- 1Department of Rehabilitation Medicine, The First Affiliated Hospital, Sun Yat-sen University, Guangzhou, Guangdong, China
- 2Department of Rehabilitation Sciences, The Hong Kong Polytechnic University, Hong Kong SAR, China
Background: Upper limb motor impairment is a common consequence of stroke, and the effectiveness and underlying mechanisms of rehabilitation therapy for improving upper limb function remain uncertain. Functional near-infrared spectroscopy, a reliable wearable neuroimaging technique, holds promise for investigating brain activity during functional tasks. This study aims to explore the synchronous oxygenation characteristics of the central cortex and upper-limb flexors during a grasping task and investigate the rehabilitation mechanisms of upper limb motor function in individuals with stroke.
Methods: Participants with stroke who demonstrate the ability to grasp and lift cubic wood blocks of different sizes (2.5cm3, 5cm3, and 10cm3) using their affected hand will be divided into three groups: A, B, and C. Each group will consist of twenty stroke patients, resulting in a total of sixty participants with stroke. Additionally, twenty matched healthy subjects will be enrolled as a control group. Comprehensive assessments will be conducted before and after the intervention, including blood oxygen parameter monitoring of the cerebral cortex and upper limb flexors using fNIRS during the grasping task. Other assessments will include MyotonPRO, the Modified Ashworth Scale, the upper extremity section of the Fugl–Meyer Assessment, the Action Research Arm Test, and the Modified Barthel Index. The study will be undertaken between January 2024 and September 2025.
Conclusions: The results of this trial will provide an in-depth understanding of the Characteristics of central cortex and upper-limb flexors synchronous oxygenation during grasping task and how it may relate to the rehabilitation mechanism of upper limb motor function in people with stroke.
Clinical trial registration: https://www.chictr.org.cn, identifier ChiCTR2400080619.
Introduction
Stroke, a leading cause of death and disability (GBD Stroke Collaborators, 2019; Stinear et al., 2020), poses a growing challenge due to the aging population and improved survival rates (Li, 2020). Studies have indicated that a significant proportion of stroke patients experience upper limb motor impairment, both in the acute and chronic stages of recovery (Grattan et al., 2019). This impairment directly affects patients’ quality of life and places a substantial economic burden on families and society.
Functional rehabilitation after stroke is believed to result from reorganization within the central nervous system. Understanding the relationship between these neurobiological changes and the recovery process is crucial for helping effective treatment approaches. Non-invasive techniques such as functional magnetic resonance imaging (fMRI), transcranial magnetic stimulation (TMS), and electroencephalography (EEG) have provided valuable insights into the functioning of the human brain (Ward, 2004). Previously, fMRI was employed to investigate intraregional activation and interregional connectivity during bilateral (antiphase and in-phase) and unilateral upper limb movements (Lin et al., 2017), while EEG was utilized to study cognitive anticipatory processes during palm grasping in subacute patients following stroke (Chen et al., 2018). However, these techniques have limitations, such as environmental requirements and human activity, which somewhat hinder the study of cortical activity during functional tasks. In this regard, near-infrared spectroscopy (NIRS) emerges as a novel and non-invasive functional neuroimaging technology. By indirectly assessing cerebral cortex and muscle tissue activity through the detection of blood oxygen parameters [Oxygenated Hemoglobin (HbO), Deoxygenated Hemoglobin (HbR), and Total Oxygenated Hemoglobin (HbT)] based on the neurovascular coupling mechanism, NIRS offers a promising method for investigating neurovascular activity during functional motor tasks. Unlike other techniques, NIRS imposes fewer physical and environmental restrictions and has minimal contraindications (Scholkmann et al., 2014), thereby facilitating the study of cortical activity characteristics in human functional activities.
Current research (Kasahara et al., 2011; Arnemann et al., 2015) in patients following stroke has focus on understanding how focal brain injuries affect brain networks and whether these changes are associated with functional recovery. However, the underlying mechanisms of functional recovery after stroke are still actively being investigated (Ward, 2004). Furthermore, there remains a notable discrepancy between clinical evaluation results and real-life functional abilities of patients (Greeley et al., 2021). To bridge this gap, there is a growing interest in portable neuroimaging techniques that can objectively measure the neurophysiology related to functional behavior (Greeley et al., 2021). Functional near infrared spectroscopy (fNIRS) is a validated and reliable neuroimaging technique that can be used as a wearable device during functional activities (Plichta et al., 2006). It has demonstrated high consistency with fMRI results in detecting cortical activities (Huppert et al., 2017). Some studies have utilized fNIRS to investigate sensorimotor stimulation of the affected hand in patients following stroke though median nerve electrical stimulation (Huo et al., 2019a). However, to date, there have been no reports on the neurophysiological activity of cerebral cortex function region detected by fNIRS during upper limb grasping function in patients following stroke.
While muscle NIRS technology has been widely used in laboratory and exercise settings to observe changes in muscle metabolism and oxygenation (Perrey and Ferrari, 2018), its application has primarily focus on athletes (Giovanelli et al., 2020), healthy people (Beever et al., 2020), and conditions such as cystic fibrosis (Werkman et al., 2016). There is currently a lack of studies utilizing NIRS to examine upper limb muscle hemodynamics in stroke patients, particularly during grasping tasks. Therefore, there is a need to investigate the immediate muscle hemodynamics of upper limb grasping tasks in stroke patients using NIRS, as this will provide valuable insights into the neurophysiological aspects of motor function recovery in this population.
Virtual reality (VR) training which can induce the active participation of patients and provide more repetitive training (Levin et al., 2015), has gained attention as a rehabilitation intervention for improving upper limb function and quality of life in patients following stroke (Lo et al., 2017a). While some studies have shown beneficial effects of VR training on upper limb motor function recovery, a multi-center randomized controlled trial using a commercial gaming system failed to demonstrate superiority over traditional occupational therapy (Saposnik et al., 2016). However, literature analysis suggests that VR systems providing task-based training can significantly enhance upper limb function in stroke patients (Lohse et al., 2014). Previous research combining VR training with conventional rehabilitation therapy in patients with cerebral infarction has shown long-lasting improvements in upper limb motor function (Laver et al., 2017). In our previous prospective clinical study on rehabilitation intervention of multi-sensory interactive training for patients with cerebral infarction, it was found that virtual reality training combined with conventional rehabilitation therapy could improve upper limb motor function of patients with cerebral infarction and the effect could last for 1 year (Lo et al., 2017a; Zhao et al., 2019c). With the increasing application of VR in stroke rehabilitation, understanding its impact on neuronal activity and motor function becomes crucial.
The primary objective of this study is to investigate the characteristics of oxygenation in the central cortex and upper-limb flexors during grasping tasks in individuals with stroke. fNIRS systems will be used to simultaneously assess blood flow dynamics in the central cortex and upper-limb flexors during grasping tasks, evaluating the heterogeneity of muscle oxygenation activity in the upper-limb flexors and analyzing the activation levels of cerebral cortex functional areas. The secondary objective is to explore the hemodynamic alterations in the cerebral cortex and flexor muscles of the affected upper limb during grasping tasks, induced by the combination of VR and conventional rehabilitation therapy, to attempt to investigate the rehabilitation mechanisms of upper limb motor function in individuals with stroke. Participants with stroke will undergo a combined intervention of VR training and conventional rehabilitation therapy. Changes of hemodynamic in cerebral cortex and upper limb flexor hemodynamics, as well as upper limb motor function, will be evaluated before and after the intervention. Furthermore, correlations between each parameter will be analyzed to gain insights into the relationship between VR training, neuronal activity, and upper limb motor function in stroke patients.
Methods and design
Study design
This study follows a longitudinal non-randomized controlled design to examine the synchronous oxygenation characteristics of the central cortex and upper-limb flexors during a grasping task in stroke patients with varying levels of grasp function.
Study setting
The study is conducted at the Department of Rehabilitation Medicine, First Affiliated Hospital of Sun Yat-sen University in China. Participants are recruited from the inpatient ward, and all interventions are delivered within the Hospital Rehabilitation Department by the hospital staff.
Recruitment
Eligible patients admitted to the inpatient ward are screened as part of routine assessment. The clinical team identifies suitable participants and provides them with written information about the study. A member of the research team then approaches the potential participants, explains the study further, and obtains written consent from those interested in participating. A screening log is maintained to record details of non-recruited patients and reasons for exclusion.
Ethics
Data collection will be performed according to the World Medical Association Declaration of Helsinki. The study has received ethical approval from the Human Subjects Ethics Subcommittee of the First Affiliated Hospital of Sun Yat-sen University (Ethical approval number: [2022] 308). Additionally, the study is registered in the Chinese Clinical Trial Registry (Registration No.: ChiCTR2400080619, registered on 2 February 2024). Any significant modifications to the study protocol will be communicated to all relevant parties.
Participants
Inclusion Criteria:
1. First stroke confirmed by CT or MRI.
2. Medically stable.
3. Age between 40 and 80 years.
4. Onset of stroke occurred between two weeks to three months.
5. Right handedness.
6. No severe cognitive impairment (MMSE ≥ 22 points) and able to follow motor commands.
7. Able to maintain an upright position in an armless seat for at least 30 min.
8. Modified Ashworth grade of the affected upper limb is equal to or less than grade 2.
9. Brunnstrom motor function grade of the affected hand is equal to or greater than grade 3, and able to grasp a 2.5cm3 wood block with effort.
10. Patient and their family members agree to participate in the study and have signed the informed consent.
Exclusion Criteria:
1. Unstable clinical condition.
2. Presence of any neuropsychiatric illness other than stroke.
3. Diagnosis of schizophrenia, bipolar disorder, obsessive-compulsive disorder, personality disorder, or major depression.
4. Presence of skin lesions or infections on the upper limb.
5. History of upper limb fractures, deformities, or finger absence.
Sample Size:
Based on the sample size of previous studies (Huo et al., 2019a), a total of 60 patients following stroke will be enrolled in this study, with 20 patients in each of the three groups.
Group A: Patients who can only grasp and lift a 2.5 cm3 wooden block with their hemiplegic hand.
Group B: Patients who can grasp and lift 2.5 cm3 and 5 cm3 blocks with their hemiplegic hand but cannot lift 10 cm3 blocks.
Group C: Patients who can pick up 2.5 cm3, 5 cm3, and 10 cm3 wood blocks with their hemiplegic hand but exhibit abnormalities in speed and coordination during the task.
In addition, a control group of 20 age-matched healthy subjects without neurological diseases or history of upper limb trauma will also be enrolled.
Experimental procedure
All stroke participants will undergo a comprehensive assessment both before and after the intervention. The assessment will involve the use of fNIRS to simultaneously monitor the blood oxygen parameters of the cerebral cortex and upper limb flexors during a grasping task. Additionally, pre- and post-intervention assessments will include the Action Research Arm Test (ARAT), the upper extremity section of the Fugl-Meyer Assessment (FMA-UE), the Modified Ashworth Scale (MAS), MyotonPRO, and the Modified Barthel Index (MBI).
During the intervention period, all stroke participants will receive 30 min of VR training and 30 min of occupational therapy once a day, five times a week, for a total of three consecutive weeks. Figure 1 shows the study procedure.
fNIRS data acquisition and analysis
The fNIRS data acquisition process is illustrated in Figures 2, 3. The participants were seated comfortably in an adjustable chair within a quiet room. For participants in Group A, they were instructed to grasp a 2.5 cm3 wooden block first with their unaffected hand and then with their affected hand. Participants in Group B performed two sets of tests, grasping a 2.5 cm3 wooden block and a 5 cm3 wooden block, using their unaffected hand first and then their affected hand. Subjects in Group C were asked to sequentially pick up three blocks of different sizes, again using their unaffected hand first and then their affected hand. The control group was required to grasp and lift three different sizes of cubic wooden blocks, first with their right hand and then with their left hand.
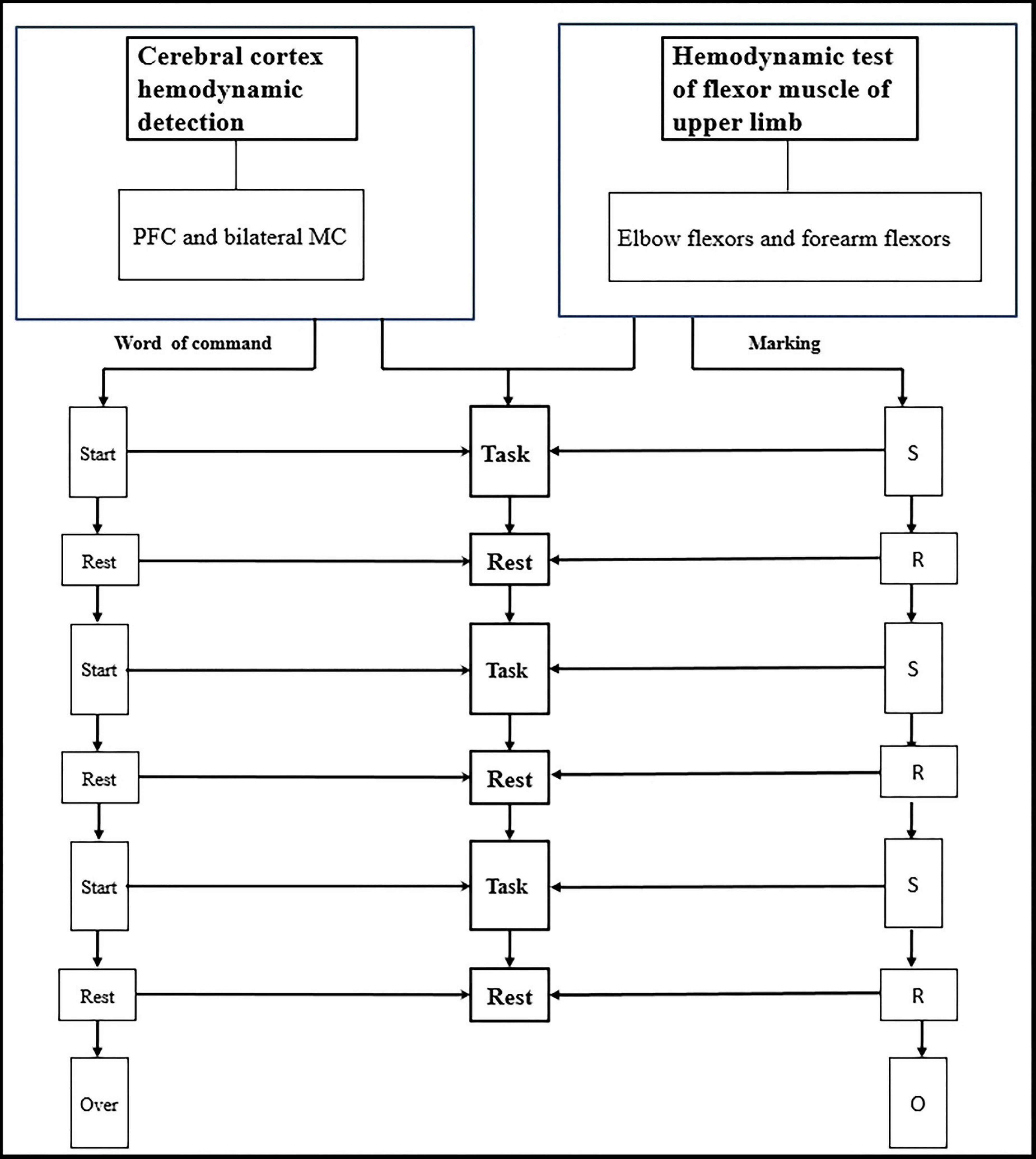
Figure 3. Blood oxygen parameters of cerebral cortex and upper limb flexors are synchronously detected during the grasping task.
Before the actual data collection, a practice session was conducted to familiarize participants with the tasks. The fNIRS data collection followed a block design paradigm. Each condition was preceded by 50 s of rest, which served as a baseline period to stabilize the fNIRS signal. Between each condition, participants were allowed a 2-min break. Each condition consisted of three blocks, with each block consisting of a 20-s motor task period followed by a 20-s rest period. During all rest periods, participants were instructed to keep their focus still on the handle of the ARAT toolbox.
fNIRS system and data analysis of cerebral cortex
In this study, the NirSmart-6000A equipment (Danyang Huichuang Medical equipment Co., Ltd, China) was used to continuously measure and record changes in the concentration of HbO and HbR in the cerebral cortex during the grasping task. The system consisted of near-infrared light sources (LEDs) and avalanche photodiodes (APDs) as detectors. The wavelengths used were 730 nm and 850 nm for the LED and APD, respectively, and the sampling rate was 11 Hz. Fourteen light sources and fourteen detectors were used, forming a total of 35 effective channels (CHs) as shown in Figure 4. The average distance between the light sources and detectors was 3 cm, ranging from 2.7 cm to 3.3 cm. To define the coordinates of the acquired channels, an NIRS-EEG compatible cap from EASYCAP, Herrsching, Germany, based on the international 10/20 system, was used for localization. The brain regions observed in this study were the bilateral prefrontal cortex (LPFC/RPFC), motor cortex (LMC/RMC), and occipital lobe (LOL/ROL), according to existing literature. No detector signal supersaturation occurred during the recording process. The middle point of each channel was defined as the main brain region detected by that channel, and the coordinates of each channel were calibrated using this point as the center of a circle. The Montreal Neurological Institute (MNI) coordinates of each channel were calculated and registered based on the 10-20 system using the NIRSpark (Danyang Huichuang Medical equipment Co., Ltd, China). The corresponding brain regions were then identified in the adult Brodmann Chris Rorden’s MRIcro brain model. The coordinates of each channel and the calibration information of the brain regions are provided in Table 1.
For data analysis, the NirSpark software package, running in MATLAB (The Mathworks, USA), was utilized. The data preprocessing involved several steps, including eliminating irrelevant time intervals, removing non-related artifacts, converting light intensity to optical density, applying a band-pass filter, converting optical density to blood oxygen concentration, and setting parameters for the hemodynamic response function (HRF). The HRF initial time was set to −2 s, and the end time was set to 40 s, with a baseline state from −2 s to 0 s and a single block paradigm from 0 s to 40 s. Both the “start” and “rest” durations were set to 20 s. The blood oxygen concentration data from the three block paradigms were then averaged to generate a block average result. These preprocessing steps allowed for meaningful analysis of the fNIRS data, providing insights into changes in cerebral blood flow and oxygenation during the experimental conditions.
In this study, the Generalized Linear Model (GLM) (Uga et al., 2014) was utilized to analyze the pre-processed time-series data of HbO for each channel and subject. A t-test was performed (P < 0.05) to compare the baseline and task states. The GLM allowed for the establishment of an ideal Hemodynamic Response Function (HRF) specific to each task and subject. The similarity between the experimental and ideal HRF values was evaluated (Chung et al., 2018). The beta value, representing the level of cortical activation for each channel, was used as an estimate of the HRF prediction for the HbO signal (Kawabata et al., 2019). It indicates the peak value of the HRF function and serves as a measure of cortical activation.
Wavelet amplitude (WA) of hemoglobin signal calculated by wavelet transform was used to quantify the intensity of cortical activity. For a single channel, 3 time windows of 20 s were divided to calculate the average WA value in the frequency domain, that is, 3 cortical activity intensity indices could be obtained. The intensity of cortical activity in a cortical region was calculated as the average WA value for all channels in that region. The WA-based laterality metric was calculated by the following equation:
where WA affected denotes the mean WA value in the cortical region of affected hemisphere and WA unaffected denotes the mean WA value in the homologous cortical region of the unaffected hemisphere. The value of WA laterality ranges from 1 to 1. Positive values imply that the affected side cortical activity is greater than the unaffected side, and negative values imply that the unaffected side is greater than the affected side.
fNIRS system and data analysis of upper limb flexors
In this study, an OctaMon M system (Artinis, LLC, New York, NY, USA) was used to continuously measure and record changes in the concentration of HbO and HbR in the upper limb flexors during the grasp task. The system consisted of two receivers and eight transmitter probes, forming a total of eight effective channels (CHs) as shown in Figure 5. The distance between the transmitter and receiver probes was 35 mm for the OctaMon M system, and the inter-optode distance was less than 3 mm. The absorption of near-infrared light at two different wavelengths (760.0 nm and 850.0 nm) was measured, and a sampling rate of 10 Hz was used during the measurements. The channel widths were adaptable and ranged from 25 mm to 40 mm. With a 2 × 4 channel configuration, changes in oxyhemoglobin, deoxyhemoglobin, and total hemoglobin values could be regionally monitored.
During the experiments, the OctaMon M system was placed on the flexors of the elbow and wrist to record the parameters of interest. When locating the muscle holder 1 on the flexors of the forearm, the receiver 1 was positioned at the upper one-third distance from the external epicondyle of the humerus to the styloid process of the ulna. When locating the muscle holder 2 on the elbow flexors, the receiver 2 was positioned at the lower one-third distance from the medial epicondyle of the humerus to the acromion. The arrangement of fNIRS channels for the upper limb flexors is shown in Figure 5.
For data analysis, dedicated software called OxySoft from Elst, The Netherlands, was used to analyze the system data. The fNIRS signal was transferred directly to a PC computer using a Bluetooth device. The collected data were exported to a text file and subsequently analyzed using MATLAB software.
Interventions
In this study, two different rehabilitation interventions were used: Virtual Reality Training (VR) and Conventional Occupational Therapy (OT).
For the VR training, the Arm Guider upper limb rehabilitation training system (Shanghai ZD Medical Technology Co., Ltd, China) was used. This system is specifically designed for individuals with upper limb dysfunction and provides various modes of training, including passive, assisting, active, and resistance training. The goal of VR training is to enhance proprioception, promote brain function remodeling, and reconstruct the neural system network. It combines exercise training with immersive game scenes to improve control, coordination, strength, and joint motion of the upper limbs, as well as train memory, understanding, and spatial structure imagination. During the first training session, the range of movement was individually set up, and the training mode was selected based on the residual motor function of the affected upper extremity. Real-time visual feedback was provided through a monitor located approximately 1 meter away from the patients to assist in task-oriented training. Additionally, real-time auditory feedback was given to indicate the quality of performance. The training sessions were conducted by an experienced occupational therapist for 30 min each, with rest intervals. The VR training was administered once a day, five times a week, for a total of three consecutive weeks.
Conventional OT was also performed by the same occupational therapist who had 15 years of experience in stroke occupational treatment. The OT treatment primarily focused on traditional neuromuscular promotion techniques, including joint compression, muscle extension, upper limb and hand functional training, and activities of daily living (ADL) training. Each OT session lasted for 30 min and was conducted once a day, five times a week, for three consecutive weeks.
Outcomes
Primary outcomes
In this study, two different applications of functional near-infrared spectroscopy (fNIRS) were employed: fNIRS of the cerebral cortex and fNIRS of the upper-limb flexors.
For fNIRS of the cerebral cortex, a Huichuang portable 35-channel fNIRS system was utilized to measure blood oxygen parameters, including HbO, HbR, and HbT. The system used the continuous wavelet transform method to calculate the wavelet amplitude (WA) of signals ranging from 0.01 to 0.08 Hz in each cortical region (Huo et al., 2019a). The phase information was extracted from the signals. The inter-hemispheric lateral degeneration index was then calculated based on WA to evaluate the degree of brain activation transfer from the affected side to the healthy side. Additionally, the extracted phase information was used for effect connection analysis based on dynamic Bayesian inference to assess the strength of the interaction between the cerebral cortex regions (Huo et al., 2019a).
For fNIRS of the upper-limb flexors, a portable muscle oxygen detector called OctaMon from Artinis Medical Systems B. V. was employed. This system utilized an 8-channel near-infrared spectroscopy setup to measure blood oxygen parameters (HbO, HbR, HbT) in the flexor muscles of the upper limb. A distribution map was created based on the measured parameters. The spatial-temporal parameters of blood oxygen were analyzed to evaluate muscle activities.
Overall, both fNIRS applications aimed to investigate blood oxygen parameters in different regions of interest (cerebral cortex and upper-limb flexors) and analyze their characteristics to understand brain activation transfer, cerebral cortex interactions, and muscle activities in the context of the study.
Secondary outcomes
The upper extremity section of the Fugl–Meyer assessment (FMA-UE): The FMA-UE has frequently been used to measure UE motor impairment (Prochazka and Kowalczewski, 2015; Qian et al., 2017) and has been reported to yield good intra-rater and inter-rater reliability and construct validity (Page et al., 2012). It is widely used to evaluate motor impairment in the upper extremity and has a Chinese version that is frequently employed in research (Wu et al., 2009).
Action Research Arm Test (ARAT): The ARAT was developed as a performance test to assess upper extremity function and dexterity after stroke (Lyle, 1981). A Chinese version of the ARAT (C-ARAT) has been translated and has been proved to have good validity, reliability (inter-rater reliability = 0.998, intra-rater reliability = 0.987), responsiveness, and predictive ability (Zhao et al., 2019c; Zhao et al., 2019a; Zhao et al., 2019b). The ARAT follows a hierarchical order, where the difficulty of items increases progressively.
MyotonPRO: Muscle tone of biceps and flexor carpi radialis of both upper limbs is evaluated using a portable MyotonPRO instrument. MyotonPRO demonstrated acceptable relative and absolute reliability in a ward setting for patients with acute stroke (Lo et al., 2017b).
Modified Ashworth scale (MAS): The MAS is an assessment tool commonly used to measure spasticity in individuals with stroke. The scale comprises six levels of spasticity severity, including grade 0, 1, 1+, 2, 3, and 4, which is in sequence scored 0, 1, 1.5, 2, 3, and 4 points in this study. This study assessed the flexors of elbow, wrist and fingers. MAS has also been shown to have excellent inter-rater reliability (ICC = 0.686-0.781) and intra-rater reliability (ICC = 0.644-0.748) in assessing spasticity in patients (Meseguer-Henarejos et al., 2018).
Modified Barthel Index (MBI): The MBI is a tool that is commonly used to assess the self-care ability of daily living, which reflects functional capacity, particularly in patients following stroke (Perez et al., 2018). In assessing patients following stroke, the MBI has shown excellent reliability (ICC = 0.88) (Yang et al., 2021).
Data management
To protect participant confidentiality, all data collected will be assigned unique codes instead of using participants’ names. Data will be recorded on the case report form. The access of all data will be restricted to the principal investigator only. To ensure accuracy in data entry, the principal investigator will meticulously review the data entries for all participants. Monitoring of study conduct and data collection will be performed by a special committee of the University Clinical Trial Unit on a biannual basis. Following the guidelines set by the journal publication policy, the raw data collected during this research study will be securely stored for a period of up to five years after the publication of the initial paper.
Statistical analysis
Before conducting parametric tests, several steps were taken to prepare and analyze the data. Outliers were identified using normal Q–Q plots and box plots, and data points that were more than three standard deviations from the mean were considered outliers and subsequently excluded from the analysis (Minati et al., 2011). Missing values were addressed using multiple imputation analysis to estimate and replace the missing data points (Blankers et al., 2010).
Descriptive statistics were used to summarize the demographic and clinical characteristics of the study participants. The normality of the data distribution was assessed using the one-sample Kolmogorov-Smirnov test. For comparisons between different groups, chi-square tests were used for categorical variables, while independent t-tests were used for continuous variables.
To evaluate the differences in outcome measures before and after the intervention for participants with stroke, paired sample t-tests were employed. The correlations between variables were assessed using either the Pearson correlation coefficient (r) or the Spearman correlation coefficient (ρ), depending on the nature of the data.
The significance of interregional coupling strength between the resting state and different grasping states was evaluated using a one-way analysis of variance (ANOVA). Additionally, χ2 tests were utilized to identify statistically significant differences in interregional coupling direction between the resting state and different grasping states.
All statistical analyses were performed using SPSS software (version 20.0; SPSS Inc., Chicago, IL, USA). All tests were two-tailed, and a significance level of p < 0.05 was considered statistically significant.
Discussion
Summary
The effectiveness and underlying mechanisms of rehabilitation therapy for improving upper limb function of stroke remain uncertain. fNIRS is a validated and reliable neuroimaging technique that can be used as a wearable device during functional activities (Plichta et al., 2006). Previous studies have utilized fNIRS to examine cortical activation and effective connectivity in stroke patients, by different interventions, such as median nerve electrical stimulation (Huo et al., 2019a), limb linkage rehabilitation tasks (Huo et al., 2019b) and musical finger movement rehabilitation gloves (Li et al., 2020). However, there is a lack of research on the neurophysiological activity of the cerebral cortex function region, as detected by fNIRS, during grasping tasks in stroke patients. Based on previous findings from EEG studies, which suggest that stroke patients have higher cognitive demands during grasping tasks compared to healthy individuals (Chen et al., 2018), it is hypothesized that the activation patterns and intensity of cerebral cortex regions in stroke patients during grasping tasks changed. Additionally, considering the weakened muscle strength and coordination disorders typically observed in the affected side of stroke patients, it is hypothesized that the hemodynamics of the upper-limb flexors undergo changes, including increased oxygen consumption, during grasping tasks after a stroke.
This study aimed to investigate the synchronous oxygenation characteristics of the central cortex and upper-limb flexors during different grasping tasks in individuals who have experienced a stroke. In addition, the study aimed to explore the hemodynamic changes in the cerebral cortex and flexor muscles of the affected upper limb during grasping tasks, brought by the combination of VR and conventional rehabilitation therapy, to try to investigate the rehabilitation mechanisms of upper limb motor function in individuals with stroke.
Limitations
There were several important limitations in this study that warrant consideration. Firstly, the trial sample size was relatively small. A small sample size can impact the statistical power of the study and may limit the ability to draw robust and generalizable conclusions from the findings. Secondly, the study design employed a non-randomized clinical trial approach, and participants were recruited using convenience sampling from the inpatient population with stroke. This could limit the generalizability of the findings. Thirdly, the study primarily focused on the assessment of hemodynamic changes during grasping motor tasks. Consequently, the understanding of the intervention’s effects on overall motor function may be limited by this narrow focus on specific upper limb tasks. Lastly, the two functional near infrared spectroscopy detection systems was at different sampling rate during the measurements.
Strengths and relevance
This study has several strengths. Firstly, it aims to investigate the synchronous oxygenation characteristics of both the central cortex and upper-limb flexors during a grasping task in individuals with stroke. The findings from this research have the potential to significantly contribute to our understanding of motor function and recovery in individuals with stroke. It may uncover valuable insights into the underlying mechanisms of motor control and rehabilitation. Secondly, the utilization of two functional near infrared spectroscopy detection systems enables simultaneous monitoring of blood oxygen parameters in both regions, providing a comprehensive assessment of oxygenation dynamics. Thirdly, the study employs a combination of quantitative and qualitative approaches to assess the outcomes. This comprehensive evaluation enhances the interpretation of the findings, capturing both the quantitative metrics and the qualitative aspects of synchronous oxygenation characteristics.
Ethics statement
The studies involving humans were approved by the Medical Ethical committee of the First Affiliated Hospital of Sun Yat-sen University in China. The studies were conducted in accordance with the local legislation and institutional requirements. The participants provided their written informed consent to participate in this study.
Author contributions
J-LZ: Writing−review and editing, Writing−original draft, Supervision, Resources, Methodology, Investigation, Funding acquisition, Formal analysis, Data curation, Conceptualization. P-MC: Methodology, Formal analysis, Writing−review and editing. TZ: Data curation, Writing−review and editing. HX: Formal analysis, Data curation, Writing−review and editing. W-WX: Data curation, Writing−review and editing. SN: Writing−review and editing. C-HW: Writing−review and editing, Funding acquisition.
Funding
The author(s) declare financial support was received for the research, authorship, and/or publication of the article. This work was funded by the National Natural Science Foundation of China (No.82102649), and the Medical Scientific Research Foundation of Guangdong Province (No. A2020426).
Acknowledgments
We would like to acknowledge the individuals for their participating in the study. We also express our gratitude to all the colleagues of the Department of Rehabilitation Medicine of the First Affiliated Hospital, Sun Yat-sen University, China.
Conflict of interest
The authors declare that the research was conducted in the absence of any commercial or financial relationships that could be construed as a potential conflict of interest.
Publisher’s note
All claims expressed in this article are solely those of the authors and do not necessarily represent those of their affiliated organizations, or those of the publisher, the editors and the reviewers. Any product that may be evaluated in this article, or claim that may be made by its manufacturer, is not guaranteed or endorsed by the publisher.
References
Arnemann, K., Chen, A., Novakovic-Agopian, T., Gratton, C., Nomura, E., and D’Esposito, M. (2015). Functional brain network modularity predicts response to cognitive training after brain injury. Neurology 84, 1568–1574. doi: 10.1212/WNL.0000000000001476
Beever, A., Tripp, T., Zhang, J., and MacInnis, M. (2020). Nirs-derived skeletal muscle oxidative capacity is correlated with aerobic fitness and independent of sex. J. Appl. Physiol. 129, 558–568. doi: 10.1152/japplphysiol.00017.2020
Blankers, M., Koeter, M., and Schippers, G. (2010). Missing data approaches in ehealth research: Simulation study and a tutorial for nonmathematically inclined researchers. J. Med. Internet Res. 12:e54. doi: 10.2196/jmir.1448
Chen, L., Mao, Y., Ding, M., Li, L., Leng, Y., Zhao, J., et al. (2018). Assessing the relationship between motor anticipation and cortical excitability in subacute stroke patients with movement-related potentials. Front. Neurol. 9:881. doi: 10.3389/fneur.2018.00881
Chung, M., Martins, B., Privratsky, A., James, G., Kilts, C., and Bush, K. (2018). Individual differences in rate of acquiring stable neural representations of tasks in fmri. PLoS One 13:e207352. doi: 10.1371/journal.pone.0207352
GBD Stroke Collaborators (2019). Global, regional, and national burden of stroke, 1990-2016: A systematic analysis for the global burden of disease study 2016. Lancet Neurol. 18, 439–458. doi: 10.1016/S1474-4422(19)30034-1
Giovanelli, N., Biasutti, L., Salvadego, D., Alemayehu, H., Grassi, B., and Lazzer, S. (2020). Changes in skeletal muscle oxidative capacity after a trail running race. Int. J. Sports Physiol. Perform. 15, 278–284. doi: 10.1123/ijspp.2018-0882
Grattan, E., Velozo, C., Skidmore, E., Page, S., and Woodbury, M. (2019). Interpreting action research arm test assessment scores to plan treatment. OTJR (Thorofare N J). 39, 64–73. doi: 10.1177/1539449218757740
Greeley, B., Hanada, G., Boyd, L., and Peters, S. (2021). The time for translation of mobile brain and body imaging to people with stroke is now. Phys. Ther. 101:zab058. doi: 10.1093/ptj/pzab058
Huo, C., Li, X., Jing, J., Ma, Y., Li, W., Wang, Y., et al. (2019a). Median nerve electrical stimulation-induced changes in effective connectivity in patients with stroke as assessed with functional near-infrared spectroscopy. Neurorehabil. Neural Repair. 33, 1008–1017. doi: 10.1177/1545968319875952
Huo, C., Xu, G., Li, Z., Lv, Z., Liu, Q., Li, W., et al. (2019b). Limb linkage rehabilitation training-related changes in cortical activation and effective connectivity after stroke: A functional near-infrared spectroscopy study. Sci. Rep. 9:6226. doi: 10.1038/s41598-019-42674-0
Huppert, T., Barker, J., Schmidt, B., Walls, S., and Ghuman, A. (2017). Comparison of group-level, source localized activity for simultaneous functional near-infrared spectroscopy-magnetoencephalography and simultaneous fnirs-fmri during parametric median nerve stimulation. Neurophotonics 4:15001. doi: 10.1117/1.NPh.4.1.015001
Kasahara, M., Menon, D., Salmond, C., Outtrim, J., Tavares, J., Carpenter, T., et al. (2011). Traumatic brain injury alters the functional brain network mediating working memory. Brain Inj. 25, 1170–1187. doi: 10.3109/02699052.2011.608210
Kawabata, D., Tokuda, T., Sato, C., Tagai, K., and Dan, I. (2019). Willingness-to-pay-associated right prefrontal activation during a single, real use of cosmetics as revealed by functional near-infrared spectroscopy. Front. Hum. Neurosci. 13:16. doi: 10.3389/fnhum.2019.00016
Laver, K., Lange, B., George, S., Deutsch, J., Saposnik, G., and Crotty, M. (2017). Virtual reality for stroke rehabilitation. Cochrane Database Syst. Rev. 11:CD8349. doi: 10.1002/14651858.CD008349.pub4
Levin, M., Weiss, P., and Keshner, E. (2015). Emergence of virtual reality as a tool for upper limb rehabilitation: Incorporation of motor control and motor learning principles. Phys. Ther. 95, 415–425. doi: 10.2522/ptj.20130579
Li, L. (2020). Support programme for secondary prevention of stroke: Inspiration to continue? Lancet Neurol. 19, 24–25. doi: 10.1016/S1474-4422(19)30410-7
Li, Q., Feng, J., Guo, J., Wang, Z., Li, P., Liu, H., et al. (2020). Effects of the multisensory rehabilitation product for home-based hand training after stroke on cortical activation by using nirs methods. Neurosci. Lett. 717:134682. doi: 10.1016/j.neulet.2019.134682
Lin, Q., Li, H., Mao, Y., Lo, W., Zhao, J., Chen, L., et al. (2017). The difference of neural networks between bimanual antiphase and in-phase upper limb movements: A preliminary functional magnetic resonance imaging study. Behav. Neurol. 2017:8041962. doi: 10.1155/2017/8041962
Lo, W., Mao, Y., Li, L., Lin, A., Zhao, J., Chen, L., et al. (2017a). Prospective clinical study of rehabilitation interventions with multisensory interactive training in patients with cerebral infarction: Study protocol for a randomised controlled trial. Trials 18:173. doi: 10.1186/s13063-017-1874-y
Lo, W., Zhao, J., Li, L., Mao, Y., and Huang, D. (2017b). Relative and absolute interrater reliabilities of a hand-held myotonometer to quantify mechanical muscle properties in patients with acute stroke in an inpatient ward. Biomed. Res. Int. 2017:4294028. doi: 10.1155/2017/4294028
Lohse, K., Hilderman, C., Cheung, K., Tatla, S., and Van der Loos, H. (2014). Virtual reality therapy for adults post-stroke: A systematic review and meta-analysis exploring virtual environments and commercial games in therapy. PLoS One 9:e93318. doi: 10.1371/journal.pone.0093318
Lyle, R. C. (1981). A performance test for assessment of upper limb function in physical rehabilitation treatment and research. Int. J. Rehabil. Res. 4, 483–492. doi: 10.1097/00004356-198112000-00001
Meseguer-Henarejos, A., Sanchez-Meca, J., Lopez-Pina, J., and Carles-Hernandez, R. (2018). Inter- and intra-rater reliability of the modified ashworth scale: A systematic review and meta-analysis. Eur. J. Phys. Rehabil. Med. 54, 576–590. doi: 10.23736/S1973-9087.17.04796-7
Minati, L., Kress, I., Visani, E., Medford, N., and Critchley, H. (2011). Intra- and extra-cranial effects of transient blood pressure changes on brain near-infrared spectroscopy (nirs) measurements. J. Neurosci. Methods 197, 283–288. doi: 10.1016/j.jneumeth.2011.02.029
Page, S., Levine, P., and Hade, E. (2012). Psychometric properties and administration of the wrist/hand subscales of the fugl-meyer assessment in minimally impaired upper extremity hemiparesis in stroke. Arch. Phys. Med. Rehabil. 93, 2373–2376. doi: 10.1016/j.apmr.2012.06.017
Perez, M., Flores, A., Castro, S., Tapia, H., Garcia, R., and Aguilar, F. (2018). Inpatient dependency in activities of daily living predicts informal caregiver strain: A cross-sectional study. J. Clin. Nurs. 27, e177–e185. doi: 10.1111/jocn.13900
Perrey, S., and Ferrari, M. (2018). Muscle oximetry in sports science: A systematic review. Sports Med. 48, 597–616. doi: 10.1007/s40279-017-0820-1
Plichta, M., Herrmann, M., Baehne, C., Ehlis, A., Richter, M., Pauli, P., et al. (2006). Event-related functional near-infrared spectroscopy (fnirs): Are the measurements reliable? Neuroimage 31, 116–124. doi: 10.1016/j.neuroimage.2005.12.008
Prochazka, A., and Kowalczewski, J. A. (2015). fully automated, quantitative test of upper limb function. J. Mot. Behav. 47, 19–28. doi: 10.1080/00222895.2014.953442
Qian, Q., Hu, X., Lai, Q., Ng, S., Zheng, Y., and Poon, W. (2017). Early stroke rehabilitation of the upper limb assisted with an electromyography-driven neuromuscular electrical stimulation-robotic arm. Front. Neurol. 8:447. doi: 10.3389/fneur.2017.00447
Saposnik, G., Cohen, L., Mamdani, M., Pooyania, S., Ploughman, M., Cheung, D., et al. (2016). Efficacy and safety of non-immersive virtual reality exercising in stroke rehabilitation (evrest): A randomised, multicentre, single-blind, controlled trial. Lancet Neurol. 15, 1019–1027. doi: 10.1016/S1474-4422(16)30121-1
Scholkmann, F., Kleiser, S., Metz, A., Zimmermann, R., Mata, P., Wolf, U., et al. (2014). A review on continuous wave functional near-infrared spectroscopy and imaging instrumentation and methodology. Neuroimage 85, 6–27. doi: 10.1016/j.neuroimage.2013.05.004
Stinear, C., Lang, C., Zeiler, S., and Byblow, W. (2020). Advances and challenges in stroke rehabilitation. Lancet Neurol. 19, 348–360. doi: 10.1016/S1474-4422(19)30415-6
Uga, M., Dan, I., Sano, T., Dan, H., and Watanabe, E. (2014). Optimizing the general linear model for functional near-infrared spectroscopy: An adaptive hemodynamic response function approach. Neurophotonics 1:15004. doi: 10.1117/1.NPh.1.1.015004
Ward, N. (2004). Functional reorganization of the cerebral motor system after stroke. Curr. Opin. Neurol. 17, 725–730. doi: 10.1097/00019052-200412000-00013
Werkman, M., Jeneson, J., Helders, P., Arets, B., van der Ent, K., Velthuis, B., et al. (2016). Exercise oxidative skeletal muscle metabolism in adolescents with cystic fibrosis. Exp. Physiol. 101, 421–431. doi: 10.1113/EP085425
Wu, Y., Min, Y., and Yan, T. (2009). Validity and reliability of wolf motor function test on assessing the upper extremities motor function of Chinese stroke patients in acute stage. Chin. J. Rehabil. Med. 24, 992–994. doi: 10.3969/j.issn.1001-1242.2009.11.011
Yang, H., Chen, Y., Wang, J., Wei, H., Chen, Y., and Jin, J. (2021). Activities of daily living measurement after ischemic stroke: Rasch analysis of the modified barthel index. Medicine (Baltimore) 100:e24926. doi: 10.1097/MD.0000000000024926
Zhao, J., Zhang, T., Xu, Z., Ding, M., Leng, Y., Bian, R., et al. (2019c). Responsiveness and predictive ability of the Chinese version of the action research arm test in people with cerebral infarction. Biomed. Res. Int. 2019:8270187. doi: 10.1155/2019/8270187
Zhao, J., Chen, P., Li, W., Bian, R., Ding, M., Li, H., et al. (2019a). Translation and initial validation of the Chinese version of the action research arm test in people with stroke. Biomed. Res. Int. 2019:5416560. doi: 10.1155/2019/5416560
Keywords: rehabilitation, stroke, motor function, functional near-infrared spectroscopy, oxygenation, virtual reality training
Citation: Zhao J-L, Chen P-M, Zhang T, Xie H, Xiao W-W, Ng SSM and Wang C-H (2024) Characteristics of central cortex and upper-limb flexors synchrony oxygenation during grasping in people with stroke: a controlled trial study protocol. Front. Hum. Neurosci. 18:1409148. doi: 10.3389/fnhum.2024.1409148
Received: 06 May 2024; Accepted: 09 August 2024;
Published: 29 August 2024.
Edited by:
Filippo Brighina, University of Palermo, ItalyReviewed by:
Shugeng Chen, Fudan University, ChinaChao-Jin-Zi Li, Capital Medical University, China
Copyright © 2024 Zhao, Chen, Zhang, Xie, Xiao, Ng and Wang. This is an open-access article distributed under the terms of the Creative Commons Attribution License (CC BY). The use, distribution or reproduction in other forums is permitted, provided the original author(s) and the copyright owner(s) are credited and that the original publication in this journal is cited, in accordance with accepted academic practice. No use, distribution or reproduction is permitted which does not comply with these terms.
*Correspondence: Jiang-Li Zhao, emhhb2psNkBtYWlsLnN5c3UuZWR1LmNu; Chu-Huai Wang, d2FuZ2NodWhAbWFpbC5zeXN1LmVkdS5jbg==