- 1Department of Obstetrics, Gynecology and Women’s Health, Division of Gynecologic Oncology, University of Minnesota School of Medicine, Minneapolis, MN, United States
- 2University of Minnesota School of Medicine, Minneapolis, MN, United States
- 3Department of Medicine, Division of Hematology, Oncology, and Transplantation, University of Minnesota School of Medicine, Minneapolis, MN, United States
Harnessing the immune system has proven an effective therapy in treating malignancies. Since the discovery of natural killer (NK) cells, strategies aimed to manipulate and augment their effector function against cancer have been the subject of intense research. Recent progress in the immunobiology of NK cells has led to the development of promising therapeutic approaches. In this review, we will focus on the recent advances in NK cell immunobiology and the clinical application of NK cell immunotherapy in ovarian, cervical, and uterine cancer.
Introduction
Exploiting the immune system has proven an effective therapeutic approach in treating a variety of malignancies. Identified in 1975, natural killer (NK) cells exist in the blood as preactivated cytolytic lymphocytes and are recognized as the most efficient antitumor effector (1–3). Distinct from T and B cells, NK cell effector function is not mediated by high-resolution antigen specificity but through signaling of multiple germ line-encoded activating and inhibiting receptors. Over the past 40 years, research has defined the regulation of NK cells and established essential roles they play in anticancer immunity. Strategies to harness and augment NK cells for cancer therapy are a relatively new and rapidly developing field. At this point, the success of NK cell-based immunotherapy has largely been confined to hematologic malignancies and has yet to translate to solid organ tumors (4). As a subset of solid organ tumors, gynecologic malignancies are a heterogeneous group of tumors derived from vulvar/vaginal, cervical, uterine, fallopian, and ovarian tissues. The treatment regimens for gynecologic cancers continue to develop with great room for improvement. With increased understanding of NK cell biology, there is renewed interest in NK cell-based immunotherapy directed against gynecologic malignancies. In this review, the advances in our understanding and clinical application of NK cell immunotherapy against ovarian, cervical, and uterine cancer is summarized.
Characteristics of NK Cells
The innate and adaptive immune systems function together to recognize and effectively eliminate aberrant cells, including cancer. Historically seen as part of the innate immune response, NK cells are large, granular lymphocytes. They were found to have the ability to kill tumor cells without any prior sensitization (thus “natural”) or restriction of major histocompatibility complex (MHC) molecule expression (1, 2, 5).
Phenotypically, NK cells are defined via flow cytometry by the absence of CD3 and the presence of CD56 surface expression and comprise approximately 5–10% of circulating lymphocytes (6, 7). NK cells can be divided into CD56brightCD16− or CD56dimCD16+ populations with different functional properties. Developmentally immature CD56brightCD16− NK cells are capable of producing abundant cytokines, particularly interferon gamma (IFN-γ) and tumor necrosis factor alpha (TNF-α), immediately after activation but possess little direct cytolytic function. In contrast, mature CD56dimCD16+ NK cells are characterized by the direct killing of transformed cells via perforin/granzyme release or death receptor pathways (Fas, TNF-related apoptosis-inducing ligand, TRAIL) (8–10).
As discussed below, NK cells are involved in tumor immunosurveillance and mediate antitumor responses (11). Their activity is highly regulated by a variety of germ line-encoded inhibitory and activating receptor expression (12, 13). Collectively, the complex balance of inhibitory and activating signals promotes self-tolerance or drives potent effector function of NK cells.
NK Cell Effector Functions
Natural killer cells identify and eliminate foreign, infected, damaged, or malignant cells through a variety of mechanisms. The most well-known is through receptor-mediated cytotoxicity. NK cells express a series of activating receptors capable of binding stress-induced ligands expressed on tumor cells. They also express a number of inhibitory receptors that interact with ligands to induce activation-limiting signals. When activating signals over-ride inhibitory mechanisms, the NK cell mediates exocytosis of stored lytic molecules. The membrane-disrupting protein perforin and serine protease granzymes then function in coordination to gain access to the target cell and induce apoptosis through the activation of caspases (14, 15).
Natural killer cells also appear to be the principal effectors for a process called antibody-dependent cell-mediated cytotoxicity (ADCC) (16). ADCC occurs when targets that become coated by antibody are recognized by NK cells via ligation to the low-affinity receptor for the Fc portion of human immunoglobulins, CD16 (FcγRIIIa). Upon binding, downstream signal transduction mechanisms lead to NK cell degranulation, cytokine secretion, and tumor cell lysis (15). The recent advances in our understanding of ADCC and NK function can be applied to augment NK cell immunotherapy. For example, monoclonal antibodies (mAbs) targeting CD20 (rituximab), Her2/neu (herceptin), epidermal growth factor receptor (cetuximab and panitumumab), and disaloganglioside (GD2) demonstrate significant antitumor contributions from NK cell-dependent ADCC in addition to the direct antitumor effect of the antibody (17). This strategy maintains the specificity against key molecular tumor targets important for cell proliferation or tumor growth with the added contribution of ADCC via NK cell effector function (16). Highlighting this role of ADCC, previous studies have demonstrated depletion in NK cell populations decreases the efficacy of mAb therapy (18). There is further evidence showing that specific FcγR polymorphisms impact responsiveness to mAb therapy and may even predict clinical outcomes for certain tumors (19–22). Today, mAb are being developed with enhanced affinity for CD16 to better activate NK cells and improve antitumor response (23, 24). Unfortunately, strategies to include the use of mAb to enhance ADCC in gynecologic malignancies have not been thoroughly investigated.
Natural killer cells can also initiate the transduction of death signals within target cells through death ligand/receptor ligation (25). NK cells are capable of expressing Fas ligand or TRAIL (26, 27). Interaction of these ligands with their respective antigens on tumor cells activates caspases and induces apoptosis (14). Recent studies have demonstrated the proteasome and histone deacetylase inhibitors upregulate the expression of death receptors and enhance NK cell-mediated cytotoxicity of tumor cells through the death receptor pathways (28–30). This is particularly interesting because this strategy was effective in both hematologic and solid tumors.
Finally, specific subsets of NK cells are capable of producing important immunoregulatory cytokines (31). NK cells expressing CD56bright are the primary source of NK cell-derived IFN-γ, TNF-α, and other cytokines that play a major role during the innate immune response to infection or tumorigenesis (8). The NK cells provide an early source of IFN-γ to induce CD8+ T cells to become cytotoxic T lymphocytes (CTLs) and drive a Th1 response of CD4+ T cells to further promote CTL differentiation (32, 33). These interactions are illustrated in Figure 1.
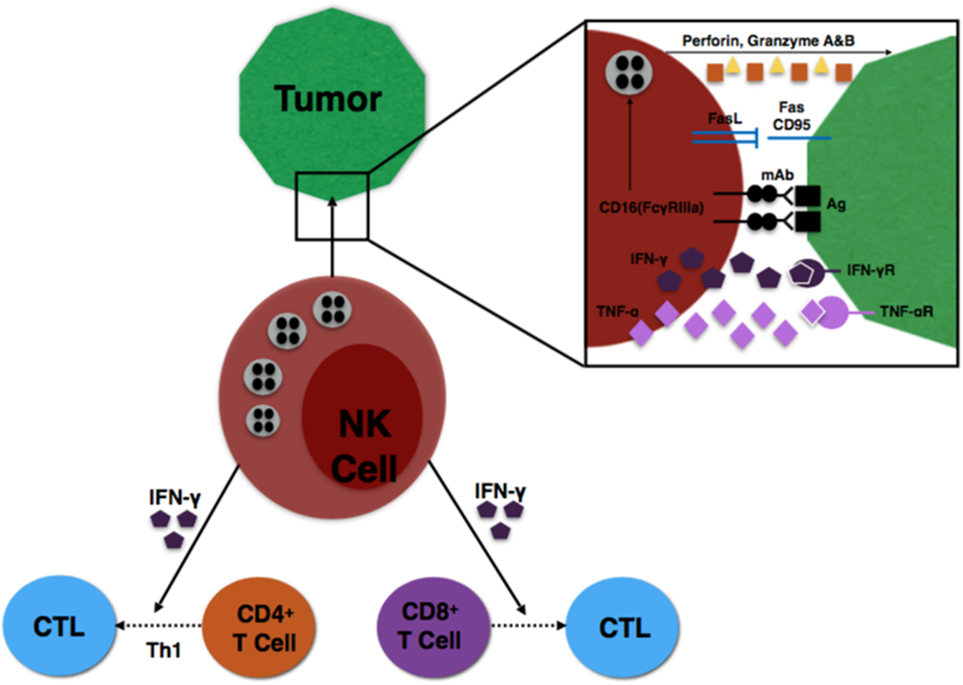
Figure 1. Illustration of NK cell interaction with tumor cells, as well as NK influence on T cell differentiation.
Important NK Cell Receptors
Due to the capability of immediate response, NK cells are tightly regulated through a combinatorial array of surface receptors. Functionally, these receptors are classified as activating or inhibitory with their ligands either members or homologs of MHC class I molecules. Structurally, they belong either to the immunoglobulin (Ig)-like receptor superfamily or the C-type lectin-like receptor (CTLR) superfamily (34). We will review a few of the selected inhibitory and activating receptors on human NK cells.
The NK cell response is dominated by a variety of germ line-encoded inhibitory receptors from three families: killer immunoglobulin-like receptors (KIRs), C-type lectins (including NKG2A-CD94), and leukocyte immunoglobulin-like receptors (35–37). Stochastic surface expression within these families leads to subsets of NK cells with a diverse repertoire of receptors (35). Ligands for these receptors are both “classical” and “non-classical” class I molecules encoded within the MHC, termed human leukocyte antigens (HLAs) (38–40). For example, inhibitory KIRs recognize the classical HLA-A, HLA-B, and HLA-C proteins but do not distinguish self from non-self peptides. The binding of inhibitory KIR on NK cells to their HLA cognate suppresses cytotoxicity and cytokine secretion. The diverse groups of inhibitory receptors are all glycoproteins that signal through the canonical immunoreceptor tyrosine-based inhibitory motif (ITIM) to suppress NK cell response. Only when sufficient activating signals are present does the NK cell initiate effector function.
The CD94 and NKG2 family of genes encode CTLRs that recognize non-classical MHC class I molecules (HLA-E, -F, -G, and -H) and play a dominant role in NK cell function. CD94 can heterodimerize with NKG2A and signal through ITIM to function as an inhibitory receptor when bound to HLA-E. However, CD94/NKG2C heterodimers serve as activating receptors. The inhibitory CD94/NKG2A and the activating CD94/NKG2C receptors are found in overlapping subsets of NK cells in the peripheral blood (38). Not surprisingly, CD94/NKG2A inhibitory receptor binds HLA-E with higher affinity compared to CD94/NKG2C activating receptor (41).
The role and function of NK inhibitory receptors are well defined. Recent research has elucidated the NK effector functions of activating and coactivating receptors. Unlike B and T cells, NK cells do not possess an activating receptor that dominates their development and effector function. Instead, they express a complement of invariant activating receptors that include the NK specific natural cytotoxicity receptors (NCRs) (NKp46, NKp30, and NKp44), C-type lectin-like (NKG2D, CD94/NKG2C), 2B4, DNAM-1, NTB-A, NKp80, CD59, and CD16 among others (36, 38, 42, 43).
The NKG2D gene encodes one activating receptor that, unlike its name, shares very little homology with NKG2A or NKG2C. NKG2D is a type II transmembrane-anchored glycoprotein constitutively expressed on all human NK cells and recognizes cell surface glycoproteins structurally related to MHC class I molecules. NK cells stimulated through NKG2D initiate cell-mediated cytotoxicity and cytokine release. Known human ligands include MICA, MICB, ULBP1, ULBP2, ULBP3, and ULBP4 and are upregulated by stress and stalled DNA replication via DNA-damage checkpoint pathways (34, 44). MICA and MICB are stress-induced antigens frequently expressed by tumors (45). However, progressive stages of cancer are associated with tumor shedding of MICA/B, which appears to systemically impair the immunological competence of individuals with cancer by causing downregulation of NKG2D. This impairment of effector function promotes tumor immune evasion (46, 47). Targeting the tumor pathways that lead to the upregulation of NKG2D ligands or alternatively maintain and/or upregulate NKG2D receptors may be a productive method to enhance NK cell-based immunotherapy.
The activating DNAM-1 receptor (CD226) belongs to the Ig superfamily and is constitutively expressed on all human NK cells (48). The specific ligands CD112 (nectin-2) and CD155 (polio virus receptor) bind and augment NK cell-mediated cytotoxicity and cytokine release (49, 50). Similarly to MICA, ovarian cancer cells ubiquitously expressing the ligand CD155 show reduced DNAM-1 expression and impaired NK cell function (51). As our understanding of these signaling and inhibitory pathways expands, our potential targets for NK immunotherapy grow.
Other triggering receptors are the NCRs, which include NKp46 (NCR1, CD335), NKp44 (NCR2, CD336), and NKp30 (NCR3, CD337) (52–56). These receptors have a variety of ligands with various structures. NKp46, the main activating receptor for human NK cells, binds the hemagglutinins on influenza virus-infected cells (57). The human cytomegalovirus pp65 tegument protein was identified as the original ligand for NKp30 and was shown to be responsible for suppression of NK cell cytotoxicity (58). Later on, HLA-B associated transcript 3 protein (BAG6) and B7-H6 (NCR3LG1) were identified as novel surface ligands of NKp30 (59–61). Importantly, B7-H6 is present on a broad spectrum of tumors and may play a role in antitumor immunity (62). In addition, tumor shedding of B7-H6 was demonstrated to be a novel mechanism of immune escape (63). A recent study in patients with ovarian cancer demonstrated B7-H6 tumor ligands were associated with decreased NKp30 expression of tumor-associated NK cells (64). These NK cells demonstrated impaired IFN-γ production and cytolytic function. Together, these findings indicate how NK cells may recognize and kill target cells without the decreased expression of MHC class I protein and serve as a template for designing molecules to stimulate NK-mediated cytotoxicity for tumor immunotherapy. It also exemplifies the important role the tumor microenvironment plays on NK cell function and immune surveillance, which will be elucidated below.
NK Role in Immunosurveillance
In 1909, the German physician Paul Ehrlich predicted the immune system routinely identified and eliminated aberrant cells that would otherwise lead to cancer (65). The theory was revisited with great interest 50 years later following a deeper understanding of tumor immunobiology (66–70). Eventually, the concept of tumor immunosurveillance was experimentally validated with the advancement in mouse genetics and the generation of mAb production (71–73). The complex relationship between the tumor and immune system further expanded to incorporate tumor immunoediting, a process where tumor cells reduce their immunogenicity thereby rendering the immune system incapable of recognizing and destroying the aberrant cells (74). Today, it is accepted both innate and adaptive immunity play vital roles in continuously monitoring tissues to eliminate aberrant tumor cells (73).
Some of the earliest experimental evidence detailing the role NK cells play in tumor control and immunosurveillance was obtained in mice. An early study demonstrated beige (bg) mice with 75 NK cell activity resulted in increased tumor growth rate and metastasis compared to control mice with normal NK cell function (75). The presence of NK cell activity also correlated with better control of in vivo tumor growth and metastasis, particularly against histocompatibility complex (MHC) class I-deficient variants (76). In addition, mice depleted of NK cells by the anti-asialo-GM1 mAb resulted in a twofold to threefold increase in 3′-methylcholanthrene (MCA)-induced tumorigenesis compared to wild-type controls (77). Similarly, mice with defective NK function due to a deficiency of NK1.1+CD3− cells but with functionally normal B, T, and NK/T cells showed impaired in vivo rejection of tumor cells (78).
More recently, a prospective cohort of 3,625 individuals were assessed for natural cytotoxic activity of peripheral blood lymphocytes and then followed for 11 years to observe the incidence of cancer (79). These results indicate individuals with impaired NK cell function display an increased risk of developing cancer. In an alternative study, NK cells were identified to also play a role in surveillance against DNA damage through checkpoint pathways (44). The DNA damage response is activated early in tumorigenesis and induces surface expression of ligands for an activating receptor of NK cells, providing a link between the innate immune system and tumor surveillance (80). Together, these studies highlight the contribution NK cell effector function plays in immune protection from tumor development.
It is now established that NK cells participate in first-line defense against tumor development. NK cells are able to discriminate self and non-self due to a wide array of cell surface receptors that control their response, particularly the MHC class I molecules (38). In a 1986 observation, NK cells eliminated MHC class I-deficient cells but not cells with normal MHC class I expression (81). This seminal observation was termed the “missing-self” hypothesis; in the absence of critical surface proteins cells are recognized and eliminated by NK cells (82, 83). This feature is important because solid tumors undergoing malignant transformation frequently reduce MHC class I expression and this process represents one of the main mechanisms for tumor cells to avoid detection by the adaptive immune system (84–88). Thus, one key function of NK cells is to monitor the integrity of MHC class I expression on tumor cells. The significance uncovered in the “missing-self” hypothesis was NK cell effector function is actively inhibited with engagement of MHC class I molecules on cells. More importantly for solid tumor malignancies, newer evidence suggests inhibitory MHC class I receptors only dampen, rather than eliminate, the effector function of NK cells (89–91). This suggests a sufficient activating signal either through a single potent stimulation or the simultaneous engagement of multiple activating receptors is capable of mediating NK cell effector function to eliminate target cells despite MHC class I expression. Thus, a modified “missing-self” hypothesis states “NK cells patrol for abnormal cells that lack MHC class I or overexpress ligands for activating NK cell receptors” (38).
The Tumor Microenvironment and Its Impact on NK Function
The malignant transformation of normal cells results from a multifactorial process resulting in genomic instability and a modification of immunosurveillance mechanisms that induce tolerance (92). As tumors evolve, they develop different strategies to escape the immune response: (i) the secretion of immunosuppressive cytokines or soluble tumor-derived inhibitory factors, (ii) the expression of co-inhibitory or loss of co-stimulatory receptors, and (iii) the loss or downregulation of MHC class I molecules (84). To be effective, NK cells must first extravasate through the vessel endothelial lining and migrate to the tumor tissue (93). Any localizing defect can lead to insufficient numbers of NK cells to the primary or metastatic sites. Once in the extravascular space, NK cells encounter hypoxia, acidic pH, and low glucose conditions that are hostile to immune effector cell function (94). Peripheral blood NK cells and tissue NK cells are notably different; tumor NK cells are functionally defective, incompletely activated, or anergic compared to peripheral blood effectors. In addition, the effectors found within solid tumors are often in limited supply. Further understanding the mechanisms of tissue immunity and its impact on NK cells will be important in our ability to treat solid tumors therapeutically with immunotherapy.
Here, we will discuss how the tumor microenvironment limits the effectiveness of the NK cell antitumor response with attention to ovarian cancer, cervical cancer and endometrial cancer.
Epithelial Ovarian Carcinoma
Ovarian cancer is the most lethal gynecologic malignancy, with an estimated 14,080 deaths expected in the United States for 2017 (95). Despite optimal treatment with surgery and adjuvant chemotherapy, the recurrence rate approaches 70–80% (96). Although the disease tends to remain confined to the abdominal cavity, women with recurrent ovarian cancer progress and ultimately die. Therefore, there is an urgency to develop new and effective therapies.
Important to effective therapeutic development is the understanding of the immunologic interactions within the tumor and its physiologic impacts, which often includes the development of profuse ascites. It is well documented that the ascites fluid from patients with advanced stage ovarian cancer suppress the function of otherwise normal immune effectors, including NK cells (97–101). The ascites contains large numbers of growth factors and cytokines that promote the proliferation of tumor cells (102–104). While fresh NK cells (CD56+CD3−CD16+) isolated from the ascites fluid are found in relatively high concentrations compared to peripheral blood, they are functionally deficient (105, 106). This population of NK cells demonstrates decreased CD16 expression and have reduced proliferative, cytolytic, and cytokine production compared to peripheral blood NK cells (107). Signaling proteins vital to interpreting the activating and inhibitory signals become defective and alter the expression of cytokine transcripts and proteins (100). As detailed above, tumor-associated ligands MICA/B and B7-H6 are often found within the peritoneal fluid of serous ovarian cancer patients and impair NK cell effector function (46, 64). These deficiencies act in combination and likely influence the ability to control the spread and proliferation of tumor cells within the peritoneal cavity of patients with advanced ovarian cancer.
For ovarian cancer, the role of the immune response has been well documented with immunohistochemistry. Multiple studies document a positive correlation between the number of tumor-infiltrating lymphocytes within the tumor and overall survival (OS) (108–110). The absence of CTL infiltration (CTL) also predicts platinum resistance (111). More recently, genomic profiling studies also support using immunophenotype as a method to predict response to therapy and clinical outcomes (112–114). However, most of the published studies document limited infiltration of NK cells within the primary ovarian tumor and cells that suppress immune response and support tumor growth dominate (108, 115–120). The presence of infiltrating NK cells impact on OS is also controversial. Infiltrating NK cells have largely not been associated with better outcomes, and in one case, predicted worse OS (121). However, it was recently shown that CD103+ tumor-infiltrating NK cells were almost always found with CD8+ T cells and were the second best predictor of positive outcomes in primary ovarian cancer (122). In light of this evidence, further study regarding the role of infiltrating NK cells is warranted. Regardless of the uncertainty of the role of NK cells and OS, freshly isolated NK cells from the tumor are drastically impaired through a variety of mechanisms (123, 124).
Within the tumor tissue, NK cells have complex interactions with other immune cells with suppressive functions, including myeloid-derived suppressor cells (MDSCs) and regulatory T cells (Treg) (125). The MDSCs typically suppress effector function of T cells, thereby promoting tumor growth. In mice, a subset of MDSCs expresses the NKG2D ligand Rae-1 and is capable of elimination through NK cell-mediated cytolysis (126). If present, NK cells are also potent producers of IFN-γ and prevent the macrophage polarization toward the M2 phenotype that support tumor progression (127).
Another mechanism interfering with NK effector function within the microenvironment is the secretion of immunosuppressive cytokines. The hypoxic environment induces transcription of interleukin (IL)-8, a chemokine important for tumor growth, angiogenesis, and metastasis (128–130). Women with ovarian cancer have significantly elevated concentrations of IL-8 compared to benign controls (131). In addition to promoting angiogenesis and tumor growth, in vitro studies demonstrate an immunosuppressive effect of IL-8 by inhibiting TNF-induced apoptosis (132). In addition, the cytokine transforming growth factor-β (TGF-β) can contribute to the immunosuppressive microenvironment (133). Overproduction of TGF-β by tumor cells suppresses CD16-mediated NK cell IFN-γ production and ADCC (134). These immunosuppressive cytokines attenuate NK cell effector function and limit the antitumor response.
Cervical Carcinoma
Cervical cancer is a human papillomavirus (HPV)-induced cancer. Mediated by an adaptive immune response against viral proteins, the greatest success involving immunotherapy in gynecologic malignancies is the development of vaccines against HPV and prevention of cancer (135). The two early viral proteins E6 and E7 are defined tumor-associated antigens and are processed and expressed on MHC class I molecules. However, HPV-induced cervical cancers often show altered expression of MHC class I molecules resulting in the inability of CTLs to recognize the peptide epitopes (88, 136–138). The HPV16 E6 and E7 oncoproteins also inhibit NK cell IL-18-induced IFN-γ production likely contributing to viral pathogenesis (139). In addition, HPV infection is non-lytic and produces only a modest inflammatory infiltrate of macrophages and lymphocytes (140). These changes likely affect the efficacy of the innate immune response and provide opportunities to escape immune surveillance. However, the “loss of self” may render these HPV-related cancers susceptible to NK cell attack.
Very little is known in regard to NK cell function and phenotype in women with cervical cancer. Freshly isolated peripheral blood NK cells in women with cervical cancer and benign healthy controls demonstrated no significant functional differences until the patient had distant metastatic disease (stage IVb) (141). Another study demonstrated infiltrating NK cells of patients with cervical cancer were present and of the CD56brightCD16− phenotype. They also observed the upregulation of the DNAM-1 ligand CD155 and the NKG2D ligand MICA in cervical cancer but not in cervical intraepithelial neoplasia or normal controls (142). A follow-up study demonstrated keratinocyte expression of HPV16 E6 and E7 produced rapid induction of intracellular adhesion molecule-1 protein levels (143). NK cells recognize expression of these ligands and adhesion molecules and may be a promising strategy to target for the treatment of cervical cancer.
Interestingly, certain combinations of KIRs and HLA loci associated with NK cell activation increase the risk of developing cervical cancer (144). Specifically, the presence of the activating KIR3DS1 on NK cells in the absence of ligands for inhibitory KIRs results in an increased risk of cervical neoplasia. In constrast, NK cell effector inhibition mediated by KIR2DL1 and KIR3DL1 in the absence of KIR3DS1 results in protection from cervical neoplasia. KIR receptors and HLA ligands interact through an epistatic relationship in which HLA ligands activate a genetic molecular cascade through the KIR receptor that influences NK functionality. This KIR/HLA interaction suggests that an inappropriate inflammatory response, mediated by NK cell KIR-ligand interactions, may lead to tumor progression. The precise role of NK cells in the context of cervical cancer is far from being defined.
Endometrial Carcinoma
Endometrial carcinoma arises from the lining of the uterine cavity and is the fourth most common malignancy in women (95). Most women are diagnosed with low-grade, early stage disease and are cured following surgery. There is very little information on the microenvironment of uterine NK (uNK) cells and cancer. There is much more known about NK cell biology and pregnancy.
Uterine NK cells are a tissue-specific, specialized population of cells that make up large percentage of both endometrial and decidual lymphocytes (145). uNK cells are almost exclusively CD56brightCD16−, although they contain cytotoxic granules (146). uNK cells are thought to contribute to immunosuppressive mechanisms during pregnancy when immune tolerance is vital. As a result, these uNK cells also display less cytotoxicity against tumor targets compared to peripheral NK cells (147). uNK cells are not only immunosuppressive during pregnancy in order to protect the fetus but also may play a key role in modulating fetal growth, with activated uNK cells at the maternal–fetal interface producing factors that play a role in the regulation of trophoblast invasion and uterine vascular remodeling. These roles are critical to placental formation and healthy gestation (148, 149). A number of interesting questions remained unanswered with respect to NK cells and uterine cancer: (i) does the immunosuppressive nature of the maternal–fetal interface contribute to developing uterine carcinoma? (ii) Do peripheral NK cells migrate into the uterine cavity with tumorigenesis? (iii) Are uterine tumor cell lines susceptible to NK cell killing? More complete knowledge of the biology and function of uNK cells in endometrial cancer is required prior to developing strategies of NK cell immunotherapy for this malignancy.
NK Immunotherapy in Gynecologic Malignancies
Advances in understanding the NK cell biology and function over the last few decades have resulted in promising new immunotherapeutic approaches for gynecologic malignancies, in particular ovarian cancer. There is scant research published on NK cell-based immunotherapy in cervical and uterine carcinoma at this time. In this section, we will review recent advances in NK cell-based immunotherapy for all gynecologic malignancies highlighting the opportunities and challenges for each cancer.
Early Trials Using Biologic Response Modifiers and Cytokine Therapy
Early clinical trials in ovarian cancer patients aimed to improve the antitumor activity of immune cells through intraperitoneal injection of biological response modifiers, including Corynebacterium parvum, bacillus Calmette-Guerin, leukocyte interferons (IFN), and irradiated autologous and allogeneic tumor cells (150). Overall, these agents had limited success in treatment response with relative toxic side effects. Another novel strategy using an attenuated strain of influenza virus to infect ovarian cancer tumor cell lines was later developed. The nonviable extracts from the tumor cells, termed viral oncolysate, were isolated and then injected intraperitoneally (IP) into patients with ovarian cancer with both clinical and pathological responses noted (151, 152). Follow-up studies noted the viral oncolysate enhanced the NK cell response (153). Although better tolerated, the treatment had limited clinical responses.
Advancement in recombinant DNA technologies led to the purified production of cytokines and their use to treat a variety of malignancies. Today, cytokines are easy to manufacture and administer. While the central goal of cytokine therapy is to potentiate the autologous antitumor response in vivo, they lack specific immunomodulatory effects (154). Table 1 lists selected clinical trials in ovarian cancer evaluating cytokine therapy and NK cell response if reported. The first generation of cytokines were recombinant (r) IFN-α, rIFN-γ, and rIL-2. The published results of IP therapy with recombinant IFN with or without chemotherapy have been comprehensively reviewed by Freedman and colleagues (155). They also reported on eight clinical trials evaluating IP therapy with IL-2 alone or in combination with cellular therapy (discussed below). The results demonstrated IP immunotherapy with cytokines was tolerated but had varying levels of success. Two important features were identified: (i) response was dependent on remaining tumor burden prior to initiation of therapy and (ii) efficacy of first-line therapy is critical in these patients. Most of these early trials did not assess NK cell response to therapy.
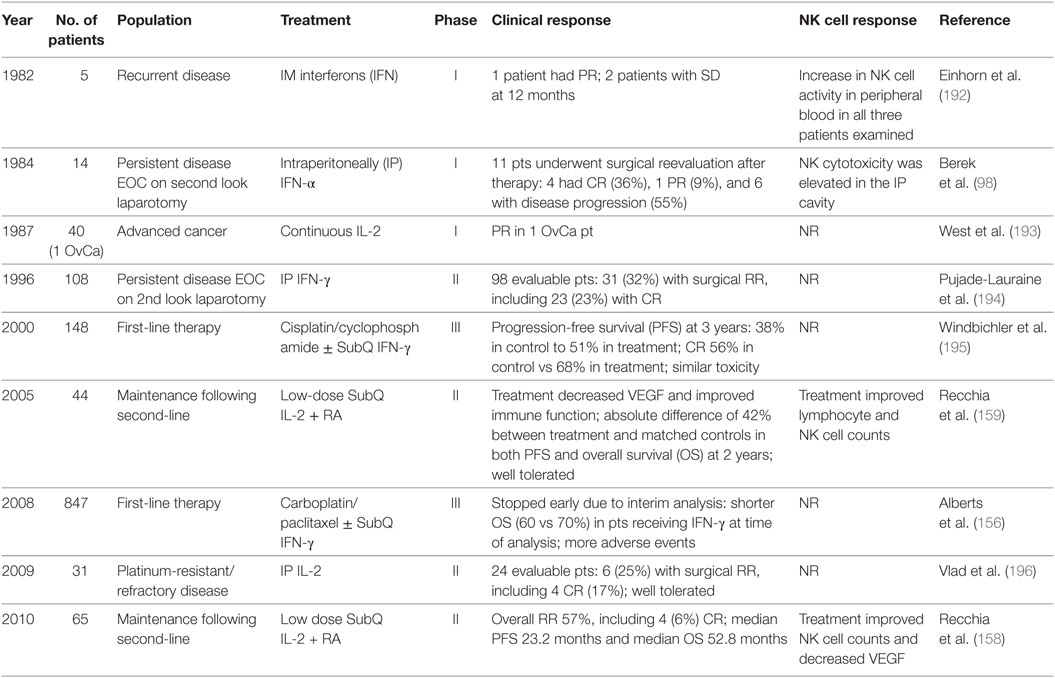
Table 1. Natural killer (NK) cell findings from clinical trials of cytokine immunotherapy for ovarian cancer.
Recently, a randomized phase III trial of 847 women with stage III or IV ovarian cancer evaluating front-line combination carboplatin/paclitaxel plus subcutaneous Escherichia coli-derived recombinant IFNγ-1b was stopped early following a second interim analysis (156). At the time of analysis, patients receiving IFNγ-1b plus chemotherapy compared to chemotherapy alone demonstrated significantly shorter OS (60 vs 70%). It should be noted IFNγ-1b has biological activity identical to natural human IFN-γ (157). The authors speculate IFNγ-1b may have resulted in activation of Tregs and immunosuppression. They also suggest treatment with IFNγ-1b is more toxic and leads to decreased treatment adherence or dose reductions of chemotherapy. The ability to complete all six cycles of chemotherapy was compromised in patients receiving IFNγ-1b (77 vs 83%). Regardless, it was concluded that IFNγ-1b does not have a role in first-line treatment of advanced ovarian cancer.
Finally, a phase II trial of advanced ovarian cancer patients treated with second-line therapy followed by maintenance low-dose subcutaneous IL-2 with oral 13-cis-retinoic acid reported an overall response rate of 57% (158, 159). Treatment was associated with an improvement of peripheral NK cell counts and a decrease in VEGF compared to baseline. In this cohort of 65 patients, the progression-free survival (PFS) and OS was 29 and 38%, respectively.
Similar to IL-2, the cytokine IL-15 can potently increase NK cell numbers. While IL-2 and IL-15 share common signaling mechanisms, they differentially control the development, activation, and proliferation of NK cells (160). IL-2 activates a broad range of T cells, including Tregs. In contrast, IL-15 preferentially stimulates CD8+ T cells and non-terminally differentiated NK cells and has been shown to enhance NK cell function in the ovarian cancer setting (101, 161). Several clinical trials evaluating IL-15 are underway (162).
In summary, biologic response modifiers and cytokine therapy have demonstrated conflicting results in the limited clinical trials. In addition, the small number and heterogeneity of study participants limit interpretations. Additional investigations examining the role of cytokines in ovarian cancer and reporting standard immune and clinical responses is warranted.
Adoptive Transfer of NK Cells
Initial efforts in adoptive transfer of immune cells aimed to improve the autologous antitumor responses through cytokine stimulation (155, 163–165). Immune cells removed from the peripheral blood of patients were activated with various cytokines and then infused back into the same patient. Table 2 lists selected clinical trials in ovarian cancer evaluating adoptive transfer of NK cell-related therapy and response if reported.
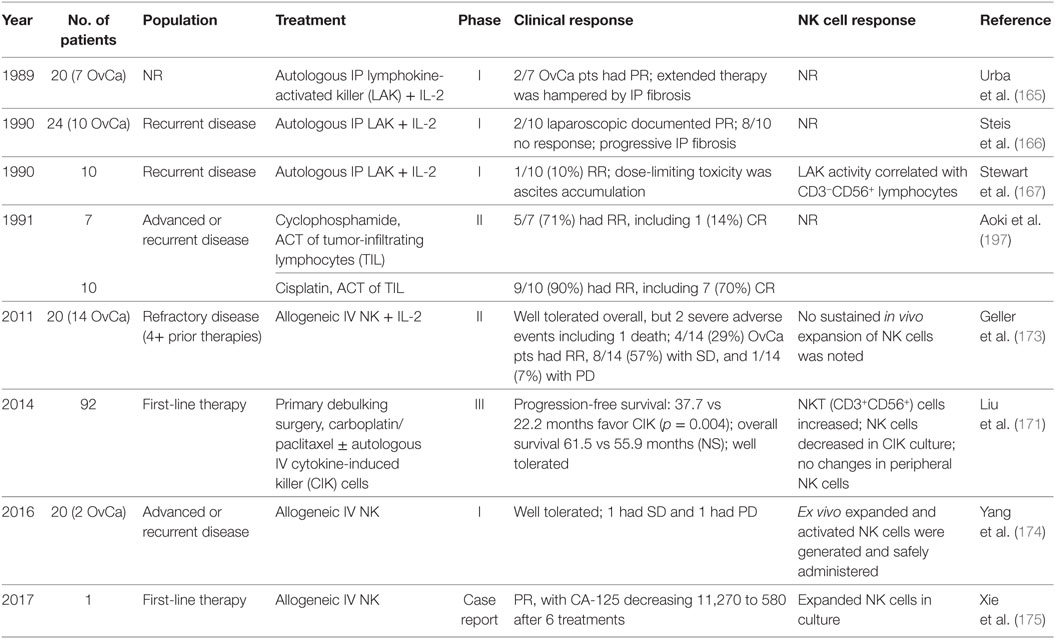
Table 2. Natural killer (NK) cell findings from clinical trials of adoptive cellular transfer for ovarian cancer.
The early phase I clinical trials evaluating the adoptive transfer of autologous lymphokine-activated killer (LAK) cells with high-dose IL-2 therapy demonstrated limited clinical responses with high rates of peritoneal fibrosis (165–167). Similar to LAK immunotherapy, cytokine-induced killer (CIK) cells arise from peripheral blood mononuclear cell cultures with stimulation of anti-CD3 mAb, IFN-γ, and IL-2 (168). CIK cells are characterized by a mixed T-NK phenotype (CD3+CD56+) and demonstrate enhanced cytotoxic activity compared to LAK cells against ovarian and cervical cancer (169, 170). A recent phase III clinical trial investigated adoptive transfer of autologous CIK cells following primary debulking surgery and adjuvant carboplatin/paclitaxel chemotherapy (171). Advanced epithelial ovarian cancer patients (n = 92) were paired to receive maintenance monthly CIK transfusions (n = 46) vs standard of care observation (n = 46). Median PFS was 37.7 months in the treatment group and 22.2 months in the control group (p = 0.004). Median OS in the treatment group was 61.5 months, compared to 55.9 months in the control group (p = 0.289). The therapy was well tolerated with no grade III or IV adverse reactions. Interestingly, the proportion of Tregs in peripheral blood decreased following two courses of immunotherapy (p = 0.006). While only a small, non-randomized phase III study, these results are promising and follow-up studies are warranted.
Recently, insights into the molecular mechanisms regulating NK cell function shifted the focus toward allogeneic NK cell immunotherapy. The mismatch between donor KIR repertoire and recipient MHC class I molecules can improve the antitumor activity of NK cells (172). In a phase II clinical trial, we studied haplo-identical related IV infused NK cells in patients with recurrent ovarian (n = 14) and breast cancer (n = 6) (173). Following a lymphodepleting chemotherapy regimen ± radiation, women received adoptive transfer of a CD3/CD19-depleted NK cell product and were treated with subcutaneous IL-2 injections. No successful NK cell persistence or expansion was noted, likely as a result from recipient Treg expansion and reconstitution following therapy. Only two other small reports using allogeneic NK cell therapy in ovarian cancer have been published (174, 175). Together, these studies suggest allogeneic NK cell therapy is feasible. However, further investigation into strategies to augment in vivo NK cell persistence and expansion are needed.
Future efforts generating novel NK cell products for adoptive transfer are likely to be investigated in the ovarian cancer setting. Ex vivo inhibition of GSK3 kinase in peripheral blood enhances CD57 expression and late-stage maturation of NK cells (176). These NK cells demonstrated significantly higher production of cytokines (TNF-α and IFN-γ) and ADCC when exposed to cancer cells. Recruitment for an ovarian cancer clinical trial using the product generated from this method has opened at the University of Minnesota. Another recent study evaluated the potency of NK cells derived from human CD34+ hematopoietic stem and progenitor cells (HSPC) against a mouse xenograft model for ovarian cancer (177). Mice that received IP HSPC-NK cell infusions had significantly reduced tumor progression compared to controls. Finally, efforts to generate ovarian cancer specific NK chimeric antigen receptors are underway (178). These engineered proteins consist of a fused single-chain variable fragment (scFv) to an intracellular signaling domain to enhance NK effector function. A combination of these different techniques of generating NK cell products hold great promise and may make IP adoptive transfer effective against ovarian cancer following primary cytoreductive surgery and adjuvant chemotherapy.
Other Immunotherapeutic Options to Enhance NK Cell Function
Other immunotherapeutic strategies are currently being characterized for antitumor activity (162). The development of drugs known to influence NK cell presence and function include mAb therapy, immunomodulatory drugs, vaccines (peptide, viral-based, tumor antigens, dendritic cells), and the adoptive transfer of T cells, dendritic cells, and macrophages. Even commonly used cytotoxic agents increase expression of NK cell-activating ligands and enhance NK cell recognition and killing more than others (179). A thorough discussion of each method is outside the scope of this review, but we will comment on several key issues.
Antibody-based immunotherapy has transformed the treatment of many malignancies, but is not yet standard of care for ovarian cancer. The mAbs function through two separate mechanisms. First, treatment is aimed at antigens present on tumor cells to facilitate an antitumor response through opsonization and activation of ADCC. Several tumor-associated antigens targeted with mAb for ovarian cancer have been identified, including NY-ESO-1, CA 125 (MUC16), MUC1, and epithelial cell adhesion molecule (EpCAM) (154). mAbs can also function in a non-immune-mediated manner to block vital growth and survival pathways, such as Her2/Neu, membrane folate receptor, and VEGF. Clinical trials evaluating the efficacy of mAbs should include investigations into both mechanisms.
A newer approach involves engineered bispecific antibodies and bispecific/trispecific killer engagers (BiKEs or TriKEs), which are molecules that cross-link antigens on tumor cells with CD16 on NK cells, activating and enhancing ADCC (180). One example utilized anti-CD16 scFv spliced to anti-EpCAM scFv (181). This BiKE promoted immune synapse and ADCC between NK cells and EpCAM-expressing tumor cells. More recently, a fully humanized TriKE utilized a modified IL-15 to cross-link the anti-CD16 scFv and EpCAM scFv (182). The 1615EpCAM TriKE was specific and active against EpCAM bearing ovarian cancer cells and mediated NK proliferation, sustained ADCC activity, improved lytic degranulation, and cytokine production. A TetraKE construct incorporating the cancer stem cell marker anti-CD133 scFv was recently engineered to simultaneously target EpCAM and CD133 bearing cells (183). These engineered small molecules combine the specificity of mAbs with the NK cell expansion and survival benefits of cytokine therapies via IL-15 into a single product. This novel strategy to target NK cells for antigen-specific immunotherapy has recently been reviewed and will hopefully prove effective in supplementing traditional therapies against gynecologic malignancies (184, 185).
Immune checkpoints are inhibitory pathways that serve to prevent self-tissue damage. During tumorigenesis, cancer cells often express ligands to bind and induce immune suppression. A number of antibodies have been developed to block checkpoint pathways expressed on certain T cells, B cells, monocytes, and NK cells, including CTL-associated protein 4, programmed death protein 1 (PD-1), TIM-3, NKG2A/CD94 complex, and CD96/CD226/TIGIT receptors (186, 187). A recent publication identified a population of NK cells within the ascites of women with ovarian cancer where PD-1 is highly expressed suggesting therapies targeting PD-1/PD-L may be effective (188). In fact, in vitro studies have shown that PD-1 and CD96/TIGIT blockade augments NK cell-mediated tumor lysis (189, 190). Future research is needed to clarify the effects checkpoint inhibitors have on the NK cell response and the potential to enhance adoptive NK cell immunotherapy.
Concluding Remarks
Here, we provide an extensive overview of NK cell-based immunobiology and therapy in gynecologic malignancies. Over the past several decades, insight into biology controlling activation or inhibition has advanced the prospect of NK cell-based immunotherapy, which is just now being realized. Today, there are strategies to harness NK cell function for immunotherapy, including: (i) adoptive transfer of alloreactive NK cells, (ii) blocking NK inhibitory signals with mAb, (iii) promoting death ligand expression, and (iv) enhancing specificity via activation of ADCC. In addition, using drugs or cytokines to promote NK cell proliferation and function or inhibit NK cell suppressors are potential strategies. Complementary approaches also exist to manipulate the genetics geared to maximize NK cell function against specific tumor targets. One example includes viral transduction and gene transfection through electroporation technologies with the goal of increasing production of cytokines or cell receptors (191).
There are several crucial issues that require consideration for adoptive NK cell-based cancer immunotherapy that need to be highlighted. These include (i) standardizing protocols and techniques in NK cell preparation, (ii) establishing firm criteria for donor selection to improve clinical response, (iii) identifying the best method of conditioning recipients to avoid rejection and promote survival of transferred NK cells, (iv) combining NK cell-based immunotherapy with other therapies to eliminate cancer cells, and (v) enhancing understanding of tissue immunity and the tumor microenvironment (3).
Finally, early clinical studies have demonstrated promise of NK cell-based immunotherapy for gynecologic malignancies. Future research will be important to identify patients that will most likely benefit from immunotherapy and define the specific role and timing of therapy. In addition, combination approaches need to be explored and optimized before therapeutic breakthroughs can realistically be envisioned.
Author Contributions
LU and CD wrote the manuscript with contributions from MF and MG. JM, MF, and MG edited the manuscript.
Conflict of Interest Statement
JM reports the following scientific advisory boards: (1) Celgene (2) Fate Therapeutics, and (3) GT BioPharma. He also receives research funding from Fate Therapeutics and GT BioPharma. The remaining coauthors declare that the research was conducted in the absence of any commercial or financial relationships that could be construed as a potential conflict of interest.
Funding
This work was supported by the following grants: NIH R35 CA197292 and U.S. Department of Defense CA150085, the Minnesota Ovarian Cancer Alliance (MOCA) (MG) “NK Cell Immunotherapy for Ovarian Cancer,” Mayo Clinic Ovarian Cancer SPORE (P50 CA136393), and by a Research Scholar Grant (RSG-14-151-01-CCE) from the American Cancer Society.
References
1. Herberman RB, Nunn ME, Holden HT, Lavrin DH. Natural cytotoxic reactivity of mouse lymphoid cells against syngeneic and allogeneic tumors. II. Characterization of effector cells. Int J Cancer (1975) 16(2):230–9. doi:10.1002/ijc.2910160204
2. Kiessling R, Klein E, Pross H, Wigzell H. “Natural” killer cells in the mouse. II. Cytotoxic cells with specificity for mouse Moloney leukemia cells. Characteristics of the killer cell. Eur J Immunol (1975) 5(2):117–21. doi:10.1002/eji.1830050209
3. Ljunggren HG, Malmberg KJ. Prospects for the use of NK cells in immunotherapy of human cancer. Nat Rev Immunol (2007) 7(5):329–39. doi:10.1038/nri2073
4. Knorr DA, Bachanova V, Verneris MR, Miller JS. Clinical utility of natural killer cells in cancer therapy and transplantation. Semin Immunol (2014) 26(2):161–72. doi:10.1016/j.smim.2014.02.002
5. Lanier LL, Phillips JH, Hackett J Jr, Tutt M, Kumar V. Natural killer cells: definition of a cell type rather than a function. J Immunol (1986) 137(9):2735–9.
6. Blum KS, Pabst R. Lymphocyte numbers and subsets in the human blood. Do they mirror the situation in all organs? Immunol Lett (2007) 108(1):45–51. doi:10.1016/j.imlet.2006.10.009
7. Westermann J, Pabst R. Distribution of lymphocyte subsets and natural killer cells in the human body. Clin Investig (1992) 70(7):539–44. doi:10.1007/BF00184787
8. Cooper MA, Fehniger TA, Turner SC, Chen KS, Ghaheri BA, Ghayur T, et al. Human natural killer cells: a unique innate immunoregulatory role for the CD56(bright) subset. Blood (2001) 97(10):3146–51. doi:10.1182/blood.V97.10.3146
9. Smyth MJ, Takeda K, Hayakawa Y, Peschon JJ, van den Brink MR, Yagita H. Nature’s TRAIL – on a path to cancer immunotherapy. Immunity (2003) 18(1):1–6. doi:10.1016/S1074-7613(02)00502-2
10. Raulet DH, Guerra N. Oncogenic stress sensed by the immune system: role of natural killer cell receptors. Nat Rev Immunol (2009) 9(8):568–80. doi:10.1038/nri2604
11. Smyth MJ, Hayakawa Y, Takeda K, Yagita H. New aspects of natural-killer-cell surveillance and therapy of cancer. Nat Rev Cancer (2002) 2(11):850–61. doi:10.1038/nrc928
12. Caligiuri MA. Human natural killer cells. Blood (2008) 112(3):461–9. doi:10.1182/blood-2007-09-077438
13. Vivier E, Raulet DH, Moretta A, Caliguiri MA, Zitvogel L, Lannier LL, et al. Innate or adaptive immunity? The example of natural killer cells. Science (2011) 331(6013):44–9. doi:10.1126/science.1198687
14. Chávez-Galán L, Arenas-Del Angel MC, Zenteno E, Chávez R, Lascurain R. Cell death mechanisms induced by cytotoxic lymphocytes. Cell Mol Immunol (2009) 6(1):15–25. doi:10.1038/cmi.2009.3
15. Smyth MJ, Cretney E, Kelly JM, Westwood JA, Street SE, Yagita H, et al. Activation of NK cell cytotoxicity. Mol Immunol (2005) 42(4):501–10. doi:10.1016/j.molimm.2004.07.034
16. Strome SE, Sausville EA, Mann D. A mechanistic perspective of monoclonal antibodies in cancer therapy beyond target-related effects. Oncologist (2007) 12(9):1084–95. doi:10.1634/theoncologist.12-9-1084
17. Alderson KL, Sondel PM. Clinical cancer therapy by NK cells via antibody-dependent cell-mediated cytotoxicity. J Biomed Biotechnol (2011) 2011:379123. doi:10.1155/2011/379123
18. van Ojik HH, Bevaart L, Dahle CE, Bakker A, Jansen MJ, van Vugt MJ, et al. CpG-A and B oligodeoxynucleotides enhance the efficacy of antibody therapy by activating different effector cell populations. Cancer Res (2003) 63(17):5595–600.
19. Cartron G, Dacheux L, Salles G, Solal-Celigny P, Bardos P, Colombat P, et al. Therapeutic activity of humanized anti-CD20 monoclonal antibody and polymorphism in IgG Fc receptor FcgammaRIIIa gene. Blood (2002) 99(3):754–8. doi:10.1182/blood.V99.3.754
20. Weng WK, Levy R. Two immunoglobulin G fragment C receptor polymorphisms independently predict response to rituximab in patients with follicular lymphoma. J Clin Oncol (2003) 21(21):3940–7. doi:10.1200/JCO.2003.05.013
21. Koene HR, Kleijer M, Algra J, Roos D, von dem Borne AE, de Haas M. Fc gammaRIIIa-158V/F polymorphism influences the binding of IgG by natural killer cell Fc gammaRIIIa, independently of the Fc gammaRIIIa-48L/R/H phenotype. Blood (1997) 90(3):1109–14.
22. Zhang W, Gordon M, Schultheis AM, Yang DY, Nagashima F, Azuma M, et al. FCGR2A and FCGR3A polymorphisms associated with clinical outcome of epidermal growth factor receptor expressing metastatic colorectal cancer patients treated with single-agent cetuximab. J Clin Oncol (2007) 25(24):3712–8. doi:10.1200/JCO.2006.08.8021
23. Bowles JA, Wang SY, Link BK, Allan B, Beuerlein G, Campbell MA, et al. Anti-CD20 monoclonal antibody with enhanced affinity for CD16 activates NK cells at lower concentrations and more effectively than rituximab. Blood (2006) 108(8):2648–54. doi:10.1182/blood-2006-04-020057
24. Oppenheim DE, Spreafico R, Etuk A, Malone D, Amofah E, Peña-Murillo C, et al. Glyco-engineered anti-EGFR mAb elicits ADCC by NK cells from colorectal cancer patients irrespective of chemotherapy. Br J Cancer (2014) 110(5):1221–7. doi:10.1038/bjc.2014.35
25. Chua HL, Serov Y, Brahmi Z. Regulation of FasL expression in natural killer cells. Hum Immunol (2004) 65(4):317–27. doi:10.1016/j.humimm.2004.01.004
26. Arase H, Arase N, Saito T. Fas-mediated cytotoxicity by freshly isolated natural killer cells. J Exp Med (1995) 181(3):1235–8. doi:10.1084/jem.181.3.1235
27. Kayagaki N, Yamaguchi N, Nakayama M, Takeda K, Akiba H, Tsutsui H, et al. Expression and function of TNF-related apoptosis-inducing ligand on murine activated NK cells. J Immunol (1999) 163(4):1906–13.
28. Lundqvist A, Abrams SI, Schrump DS, Alvarez G, Suffredini D, Berg M, et al. Bortezomib and depsipeptide sensitize tumors to tumor necrosis factor-related apoptosis-inducing ligand: a novel method to potentiate natural killer cell tumor cytotoxicity. Cancer Res (2006) 66(14):7317–25. doi:10.1158/0008-5472.CAN-06-0680
29. Sayers TJ, Brooks AD, Koh CY, Ma W, Seki N, Raziuddin A, et al. The proteasome inhibitor PS-341 sensitizes neoplastic cells to TRAIL-mediated apoptosis by reducing levels of c-FLIP. Blood (2003) 102(1):303–10. doi:10.1182/blood-2002-09-2975
30. VanOosten RL, Moore JM, Karacay B, Griffith TS. Histone deacetylase inhibitors modulate renal cell carcinoma sensitivity to TRAIL/Apo-2L-induced apoptosis by enhancing TRAIL-R2 expression. Cancer Biol Ther (2005) 4(10):1104–12. doi:10.4161/cbt.4.10.2022
31. Cooper MA, Fehniger TA, Caligiuri MA. The biology of human natural killer-cell subsets. Trends Immunol (2001) 22(11):633–40. doi:10.1016/S1471-4906(01)02060-9
32. Martín-Fontecha A, Thomsen LL, Brett S, Gerard C, Lipp M, Lanzavecchia A, et al. Induced recruitment of NK cells to lymph nodes provides IFN-gamma for T(H)1 priming. Nat Immunol (2004) 5(12):1260–5. doi:10.1038/ni1138
33. Mocikat R, Braumüller H, Gumy A, Egeter O, Ziegler H, Reusch U, et al. Natural killer cells activated by MHC class I(low) targets prime dendritic cells to induce protective CD8 T cell responses. Immunity (2003) 19(4):561–9. doi:10.1016/S1074-7613(03)00264-4
34. Radaev S, Sun PD. Structure and function of natural killer cell surface receptors. Annu Rev Biophys Biomol Struct (2003) 32:93–114. doi:10.1146/annurev.biophys.32.110601.142347
35. Long EO. Negative signaling by inhibitory receptors: the NK cell paradigm. Immunol Rev (2008) 224:70–84. doi:10.1111/j.1600-065X.2008.00660.x
36. Lanier LL. Up on the tightrope: natural killer cell activation and inhibition. Nat Immunol (2008) 9(5):495–502. doi:10.1038/ni1581
37. Parham P. MHC class I molecules and KIRs in human history, health and survival. Nat Rev Immunol (2005) 5(3):201–14. doi:10.1038/nri1570
38. Lanier LL. NK cell recognition. Annu Rev Immunol (2005) 23:225–74. doi:10.1146/annurev.immunol.23.021704.115526
39. López-Botet M, Llano M, Navarro F, Bellón T. NK cell recognition of non-classical HLA class I molecules. Semin Immunol (2000) 12(2):109–19. doi:10.1006/smim.2000.0213
40. Moretta A, Bottino C, Vitale M, Pende D, Biassoni R, Mingari MC, et al. Receptors for HLA class-I molecules in human natural killer cells. Annu Rev Immunol (1996) 14:619–48. doi:10.1146/annurev.immunol.14.1.619
41. Valés-Gómez M, Reyburn HT, Erskine RA, López-Botet M, Strominger JL. Kinetics and peptide dependency of the binding of the inhibitory NK receptor CD94/NKG2-A and the activating receptor CD94/NKG2-C to HLA-E. EMBO J (1999) 18(15):4250–60. doi:10.1093/emboj/18.15.4250
42. Moretta A, Bottino C, Vitale M, Pende D, Cantoni C, Mingari MC, et al. Activating receptors and coreceptors involved in human natural killer cell-mediated cytolysis. Annu Rev Immunol (2001) 19:197–223. doi:10.1146/annurev.immunol.19.1.197
43. Marcenaro E, Augugliaro R, Falco M, Castriconi R, Parolini S, Sivori S, et al. CD59 is physically and functionally associated with natural cytotoxicity receptors and activates human NK cell-mediated cytotoxicity. Eur J Immunol (2003) 33(12):3367–76. doi:10.1002/eji.200324425
44. Gasser S, Orsulic S, Brown EJ, Raulet DH. The DNA damage pathway regulates innate immune system ligands of the NKG2D receptor. Nature (2005) 436(7054):1186–90. doi:10.1038/nature03884
45. Groh V, Rhinehart R, Secrist H, Bauer S, Grabstein KH, Spies T. Broad tumor-associated expression and recognition by tumor-derived gamma delta T cells of MICA and MICB. Proc Natl Acad Sci U S A (1999) 96(12):6879–84. doi:10.1073/pnas.96.12.6879
46. Groh V, Wu J, Yee C, Spies T. Tumour-derived soluble MIC ligands impair expression of NKG2D and T-cell activation. Nature (2002) 419(6908):734–8. doi:10.1038/nature01112
47. Salih HR, Rammensee HG, Steinle A. Cutting edge: down-regulation of MICA on human tumors by proteolytic shedding. J Immunol (2002) 169(8):4098–102. doi:10.4049/jimmunol.169.8.4098
48. Shibuya A, Campbell D, Hannum C, Yssel H, Franz-Bacon K, McClanahan T, et al. DNAM-1, a novel adhesion molecule involved in the cytolytic function of T lymphocytes. Immunity (1996) 4(6):573–81. doi:10.1016/S1074-7613(00)70060-4
49. Bottino C, Castriconi R, Pende D, Rivera P, Nanni M, Carnemolla B, et al. Identification of PVR (CD155) and nectin-2 (CD112) as cell surface ligands for the human DNAM-1 (CD226) activating molecule. J Exp Med (2003) 198(4):557–67. doi:10.1084/jem.20030788
50. Tahara-Hanaoka S, Shibuya K, Onoda Y, Zhang H, Yamazaki S, Miyamoto A, et al. Functional characterization of DNAM-1 (CD226) interaction with its ligands PVR (CD155) and nectin-2 (PRR-2/CD112). Int Immunol (2004) 16(4):533–8. doi:10.1093/intimm/dxh059
51. Carlsten M, Norell H, Bryceson YT, Poschke I, Schedvins K, Ljunggren HG, et al. Primary human tumor cells expressing CD155 impair tumor targeting by down-regulating DNAM-1 on NK cells. J Immunol (2009) 183(8):4921–30. doi:10.4049/jimmunol.0901226
52. Sivori S, Vitale M, Morelli L, Sanseverino L, Augugliaro R, Bottino C, et al. p46, a novel natural killer cell-specific surface molecule that mediates cell activation. J Exp Med (1997) 186(7):1129–36. doi:10.1084/jem.186.7.1129
53. Pessino A, Sivori S, Bottino C, Malaspina A, Morelli L, Moretta L, et al. Molecular cloning of NKp46: a novel member of the immunoglobulin superfamily involved in triggering of natural cytotoxicity. J Exp Med (1998) 188(5):953–60. doi:10.1084/jem.188.5.953
54. Vitale M, Bottino C, Sivori S, Sanseverino L, Castriconi R, Marcenaro E, et al. NKp44, a novel triggering surface molecule specifically expressed by activated natural killer cells, is involved in non-major histocompatibility complex-restricted tumor cell lysis. J Exp Med (1998) 187(12):2065–72. doi:10.1084/jem.187.12.2065
55. Cantoni C, Bottino C, Vitale M, Pessino A, Augugliaro R, Malaspina A, et al. NKp44, a triggering receptor involved in tumor cell lysis by activated human natural killer cells, is a novel member of the immunoglobulin superfamily. J Exp Med (1999) 189(5):787–96. doi:10.1084/jem.189.5.787
56. Pende D, Parolini S, Pessino A, Sivori S, Augugliaro R, Morelli L, et al. Identification and molecular characterization of NKp30, a novel triggering receptor involved in natural cytotoxicity mediated by human natural killer cells. J Exp Med (1999) 190(10):1505–16. doi:10.1084/jem.190.10.1505
57. Mandelboim O, Lieberman N, Lev M, Paul L, Arnon TI, Bushkin Y, et al. Recognition of haemagglutinins on virus-infected cells by NKp46 activates lysis by human NK cells. Nature (2001) 409(6823):1055–60. doi:10.1038/35059110
58. Arnon TI, Achdout H, Levi O, Markel G, Saleh N, Katz G, et al. Inhibition of the NKp30 activating receptor by pp65 of human cytomegalovirus. Nat Immunol (2005) 6(5):515–23. doi:10.1038/ni1190
59. Pogge von Strandmann E, Simhadri VR, von Tresckow B, Sasse S, Reiners KS, Hansen HP, et al. Human leukocyte antigen-B-associated transcript 3 is released from tumor cells and engages the NKp30 receptor on natural killer cells. Immunity (2007) 27(6):965–74. doi:10.1016/j.immuni.2007.10.010
60. Simhadri VR, Reiners KS, Hansen HP, Topolar D, Simhadri VL, Nohroudi K, et al. Dendritic cells release HLA-B-associated transcript-3 positive exosomes to regulate natural killer function. PLoS One (2008) 3(10):e3377. doi:10.1371/journal.pone.0003377
61. Brandt CS, Baratin M, Yi EC, Kennedy J, Gao Z, Fox B, et al. The B7 family member B7-H6 is a tumor cell ligand for the activating natural killer cell receptor NKp30 in humans. J Exp Med (2009) 206(7):1495–503. doi:10.1084/jem.20090681
62. Kaifu T, Escalière B, Gastinel LN, Vivier E, Baratin M. B7-H6/NKp30 interaction: a mechanism of alerting NK cells against tumors. Cell Mol Life Sci (2011) 68(21):3531–9. doi:10.1007/s00018-011-0802-7
63. Schlecker E, Fiegler N, Arnold A, Altevogt P, Rose-John S, Moldenhauer G, et al. Metalloprotease-mediated tumor cell shedding of B7-H6, the ligand of the natural killer cell-activating receptor NKp30. Cancer Res (2014) 74(13):3429–40. doi:10.1158/0008-5472.CAN-13-3017
64. Pesce S, Tabellini G, Cantoni C, Patrizi O, Coltrini D, Rampinelli F, et al. B7-H6-mediated downregulation of NKp30 in NK cells contributes to ovarian carcinoma immune escape. Oncoimmunology (2015) 4(4):e1001224. doi:10.1080/2162402X.2014.1001224
65. Ehrlich P. Über den jetzigen Stand der Karzinomforschung. Beiträge zur experimentellen Pathologie und Chemotherapie. Leipzig: Verlagsgesellschaft (1909). p. 117–64.
66. Burnet M. Cancer; a biological approach. I. The processes of control. Br Med J (1957) 1(5022):779–86. doi:10.1136/bmj.1.5022.779
67. Burnet M. Immunological factors in the process of carcinogenesis. Br Med Bull (1964) 20:154–8. doi:10.1093/oxfordjournals.bmb.a070310
68. Burnet FM. The concept of immunological surveillance. Prog Exp Tumor Res (1970) 13:1–27. doi:10.1159/000386035
70. Thomas L. Delayed Hypersensitivity in Health and Disease. Cellular and Humoral Aspects of the Hypersensitive States. New York: Hoeber-Harper (1959). p. 529–32.
71. Smyth MJ, Godfrey DI, Trapani JA. A fresh look at tumor immunosurveillance and immunotherapy. Nat Immunol (2001) 2(4):293–9. doi:10.1038/86297
72. Dunn GP, Bruce AT, Ikeda H, Old LJ, Schreiber RD. Cancer immunoediting: from immunosurveillance to tumor escape. Nat Immunol (2002) 3(11):991–8. doi:10.1038/ni1102-991
73. Dunn GP, Old LJ, Schreiber RD. The immunobiology of cancer immunosurveillance and immunoediting. Immunity (2004) 21(2):137–48. doi:10.1016/j.immuni.2004.07.017
74. Shankaran V, Ikeda H, Bruce AT, White JM, Swanson PE, Old LJ, et al. IFNgamma and lymphocytes prevent primary tumour development and shape tumour immunogenicity. Nature (2001) 410(6832):1107–11. doi:10.1038/35074122
75. Talmadge JE, Meyers KM, Prieur DJ, Starkey JR. Role of NK cells in tumour growth and metastasis in beige mice. Nature (1980) 284(5757):622–4. doi:10.1038/284622a0
76. Smyth MJ, Thia KY, Street SE, Cretney E, Trapani JA, Taniguchi M, et al. Differential tumor surveillance by natural killer (NK) and NKT cells. J Exp Med (2000) 191(4):661–8. doi:10.1084/jem.191.4.661
77. Smyth MJ, Crowe NY, Godfrey DI. NK cells and NKT cells collaborate in host protection from methylcholanthrene-induced fibrosarcoma. Int Immunol (2001) 13(4):459–63. doi:10.1093/intimm/13.4.459
78. Kim S, Iizuka K, Aguila HL, Weissman IL, Yokoyama WM. In vivo natural killer cell activities revealed by natural killer cell-deficient mice. Proc Natl Acad Sci U S A (2000) 97(6):2731–6. doi:10.1073/pnas.050588297
79. Imai K, Matsuyama S, Miyake S, Suga K, Nakachi K. Natural cytotoxic activity of peripheral-blood lymphocytes and cancer incidence: an 11-year follow-up study of a general population. Lancet (2000) 356(9244):1795–9. doi:10.1016/S0140-6736(00)03231-1
80. Gasser S, Raulet D. The DNA damage response, immunity and cancer. Semin Cancer Biol (2006) 16(5):344–7. doi:10.1016/j.semcancer.2006.07.004
81. Kärre K, Ljunggren HG, Piontek G, Kiessling R. Selective rejection of H-2-deficient lymphoma variants suggests alternative immune defence strategy. Nature (1986) 319(6055):675–8. doi:10.1038/319675a0
82. Karre K. On the Immunobiology of Natural Killer Cells. Stockholm, Sweden: Karolinska Institute (1981).
83. Ljunggren HG, Karre K. In search of the ‘missing self’: MHC molecules and NK cell recognition. Immunol Today (1990) 11(7):237–44.
84. Seliger B. Different regulation of MHC class I antigen processing components in human tumors. J Immunotoxicol (2008) 5(4):361–7. doi:10.1080/15476910802482870
85. Chang CC, Campoli M, Ferrone S. HLA class I defects in malignant lesions: what have we learned? Keio J Med (2003) 52(4):220–9. doi:10.2302/kjm.52.220
86. Karre K. Express yourself or die: peptides, MHC molecules, and NK cells. Science (1995) 267(5200):978–9. doi:10.1126/science.7863341
87. Raulet DH, Held W. Natural killer cell receptors: the offs and ons of NK cell recognition. Cell (1995) 82(5):697–700. doi:10.1016/0092-8674(95)90466-2
88. Aptsiauri N, Cabrera T, Mendez R, Garcia-Lora A, Ruiz-Cabello F, Garrido F. Role of altered expression of HLA class I molecules in cancer progression. Adv Exp Med Biol (2007) 601:123–31. doi:10.1007/978-0-387-72005-0_13
89. Lanier LL, Corliss B, Phillips JH. Arousal and inhibition of human NK cells. Immunol Rev (1997) 155:145–54. doi:10.1111/j.1600-065X.1997.tb00947.x
90. Diefenbach A, Jensen ER, Jamieson AM, Raulet DH. Rae1 and H60 ligands of the NKG2D receptor stimulate tumour immunity. Nature (2001) 413(6852):165–71. doi:10.1038/35093109
91. Cerwenka A, Baron JL, Lanier LL. Ectopic expression of retinoic acid early inducible-1 gene (RAE-1) permits natural killer cell-mediated rejection of a MHC class I-bearing tumor in vivo. Proc Natl Acad Sci U S A (2001) 98(20):11521–6. doi:10.1073/pnas.201238598
92. Hanahan D, Weinberg RA. The hallmarks of cancer. Cell (2000) 100(1):57–70. doi:10.1016/S0092-8674(00)81683-9
93. Timonen T. Natural killer cells: endothelial interactions, migration, and target cell recognition. J Leukoc Biol (1997) 62(6):693–701.
94. Lardner A. The effects of extracellular pH on immune function. J Leukoc Biol (2001) 69(4):522–30.
95. Siegel RL, Miller KD, Jemal A. Cancer statistics, 2017. CA Cancer J Clin (2017) 67(1):7–30. doi:10.3322/caac.21387
96. du Bois A, Reuss A, Pujade-Lauraine E, Harter P, Ray-Coquard I, Pfisterer J. Role of surgical outcome as prognostic factor in advanced epithelial ovarian cancer: a combined exploratory analysis of 3 prospectively randomized phase 3 multicenter trials: by the Arbeitsgemeinschaft Gynaekologische Onkologie Studiengruppe Ovarialkarzinom (AGO-OVAR) and the Groupe d’Investigateurs Nationaux Pour les Etudes des Cancers de l’Ovaire (GINECO). Cancer (2009) 115(6):1234–44. doi:10.1002/cncr.24149
97. Badger AM, Oh SK, Moolten FR. Differential effects of an immunosuppressive fraction from ascites fluid of patients with ovarian cancer on spontaneous and antibody-dependent cytotoxicity. Cancer Res (1981) 41(3):1133–9.
98. Berek JS, Bast RC Jr, Lichtenstein A, Hacker NF, Spina CA, Lagasse LD, et al. Lymphocyte cytotoxicity in the peritoneal cavity and blood of patients with ovarian cancer. Obstet Gynecol (1984) 64(5):708–14.
99. Heo DS, Whiteside TL, Kanbour A, Herberman RB. Lymphocytes infiltrating human ovarian tumors. I. Role of Leu-19 (NKH1)-positive recombinant IL-2-activated cultures of lymphocytes infiltrating human ovarian tumors. J Immunol (1988) 140(11):4042–9.
100. Rabinowich H, Suminami Y, Reichert TE, Crowley-Nowick P, Bell M, Edwards R, et al. Expression of cytokine genes or proteins and signaling molecules in lymphocytes associated with human ovarian carcinoma. Int J Cancer (1996) 68(3):276–84. doi:10.1002/(SICI)1097-0215(19961104)68:3<276::AID-IJC2>3.0.CO;2-Z
101. Felices M, Chu S, Kodal B, Bendzick L, Ryan C, Lenvik AJ, et al. IL-15 super-agonist (ALT-803) enhances natural killer (NK) cell function against ovarian cancer. Gynecol Oncol (2017) 145(3):453–61. doi:10.1016/j.ygyno.2017.02.028
102. Mills GB, May C, McGill M, Roifman CM, Mellors A. A putative new growth factor in ascitic fluid from ovarian cancer patients: identification, characterization, and mechanism of action. Cancer Res (1988) 48(5):1066–71.
103. Mills GB, May C, Hill M, Campbell S, Shaw P, Marks A. Ascitic fluid from human ovarian cancer patients contains growth factors necessary for intraperitoneal growth of human ovarian adenocarcinoma cells. J Clin Invest (1990) 86(3):851–5. doi:10.1172/JCI114784
104. Westermann AM, Beijnen JH, Moolenaar WH, Rodenhuis S. Growth factors in human ovarian cancer. Cancer Treat Rev (1997) 23(2):113–31. doi:10.1016/S0305-7372(97)90024-4
105. Ioannides CG, Platsoucas CD, Rashed S, Wharton JT, Edwards CL, Freedman RS. Tumor cytolysis by lymphocytes infiltrating ovarian malignant ascites. Cancer Res (1991) 51(16):4257–65.
106. Lukesova S, Vroblova V, Tosner J, Kopecky J, Sedlakova I, Cermakova E, et al. Comparative study of various subpopulations of cytotoxic cells in blood and ascites from patients with ovarian carcinoma. Contemp Oncol (Pozn) (2015) 19(4):290–9. doi:10.5114/wo.2015.54388
107. Lai P, Rabinowich H, Crowley-Nowick PA, Bell MC, Mantovani G, Whiteside TL. Alterations in expression and function of signal-transducing proteins in tumor-associated T and natural killer cells in patients with ovarian carcinoma. Clin Cancer Res (1996) 2(1):161–73.
108. Zhang L, Conejo-Garcia JR, Katsaros D, Gimotty PA, Massobrio M, Regnani G, et al. Intratumoral T cells, recurrence, and survival in epithelial ovarian cancer. N Engl J Med (2003) 348(3):203–13. doi:10.1056/NEJMoa020177
109. Sato E, Olson SH, Ahn J, Bundy B, Nishikawa H, Qian F, et al. Intraepithelial CD8+ tumor-infiltrating lymphocytes and a high CD8+/regulatory T cell ratio are associated with favorable prognosis in ovarian cancer. Proc Natl Acad Sci U S A (2005) 102(51):18538–43. doi:10.1073/pnas.0509182102
110. Hamanishi J, Mandai M, Iwasaki M, Okazaki T, Tanaka Y, Yamaguchi K, et al. Programmed cell death 1 ligand 1 and tumor-infiltrating CD8+ T lymphocytes are prognostic factors of human ovarian cancer. Proc Natl Acad Sci U S A (2007) 104(9):3360–5. doi:10.1073/pnas.0611533104
111. Mariya T, Hirohashi Y, Torigoe T, Asano T, Kuroda T, Yasuda K, et al. Prognostic impact of human leukocyte antigen class I expression and association of platinum resistance with immunologic profiles in epithelial ovarian cancer. Cancer Immunol Res (2014) 2(12):1220–9. doi:10.1158/2326-6066.CIR-14-0101
112. Cancer Genome Atlas Research Network. Integrated genomic analyses of ovarian carcinoma. Nature (2011) 474(7353):609–15. doi:10.1038/nature10166
113. Tothill RW, Tinker AV, George J, Brown R, Fox SB, Lade S, et al. Novel molecular subtypes of serous and endometrioid ovarian cancer linked to clinical outcome. Clin Cancer Res (2008) 14(16):5198–208. doi:10.1158/1078-0432.CCR-08-0196
114. Eng KH, Tsuji T. Differential antigen expression profile predicts immunoreactive subset of advanced ovarian cancers. PLoS One (2014) 9(11):e111586. doi:10.1371/journal.pone.0111586
115. Haskill S, Becker S, Fowler W, Walton L. Mononuclear-cell infiltration in ovarian cancer. I. Inflammatory-cell infiltrates from tumour and ascites material. Br J Cancer (1982) 45(5):728–36. doi:10.1038/bjc.1982.115
116. Introna M, Allavena P, Biondi A, Colombo N, Villa A, Mantovani A. Defective natural killer activity within human ovarian tumors: low numbers of morphologically defined effectors present in situ. J Natl Cancer Inst (1983) 70(1):21–6.
117. Kabawat SE, Bast RC Jr, Welch WR, Knapp RC, Bhan AK. Expression of major histocompatibility antigens and nature of inflammatory cellular infiltrate in ovarian neoplasms. Int J Cancer (1983) 32(5):547–54. doi:10.1002/ijc.2910320505
118. Ferguson A, Moore M, Fox H. Expression of MHC products and leucocyte differentiation antigens in gynaecological neoplasms: an immunohistological analysis of the tumour cells and infiltrating leucocytes. Br J Cancer (1985) 52(4):551–63.
119. Negus RP, Stamp GW, Hadley J, Balkwill FR. Quantitative assessment of the leukocyte infiltrate in ovarian cancer and its relationship to the expression of C-C chemokines. Am J Pathol (1997) 150(5):1723–34.
120. Santin AD, Hermonat PL, Ravaggi A, Bellone S, Roman JJ, Smith CV, et al. Phenotypic and functional analysis of tumor-infiltrating lymphocytes compared with tumor-associated lymphocytes from ascitic fluid and peripheral blood lymphocytes in patients with advanced ovarian cancer. Gynecol Obstet Invest (2001) 51(4):254–61. doi:10.1159/000058060
121. Dong HP, Elstrand MB, Holth A, Silins I, Berner A, Trope CG, et al. NK- and B-cell infiltration correlates with worse outcome in metastatic ovarian carcinoma. Am J Clin Pathol (2006) 125(3):451–8. doi:10.1309/15B66DQMFYYM78CJ
122. Webb JR, Milne K, Watson P, Deleeuw RJ, Nelson BH. Tumor-infiltrating lymphocytes expressing the tissue resident memory marker CD103 are associated with increased survival in high-grade serous ovarian cancer. Clin Cancer Res (2014) 20(2):434–44. doi:10.1158/1078-0432.CCR-13-1877
123. Miescher S, Whiteside TL, Carrel S, von Fliedner V. Functional properties of tumor-infiltrating and blood lymphocytes in patients with solid tumors: effects of tumor cells and their supernatants on proliferative responses of lymphocytes. J Immunol (1986) 136(5):1899–907.
124. Moy PM, Holmes EC, Golub SH. Depression of natural killer cytotoxic activity in lymphocytes infiltrating human pulmonary tumors. Cancer Res (1985) 45(1):57–60.
125. Stojanovic A, Cerwenka A. Natural killer cells and solid tumors. J Innate Immun (2011) 3(4):355–64. doi:10.1159/000325465
126. Nausch N, Galani I, Schlecker E, Cerwenka A. Mononuclear myeloid-derived “suppressor” cells express RAE-1 and activate natural killer cells. Blood (2008) 112(10):4080–9. doi:10.1182/blood-2008-03-143776
127. Solinas G, Germano G, Mantovani A, Allavena P. Tumor-associated macrophages (TAM) as major players of the cancer-related inflammation. J Leukoc Biol (2009) 86(5):1065–73. doi:10.1189/jlb.0609385
128. Matsushima K, Oppenheim JJ. Interleukin 8 and MCAF: novel inflammatory cytokines inducible by IL 1 and TNF. Cytokine (1989) 1(1):2–13. doi:10.1016/1043-4666(89)91043-0
129. Koch AE, Polverini PJ, Kunkel SL, Harlow LA, DiPietro LA, Elner VM, et al. Interleukin-8 as a macrophage-derived mediator of angiogenesis. Science (1992) 258(5089):1798–801. doi:10.1126/science.1281554
130. Xu L, Pathak PS, Fukumura D. Hypoxia-induced activation of p38 mitogen-activated protein kinase and phosphatidylinositol 3’-kinase signaling pathways contributes to expression of interleukin 8 in human ovarian carcinoma cells. Clin Cancer Res (2004) 10(2):701–7. doi:10.1158/1078-0432.CCR-0953-03
131. Radke J, Schmidt D, Böhme M, Schmidt U, Weise W, Morenz J. Cytokine level in malignant ascites and peripheral blood of patients with advanced ovarian carcinoma. Geburtshilfe Frauenheilkd (1996) 56(2):83–7. doi:10.1055/s-2007-1022247
132. Abdollahi T, Robertson NM, Abdollahi A, Litwack G. Identification of interleukin 8 as an inhibitor of tumor necrosis factor-related apoptosis-inducing ligand-induced apoptosis in the ovarian carcinoma cell line OVCAR3. Cancer Res (2003) 63(15):4521–6.
133. Flavell RA, Sanjabi S, Wrzesinski SH, Licona-Limón P. The polarization of immune cells in the tumour environment by TGFbeta. Nat Rev Immunol (2010) 10(8):554–67. doi:10.1038/nri2808
134. Trotta R, Dal Col J, Yu J, Ciarlariello D, Thomas B, Zhang X, et al. TGF-beta utilizes SMAD3 to inhibit CD16-mediated IFN-gamma production and antibody-dependent cellular cytotoxicity in human NK cells. J Immunol (2008) 181(6):3784–92. doi:10.4049/jimmunol.181.6.3784
135. FUTURE II Study Group. Quadrivalent vaccine against human papillomavirus to prevent high-grade cervical lesions. N Engl J Med (2007) 356(19):1915–27. doi:10.1056/NEJMoa061741
136. Koopman LA, Corver WE, van der Slik AR, Giphart MJ, Fleuren GJ. Multiple genetic alterations cause frequent and heterogeneous human histocompatibility leukocyte antigen class I loss in cervical cancer. J Exp Med (2000) 191(6):961–76. doi:10.1084/jem.191.6.961
137. de Boer MA, Jordanova ES, van Poelgeest MI, van den Akker BE, van der Burg SH, Kenter GG, et al. Circulating human papillomavirus type 16 specific T-cells are associated with HLA class I expression on tumor cells, but not related to the amount of viral oncogene transcripts. Int J Cancer (2007) 121(12):2711–5. doi:10.1002/ijc.23035
138. Koopman LA, Mulder A, Corver WE, Anholts JD, Giphart MJ, Claas FH, et al. HLA class I phenotype and genotype alterations in cervical carcinomas and derivative cell lines. Tissue Antigens (1998) 51(6):623–36. doi:10.1111/j.1399-0039.1998.tb03005.x
139. Lee SJ, Cho YS, Cho MC, Shim JH, Lee KA, Ko KK, et al. Both E6 and E7 oncoproteins of human papillomavirus 16 inhibit IL-18-induced IFN-gamma production in human peripheral blood mononuclear and NK cells. J Immunol (2001) 167(1):497–504. doi:10.4049/jimmunol.167.1.497
140. Davidson B, Goldberg I, Kopolovic J. Inflammatory response in cervical intraepithelial neoplasia and squamous cell carcinoma of the uterine cervix. Pathol Res Pract (1997) 193(7):491–5. doi:10.1016/S0344-0338(97)80102-1
141. Vaquer S, Jordá J, López de la Osa E, Alvarez de los Heros J, López-García N, Alvarez de Mon M. Clinical implications of natural killer (NK) cytotoxicity in patients with squamous cell carcinoma of the uterine cervix. Gynecol Oncol (1990) 36(1):90–2. doi:10.1016/0090-8258(90)90114-Z
142. Textor S, Dürst M, Jansen L, Accardi R, Tommasino M, Trunk MJ, et al. Activating NK cell receptor ligands are differentially expressed during progression to cervical cancer. Int J Cancer (2008) 123(10):2343–53. doi:10.1002/ijc.23733
143. Colucci F, Kieckbusch J, NF-kappa B-dependent upregulation of ICAM-1 by HPV16-E6/E7 facilitates NK cell/target cell interaction. Int J Cancer (2011) 128(5):1104–13. doi:10.1002/ijc.25442
144. Carrington M, Wang S, Martin MP, Gao X, Schiffman M, Cheng J, et al. Hierarchy of resistance to cervical neoplasia mediated by combinations of killer immunoglobulin-like receptor and human leukocyte antigen loci. J Exp Med (2005) 201(7):1069–75. doi:10.1084/jem.20042158
145. Manaster I, Mizrahi S, Goldman-Wohl D, Sela HY, Stern-Ginossar N, Lankry D, et al. Endometrial NK cells are special immature cells that await pregnancy. J Immunol (2008) 181(3):1869–76. doi:10.4049/jimmunol.181.3.1869
146. El Costa H, Casemayou A, Aguerre-Girr M, Rabot M, Berrebi A, Parant O, et al. Critical and differential roles of NKp46- and NKp30-activating receptors expressed by uterine NK cells in early pregnancy. J Immunol (2008) 181(5):3009–17. doi:10.4049/jimmunol.181.5.3009
147. Kopcow HD, Allan DS, Chen X, Rybalov B, Andzelm MM, Ge B, et al. Human decidual NK cells form immature activating synapses and are not cytotoxic. Proc Natl Acad Sci U S A (2005) 102(43):15563–8. doi:10.1073/pnas.0507835102
148. Colucci F, Kieckbusch J. Maternal uterine natural killer cells nurture fetal growth: in medio stat virtus. Trends Mol Med (2015) 21(2):60–7. doi:10.1016/j.molmed.2014.12.009
149. Moffett A, Colucci F. Uterine NK cells: active regulators at the maternal-fetal interface. J Clin Invest (2014) 124(5):1872–9. doi:10.1172/JCI68107
150. Freedman RS. Recent immunologic advances affecting the management of ovarian cancer. Clin Obstet Gynecol (1985) 28(4):853–71. doi:10.1097/00003081-198528040-00020
151. Freedman RS, Edwards CL, Bowen JM, Lotzova E, Katz R, Lewis E, et al. Viral oncolysates in patients with advanced ovarian cancer. Gynecol Oncol (1988) 29(3):337–47. doi:10.1016/0090-8258(88)90233-8
152. Freedman RS, Kouttab NM, Bowen JM, Edwards CL, Katz RL. Lymphokine activity in malignant effusions after intracavitary viral oncolysate. Lymphokine Res (1989) 8(2):115–22.
153. Lotzová E, Savary CA, Freedman RS, Bowen JM. Natural killer cell cytotoxic potential of patients with ovarian carcinoma and its modulation with virus-modified tumor cell extract. Cancer Immunol Immunother (1984) 17(2):124–9. doi:10.1007/BF00200048
154. Mantia-Smaldone GM, Corr B, Chu CS. Immunotherapy in ovarian cancer. Hum Vaccin Immunother (2012) 8(9):1179–91. doi:10.4161/hv.20738
155. Freedman RS, Lenzi R, Kudelka AP, Lawrence DD, Rosenblum M, Platsoucas CD. Intraperitoneal immunotherapy of peritoneal carcinomatosis. Cytokines Cell Mol Ther (1998) 4(2):121–40.
156. Alberts DS, Marth C, Alvarez RD, Johnson G, Bidzinski M, Kardatzke DR, et al. Randomized phase 3 trial of interferon gamma-1b plus standard carboplatin/paclitaxel versus carboplatin/paclitaxel alone for first-line treatment of advanced ovarian and primary peritoneal carcinomas: results from a prospectively designed analysis of progression-free survival. Gynecol Oncol (2008) 109(2):174–81. doi:10.1016/j.ygyno.2008.01.005
157. Todd PA, Goa KL. Interferon gamma-1b. A review of its pharmacology and therapeutic potential in chronic granulomatous disease. Drugs (1992) 43(1):111–22. doi:10.2165/00003495-199243010-00008
158. Recchia F, Di Orio F, Candeloro G, Guerriero G, Piazze J, Rea S. Maintenance immunotherapy in recurrent ovarian cancer: long term follow-up of a phase II study. Gynecol Oncol (2010) 116(2):202–7. doi:10.1016/j.ygyno.2009.09.042
159. Recchia F, Saggio G, Cesta A, Candeloro G, Nuzzo A, Lombardo M, et al. Interleukin-2 and 13-cis retinoic acid as maintenance therapy in advanced ovarian cancer. Int J Oncol (2005) 27(4):1039–46.
160. Pillet AH, Bugault F, Thèze J, Chakrabarti LA, Rose T. A programmed switch from IL-15- to IL-2-dependent activation in human NK cells. J Immunol (2009) 182(10):6267–77. doi:10.4049/jimmunol.0801933
161. Leclercq G, Debacker V, de Smedt M, Plum J. Differential effects of interleukin-15 and interleukin-2 on differentiation of bipotential T/natural killer progenitor cells. J Exp Med (1996) 184(2):325–36. doi:10.1084/jem.184.2.325
162. Childs RW, Carlsten M. Therapeutic approaches to enhance natural killer cell cytotoxicity against cancer: the force awakens. Nat Rev Drug Discov (2015) 14(7):487–98. doi:10.1038/nrd4506
163. Kamada M, Sakamoto Y, Furumoto H, Mori K, Daitoh T, Irahara M, et al. Treatment of malignant ascites with allogeneic and autologous lymphokine-activated killer cells. Gynecol Oncol (1989) 34(1):34–7. doi:10.1016/0090-8258(89)90101-7
164. Rosenberg SA. Immunotherapy of cancer by systemic administration of lymphoid cells plus interleukin-2. J Biol Response Mod (1984) 3(5):501–11.
165. Urba WJ, Clark JW, Steis RG, Bookman MA, Smith JW II, Beckner S, et al. Intraperitoneal lymphokine-activated killer cell/interleukin-2 therapy in patients with intra-abdominal cancer: immunologic considerations. J Natl Cancer Inst (1989) 81(8):602–11. doi:10.1093/jnci/81.8.602
166. Steis RG, Urba WJ, VanderMolen LA, Bookman MA, Smith JW II, Clark JW, et al. Intraperitoneal lymphokine-activated killer-cell and interleukin-2 therapy for malignancies limited to the peritoneal cavity. J Clin Oncol (1990) 8(10):1618–29. doi:10.1200/JCO.1990.8.10.1618
167. Stewart JA, Belinson JL, Moore AL, Dorighi JA, Grant BW, Haugh LD, et al. Phase I trial of intraperitoneal recombinant interleukin-2/lymphokine-activated killer cells in patients with ovarian cancer. Cancer Res (1990) 50(19):6302–10.
168. Schmidt-Wolf IG, Negrin RS, Kiem HP, Blume KG, Weissman IL. Use of a SCID mouse/human lymphoma model to evaluate cytokine-induced killer cells with potent antitumor cell activity. J Exp Med (1991) 174(1):139–49. doi:10.1084/jem.174.1.139
169. Kim HM, Kang JS, Lim J, Park SK, Lee K, Yoon YD, et al. Inhibition of human ovarian tumor growth by cytokine-induced killer cells. Arch Pharm Res (2007) 30(11):1464–70. doi:10.1007/BF02977372
170. Kim HM, Lim J, Kang JS, Park SK, Lee K, Kim JY, et al. Inhibition of human cervical carcinoma growth by cytokine-induced killer cells in nude mouse xenograft model. Int Immunopharmacol (2009) 9(3):375–80. doi:10.1016/j.intimp.2008.12.001
171. Liu J, Li H, Cao S, Zhang X, Yu J, Qi J, et al. Maintenance therapy with autologous cytokine-induced killer cells in patients with advanced epithelial ovarian cancer after first-line treatment. J Immunother (2014) 37(2):115–22. doi:10.1097/CJI.0000000000000021
172. Miller JS, Soignier Y, Panoskaltsis-Mortari A, McNearney SA, Yun GH, Fautsch SK, et al. Successful adoptive transfer and in vivo expansion of human haploidentical NK cells in patients with cancer. Blood (2005) 105(8):3051–7. doi:10.1182/blood-2004-07-2974
173. Geller MA, Cooley S, Judson PL, Ghebre R, Carson LF, Argenta PA, et al. A phase II study of allogeneic natural killer cell therapy to treat patients with recurrent ovarian and breast cancer. Cytotherapy (2011) 13(1):98–107. doi:10.3109/14653249.2010.515582
174. Yang Y, Lim O, Kim TM, Ahn YO, Choi H, Chung H, et al. Phase I study of random healthy donor-derived allogeneic natural killer cell therapy in patients with malignant lymphoma or advanced solid tumors. Cancer Immunol Res (2016) 4(3):215–24. doi:10.1158/2326-6066.CIR-15-0118
175. Xie S, Chen J, Zhang M, Wu Z. Allogenic natural killer cell immunotherapy of sizeable ovarian cancer: a case report. Mol Clin Oncol (2017) 6(6):903–6. doi:10.3892/mco.2017.1230
176. Cichocki F, Valamehr B, Bjordahl R, Zhang B, Rezner B, Rogers P, et al. GSK 3 inhibition drives maturation of NK cells and enhances their antitumor activity. Cancer Res (2017) 77(20):5664–75. doi:10.1158/0008-5472.CAN-17-0799
177. Hoogstad-van Evert JS, Cany J, van den Brand D, Oudenampsen M, Brock R, Torensma R, et al. Umbilical cord blood CD34+ progenitor-derived NK cells efficiently kill ovarian cancer spheroids and intraperitoneal tumors in NOD/SCID/IL2Rgnull mice. Oncoimmunology (2017) 6(8):e1320630. doi:10.1080/2162402X.2017.1320630
178. Hermanson DL, Kaufman DS. Utilizing chimeric antigen receptors to direct natural killer cell activity. Front Immunol (2015) 6:195. doi:10.3389/fimmu.2015.00195
179. Bracci L, Schiavoni G, Sistigu A, Belardelli F. Immune-based mechanisms of cytotoxic chemotherapy: implications for the design of novel and rationale-based combined treatments against cancer. Cell Death Differ (2014) 21(1):15–25. doi:10.1038/cdd.2013.67
180. Gleason MK, Verneris MR, Todhunter DA, Zhang B, McCullar V, Zhou SX, et al. Bispecific and trispecific killer cell engagers directly activate human NK cells through CD16 signaling and induce cytotoxicity and cytokine production. Mol Cancer Ther (2012) 11(12):2674–84. doi:10.1158/1535-7163.MCT-12-0692
181. Vallera DA, Zhang B, Gleason MK, Oh S, Weiner LM, Kaufman DS, et al. Heterodimeric bispecific single-chain variable-fragment antibodies against EpCAM and CD16 induce effective antibody-dependent cellular cytotoxicity against human carcinoma cells. Cancer Biother Radiopharm (2013) 28(4):274–82. doi:10.1089/cbr.2012.1329
182. Schmohl JU, Felices M, Taras E, Miller JS, Vallera DA. Enhanced ADCC and NK cell activation of an anticarcinoma bispecific antibody by genetic insertion of a modified IL-15 cross-linker. Mol Ther (2016) 24(7):1312–22. doi:10.1038/mt.2016.88
183. Schmohl JU, Felices M, Todhunter D, Taras E, Miller JS, Vallera DA. Tetraspecific scFv construct provides NK cell mediated ADCC and self-sustaining stimuli via insertion of IL-15 as a cross-linker. Oncotarget (2016) 7(45):73830–44. doi:10.18632/oncotarget.12073
184. Felices M, Lenvik TR, Davis ZB, Miller JS, Vallera DA. Generation of BiKEs and TriKEs to improve NK cell-mediated targeting of tumor cells. Methods Mol Biol (2016) 1441:333–46. doi:10.1007/978-1-4939-3684-7_28
185. Davis ZB, Vallera DA, Miller JS, Felices M. Natural killer cells unleashed: checkpoint receptor blockade and BiKE/TriKE utilization in NK-mediated anti-tumor immunotherapy. Semin Immunol (2017) 31:64–75. doi:10.1016/j.smim.2017.07.011
186. Carotta S, Targeting NK. Cells for anticancer immunotherapy: clinical and preclinical approaches. Front Immunol (2016) 7:152. doi:10.3389/fimmu.2016.00152
187. Davis ZB, Felices M, Verneris MR, Miller JS. Natural killer cell adoptive transfer therapy: exploiting the first line of defense against cancer. Cancer J (2015) 21(6):486–91. doi:10.1097/PPO.0000000000000156
188. Pesce S, Greppi M, Tabellini G, Rampinelli F, Parolini S, Olive D, et al. Identification of a subset of human natural killer cells expressing high levels of programmed death 1: a phenotypic and functional characterization. J Allergy Clin Immunol (2017) 139(1):335–46.e3. doi:10.1016/j.jaci.2016.04.025
189. Benson DM Jr, Bakan CE, Mishra A, Hofmeister CC, Efebera Y, Becknell B, et al. The PD-1/PD-L1 axis modulates the natural killer cell versus multiple myeloma effect: a therapeutic target for CT-011, a novel monoclonal anti-PD-1 antibody. Blood (2010) 116(13):2286–94. doi:10.1182/blood-2010-02-271874
190. Chan CJ, Martinet L, Gilfillan S, Souza-Fonseca-Guimaraes F, Chow MT, Town L, et al. The receptors CD96 and CD226 oppose each other in the regulation of natural killer cell functions. Nat Immunol (2014) 15(5):431–8. doi:10.1038/ni.2850
191. Carlsten M, Childs RW. Genetic manipulation of NK cells for cancer immunotherapy: techniques and clinical implications. Front Immunol (2015) 6:266. doi:10.3389/fimmu.2015.00266
192. Einhorn N, Cantell K, Einhorn S, Strander H. Human leukocyte interferon therapy for advanced ovarian carcinoma. Am J Clin Oncol (1982) 5(2):167–72.
193. West WH, Tauer KW, Yannellli JR, Marshall GD, Orr DW, Thurman GB, et al. Constant-infusion recombnant interleukin-2 in adoptive immunotherapy of advanced cancer. N Engl J Med (1987) 316(15):898–905. doi:10.1056/NEJM198704093161502
194. Pujade-Lauraine E, Guastalla JP, Colombo N, Devillier P, François E, Fumoleau P, et al. Intraperitoneal recombinant interferon gamma in ovarian cancer patients with residual disease at second-look laparotomy. J Clin Oncol. (1996) 14(2):343–50. doi:10.1200/JCO.1996.14.2.343
195. Windbichler GH, Hausmaninger H, Stummvoll W, Graf AH, Kainz C, Lahodny J, et al. Interferon-gamma in the first-line therapy of ovarian cancer: a randomized phase III trial. Br J Cancer. (2000) 82(6):1138–44. doi:10.1054/bjoc.1999.1053
196. Vlad AM, Budiu RA, Lenzner DE, Wang Y, Thaller JA, Colonello K, et al. A phase II trial of intraperitoneal interleukin-2 in patients with platinum-resistant or platinum-refractory ovarian cancer. Cancer Immunol Immunother. (2010) 59(2):293–301. doi:10.1007/s00262-009-0750-3
Keywords: natural killer cells, immunotherapy, gynecologic malignancy, ovarian cancer, cervical cancer
Citation: Uppendahl LD, Dahl CM, Miller JS, Felices M and Geller MA (2018) Natural Killer Cell-Based Immunotherapy in Gynecologic Malignancy: A Review. Front. Immunol. 8:1825. doi: 10.3389/fimmu.2017.01825
Received: 06 October 2017; Accepted: 04 December 2017;
Published: 05 January 2018
Edited by:
Rohtesh S. Mehta, University of Texas MD Anderson Cancer Center, United StatesReviewed by:
Sarah M. Temkin, Virginia Commonwealth University, United StatesFrancesco Colucci, University of Cambridge, United Kingdom
Alessandro Moretta, Università di Genova, Italy
Copyright: © 2018 Uppendahl, Dahl, Miller, Felices and Geller. This is an open-access article distributed under the terms of the Creative Commons Attribution License (CC BY). The use, distribution or reproduction in other forums is permitted, provided the original author(s) or licensor are credited and that the original publication in this journal is cited, in accordance with accepted academic practice. No use, distribution or reproduction is permitted which does not comply with these terms.
*Correspondence: Melissa A. Geller, gelle005@umn.edu