- 1Cincinnati Children's Hospital Medical Center, Cancer and Blood Diseases Institute, Cincinnati, OH, United States
- 2Division of Immunobiology, Cincinnati Children's Hospital Research Foundation, Cincinnati, OH, United States
- 3Department of Pediatrics, University of Cincinnati College of Medicine, Cincinnati, OH, United States
In cancer, immune exhaustion contributes to the immunosuppressive tumor microenvironment. Exhausted immune cells demonstrate poor effector function and sustained expression of certain immunomodulatory receptors, which can be therapeutically targeted. CD244 is a Signaling Lymphocyte Activation Molecule (SLAM) family immunoregulatory receptor found on many immune cell types—including NK cells, a subset of T cells, DCs, and MDSCs—that represents a potential therapeutic target. Here, we discuss the role of CD244 in tumor-mediated immune cell regulation.
Introduction
In cancer, immune exhaustion contributes to the immunosuppressive tumor microenvironment. Immune exhaustion is defined by poor effector function with decreased pro-inflammatory cytokine production and diminished cytolytic activity caused by soluble and membrane-associated stimuli derived from tumor and immune cells. In the last decade, research into mechanisms underlying immune exhaustion, particularly in T cells, has revealed a variety of immunomodulatory receptors whose sustained expression is associated with chronic infection and cancer. Examples include Programmed cell Death protein-1 (PD-1) and its ligands (PD-L1 and PD-L2), Cytotoxic T-lymphocyte- Associated protein-4 (CTLA-4), Lymphocyte-activation Gene-3 (LAG-3), T-cell Immunoglobulin and Mucin domain-3 (TIM-3), and CD160. Recently, therapeutic checkpoint inhibitors that target PD-1, PD-L1, and CTLA-4 have been used successfully in a variety of cancers to ameliorate immune exhaustion and improve patient outcomes. However, treatment responses remain suboptimal for many patients and further targets for immunotherapy are needed.
CD244 is an immunoregulatory transmembrane receptor in the Signaling Lymphocyte Activation Molecule (SLAM) family that offers a potential target for immunotherapy. CD244 expression has been demonstrated on natural killer (NK) cells, γδ T cells, basophils, monocytes, a subset of CD8+ αβ T cells, dendritic cells (DC), and myeloid-derived suppressor cells (MDSC) (1–7). After early studies established that CD244 expression on these cell types is altered under specific pathologic conditions, more recent research has linked CD244 inhibitory signaling to the maintenance of an exhausted phenotype in NK cells and T cells in chronic infection and cancer (8–11). However, knowledge of CD244 signaling pathways still derives largely from NK cell studies, although expression of CD244 adaptor molecules differs between cell types and under various conditions (see Specific adaptor molecules) (12). Thus, further investigations are needed to elucidate CD244 signaling mechanisms in the broad repertoire of immune cells on which the receptor is found. In addition, more studies are needed to delineate the specific functions of CD244 signaling in tumor-associated immunosuppression. Here, we discuss the role of the CD244 receptor in tumor-mediated immune cell regulation.
CD244 Receptor
CD244 (2B4) was first identified on mouse NK cells and a subset of T cells that mediated non-MHC-restricted cytotoxicity.(1) Subsequently, the structures of mouse and human CD244 were elucidated, the receptor's ligand was identified as CD48, and initial observations regarding CD244 functionality were reported. Because CD244 is expressed on all NK cells (13), its signaling mechanisms have been best elucidated in these lymphocytes, although recent work suggests parallel mechanisms in other cell types (5, 6, 11, 14, 15). The following sections review the structure of CD244 and its signaling mechanisms.
CD244 Structure
CD244 is an Ig Superfamily Signaling Lymphocyte Activation Molecule (SLAM) family receptor. Like all SLAM family receptors, it is a transmembrane receptor comprised of an extracellular segment with two immunoglobulin (Ig)-like domains, a transmembrane region, and a cytoplasmic domain containing tyrosine-based motifs. Unlike other SLAM family receptors, it does not act as a self-ligand; instead, it binds CD48, a transmembrane receptor ubiquitously expressed on hematopoietic cells (16–18). Its cytoplasmic domain includes four Immunoreceptor Tyrosine-based Switch Motifs (ITSMs) that interact with a variety of specific adaptor molecules and are capable of propagating both inhibitory and activating signals (19, 20).
In mice, two isoforms of CD244 are expressed via alternative splicing: a long isoform with four ITSMs and a short isoform with only one ITSM (13, 21). In humans, two isoforms of CD244 are also expressed via differential splicing of hnRNA, but both human isoforms have identical intracellular domains with four ITSMs (22). Structurally, the human isoforms differ extracellularly by the presence or absence of five amino acids between the immunoglobulin V and C2 domains. Functionally, the shorter human isoform has increased affinity for CD48, and its engagement results in increased calcium flux and increased NK-cell mediated cytotoxicity in vitro (23).
Specific Adaptor Molecules
The ITSMs of human and murine CD244 bind Src homology 2 (SH2) domain-containing proteins, including SLAM-associated protein (SAP), associated with activating signaling (24), and Ewing sarcoma-activated transcript 2 (EAT2) (25), associated with activating and inhibitory signaling (26, 27), and phosphatases SHP1 (28), SHP2 (29), and SHIP-1 (30), associated with inhibitory signaling (Figure 1). In mice only, the EAT-2-related transducer (ERT) also binds CD244 ITSMs (25). In human NK cells, the c-Src kinase (Csk) binds the second and third ITSMs (31). CD244 signaling studies in other immune cell types, which express different levels of these adaptor molecules, are lacking. Based on NK cell studies, it is thought that adaptor molecule expression levels, availability, and competitive binding determine whether CD244 propagates an activating or inhibitory signal (8, 24–27, 31–38).
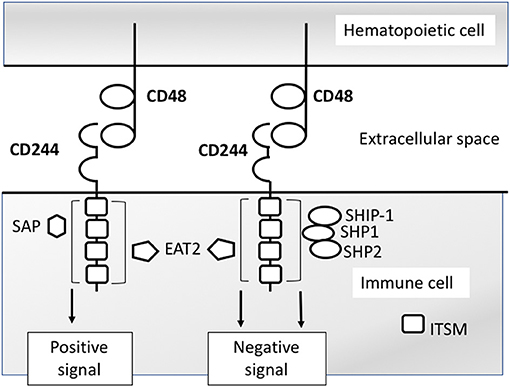
Figure 1. CD244 signaling model based on NK cell studies. CD244 binds CD48 with high affinity. Intracellular signaling is propagated via interactions with any of several SH2 domain- containing signaling molecules. Interactions with SAP (SH2D1A) propagate activating signals in NK cells. Interactions with SH2 phosphatases SHP1,SHP2, SHIP-1 propagate inhibitory signals in NK cells. Interactions with EAT2 (SH2D1B) have been shown to propagate both activating and inhibitors signals in separate studies.
CD244 can mediate activating signals in NK cells in the presence of adequate concentrations of functional SAP (24) (Figure 1). In the absence of functional SAP, CD244 is unable to initiate activating signals in mouse and human NK cells (32, 33). When SAP is unavailable for recruitment, CD244 instead recruits phosphatases (e.g., : SHP-1, SHP-2), which leads to the propagatation of inhibitory signals (8, 34–37). In subsequent investigations, specific adaptor molecule EAT-2 was also found to produce inhibitory signaling upon binding with CD244 in C57BL/6 mouse NK cells, reflected by decreased production of IFN-γ and reduced killing of targets (27). However, a later set of experiments demonstrated that C57BL/6 mouse EAT-2A−/− and EAT-2A−/B− NK cells lose CD244-specific cytotoxicity and IFNγ production compared with WT NK cells, providing evidence for an activating role (26). Of note, the initial study demonstrating inhibitory function of EAT-2 in NK cells did not demonstrate the effect of EAT-2 deficiency on CD244-CD48 mediated signaling specifically, whereas the later study demonstrated a CD244-CD48 specific effect. Comparing the function of SAP and EAT-2 in CD244 signaling, SAP is able to bind both non-phosphorylated and phosphorylated ITSMs, while EAT-2 only binds phosphorylated SLAM family ITSMs (25), which may limit the contribution of EAT-2 to the determination of activating versus inhibitory CD244 signaling. For example, in the presence of SAP, the association of inhibitory adaptor molecule SHP-2 is decreased, while EAT-2 partially inhibits the binding of SHP-2, but to a lesser degree than SAP (25, 38). Likewise, in humans, the association of CD244 with SHP-2 and SAP in transfected NK cells is mutually exclusive (38).
A mechanistic model demonstrating inhibitory signaling by CD244 in human NK cells showed that while the first, second, and fourth ITSMs of CD244 activate NK-mediated cytotoxicity by binding SAP, the third ITSM was able to bind phosphatases SHP-1, SHP-2, SHIP, and Csk, and inhibit NK cytotoxicity (31). However, only one molecule associates with the ITSM at a time, and the presence of SAP prevented binding of these phosphatases. This competitive interaction makes SAP essential to the regulation of activating versus inhibitory signaling from CD244 in human NK cells.
CD244 Expression Levels and Signal Outcome
CD244 expression is altered on different cell types under various physiologic and pathologic conditions (discussed in later sections). Alterations in the level of CD244 expression and the degree of CD244-CD48 ligation appear to contribute to determination of activating versus inhibitory signaling. CD244 has been shown in vitro to produce an activating function in murine NK cells when expressed at low surface levels, and an inhibitory function when expressed at high levels (39), although the pathway leading to increases in CD244 expression has not been determined. The inhibitory function can be overcome when fewer CD244 molecules are engaged or when SAP is over-expressed in transfectants expressing high surface concentrations of CD244 (39). This suggests that the CD244-to-SAP ratio is crucial in determining whether CD244 binding propagates an activating or inhibitory signal (Figure 2). The role that relative concentrations of the other CD244-associated adaptor molecules may play in determining signal type has not yet been elucidated.
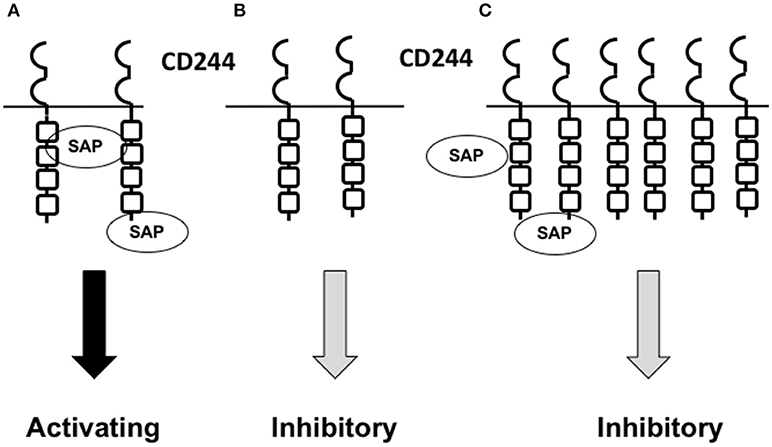
Figure 2. Model showing how the relative concentrations of CD244 and SAP may contribute to the determination of activating versus inhibitory CD244 signaling. (A) Under normal physiologic conditions, NK cells and CD8+ T cells express CD244 at low concentrations. Provided a normal intracellular concentration of SAP is present,activating signals are propagated upon CD244-CD48 interaction. (B) When SAP concentrations are low,absent,or dysfunctional (unable to bind), such as in X-linked proliferative disease,CD244 propagates an inhibitory signal upon CD244-CD48 interaction. (C) In the setting of cancer or chronic viral infection,NK cells and CD8+ T cells express high concentrations of CD244,and normal concentrations of SAP become insufficient to saturate CD244 binding sites upon CD244-CD48 interaction; an inhibitory signal is propagated.
CD244 in NK Cells
Early Studies of CD244 as an Activating Receptor in NK Cells in vitro
NK cells are critical for surveillance and eradication of cancer cells. CD244 is expressed on all NK cells (4, 20), where its signaling mechanisms were initially characterized. Early experiments showed that treatment of mouse NK cells with anti-2B4 monoclonal antibody (mAb) led to increased IFNγ production and augmented non-MHC-restricted killing of tumor cells in vitro (1). In addition, CD244-CD48 homotypic interactions were shown to be essential for optimal human NK cell proliferation in response to IL-2, as well as contributing to murine NK cell proliferation, lytic potential, and cytokine secretion (40, 41). Furthermore, cross-linking of CD244 on human NK cells using anti-CD244 mAb induced NK cell-mediated lysis of target cells (2). However, the same study also found that treatment of cultured human NK cells with anti-CD244 had an antagonistic effect on IL-2-stimulated proliferation, suggesting that CD244 could mediate activating or inhibitory signaling.
CD244 as an Inhibitory Receptor in NK Cells in vitro and in vivo
Soon after identification of this dual functionality, it was determined that CD48+ target cells inhibit murine NK cell effector function, and blocking the CD244-CD48 interaction with CD244 or CD48 mAb relieves this inhibition, causing enhanced target cell lysis (36). In addition, CD244 preferentially accumulates at the interface between NK and target cells during non-lytic events (36). These results introduced an inhibitory role for CD244 in mouse NK cells. Findings by Lee et al. further showed that CD244 ligation inhibits NK cell-mediated lysis of CD48+ tumor cells and NK cell production of IFNγ in vitro (37). Correspondingly, in vivo administration of anti-CD244 mAb significantly decreased the number of B16F10 syngeneic melanoma lung nodules in wildtype (WT) mice following intravenous injection (42). However, female CD244−/− mice showed poor rejection of both CD48(+) and CD48(–) B16 melanoma cells, suggesting a gender-based difference in these genetically-modified mice. Further studies are needed to determine whether this difference exists in other genetic backgrounds. In both of these studies, CD244−/− mice demonstrated increased ability to reject CD48(+) B16 melanoma cells compared with WT. Taken together, these data strongly support an inhibitory role for CD244 in NK cells.
Inhibitory CD244 signaling in NK cells has also been demonstrated in human cancer patients. When CD244+ NK cells were co-cultured with tumor-infiltrating, CD48+CD68+ monocytes/macrophages obtained from patients with hepatocellular carcinoma, they initially demonstrated increased TNFα and IFNγ production, followed by exhaustion with significantly decreased cytokine production and increased apoptosis (9). This exhaustion did not occur in CD244+ NK cells co-cultured with non-tumor liver-infiltrating monocyte/macrophages, which exhibited significantly lower expression of CD48 than those from tumor. Importantly, the exhaustion seen in NK cells co-cultured with tumor-associated CD48hi monocytes/macrophages could be overcome by blocking the CD244-CD48 interaction using anti-CD48 mAb. These observations support a role for CD244 signaling in the development of NK cell exhaustion in the tumor microenvironment (Figure 3A).
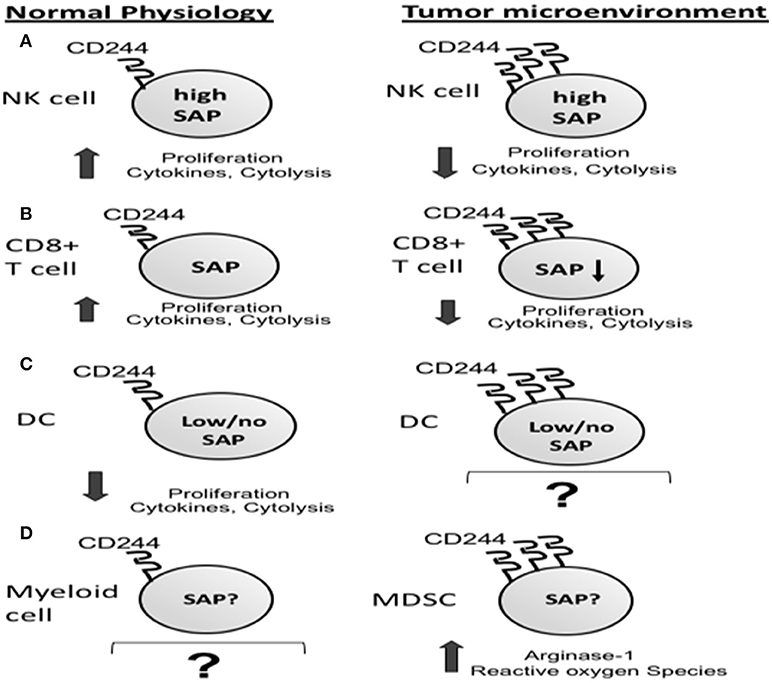
Figure 3. Increased CD244 expression on immune cells in the tumor microenvironment corresponds to increased immunosuppression via effector cell exhaustion (A,B) and increased production of immunosuppressors by myeloid derived suppressor cells (MDSCs) (C).
CD244 Function in CD8+ T Cells
CD244 Signaling and CD8+ T Cell Exhaustion in Chronic Infection
CD244 is co-expressed on a subset of antigen-experienced, effector and effector memory CD8+ T cells with other immunoregulatory receptors including PD-1, LAG3, CD160, CTLA-4, and TIM-3 in mice and humans (11, 43–45). In mouse models of chronic viral infection using lymphocytic choriomeningitis virus (LCMV) clone 13, these T-cells exhibit an exhausted phenotype (10, 11). As in NK cells, CD244 receptor concentration affects whether activating or inhibitory signaling occurs. In LCMV-specific CD8+ T cells with low-intermediate CD244 expression, blocking CD244 with anti-CD48 mAb decreases IFNγ production (reflecting activating signaling), while blocking CD244 on exhausted CD8+ T cells with high CD244 expression significantly increases IFNγ production (reflecting inhibitory signaling) (11). Of note, SAP expression decreases in effector CD8+ T cells over time (12), suggesting that, as in NK cells, relative decreases in SAP concentration may lead to inhibitory CD244 signaling in CD8+ T cells. These findings support increased CD244 expression as a mediator of CD8+ T cell exhaustion in the setting of persistent antigen exposure.
In humans, CD244 expression has been shown to be increased on CD8+ T cells in chronic infection (15, 46–51). In these infections, CD244 expression correlates with CD8+CD45RA+CCR7- effector or effector memory subtypes (46, 47). CD244 expression is higher on virus-specific CD8+ T cells in patients with chronic infection than with acute infection and correlates with PD1 expression (15, 47). Human CD8+ T cells with high CD244 expression show evidence of exhaustion that can be reversed with CD244 blockade (15, 47). Ex vivo, blockade of CD244 using anti-CD244 mAb on human CD244+CD8+T cells leads to increased virus-specific T cell proliferation, increased expression of CD107a and perforin (markers of degranulation), and IFNy, and increased TNFα and IL-6 in culture supernatants (15, 47, 50, 51). Similarly, the response to CD244 cross-linking in vitro seems to depend on the relative expression of CD244, with decreased proliferation in response to CD244 activation in CD244hi CD8+ T cells compared to CD244lo/intCD8+ T cells (47). Likewise, the combination of anti-CD3, anti-CD28, and CD244 cross-linking increased IFNγ and degranulation only in CD244lo CD8+ T cells, but not in CD244hi CD8+ T cells. These findings suggest that, as in mice, CD244 in humans is a marker of CD8+ T cell exhaustion in chronic infection, and when expressed at high concentrations, acts as an inhibitory receptor.
CD244 Signaling and CD8+ T Cell Exhaustion in Cancer
In mouse models of cancer, CD244 is expressed on CD8+ T cells with an exhausted phenotype. In syngeneic C57BL/6 mouse models of pancreatic adenocarcinoma and lung carcinoma, frequencies of T-cells exhibiting co-inhibitory receptors CD244, PD-1, and BTLA were increased in tumor-bearing mice compared with naïve controls (52). Consistent with a role in T-cell exhaustion, increased CD244 expression on antigen-specific CD8+ T cells from the spleens of tumor-bearing mice correlated with reduced IL-2 and IFN-γ production (Figure 3B).
In human cancers, CD244 also shows increased expression on exhausted CD8+ T cells. In melanoma, CD244 is increased in tumor-infiltrating lymphocytes compared with peripheral blood, and CD8+ T cells from tumor and peripheral blood show increased CD244 expression compared with CD8+ T cells from healthy controls (43, 44). CD244 is also co-expressed on melanoma-associated CD8+ T cells with other inhibitory receptors, including PD-1, TIM-3 (43, 44), CD160 (43, 45), KLRG1 (44). Because non-hematopoietic cancers do not generally express CD48, T-cell CD244 signaling likely depends on the availability of immune cell-cell interactions within these tumor microenvironments, paralleling the dependence of CD244 signaling in NK cells on local macrophages in hepatocellular carcinoma (9). In multiple myeloma patients, CD8+ T cells demonstrated an exhausted phenotype with decreased CD28 expression, decreased proliferation, and decreased degranulation as measured by mobilization of CD107a (45). These exhausted CD8+ T-cells co-expressed increased CD244, PD-1, CTLA-4, and CD160 compared with non-exhausted CD8+ T cells from healthy controls. Similarly, in AML patients, CD244 expression was increased on peripheral blood T cells compared to healthy controls and correlated with PD-1 expression (53). In another series of AML patients, CD244 expression on T cells was higher in relapse than in new diagnosis, and the degree of CD244 expression in relapsed AML was equivalent to that seen in untreated HIV patients (54). The authors of this second study did not see any correlation between increased CD244 or PD-1 expression and proliferation or cytokine secretion in vitro. However, a study by Epling-Burnette et al. demonstrated increased expression of CD244 and decreased expression of activation markers CD28 and CD62L on circulating T cells in myelodysplastic syndrome (MDS) patients, suggesting a link between increased CD244 expression and T cell exhaustion in this segment of the MDS/AML spectrum (55).
CD244 Function in Myeloid Cells
Dendritic cells are professional antigen-presenting cells that play a crucial role in the induction and maintenance of anti-tumor immunity by cross-presenting tumor antigens and cross-priming tumor-specific T cells. Importantly, the presence of intratumoral DCs is known to correlate positively with prognosis in multiple human cancers (56). However, recent studies have shown that DCs can play an activating or inhibitory role in the tumor microenvironment depending on DC subset, maturation status, and presence of (co-)stimulatory and inhibitory receptors and cytokines (57). Both mouse and human DC populations express CD244. Expression is higher on so-called conventional DC populations compared with plasmacytoid DCs (6). Functionally, CD244−/− DCs from C57BL/6 mice produce significantly higher levels of pro-inflammatory cytokines than WT DCs upon TLR stimulation in vitro (6). In addition to priming T cells, DCs contribute to anti-tumor immunity by activation of NK cells. CD244−/− signaling in DCs appears to affect this function: in vitro, CD244−/− DCs elicit greater NK cell activation than WT DCs.(6) Notably, SAP is not expressed at significant levels in DCs, while EAT-2, SHIP-1, SHP-1, and SHP-2 are all expressed, which may account for the inhibitory role of CD244 signaling in DCs. Further studies are required to elucidate the CD244 signaling pathways in DCs and to determine the influences of the tumor microenvironment on those pathways.
Myeloid-derived suppressor cells (MDSCs) are highly suppressive immune cells found in the tumor microenvironment and in the peripheral blood and spleens of tumor-bearing hosts. Increased numbers of MDSCs have been associated with tumor progression (58, 59), metastases (60, 61), and poor response to current therapies (62–64). Two morphologically distinct subtypes of MDSCs have been identified in both mice and humans: monocytic MDSC (Mo-MDSC: CD11b+ Ly6Chi Ly6G- in mice; CD33+ HLA-DRlo/− CD14+ in humans) and granulocytic MDSC (Gr-MDSC: CD11b+ Ly6Clo/− Ly6G+ in mice; CD33+ HLA-DRlo/− CD15+ in humans) (65). Both subtypes have been shown to produce suppressive mediators including inducible nitric oxide synthase (iNOS), arginase-1, indoleamine 2,3 dioxygenase (IDO), IL-10, and TGFβ1 (66–70). Both MDSC subtypes are known to suppress CD8+ T-cell function (71–73). Recently, the expression of CD244 has been described on MDSCs in tumor-bearing mice, with 30–50% of Gr-MDSC expressing CD244 in four syngeneic tumor models. Distinct differences in Gr-MDSC function were observed between CD244+ and CD244– populations. CD244+ Gr-MDSC significantly suppressed antigen-specific CD8+ T cell response compared to CD244- Gr-MDSC, which did not suppress. Additionally, expression of CD244 on Gr-MDSC correlated with reactive oxygen species (ROS) production and myeloperoxidase (7) (Figure 3C). The correlation between CD244 expression and immunosuppressive capacity in these tumor-associated MDSCs is consistent with the inhibitory role of CD244 signaling in NK cells and CD8+ T cells in the tumor microenvironment.
Therapeutic Considerations and Discussion
CD244 is a SLAM family receptor with activating and inhibitory signaling capacities implicated in the functions of NK cells, T cells, DCs and MDSCs in the tumor microenvironment. CD244 appears to predominantly propagate inhibitory signaling in tumor-associated immune cells, but the interplay of factors determining activating versus inhibitory signaling has not been fully elucidated. Increased cell suface density of CD244 and decreased or absent concentrations of functional SAP have both been identified as factors associated with inhibitory signaling (39), while conversely, decreased CD244 density and normal concentrations of functional SAP have been associated with activating signaling (8, 36, 37, 42, 74, 75). In addition, binding to the ITSM domains of the CD244 receptor is a competitive process with SAP preferentially binding over the adaptor molecules associated with inhibitory CD244 signaling (8, 34, 35, 38). These patterns suggest that the ratio of SAP to CD244 is critical to the determination of activating versus inhibitory signaling by this receptor. We theorize that the inhibitory signaling seen in exhausted immune cells with increased CD244 expression occurs because the increased density of CD244 decreases the ratio of SAP to CD244, allowing binding of one of the other adaptor molecules and propagation of an inhibitory signal. Future studies will test this hypothesis in tumor-associated T-cells, DCs, and MDSCs, as differential expression of these molecules is expected to affect the proposed mechanism.
Corresponding to inhibitory signaling in NK cells, CD244 demonstrates an inhibitory function when expressed at higher concentrations on CD8+ T cells that demonstrate an exhausted phenotype in chronic viral infection and cancer (10, 11, 46, 47, 50, 52, 76). Furthermore, in MDSCs from tumor-bearing mice, CD244 expression correlates with suppression of antigen-specific CD8+ T cell function and production of suppressive molecules, suggesting a role for CD244 signaling in the immunosuppressive function of these cells. CD244−/− DCs produce increased levels of pro-inflammatory cytokines and increased activation of NK cells, reflecting inhibitory CD244 signaling in DCs. Taken together, these findings suggest that CD244 signaling on NK cells, CD8+ T cells, DCs, and MDSCs may contribute to immunosuppression in the tumor microenvironment.
The evidence that inhibitory CD244 signaling contributes to immunosuppression in the tumor microenvironment suggests that targeting CD244 could provide a strategy for overcoming resistance to existing checkpoint inhibitors by multiple mechanisms. For example, blocking CD244 signaling on exhausted CD8+ T-cells may ameliorate the exhausted phenotype and contribute to re-activation of memory CD8+ T cells in cancer. Blocking CD244 signaling on DCs may increase pro-inflammatory cytokine release and activation NK cells and CD4+ T cells. Finally, blocking CD244 signaling in MDSCs may decrease the suppressive capacity of these cells, which are known to correspond with more aggressive disease and resistance to current therapies (62–64, 77–79). Importantly, CD244−/− mice appear phenotypically normal with normal maturation of the immune cell repertoire and a normal lifespan compared with other C57BL/6 laboratory mice, suggesting that therapeutic CD244 blockade could be feasible from an adverse effects standpoint. Thus, targeted anti-CD244 therapy could be of benefit as an adjunct to existing checkpoint inhibitors or even conventional chemotherapy strategies with limited myelosuppression.
Besides the effect on immune cells, recent evidence suggests a direct effect of CD244 signaling on CD244-expressing tumor cells. Specifically, CD244 signaling may also play a role in leukemogenesis, adding to its potential as a therapeutic target. A recent study shows that knock-down of CD244 in human leukemia cell lines produces markedly impaired proliferation in vitro and in vivo, while the repopulation ability of hematopoietic stem cells remains unimpaired following CD244 knockdown (80). Furthermore, in a mouse model of AML, leukemogenesis is dramatically delayed upon CD244 deletion. CD244 may therefore represent a unique therapeutic target if future studies confirm its role as a direct anti-leukemia target and support the hypothesis that it mediates immunosuppressive function in the tumor microenvironment.
Author Contributions
All authors listed have made a substantial, direct and intellectual contribution to the work, and approved it for publication.
Funding
The research was supported by the Office of the Assistant Secretary of Defense for Health Affairs through the Peer Reviewed Cancer Research Program under Award No. W81XWH-17-1-0374, the National Cancer Institute (CA201918-01), and the National Institute on Aging (AG053498).
Conflict of Interest Statement
The authors declare that the research was conducted in the absence of any commercial or financial relationships that could be construed as a potential conflict of interest.
References
1. Garni-Wagner BA, Purohit A, Mathew PA, Bennett M, Kumar V. A novel function-associated molecule related to non-MHC-restricted cytotoxicity mediated by activated natural killer cells and T cells. J Immunol. (1993) 151:60–70.
2. Valiante NM, and Trinchieri G. Identification of a novel signal transduction surface molecule on human cytotoxic lymphocytes. J Exp Med. (1993) 178:1397–406.
3. Schuhmachers G, Ariizumi K, Mathew PA, Bennett M, Kumar V, Takashima A. 2B4, a new member of the immunoglobulin gene superfamily, is expressed on murine dendritic epidermal T cells and plays a functional role in their killing of skin tumors. J Invest Dermatol. (1995) 105:592–6. doi: 10.1111/1523-1747.ep12323533
4. Nakajima H, Cella M, Langen H, Friedlein A, Colonna M. Activating interactions in human NK cell recognition: the role of 2B4-CD48. Eur J Immunol. (1999) 29:1676–83.
5. Straub C, Neulen ML, Viertlboeck BC, Göbel TW. Chicken SLAMF4 (CD244, 2B4), a receptor expressed on thrombocytes, monocytes, NK cells, and subsets of alphabeta-, gammadelta- T cells and B cells binds to SLAMF2. Dev Comp Immunol. (2014) 42:159–68. doi: 10.1016/j.dci.2013.09.007
6. Georgoudaki AM, Khodabandeh S, Puiac S, Persson CM, Larsson MK, Lind M, et al. CD244 is expressed on dendritic cells and regulates their functions. Immunol Cell Biol. (2015) 93:581–90. doi: 10.1038/icb.2014.124
7. Youn JI, Collazo M, Shalova IN, Biswas SK, Gabrilovich DI. Characterization of the nature of granulocytic myeloid-derived suppressor cells in tumor-bearing mice. J Leukoc Biol. (2012) 91:167–81. doi: 10.1189/jlb.0311177
8. Parolini S, Bottino C, Falco M, Augugliaro R, Giliani S, Franceschini R, et al. X-linked lymphoproliferative disease. 2B4 molecules displaying inhibitory rather than activating function are responsible for the inability of natural killer cells to kill Epstein-Barr virus-infected cells. J Exp Med. (2000) 192:337–46. doi: 10.1084/jem.192.3.337
9. Wu Y, Kuang DM, Pan WD, Wan YL, Lao XM, Wang D, et al. Monocyte/macrophage-elicited natural killer cell dysfunction in hepatocellular carcinoma is mediated by CD48/2B4 interactions. Hepatology (2013) 57:1107–16. doi: 10.1002/hep.26192
10. Wherry EJ, Kurachi M. Molecular and cellular insights into T cell exhaustion. Nat Rev Immunol. (2015) 15:486–99. doi: 10.1038/nri3862
11. Blackburn SD, Shin H, Haining WN, Zou T, Workman CJ, Polley A, et al. Coregulation of CD8+ T cell exhaustion by multiple inhibitory receptors during chronic viral infection. Nat Immunol. (2009) 10:29–37. doi: 10.1038/ni.1679
12. Heng TS, Painter MW, Immunological Genome Project Consortium The Immunological Genome Project: networks of gene expression in immune cells. Nat Immunol. (2008) 9:1091–4. doi: 10.1038/ni1008-1091
13. Mathew PA, Garni-Wagner BA, Land K, Takashima A, Stoneman E, Bennett M, et al. Cloning and characterization of the 2B4 gene encoding a molecule associated with non-MHC-restricted killing mediated by activated natural killer cells and T cells. J Immunol. (1993) 151:5328–37.
14. Youngblood B, Wherry EJ, Ahmed R. Acquired transcriptional programming in functional and exhausted virus-specific CD8 T cells. Curr Opin HIV AIDS (2012) 7:50–7. doi: 10.1097/COH.0b013e32834ddcf2
15. Raziorrouh B, Schraut W, Gerlach T, Nowack D, Grüner NH, Ulsenheimer A, et al. The immunoregulatory role of CD244 in chronic hepatitis B infection and its inhibitory potential on virus-specific CD8+ T-cell function. Hepatology (2010) 52:1934–47. doi: 10.1002/hep.23936
16. Yokoyama S, Staunton D, Fisher R, Amiot M, Fortin JJ, Thorley-Lawson DA. Expression of the Blast-1 activation/adhesion molecule and its identification as CD48. J Immunol. (1991) 146:2192–200.
17. Brown MH, Boles K, van der Merwe PA, Kumar V, Mathew PA, Barclay AN. 2B4, the natural killer and T cell immunoglobulin superfamily surface protein, is a ligand for CD48. J Exp Med. (1998) 188:2083–90. doi: 10.1084/jem.188.11.2083
18. Latchman Y, McKay PF, Reiser H. Identification of the 2B4 molecule as a counter-receptor for CD48. J Immunol. (1998) 161:5809–12.
19. Kubin MZ, Parshley DL, Din W, Waugh JY, Davis-Smith T, Smith CA, et al. Molecular cloning and biological characterization of NK cell activation-inducing ligand, a counterstructure for CD48. Eur J Immunol. (1999) 29:3466–77.
20. Boles KS, Nakajima H, Colonna M, Chuang SS, Stepp SE, Bennett M, et al. Molecular characterization of a novel human natural killer cell receptor homologous to mouse 2B4. Tissue Antigens (1999) 54:27–34.
21. Stepp SE, Schatzle JD, Bennett M, Kumar V, Mathew PA. Gene structure of the murine NK cell receptor 2B4: presence of two alternatively spliced isoforms with distinct cytoplasmic domains. Eur J Immunol. (1999) 29:2392–9. doi: 10.1002/(SICI)1521-4141(199908)29:08<2392::AID-IMMU2392>3.0.CO;2-R
22. Kumaresan PR, Mathew PA. Structure of the human natural killer cell receptor 2B4 gene and identification of a novel alternative transcript. Immunogenetics (2000) 51:987–92. doi: 10.1007/s002510000237
23. Mathew SO, Rao KK, Kim JR, Bambard ND, Mathew PA. Functional role of human NK cell receptor 2B4 (CD244) isoforms. Eur J Immunol. (2009) 39:1632–41. doi: 10.1002/eji.200838733
24. Sayos J, Wu C, Morra M, Wang N, Zhang X, Allen D, et al. The X-linked lymphoproliferative-disease gene product SAP regulates signals induced through the co-receptor SLAM. Nature (1998) 395:462–9.
25. Morra M, Lu J, Poy F, Martin M, Sayos J, Calpe S, et al. Structural basis for the interaction of the free SH2 domain EAT-2 with SLAM receptors in hematopoietic cells. Embo J. (2001) 20:5840–52. doi: 10.1093/emboj/20.21.5840
26. Wang N, Calpe S, Westcott J, Castro W, Ma C, Engel P, et al. Cutting edge: the adapters EAT-2A and−2B are positive regulators of CD244- and CD84-dependent NK cell functions in the C57BL/6 mouse. J Immunol. (2010) 185:5683–7. doi: 10.4049/jimmunol.1001974
27. Roncagalli R, Taylor JE, Zhang S, Shi X, Chen R, Cruz-Munoz ME, et al. Negative regulation of natural killer cell function by EAT-2, a SAP-related adaptor. Nat Immunol. (2005) 6:1002–10. doi: 10.1038/ni1242
28. Wahle JA, Paraiso KH, Kendig RD, Lawrence HR, Chen L, Wu J, et al. Inappropriate recruitment and activity by the Src homology region 2 domain-containing phosphatase 1 (SHP1) is responsible for receptor dominance in the SHIP-deficient NK cell. J Immunol. (2007) 179:8009–15. doi: 10.4049/jimmunol.179.12.8009
29. Schatzle JD, Sheu S, Stepp SE, Mathew PA, Bennett M, Kumar V. Characterization of inhibitory and stimulatory forms of the murine natural killer cell receptor 2B4. Proc Natl Acad Sci. (1999) 96:3870–5. doi: 10.1073/pnas.96.7.3870
30. Chen R, Relouzat F, Roncagalli R, Aoukaty A, Tan R, Latour S, et al. Molecular dissection of 2B4 signaling: implications for signal transduction by SLAM-related receptors. Mol Cell Biol. (2004) 24:5144–56. doi: 10.1128/MCB.24.12.5144-5156.2004
31. Eissmann P, Beauchamp L, Wooters J, Tilton JC, Long EO, Watzl C. Molecular basis for positive and negative signaling by the natural killer cell receptor 2B4 (CD244). Blood (2005) 105:4722–9. doi: 10.1182/blood-2004-09-3796
32. Nakajima H, Cella M, Bouchon A, Grierson HL, Lewis J, Duckett CS, et al. Patients with X-linked lymphoproliferative disease have a defect in 2B4 receptor-mediated NK cell cytotoxicity. Eur J Immunol. (2000) 30:3309–18.doi: 10.1002/1521-4141(200011)30:11 < 3309::AID-IMMU3309>3.0.CO;2-3
33. Bloch-Queyrat C, Fondanèche MC, Chen R, Yin L, Relouzat F, Veillette A, et al. Regulation of natural cytotoxicity by the adaptor SAP and the Src-related kinase Fyn. J Exp Med. (2005) 202:181–92. doi: 10.1084/jem.20050449
34. Tangye SG, Phillips JH, Lanier LL, Nichols KE. Functional requirement for SAP in 2B4-mediated activation of human natural killer cells as revealed by the X-linked lymphoproliferative syndrome. J Immunol. (2000) 165:2932–6. doi: 10.4049/jimmunol.165.6.2932
35. Sayós J, Nguyen KB, Wu C, Stepp SE, Howie D, Schatzle JD, et al. Potential pathways for regulation of NK and T cell responses: differential X-linked lymphoproliferative syndrome gene product SAP interactions with SLAM and 2B4. Int Immunol. (2000) 12:1749–57.
36. Mooney JM, Klem J, Wülfing C, Mijares LA, Schwartzberg PL, Bennett M, et al. The murine NK receptor 2B4 (CD244) exhibits inhibitory function independent of signaling lymphocytic activation molecule-associated protein expression. J Immunol. (2004) 173:3953–61. doi: 10.4049/jimmunol.173.6.3953
37. Lee KM, McNerney ME, Stepp SE, Mathew PA, Schatzle JD, Bennett M, et al. 2B4 acts as a non-major histocompatibility complex binding inhibitory receptor on mouse natural killer cells. J Exp Med. (2004) 199:1245–54. doi: 10.1084/jem.20031989
38. Tangye SG, Lazetic S, Woollatt E, Sutherland GR, Lanier LL, Phillips JH. Cutting edge: human 2B4, an activating NK cell receptor, recruits the protein tyrosine phosphatase SHP-2 and the adaptor signaling protein SAP. J Immunol. (1999) 162:6981–5.
39. Chlewicki LK, Velikovsky CA, Balakrishnan V, Mariuzza RA, Kumar V. Molecular basis of the dual functions of 2B4 (CD244). J Immunol. (2008) 180:8159–67. doi: 10.4049/jimmunol.180.12.8159
40. Lee KM, Forman JP, McNerney ME, Stepp S, Kuppireddi S, Guzior D, et al. Requirement of homotypic NK-cell interactions through 2B4(CD244)/CD48 in the generation of NK effector functions. Blood (2006) 107:3181–8. doi: 10.1182/blood-2005-01-0185
41. Kim EO, Kim TJ, Kim N, Kim ST, Kumar V, Lee KM. Homotypic cell to cell cross-talk among human natural killer cells reveals differential and overlapping roles of 2B4 and CD2. J Biol Chem. (2010) 285:41755–64. doi: 10.1074/jbc.M110.137976
42. Johnson LA, Vaidya SV, Goldfarb RH, Mathew PA. 2B4(CD244)-mediated activation of NK cells reduces metastases of B16F10 melanoma in mice. Anticancer Res. (2003) 23:3651–5.
43. Lichtenegger FS, Schnorfeil FM, Emmerig K, Neitz JS, Beck B, Draenert R, et al. Pseudo-exhaustion of CD8+ T cells in AML. Blood (2013) 122:2615.
44. Baitsch L, Baumgaertner P, Devêvre E, Raghav SK, Legat A, Barba L, et al. Exhaustion of tumor-specific CD8(+) T cells in metastases from melanoma patients. J Clin Invest. (2011) 121:2350–60. doi: 10.1172/JCI46102
45. Zelle-Rieser C, Thangavadivel S, Biedermann R, Brunner A, Stoitzner P, Willenbacher E, et al. T cells in multiple myeloma display features of exhaustion and senescence at the tumor site. J Hematol Oncol. (2016) 9:116. doi: 10.1186/s13045-016-0345-3
46. de Mare-Bredemeijer EL, Shi XL, Mancham S, van Gent R, van der Heide-Mulder M, de Boer R, et al. Cytomegalovirus-induced expression of CD244 after liver transplantation is associated with CD8+ T cell hyporesponsiveness to alloantigen. J Immunol. (2015) 195:1838–48. doi: 10.4049/jimmunol.1500440
47. Schlaphoff V, Lunemann S, Suneetha PV, Jaroszewicz J, Grabowski J, Dietz J, et al. Dual function of the NK cell receptor 2B4 (CD244) in the regulation of HCV-specific CD8+ T cells. PLoS Pathog. (2011) 7:e1002045. doi: 10.1371/journal.ppat.1002045
48. Aldy KN, Horton NC, Mathew PA, Mathew SO. 2B4+ CD8+ T cells play an inhibitory role against constrained HIV epitopes. Biochem Biophys Res Commun. (2011) 405:503–7. doi: 10.1016/j.bbrc.2011.01.062
49. Enose-Akahata Y, Matsuura E, Oh U, Jacobson S. High expression of CD244 and SAP regulated CD8 T cell responses of patients with HTLV-I associated neurologic disease. PLoS Pathog. (2009) 5:e1000682. doi: 10.1371/journal.ppat.1000682
50. Wang Y, Zhong H, Xie X, Chen CY, Huang D, Shen L, et al. Long noncoding RNA derived from CD244 signaling epigenetically controls CD8+ T-cell immune responses in tuberculosis infection. Proc Natl Acad Sci USA. (2015) 112:E3883–92. doi: 10.1073/pnas.1501662112
51. Ezinne CC, Yoshimitsu M, White Y, Arima N. HTLV-1 specific CD8+ T cell function augmented by blockade of 2B4/CD48 interaction in HTLV-1 infection. PLoS ONE (2014) 9:e87631. doi: 10.1371/journal.pone.0087631
52. Mittal R, Wagener M, Breed ER, Liang Z, Yoseph BP, Burd EM, et al. Phenotypic T cell exhaustion in a murine model of bacterial infection in the setting of pre-existing malignancy. PLoS ONE (2014) 9:e93523. doi: 10.1371/journal.pone.0093523
53. Tan J, Chen S, Lu Y, Yao D, Xu L, Zhang Y, et al. Higher PD-1 expression concurrent with exhausted CD8+ T cells in patients with de novo acute myeloid leukemia. Chin J Cancer Res. (2017) 29:463–470. doi: 10.21147/j.issn.1000-9604.2017.05.11
54. Schnorfeil FM, Lichtenegger FS, Emmerig K, Schlueter M, Neitz JS, Draenert R, et al. T cells are functionally not impaired in AML: increased PD-1 expression is only seen at time of relapse and correlates with a shift towards the memory T cell compartment. J Hematol Oncol. (2015) 8:93. doi: 10.1186/s13045-015-0189-2
55. Epling-Burnette PK, Painter JS, Rollison DE, Ku E, Vendron D, Widen R, et al. Prevalence and clinical association of clonal T-cell expansions in Myelodysplastic Syndrome. Leukemia (2007) 21:659–67. doi: 10.1038/sj.leu.2404590
56. Lotze MT. Getting to the source: dendritic cells as therapeutic reagents for the treatment of patients with cancer. Ann Surg. (1997) 226:1–5.
57. Liu Y, Cao X. Intratumoral dendritic cells in the anti-tumor immune response. Cell Mol Immunol. (2015) 12:387–90. doi: 10.1038/cmi.2014.130
58. Bunt SK, Sinha P, Clements VK, Leips J, Ostrand-Rosenberg S. Inflammation induces myeloid-derived suppressor cells that facilitate tumor progression. J Immunol. (2006) 176:284–90. doi: 10.4049/jimmunol.176.1.284
59. Chevolet I, Speeckaert R, Schreuer M, Neyns B, Krysko O, Bachert C, et al. Clinical significance of plasmacytoid dendritic cells and myeloid-derived suppressor cells in melanoma. J Transl Med. (2015) 13:9. doi: 10.1186/s12967-014-0376-x
60. Sinha P, Clements VK, Ostrand-Rosenberg S. Reduction of myeloid-derived suppressor cells and induction of M1 macrophages facilitate the rejection of established metastatic disease. J Immunol. (2005) 174:636–45. doi: 10.4049/jimmunol.174.2.636
61. Huang A, Zhang B, Wang B, Zhang F, Fan KX, Guo YJ. Increased CD14(+)HLA-DR (-/low) myeloid-derived suppressor cells correlate with extrathoracic metastasis and poor response to chemotherapy in non-small cell lung cancer patients. Cancer Immunol Immunother. (2013) 62:1439–51. doi: 10.1007/s00262-013-1450-6
62. Sun H, Li Y, Zhang ZF, Ju Y, Li L, Zhang BC, et al. Increase in myeloid-derived suppressor cells (MDSCs) associated with minimal residual disease (MRD) detection in adult acute myeloid leukemia. Int J Hematol. (2015) 102:579–86. doi: 10.1007/s12185-015-1865-2
63. Napolitano M, D'Alterio C, Cardone E, Trotta AM, Pecori B, Rega D, et al. Peripheral myeloid-derived suppressor and T regulatory PD-1 positive cells predict response to neoadjuvant short-course radiotherapy in rectal cancer patients. Oncotarget (2015) 6:8261–70. doi: 10.18632/oncotarget.3014
64. Poschke I, Mao Y, Adamson L, Salazar-Onfray F, Masucci G, Kiessling R. Myeloid-derived suppressor cells impair the quality of dendritic cell vaccines. Cancer Immunol Immunother. (2012) 61:827–38. doi: 10.1007/s00262-011-1143-y
65. Bronte V, Brandau S, Chen SH, Colombo MP, Frey AB, Greten TF, et al. Recommendations for myeloid-derived suppressor cell nomenclature and characterization standards. Nat Commun. (2016) 7:12150. doi: 10.1038/ncomms12150
66. Redd PS, Ibrahim ML, Klement JD, Sharman SK, Paschall AV, Yang D, et al. SETD1B activates iNOS expression in myeloid-derived suppressor cells. Cancer Res. (2017) 77:2834–43. doi: 10.1158/0008-5472.CAN-16-2238
67. Rastad JL, Green WR. Myeloid-derived suppressor cells in murine AIDS inhibit B-cell responses in part via soluble mediators including reactive oxygen and nitrogen species, and TGF-beta. Virology (2016) 499:9–22. doi: 10.1016/j.virol.2016.08.031
68. Gielen PR, Schulte BM, Kers-Rebel ED, Verrijp K, Bossman SA, Ter Laan M, et al. Elevated levels of polymorphonuclear myeloid-derived suppressor cells in patients with glioblastoma highly express S100A8/9 and arginase and suppress T cell function. Neuro Oncol. (2016) 18:1253–64. doi: 10.1093/neuonc/now034
69. Chevolet I, Speeckaert R, Schreuer M, Neyns B, Krysko O, Bachert C, et al. Characterization of the in vivo immune network of IDO, tryptophan metabolism, PD-L1, and CTLA-4 in circulating immune cells in melanoma. Oncoimmunology (2015) 4:e982382. doi: 10.4161/2162402X.2014.982382
70. Mougiakakos D, Jitschin R, von Bahr L, Poschke I, Gary R, Sundberg B, et al. Immunosuppressive CD14+HLA-DRlow/neg IDO+ myeloid cells in patients following allogeneic hematopoietic stem cell transplantation. Leukemia (2013) 27:377–88. doi: 10.1038/leu.2012.215
71. Pinton L, Solito S, Damuzzo V, Francescato S, Pozzuoli A, Berizzi A, et al. Activated T cells sustain myeloid-derived suppressor cell-mediated immune suppression. Oncotarget (2016) 7:1168–84. doi: 10.18632/oncotarget.6662
72. Movahedi K, Guilliams M, Van den Bossche J, Van den Bergh R, Gysemans C, Beschin A, et al. Identification of discrete tumor-induced myeloid-derived suppressor cell subpopulations with distinct T cell-suppressive activity. Blood (2008) 111:4233–44.
73. Kusmartsev S, Nefedova Y, Yoder D, Gabrilovich DI. Antigen-specific inhibition of CD8+ T cell response by immature myeloid cells in cancer is mediated by reactive oxygen species. J Immunol. (2004) 172:989–99. doi: 10.4049/jimmunol.172.2.989
74. Vaidya SV, Stepp SE, McNerney ME, Lee JK, Bennett M, Lee KM, et al. Targeted disruption of the 2B4 gene in mice reveals an in vivo role of 2B4 (CD244) in the rejection of B16 melanoma cells. J Immunol. (2005) 174:800–7. doi: 10.4049/jimmunol.174.2.800
75. Vacca P, Pietra G, Falco M, Romeo E, Bottino C, Bellora F, et al. Analysis of natural killer cells isolated from human decidua: evidence that 2B4 (CD244) functions as an inhibitory receptor and blocks NK-cell function. Blood (2006) 108:4078–85. doi: 10.1182/blood-2006-04-017343
76. West EE, Youngblood B, Tan WG, Jin HT, Araki K, Alexe G, et al. Tight regulation of memory CD8 T cells limits their effectiveness during sustained high viral load. Immunity (2011) 35:285–298. doi: 10.1016/j.immuni.2011.05.017
77. Ding ZC, Munn DH, Zhou G. Chemotherapy-induced myeloid suppressor cells and antitumor immunity: the Janus face of chemotherapy in immunomodulation. Oncoimmunology (2014) 3:e954471. doi: 10.4161/21624011.2014.954471
78. Long AH, Highfill SL, Cui Y, Smith JP, Walker AJ, Ramakrishna S, et al. Reduction of MDSCs with all-trans retinoic acid improves CAR therapy efficacy for sarcomas. Cancer Immunol Res. (2016) 4:869–80. doi: 10.1158/2326-6066.CIR-15-0230
79. Sasso MS, Lollo G, Pitorre M, Solito S, Pinton L, Valpione S, et al. Low dose gemcitabine-loaded lipid nanocapsules target monocytic myeloid-derived suppressor cells and potentiate cancer immunotherapy. Biomaterials (2016) 96:47–62. doi: 10.1016/j.biomaterials.2016.04.010
Keywords: CD244, tumor immune escape, immune exhaustion (IE), signaling lymphocyte activation molecule, MDSC, CD8 cytotoxic T lymphocytes +, NK cells and cancer, tumor microenviroment
Citation: Agresta L, Hoebe KHN and Janssen EM (2018) The Emerging Role of CD244 Signaling in Immune Cells of the Tumor Microenvironment. Front. Immunol. 9:2809. doi: 10.3389/fimmu.2018.02809
Received: 13 July 2018; Accepted: 14 November 2018;
Published: 28 November 2018.
Edited by:
Fabrizio Mattei, Istituto Superiore di Sanità (ISS), ItalyReviewed by:
Rodabe N. Amaria, University of Texas MD Anderson Cancer Center, United StatesAmorette Barber, Longwood University, United States
Copyright © 2018 Agresta, Hoebe and Janssen. This is an open-access article distributed under the terms of the Creative Commons Attribution License (CC BY). The use, distribution or reproduction in other forums is permitted, provided the original author(s) and the copyright owner(s) are credited and that the original publication in this journal is cited, in accordance with accepted academic practice. No use, distribution or reproduction is permitted which does not comply with these terms.
*Correspondence: Edith M. Janssen, ZWRpdGguamFuc3NlbkBjY2htYy5vcmc=