- Department of Infection Biology, University Hospital Erlangen and Friedrich-Alexander University Erlangen-Nuremberg (FAU), Erlangen, Germany
Type 2 innate lymphoid cells (ILC2s) are a major source of cytokines, which are also produced by Th2 cells and several cell types of the innate immune system. Work over the past few years indicates that ILC2s play a central role in regulating type 2 immune responses against allergens and helminths. ILC2s can interact with a variety of cells types of the innate and adaptive immune system by cell–cell contacts or by communication via soluble factors. In this review, we provide an overview about recent advances in our understanding how ILC2s orchestrate type 2 immune responses with focus on direct interactions between ILC2s and other cells of the immune system.
Introduction
Innate lymphoid cells (ILCs) are characterized by their lack of expression of rearranged antigen-receptors and absence of cell surface markers present on other common hematopoietic cell lineages. They arise from a common lymphoid progenitor, requiring expression of the transcriptional repressor Id2 and relying on cytokine signaling through the common gamma-chain (γc chain) of the IL-2 receptor family. ILCs are classified into three distinct populations termed group 1, 2, and 3 ILCs based on their ability to produce effector cytokines associated with Th1, Th2, or Th17 cells, respectively (1–4).
Group 2 ILCs (ILC2s) share the expression of the transcription factor GATA-3 and characteristic cytokines with Th2 cells indicating a similar role for both cell types in type 2 immune responses (5, 6). ILC2s respond extremely rapidly to epithelial cell-derived cytokines, such as IL-25, IL-33, and thymic stromal lymphopoietin (TSLP), associated with barrier disruption (7) and act as “early sentinel” cells of the innate immune system orchestrating type 2 immune responses at mucosal surfaces and adipose tissue (8–10).
The canonical type 2 immunity-associated cytokines IL-4, IL-5, and IL-13 are pivotal in immunity toward gastrointestinal helminths inducing eosinophilia, elevated IgE levels, goblet cell metaplasia with enhanced mucus production, and smooth muscle hyperreactivity (11, 12). It is evident that cells of the adaptive and innate immune system, including Th2 cells, ILC2s, eosinophils, basophils, and mast cells, produce significant levels of these cytokines inducing and sustaining ongoing type 2 responses (13, 14). Although the prominent role of Th2 cells in type 2 immune responses is well established, the secretion of IL-13 from ILC2s rather than Th2 cells is of particular importance for controlling the intestinal immune response and worm expulsion during infection of mice with the helminth Nippostrongylus brasiliensis (9, 10, 15). On the other hand, T cell-derived IL-4/IL-13 are needed for effector cell recruitment, germinal center formation, IgE switching, and paracrine Th2 differentiation (16, 17).
In contrast to the protective function of ILC2s, dysregulated ILC2 responses contribute to inflammatory processes, such as airway hyperreactivity (18), allergen-induced lung inflammation (19, 20), and atopic dermatitis (21). Despite the substantial gain of knowledge about ILC2s development and mediators that positively or negatively modulate ILC2 homeostasis, activation, and functions (22, 23), the regulation of ILC2 functions is becoming more complex, and it is of high importance to understand the immunoregulatory mechanisms to improve therapeutic treatments of pathological type 2 immune responses. Besides producing cytokines, ILC2s may interact with other effector immune cells and coordinate immune responses as part of the complex immune system network important for immune defense and allergic reactions. Recent data indicate that ILC2s can influence T cell responses in a reciprocal manner, either through cytokines, indirect effects on accessory cells, or direct cell–cell contact relaying signals to the adaptive immune system. Additionally, ILC2s also contribute to the maintenance of eosinophils (24) and affect the functions of cells such as basophils (25), macrophages (26), dendritic cells (DCs) (27, 28), and mast cells (29), which on the other hand can also activate ILC2s (30) or suppress their activity (31).
Defining the complex network of interactions and mutual communications of ILC2s with immune cells from the innate and adaptive immune system and understanding the specific contributions of ILC2s leading to protective immunity against helminths or development of pathologic responses may reveal critical checkpoints that can be manipulated for controlling type 2 immunity-mediated responses and will be important to investigate new possible therapeutic interventions.
Interactions of ILC2s with Cells of the Adaptive Immune System
ILC2s and T Cells
Th2 cells are a major source of IL-4 and IL-13 and they play an important role in type 2 immune responses. Recently, our group revealed that specific depletion of IL-4/IL-13 in CD4+ T cells results in reduced accumulation of innate effector cells (eosinophils, basophils, ILC2s) in the lung of N. brasiliensis-infected mice as compared to wild-type mice and that CD4+ T cell-derived IL-4/IL-13 cytokines promote Th2 polarization in a paracrine manner (16, 17). Beside eosinophils, basophils, and mast cells, mouse ILC2s are also known to transcribe and produce low amounts of IL-4 induced by the lipid mediator leukotriene D4 (15, 32, 33). Leukotrienes can act in a synergistic manner together with IL-33 to activate ILC2s (34, 35). Human ILC2s appear to secrete larger quantities of IL-4 as compared to mouse ILC2s especially upon IL-33 stimulation and in the presence of TSLP (36). During infection with the helminth Heligmosomoides polygyrus, ILC2-derived IL-4 has been shown to contribute to ILC2 expansion and to drive early Th2 differentiation without direct cell–cell contact (33). Furthermore, mice lacking ILC2s showed impaired Th2-skewed inflammatory responses following helminth infection or local exposure to the protease-allergen papain, or house dust mite antigens (27, 37, 38). This suggests that ILC2s can promote Th2 responses under certain experimental conditions. In addition, IL-13-producing ILC2s were shown to cooperate with CD4+ T cells during N. brasiliensis infection to mediate larval killing in the small intestine during primary infection (38) and in the lung following secondary infection (26). Furthermore, N. brasiliensis could be expelled by transfer of ILC2s into IL-13-deficient mice, but not into Rag2-deficient mice (9). This indicates that IL-13 from ILC2s is sufficient for clearance of primary N. brasiliensis infection, but CD4+ T cells are still required for effective worm expulsion Interestingly, T cell-derived IL-2 can induce ILC2 proliferation and IL-13 secretion (39). In addition, it was shown that in mice exposed to the pro-allergic protease papain ILC2-derived IL-13 rather than IL-4 promotes migration of DCs into lung-draining lymph nodes, where activated DCs support Th2 cell differentiation (27).
Innate lymphoid cells not only contribute to Th2 cell differentiation by cytokine secretion but can also directly interact with CD4+ T cells. Using an in vitro culture system, it was reported that ILC2s promote Th2 polarization in a cell–cell contact-dependent manner (39). In addition, both costimulation by OX40/OX40-L interaction and ILC2-derived IL-4 was shown to enhance Th2 cell proliferation and Th2 cytokine production when the isolated cell populations were cultured together (40). Beside expressing costimulatory molecules, ILC2s have also been shown to express MHC class II (9, 39, 41). Recent data identified ILC2s as antigen-presenting cells (APC) able to process and present peptide antigens and modulate naive CD4+ T cell activation in a cell contact-dependent manner (38, 39, 42). Expression of MHC-II on ILC2s was required to receive activating signals by T cell-derived IL-2 causing efficient secretion of IL-13 (38). This suggests that ILC2s and T cells can communicate in an antigen-dependent manner. However, whether ILC2s play a significant role as APC during priming of the Th2 response remains to be investigated.
ILC2s and Treg Cells
Subsequent studies demonstrated that Treg cells and ILC2s engage in reciprocal regulation. Treg cells are regulators of adaptive immune responses through direct cell–cell contact, as well as through the suppressive activities of IL-10 and TGF-β. The importance of Treg cells on control of ILC2 activity and homeostasis has recently been shown by inhibition of the transcription factors Id2 and Id3 in Treg cells, which lead to a spontaneous increase in ILC2 counts, as well as accumulation of eosinophils in the lungs and resulted in the development of fatal inflammatory disease (43). While Treg cells regulate ILC2 expansion and suppress their pro-inflammatory cytokine secretion in vivo and in vitro, ILC2-derived IL-4 plays a requisite role in mediating sensitization to food allergens by compromising allergen-specific regulatory T cell function and thereby promoting food allergy (44). In contrast, ILC2-derived IL-9 was required for Treg activation and resolution of inflammation in an arthritis model (45). In addition, ILC2s may enhance the suppressive activity of Treg cells by secretion of amphiregulin as it has been described for mast cell-derived amphiregulin (46).
IL-33 can either directly activate ST2+ Treg cells in the intestine (47) or promote their expansion indirectly by inducing IL-2 secretion from DCs (48). Others have shown that accumulation of Treg cells in adipose tissue by IL-33 was directly dependent on ILC2s by a process requiring ILC2-intrinsic MyD88 signaling and cognate interactions between inducible T cell co-stimulator (ICOS) on Treg and ICOS ligand (ICOS-L) on ILC2s (49). As IL-33 alone can activate significant Treg cell proliferation independently of ILC2s, one can assume that ILC2s act mainly to promote Treg survival. Coculture experiments revealed that ICOS-L/ICOS interaction is important for the ILC2-mediated survival of Treg cells (49). Furthermore, ILC2-derived IL-4 may promote the conversion of Treg cells to Th2 cells after H. polygyrus infection (50). A recent study demonstrated that induced Treg cells (iTreg), but not natural Treg cells, effectively suppressed human and mouse ILC2 function and this effect was dependent on ICOS/ICOS-L interactions (51). Autocrine ICOS/ICOS-L interactions on ILC2s were also reported to play an important role to enhance survival of ILC2s (42, 52). IFN-γ was found to counter-regulate the effects of IL-33 mediated ILC2 activation and Treg cell expansion (49), indicating that Th1-dominated immune responses can actively suppress the IL-33-ILC2-Treg axis and thereby cause loss of barrier tissue integrity and shifts in fat metabolism. Understanding the contributions of ILC2s and Tregs to optimal immune regulation might help preventing excessive tissue damage and the development of chronic inflammations.
ILC2s and B Cells
In addition to their mutual interactions with T cells, ILC2s may also interact with B cells. It is known that fat-associated lymphoid clusters (FALCs), found in human and in mouse mesentery, contain large proportion of B1 cells and contribute to innate B cell activation and germinal center differentiation (53). So far, it is described that ILC2s from FALCs maintain homeostasis of the self-renewing B1 cells in an IL-5-dependent manner and support production of parasite-specific antibodies by B cells (8, 54). Interestingly, it was described that lung ILC2s not only enhance the proliferation of B1 but also follicular B2 type B cells and promote the secretion of IgM, IgG1, IgA, and IgE by these cells in the absence of T cells (55). It is also assumed that ILC2s have the ability to promote T cell-dependent antibody responses as serum IgE levels are reduced in ILC2-deficient mice challenged with papain (27). Human ILC2s were found to directly activate B cells isolated from tonsils (56).
Furthermore, it has been described that ILC2s can enhance IgE production from B cells in vitro, raising the possibility that ILC2s orchestrate IgE-dependent allergic sensitization (57). Whether ILC2s can interact with ICOS-L-expressing B cells is still an open question and additional studies are required to identify B cell-derived mediators that feedback on ILC2s. However, the addition of anti-ICOS antibodies showed no effect on the ability of ILC2s to enhance B cell IgM and IgG1 production indicating that cytokines like IL-5 and other soluble factors that are secreted by ILC2s may play a key role (55).
Still, many questions remain to be answered regarding the interactions between ILC2s and cells of the adaptive immune system. Taken together, ILC2s regulate and dictate the nature of downstream T cell- and B cell-mediated immune responses, while T cells also influence the survival, proliferation, and function of ILC2s (Figure 1).
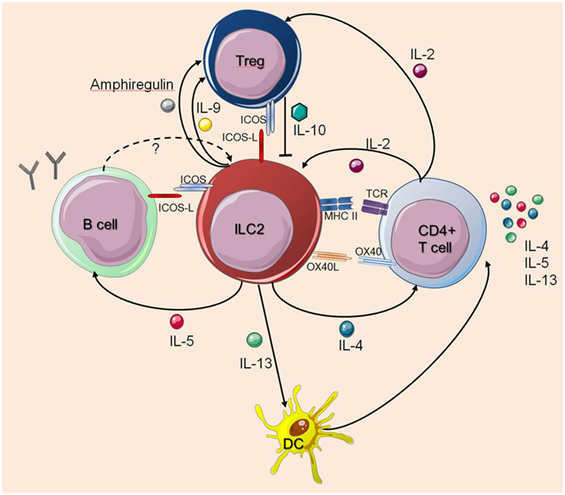
Figure 1. Interactions between innate lymphoid cells (ILC2s) and T cells or B cells. The figure illustrates how ILC2s interact with T cells or B cells as described in the main text. The dashed arrow indicates a potential feedback regulation for which there is currently no experimental evidence. Solid arrows indicate published evidence for activating mechanisms whereas Treg-derived IL-10 was shown to suppress ILC2s. Cell symbols where taken from http://smart.servier.com website.
Interactions of ILC2s with Cells of the Innate Immune System
The alarmins IL-25, IL-33, and TSLP initiate a local inflammatory response through the recruitment and activation of ILC2s and other innate effector cells. Investigating the role of ILC2s during coordination of this early innate immune response is critical to uncover their contribution for type 2 immunity during infections, allergic responses, or autoimmune diseases.
ILC2s and Macrophages
Innate lymphoid cells can promote the differentiation of so-called “alternatively activated” or M2 macrophages, which have protective functions in some helminth infection models and contribute to tissue repair responses (58). Using a mouse model for cerebral malaria, IL-33-elicited ILC2s were found to promote M2 polarization and Treg cell expansion resulting in protective immunity (59). It was further described that IL-33- or IL-2-elicited ILC2s are sufficient to induce M2 macrophage-mediated larval killing of N. brasiliensis helminths in the lung (26). Antifungal type 2 immune responses are also regulated by ILC2-mediated M2 polarization as demonstrated with a Cryptococcus neoformans infection model (60). In addition, IL-25-elicited ILC2-derived IL-13 was shown to promote activation of lung-resident macrophages to a profibrotic phenotype, driving collagen deposition from fibroblasts (61).
M2 macrophages in visceral adipose tissue (VAT) play an important role for glucose and fat metabolism and ILC2s as well as eosinophils have been described to promote M2 macrophage accumulation in VAT during helminth infection (62, 63). Furthermore, it has been reported that IL-33-activated ILC2s elicit the differentiation of alternatively activated macrophages through IL-4 receptor signaling and regulate directly beige fat biogenesis (64). However, recent studies indicate that IL-4-mediated macrophages have no relevant effect on white and brown adipocyte function and do not likely modulate adipocyte metabolism by catecholamine production (65, 66).
The other way round, alveolar macrophages are able to secrete IL-33, which is likely important for direct activation of ILC2s to produce substantial amounts of IL-13, as mice lacking the IL-33 receptor failed to develop AHR or airway inflammation independently of adaptive immunity (67). Thus, cross talk between macrophages and ILC2s might be critical to promote an early feed forward process during type 2 immune responses.
ILC2s and DCs
Type 2 innate lymphoid cell (ILC2)-derived IL-13 was reported to induce migration of DCs into the draining lymph node, where DCs drive naive T cells to become Th2 cells (27). However, how IL-13 controls the migratory function of DCs still remains to be defined. Furthermore, ILC2-derived IL-13 promotes the secretion of the chemokine CCL17 from DCs for the recruitment of CCR4+ memory Th2 cells to the site of allergen exposure (28). Eosinophils recruited into tissues and lymph nodes can also control DC activation and migration and promote Th2-cell-mediated immunity via degranulation of eosinophil peroxidase (68), demonstrating how innate cells work in a complex network to drive type 2 inflammatory responses.
The tumor necrosis factor (TNF) family cytokine TL1A is known to be produced by DCs and macrophages in response to toll-like-receptor and Fc receptor cross-linking and regulates the adaptive immune response by co-stimulating T cells (69). It was demonstrated that TL1A synergizes with IL-25 in vivo to directly promote ILC2 expansion, survival, and function (70, 71). The activation of ILC2s by TL1A could provide new insight into interaction of ILC2s and activated myeloid cells.
Recent observations revealed that type I and type II IFNs as well as IL-27 play a critical role as negative regulators of ILC2s to restrict type 2 immunity and its associated pathologies (49, 72–74). It has been reported that plasmacytoid dendritic cells (pDC) play a critical role in dampening the function and survival of ILC2s in the context of allergic pulmonary inflammation. IFN-α production by activated pDCs can inhibit proliferation and increases the apoptosis rate of ILC2s (31). Similarly, polyinosinic–polycytidylic acid (pI:C) activated NK cells inhibit the proliferation and cytokine production of ILC2s via IFN-γ during the early stage of lung inflammation, reminescent of the classic antagonism between Th1 and Th2 differentiation (75). As interferons are produced by many different cell types including Th1 cells, NKT cells, and others, one can assume that ILC2s may be suppressed by various cellular sources of these mediators.
ILC2s and Eosinophils
Innate lymphoid cells are a major source of IL-5 and thereby enhance proliferation, survival, and recruitment of eosinophils (24, 49). By comparing Rag2−/− and Rag2−/− γc−/− mice, lung ILC2s were shown to promote eosinophilia independently of signals from the adaptive immune system (76). Naive lung ILC2s secrete IL-5 constitutively, as indicated by elevated Il5 mRNA levels and fluorescent signals in Il5-reporter mice (24, 77). ILC2-derived IL-5 is required for systemic maintenance of eosinophils and during type 2 inflammation ILC2s are induced to co-express IL-13, resulting in localized eotaxin production for recruitment and activation of eosinophils during allergic inflammation and helminth infection (24, 62, 78). It was further shown that vasoactive intestinal peptide, a hormone regulated by caloric intake, stimulates ILC2s via the vasoactive intestinal peptide receptor 2 receptor to release IL-5, linking eosinophil levels with metabolic cycling (24). IL-33 can directly stimulate eosinophil survival (79) and activate the production of IL-4 (80), which can stimulate ILC2s and thereby mediate the cross talk between eosinophils and IL-5-producing ILC2s (81).
ILC2s and Basophils
Basophils were shown to promote ILC2 proliferation by secretion of IL-4 in lung and skin inflammation models (25, 82). Basophils are relatively short-lived circulating cells, which have specified effector functions in type 2 immunity such as protective functions against helminths and ticks as well as pro-inflammatory functions in response to allergens. Lung ILC2 activation and numbers are reduced in basophil-specific IL-4-deficient mice, indicating that the ILC2s respond to basophil-produced IL-4 (25, 83). Furthermore, clusters of basophils and ILC2s where shown to accumulate in a mouse model for atopic dermatitis where basophil-derived IL-4 was required for ILC2 accumulation and proliferation in inflamed skin (82). Whether basophil activity, survival, or tissue recruitment is regulated by ILC2-derived factors remains to be analyzed.
ILC2s and Mast Cells
Innate lymphoid cells have been found in proximity to tissue mast cells in human lung (84). Furthermore, dermal ILC2s and skin-resident mast cells have been reported to physically interact in contact dermatitis models in mice using intravital multiphoton microscopy, supporting the idea that ILC2s can directly communicate with mast cells (85). It was shown that ILC2s have the potential to dampen pro-inflammatory mast cell response through the production of IL-13, thereby reducing IL-6 and TNF-α production by mast cells (85).
The close proximity of ILC2s and mast cells could be caused by production of inflammatory mediators such as PGD2 by mast cells, which induces the chemotaxis of ILC2s through activation of cysteine-three-histidine protein 2 and promotes IL-13 production in a synergistic manner with airway epithelial cytokines IL-25 and IL-33 leading to tissue eosinophilia (84, 86–88). Moreover, mast cells can influence ILC2 activity indirectly by releasing non-caspase proteases chymase and tryptase that cleave IL-33 into a more bioactive isoform (89).
Mast cells can also release IL-33 upon antigen-specific IgE-mediated activation (90) and in response to extracellular ATP, which in turn activates ILC2s to produce IL-13 resulting in clearance of helminth infection (30). Conversely, recombinant IL-33 can directly activate mast cells to produce several cytokines including IL-4 and IL-5. IL-33-activated mast cells are known to produce IL-2, which was shown to indirectly limit ILC2 proliferation by the expansion of Treg cells and their production of IL-10 (91). On the other hand, mast cells also produce IL-2 when activated by IL-9 from IL-33-elicited ILC2s. This mast cell-derived IL-2 leads to expansion of pro-inflammatory CD25+ ILC2s, which in turn activate Th9 cells leading to an amplified allergic inflammation (92). However, comparing N. brasiliensis-infected wild-type mice with IL-9 receptor-deficient mice showed similar mast cell accumulation in the lung arguing against a major role of ILC2-derived IL-9 for mastocytosis in this model (93).
Conclusion
Innate lymphoid cells are important “early sentinel” cells, which bridge the gap between the innate and adaptive type 2 immune response by sensing environmental changes and releasing immune-regulatory cytokines. A large variety of pathways that regulate the functions of ILC2s have been identified in the recent past and key interactions between ILC2s and cells of the innate and adaptive immune system were characterized (Figure 2). Apart from cross talk with various immune cell types, ILC2s may also have effects on structural cells including epithelial cells, smooth muscle cells, and fibroblasts. These interactions in mice and humans include communications by direct cell–cell contact and by secretion and recognition of soluble factors like cytokines, chemokines, hormones, and lipid mediators. However, regulation of ILC2 functions in various tissues during steady state conditions and upon infection with different pathogens becomes more and more complex and many pathways are still unknown. Further investigations will help to improve our understanding of how interactions between ILC2s and other immune cells are regulated. This information is essential to dissect the complexity of type 2 immune responses with the hope to identify critical checkpoints that are accessible for therapeutic interventions in allergic inflammation and immunity against helminths.
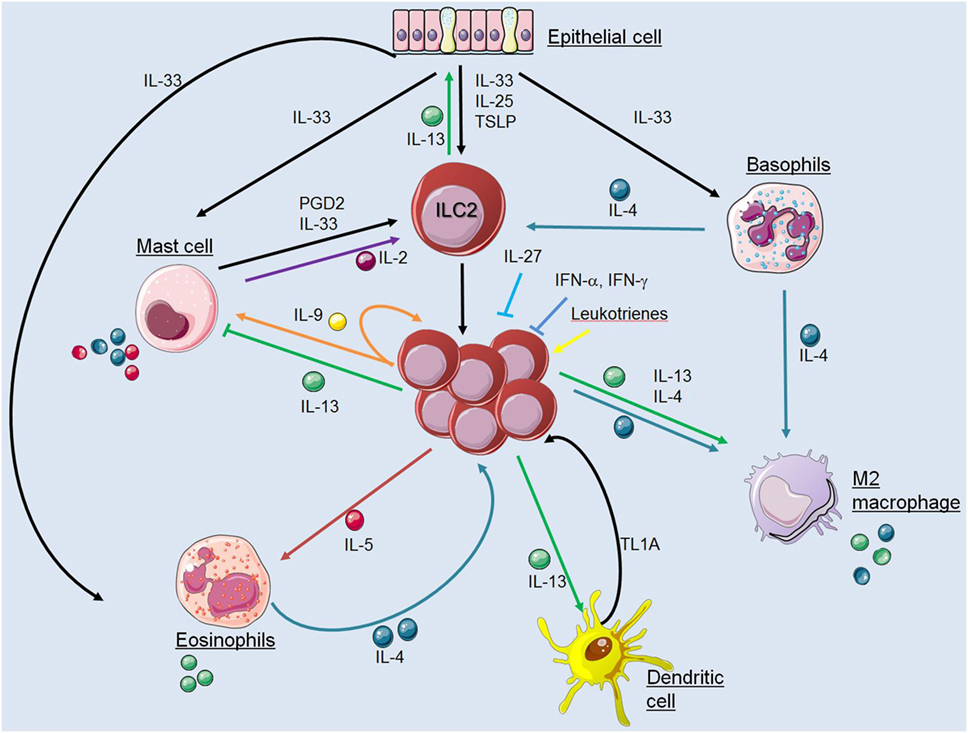
Figure 2. Interactions between innate lymphoid cells (ILC2s) and cells of the innate immune system. The figure illustrates communication pathways between ILC2s and other cells of the innate immune system by secretion and recognition of soluble factors as described in the main text. Solid arrows indicate published evidence for activating mechanisms whereas ILC2-derived IL-13 was shown to suppress mast cells and IL-27 or interferons inhibit ILC2 functions. Cell symbols where taken from http://smart.servier.com website.
Author Contributions
All authors listed have made a substantial, direct, and intellectual contribution to the work and approved it for publication.
Conflict of Interest Statement
The authors declare that the research was conducted in the absence of any commercial or financial relationships that could be construed as a potential conflict of interest.
Funding
This work was supported in part by the Deutsche Forschungsgemeinschaft DFG (grant VO944/8-1).
Abbreviations
ILC, innate lymphoid cells; PGD2, prostaglandin 2; CRTH2, cysteine-three-histidine protein 2; NK cell, natural killer cell; VAT, visceral adipose tissue; CLP, common lymphoid progenitor; ICOS, inducible T cell co-stimulator; VPAC2, vasoactive intestinal peptide receptor 2; FALC, fat-associated lymphoid cluster.
References
1. Spits H, Artis D, Colonna M, Diefenbach A, Di Santo JP, Eberl G, et al. Innate lymphoid cells – a proposal for uniform nomenclature. Nat Rev Immunol (2013) 13(2):145–9. doi:10.1038/nri3365
2. Hazenberg MD, Spits H. Human innate lymphoid cells. Blood (2014) 124(5):700–9. doi:10.1182/blood-2013-11-427781
3. Spits H, Di Santo JP. The expanding family of innate lymphoid cells: regulators and effectors of immunity and tissue remodeling. Nat Immunol (2011) 12(1):21–7. doi:10.1038/ni.1962
4. Yokota Y, Mansouri A, Mori S, Sugawara S, Adachi S, Nishikawa S, et al. Development of peripheral lymphoid organs and natural killer cells depends on the helix-loop-helix inhibitor Id2. Nature (1999) 397(6721):702–6. doi:10.1038/17812
5. Spits H, Cupedo T. Innate lymphoid cells: emerging insights in development, lineage relationships, and function. Annu Rev Immunol (2012) 30:647–75. doi:10.1146/annurev-immunol-020711-075053
6. Eberl G, Di Santo JP, Vivier E. The brave new world of innate lymphoid cells. Nat Immunol (2015) 16(1):1–5. doi:10.1038/ni.3059
7. Fort MM, Cheung J, Yen D, Li J, Zurawski SM, Lo S, et al. IL-25 induces IL-4, IL-5, and IL-13 and Th2-associated pathologies in vivo. Immunity (2001) 15(6):985–95. doi:10.1016/S1074-7613(01)00243-6
8. Moro K, Yamada T, Tanabe M, Takeuchi T, Ikawa T, Kawamoto H, et al. Innate production of T(H)2 cytokines by adipose tissue-associated c-Kit(+)Sca-1(+) lymphoid cells. Nature (2010) 463(7280):540–4. doi:10.1038/nature08636
9. Neill DR, Wong SH, Bellosi A, Flynn RJ, Daly M, Langford TK, et al. Nuocytes represent a new innate effector leukocyte that mediates type-2 immunity. Nature (2010) 464(7293):1367–70. doi:10.1038/nature08900
10. Price AE, Liang HE, Sullivan BM, Reinhardt RL, Eisley CJ, Erle DJ, et al. Systemically dispersed innate IL-13-expressing cells in type 2 immunity. Proc Natl Acad Sci U S A (2010) 107(25):11489–94. doi:10.1073/pnas.1003988107
11. Finkelman FD, Shea-Donohue T, Morris SC, Gildea L, Strait R, Madden KB, et al. Interleukin-4- and interleukin-13-mediated host protection against intestinal nematode parasites. Immunol Rev (2004) 201:139–55. doi:10.1111/j.0105-2896.2004.00192.x
12. Anthony RM, Rutitzky LI, Urban JF Jr, Stadecker MJ, Gause WC. Protective immune mechanisms in helminth infection. Nat Rev Immunol (2007) 7(12):975–87. doi:10.1038/nri2199
13. Coffman RL, von der Weid T. Multiple pathways for the initiation of T helper 2 (Th2) responses. J Exp Med (1997) 185(3):373–5. doi:10.1084/jem.185.3.373
14. Fallon PG, Jolin HE, Smith P, Emson CL, Townsend MJ, Fallon R, et al. IL-4 induces characteristic Th2 responses even in the combined absence of IL-5, IL-9, and IL-13. Immunity (2002) 17(1):7–17. doi:10.1016/S1074-7613(02)00332-1
15. Fallon PG, Ballantyne SJ, Mangan NE, Barlow JL, Dasvarma A, Hewett DR, et al. Identification of an interleukin (IL)-25-dependent cell population that provides IL-4, IL-5, and IL-13 at the onset of helminth expulsion. J Exp Med (2006) 203(4):1105–16. doi:10.1084/jem.20051615
16. Voehringer D, Reese TA, Huang X, Shinkai K, Locksley RM. Type 2 immunity is controlled by IL-4/IL-13 expression in hematopoietic non-eosinophil cells of the innate immune system. J Exp Med (2006) 203(6):1435–46. doi:10.1084/jem.20052448
17. Oeser K, Schwartz C, Voehringer D. Conditional IL-4/IL-13-deficient mice reveal a critical role of innate immune cells for protective immunity against gastrointestinal helminths. Mucosal Immunol (2015) 8(3):672–82. doi:10.1038/mi.2014.101
18. Kim HY, Chang YJ, Subramanian S, Lee HH, Albacker LA, Matangkasombut P, et al. Innate lymphoid cells responding to IL-33 mediate airway hyperreactivity independently of adaptive immunity. J Allergy Clin Immunol (2012) 129(1): 216–27.e1–6. doi:10.1016/j.jaci.2011.10.036
19. Chang YJ, DeKruyff RH, Umetsu DT. The role of type 2 innate lymphoid cells in asthma. J Leukoc Biol (2013) 94(5):933–40. doi:10.1189/jlb.0313127
20. Barlow JL, Bellosi A, Hardman CS, Drynan LF, Wong SH, Cruickshank JP, et al. Innate IL-13-producing nuocytes arise during allergic lung inflammation and contribute to airways hyperreactivity. J Allergy Clin Immunol (2012) 129(1):e1–4. doi:10.1016/j.jaci.2011.09.041
21. Imai Y, Yasuda K, Sakaguchi Y, Haneda T, Mizutani H, Yoshimoto T, et al. Skin-specific expression of IL-33 activates group 2 innate lymphoid cells and elicits atopic dermatitis-like inflammation in mice. Proc Natl Acad Sci U S A (2013) 110(34):13921–6. doi:10.1073/pnas.1307321110
22. Duerr CU, Fritz JH. Regulation of group 2 innate lymphoid cells. Cytokine (2016) 87:1–8. doi:10.1016/j.cyto.2016.01.018
23. Halim TYF. Group 2 innate lymphoid cells in disease. Int Immunol (2016) 28(1):13–22. doi:10.1093/intimm/dxv050
24. Nussbaum JC, Van Dyken SJ, von Moltke J, Cheng LE, Mohapatra A, Molofsky AB, et al. Type 2 innate lymphoid cells control eosinophil homeostasis. Nature (2013) 502(7470):245–8. doi:10.1038/nature12526
25. Motomura Y, Morita H, Moro K, Nakae S, Artis D, Endo TA, et al. Basophil-derived interleukin-4 controls the function of natural helper cells, a member of ILC2s, in lung inflammation. Immunity (2014) 40(5):758–71. doi:10.1016/j.immuni.2014.04.013
26. Bouchery T, Kyle R, Camberis M, Shepherd A, Filbey K, Smith A, et al. ILC2s and T cells cooperate to ensure maintenance of M2 macrophages for lung immunity against hookworms. Nat Commun (2015) 6:6970. doi:10.1038/ncomms7970
27. Halim TY, Steer CA, Mathä L, Gold MJ, Martinez-Gonzalez I, McNagny KM, et al. Group 2 innate lymphoid cells are critical for the initiation of adaptive T helper 2 cell-mediated allergic lung inflammation. Immunity (2014) 40(3):425–35. doi:10.1016/j.immuni.2014.01.011
28. Halim TY, Hwang YY, Scanlon ST, Zaghouani H, Garbi N, Fallon PG, et al. Group 2 innate lymphoid cells license dendritic cells to potentiate memory TH2 cell responses. Nat Immunol (2016) 17(1):57–64. doi:10.1038/ni.3294
29. Shik D, Tomar S, Lee JB, Chen CY, Smith A, Wang YH. IL-9-producing cells in the development of IgE-mediated food allergy. Semin Immunopathol (2017) 39(1):69–77. doi:10.1007/s00281-016-0605-x
30. Shimokawa C, Kanaya T, Hachisuka M, Ishiwata K, Hisaeda H, Kurashima Y, et al. Mast cells are crucial for induction of group 2 innate lymphoid cells and clearance of helminth infections. Immunity (2017) 46(5):863–74.e4. doi:10.1016/j.immuni.2017.04.017
31. Maazi H, et al. Activated plasmacytoid dendritic cells regulate type 2 innate lymphoid cells-mediated airway hyperreactivity. J Allergy Clin Immunol (2017). doi:10.1016/j.jaci.2017.04.043
32. Doherty TA, Khorram N, Lund S, Mehta AK, Croft M, Broide DH. Lung type 2 innate lymphoid cells express cysteinyl leukotriene receptor 1, which regulates TH2 cytokine production. J Allergy Clin Immunol (2013) 132(1):205–13. doi:10.1016/j.jaci.2013.03.048
33. Pelly VS, Kannan Y, Coomes SM, Entwistle LJ, Rückerl D, Seddon B, et al. IL-4-producing ILC2s are required for the differentiation of TH2 cells following Heligmosomoides polygyrus infection. Mucosal Immunol (2016) 9(6):1407–17. doi:10.1038/mi.2016.4
34. von Moltke J, O’Leary CE, Barrett NA, Kanaoka Y, Austen KF, Locksley RM. Leukotrienes provide an NFAT-dependent signal that synergizes with IL-33 to activate ILC2s. J Exp Med (2017) 214(1):27–37. doi:10.1084/jem.20161274
35. Lund SJ, Portillo A, Cavagnero K, Baum RE, Naji LH, Badrani JH, et al. Leukotriene C4 potentiates IL-33-induced group 2 innate lymphoid cell activation and lung inflammation. J Immunol (2017) 199(3):1096–104. doi:10.4049/jimmunol.1601569
36. Mjösberg J, Bernink J, Golebski K, Karrich JJ, Peters CP, Blom B, et al. The transcription factor GATA3 is essential for the function of human type 2 innate lymphoid cells. Immunity (2012) 37(4):649–59. doi:10.1016/j.immuni.2012.08.015
37. Gold MJ, Antignano F, Halim TY, Hirota JA, Blanchet MR, Zaph C, et al. Group 2 innate lymphoid cells facilitate sensitization to local, but not systemic, TH2-inducing allergen exposures. J Allergy Clin Immunol (2014) 133(4):1142–8. doi:10.1016/j.jaci.2014.02.033
38. Oliphant CJ, Hwang YY, Walker JA, Salimi M, Wong SH, Brewer JM, et al. MHCII-mediated dialog between group 2 innate lymphoid cells and CD4(+) T cells potentiates type 2 immunity and promotes parasitic helminth expulsion. Immunity (2014) 41(2):283–95. doi:10.1016/j.immuni.2014.06.016
39. Mirchandani AS, Besnard AG, Yip E, Scott C, Bain CC, Cerovic V, et al. Type 2 innate lymphoid cells drive CD4+ Th2 cell responses. J Immunol (2014) 192(5):2442–8. doi:10.4049/jimmunol.1300974
40. Drake LY, Iijima K, Kita H. Group 2 innate lymphoid cells and CD4+ T cells cooperate to mediate type 2 immune response in mice. Allergy (2014) 69(10):1300–7. doi:10.1111/all.12446
41. Hepworth MR, Monticelli LA, Fung TC, Ziegler CG, Grunberg S, Sinha R, et al. Innate lymphoid cells regulate CD4+ T-cell responses to intestinal commensal bacteria. Nature (2013) 498(7452):113–7. doi:10.1038/nature12240
42. Maazi H, Patel N, Sankaranarayanan I, Suzuki Y, Rigas D, Soroosh P, et al. ICOS:ICOS-ligand interaction is required for type 2 innate lymphoid cell function, homeostasis, and induction of airway hyperreactivity. Immunity (2015) 42(3):538–51. doi:10.1016/j.immuni.2015.02.007
43. Miyazaki M, Miyazaki K, Chen S, Itoi M, Miller M, Lu LF, et al. Id2 and Id3 maintain the regulatory T cell pool to suppress inflammatory disease. Nat Immunol (2014) 15(8):767–76. doi:10.1038/ni.2928
44. Noval Rivas M, Burton OT, Oettgen HC, Chatila T. IL-4 production by group 2 innate lymphoid cells promotes food allergy by blocking regulatory T-cell function. J Allergy Clin Immunol (2016) 138(3):801–11.e9. doi:10.1016/j.jaci.2016.02.030
45. Rauber S, Luber M, Weber S, Maul L, Soare A, Wohlfahrt T, et al. Resolution of inflammation by interleukin-9-producing type 2 innate lymphoid cells. Nat Med (2017) 23(8):938–44. doi:10.1038/nm.4373
46. Zaiss DM, van Loosdregt J, Gorlani A, Bekker CP, Gröne A, Sibilia M, et al. Amphiregulin enhances regulatory T cell-suppressive function via the epidermal growth factor receptor. Immunity (2013) 38(2):275–84. doi:10.1016/j.immuni.2012.09.023
47. Schiering C, Krausgruber T, Chomka A, Fröhlich A, Adelmann K, Wohlfert EA, et al. The alarmin IL-33 promotes regulatory T-cell function in the intestine. Nature (2014) 513(7519):564–8. doi:10.1038/nature13577
48. Matta BM, Lott JM, Mathews LR, Liu Q, Rosborough BR, Blazar BR, et al. IL-33 is an unconventional alarmin that stimulates IL-2 secretion by dendritic cells to selectively expand IL-33R/ST2+ regulatory T cells. J Immunol (2014) 193(8):4010–20. doi:10.4049/jimmunol.1400481
49. Molofsky AB, Van Gool F, Liang HE, Van Dyken SJ, Nussbaum JC, Lee J, et al. Interleukin-33 and interferon-gamma counter-regulate group 2 innate lymphoid cell activation during immune perturbation. Immunity (2015) 43(1):161–74. doi:10.1016/j.immuni.2015.05.019
50. Pelly VS, Coomes SM, Kannan Y, Gialitakis M, Entwistle LJ, Perez-Lloret J, et al. Interleukin 4 promotes the development of ex-Foxp3 Th2 cells during immunity to intestinal helminths. J Exp Med (2017) 214(6):1809–26. doi:10.1084/jem.20161104
51. Rigas D, Lewis G, Aron JL, Wang B, Banie H, Sankaranarayanan I, et al. Type 2 innate lymphoid cell suppression by regulatory T cells attenuates airway hyperreactivity and requires inducible T-cell costimulator-inducible T-cell costimulator ligand interaction. J Allergy Clin Immunol (2017) 139(5):1468–77.e2. doi:10.1016/j.jaci.2016.08.034
52. Paclik D, Stehle C, Lahmann A, Hutloff A, Romagnani C. ICOS regulates the pool of group 2 innate lymphoid cells under homeostatic and inflammatory conditions in mice. Eur J Immunol (2015) 45(10):2766–72. doi:10.1002/eji.201545635
53. Bénézech C, Luu NT, Walker JA, Kruglov AA, Loo Y, Nakamura K, et al. Inflammation-induced formation of fat-associated lymphoid clusters. Nat Immunol (2015) 16(8):819–28. doi:10.1038/ni.3215
54. Jackson-Jones LH, Duncan SM, Magalhaes MS, Campbell SM, Maizels RM, McSorley HJ, et al. Fat-associated lymphoid clusters control local IgM secretion during pleural infection and lung inflammation. Nat Commun (2016) 7:12651. doi:10.1038/ncomms12651
55. Drake LY, Iijima K, Bartemes K, Kita H. Group 2 innate lymphoid cells promote an early antibody response to a respiratory antigen in mice. J Immunol (2016) 197(4):1335–42. doi:10.4049/jimmunol.1502669
56. Feldman S, Kasjanski R, Poposki J, Hernandez D, Chen JN, Norton JE, et al. Chronic airway inflammation provides a unique environment for B cell activation and antibody production. Clin Exp Allergy (2017) 47(4):457–66. doi:10.1111/cea.12878
57. Fukuoka A, Futatsugi-Yumikura S, Takahashi S, Kazama H, Iyoda T, Yoshimoto T, et al. Identification of a novel type 2 innate immunocyte with the ability to enhance IgE production. Int Immunol (2013) 25(6):373–82. doi:10.1093/intimm/dxs160
58. Van Dyken SJ, Locksley RM. Interleukin-4- and interleukin-13-mediated alternatively activated macrophages: roles in homeostasis and disease. Annu Rev Immunol (2013) 31:317–43. doi:10.1146/annurev-immunol-032712-095906
59. Besnard AG, Guabiraba R, Niedbala W, Palomo J, Reverchon F, Shaw TN, et al. IL-33-mediated protection against experimental cerebral malaria is linked to induction of type 2 innate lymphoid cells, M2 macrophages and regulatory T cells. PLoS Pathog (2015) 11(2):e1004607. doi:10.1371/journal.ppat.1004607
60. Piehler D, Eschke M, Schulze B, Protschka M, Müller U, Grahnert A, et al. The IL-33 receptor (ST2) regulates early IL-13 production in fungus-induced allergic airway inflammation. Mucosal Immunol (2016) 9(4):937–49. doi:10.1038/mi.2015.106
61. Hams E, Armstrong ME, Barlow JL, Saunders SP, Schwartz C, Cooke G, et al. IL-25 and type 2 innate lymphoid cells induce pulmonary fibrosis. Proc Natl Acad Sci U S A (2014) 111(1):367–72. doi:10.1073/pnas.1315854111
62. Molofsky AB, Nussbaum JC, Liang HE, Van Dyken SJ, Cheng LE, Mohapatra A, et al. Innate lymphoid type 2 cells sustain visceral adipose tissue eosinophils and alternatively activated macrophages. J Exp Med (2013) 210(3):535–49. doi:10.1084/jem.20121964
63. Wu D, Molofsky AB, Liang HE, Ricardo-Gonzalez RR, Jouihan HA, Bando JK, et al. Eosinophils sustain adipose alternatively activated macrophages associated with glucose homeostasis. Science (2011) 332(6026):243–7. doi:10.1126/science.1201475
64. Lee MW, Odegaard JI, Mukundan L, Qiu Y, Molofsky AB, Nussbaum JC, et al. Activated type 2 innate lymphoid cells regulate beige fat biogenesis. Cell (2015) 160(1–2):74–87. doi:10.1016/j.cell.2014.12.011
65. Fischer K, Ruiz HH, Jhun K, Finan B, Oberlin DJ, van der Heide V, et al. Alternatively activated macrophages do not synthesize catecholamines or contribute to adipose tissue adaptive thermogenesis. Nat Med (2017) 23(5):623–30. doi:10.1038/nm.4316
66. Reitman ML. How does fat transition from white to beige? Cell Metab (2017) 26(1):14–6. doi:10.1016/j.cmet.2017.06.011
67. Chang YJ, Kim HY, Albacker LA, Baumgarth N, McKenzie AN, Smith DE, et al. Innate lymphoid cells mediate influenza-induced airway hyper-reactivity independently of adaptive immunity. Nat Immunol (2011) 12(7):631–8. doi:10.1038/ni.2045
68. Chu DK, Jimenez-Saiz R, Verschoor CP, Walker TD, Goncharova S, Llop-Guevara A, et al. Indigenous enteric eosinophils control DCs to initiate a primary Th2 immune response in vivo. J Exp Med (2014) 211(8):1657–72. doi:10.1084/jem.20131800
69. Meylan F, Davidson TS, Kahle E, Kinder M, Acharya K, Jankovic D, et al. The TNF-family receptor DR3 is essential for diverse T cell-mediated inflammatory diseases. Immunity (2008) 29(1):79–89. doi:10.1016/j.immuni.2008.04.021
70. Meylan F, Hawley ET, Barron L, Barlow JL, Penumetcha P, Pelletier M, et al. The TNF-family cytokine TL1A promotes allergic immunopathology through group 2 innate lymphoid cells. Mucosal Immunol (2014) 7(4):958–68. doi:10.1038/mi.2013.114
71. Yu X, Pappu R, Ramirez-Carrozzi V, Ota N, Caplazi P, Zhang J, et al. TNF superfamily member TL1A elicits type 2 innate lymphoid cells at mucosal barriers. Mucosal Immunol (2014) 7(3):730–40. doi:10.1038/mi.2013.92
72. Duerr CU, McCarthy CD, Mindt BC, Rubio M, Meli AP, Pothlichet J, et al. Type I interferon restricts type 2 immunopathology through the regulation of group 2 innate lymphoid cells. Nat Immunol (2016) 17(1):65–75. doi:10.1038/ni.3308
73. Moro K, Kabata H, Tanabe M, Koga S, Takeno N, Mochizuki M, et al. Interferon and IL-27 antagonize the function of group 2 innate lymphoid cells and type 2 innate immune responses. Nat Immunol (2016) 17(1):76–86. doi:10.1038/ni.3309
74. Mchedlidze T, Kindermann M, Neves AT, Voehringer D, Neurath MF, Wirtz S. IL-27 suppresses type 2 immune responses in vivo via direct effects on group 2 innate lymphoid cells. Mucosal Immunol (2016) 9(6):1384–94. doi:10.1038/mi.2016.20
75. Bi J, Cui L, Yu G, Yang X, Chen Y, Wan X. NK cells alleviate lung inflammation by negatively regulating group 2 innate lymphoid cells. J Immunol (2017) 198(8):3336–44. doi:10.4049/jimmunol.1601830
76. Kumagai K, Lewandowski R, Jackson-Humbles DN, Li N, Van Dyken SJ, Wagner JG, et al. Ozone-induced nasal type 2 immunity in mice is dependent on innate lymphoid cells. Am J Respir Cell Mol Biol (2016) 54(6):782–91. doi:10.1165/rcmb.2015-0118OC
77. Halim TY, Krauss RH, Sun AC, Takei F. Lung natural helper cells are a critical source of Th2 cell-type cytokines in protease allergen-induced airway inflammation. Immunity (2012) 36(3):451–63. doi:10.1016/j.immuni.2011.12.020
78. Blanchard C, Rothenberg ME. Biology of the eosinophil. Adv Immunol (2009) 101:81–121. doi:10.1016/S0065-2776(08)01003-1
79. Stolarski B, Kurowska-Stolarska M, Kewin P, Xu D, Liew FY. IL-33 exacerbates eosinophil-mediated airway inflammation. J Immunol (2010) 185(6):3472–80. doi:10.4049/jimmunol.1000730
80. Matsuba-Kitamura S, Yoshimoto T, Yasuda K, Futatsugi-Yumikura S, Taki Y, Muto T, et al. Contribution of IL-33 to induction and augmentation of experimental allergic conjunctivitis. Int Immunol (2010) 22(6):479–89. doi:10.1093/intimm/dxq035
81. Bal SM, Bernink JH, Nagasawa M, Groot J, Shikhagaie MM, Golebski K, et al. IL-1beta, IL-4 and IL-12 control the fate of group 2 innate lymphoid cells in human airway inflammation in the lungs. Nat Immunol (2016) 17(6):636–45. doi:10.1038/ni.3444
82. Kim BS, Wang K, Siracusa MC, Saenz SA, Brestoff JR, Monticelli LA, et al. Basophils promote innate lymphoid cell responses in inflamed skin. J Immunol (2014) 193(7):3717–25. doi:10.4049/jimmunol.1401307
83. Doherty TA, Khorram N, Chang JE, Kim HK, Rosenthal P, Croft M, et al. STAT6 regulates natural helper cell proliferation during lung inflammation initiated by Alternaria. Am J Physiol Lung Cell Mol Physiol (2012) 303(7):L577–88. doi:10.1152/ajplung.00174.2012
84. Barnig C, Cernadas M, Dutile S, Liu X, Perrella MA, Kazani S, et al. Lipoxin A4 regulates natural killer cell and type 2 innate lymphoid cell activation in asthma. Sci Transl Med (2013) 5(174):174ra26. doi:10.1126/scitranslmed.3004812
85. Roediger B, Kyle R, Yip KH, Sumaria N, Guy TV, Kim BS, et al. Cutaneous immunosurveillance and regulation of inflammation by group 2 innate lymphoid cells. Nat Immunol (2013) 14(6):564–73. doi:10.1038/ni.2584
86. Xue L, Salimi M, Panse I, Mjösberg JM, McKenzie AN, Spits H, et al. Prostaglandin D2 activates group 2 innate lymphoid cells through chemoattractant receptor-homologous molecule expressed on TH2 cells. J Allergy Clin Immunol (2014) 133(4):1184–94. doi:10.1016/j.jaci.2013.10.056
87. Chang JE, Doherty TA, Baum R, Broide D. Prostaglandin D2 regulates human type 2 innate lymphoid cell chemotaxis. J Allergy Clin Immunol (2014) 133(3):899–901.e3. doi:10.1016/j.jaci.2013.09.020
88. Mjösberg JM, Trifari S, Crellin NK, Peters CP, van Drunen CM, Piet B, et al. Human IL-25- and IL-33-responsive type 2 innate lymphoid cells are defined by expression of CRTH2 and CD161. Nat Immunol (2011) 12(11):1055–62. doi:10.1038/ni.2104
89. Lefrançais E, Duval A, Mirey E, Roga S, Espinosa E, Cayrol C, et al. Central domain of IL-33 is cleaved by mast cell proteases for potent activation of group-2 innate lymphoid cells. Proc Natl Acad Sci U S A (2014) 111(43):15502–7. doi:10.1073/pnas.1410700111
90. Hsu CL, Neilsen CV, Bryce PJ. IL-33 is produced by mast cells and regulates IgE-dependent inflammation. PLoS One (2010) 5(8):e11944. doi:10.1371/journal.pone.0011944
91. Morita H, Arae K, Unno H, Miyauchi K, Toyama S, Nambu A, et al. An interleukin-33-mast cell-interleukin-2 axis suppresses papain-induced allergic inflammation by promoting regulatory T cell numbers. Immunity (2015) 43(1):175–86. doi:10.1016/j.immuni.2015.06.021
92. Moretti S, Renga G, Oikonomou V, Galosi C, Pariano M, Iannitti RG, et al. A mast cell-ILC2-Th9 pathway promotes lung inflammation in cystic fibrosis. Nat Commun (2017) 8:14017. doi:10.1038/ncomms14017
Keywords: allergic inflammation, helminths, mast cells, innate lymphoid cells, eosinophils, basophils, Th2 cells, alternatively activated macrophages
Citation: Symowski C and Voehringer D (2017) Interactions between Innate Lymphoid Cells and Cells of the Innate and Adaptive Immune System. Front. Immunol. 8:1422. doi: 10.3389/fimmu.2017.01422
Received: 28 July 2017; Accepted: 12 October 2017;
Published: 30 October 2017
Edited by:
Chiara Romagnani, Deutsches Rheuma-Forschungszentrum (DRFZ), GermanyReviewed by:
Jorg Hermann Fritz, McGill University, CanadaChristoph Wilhelm, University of Bonn, Germany
Copyright: © 2017 Symowski and Voehringer. This is an open-access article distributed under the terms of the Creative Commons Attribution License (CC BY). The use, distribution or reproduction in other forums is permitted, provided the original author(s) or licensor are credited and that the original publication in this journal is cited, in accordance with accepted academic practice. No use, distribution or reproduction is permitted which does not comply with these terms.
*Correspondence: David Voehringer, david.voehringer@uk-erlangen.de