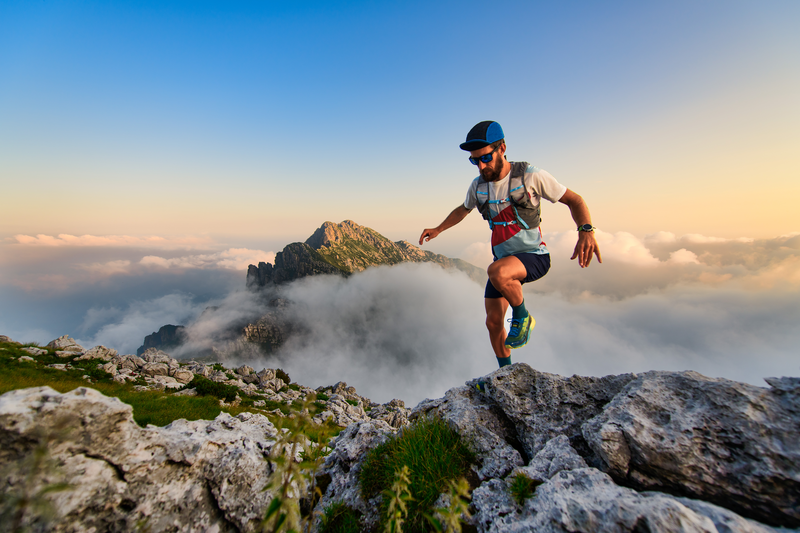
95% of researchers rate our articles as excellent or good
Learn more about the work of our research integrity team to safeguard the quality of each article we publish.
Find out more
ORIGINAL RESEARCH article
Front. Hortic. , 21 August 2024
Sec. Sustainable Pest and Disease Management
Volume 3 - 2024 | https://doi.org/10.3389/fhort.2024.1447425
Four Plant Growth-Promoting Rhizobacteria (PGPR) consortia were assembled based on their plant growth-promoting (PGP) traits and biocontrol properties, previously characterized in vitro, and tested to protect tomato plants against Alternaria spp. infections. Results indicated that PGPR consortia significantly reduced Alternaria disease severity on tomato plants. Consortia C2 and C3 were particularly effective, demonstrating disease control rates similar to or exceeding those achieved with full-dose chemical fungicides. Furthermore, PGPR treatments markedly decreased fungal colonization in both leaves and fruits, with the best performing consortia reducing fungal presence by up to 98% shortly after inoculation in the short term. Only tenuazonic acid (TeA) was detected among the tested mycotoxins, and its levels were significantly lower in fruits from PGPR-treated plants. The combination of PGPR consortia with a reduced dose of fungicide also showed promising results, indicating a synergistic effect in controlling both fungal spread and mycotoxin production in the long run. Findings support the potential of PGPR as sustainable alternative to chemical fungicides, enhancing plant health while reducing environmental impact, aligning with current agricultural policies.
Tomato (Solanum lycopersicum L.) is one of the most cultivated vegetable crops worldwide (Khalil et al., 2021). The main producers are the United States, followed by Italy and China, which together cover 56% of the total production. Italy is the leading world exporter of pulps and peeled tomatoes, with a share of 76% of the world value of production (2022 data) (World Processing Tomato Council, 2022). Moreover, tomato is one of the most consumed agri-food products in Italy, often necessary for the preparation of different typical dishes. For all these reasons, any danger or loss regarding tomato cultivations represents an important issue for the Italian economy. Currently, several diseases can affect tomato plants during the growing season and fungi can determine great crop losses. One of the most devastating fungi able to infect tomato plants is Alternaria spp., with the most frequently reported species being A. solani, A. alternata and A. tenuissima (Garganese et al., 2019; Sanzani et al., 2019). Tomato plants can be colonized by Alternaria species throughout the growth cycle, with damage to leaves, stems and fruits. In particular, environmental conditions favorable for Alternaria spp. infections at the time of fruit ripening can cause severe yield losses (Nash and Gardner, 1988) ranging from 35 up to 78% (Parvin et al., 2021). In addition to crop losses, Alternaria species pose a serious toxicological risk, due to the production of a wide range of mycotoxins, including alternariol (AOH), alternariol methyl ether (AME), altenuene (ALT), tenuazonic acid (TeA) and tentoxin (TEN), which are associated with various toxic activities on human health (Habib et al., 2021).
Even though the evaluation of their toxicological capabilities is essential for a valid risk assessment, this is still ongoing by the European Union (EU), data on the incidence and the quantity of Alternaria mycotoxins found in food products are necessary to evaluate human dietary exposure. The European Food Safety Authority (EFSA) performed a risk assessment for four known Alternaria mycotoxins (AOH, AME, TeA and TEN) and established the threshold for toxicological concern (TTC) (Solfrizzo, 2017). Indeed, in 2022, the European Union issued Recommendation (EU) 2022/553, supplementing the Regulation (EU) 1881/2006, setting limits for Alternaria toxins in foodstuff, including products derived from processed tomatoes (EU Commission, 2022).
Therefore, it is of primary importance to adopt strategies for containing Alternaria spp. and their related mycotoxins in tomato cultivation, especially in a scenario of climate change where mycotoxigenic fungi could find suitable environmental conditions for easier growth.
Preventive methods, such as good agricultural practices and the use of appropriate chemical products, are considered the best choices to reduce mycotoxin contamination (Giorni et al., 2023). Chemical Cu-based fungicides have always been used to contain economic losses due to the presence of fungal species on tomato. However, recent policies advocate for alternative solutions that could be more in accordance with an environment friendly approach (Parvin et al., 2021). Following the European Green Deal policies, many studies were indeed conducted on the possible use of Plant Growth-Promoting Rhizobacteria (PGPRs) as biocontrol agents for sustainable and biological management of tomato’s diseases and better fruit quality (Boukhatem et al., 2022; El-Saadony et al., 2022; Bellotti et al., 2023; Timmusk et al., 2023).
PGPRs are mainly known for their ability to enhance plant growth and development thanks to their plant growth-promoting (PGP) traits, such as siderophores and indole acetic acid (IAA) production, 1-aminocyclopropane-1-carboxylic acid (ACC) deaminase activity, inorganic phosphate solubilization, and nitrogen fixation. Additionally, many studies underline that some PGPRs can directly inhibit plant pathogens by producing antimicrobial compounds or outcompeting pathogens for space and nutrients (Lee et al., 2023). Some PGPRs were also found to protect plants against fungal diseases through an indirect mechanism known as Induced Systemic Resistance (ISR) (Pieterse et al., 2014). Since PGPRs can exhibit both biostimulant and biocontrol activity, they represent a promising alternative for sustainable crop management.
This study aimed to evaluate, through in planta pot trials, the antifungal potential of four different PGPR consortia. The rhizobacteria involved in this research belong to a larger set of isolates that were previously selected and screened in Bellotti et al. (2023) using in vitro tests for the characterization of PGP traits and biocontrol properties against different mycotoxigenic Alternaria species (A. tenuissima, A. solani and A. alternata).
Four rhizobacterial consortia were assembled on the base of results obtained with the in vitro tests reported by Bellotti et al. (2023). Briefly, a total of 106 bacteria were collected from the rhizosphere of Solanum lycopersicum L., comprising 85 rhizosphere bacteria and 21 endophytic bacteria, and screened for their antagonism versus the main Alternaria species associated with tomato cultivation in Italy (A. alternata, A. solani and A. tenuissima). The PGP traits, including indole acetic acid (IAA), siderophores production, nitrogen fixation, and phosphate solubilization, were assessed, as well as the bacteria strains’ ability to reduce Alternaria growth and mycotoxin production in dual culture assays. As reported in Bellotti et al. (2023), following a ranking system to highlight strains with the best antifungal properties and various PGP characteristics, 10 bacterial isolates were selected for this study, and 4 consortia were built and tested as plant inoculants on tomatoes (Table 1). Combinations were chosen so that each consortium contained individuals active versus each of the Alternaria species used for the pot trials, either for fungal biomass control or Alternaria toxins reduction.
To assemble the four consortia, each strain was individually prepared by inoculation in 10 ml tubes containing 5 ml Tryptic Soy Broth and incubation at 30°C in shaking conditions for 48h. Tubes were then centrifuged at 3500 rpm for 10 mins and washed three times with sterile saline solution to remove medium traces and metabolites. The washed pellet was finally resuspended with 10% sucrose solution up to the equal cell density adjusted using Mc Farland 1.0 standard. Then an equal volume of each strain was added to a 50 ml falcon tube, up to the final volume of 10 ml total for each consortium. Five grams of seeds were added in each falcon tube and seeds were soaked in the liquid bacterial culture suspensions for 1.5 h at 30°C. Then the bacterial suspensions with seeds were spread separately onto petri dish to allow water to evaporate. The presence of sucrose on the seed surface, once dried, provides a sticky matrix that helps the bacteria to adhere more effectively to the seed surface and potentially increases its survival rate (Deaker et al., 2004). Finally, 1g of seeds (approximately 100 seeds) were used to quantify the level of colonization (CFU/seed) before sowing. Control seeds were treated using a 10% sucrose solution.
Treated and untreated dry tomato seeds were placed in a polystyrene container (280 inverted pyramid cells) filled with a standard organic peat-based germination mix. Seedlings were grown under typical greenhouse growing conditions (temperatures of 18°C at night and 24°C during the day, 80% relative humidity) for a total of 40 days, until the phenological stage of second/third pair of true leaves was visible (BBCH 13) (Cardoso et al., 2021).
Tomato plants at BBCH 13 stage were transplanted in pots of about 40 cm in diameter filled with a commercial topsoil substrate having the following composition: medium peat texture enriched with 1500 g/m³ NPK plus 2000 g/m³ organic nitrogen fertilizer plus microelements; pH 6.0; electrical conductivity 0.25 dS/m; bulk density 280 kg/m3. Pots were placed in an outside area following a randomized block pattern (4 replicates). Plants were then grown for 93 days starting from May 31st with a photoperiod ranging from approximately 15 hours of natural daylight at transplanting date to 13 hours at harvesting date (September 1st), and with day/night temperatures that spanned from 21°C to 34°C. Drip irrigation was applied to prevent water stress by plants. For the transplantation, an organic Cu-based pesticide was used.
For the pot experiment 11 different plant treatments were considered (Table 2). The untreated plants consisted of plants developed from seeds exposed to 10% sucrose. All the other plants were developed from seeds treated with a bacterial consortium, with and without the use of copper-based fungicide applied at the plant developing stage BBCH 69 (50% dose reported on the label).
Table 2. Description of the treatments considered in the study on tomato plants transplanted in pots and conducted during the growing season in year 2021.
Moreover, two other treatments considering plants treated only with the Cu-fungicide at full-dose (100% dose reported in label) and at a reduced dose (50% dose reported in label) were also prepared.
Four plants were used for each treatment (biological replicates) for a total of 44 plants used for the trial.
One strain of A. alternata (CBS 118814), one strain of A. solani (CBS 109157) and one strain of A. tenuissima (CBS 117.44), obtained from the Westerdijk Fungal Biodiversity Institute (Utrecht, the Netherlands) were used to prepare the fungal inoculum. The same strains were previously tested in the in vitro experiment and resulted able to produce different Alternaria toxins such as tenuazonic acid (TeA), alternariol (AOH), alternariol monomethyl ether (AME) and tentoxin (Bellotti et al., 2023). The fungal strains were singularly inoculated on Petri dishes (Ø 9 cm) with Potato Dextrose Agar (PDA, Biolife, Milano, Italy) and incubated at 25°C for 7 days (12 h light/12 h dark photoperiod). After incubation, developed fungal colonies were washed with sterile water and spores were collected and adjusted to a concentration of 104 CFU/ml by mixing together the three Alternaria strains. The inoculation on plants with the spore mix was carried out in two phases: at the development phase (BBCH 51) and at the flowering/fruit set phase (BBCH 65). In both stages, 10 mL of the inoculum was sprayed directly on the leaves on each plant.
One week after the first artificial treatment with Alternaria spp., visual checks were carried out by assessing the incidence of disease on plants in percentages ranging from 0% to 100%, based on the scale published by Bessadat et al. (2016). The visual checks took place weekly for the entire growing season, were conducted by the same operators to reduce variability in observations, and were based on the assessment of the disease symptoms on the plant area. Samples of leaves were harvested 7 days after the second fungal infection and at harvest time when also berries were collected. Fruits harvested from each plant were weighed to check differences in production. Both berries and leaves were kept at 4°C until biological analysis.
Colonization by fungi was determined by diluting 1 g of leaves for each treatment and replicate in 9 ml of sterilized water-peptone (1%) solution. Following the Colony Forming Units procedure, dilutions from 102 to 107 were prepared, and 1 ml of each was distributed in plates with Dichloran Rose Bengal Chloramphenicol (DRBC, Oxoid, UK) conducting the analysis with 3 replicates.
After 7 days of incubation at 25°C in darkness, developed fungal colonies were counted and reported as CFU/g of leaves. In the same way, tomato fruits collected at harvest time were washed, dried with sterile paper and then smashed. Pulp obtained was analyzed following the same procedure of leaves but starting with 1 mL of sample. After incubation, developed fungal colonies were counted and results reported as CFU/ml of tomato pulp.
Tomato berries collected at harvest time were kept at 4°C until chemical analysis. Alternaria toxins were extracted according to the method of Bertuzzi et al. (2021). Briefly, Alternaria toxins were extracted using acetonitrile:water 80 + 20 v/v for 60 min using a rotary shaker; after filtration, 1 ml was evaporated under N2, redissolved in acetonitrile:water 1 + 9 v/v and injected in a HPLC-MS/MS system (Thermofisher). Alternaria toxins were chromatographed on a HSS-T3 RP-18 column (5 µm particle size, 150 x 2.1 mm, Waters) using a mobile-phase gradient acetonitrile-water (both acidified with 0.2% formic acid) from 30:70 to 65:35 in 6 min, then isocratic for 3 min; gradient to 30:70 in 1 min and isocratic for 6 min (equilibration-step). The ionization was carried out with an ESI interface (Thermo-Fisher) in positive mode as follows: spray capillary voltage 4.5 kV, sheath and auxiliary gas 35 and 14 psi, respectively, temperature of the heated capillary 270°C. The LOD and the LOQ were 0.5 µg/kg and 1.5 µg/kg for AOH, AME and TEN, 5 and 15 µg/kg for TeA.
We used generalized linear models (GLMs) to assess the effects of different kind of treatments (biological and chemical approaches considered) (factors) on visible Alternaria symptoms on tomato plants and Alternaria fungal colonization (CFU/g) on plants and fruits at different sampling times (7 days after artificial inoculation and at harvest time) (response variables). Response and explanatory variables are listed in Table 3.
Table 3. The response variables and the factors used in the present study for generalized linear model (GLM).
Analysis of variance (ANOVA) was calculated using the generalized linear model (GLM) procedure of the statistical package IBM SPSS Statistics 27 (IBM Corp., Armonk, NY, USA) while significant differences were highlighted using the Tukey test (p ≤ 0.05) for mean separation.
Data on Alternaria toxins (values+1) were logarithmically transformed before statistical analysis (Clewer and Scarisbrick, 2001).
The visual assessment of Alternaria symptoms on tomato plants showed a significant increase along the growing season (Table 4) (Supplementary Table 1). At 7 days after the artificial inoculation with Alternaria species (A. alternata, A. solani and A. tenuissima) significant differences in symptoms presence were found among the different treatments (p ≤ 0.05) (Supplementary Table 2). In particular, the lowest presence of the disease was shown by C1 + 50% and C3 (p ≤ 0.05) (Figure 1) with a visual assessment of 30% and 35%, respectively. The worst performance was obtained by plants treated with the reduced dose of fungicide (50%), which presented a Alternaria visual disease assessment of 58% (p ≤ 0.05) (Figure 1).
Table 4. Analysis of variance (ANOVA) of Alternaria disease symptoms on tomato leaves after artificial inoculation with Alternaria strains (A. alternata, A. solani and A. tenuissima) assessed following the disease severity scale reported by Bessadat et al. (2016) at 7 days and at harvest time in tomato plants treated with different biological and chemical approaches.
Figure 1. Box plots of Alternaria disease severity on tomato plants after 7 days from the artificial inoculation with A. alternata, A. tenuissima and A. solani following the scale suggested by Bessadat et al. (2016). Within each box, horizontal black lines denote median values; boxes extend from the 25th to the 75th percentile of each group’s distribution of values; vertical extending lines denote the most extreme values. Different letters mean significant differences according to Tukey’s test (p ≤ 0.05).
The same assessment on tomato plants at the end of the experiment (full-ripening stage) did not present significant differences among treatments (Supplementary Table 3). However, tomato plants treated with C2 and C3 appeared comparable to plants treated with the full dose of chemical Cu-fungicide (Figure 2) (n.s.). For these plants, the visual presence of the disease was around 83% while for all other treatments, the disease resulted higher in C4, C3 + 50% and C4 + 50%, which visually presented damages higher than 90% (Figure 2).
Figure 2. Box plots of Alternaria disease severity on tomato plants artificially inoculated with A. alternata, A. tenuissima e A. solani at the end of the growing season (harvest time) following the scale suggested by Bessadat et al., 2016. Within each box, horizontal black lines denote median values; boxes extend from the 25th to the 75th percentile of each group’s distribution of values; vertical extending lines denote the most extreme values. Means separation resulted statistically not significant (ns) according to Tukey’s test.
Morphological characteristics analysis (spores shape and length), observed with an optical microscope on randomly selected fungal colonies confirmed they belonged to the Genus Alternaria. At least ten fungal strains isolated for each plant were observed. Fungal presence on tomato leaves was found both after 7 days from the artificial inoculation (Figure 3) and at harvest time (Figure 4) (Supplementary Table 4), with the highest fungal colonization at the first sampling time (Table 5). After 7 days from the artificial fungal inoculation, untreated plants, as expected, showed the highest fungal colonization while all the plants treated with bacterial consortia or Cu-fungicide showed a significant reduction in the presence of fungal species (p ≤ 0.01) (Supplementary Table 5). Interestingly, the best results in Alternaria containment were obtained when the consortia C2 (-98%) and C3 (-96%) were applied, while the full dose of Cu-fungicide showed a reduction of -95% after 7 days from the inoculation. Similarly, all bacterial consortia applied with 50% dose of the Cu- fungicide showed a reduction of fungal presence ranging from -85% to -89%, thus resulting less efficient than the same consortia used without the Cu-fungicide (Figure 3).
Figure 3. Box plots of fungal colonization of tomato plants leaves (CFU/g) due to Alternaria species counted 7 days after the second artificial inoculation with fungal strains A. alternata, A. tenuissima e A. solani. Within each box, horizontal black lines denote median values; boxes extend from the 25th to the 75th percentile of each group’s distribution of values; vertical extending lines denote the most extreme values. Different letters indicate significant differences according to Tukey’s test (p ≤ 0.01).
Figure 4. Box plots of fungal colonization of tomato plants leaves (CFU/g) due to Alternaria species at harvest time. Within each box, horizontal black lines denote median values; boxes extend from the 25th to the 75th percentile of each group’s distribution of values; vertical extending lines denote the most extreme values. Different letters indicate significant differences according to Tukey’s test (p ≤ 0.05).
Table 5. Analysis of variance (ANOVA) of fungal colonies (CFU/g) obtained from tomato leaves after artificial inoculation with Alternaria strains (A. alternata, A. solani and A. tenuissima) collected after 7 days and at harvest time in tomato plants treated with different biological and chemical approaches.
At harvest time, the level of Alternaria spp. colonization of tomato plants generally resulted lower compared to 7 days after the inoculation. However, C4 treatments showed the lowest performance with a fungal concentration significantly higher than the untreated samples (p ≤ 0.05) (Figure 4; Supplementary Table 6). The plants treated with C3 + 50% showed the lowest fungal presence (64%) compared to the untreated plants (p ≤ 0.05) (Figure 4; Supplementary Table 6).
When considering tomato berries, results on fungal contamination differed from those in leaves (Supplementary Table 7). In particular, consortium C1 was the most effective in reducing the presence of fungal species on fruits both alone and in combination with 50% of Cu-fungicide, without significant differences between the two treatments. Consortium C3 was also effective in reducing fungal colonization although the addition of a 50% dose of Cu-fungicide seemed to reduce its efficacy (Figure 5).
Figure 5. Box plots of fungal colonization of tomato berries (CFU/g) due to Alternaria species at harvest time and full ripening. Within each box, horizontal black lines denote median values; boxes extend from the 25th to the 75th percentile of each group’s distribution of values; vertical extending lines denote the most extreme values. Different letters indicate significant differences according to Tukey’s test (p ≤ 0.05).
Overall, the best performing bacterial consortia for containing Alternaria development on tomato fruits were C1 (-83%) and C3 (-70%), with C1 achieving similar results also in combination with 50% dose of Cu-fungicide (-68%) (Figure 5).
Among the Alternaria toxins, only TeA was detected, while AOH, AME and TEN were never found on berries. Comparing the results between bacterial, Cu-pesticide, and Cu- pesticide + bacteria, all were able to reduce TeA to different extents compared to untreated plants, with the only exceptions being C2 + 50% and C3 + 50%, which had TeA content similar to untreated tomatoes (Figure 6; Supplementary Table 8). Overall, the TeA average reduction varied from 9% to 98%, with C1 + 50% being the most effective in reducing TeA content in tomato berries (Figure 6). Berries treated with C2 and with C4 + 50% also showed good results in TeA reduction (-82% and -94%, respectively), compared to the full dose Cu-fungicide treatment (-88%) (p ≤ 0.05) (Figure 6; Supplementary Table 8).
Figure 6. Box plots of tenuazonic acid (TeA) content in tomato berries (CFU/g) collected at full ripening. Within each box, horizontal black lines denote median values; boxes extend from the 25th to the 75th percentile of each group’s distribution of values; vertical extending lines denote the most extreme values. Different letters indicate significant differences according to Tukey’s test (p ≤ 0.05).
Alternaria is a widespread ubiquitous pathogen able to survive in soil and in crop debris and is preferably adapted to humid and temperate climates. In general, Alternaria can be considered a foliar pathogen capable of causing a slow devastation of host tissues through the reduction of photosynthetic potential (Thomma, 2003). In conducive year, losses caused by Alternaria spp. can range from 35 to 80% (Bessadat et al., 2016). Tomato plants presenting this fungal disease are characterized by the appearance of chlorotic and necrotic symptoms on all the aboveground parts of the plant (Meena et al., 2016).
In our trial, the visual assessment of Alternaria symptoms on leaves and stems showed, as expected, a significant increment along the growing season. Our plants were treated with PGPR only once during the season by inoculating the seeds with bacterial consortia, while the Cu-fungicide was sprayed on leaves. Normally, treatments in field against Alternaria can be repeated in case of conducive weather conditions or when symptoms appear in cultivations. In other tomato field trials, to assess the efficacy of chemical products against Alternaria spp., including Cu-based products, treatments were repeated weekly (Egel et al., 2019; Trebbi et al., 2021). Cu-based products, like the one used in this trial, have a long history in the management of plant diseases and have recently become a standard for disease management in organic agriculture. However, alternative disease control approaches are needed as repeated applications of products containing copper. This can negatively affect soil and water quality and some pathogens have evolved resistance to copper (Egel et al., 2019). Moreover, excessive applications can be phytotoxic to plants and harmful to other micro- and macro-organisms (Dias, 2012).
When plants were treated with a 50% dose of Cu-fungicide alone, plant defense had the worst performance. This is not surprising since chemical fungicides show the best performance at recommended label doses. In fact, the higher the dose, the greater the reduction in fitness of the sensitive Alternaria strains follows. Of course, the fitness of the resistant strains does not change or changes to a lesser extent compared to the resistant strain, increasing the fitness difference and thus increasing selection (van der Bosch et al., 2014a).
The differences between the short-term (7 days from the artificial inoculation) and the long-term data (harvest time, full ripening stage) were probably due to a limited efficacy of the bacterial consortia and Cu-based products over time. In the case of high fungal presence, as in our trial where Alternaria spores were inoculated at a very high concentration, it would be necessary to repeat the treatments along the growing season to improve the treatment’s efficacy.
From a resistance management perspective, a larger number of applications and/or a higher dose are expected to increase the rate of selection for fungicide/BCA resistance (van den Bosch et al., 2011, 2014a, 2014b). From a disease control perspective, a larger number of applications and higher dosages are expected to improve pathogens control. Thus, the conditions required for effective resistance management are in opposition to those for effective disease control; however, there should be an optimal combination of the number of applications and dosage that leads to effective disease control with a long effective life that needs to be determined with further trials (van den Berg et al., 2016).
Another important point is whether treatment is applied to the leaves or on the seed. In our trial, bacterial consortia were applied directly on seeds while the Cu-fungicide was sprayed on leaves. Normally, seed coating treatments are more active in the lower part of the canopy compared to fungicides applied with foliar sprays later in the crop growing season (Kitchen et al., 2016). Seed treatments with control agents able to induce a foliar-active systemic action can be very important because they can provide additional disease control and greater flexibility in the following foliar treatments program (Bartlett et al., 2002). Although not for all treatments, our results underline a similar trend, with plants showing a lower disease severity when treated with some bacterial consortia (C3), or with both bacteria consortia and a lower dose of Cu-fungicide (C1 + 50%). Containment of Alternaria species on leaves and fruits had the best results with consortium C2 and consortium C3 at 7 days after artificial inoculation. C2 is composed of Bacillus subtilis TR62, Bacillus amyloliquefaciens TE106, Pseudomonas fluorescens TR30 and Pseudomonas brassicacearum TR88. (Table 1). Generally, Bacillus species are well known to be effective BCA and research has demonstrated their efficacy many times, with both in vitro and in vivo experiments, where plant defense was enhanced by their biocontrol activity (Yang et al., 2023). Similarly, the Genus Pseudomonas is well established among the BCAs, with their main effects attributed to the production of antimicrobial compounds (Rezzonico et al., 2007) and other secondary metabolites (Haas and Keel, 2003).
Consortium C3 is characterized by B. amyloliquefaciens TR106 (in common with C1 and C2) but also by one Variovorax paradoxus TR65 and Chitinophaga arvensicola TR88. These bacteria species are less known for their biocontrol activity; however, these two strains had already showed their potential versus Alternaria spp. containment in a previous in vitro experiment (Bellotti et al., 2023).
Seed treatment can be important in enhancing plant defense in the first development phases, while foliar treatments can be modulated, especially as number of applications, and are extremely important in containing diseases throughout the growing season, especially in years when environmental conditions are more conducive to certain pathogens (Andreotti et al., 2022; Jaskulska et al., 2023). In our trial, the combination of seed treatment with bacterial consortia and a reduced dose of Cu-fungicide often proved to be the most effective, likely because, as generally accepted, mixing two different modes of action reduces the rate of build-up of fungal resistance (Kitchen et al., 2016; Lavrukaite et al., 2021).
In general a significant reduction in fungal presence was noticed in all treatments at the end of the growing season, also in the untreated plants. This was probably due to the meteorological conditions registered during the period of the trial with mean daily temperatures very high (29°C) and an almost total absence of rainfalls (less than 5 mm during the experiment) (Figure 7). It is well known, that Alternaria spp. are more prevalent in regions with high humidity or in semi-arid climates where frequent and prolonged night dews are common (Chaerani and Voorrips, 2006). The development of the disease in the field is mainly dependent by climatic conditions, as Alternaria species are susceptible to extreme temperatures and humidity levels (Simmons, 2007; Sudarshan et al., 2022). Moreover, weather parameters can also indirectly influence the resistance of the host (Rotem, 1994). Cooler temperatures may decrease the growth of the plant while short photoperiod can decrease sugar content in leaves, increasing the plant’s susceptibility as a consequence (Rotem, 1994). In our experiment, we used only one variety of tomato in order to reduce this variability in plant response to the disease.
Figure 7. Data on mean daily temperature (°C) and rainfall (mm) registered in field during the period of artificial inoculation with Alternaria spp.
In plants of all treatments, Alternaria strains were always found on both leaves and tomato berries. However, considering the same plants at the end of the growing season, the different capability to contain the disease became more evident. Over the long term, bacterial consortia seem to reduce their ability to contain fungi, while consortia+50% fungicide treatments maintained their efficacy, particularly for C3, which showed the lowest number of Alternaria on leaves at the harvest time.
In previous studies, the efficacy of bacteria against mycotoxigenic fungi have already been demonstrated, even if with different results depending on bacteria species and target pathogen (Bellotti et al., 2023). The mechanism by which interaction occurs between PGPR and phytopathogenic fungi are not thoroughly addressed, however the development of a consortium made up by several strains can guarantee a broader spectrum of action and a lower impact of environmental variabilities to which a field is normally subjected throughout the crop season (Singh et al., 2023).
The presence of Alternaria spp. on tomato berries was lower than on leaves but it can lead to the presence of secondary metabolites that pose a risk for final consumers. It is also important to remember that Alternaria species can produce about 70 toxic metabolites with possible significance for human and animal health (Sanzani et al., 2019).
In our trial, the highest presence of Alternaria species on berries was found in treatments with 50% dose of Cu-fungicide. The presence of mycotoxigenic strains and the level of mycotoxins is not always correlated. Many studies underline that under stress conditions, mycotoxigenic fungi can increase the production of mycotoxins (Medina et al., 2015; Ponts, 2015). During the trial, Alternaria strains present on tomato plants were subjected to ecological conditions characterized by extreme temperatures and lack of rainfalls considered not optimal for their development. Moreover, plants were treated with substances able to contrast fungal growth (Cu-fungicide treatment or consortia treatment) and this can result in a stress able to drive Alternaria strains to an increment in mycotoxins production. However, antifungal activity of bacteria against some mycotoxigenic fungi such as Aspergillus flavus and Aspergillus parasiticus was already shown by iturin produced by Bacillus pumilus isolated from soybean sauce (Cho et al., 2009) or by some lipopeptides produced by Bacillus subtilis (Bertuzzi et al., 2022) or by Bacillus vallismortis successful against Alternata alternata (Kaur et al., 2017).
The presence of Alternaria toxins in fresh tomatoes and in its derived products is an emerging risk for consumers. In 2016, the European Food Safety Authority (EFSA) assessed that TeA was the most prevalent and relevant mycotoxin among the Alternaria toxins, pointing out that some age group (such as infants or toddlers) and some specific people (like vegetarians) could be more exposed to this mycotoxin than the general population (Arcella et al., 2016). In 2022, the European Union emanated a recommendation (2022/553) setting the limits for Alternaria toxins in foodstuff, including products from processed tomatoes. More studies on these mycotoxins and on their toxicology are needed to protect consumers. The use of microbial populations to protect plants may be a safe and promising alternative to synthetic fungicides able to combine the necessity to reduce environmental pollution but, at the same time, find an effective tool to contain pathogens in field.
This study supports the potential use of PGPR consortia as for the biocontrol of Alternaria spp. infections in tomato plants. The key findings indicated that PGPRs consortia were effective in reducing both fungal colonization and Alternaria toxins (TeA) in tomato plants and berries. In the short term, some PGPR consortia alone showed a higher effectiveness in containing Alternaria, but over time the combination of PGPR consortia with 50% of Cu-based fungicide seemed to have a more solid effect on the biological control of Alternaria. Given the growing concerns over Cu-fungicide resistance and environmental impact, the integration of PGPR consortia into disease management programs offers a promising strategy to enhance plant health while reducing farmers reliance on synthetic organic or conventional products.
The raw data supporting the conclusions of this article will be made available by the authors, without undue reservation.
PG: Writing – review & editing, Writing – original draft, Methodology, Investigation, Data curation, Conceptualization. GBu: Writing – original draft, Investigation, Data curation. GBe: Writing – review & editing, Methodology, Investigation. MA: Writing – review & editing. MG: Writing – review & editing, Methodology. AF: Writing – original draft, Conceptualization. TB: Writing – review & editing, Writing – original draft, Investigation, Data curation, Conceptualization. EP: Writing – review & editing, Supervision, Project administration, Funding acquisition, Conceptualization.
The author(s) declare financial support was received for the research, authorship, and/or publication of this article. The authors would like to acknowledge the financial support provided by project PROBIOPOM (CUP J34I20000790009) funded by the region of Lombardy, Italy.
The authors declare that the research was conducted in the absence of any commercial or financial relationships that could be construed as a potential conflict of interest.
The author(s) declared that they were an editorial board member of Frontiers, at the time of submission. This had no impact on the peer review process and the final decision.
All claims expressed in this article are solely those of the authors and do not necessarily represent those of their affiliated organizations, or those of the publisher, the editors and the reviewers. Any product that may be evaluated in this article, or claim that may be made by its manufacturer, is not guaranteed or endorsed by the publisher.
The Supplementary Material for this article can be found online at: https://www.frontiersin.org/articles/10.3389/fhort.2024.1447425/full#supplementary-material
Andreotti C., Rouphael Y., Colla G., Basile B. (2022). Rate and timing of application of biostimulant substances to enhance fruit tree tolerance toward environmental stresses and fruit quality. Agronomy 12, 603. doi: 10.3390/agronomy12030603
Arcella D., Eskola M., Gómez Ruiz J. A. (2016). Dietary exposure assessment to Alternaria toxins in the European population. EFSA J. 14, e04654. doi: 0.2903/j.efsa.2016.4654
(2022). EU Commission recommendation (EC) no 553/2022 of 5 April 2022 related to Alternaria toxins in certain foodstuffs (Bruxelles, Belgium: Official J. European Union).
Bartlett D. W., Clough J. M., Godwin J. R., Hall A. A., Hamer M., Parr-Dobrzanski B. (2002). The strobilurin fungicides. Pest Manage. Sci. 58, 649–662. doi: 10.1002/ps.520
Bellotti G., Guerrieri M. C., Giorni P., Bulla G., Fiorini A., Bertuzzi T., et al. (2023). Enhancing plant defense using rhizobacteria in processing tomatoes: a bioprospecting approach to overcoming Early Blight and Alternaria toxins. Front. Microbiol. 14, 1221633. doi: 10.3389/fmicb.2023.1221633
Bertuzzi T., Leni G., Bulla G., Giorni P. (2022). Reduction of mycotoxigenic fungi growth and their mycotoxins production by Bacillus subtilis QST 713. Toxins 14, 797. doi: 10.3390/toxins14110797
Bertuzzi T., Rastelli S., Pietri A., Giorni P. (2021). Alternaria toxins in tomato products in Northern Italy in the period 2017-2019. Food Addit. Contam. B. 14, 170–176. doi: 10.1080/19393210.2021.1895325
Bessadat N., Hamon B., Henni J. E., Simoneau P. (2016). First report of tomato early blight caused by Alternaria grandis in Algeria. Plant Dis. 102, 2651. doi: 10.1094/PDIS-05-15-0613-PDN
Boukhatem Z. F., Merabet C., Tsaki H. (2022). Plant growth promoting actinobacteria, the most promising candidates as bioinoculants? Front. Agron. 4, 849911. doi: 10.3389/fagro.2022.849911
Cardoso E. F., Lopes A. R., Dotto M., Pirola K., Giarola C. M. (2021). Phenological growth stages of Gaúcho tomato based on the BBCH scale. Comunicata Scientiae. 12, e3490–e3490. doi: 10.14295/cs.v12.3490
Chaerani R., Voorrips R. E. (2006). Tomato early blight (Alternaria solani): the pathogen, genetics and breeding for resistance. J. Gen. Plant Pathol. 72, 335–347. doi: 10.1007/s10327-006-0299-3
Cho K. M., Math R. K., Hong S. Y., Asraful Islam S. M., Mandanna D. K., Cho J. J., et al. (2009). Iturin produced by bacillus pumilus HY1 from korean soybean sauce (Kanjang) inhibits growth of aflatoxin producing fungi. Food Control. 20, 402–406. doi: 10.1016/j.foodcont.2008.07.010
Clewer A. G., Scarisbrick D. H. (2001). Practical statistics and experimental design for plant and crop science (England: PublisherL John Wiley & Sons, Ltd).
Deaker R., Roughley R. J., Kennedy I. R. (2004). Legume seed inoculation technology—a review. Soil Biol. Biochem. 36, 1275–1288. doi: 10.1016/j.soilbio.2004.04.009
Dias M. C. (2012). Phytotoxicity: An overview of the physiological responses of plants exposed to fungicides. J. Bot. 2012, 135479. doi: 10.1155/2012/135479
Egel D. S., Hoagland L., Davis J., Marchino C., Bloomquist M. (2019). Efficacy of organic disease control products on common foliar disease of tomato in field and greenhouse trials. Crop Prot. 122, 90–97. doi: 10.1016/j.cropro.2019.04.022
El-Saadony M. T., Saad A. M., Soliman S. M., Salem H. M., Ahmed A. I., Mahmood M., et al. (2022). Plant growth-promoting microorganisms as biocontrol agents of plant diseases: mechanisms, challenges and future perspectives. Front. Plant Sci. 13, 923880. doi: 10.3389/fpls.2022.923880
Garganese F., Sanzani S. M., Di Rella D., Schena L., Ippolito A. (2019). Pre- and postharvest application of alternative means to control Alternaria Brown spot of citrus. Crop Prot. 121, 73–79. doi: 10.1016/j.cropro.2019.03.014
Giorni P., Bulla G., Leni G., Soldano M., Tacchini M., Guerrini A., et al. (2023). Enhancement of agri-food by-products: green extractions of bioactive molecules with fungicidal action against mycotoxigenic fungi and their mycotoxins. Front. Nutr. 10, 1196812. doi: 10.3389/fnut.2023.1196812
Haas D., Keel C. (2003). Regulation of antibiotic production in root-colonizing Pseudomonas spp. and relevance for biological control of plant disease. Annu. Rev. Phytopathol. 41, 117–153. doi: 10.1146/annurev.phyto.41.052002.095656
Habib W., Masiello M., El-Ghorayeb R., Gerges E., Susca A., Meca G., et al. (2021). Mycotoxin profile and phylogeny of pathogenic Alternaria species isolated from symptomatic tomato plants in Lebanon. Toxins 13, 513. doi: 10.3390/toxins13080513
Jaskulska I., Kamieniarz J., Jaskulski D., Radziemska M., Brtnicky M. (2023). Fungicidal protection as part of the integrated cultivation of sugar beet: an assessment of the influence on root yield in a long-term study. Agric 13, 346. doi: 10.3390/agriculture13071449
Kaur P. K., Joshi N., Singh I. P., Saini H. S. (2017). Identification of cyclic lipopeptides produced by Bacillus vallismortis R2 and their antifungal activity against Alternaria alternata. J. Appl. Microbiol. 122, 139–152. doi: 10.1111/jam.2017.122.issue-1
Khalil M. I. I., Youssef S. A., Tartoura K. A., Eldesoky A. A. (2021). Comparative evaluation of physiological and biochemical alteration in tomato plants infected by Alternaria alternata in response to Trichoderma viride and Chaetomium globosum application. Physiol. Mol. Plant Pathol. 115, 101671. doi: 10.1016/j.pmpp.2021.101671
Kitchen J. L., van den Bosch F., Paveley N. D., Helps J., van den Berg F. (2016). The evolution of fungicide resistance resulting from combinations of foliar-acting systemic seed treatments and foliar-applied fungicides: a modeling analysis. PloS One. doi: 10.1371/journal.pone.0161887
Lavrukaite K., Heick T. M., Ramanauskiene J., Armoniene R., Ronis A. (2021). Fungicide sensitivity levels in the Lithuanian Zymoseptoria tritici population in 2021. Front. Plant Sci. 14, 36714691. doi: 10.3389/fpls.2022.1075038
Lee J., Kim S., Jung H., Koo B. K., Han J. A., Lee H. S. (2023). Exploiting bacterial genera as biocontrol agents: Mechanisms, interactions and applications in sustainable agriculture. J. Plant Biol. 66, 485–498. doi: 10.1007/s12374-023-09404-6
Medina A., Rodríguez A., Sultan Y., Magan N. (2015). Climate change factors and A. flavus: effects on gene expression, growth and aflatoxin production. World Mycotoxin J. 8, 171–179. doi: 10.3920/WMJ2014.1726
Meena M., Zehra A., Dubey M. K., Aamir M., Gupta V. K., Upadhyay R. S. (2016). Comparative evaluation of biochemical changes in tomato (Lycopersicon esculentum Mill.) infected by Alternaria alternata and its toxic metabolites (TeA, AOH, and AME). Front. Plant Sci. 7, 1408. doi: 10.3389/fpls.2016.01408
Nash A. F., Gardner R. G. (1988). Tomato early blight resistance in a breeding line derived from Lycopersicon hirsutum PI 126445. Plant Dis. 72, 206–209. doi: 10.1094/PD-72-0206
Parvin I., Mondal C., Sultana S., Sultana N., Aminuzzaman F. M. (2021). Pathological survey on early leaf blight of tomato and in vitro effect of culture media, temperature and pH on growth and sporulation of Alternaria solani. Open Access Library J. 8, 1–17. doi: 10.4236/oalib.1107219
Pieterse C. M. J., Zamioudis C., Berendsen R. L., Weller D. M., Van Wees S. C. M., Bakker P. A. H. M. (2014). Induced systemic resistance by beneficial microbes. Annu. Rev. Phytopathol. 52, 347–375. doi: 10.1146/annurev-phyto-082712-102340
Ponts N. (2015). Mycotoxins are a component of Fusarium graminearum stress-response system. Front. Microbiol. 6, 1234. doi: 10.3389/fmicb.2015.01234
Rezzonico F., Zala M., Keel C., Duffy B., Moënne-Loccoz Y., Défago G. (2007). Is the ability of biocontrol fluorescent pseudomonads to produce the antifungal metabolite 2, 4-diacetylphloroglucinol really synonymous with higher plant protection? New Phytol. 173, 861–872. doi: 10.1111/j.1469-8137.2006.01955.x
Rotem J. (1994). The genus alternaria. Biology, epidemiology and pathogenicity (Saint Paul: APS Press).
Sanzani S. M., Gallone T., Garganese F., Caruso A. G., Amenduni M., Ippolito A. (2019). Contamination of fresh and dried tomato by Alternaria toxins in southern Italy. Food Addit. Contam. A. 36, 789–799. doi: 10.1080/19440049.2019.1588998
Simmons E. G. (2007). Alternaria. An identification manual (Utrecht, Netherlands: CBS Fungal Biodiversity Centre).
Singh A., Yadav V. K., Chundawat R. S., Soltane R., Awwad N. S., Ibrahium H. A., et al. (2023). Enhancing plant growth promoting rhizobacterial activities through consortium exposure: A review. Front. Bioeng. Biotechnol. 11, 1099999. doi: 10.3389/fbioe.2023.1099999
Solfrizzo M. (2017). Recent advances on Alternaria mycotoxins. Curr. Opin. Food Sci. 17, 57–61. doi: 10.1016/j.cofs.2017.09.012
Sudarshan G. K., Nagaraj M. S., Yogananda S. B., Gowda A. P. M. (2022). Role of weather parameters on development of early blight of tomato caused by Alternaria solani. Indian J. Ecol. 49, 543–548. doi: 10.55362/IJE/2022/3558
Thomma B. P. H. J. (2003). Alternaria spp.: from general saprophyte to specific parasite. Mol. Plant Pathol. 4, 225–236. doi: 10.1046/j.1364-3703.2003.00173.x
Timmusk S., Pall T., Raz S., Fetsiukh A., Nevo E. (2023). The potential for plant growth-promoting bacteria to impact crop productivity in future agricultural systems is linked to understanding the principles of microbial ecology. Front. Microbiol. 14, 1141862. doi: 10.3389/fmicb.2023.1141862
Trebbi G., Negri L., Bosi S., Dinelli G., Cozzo R., Marotti I. (2021). Evaluation of Equisetum arvense (horsetail macerate) as a copper substitute for pathogen management in field-grown organic tomato and durum wheat cultivations. Agric 11, 5. doi: 10.3390/agriculture11010005
van den Berg F., Paveley N. D., van den Bosch F. (2016). Dose and number of applications that maximize fungicide effective life exemplified by Zymoseptoria tritici on wheat – a model analysis. Plant Pathol. 65, 1380–1389. doi: 10.1111/ppa.12558
van den Bosch F., Oliver R., van den Berg F., Paveley N. (2014a). Governing principles can guide fungicide-resistance management tactics. Ann. Rev. Phytopathol. 52, 175–195. doi: 10.1146/annurev-phyto-102313-050158
van den Bosch F., Paveley N., Shaw M., Hobbelen P., Oliver R. (2011). The dose rate debate: does the risk of fungicide resistance increase or decrease with dose? Plant Pathol. 60, 597–606. doi: 10.1111/j.1365-3059.2011.02439.x
van den Bosch F., Paveley N. D., van den Berg F., Hobbelen P., Oliver R. (2014b). Mixtures as a fungicide resistance management tactic. Phytopathol 104, 1264–1273. doi: 10.1094/PHYTO-04-14-0121-RVW
World Processing Tomato Council (2022). Available online at: https://www.wptc.to/trade/. access date: January 21st, 2024
Keywords: plant disease control, early blight, Alternaria toxins, biological control, PGPR, plant-microbe interactions, crop protection
Citation: Giorni P, Bulla G, Bellotti G, Antinori ME, Guerrieri MC, Fiorini A, Bertuzzi T and Puglisi E (2024) In planta evaluation of different bacterial consortia for the protection of tomato plants against Alternaria spp. infection and Alternaria toxins presence in fruits. Front. Hortic. 3:1447425. doi: 10.3389/fhort.2024.1447425
Received: 11 June 2024; Accepted: 02 August 2024;
Published: 21 August 2024.
Edited by:
Luisa Ghelardini, University of Florence, ItalyReviewed by:
Loukas I. Kanetis, Cyprus University of Technology, CyprusCopyright © 2024 Giorni, Bulla, Bellotti, Antinori, Guerrieri, Fiorini, Bertuzzi and Puglisi. This is an open-access article distributed under the terms of the Creative Commons Attribution License (CC BY). The use, distribution or reproduction in other forums is permitted, provided the original author(s) and the copyright owner(s) are credited and that the original publication in this journal is cited, in accordance with accepted academic practice. No use, distribution or reproduction is permitted which does not comply with these terms.
*Correspondence: Terenzio Bertuzzi, dGVyZW56aW8uYmVydHV6emlAdW5pY2F0dC5pdA==
Disclaimer: All claims expressed in this article are solely those of the authors and do not necessarily represent those of their affiliated organizations, or those of the publisher, the editors and the reviewers. Any product that may be evaluated in this article or claim that may be made by its manufacturer is not guaranteed or endorsed by the publisher.
Research integrity at Frontiers
Learn more about the work of our research integrity team to safeguard the quality of each article we publish.