- 1Department of Postharvest Science, Agricultural Research Organization (ARO), Volcani Center, Rishon LeZion, Israel
- 2The Robert H. Smith Faculty of Agriculture, Food and Environment, The Hebrew University of Jerusalem, Rehovot, Israel
- 3Department of Vegetables, Agricultural Extension Service of the Ministry of Agriculture, Bet Dagan, Israel
- 4Southern Research and Development, Besor Experiment Station, Negev, Israel
- 5Newe Ya’ar Research Center, Agricultural Research Organization, Ramat Yishay, Israel
Acorn squash (Cucurbita pepo) have been a familiar item at produce stands for decades in the United States and Canada, but little known or appreciated elsewhere. Following the breeding and development of sweet-fleshed acorn squash in Israel and its commercial introduction in 2007, acorn squash became a high-priced, popular produce item there. Scarcity of supply in winter has led to attempts to fill the consumer demand by using available protected cultivation infrastructure in the relatively mild area of southwestern Israel for production. Such production has proven feasible but it was not determined whether it would be preferable to allow the plants to simply grow sprawling on the ground or vertically, using trellises to train the plants to grow erect. Two sweet acorn squash hybrid cultivars differing in fruit size were compared, growing on the ground or on trellises, for yield, quality, and storability of the fruits. The hybrids bore fully ripe fruits from December through February, producing 56% higher yields when trellised rather than when allowed to grow on the ground. The fruits of trellised plants of both hybrids were more uniformly black-green and firmer than those of ground-grown plants. Their dry matter content at harvest and after 78 days of storage was very high, averaging 28% and 25%, respectively. Total soluble solids content of the fruit flesh from trellised plants was 19% at harvest and an extraordinary 20% after storage at 10°C, 70% RH. The fruit flesh of trellised plants was also more highly colored and had higher carotenoid, ascorbate, and anti-oxidant contents. Overall, trellising of sweet acorn squash during the winter under protected cultivation resulted in significantly higher yields and the finest fruit quality.
1 Introduction
Vegetable crops have been cultivated under protected conditions for 2000 years (Janick and Paris, 2022). Production has been steadily increasing and is expected to continue increasing as new technologies are developed (Mitchell, 2022; Nemali, 2022). Protected conditions can allow out-of-season production and afford exclusion of pests and diseases, saving or reducing the need for insecticide or fungicide applications. The high-value, warm-season fruit vegetables, including various Cucurbitaceae and Solanaceae, are grown protected, in greenhouses and screenhouses, where open-field conditions are too cool, or pests and diseases too prevalent, for their profitable production (Russo et al., 1991; Cantliffe and Vansickle, 2001; Jovicich et al., 2004; Cook and Calvin, 2005; Parker et al., 2019; Batziakas et al., 2022; Rutgers New Jersey Agricultural Experiment Station, 2022). Given the various inputs required for construction and maintenance of the infrastructure, the protected space and the time required for production of the crop are at a premium. Profitable, efficient production requires that yield per unit area and quality of the product be maximized over time.
In the Besor area of southwestern Israel, there are approximately 1000 ha of 50-mesh screened greenhouses (Avraham et al., 2020). The 50-mesh screening is critical for preventing entrance of whiteflies (Bemisia spp.), which by vectoring virus diseases are a production-limiting factor for open-field production in this area. Most of these screenhouses are planted in March for production of Dutch-trellised tomatoes (Solanum lycopersicum L.) during the ensuing summer months. Tomato production ends in late summer, at which time many of the screenhouses become vacant. Nonetheless, autumn and early winter conditions in this area are frost-free with relatively mild temperatures, allowing for profitable out-of-season production of some warm-season vegetables in these structures. Among the vegetable crops that have been considered fit for possibly taking advantage of this available infrastructure is sweet acorn squash.
Acorn squash (Cucurbita pepo L. subsp. ovifera Acorn Group) were developed by indigenous people of eastern North America in pre-Columbian times (Paris, 2000). Early records are scant but nonetheless suggest that acorn squash were grown in this region over the centuries following the European contact and, by the early 20th century, they had become a familiar and esteemed produce item there. Acorn squash are one of the eight edible-fruited morphotypes (cultivar-groups) of Cucurbita pepo. They are distinguished from the others by their turbinate shape and alternating radial ridges and furrows (Paris, 1986). Acorn squash are quite popular today in the United States and Canada because of their attractive appearance and convenient small size (≤ 1 kg.). However, they are usually inferior in sweetness as compared with the larger and less attractive buttercup and kabocha squash (C. maxima Duchesne) and butternut squash (C. moschata Duchesne) (Loy, 2004). All of these winter squash are grown in open fields and usually have a viney, prostrate growth, requiring much space for plant development and fruit production (Wehner et al., 2020). Usually, three or four months are required from planting until the fruits mature and ripen (Loy, 2004; Ferriol and Pico, 2008). The harvested fruits, if properly handled and stored, can be kept for two months or more (Ferriol and Pico, 2008; Michigan State University Extension, 2021). Consumer perception of sweetness and quality of the fruits can be objectively, quickly, and easily predicted by measuring their total soluble solids (TSS) and dry matter (DM) contents (Loy, 2004, Loy, 2012; Silva and Bruce, 2016).
A long-term breeding effort was conducted in Israel to combine greater sweetness, bush growth habit, black-green rind color and powdery mildew resistance (PMR) in acorn squash. With the 2007 introduction of the sweet, bush-habit, black-green PMR hybrid ‘Table Sugar’, a consumer demand specifically for acorn squash began in this country (Paris and Godinger, 2016). Among the various winter squash and pumpkins found in Israeli markets, there has developed a strong consumer preference and appreciation for this sweet acorn squash, its price often double or more that of all other winter squash and pumpkins. During the winter, when supply has been lacking, its retail price has reached record levels, exceeding the equivalent of US$10.00 per kg. The fruits of ‘Table Sugar’ are quite small, averaging 350 g, but its commercial success led to the breeding of the larger fruited, sweet, bush-habit, black-green, PMR hybrid acorn squash ‘Table Confection’, which entered Israeli markets in 2017.
The strong consumer demand coupled with lack of supply of sweet acorn squash led us to attempt to utilize the available screenhouses in the Besor area for winter production. We have determined that such production is indeed quite feasible (Adeeko et al., 2020) but until now have not focused on determining whether growing the plants vertically, on trellises, would be advantageous to simply allowing them to grow sprawling on the ground. Our objective was to determine if trellising would be worthwhile for management of sweet acorn squash production in this environment, with regard to yield and quality of freshly harvested and stored fruits.
2 Materials and methods
2.1 Plant material, growing conditions and harvest
Seeds of two acorn squash hybrids, ‘Table Sugar’ (Hybrid 1700) and ‘Table Confection’ (Hybrid 2005), which were bred by the Cucurbits Section of the Agricultural Research Organization at the Newe Ya‘ar Research Center (northern Israel), were obtained from Origene Seeds (Giv‘at Brenner, Israel). Descriptors according to the Plant Variety Protection Office of the U.S.D.A. (United States Department of Agriculture, 2008) are listed for these hybrids in Table 1. The seeds were sown on 27 August, 2018, in styrofoam trays consisting of 128 34 mm × 34 mm cells (measured at the top), in a commercial greenhouse at Hishtil Nurseries, Ashqelon, Israel. On 17 September 2018, the seedlings were transplanted into a sandy soil covered with gray-silver plastic mulch in a 50-mesh screenhouse at the Besor Experiment Station, Southern Research and Development, in southwestern Israel (31°16′20″ N, 34°23′15″ E, elev. 102 m) (Avraham et al., 2018). They were set one every 40 cm within rows, with 2 m between rows, resulting in a density of 1.25 plants/m2. The plants were irrigated and fertilized via drip lines that had been laid under the plastic mulch.
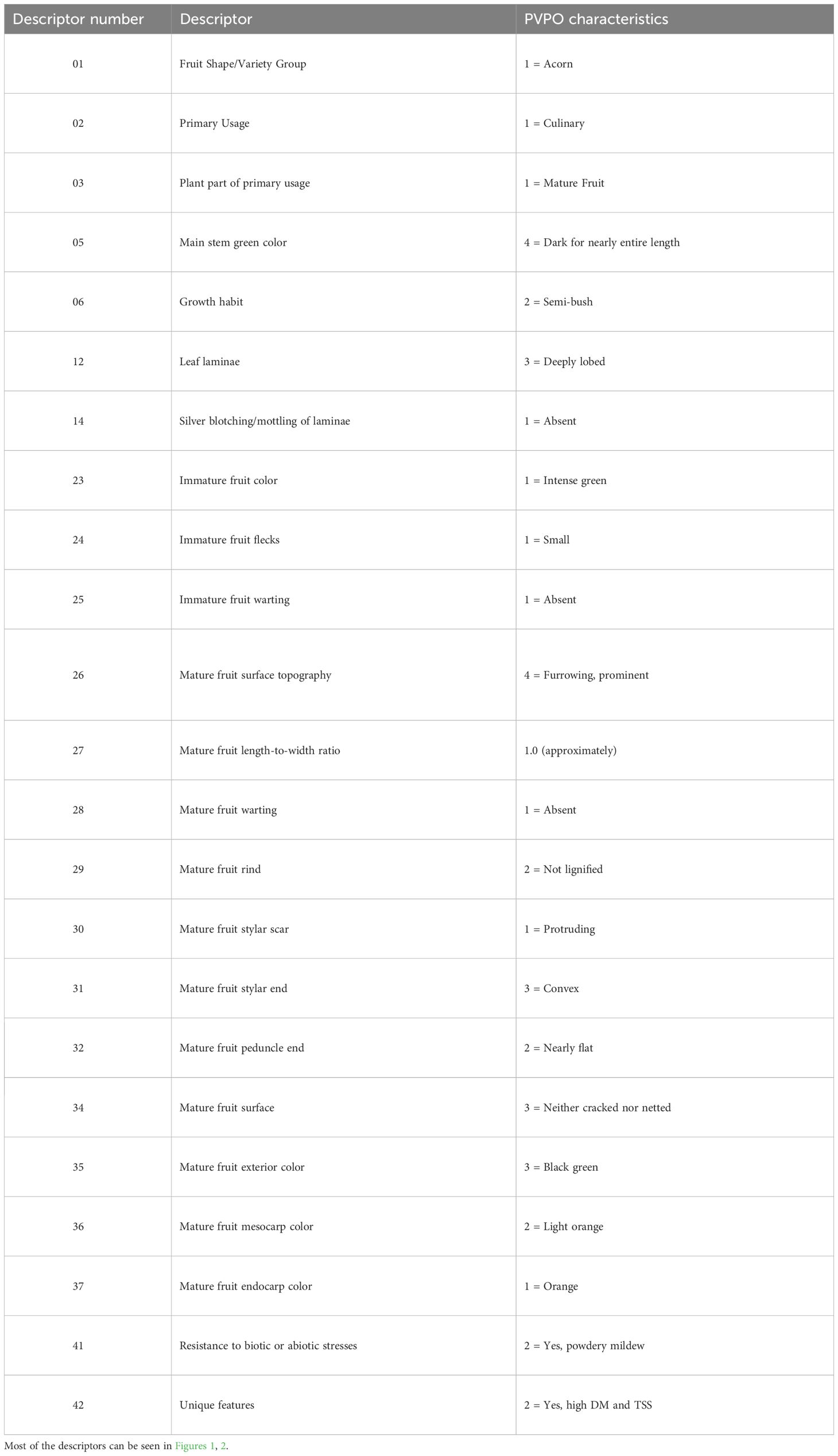
Table 1 Descriptors of ‘Table Sugar’ and ‘Table Confection’ acorn squash, based on the “Objective Description of Variety” for Cucurbita pepo, Exhibit C, of the Plant Variety Protection Office (PVPO), Agricultural Marketing Service (United States Department of Agriculture, 2008).
The plants were grown by either being freely allowed to develop on the ground or by training vertically using the Dutch trellis system (Avraham et al., 2018), the latter method including nipping off all side branches when each had developed two leaves (Figure 1). Flowering began approximately three weeks after transplanting. On October 07, a hive of bumblebees (Bombus spp., Bio Bee Ltd., Sede Eliyyahu, Israel) was placed in the screenhouse. Three waves of fruit set occurred over the course of October and November, in both hybrids and in both growing regimes, that is, in all 4 treatments. The plants weakened, became chlorotic, and ceased bearing fruits during the winter months, probably due to the heavy load of maturing fruits combined with lower temperatures and less insolation.
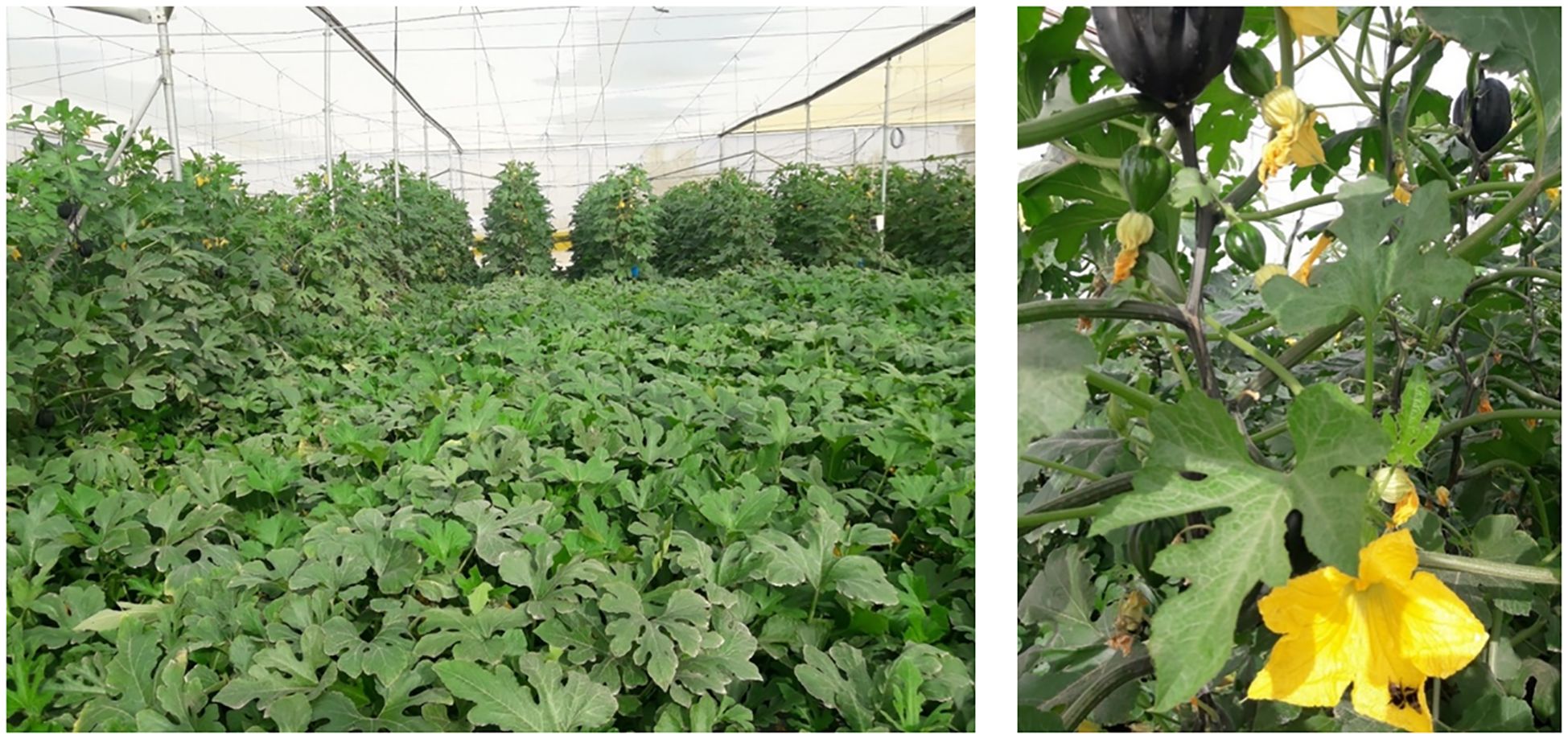
Figure 1 Out-of-season protected cultivation of sweet acorn squash in a 50-mesh-screened greenhouse at the Besor Experiment Station. Left: Ground-grown, sprawling (foreground) and vertically grown, trellised (left and background) sweet acorn squash plants. Right: Trellised plants with developing fruits of the ‘Table Confection’ sweet acorn squash.
For assessing yield and its components, the experiment consisted of split blocks of trellis-grown and ground-grown plants (Figure 1) and each of the 2 hybrids was represented by 4 randomized plots that had been demarcated within the split blocks. Each plot consisted of 30 plants from 12 m length of row (24 m2), for a total of 120 plants per treatment, there being 4 treatments based on the 2 hybrids × 2 growing regimes.
2.2 Harvesting and postharvest handling
Decisions concerning when to harvest were taken on the basis of internal color and seed maturity observed in cut fruits taken from plants bordering the experiment. There were 3 harvests from all 4 treatments, on 10 December 2018, 15 January 2019, and 20 February 2019. All fruits from the treatment plots were counted and weighed for all three harvests.
From the second harvest (15 January 2019), which was the largest, being comprised of nearly half of the fruits (Supplementary Figure S1), random samplings of 15–20 fruits from each treatment were taken to the fruit storage laboratory of the Department of Postharvest and Food Sciences at the Volcani Center. There, the fruits were rinsed with tap water, air-dried, weighed, and evaluated for the quality parameters enumerated below on the day of harvest, or after storage for 78 days at either 15 °C and 95% relative humidity (RH) or 10 °C and 70% RH. This long storage period was chosen to determine whether or not the fruits would remain in acceptable condition until spring, when sweet acorn squash are still in short supply at markets.
2.3 Quality components and their evaluation
The quality traits that were evaluated at harvest included fruit firmness, rind (peel, exocarp) and fruit flesh (pulp, mesocarp) color, percent dry weight (%DW) and total soluble solids (TSS), sensorial testing, and total carotenoid, ascorbate, and antioxidant contents. Rind color was evaluated for all the fruits, while internal quality was determined for at least five fruits per treatment. After storage, a sample of five fruits from each treatment was evaluated for firmness, rind and flesh color, weight loss, percent dry weight (%DW), total soluble solids (TSS), and sensorially.
2.3.1 Fruit firmness
Fruit firmness was measured in Newtons (N) using a motorized Chatillon penetrometer equipped with a 6-mm conic plunger (John Chatillon & Sons, Kew Gardens, Queens, NY, USA). Measurements were taken at two points, on opposite sides of each fruit at the equatorial region (Chalupowicz et al., 2018).
2.3.2 Rind color
Fruit exocarp (rind) color before storage and at the end of storage was evaluated quantitatively using a Chromo Meter CR-400/410 (Konica Minolta, Osaka, Japan). Two measurements, using the color space L*C*h were taken near the blossom (stylar) end of each fruit and averaged (Adeeko et al., 2020). The color of the fruit rind is reported as hue angle (Hue°), corresponding to green and blue, which are positioned at 180° and 270°, respectively. The lightness of fruit rind color is reported with value L* defining black as 0 and white as 100.
2.3.3 Fruit-flesh color
Fruit mesocarp (flesh, pulp) color was also evaluated quantitatively using the Chromo Meter CR-400/410 (Konica Minolta, Osaka, Japan). Fruits were cut equatorially at the approximate middle of the length of the fruit and three measurements were taken from three approximately equidistant points and averaged using color space L*a*b*. Values a* and b* represent the colors green to red and blue to yellow, respectively. Negative values a* and b* were reflective of a more greenish coloration of the fruit flesh while positive values were more toward yellow and orange coloration (Adeeko et al., 2020). These color-space values have been shown to be strongly correlated with carotenoid content in pumpkins and squash (Itle and Kabelka, 2009).
2.3.4 Percent dry weight of the fruit flesh
The fruits were cut open and the seed cavity (containing the seeds and placenta) of each scooped out and the rind peeled off. A fresh sample of fruit flesh 3–5 mm thick (approximately 10 g) was precisely weighed. This sample piece was then placed on an aluminum dish and dried in an oven at 55°C for 3 days. The dried sample was then weighed (Adeeko et al., 2020). Percent dry weight was determined by dividing the weight of the sample after drying by its weight when fresh, multiplied by 100.
2.3.5 Total soluble solids
Cut samples of fruit flesh were frozen overnight and thawed. After thawing, the fruit flesh samples were squeezed and their juice thereby extracted onto a Palette Digital Refractometer PR-1 (Model DBX-55, Atago, Tokyo, Japan) (Chalupowicz et al., 2018). The results are expressed as percent total soluble solids (TSS).
2.3.6 Sensory evaluation
Sensory quality of the fruit flesh was assessed by a panel of 28–30 untrained people (Adeeko et al., 2020). The analysis was for four parameters on a scale of 1 to 4: Sweetness 1 = not sweet, 2 = moderately sweet, 3 = sweet, 4 = very sweet. Texture 1 = very fibrous, 2 = fibrous, 3 = slightly fibrous, 4 = smooth. General impression 1 = not tasty, 2 = moderately tasty, 3 = tasty, 4 = very tasty. Non-bitterness 1 = very bitter, 2 = moderately bitter, 3 = slightly bitter, 4 = not bitter.
2.3.7 Weight loss after storage
Fruits were weighed on the day of harvest (prior to storage) and at the end of the storage period, 78 days after harvest. Weight loss was calculated by subtraction; percent weight loss was then calculated by dividing the remainder by the weight on the day of harvest, multiplied by 100 (Chalupowicz et al., 2018).
2.3.8 Total carotenoid content
Carotenoid extraction was performed using established protocols (Tadmor et al., 2005; Azevedo-Moleiro and Rodriguez-Amaya, 2007; Itle and Kabelka, 2009; Bonita-Noseworthy et al., 2016). Carotenoids were extracted and analyzed by HPLC in five fruits of each of the four treatments. Fresh mesocarp tissue was ground under liquid nitrogen. Total carotenoids from frozen ground samples (0.5 g) were extracted with a hexane:acetone:ethanol (2:1:1, v/v/v) mixture according to the method described by Tadmor et al. (2005). Extracted carotenoids were saponified with 80% w/v KOH methanolic solution for 5 min at 85°C to hydrolyze esterified carotenoids. The extracted pigments were separated and detected using a C18 Nova-Pak (Waters) column (250 × 4.6 mm i.d., 60 A, 4 mm) and a Nova-Pak Sentry Guard cartridge (Waters) using Waters 2695 HPLC apparatus equipped with a Waters 996 photodiode array detector, as described previously (Chayut et al., 2017). Quantification was performed using commercial β-carotene as the calibration standard.
2.3.9 Ascorbate content
Ascorbic acid content was measured using the Folin reagent method (Jagota and Dani, 1982). Fruit tissue (200 mg) was ground and mixed with 0.8 ml 10% (w/v) trichloroacetic acid (TCA) and centrifuged for 20 minutes at 13000 g and the supernatant transferred to a new tube; all steps were performed at below 4°C and in minimum light. The reaction mixture was made with 160 μl double distilled water and 20 μl of 10-fold diluted Folin reagent (Sigma Aldrich, Louis MO, USA), and was then mixed with 40 μl of the fruit extract. The reaction mixture was incubated at room temperature in the dark for 10 min and the absorbance was thereafter measured in a spectrophotometer (Ultrospec 2100 pro, Amersham Bioscience, England) at 760 nm. The concentration of ascorbate per 100 g was calculated to a standard calibration curve prepared from commercial L-ascorbic acid (Sigma-Aldrich, St. Louis MO, USA). Low pH is an important factor in stabilizing ascorbic acid in plant extracts and using TCA reduced interference by other substances such as phenols, tannins, and glutathione (Kim et al., 1987).
2.3.10 Antioxidant activity
Antioxidant activity was measured using the Trolox Equivalent Capacity (TEAC) assay. This protocol is based on the reduction of ABTS+ [2,2’-azino-bis(3-ethylbenzothiazoline-6-sulfonic acid)] radicals by antioxidants of plant extract samples, as according to Xiao et al. (2020).
Fruit sample extractions were performed in accordance with Vinokur and Rodov (2006) to separate hydrophilic and lipophilic compounds. Briefly, acorn squash sample tissue (200 mg) was ground to powder in liquid nitrogen and extracted with 1 ml of 0.2 M acetate buffer (pH 4.5) for hydrophilic compounds or acidified ethanol for lipophilic/hydrophobic compounds. Measurements were conducted in the spectrophotometer at 734 nm and the ABTS value was calculated according to Xiao et al. (2020). The spectrophotometer readings were baselined using 0.2 M acetate buffer (pH 4.3) and Trolox was used as the standard for the calibration curve. The reagents were purchased from Sigma-Aldrich, Rehovot, Israel. The results are expressed as μmol TE/g FW according to the formula:
where C is Trolox equivalents (μmol/ml) calculated using the regression equation between absorbance at 734 nm and Trolox standards (with concentrations ranging from 0.0-0.6 μM/ml), V is the sample volume (ml), t is the dilution factor, and m is the weight of the sample (in g of fresh tissue).
2.4 Statistical analysis
The data were subjected to Tukey-Kramer tests by one- or two-tailed ANOVA from 0.05 to 0.001, or to Student’s t tests, using the JMP 16 Statistical Analysis Software Program (JMP Pro 16.0 SAS Institute, Cary, NC U.S.A.). Significance was set at P ≤ 0.05.
3 Results
3.1 Yield
Trellised plants of ‘Table Sugar’ outyielded their ground-grown counterparts by 35%. The fruits from both growing methods were small, averaging 350 g. The greater yield of the trellised plants was attributable entirely to the greater number of fruits they produced (Table 2).
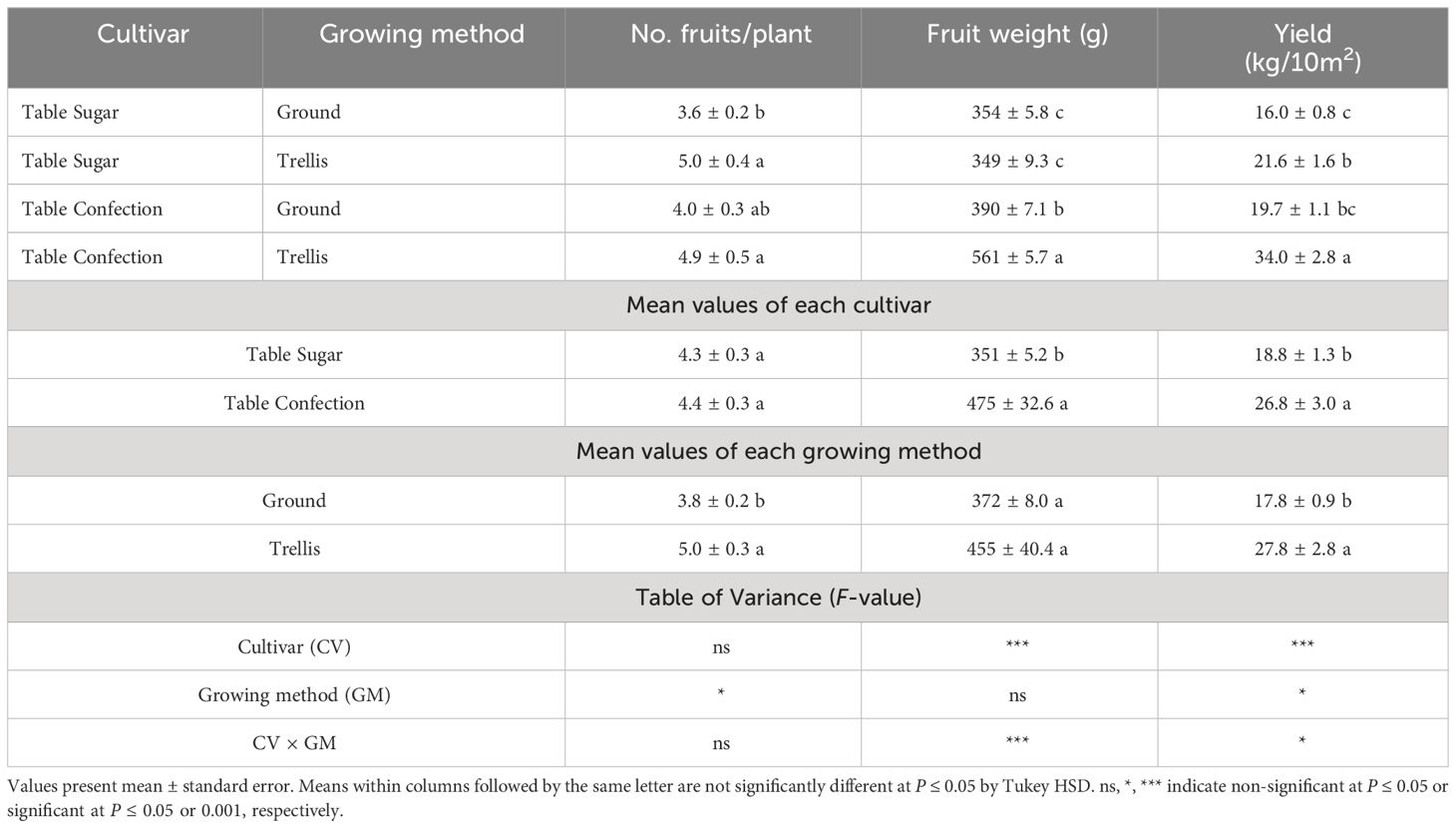
Table 2 Total yield and yield components from three harvests of two cultivars of sweet acorn squash grown on the ground or trellised.
Trellised plants of ‘Table Confection’ outyielded their ground-grown counterparts by 73% (Table 2). The greater yield of the trellised plants of this cultivar is attributable to both, larger fruit size and greater fruit number. The fruits of the trellis-grown plants averaged 171 g larger than their ground-grown counterparts. ‘Table Confection’ produced about as many fruits as ‘Table Sugar’ but they averaged 35% larger, thus resulting in greater yield.
On average, trellis-grown plants produced nearly one-third more fruits that were 22% larger, resulting in a yield increase of 56%, 1 kg more per square meter, than the ground-grown plants (Table 2).
3.2 Quality of fruits at harvest
At harvest, the fruits of trellis-grown plants were firmer and had higher DM and TSS contents than those of ground-grown plants (Table 3). Rind color, at harvest, did not differ significantly between the two growing methods. However, the entire surface of the fruits of trellis-grown plants was exposed to sunlight and hence did not have the characteristic orange “ground spot” of the ground-grown plants (Figure 2).
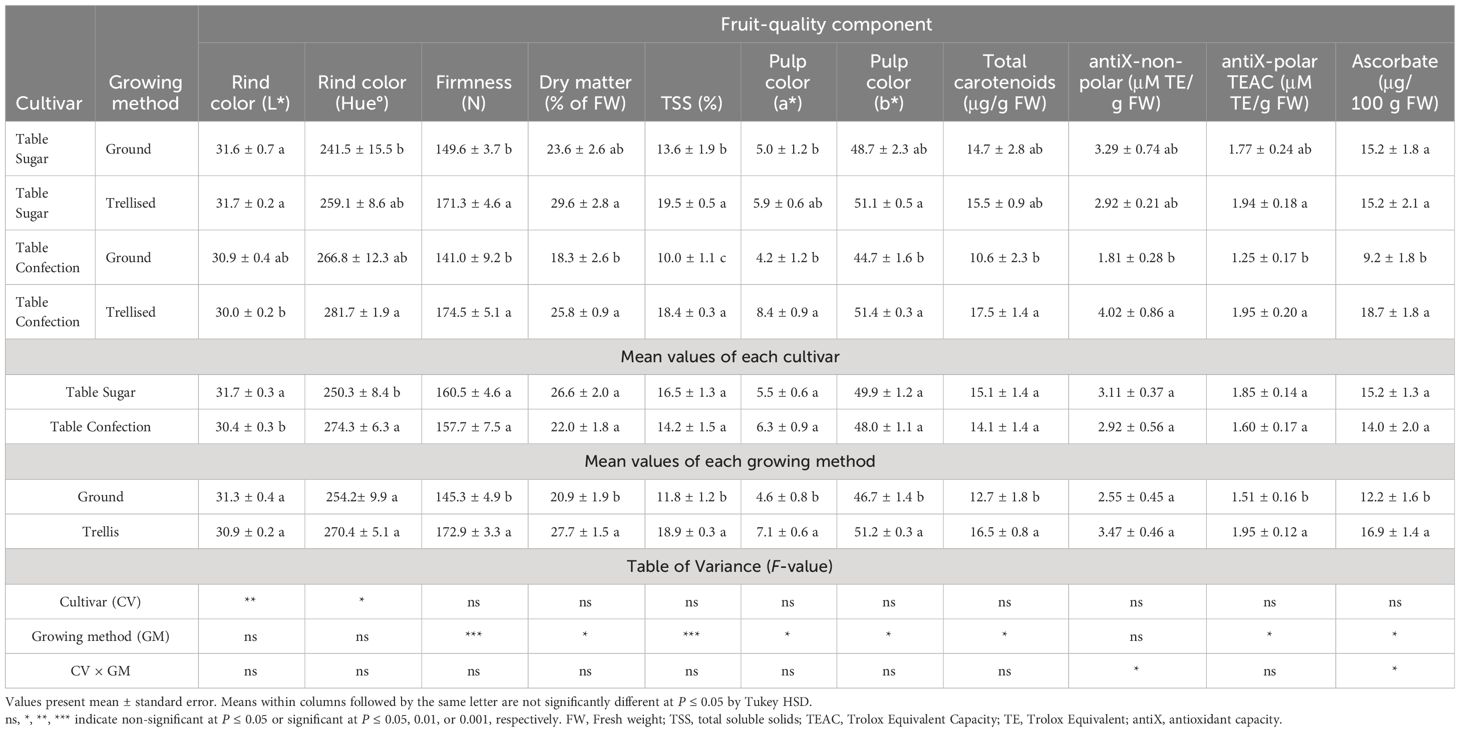
Table 3 Fruit-quality components at harvest of two cultivars of sweet acorn squash grown on the ground or trellised.
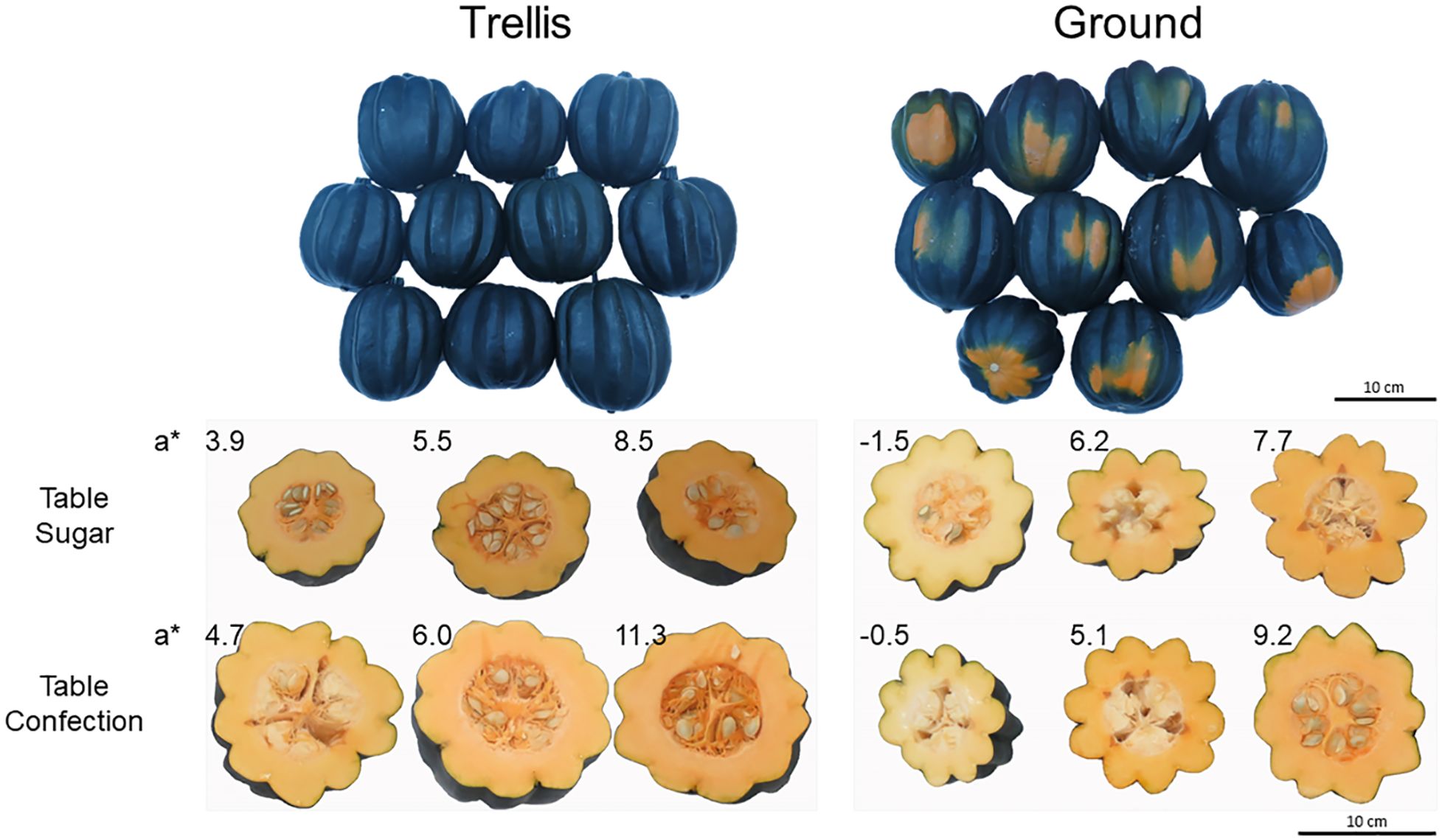
Figure 2 External and internal appearance of sweet acorn squash fruits at harvest. At left, fruits from vertically grown, trellised plants. At right, fruits from sprawling ground-grown plants. Top images indicate external appearance of whole fruits. Notice the fruits from trellised plants are entirely black-green while those from ground-grown plants are mostly black-green and partially orange; the orange areas result from lack of exposure to sunlight where the fruits were laying on the ground. Bottom images indicate internal appearance of fruits cut equatorially at harvest to show mesocarp color, labelled with corresponding a* values, for fruits of minimum, middle, and maximum values of a* for each of the 4 treatments.
Interestingly, for trellised plants, the fruit mesocarp exhibited higher a* and b* color-space values, that is, the fruit flesh was more intensely yellow (Table 3, Figure 2). The fruits of trellis-grown plants also had a significantly higher carotenoid content and a higher polar antioxidant capacity, specifically ascorbate, than the ground-grown fruits (Table 3).
The taste panel assessed the freshly harvested fruits as being moderately sweet to sweet, slightly fibrous, almost completely non-bitter, and moderately tasty to tasty (Figure 3A). Those of ‘Table Sugar’ tended to have higher scores for sweetness and taste than those of ‘Table Confection’. The trellis-grown fruits were significantly sweeter than ground-grown fruits.
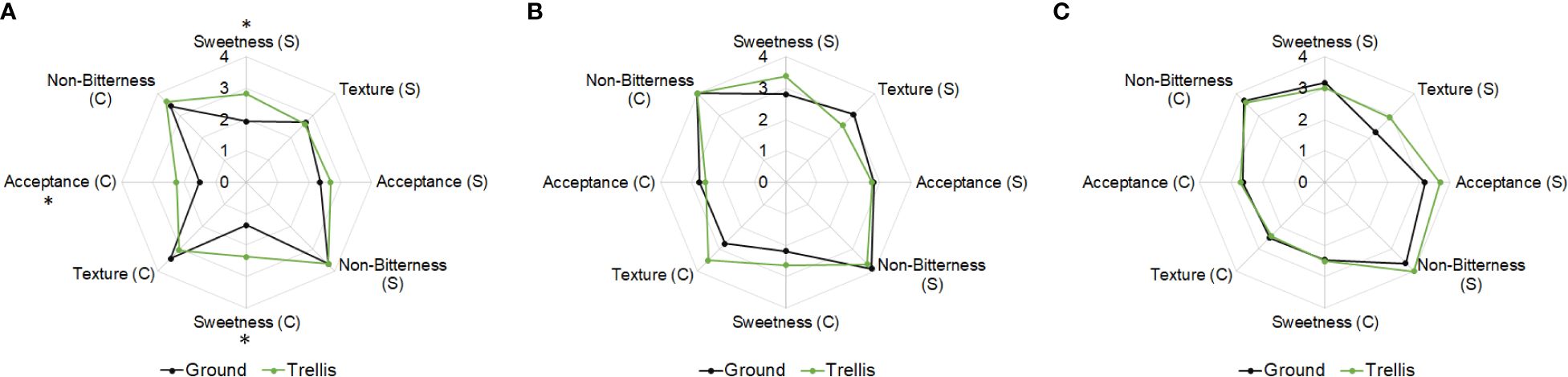
Figure 3 Sensory test of fruits of two cultivars of sweet acorn squash (S = ‘Table Sugar’; C = ‘Table Confection’) grown on the ground or trellised; (A) at harvest, (B) after storage at 15°C, RH 95%, and (C) after storage at 10°C, RH 70%. *Means significantly different based on Students’ t-test, P ≤ 0.05.
3.3 Quality of fruits postharvest
3.3.1 Storage at 15°C, 95% RH
After storage for 78 days at 15°C, 95% RH, the fruits of ‘Table Confection’ lost 6% and those of ‘Table Sugar’ lost 8% of their weight (Table 4). Ground-grown fruits lost more of their weight than trellis-grown fruits in this storage regime. L* values for rind color increased noticeably in this storage regime, while hue angle decreased (Tables 3, 4), meaning that the fruits lost some of their blackness, making them more greenish and less attractive. The fruits were nearly as firm as when freshly harvested, and those from trellised plants remained significantly firmer than those from ground-grown plants. The fruits had 2–3% less DM than when freshly harvested, but the trellis-grown plants retained significantly 6% more DM than ground-grown fruits. The stored fruits had higher %TSS than those freshly harvested, especially those from ground-grown plants. Nonetheless, %TSS was higher in trellis-grown plants. The a* values for mesocarp color increased appreciably after storage but did not differ significantly among the treatments (Table 4). Results from the taste panel assessments for sweetness, texture, non-bitterness, and acceptance between trellised and ground-grown fruits were small and non-significant (Figure 3B).
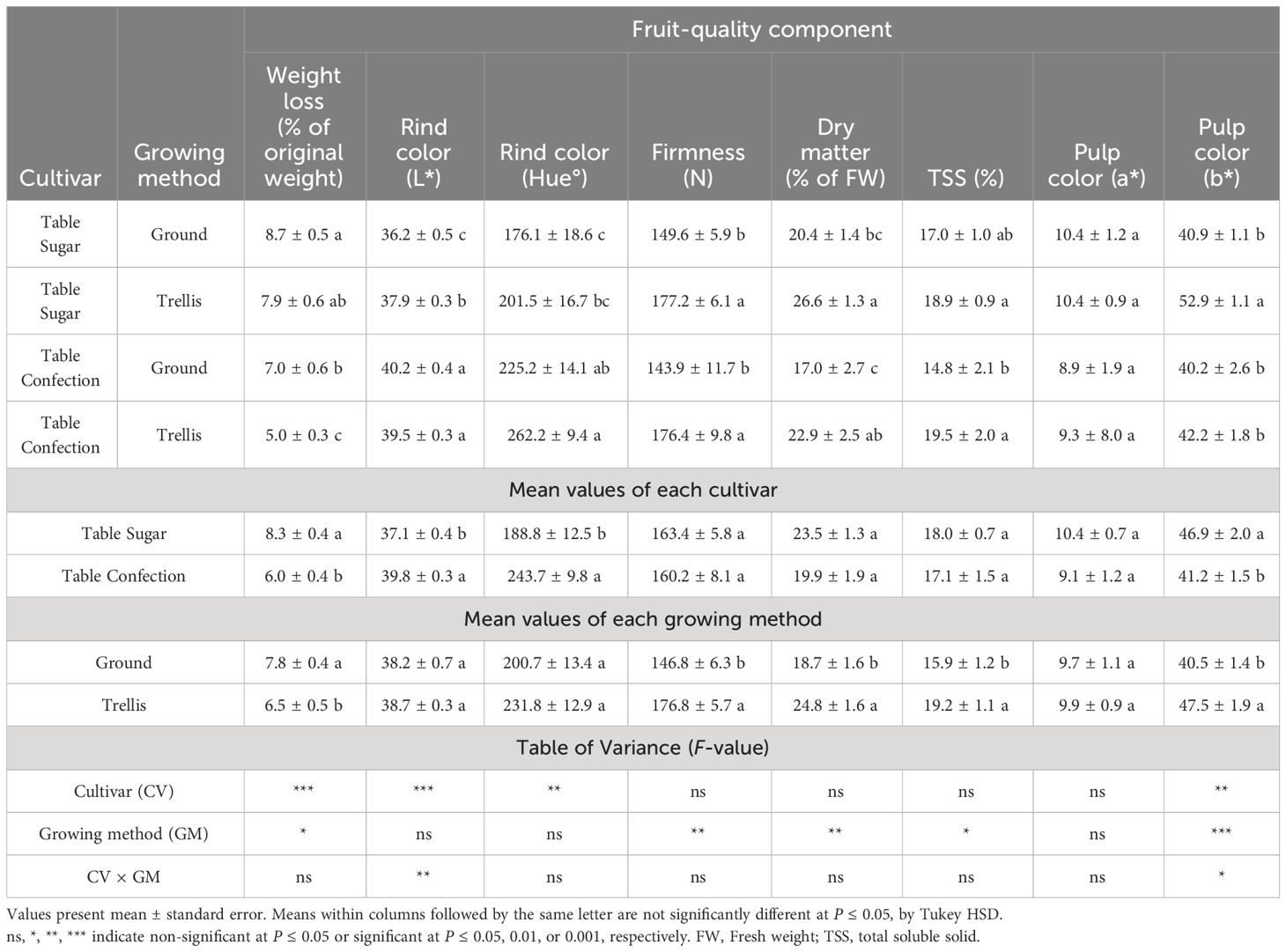
Table 4 Postharvest fruit-quality components of two cultivars of sweet acorn squash, grown on the ground or trellised, after 78 days of storage at 15°C and 95% relative humidity.
3.3.2 Storage at 10°C, 70% RH
After storage for 78 days at 10°C, 70% RH, the fruits of both cultivars lost approximately 11% of their weight regardless of whether they were trellised or not (Table 5). However, the blackness of the rind color was not compromised in this storage regime, as the L* values and hue angle were similar to those of freshly harvested fruits (Tables 3, 5). The fruits lost some of their firmness and %DM but, still, the fruits that developed on trellis-grown plants were significantly firmer and had significantly higher %DM than those that developed on ground-grown plants. The maximum DM was 26.6% in trellis-grown ‘Table Sugar’ fruits. The fruits of both cultivars showed increased TSS in this storage regime, the increase being more marked for ground-grown plants, which had averaged only 11.8% prior to storage but 14.7% after storage. The highest average TSS, 20.8%, was achieved in trellis-grown ‘Table Sugar’ fruits, with 18.2% in trellis-grown ‘Table Confection’ fruits. The a* values for mesocarp color noticeably increased in this storage regime too, again remaining significantly higher in trellis-grown fruits (Table 5).
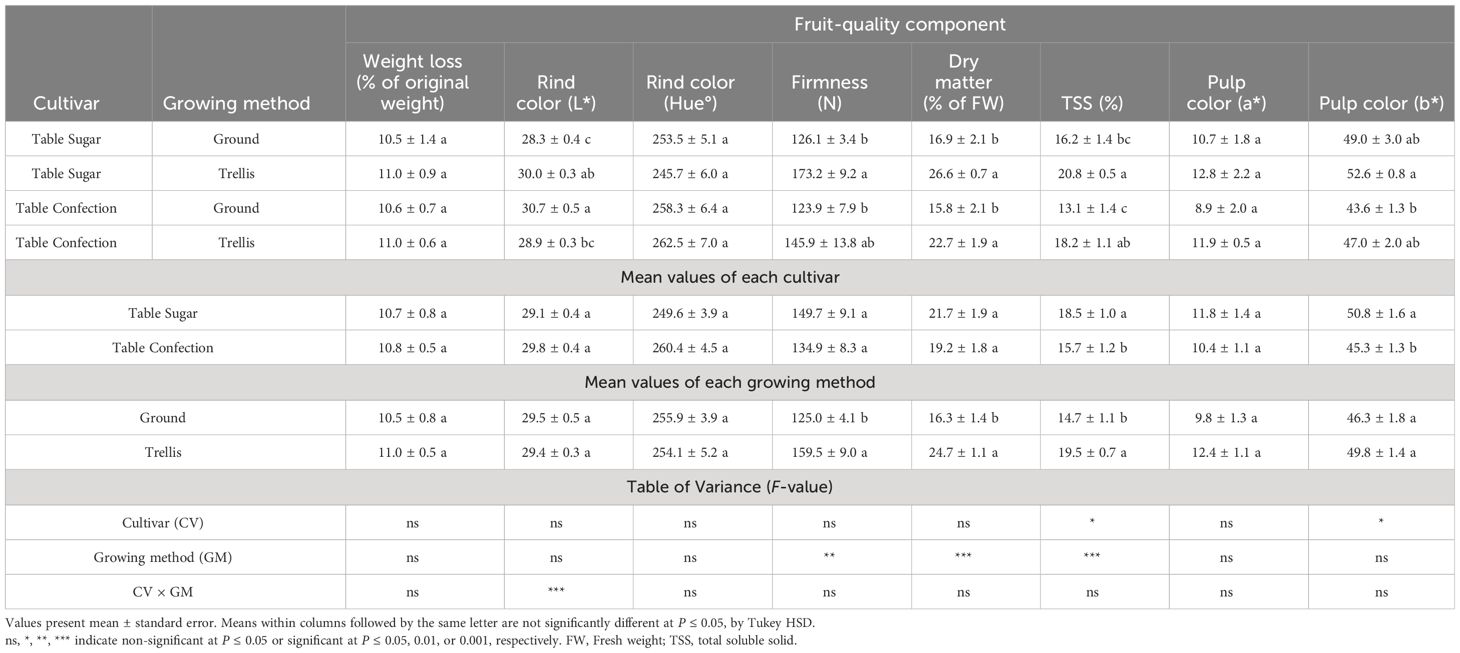
Table 5 Postharvest fruit-quality components of two cultivars of sweet acorn squash, grown on the ground or trellised, after 78 days of storage at 10°C and 70% relative humidity.
Results from the taste panel assessments for sweetness, texture, non-bitterness, and acceptance between trellised and ground-grown fruits were small and non-significant (Figure 3C). The taste panel assessed the stored fruits of ‘Table Sugar’ as being sweet to very sweet, moderately to slightly fibrous, almost completely non-bitter, and tasty to very tasty (Figure 3C). Those of ‘Table Confection’ were assessed as moderately sweet to sweet, slightly fibrous to smooth, almost completely non-bitter, and tasty.
4 Discussion
4.1 Yield
The results show a clear advantage in yield by growing sweet acorn squash vertically on trellises rather than sprawling on the ground. The yields of the two cultivars averaged 56% higher when grown on trellises (Table 2). The fruits of the trellised plants were uniformly black-green over their entire surface, without a light-colored ground spot (Figure 2). Similar advantages in yield and appearance of trellis-grown cucumbers as compared with ground-grown cucumbers have long been appreciated (Konsler and Strider, 1973; Hanna et al., 1987; Russo et al., 1991; Nair et al., 2013). Growing cucurbit plants vertically increases yield and improves fruit quality by increasing the exposure of the plant foliage to sunlight, thereby increasing photosynthesis, with increased ventilation and reduced exposure of the fruits to soil-borne pathogens and damage-causing ground-dwelling arthropods (Konsler and Strider, 1973; Pradhan et al., 2021; Buck-Sorlin et al., 2023). Trellising is today a widely commercially applied method for growing fresh-market cucumbers (Parker et al., 2019; Rutgers New Jersey Agricultural Experiment Station, 2022). However, we are not aware of commercial-scale, out-of-season trellised production of winter squash or pumpkins elsewhere. This is probably due to a combination of economic factors and the large plant size and rank, viney plant growth of other high-quality market types, which would make screenhouse or greenhouse trellising unwieldy. The internode length of buttercup, kabocha, and butternut squash is typically >15 cm. Acorn squash plants have an internode length of 9 cm, and the new bush cultivars, such as ‘Table Sugar’ and ‘Table Confection’, have a mature-plant internode length of only 3.5 cm, with the first 17 internodes having a length of 1 cm (Loy, 2012). Thus, of the high-quality market types of winter squash, the acorn squash would be expected to be the most feasible for trellising under protected conditions.
4.2 Quality of fruits at harvest
Percent DM and TSS of the fruit mesocarp are easily measured parameters that are closely reflective of starch and sugar contents (Culpepper and Moon, 1945; Nerson, 1995; Harvey et al., 1997; Loy, 2004, Loy, 2012). Contents of starch and especially sugars are primarily responsible for eating quality and consumer preference, positively affecting perceived smoothness of texture and sweetness, though color and flavor, which are partially species-specific, also have a role (Haber and Argue, 1927; Culpepper and Moon, 1945; Merrow and Hopp, 1961; Corrigan et al., 2001; Stevenson, 2003; Loy, 2004; Corrigan et al., 2006). Percent DM peaks from 30 to 40 days past anthesis, two to three weeks prior to fruit maturity, at which time %TSS is insufficient for good eating quality (Loy, 2004; Loy et al., 2004; Loy, 2006; Noseworthy and Loy, 2008). As the fruits approach maturity, at 50 or so days after anthesis, %DM tends to decrease but %TSS increases (Harvey et al., 1997; Loy, 2004). Buttercup- and kabocha-type fruits (Cucurbita maxima) have higher DM contents, 20–30% (Harvey et al., 1997), than butternut (C. moschata) and acorn squash (C. pepo) fruits, which range from 11–16% (Hultengren et al., 2016) and 12–20% (Loy, 2004), respectively. To have acceptable eating quality, acorn squash must have ≥16% DM at maturity (Loy, 2005), otherwise the product will have a texture that is objectionably fibrous with a thin, watery consistency (Loy, 2004). Although acorn squash are preferred over the other market types by many consumers because of their attractive appearance, conveniently small size and more pleasing flavor, their relatively low range for DM content provides limited buffering capacity for assuring smooth texture and acceptable eating quality (Loy, 2004). However, the mature fruits of some new cultivars and breeding germplasm of acorn squash have been shown to well exceed the 16% DM minimum requirement for consumer acceptability, attaining averages of 20–21% DM (Loy, 2006; Wyatt et al., 2016). The DM contents of the mature fruits harvested during the present experiments similarly averaged 21% from plants grown sprawling on the ground but attained an extraordinary average of 28% from those grown vertically on the trellises (Table 3).
A TSS content of at least 10% is required for acceptable eating quality of winter squash (Loy, 2005; Silva and Bruce, 2016). Among the three species, Cucurbita pepo, C. maxima, and C. moschata, the fruits of C. pepo tend to reach their maximum TSS first, from 45–55 days past anthesis. In buttercup and kabocha (C. maxima) and in butternut (C. moschata), the conversion of starch to sugar is slower. Fruits of the kabocha market type have a TSS content of 11–13% at harvest (Harvey et al., 1997; Loy, 2004) and butternut squash attain levels of 10–13% at harvest (Hultengren et al., 2016). Acorn squash often have 8–11% (Loy, 2005; Silva and Bruce, 2016) but the mature fruits of some new cultivars and breeding germplasm of acorn squash have been shown to attain averages of 12–14% TSS at harvest (Loy, 2006). Field-grown fruits of ‘Table Sugar’ average approximately 15% (Paris and Godinger, 2016). Under the protected conditions of the current experiment, ‘Table Sugar’ and ‘Table Confection’ averaged approximately 12% with ground culture and 19% with trellis culture (Table 3). As sensory quality is largely dependent on sugar content (Corrigan et al., 2000; Loy, 2004, Loy, 2012), it is not surprising that the freshly harvested trellis-grown squash, which had markedly higher TSS contents than the freshly harvested ground-grown squash, were given higher scores for perceived sweetness and overall acceptance by the panel of tasters (Figure 3).
Other components of consumer preference in pumpkins and winter squash include external and internal fruit colors (Schales and Isenberg, 1963; Murphy et al., 1966; Carbonell et al., 1990). There are many market types of pumpkins and winter squash having differing desired external color and color patterns (Ferriol and Pico, 2008). For acorn squash, dark green was the most widely accepted exterior color for many years but it was recently superseded by an even darker, black-green color, derived by introgression of the L-1 allele from zucchini squash (Paris and Nerson, 1986; Loy, 2012; Paris and Godinger, 2016). The green hue of the black-green cultivars is maintained by the fruits for a longer period of time after harvest than the regular dark green (Paris, 1997). Both, ‘Table Sugar’ and ‘Table Confection’, have a black-green exterior, except for the ground spot. Fruits that grew on the trellised plants did not show the ground spot and were entirely black-green (Figure 2).
For pumpkins and winter squash almost everywhere, intense color of the fruit flesh is highly desired by consumers (Carbonell et al., 1990; Nerson, 1995; Ferriol and Pico, 2008; Obrero et al., 2013). The fruits harvested from the trellised plants had a significantly more intense orange-yellow fruit-flesh color, measured in a* and b* values, than those harvested from the ground-grown plants (Table 3). Thus, for both exterior and interior color, the trellised plants provided a more desirable product.
The intensity of the yellow or orange color of the mesocarp is positively correlated with its total carotenoid content (Murphy et al., 1966; Kubicki and Walczak, 1976; Itle and Kabelka, 2009). Carotenoids can be divided into hydrocarbons, referred to as carotenes, and oxygenated compounds, referred to as xanthophylls (Maoka, 2020). The carotenes are vitamin A precursors while both they and the xanthophyll lutein function as anti-oxidants; lutein also slows progression of age-related macular eye disease (Eggersdorfer and Wyss, 2018). Cucurbita moschata has primarily beta-carotene and alpha-carotene while C. maxima and C. pepo have mostly lutein and beta-carotene (Azevedo-Moleiro and Rodriguez-Amaya, 2007; Wyatt et al., 2016; Reddy et al., 2023). Total carotenoid content in acorn squash varies among cultivars, ranging from 2 μg/g in ‘Fordhook Acorn’ and ‘Thelma Sanders’ to 14.7 μg/g in ‘Table Gold’ (Schaffer et al., 1986; Paris et al., 1989; Itle and Kabelka, 2009). The carotenoid contents for trellised ‘Table Sugar’ and ‘Table Confection’ (Table 3) exceed those that have been reported for other acorn squash.
The non-polar antioxidant capacity of the fruit pulp, which is mostly attributed to the carotenoids, was partially in agreement with the pulp-color a* and b* values (Table 3). Trellis-grown fruits of both cultivars showed significantly greater polar antioxidant capacity, including higher ascorbate content (Table 3). With their higher carotenoid and antioxidant contents, the trellis-grown squash were superior in nutritional quality to ground-grown squash.
4.3 Quality of fruits postharvest
During storage of winter squash, DM and starch contents decrease while TSS and sugar contents increase (Phillips, 1946; Holmes et al., 1954; Merrow and Hopp, 1961; Nerson, 1995; Harvey et al., 1997; Corrigan et al., 2001; Loy, 2004; Kami et al., 2011). Cultivars that are high in starch at the time of maturity become high in sugar during storage (Culpepper and Moon, 1945; Loy, 2004, Loy, 2006, Loy, 2012). After 30 or 60 days of storage at 15°C of mature, high-starch, kabocha-type fruits, the maximum TSS attained was 13.5% (Noseworthy and Loy, 2008). Fully mature butternut squash stored under the same conditions attained a maximum of 11.5% TSS. In a warm climate, butternut squash obtained a maximum of 12.2% after two months of storage and 12.8% TSS after four months of storage (Nerson, 1995). In contrast, for acorn squash, the increase in TSS during storage is faster (Noseworthy and Loy, 2008). If stored after harvest for three weeks, acorn squash can attain 10–11% TSS and the new, sweet-acorn germplasm and cultivars can attain 12–14% (Loy, 2004, Loy, 2006). After two months of cool-temperature storage, regular acorn squash achieved 12% and two new, sweet-acorn cultivars attained 14–15% TSS (Baggett and Kean, 1990). Presently, after 78 days of storage at 10°C or 15°C, the fruits of two sweet-acorn squash cultivars grown with ground culture averaged 15% TSS (Tables 4, 5) but with trellis culture the fruits averaged 19% TSS which, to our knowledge, is the highest value reported for any winter squash or pumpkin. No less striking was that the trellised fruits, even after 78 days of storage, averaged 25% DM (Tables 4, 5).
The fruits lost weight during storage. Over the 78 days of storage of sweet acorn squash, a weight loss of 7–8% occurred at 15°C, 95% RH while a weight loss of 10–11% occurred at 10°C, 70% RH, with trellis-grown fruits under the former conditions undergoing slightly less weight loss than their ground-grown counterparts (Tables 4, 5). Weight loss over the course of postharvest storage is due to respiration and desiccation (Phillips, 1946; Nerson, 1995; Harvey et al., 1997). Respiration, as measured by CO2 production and ethylene emission rates, was observed to be slow in sweet acorn squash and the differences between treatments were small and non-significant (Supplementary Table S1). Winter squash and pumpkins are considered to be non-climacteric and non-sensitive to ethylene (Paul et al., 2012; Michigan State University Extension, 2021). Desiccation tends to be greater in drier storage environments (Adeeko et al., 2020) and, indeed, the squash stored at 70% RH lost more weight than those stored at 95% RH (Tables 4, 5). High RHs though, can result in a greater incidence of fruit decay (Schales and Isenberg, 1963; Adeeko et al., 2020). Although the particular fruits that were sampled showed no signs of decay, some of the other stored fruits did show decay, the incidences being similar to those reported by Adeeko et al. (2021). There was approximately 25% incidence of decay at 95% RH but < 5% at 70% RH. Decay incidence, though, did not significantly differ between ground-grown and trellis-grown fruits (Supplementary Figure S2). Storage at 10°C 70% RH, with its very low incidence of fruit decay, would be preferred and is also more consistent with the recommendations for storage of pumpkins and winter squash indicated elsewhere, 10–16°C and 50–75% RH being considered optimal (Michigan State University Extension, 2021).
Storage can result in softening of fruits (Lara et al., 2019). The trellised acorn squash fruits were firmer than those from ground-grown plants in both storage regimes (Tables 4, 5). Fruit firmness has been observed to be positively correlated with starch content in winter squash (Stevenson, 2003) and, interestingly, we identified a positive correlation between firmness and %DW as well as between firmness and TSS (r2 = 0.58 and 0.52, respectively, with P ≤ 0.0001 for both) when considering all of the fruits sampled in this experiment (n = 60), but no significant correlation was identified between firmness and carotenoids or firmness and fruit weight.
4.4 Evolution and adaptation of acorn squash to out-of-season, vertical cultivation
The long-day, temperate-climate, field conditions at which acorn squash evolved under centuries of cultivation (Paris, 2000) are probably optimal for the development of high-quality fruits of this group of cultivars. In contrast, in the current experiment, the plants were grown under protection during the short days of autumn and winter of a Mediterranean climate, conditions to which this crop has not had long-standing adaptation. Nonetheless, the plants produced high-quality fruits. The trellised plants bore fruits of a quality, measured by DM and TSS contents, far superior to any reported elsewhere for acorn squash. Indeed, the TSS content of the fruit-flesh of trellised plants was, to our knowledge, higher than that reported anywhere for any kind of squash or pumpkin, the peak being an astounding 20.8% TSS for ‘Table Sugar’ after storage at 10°C, 70% RH (Table 5). Recently, growers in the Besor (southwestern) and the ‘Arava (southeastern) regions of Israel have begun applying trellising for growing protected, autumn-planted sweet-acorn squash, for winter production. Over the past two years, production of trellis-grown, winter-produced, sweet-acorn squash has increased markedly, and this product has become more easily available at specialty produce stands and high-end supermarkets during winter and early spring.
Yield of winter squash is determined by its photosynthetic capacity, the length of time needed for fruit development, and the efficiency with which photosynthates are directed and converted to fruit development (Loy, 2011). Growing methods can be altered so that more sunlight is absorbed by the plant but growers are presented with a paradox when it comes to choosing a variety because their profits are based on yields measured as fresh weight; hence, they will tend to choose a high-yielding cultivar. However, cultivars with good eating quality have flesh with high dry matter, meaning high in carbohydrates (starch and sugar), which are products of photosynthesis. Traditional acorn squash have approximately 10–14% dry matter in their fruit flesh while a sweet acorn squash would have nearly double that, meaning that the two cultivars produced an nearly amount in dry weight biomass but the traditional cultivar would have about double the yield of the sweet cultivar. Obviously, growers for wholesale markets will choose the higher yielding, mediocre quality, traditional acorn squash. And therein lies the conundrum of the traditional acorn squash: it is a nutritious, attractive, convenient size, easy-to-produce and inexpensive vegetable that requires the know-how, tools, time, and effort to prepare, and then often rewards the preparer with mediocre quality (Jones et al., 2021). The sweet acorn squash has solved that conundrum in Israel, becoming a recognized premium vegetable, and may have the potential to do so elsewhere. Combining breeding for utmost fruit quality and plant architecture adapted to vertical farming (trellising), with adoption of optimal postharvest storage conditions, has been a boon to production and marketing of this nutritious, high-quality produce item during the off-season. This approach has resulted in an added-value vegetable being widely produced in an economically viable manner, for the benefit of growers and consumers alike.
5 Conclusions
Trellised cultivation was advantageous to ground cultivation in yield and fruit production of winter-grown, sweet acorn squash. Fruit quality, as measured by firmness, external and internal color, dry matter and total soluble solids contents, was superior in fruits grown on trellised plants, and these advantages were maintained over a two-and-a-half month postharvest storage period. Nutritional quality, as measured by carotenoid, ascorbate, and anti-oxidant contents, was also superior in trellis-grown fruits. After storage at 10°C, 70% RH, fruits derived from trellised plants averaged 20% total soluble solids.
Data availability statement
The original contributions presented in the study are included in the article/Supplementary Material. Further inquiries can be directed to the corresponding author.
Author contributions
AA: Investigation, Writing – original draft. FY: Investigation, Writing – review & editing. GR: Investigation, Writing – review & editing. LA: Conceptualization, Funding acquisition, Investigation, Writing – review & editing. HA: Conceptualization, Funding acquisition, Investigation, Supervision, Writing – review & editing. MZP: Investigation, Writing – review & editing. SA-T: Investigation, Writing – review & editing. EF: Conceptualization, Supervision, Writing – original draft, Writing – review & editing. HP: Data curation, Writing – original draft, Writing – review & editing. CZ: Conceptualization, Funding acquisition, Investigation, Supervision, Writing – original draft, Writing – review & editing.
Funding
The author(s) declare financial support was received for the research, authorship, and/or publication of this article. This research was funded by the Vegetable Division, Israel Plant Production and Marketing Board (Yehud, Israel) for years 2018-2021.
Acknowledgments
We thank Origene Seeds (Giv‘at Brenner, Israel) for providing the seeds for the experiment.
Conflict of interest
The authors declare that the research was conducted in the absence of any commercial or financial relationships that could be construed as a potential conflict of interest.
Publisher’s note
All claims expressed in this article are solely those of the authors and do not necessarily represent those of their affiliated organizations, or those of the publisher, the editors and the reviewers. Any product that may be evaluated in this article, or claim that may be made by its manufacturer, is not guaranteed or endorsed by the publisher.
Supplementary material
The Supplementary Material for this article can be found online at: https://www.frontiersin.org/articles/10.3389/fhort.2024.1365147/full#supplementary-material
References
Adeeko A., Yudelevich F., Raphael G., Avraham L., Alon H., Zaaroor Presman M., et al. (2020). Quality and storability of trellised, greenhouse-grown, winter-harvested, new sweet acorn squash hybrids. Agronomy 10, 1443. doi: 10.3390/agronomy10091443
Adeeko A., Yudelevich F., Regev R., Avraham L., Alkalai-Tuvia S., Paris H. S., et al. (2021). The effect of postharvest treatments on produce losses during long-term storage of acorn squash. Acta Hortic. 1325, 159–166. doi: 10.17660/ActaHortic.2021.1325.24
Avraham L., Omer S., Alon H., Ben-Khalifa H., Ziv C., Fallik E., et al. (2018). Cultivar trial of trellised sweet acorn squash in 50-mesh screenhouses during the autumn. Mivzaq Yeraqot 320, 37–40.
Avraham L., Omer S., Alon H., Ben-Khalifa H., Ziv C., Fallik E., et al. (2020). Cultivar trial and agrotechniques for sweet acorn squash in 50-mesh screenhouses. Mivzaq Yeraqot 332, 46–50.
Azevedo-Moleiro C. H., Rodriguez-Amaya D. B. (2007). Qualitative and quantitative differences in carotenoid composition among Cucurbita moschata, Cucurbita maxima, and Cucurbita pepo. J. Agric. Food Chem. 55, 4037–4053. doi: 10.1021/jf063413d
Baggett J. R., Kean D. (1990). ‘Sugar Loaf’ and ‘Honey Boat’ winter squashes. HortScience 25, 369–370. doi: 10.21273/HORTSCI.25.3.369
Batziakas K. G., Jenkins T., Stanley H., Cunningham B. M., Kang Q., Rivard C. L., et al. (2022). Effect of high-tunnel production systems on the preharvest losses and harvest quality of ‘BHN 589’ and ‘Cherokee Purple’ tomatoes. HortTechnology 32, 507–509. doi: 10.21273/HORTTECH05082-22
Bonita-Noseworthy J., Loy J. B., Curran-Celentano J., Sideman R., Kopsell D. A. (2016). Carotenoid concentration and composition in winter squash: Variability associated with different cultigens, harvest maturities, and storage times. HortScience 51, 472–480. doi: 10.21273/HORTSCI.51.5.472
Buck-Sorlin G., Bournet P. E., Rossdeutsch L., Truffault V. (2023). Optimizing photosynthetic activity of high-wire cucumber production systems using a functional-structural plant modeling approach. Acta Hortic. 1377, 85–92. doi: 10.17660/ActaHortic.2023.1377.10
Cantliffe D. J., Vansickle J. J. (2001). Competitiveness of the Spanish and Dutch greenhouse industries with the Florida fresh vegetable industry. Proc. Fla. State Hortic. Soc. 114, 283–287.
Carbonell M. E., Wessel-Beaver L., Varela F., Luciano B. (1990). Pumpkin (Cucurbita moschata) breeding priorities based on a survey among Puerto Rican consumers. J. Agric. Univ. Puerto Rico 74, 229–236.
Chalupowicz D., Alkalai-Tuvia S., Zaaroor-Presman M., Fallik E. (2018). The potential use of hot water rinsing and brushing technology to extend storability and shelf life of sweet acorn squash (Cucurbita pepo L.). Horticulturae 4, 19. doi: 10.3390/horticulturae4030019
Chayut N., Yuan H., Ohali S., Meir A., Sa’ar U., Tzuri G., et al. (2017). Distinct mechanisms of the ORANGE protein in controlling carotenoid flux. Plant Physiol. 173, 376–389. doi: 10.1104/pp.16.01256
Cook R., Calvin L. (2005). Greenhouse tomatoes change the dynamics of the North American fresh tomato industry. (USDA Economic Research Report). Number 2.
Corrigan V. K., Hedderley D. I., Hurst P. L. (2006). Assessment of objective texture measurements for characterising and predicting the sensory quality of squash (Cucurbita maxima). New Z. J. Crop Hortic. Sci. 34, 369–379. doi: 10.1080/01140671.2006.9514428
Corrigan V. K., Hurst P. L., Potter J. F. (2001). Winter squash (Cucurbita maxima) texture: Sensory, chemical, and physical measures. New Z. J. Crop Hortic. Sci. 29, 111–124. doi: 10.1080/01140671.2001.9514169
Corrigan V. K., Irving D. E., Potter J. F. (2000). Sugars and sweetness in buttercup squash. Food Qual. Pref. 11, 313–322. doi: 10.1016/S0950-3293(99)00077-4
Culpepper C. W., Moon H. H. (1945). Differences in the composition of the fruits of Cucurbita varieties at different ages in relation to culinary use. J. Agric. Res. 71, 111–136.
Eggersdorfer M., Wyss A. (2018). Carotenoids in human nutrition and health. Arch. Biochem. Biophys. 652, 18–26. doi: 10.1016/j.abb.2018.06.001
Ferriol M., Pico B. (2008). “Pumpkin and winter squash” in Handbook of plant breeding, vegetables I. Eds. Prohens J., Nuez F. (Springer Science+Business Media), 317–349.
Haber E. S., Argue C. W. (1927). The chemical composition of the Des Moines (Table Queen) squash as affected by the age of the specimen. Am. Soc Hortic. Sci. 23, 203–207.
Hanna H. Y., Adams A. J., Story R. N. (1987). Increased yield in slicing cucumbers with vertical training of plants and reduced plant spacing. HortScience 22, 32–34. doi: 10.21273/HORTSCI.22.1.32
Harvey W. J., Grant D. G., Lammerink J. P. (1997). Physical and sensory changes during the development and storage of buttercup squash. New Z. J. Crop Hortic. Sci. 25, 341–351. doi: 10.1080/01140671.1997.9514025
Holmes A. D., Spelman A. F., Wetherbee R. T. (1954). Effect of storage on butternut squash and its seeds. J. Am. Dietet. Assoc. 30, 138–141. doi: 10.1016/S0002-8223(21)29117-7
Hultengren R. L., Wyatt L., Mazourek M. (2016). A suite of high-quality butternut squash. HortScience 51, 1435–1437. doi: 10.21273/HORTSCI10987-16
Itle R. A., Kabelka E. A. (2009). Correlation between L*a*b* color space values and carotenoid content in pumpkins and squash (Cucurbita spp.). HortScience 44, 633–637. doi: 10.21273/HORTSCI.44.3.633
Jagota S. K., Dani H. M. (1982). A new calorimetric technique for the estimation of vitamin C using folin phenol reagent. Analyt. Biochem. 127, 178–182. doi: 10.1016/0003-2697(82)90162-2
Janick J., Paris H. S. (2022). History of controlled environment horticulture: Ancient origins. HortScience 57, 236–238. doi: 10.21273/HORTSCI16169-21
Jones J. C., Christaldi J., Castellanos D. C. (2021). The acorn squash problem: a digestible conceptualisation of barriers to emergency food assistance. Public Health Nutr. 25, 1045–1049. doi: 10.1017/S1368980021003748
Jovicich E., Cantliffe D. J., Stoffella P. J. (2004). Fruit yield and quality of greenhouse-grown bell pepper as influenced by density, container, and trellis system. HortTechnology 14, 507–509. doi: 10.21273/HORTTECH.14.4.0507
Kami D., Muro T., Sugiyama K. (2011). Changes in starch and soluble sugar concentrations in winter squash mesocarp during storage at different temperatures. Scient. Hortic. 127, 444–446. doi: 10.1016/j.scienta.2010.10.025
Kim S. R., Lane R. H., Abdel‐Ghany M., Stitt K. R. (1987). Influence of extractant on L-ascorbic acid recovery from selected foods and beverages. J. Food Qual 10, 1–7. doi: 10.1111/j.1745-4557.1987.tb00284.x
Konsler T. R., Strider D. L. (1973). The response of cucumber to trellis vs. ground culture. HortScience 8, 220–221. doi: 10.21273/HORTSCI.8.3.220
Kubicki B., Walczak B. (1976). Variation and heritability of beta-carotene content in some cultivars of the Cucurbita species. Genet. Polon 17, 531–544.
Lara I., Heredia A., Dominguez E. (2019). Shelf life potential and the fruit cuticle: the unexpected player. Front. Plant Sci. 10. doi: 10.3389/fpls.2019.00770
Loy J. B. (2004). Morpho-physiological aspects of productivity and quality in squash and pumpkins (Cucurbita spp.). Crit. Rev. Plant Sci. 23, 337–363. doi: 10.1080/07352680490490733
Loy J. B. (2005). Improving eating quality and storage life of acorn squash. HortScience 40, 1099. doi: 10.21273/HORTSCI.40.4.1099B
Loy J. B. (2006). “Harvest period and storage affect biomass partitioning and attributes of eating quality in acorn squash (Cucurbita pepo)” in Proceedings of Cucurbitaceae 2006. Ed. Holmes G. J. (Universal Press, Raleigh, NC), 568–577.
Loy J. B. (2011). Maximizing yield and eating quality in winter squash – a grower’s paradox. Available online at: www.hort.cornell.edu/expo/proceedings/2011/Vine%20Crops/Maximizing%20Yield%20and%20Eating%20Quality%20in%20Winter%20Squash%2011.pdf.
Loy J. B. (2012). “Breeding squash and pumpkins” in Genetics, genomics and breeding of cucurbits. Eds. Wang Y.-H., Behera T. K., Kole C. (CRC Press, Boca Raton), 93–139. Available at: doi: 10.1201/b11436-5
Loy J. B., Clark S., Xiao Q., Loy R. (2004). Physiological changes in acorn squash during development and storage as related to eating quality. HortScience 39, 460–461.
Maoka A. (2020). Carotenoids as natural functional pigments. J. Natural Medic 74, 1–16. doi: 10.1007/s11418-019-01364-x
Merrow S. B., Hopp R. J. (1961). Associations between the sugar and starch content and the degree of preference for winter squashes. J. Agric. Food Chem. 9, 321–326. doi: 10.1021/jf60116a024
Michigan State University Extension. (2021). Food preservation. All fruits and vegetables are not created equal when it comes to proper storage conditions. Available online at: https://www.canr.msu.edu/news/all-fruit-and-vegetables-are-not-created-equal-when-it-comes-to-proper-storage-conditions.
Mitchell C. A. (2022). History of controlled environment horticulture: Indoor farming and its key technologies. HortScience 57, 247–256. doi: 10.21273/HORTSCI16159-21
Murphy E. F., Hepler P. R., True R. H. (1966). An evaluation of the sensory qualities of inbred lines of squash (Cucurbita maxima). Proc. Am. Soc Hortic. Sci. 89, 483–490.
Nair A., Carpenter B. H., Weieneth L. K. (2013). “Effect of plastic mulch and trellises on cucumber production in high tunnels,” in Iowa State Research Farm Progress Report. Available at: http://lib.dr.iastate.edu/farms_reports/1909.
Nemali K. (2022). History of controlled environment horticulture: Greenhouses. HortScience 57, 239–246. doi: 10.21273/HORTSCI16160-21
Nerson H. (1995). Yield, quality and shelf-life of winter squash harvested at different fruit ages. Adv. Hortic. Sci. 9, 106–111.
Noseworthy J., Loy J. B. (2008). “Improving eating quality and carotenoid content of squash” in Proceedings of Cucurbitaceae 2008. Ed. Pitrat M. (I. N. R. A., Avignon), 521–528.
Obrero A., Gonzalez-Verdejo C. I., Die J. V., Gomez P., Del Rio-Celestino M., Roman B. (2013). Carotenogenic gene expression and carotenoid accumulation in three varieties of Cucurbita pepo during fruit development. J. Agric. Food Chem. 61, 6393–6403. doi: 10.1021/jf4004576
Paris H. S. (1986). A proposed subspecific classification for Cucurbita pepo. Phytologia 61, 133–138.
Paris H. S. (1997). Genes for developmental fruit coloration of acorn squash. J. Hered 88, 52–56. doi: 10.1093/oxfordjournals.jhered.a023056
Paris H. S. (2000). History of the cultivar‐groups of Cucurbita pepo. Hortic. Rev. 25, 71–170. doi: 10.1002/9780470650783.ch2
Paris H. S., Godinger D. (2016). Sweet acorn squash, a new vegetable on the Israeli market. Acta Hortic. 1127, 451–456. doi: 10.17660/ActaHortic.2016.1127.70
Paris H. S., Nerson H. (1986). Genes for intense fruit pigmentation of squash. J. Hered 77, 403–409. doi: 10.1093/oxfordjournals.jhered.a110270
Paris H. S., Schaffer A. A., Ascarelli I. M., Burger Y. (1989). Heterozygosity of gene B and the carotenoid content of Cucurbita pepo. Crop Res. 29, 11–18.
Parker J. B., James L., Parks S., Tesoriero L., Ryland A., Ekman J., et al. (2019). Greenhouse cucumber production (New South Wales: National Vegetable Extension Network). 224 pp.
Paul V., Pandey R., Srivastava G. C. (2012). The fading distinctions between classical patterns of ripening in climacteric and non-climacteric fruit and the ubiquity of ethylene—An overview. J. Food Sci. Technol. 49, 1–21. doi: 10.1007/s13197-011-0293-4
Phillips T. G. (1946). Changes in the composition of squash during storage. Plant Physiol. 21, 533–541. doi: 10.1104/pp.21.4.533
Pradhan R., Nayak D. A., Rao K. M., Mohapatra P. (2021). A critical review on effect of trailing and staking on growth and yield of cucurbitaceous crops. J. Pharmacognosy Phytochem. 10, 2151–2154.
Reddy U. K., Lopez-Ortiz C., Talavera-Caro A. C., Natarajan P., Tomason Y., Alaparthi S., et al. (2023). GWAS resolves molecular mechanisms underlying natural variation for carotenoids in Cucurbita maxima Duchesne. Scient. Hortic. 312, 111881. doi: 10.1016/j.scienta.2023.111881
Russo V. M., Roberts B. W., Schatzer R. J. (1991). Feasibility of trellised cucumber production. HortScience 26, 1156–1158. doi: 10.21273/HORTSCI.26.9.1156
Rutgers New Jersey Agricultural Experiment Station (2022). 2022/2023 Mid-Atlantic commercial vegetable production recommendations (New Brunswick: Rutgers—State University of New Jersey).
Schaffer A. A., Paris H. S., Ascarelli I. M. (1986). Carotenoid and starch content of near-isogenic B+B+ and BB genotypes of Cucurbita. J. Am. Soc Hortic. Sci. 111, 780–783. doi: 10.21273/JASHS.111.5.780
Schales F. D., Isenberg F. M. (1963). The effect of curing and storage on chemical composition and taste acceptability of winter squash. Proc. Am. Soc Hortic. Sci. 83, 667–674.
Silva E., Bruce D. (2016). Variety trial report: 2016 northern organic vegetable improvement collaborative. Organic acorn and delicata winter squash (University of Wisconsin-Madison Organic Research and Extension Program).
Stevenson D. G. (2003). Role of starch structure in texture of winter squash (Cucurbita maxima D.) fruit and starch functional properties. Iowa State University, Ames.
Tadmor Y., Paris H. S., Meir A., Schaffer A. A., Lewinsohn E. (2005). Dual role of the pigmentation gene B in affecting carotenoid and vitamin E content in squash (Cucurbita pepo) mesocarp. J. Agric. Food Chem. 53, 9759–9763. doi: 10.1021/jf0520591
United States Department of Agriculture (2008). Objective description of variety Pumpkin/Squash/Gourd (Cucurbita pepo L.), Exhibit C. (Beltsville, MD: Plant Variety Protection Office, Agricultural Marketing Service, Science and Technology).
Vinokur Y., Rodov V. (2006). Method for determining total (hydrophilic and lipophilic) radical scavenging activity in the same sample of fresh produce. Acta Hortic. 709, 53–60. doi: 10.17660/ActaHortic.2006.709.6
Wehner T. C., Naegele R. P., Myers J. R., Dhillon N. P. S., Crosby K. (2020). Cucurbits. 2nd edition (Wallingford: CABI). doi: 10.1079/9781786392916.0000
Wyatt L. E., Strickler S. R., Mueller L. A., Mazourek M. (2016). Comparative analysis of Cucurbita pepo metabolism throughout fruit development in acorn squash and oilseed pumpkin. Hortic. Res. 3, 16045. doi: 10.1038/hortres.2016.45
Keywords: Cucurbitaceae, protected cultivation, fruit yield and quality, fruit nutritional value, vertical farming, screenhouse, vegetable production, postharvest storage
Citation: Adeeko A, Yudelevich F, Raphael G, Avraham L, Alon H, Zaaroor Presman M, Alkalai-Tuvia S, Fallik E, Paris HS and Ziv C (2024) Trellising is advantageous over ground culture for out-of-season, protected production and storage of sweet acorn squash. Front. Hortic. 3:1365147. doi: 10.3389/fhort.2024.1365147
Received: 03 January 2024; Accepted: 22 April 2024;
Published: 17 May 2024.
Edited by:
Marwa Moumni, Marche Polytechnic University, ItalyReviewed by:
María Serrano, Miguel Hernández University of Elche, SpainXiaomeng Guo, Hezhou University, China
Copyright © 2024 Adeeko, Yudelevich, Raphael, Avraham, Alon, Zaaroor Presman, Alkalai-Tuvia, Fallik, Paris and Ziv. This is an open-access article distributed under the terms of the Creative Commons Attribution License (CC BY). The use, distribution or reproduction in other forums is permitted, provided the original author(s) and the copyright owner(s) are credited and that the original publication in this journal is cited, in accordance with accepted academic practice. No use, distribution or reproduction is permitted which does not comply with these terms.
*Correspondence: Carmit Ziv, Q2FybWl0LlppdkBhZ3JpLmdvdi5pbA==