberculosis in Patients With Coincident Filarial and/or Intestinal Helminth Infections Followed Longitudinally in South India. PloS One (2014) 9(4):e94603. doi: 10.1371/journal.pone.0094603
67. Hübner MP, Killoran KE, Rajnik M, Wilson S, Yim KC, Torrero MN, et al. Chronic Helminth Infection Does Not Exacerbate Mycobacterium Tuberculosis Infection. PloS Neglect Trop Dis (2012) 6(12):e1970. doi: 10.1371/journal.pntd.0001970
68. Metenou S, Babu S, Nutman TB. Impact of Filarial Infections on Coincident Intracellular Pathogens: Mycobacterium Tuberculosis and Plasmodium Falciparum. Curr Opin HIV AIDS (2012) 7(3):231–8. doi: 10.1097/COH.0b013e3283522c3d
69. Salgame P. Host Innate and Th1 Responses and the Bacterial Factors That Control Mycobacterium Tuberculosis Infection. Curr Opin Immunol (2005) 17(4):374–80. doi: 10.1016/j.coi.2005.06.006
70. O’Garra A, Redford PS, McNab FW, Bloom CI, Wilkinson RJ, Berry MPR. The Immune Response in Tuberculosis. Annu Rev Immunol (2013) 31:475–527. doi: 10.1146/annurev-immunol-032712-095939
71. Levy MM, Fink MP, Marshall JC, Abraham E, Angus D, Cook D, et al. 2001 SCCM/ESICM/ACCP/ATS/SIS International Sepsis Definitions Conference. Crit Care Med (2003) 31(4):1250–6. doi: 10.1097/01.CCM.0000050454.01978.3B
72. Bone RC, Sibbald WJ, Sprung CL. The ACCP-SCCM Consensus Conference on Sepsis and Organ Failure. Chest (1992) 101(6):1481–3. doi: 10.1378/chest.101.6.1481
73. Roger PM, Hyvernat H, Breittmayer JP, Dunais B, Dellamonica J, Bernardin G, et al. Enhanced T-Cell Apoptosis in Human Septic Shock Is Associated With Alteration of the Costimulatory Pathway. Eur J Clin Microbiol Infect Dis (2009) 28(6):575–84. doi: 10.1007/s10096-008-0673-5
74. Hotchkiss RS, Opal S. Immunotherapy for Sepsis — A New Approach Against an Ancient Foe. New Engl J Med (2010) 363(1):87–9. doi: 10.1056/nejmcibr1004371
75. Schwandt T, Schumak B, Gielen GH, Jüngerkes F, Schmidbauer P, Klocke K, et al. Expression of Type I Interferon by Splenic Macrophages Suppresses Adaptive Immunity During Sepsis. EMBO J (2012) 31(1):201–13. doi: 10.1038/emboj.2011.380
76. Buerfent BC, Gondorf F, Wohlleber D, Schumak B, Hoerauf A, Hübner MP. Escherichia Coli-Induced Immune Paralysis is Not Exacerbated During Chronic Filarial Infection. Immunology (2015) 145(1):150–60. doi: 10.1111/imm.12435
77. Salti SM, Hammelev EM, Grewal JL, Reddy ST, Zemple SJ, Grossman WJ, et al. Granzyme B Regulates Antiviral CD8+ T Cell Responses. J Immunol (Baltimore Md.: 1950) (2011) 187(12):6301–9. doi: 10.4049/jimmunol.1100891
78. Hartmann W, Marsland BJ, Otto B, Urny J, Fleischer B, Korten S. A Novel and Divergent Role of Granzyme A and B in Resistance to Helminth Infection. J Immunol (Baltimore Md.: 1950) (2011) 186(4):2472–81. doi: 10.4049/jimmunol.0902157
79. Yamamoto N, Watanabe M, Matsuzuka F, Miyauchi A, Iwatani Y. Lower Concentration of Serum Soluble CD8 in Severe Hashimoto’s Disease. Clin Exp Immunol (2004) 137(3):601–5. doi: 10.1111/j.1365-2249.2004.02576.x
80. Ichimori K, King JD, Engels D, Yajima A, Mikhailov A, Lammie P, et al. Global Programme to Eliminate Lymphatic Filariasis: The Processes Underlying Programme Success. PloS Negl Trop Dis (2014) 8:e3328. doi: 10.1371/journal.pntd.0003328
81. Babayan SA, Allen JE, Taylor DW. Future Prospects and Challenges of Vaccines Against Filariasis. Parasit Immunol (2012) 34:243–53. doi: 10.1111/j.1365-3024.2011.01350.x
82. Morris CP, Evans H, Larsen SE, Mitre E. A Comprehensive, Model- Based Review of Vaccine and Repeat Infection Trials for Filariasis. Clin Microbiol Rev (2013) 26:381–421. doi: 10.1128/CMR.00002-13
83. Kalyanasundaram R, Khatri V, Chauhan N. Advances in Vaccine Development for Human Lymphatic Filariasis. Trends Parasitol (2020) 36(2):195–205. doi: 10.1016/j.pt.2019.11.005
84. Cho-Ngwa F, Liu J, Lustigman S. The Onchocerca Volvulus Cysteine Proteinase Inhibitor, Ov-CPI-2, Is a Target of Protective Antibody Response That Increases With Age. PloS Neglect Trop Dis (2010) 4(8):2–9. doi: 10.1371/journal.pntd.0000800
85. Anugraha G, Madhumathi J, Prince PR, Jeya Prita PJ, Khatri VK, Amdare NP, et al. Chimeric Epitope Vaccine From Multistage Antigens for Lymphatic Filariasis. Scand J Immunol (2015) 82(4):380–9. doi: 10.1111/sji.12340
86. Chauhan N, Khatri V, Banerjee P, Kalyanasundaram R. Evaluating the Vaccine Potential of a Tetravalent Fusion Protein (Rbmhaxt) Vaccine Antigen Against Lymphatic Filariasis in a Mouse Model. Front Immunol (2018) 9:1520(July 2018). doi: 10.3389/fimmu.2018.01520
87. Dakshinamoorthy G, Munirathinam G, Stoicescu K, Reddy MV, Kalyanasundaram R. Large Extracellular Loop of Tetraspanin as a Potential Vaccine Candidate for Filariasis. PloS One (2013) 8(10):1–12. doi: 10.1371/journal.pone.0077394
88. Dakshinamoorthy G, Samykutty AK, Munirathinam G, Reddy MV, Kalyanasundaram R. Multivalent Fusion Protein Vaccine for Lymphatic Filariasis. Vaccine (2013) 31(12):1616–22. doi: 10.1016/j.vaccine.2012.09.055
89. Kalyanasundaram R, Vittel P. Multivalent Vaccine Formulation With BmVAL-1 and BmALT-2 Confer Significant Protection Against Challenge Infections With Brugia Malayi in Mice and Jirds. Res Rep Trop Med (2011) 45:45–56. doi: 10.2147/rrtm.s13679
90. Singh PK, Kushwaha S, Rana AK, Misra-Bhattacharya S. Cofactor Indepen- Dent Phosphoglycerate Mutase of Brugia Malayi Induces a Mixed Th1/Th2 Type Immune Response and Inhibits Larval Development in the Host. BioMed Res Int (2014) 2014:1–19. doi: 10.1155/2014/590281
91. Anand SB, Kodumudi KN, Reddy MV, Kaliraj P. A Combination of Two Brugia Malayi Filarial Vaccine Candidate Antigens (BmALT-2 and BmVAH) Enhances Immune Responses and Protection in Jirds. J Helminthol (2011) 85(4):442–52. doi: 10.1017/S0022149X10000799
92. Verma SK, Arora A, Murthy PK. Recombinant Calponin of Human Filariid Brugia Malayi: Secondary Structure and Immunoprophylactic Potential. Vaccine (2017) 35(38):5201–8. doi: 10.1016/j.vaccine.2017.07.105
93. Kushwaha V, Kumar V, Verma SK, Sharma R, Siddiqi MI, Murthy PK. Disorganized Muscle Protein-1 (DIM-1) of Filarial Parasite Brugia Malayi: CDNA Cloning, Expression, Purification, Structural Modeling and its Potential as Vaccine Candidate for Human Filarial Infection. Vaccine (2014) 32(15):1693–9. doi: 10.1016/j.vaccine.2014.01.064
94. Khatri V, Chauhan N, Vishnoi K, von Gegerfelt A, Gittens C, Kalyanasundaram R. Prospects of Developing a Prophylactic Vaccine Against Human Lymphatic Filariasis - Evaluation of Protection in Non-Human Primates. Int J Parasitol (2018) 48(9–10):773–83. doi: 10.1016/j.ijpara.2018.04.002
95. Dakshinamoorthy G, Von Gegerfelt A, Andersen H, Lewis M, Kalyanasundaram R. Evaluation of a Multivalent Vaccine Against Lymphatic Filariasis in Rhesus Macaque Model. PloS One (2014) 9(11):1–14. doi: 10.1371/journal.pone.0112982
96. Paul R, Karthik S, Vimalraj P, Meenakshisundaram S, Kaliraj P. Cloning, Large-Scale Production and Characterization of Fusion Protein (P-TUFT-ALT- 2) of Brugian Abundant Larval Transcript-2 With Tuftsin in Pichia Pastoris. Prep Biochem Biotechnol (2018) 48:823–33. doi: 10.1080/10826068.2018.1514511
97. Chauhan N, Banerjee P, Khatri VK, Canciamille A, Gilles J, Kalyanasundaram R. Improving the Efficacy of a Prophylactic Vaccine Formulation Against Lymphatic Filariasis. Parasitol Res (2017) 116:2821–30. doi: 10.1007/s00436-017-5593-9
98. Kwarteng A, Asiedu E, Mubarik Y, Katawa G, Asiedu SO. Exploring Onchocerca Volvulus Cysteine Protease Inhibitor for Multi-Epitope Subunit Vaccine Against Onchocerciasis: An Immunoinformatics Approach. Int J Pept Res Ther (2021) 27:1953–66. doi: 10.1007/s10989-021-10224-w
99. Lagatie O, Verheyen A, Van Dorst B, Batsa Debrah L, Debrah A, Stuyver LJ. Linear Epitopes in Onchocerca Volvulus Vaccine Candidate Proteins and Excretory-Secretory Proteins. Parasit Immunol (2018) 40(11):1–8. doi: 10.1111/pim.12587
100. Shey RA, Ghogomu SM, Esoh KK, Nebangwa ND, Shintouo CM, Nongley NF, et al. In-Silico Design of a Multi-Epitope Vaccine Candidate Against Onchocerciasis and Related Filarial Diseases. Sci Rep (2019) 9(1):1–18. doi: 10.1038/s41598-019-40833-x
101. Lei Y, Zhao F, Shao J, Li Y, Li S, Chang H, et al. Application of Built-in Adjuvants for Epitope-Based Vaccines. PeerJ (2019) 2019(1):1–48. doi: 10.7717/peerj.6185
Keywords: anti-filarial immunity, T cell, CD8+, filarial co-infections, filarial pathology
Citation: Kwarteng A, Asiedu E, Koranteng KK and Asiedu SO (2021) Highlighting the Relevance of CD8+ T Cells in Filarial Infections. Front. Immunol. 12:714052. doi: 10.3389/fimmu.2021.714052
Received: 24 May 2021; Accepted: 31 August 2021;
Published: 16 September 2021.
Edited by:
Manuel Elkin Patarroyo, Colombian Institute of Immunology Foundation, ColombiaReviewed by:
Lucia Elena Alvarado-Arnez, Franz Tamayo University, BoliviaSuprabhat Mukherjee, Kazi Nazrul University, India
Gaurav Gupta, NIIT University, India
Kuladip Jana Jana, Bose Institute, India
Copyright © 2021 Kwarteng, Asiedu, Koranteng and Asiedu. This is an open-access article distributed under the terms of the Creative Commons Attribution License (CC BY). The use, distribution or reproduction in other forums is permitted, provided the original author(s) and the copyright owner(s) are credited and that the original publication in this journal is cited, in accordance with accepted academic practice. No use, distribution or reproduction is permitted which does not comply with these terms.
*Correspondence: Alexander Kwarteng, YWt3YXJ0ZW5nQGtudXN0LmVkdS5naA==