- United States Department of Agriculture, Agricultural Research Service, Sugarbeet and Potato Research Unit, Edward T. Schafer Agricultural Research Center, Fargo, ND, United States
Introduction: Wounding of potato (Solanum tuberosum L.) tubers affects the postharvest storage qualities and marketability. To mitigate wound-related losses, it is necessary to accelerate wound healing (WH) responses of potato tubers. While role of nitric oxide (NO) in healing of wounded biological tissues is well known, its impact on WH responses of potato tubers has not been examined thoroughly. The aim of this study was to elucidate the potential modulatory role of NO in WH response of potato tubers by determining the activity of key enzymes associated with NO biosynthesis.
Methods: Using a model wounding system, tuber tissue discs were extracted from mini tubers of cv. Russet Burbank to examine their WH responses. Tuber discs were treated with NO related chemicals, such as NO scavenger, nitric oxide synthase (NOS) inhibitor, and NO donors. The effect of these treatments on in vitro production of NO, activity of nitrate reductase, NOS, and phenylalanine ammonia-lyase were determined. The accumulation of suberin polyphenolics (SPP) in wounded parenchyma cells, an indicator of early suberization process, was analyzed histologically.
Results: Treatment of tuber tissues with the higher dose of an NO scavenger (2-phenyl-4,4,5,5-tetramethylimidazoline-1-oxyl-3-oxide) at the time of wounding completely ceased SPP accumulation between 0 h to 3 d after wounding. Increased production of NO and enhanced activity of NOS were also observed immediately after wounding of the tuber tissues. The results suggest that NO is a key biological modulator in WH responses of potato tubers and could be optimized as a potential exogenous treatment to counter wound-related losses.
1 Introduction
Wound-related damage to potato tubers can cause significant postharvest losses (estimated ≥ $300 million U.S. dollars per year) affecting the potato industry (Thornton and Bohl, 1995). While there are several postharvest challenges that can negatively impact the long-term storage of potato tubers (e.g., premature sprout growth, pressure bruise, storage diseases), wounding during harvest and postharvest operations severely affects tuber quality and its marketability. Additionally, when potato seed tubers are cut for planting, rapid wound healing (WH) of cut surfaces of seed pieces is critical for overall crop yield and performance. The potato industry is in need of finding benign and practical strategies to address these wound-related challenges. In this context, determining the regulatory mechanisms associated with WH responses of potato tubers is essential. Although there have been advancements in our understanding of the physiological mechanisms and regulation of WH processes of potato tubers (Lulai et al., 2008; Lulai et al., 2016), further research is needed for development of new technologies to accelerate WH of cut or wounded tubers.
Exogenous application of nitric oxide (NO) has gained interest in agriculture and biotechnology due to its putative role in diverse developmental and physiological processes of plants. NO is an endogenous signaling molecule known to play distinct roles in the formation and differentiation of xylem (xylogenesis) (Neill, 2005), plant senescence and cellular apoptosis (Hussain et al., 2022), flower development (Senthil Kumar et al., 2016), stomatal movement (Sun et al., 2019), breaking dormancy and promoting germination (Signorelli and Considine, 2018), maintaining cellular redox homeostasis (Kolupaev et al., 2023), and providing protection against several biological and abiotic stresses (Shi et al., 2012; Wani et al., 2021). The role of NO in plant stress adaptation, especially crosstalk with phytohormones, interaction with reactive oxygen and nitrogen species, modulation of stress-related gene expression and protein activity have been investigated in different plant models (Sanz et al., 2015; Simontacchi et al., 2015).
Wounding is a form of stress, and most plant species have an intricate defense system and response mechanism to counter and repair wound related tissue damages, and NO is a known modulator in plants’ response to wounding (Yin et al., 2012; Marvasi, 2017). Previous research has implicated enhanced deposition of glucan-callose (a polysaccharide in plant cell walls which provides protection against pathogens) in wounded potato leaflets treated with an NO donor sodium nitroprusside (SNP) (París et al., 2007). The same study also found an increase of the extensin and phenylalanine ammonia-lyase (PAL) transcript levels in wounded leaflets with SNP treatment (París et al., 2007). Induction of a phytoalexin (i.e., rishitin) was observed in potato tuber tissues with treatment of an NO releasing compound (i.e., NOC-18) (Noritake et al., 1996). Exogenous application of NO donors (i.e., SNP and S-nitroso-N-acetylpenicillamine-SNAP) showed enhanced protection against oxidative stress associated ion leakage in Diquat treated potato leaflets (Beligni and Lamattina, 2002). Similarly, partial reversal of chlorophyll formation was reported in Phytophthora infestans infected potato leaves when treated with SNP (Lazalt et al., 1997). These studies indicated that NO plays a modulatory role in wound-induced physiological responses in various potato plant tissues and might provide protection against infection and herbicide damages. However, the regulatory role of NO or NO- related biology on potato tuber WH has not been thoroughly investigated.
In plant cells, NO is mostly generated from L-arginine through the activity of nitric oxide synthase (NOS) enzymes or can be produced from nitrite (NO2-) by activity of nitrate reductase (NR) (Neill et al., 2008; Simontacchi et al., 2015). Analogues of arginine such as NG-nitro-L-arginine methyl ester (L-NAME) and NG-monomethyl-L-arginine acetate (L-NMMA), which are known to work as competitive inhibitors of NOS were previously used to investigate the generation of NO in plant cells and its biological function in plant development (Tamás et al., 2018; Krasylenko et al., 2019). Similarly, 2-4-carboxyphenyl-4,4,5,5-tetramethylimidazoline-1-oxyl-3-oxide (cPTIO) and 2-phenyl-4,4,5,5-tetramethylimidazoline-1-oxyl-3-oxide (PTIO), two NO scavengers, have been widely used to study the role of NO in stress responses or in plants developmental process (D’Alessandro et al., 2013; Gupta et al., 2020).
Previous research also highlighted the importance of suberin polyphenolic (SPP) formation in the outer layer of wounded cells as an immediate physiological response of potato tubers to wounding, particularly during the critical WH phase of 0-8 d (Lulai et al., 2016). Along with suberin polyaliphatics (SPA), SPP accumulation in wounded surfaces is part of the closing layer formation that provides initial protection to wounded potato tuber tissues (Lulai and Corsini, 1998). Formation of SPP and SPA in wounded surface during closing layer formation is closely associated with phytohormone regulation and key metabolic changes associated with plant’s stress responses (Lulai et al., 2016). Accelerated accumulation of SPP and lignin and enhanced activity of PAL were previously observed in wounded potato tissues (Zhu et al., 2022). Up-regulation of the phenylpropanoid pathway (PAL catalyzes the first step of phenylpropanoid biosynthesis) is a common response of plant cells to wounding and is related to formation of SPP and other WH responses (Yin et al., 2012). Therefore, determination of PAL activity at early stages of closing layer formation could provide an indication on biosynthesis and accumulation of SPP during WH.
Here, we investigated the potential modulatory roles of NO in WH responses by utilizing NO related chemicals to enhance or inhibit its abundance in mechanically wounded tuber tissues. To determine the response of enzymes involved in NO biosynthesis in wounded potato tuber tissues, NO- donors, scavengers, and enzyme inhibitors were used. The changes in WH responses due to these treatments were also determined using histological observation of SPP accumulation at early stages of closing layer formation (0-3 d after wounding) in potato tuber tissues. Outcomes of this research provide insights into the role of NO in potato WH processes and could lead to novel management strategies for mitigating wound-related losses of potato tubers.
2 Materials and methods
2.1 Plant material and sampling process
Certified seed mini tubers of cv. Russet Burbank were used for the experiment. The mini tubers were obtained from a commercial grower (Valley Tissue Culture, Halstad, MN, USA), and stored in the dark at 4°C and ~95% relative humidity (RH). Potato tubers were washed with distilled water 48 h prior to the wounding treatment and equilibrated at 20°C and ~95% RH. A tuber disc model system as described by Lulai et al. (2008) was adapted to study the WH responses of potato tubers. In brief, a cork borer was used to laterally excise a cylinder (11 mm diameter) of tuber flesh tissues, and discs of parenchyma tissues were cut using a fixed width blade (3 mm thick).
Directly after wounding, tuber discs were treated with different NO related chemicals dissolved in 0.01 M MES (2-(N-morpholino) ethanesulfonic acid) buffer, and MES was used as the control treatment. Different concentrations (0.5, 1, 2, and 5 mM) of NO scavenger (PTIO), NOS inhibitor (5, 25, 50, 100, and 200 mM L-NAME), and NO donors (0.5, 1, 5 mM SNP and SNAP) alone or in combinations were used to determine dose dependent responses of NO-associated changes during tuber WH. Tuber discs were placed in the respective treatment solutions (20 discs in 100 mL) and allowed to imbibe for 1 h on a rotary shaker (50 cycles min-1). Treatment solutions were replaced with fresh solution every 20 min. Then, treated tuber discs were transferred and kept as a single layer on a non-reacting mesh surface in Pyrex trays and allowed to wound-heal in the dark at 20°C and ~95% RH. A subset of discs was snap frozen at 0 h and remaining discs were collected at different time points (2 h, 4 h, 6 h, 12 h, 1 d, 3 d, 5 d, and 7 d) for determination of NO content and in vitro enzyme analysis.
2.2 Histological determination of suberin polyphenolics accumulation
A subset of discs (4 discs for each biological replicate) from 0 and 3 d after the treatments were also placed in Farmer’s fixative (absolute ethanol: acetic acid in 3:1 v/v) for microscopical analysis of SPP. The 3 d time point was chosen to determine the impact of experimental treatments on WH responses of potato tubers, because SPP is known to accumulate at the first cell layer of wounded surface within 3 d of WH process (Lulai and Corsini, 1998; Lulai et al., 2008). A microscope (Zeiss Axioscope) configured with fluorescent illumination was used to determine the suberization rating based on the SPP accumulation at the wound-healed surface of the tuber discs. The suberization rating system developed by Lulai and Corsini (1998) was employed which indicated 0= none; 3.0= contiguous accumulation of SPP on outer tangential layer of first cell, and 5= complete accumulation of SPP around first cell layer.
2.3 Determination of in vitro production of nitric oxide
NO concentrations of treated tuber discs were determined using Arbor Assay kits (Arbor Assays LLC, Ann Arbor, MI, USA) based on colorimetric method. Two stable break-down products, nitrate and nitrite, were measured quantitively to determine total NO content in the tuber tissues. For this assay, cryogenically ground potato tuber samples were extracted using distilled water and centrifuged 2 times at 10,000 rpm for 15 min. Collected supernatant was stored at -20°C prior to carrying out the assay following the manufacturer’s protocol. In brief, 50 µL of sample was added to a single well of a 96-well plate and mixed with 50 µL assay buffer. Then 10 µL of nicotinamide adenine dinucleotide hydride (NADH) and 10 µL of nitrate reductase (NR) were added in the wells and incubated at room temperature (~21°C) for 20 min. After incubation, 25 µL of color agent A (sulfanilamide in acid) and 25 µL of color agent B (N-(1-Napthyl) ethylenediamine in acid) were added in each well. The mixture was then incubated at room temperature for 5 min. The absorbance of the sample was recorded at 540 nm (Molecular Devices Spectra Max, San Jose, CA, USA). Total NO content of potato tuber tissues was determined based on the standard curve prepared with nitrate standard and expressed as mM g-1 fresh weight.
2.4 Nitrate reductase (NR) activity
Activity of NR of the potato tissue samples was determined using a microplate spectrophotometry assay (MyBioSource Assay kit, San Diego, CA, USA). The catalyzed reaction product (azo-compound) from the enzymatic hydrolysis of nitrate by NR was measured using colorimetric readout at 540 nm. For this assay, 0.1 g of frozen tissue was homogenized with 1 mL of assay buffer (proprietary information of the assay kit) and then centrifuged at 10,000 rpm for 10 min. Initially, 20 µL of sample was added to microplate wells and then mixed with 80 µL substrate solutions. Following the manufacturer protocol, a control, blank and standard curve were also prepared. For controls 80 µL of distilled water (replacing the substrates), and for blanks 20 µL of distilled water (replacing sample) were used. The microplate was then incubated in oven at 37°C for 30 min. After incubation, 50 µL of two dye reagents (color agent A and B of the assay kit) were added in the wells and mixed thoroughly. For development of a standard curve, 20 µL of enzyme standard was used instead of the sample. The mixture was kept at room temperature for 20 min and then absorbance was recorded at 540 nm using a microplate reader (Molecular Devices Spectra Max, San Jose, CA, USA).
2.5 Nitric oxide synthase activity
The activity of NOS of tuber discs was determined using a microplate based spectrophotometric assay (Oxford Biomedical Research Kit, Rochester Hills, MI, USA). The assay principle is based on the use of recombinant NR for conversion of nitrate to nitrite and quantification of nitrite using Griess color reagent. To prepare reaction mixture, 30 µL of recombinant NOS was added in a 2 mL microcentrifuge tube and mixed with 200 µL of 20 mM HEPES buffer (with 0.5 mM EDTA), 10 µL of NADPH part A (NADP+, Glucose-6-phosphate, L-arginine), 10 µL of NADPH part B (Glucose 6-phosphate dehydrogenase), and 10 µL of NS70 (NOS Cofactor Mix); information on the concentration of the chemicals is protected through manufacturer proprietary. After mixing thoroughly, the reaction mixture was incubated at 37°C for 6 h. Then the mixture was chilled on ice for 5 min and 10 µL of reconstituted NR was added and mixed. The mixture was incubated at room temperature for 20 min and then centrifuged at 12,500 rpm for 5 min at 4°C. Then, 100 µL samples were added in microplate wells and mixed with the reaction mixture, while nitrite standard was used instead of samples for preparing the standard curve. After that, 50 µL of color reagent 1 (sulfanilamide in acid) and 50 µL of color reagent 2 (N-(1-Napthyl) ethylenediamine in acid) were added and mixed and the plate was allowed to shake for 5 min at room temperature. Absorbance of the samples and standards was measured at 540 nm (Molecular Devices Spectra Max, San Jose, CA, USA). The activity of the NOS of the sample was expressed as mM g-1 fresh weight of the sample and based on the conversion of 1 mM of substrate (L-arginine) per min.
2.6 Phenylalanine-ammonia lyase activity
The PAL activity of the tuber discs 3 d after wounding was determined using a spectrophotometric assay as described by Lulai et al. (2008). The sample from 3 d time point was selected for PAL assay to align with the results of SPP accumulation from histological analysis. Frozen disc tissues were ground to powder using mortar and pestle, and then extracted using 0.025 M cold borate buffer (pH 8.8, containing 1 mM dithiothreitol) and centrifuged for 15 min at 10,000 rpm. The formation of trans-cinnamic acid from L-phenylalanine was detected at 290 nm (Shimadzu model UV-1601 Spectrophotometer, Kyoto, Japan). The activity of PAL was expressed as unit g-1 fresh weight; one unit was defined as the number of enzymes needed for the conversion of 1 mM of L-phenylalanine into trans-cinnamic acid (molar extinction coefficient of 9630/M/cm) per min.
2.7 Statistical analysis
All experiments were repeated, and 4 biological replicates (8 tuber discs represent one biological replicate for biochemical assays) were used for each experimental unit. Mean, standard deviation, and standard errors were calculated using Microsoft Excel tools. The data were analyzed statistically using R software and one-way analysis of variance (ANOVA). Least significant difference (LSD) between treatments and time points was performed by Tukey’s HSD test at 95% confidence level.
3 Results and discussion
3.1 Histological determination of the accumulation of SPP in wounded surface of potato tubers
Response of plant tissues to wounding involves myriad of physiological and biochemical adjustments and changes in stress-associated molecular signaling (Ikeuchi et al., 2020). Formation of wound-protective barrier layer through synthesis of SPP and SPA in wounded surface is a common response of potato tuber tissues to wounding (Lulai et al., 2016). Exogenous chemical treatments that alter the WH responses of potato tubers potentially influence the accumulation of stress protective compounds such as SPP and SPA during closing layer and wound periderm formation. In our results, there were no accumulations of SPP in cells of parenchymal tissues at the wounded surface of potato tuber discs at 0 h time point. After 3 d of wounding, control treated tissues had a suberization rating of 3.8, which indicated accumulation of SPP around 50-60% in the first cell layer of the developing closing layer (Figure 1). Previous studies (Lulai et al., 2008; Kumar et al., 2010), using a similar potato tuber wounding model, reported suberization rating of 3.0-4.0 after 3 d of wounding. Because SPP accumulates at the outer tangential layer of first cell within 3 d of WH process (Lulai et al., 2008), we selected this time point to identify the effect of NO related treatments on WH responses of potato tubers during early stage of closing layer formation.
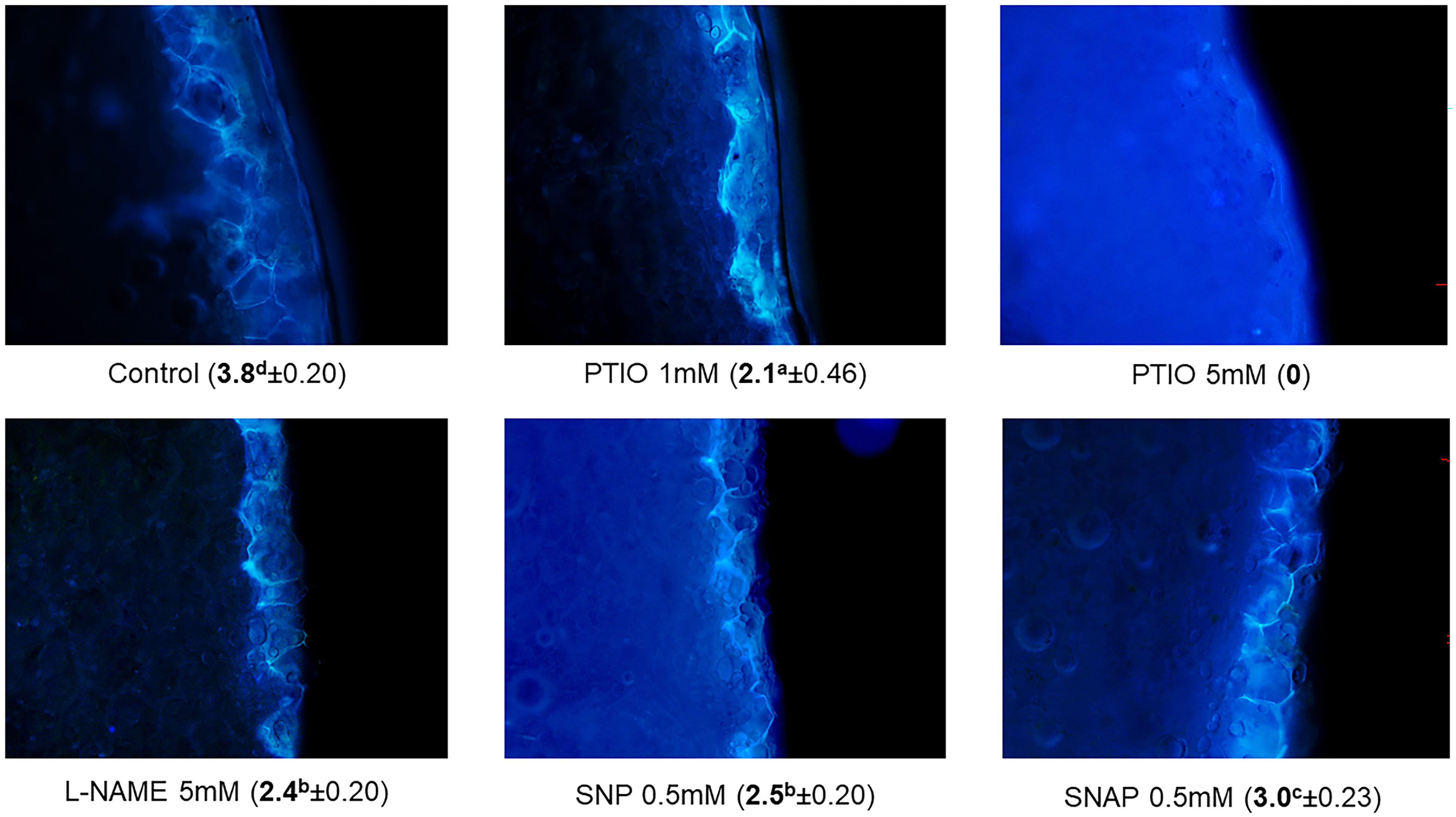
Figure 1 Accumulation of suberin polyphenolics (SPP) in first cell layer of the wounded surface (during closing layer formation) of potato tuber flesh tissues. Effect of different NO-related chemical treatments on SPP rating (in parenthesis ± standard error) 3 d after wounding. Different lowercase letters represent statistically significant differences in SPP ratings between treatments at 95% confidence level.
Treatment of tuber disc tissues with NO scavenger (PTIO) significantly hindered SPP accumulation 3 d after wounding (Figure 1). A higher concentration of PTIO treatment (5 mM) completely inhibited the formation of SPP, while treatment of 1 mM of PTIO considerably reduced the formation of SPP (rating of 2.1) when compared to the suberization rating of the control (rating of 3.8). These suberization rating results suggest that NO is an essential modulator for the formation of SPP, because scavenging of NO by PTIO inhibited SPP accumulation. In previous reports, NO scavenger (cPTIO) treatment also reduced WH responses in sweet potato roots (Yin et al., 2012) and potato leaflets (París et al., 2007). Furthermore, suppression of NO production with cPTIO treatment immediately after wounding was reported in geranium (Pelargonium peltatum L.) leaves (Arasimowicz et al., 2009). This study also highlighted the modulatory role of NO in WH responses, which involves oxidative stress-countering metabolic regulation and induction of cellular redox homeostasis (Arasimowicz et al., 2009). Similarly, Liu et al. (2015) found that cadmium induced toxicity and associated oxidative stress could be alleviated by application of NO donor (SNP), while NO scavenger (cPTIO) and NOS inhibitor (L-NAME) treatments counteracted the positive impacts of SNP in white clover (Trifolium repens L.).
Interestingly, treatment of tuber discs with NOS inhibitor (L-NAME) also impeded SPP accumulation (rating of 2.4) 3 d after wounding (Figure 1). Previously, impairment in NR dependent NO production was observed with L-NAME treatment in Arabidopsis (Rasul et al., 2012). These results indicate that scavenging of NO or inhibiting its biosynthesis can hinder SPP accumulation and subsequently WH. In the contrary, application of exogenous NO through NO donor treatments (SNP and SNAP) did not further contribute to SPP accumulation between 0 h to 3 d after wounding (Table 1). Collectively these results, inhibition of SPP accumulation by an NO scavenger and lack of improvement in SPP accumulation by NO donors, suggest that endogenous accumulation of NO is critical for healing processes of wounded potato tubers. It is well recognized that NO induces biphasic dose relationship during modulation of physiological processes of plant and animal cells (Calabrese and Agathokleous, 2023; Calabrese et al., 2023). Instead of accelerating, higher concentration of NO likely inhibit the WH response of plant tissues. The biphasic dose response of exogenous NO is considered as part of the hormesis phenomenon, where plant’s response to chemicals depends on their optimal concentration (Belz and Duke, 2022). For example, inhibition of wound-induced accumulation of proteinase was observed in wounded tomato (Solanum lycopersicum L.) seedlings with higher concentration of NO donor (SNP) treatment (Orozco-Cárdenas and Ryan, 2002). Inhibition of root growth, lignification, and loss of cell viability were also reported in soybean (Glycine max L.) seedling with higher concentration of SNP treatment (Böhm et al., 2010). Therefore, manipulation of endogenous NO content through optimized treatments (e.g., NO related chemicals) can be instrumental for improving WH responses of potato tubers. It is important to note that for commercial application of such treatments, careful examination of their overall impact on other critical postharvest factors, such as tuber dormancy and sprout growth or storage diseases, is warranted.
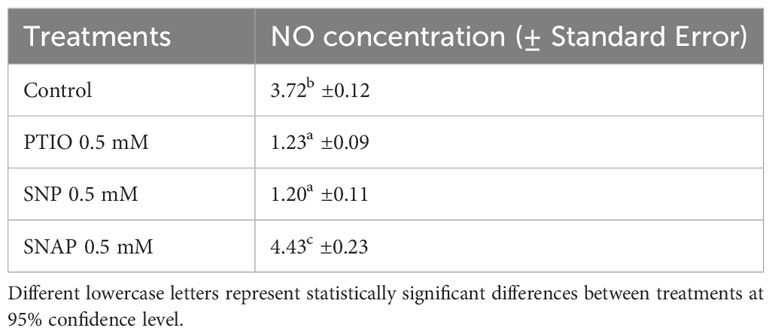
Table 1 Concentration (mM g-1 FW) of NO in cell free extracts of wounded potato tuber tissues treated with NO scavenger (PTIO) and NO donors (SNP and SNAP) at 0 h and assayed 7 d after wounding.
3.2 Changes in NO concentration during WH of potato tubers
Immediately after wounding, a rapid increase in content of NO in cell free extracts was observed (Figure 2). The results reflect the immediate NO production in potato tuber tissues at specific time points; however, since gaseous form of NO was not quantified the results do not represent the collective accumulation over time. The NO concentration peaked after 4 h and 12 h and sustained at basal level between 1 d and 7 d after wounding. A sustained level of NO concentration suggests a putative role of NO in WH response, particularly during the closing layer formation of potato tuber tissues. Rapid increase in NO production (often along with other stress-induced metabolites) at the wounded surface of leaf tissues was reported in other plant species (Arasimowicz et al., 2009; Si et al., 2017). Similarly, Huang et al. (2004) reported wound-induced accumulation of NO in Arabidopsis plants. They also suggested that NO might be involved in jasmonic acid (JA) related stress responses in plants (Huang et al., 2004). These results indicate that accumulation of NO is an immediate response to wounding and might correlate with other stress responses in plant tissues.
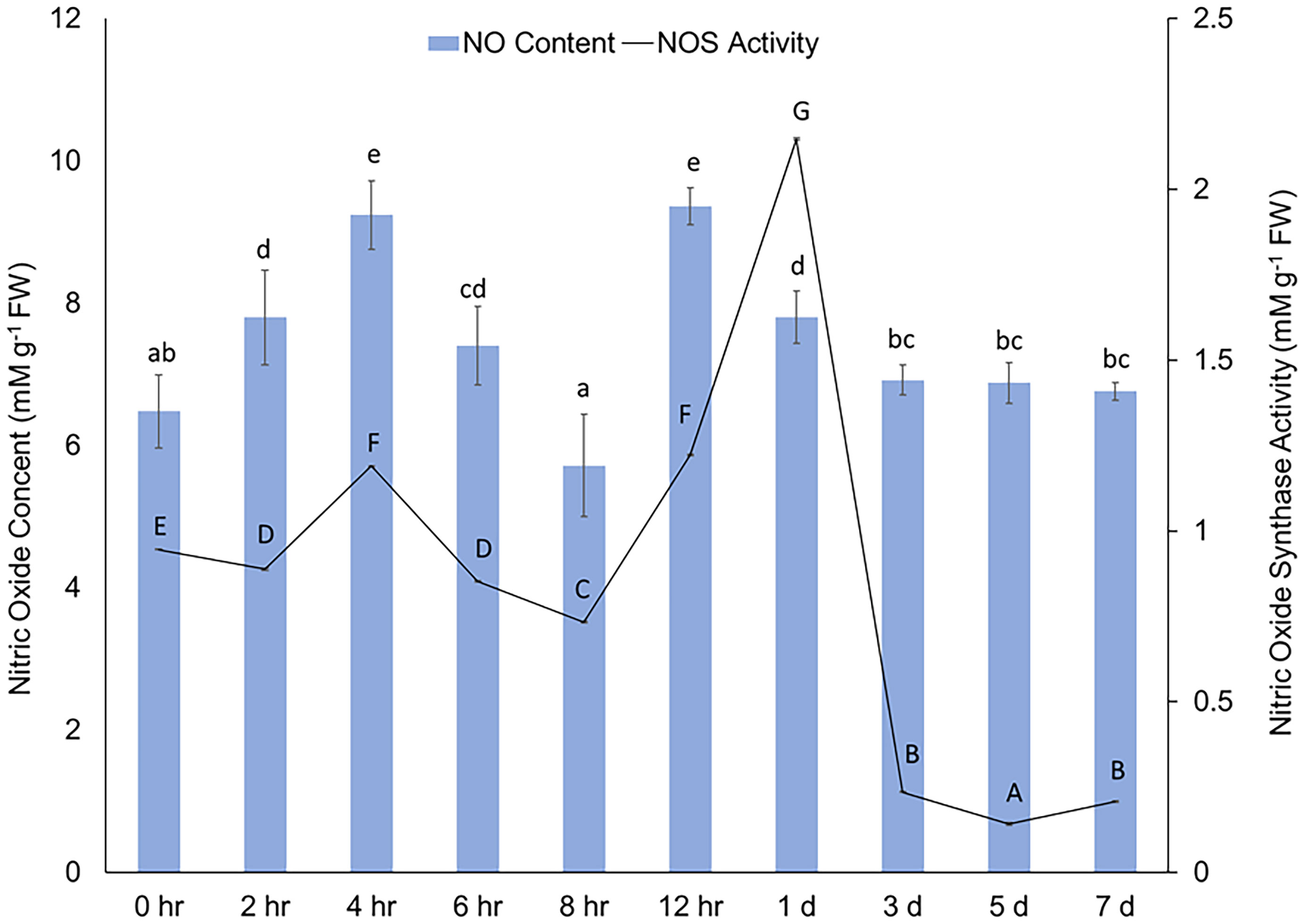
Figure 2 Concentration (mM g-1 FW) of NO (y-axis) in cell free extracts and changes in activity of NOS [mM g-1 FW based on the conversion of 1 mM substrate (L-arginine) per minute] (z-axis) in wounded potato tuber tissues between 0 h to 7 d after wounding. The vertical bars represent ± standard error (the standard error bars of the NOS activity are not distinctly visible due to very negligible value). Different lowercase letters represent statistically significant differences in NO concentration, while different uppercase letters represent statistically significant in NOS activity between different time points (0 hr to 7 d) at 95% confidence level.
We also quantified NO concentration in treated tuber tissues after 7 d of wounding, which coincides with the last phase of closing layer formation. Lower concentration of NO (1.23 mM g-1 FW) was found in PTIO treated tuber tissues when compared to the control (MES) treatment (3.72 mM g-1 FW) (Table 1). Interestingly, different responses in NO content were observed between the NO donor treatments (SNP and SNAP); while SNAP resulted in higher, and SNP resulted in lower NO content. Differential response in NO concentration due to different NO donor treatments was previously reported in wounded tissues of sunflower (Helianthus annus L.) (Singh and Bhatla, 2017). Under low light condition, higher rates of NO generation were reported in leaf tissues of geranium with only SNAP treatment, while SNP treatment yielded lower NO content (Floryszak-Wieczorek et al., 2006). We hypothesize that the efficacy of NO donor treatments to enhance NO content might be dependent on light in addition to the specific dose dependent response. Therefore, as potato tubers develop underground and stored in dark, it is necessary to determine optimum doses of effective NO donor treatments (such as SNAP) to enhance WH responses of tubers under dark conditions.
3.3 Activity of NR during WH of potato tubers
No detectable NR activity was observed in wounded potato tissues between 0 h to 7 d after wounding. The absence of detectable NR activity could be due to either low NR activity in wounded potato tissues or the potential limitations of the assay kit used. Garcês et al. (2001) previously reported that rapid production of NO after mechanical wounding is not a by-product of NR activity in Arabidopsis thaliana NR mutant. Therefore, the NO synthesis in plants whether through NR or NOS might be specific to tissue types or depending on type of stresses. The specific role of NR for biosynthesis of NO in wounded potato tuber tissues will need to be further investigated with other enzyme assay protocols.
3.4 Changes in activity of NOS during WH of potato tubers
A rapid increase in NOS activity in potato tuber discs following wounding was observed (Figure 2). The activity of NOS increased 4 h and 12 h after wounding and the increase was almost 3 folds at 1 d. The alignment between rapid induction of NOS activity and subsequent generation of NO immediately after wounding indicates a modulatory role of NO and its biosynthesis from L-arginine during potato tuber WH. Catalysis of L-arginine by NOS is one of the primary sources of endogenous NO in plant cells (León and Costa-Broseta, 2020; Sun et al., 2023). While NO content remained abundant after 1 d of wounding, a rapid decrease in NOS activity was observed after this time point. Previously, Chaki et al. (2011) reported a significant reduction in NOS activity after 4h of mechanical wounding of sunflower seedling. However, S-nitrosothiol content enhanced following the wounding of sunflower seedling in the same study (Chaki et al., 2011), which indicated a complex relationship between activity of enzymes related to NO biosynthesis and accumulation process of wound protective compounds in plant tissues. Due to the peak NOS activity at 1 d and well-known significance of 3 d in studying initial WH responses of potato tubers (Lulai et al., 2008; Kumar et al., 2010), we used timepoints of 12 h, 1 d, and 3 d to investigate the effect of NO related chemical treatments on NOS activity in wounded potato tuber tissues (Table 2).
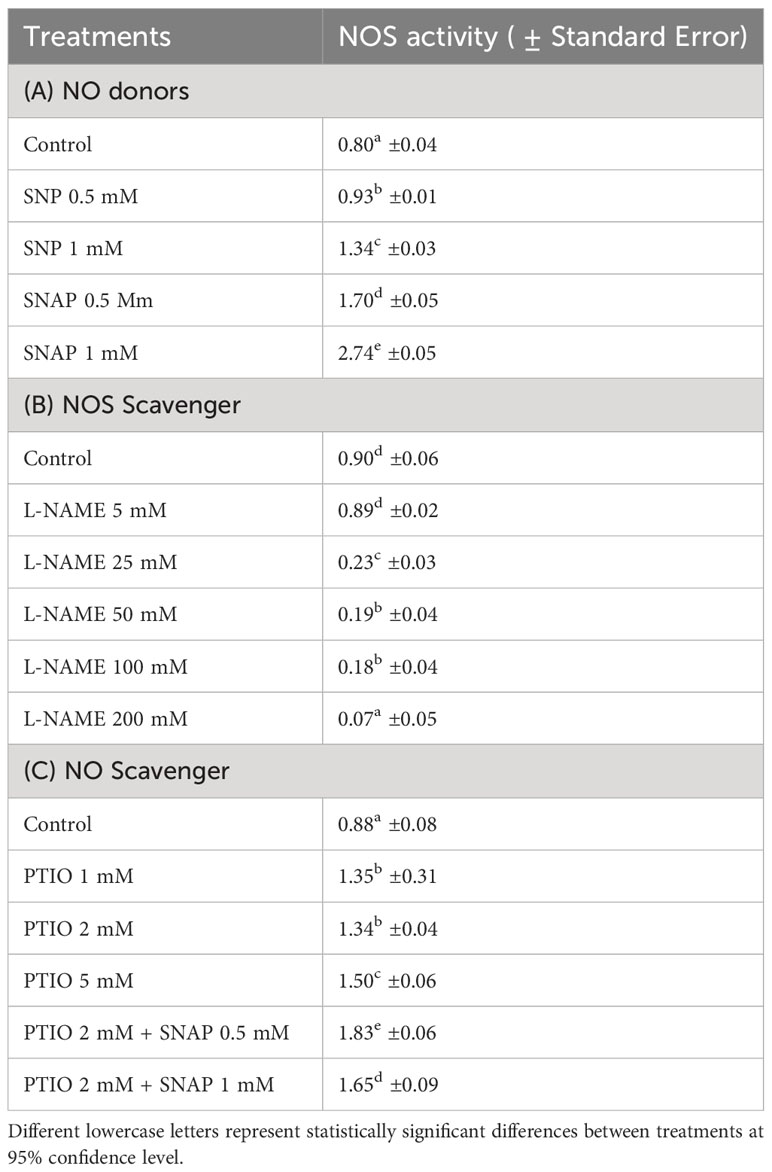
Table 2 Changes in activity of NOS [mM g-1 FW based on the conversion of 1 mM substrate (L-arginine) per minute] in wounded potato tuber tissues with treatment of NO donors (SNP and SNAP) at 0 h and assayed 12 h after wounding (A); with treatments of different concentrations of NOS scavenger (L-NAME) at 0 h and assayed 1 d after wounding (B); and with NO scavenger (PTIO) alone or combination of NO scavenger (PTIO) and NO donor (SNAP) treatment at 0 h and assayed 3 d after wounding (C).
After 12 h of wounding, higher NOS activity was observed with treatment of the NO donor (1 mM SNAP) (Table 2A). Similar to the result of NO concentration, SNP treatment did not yield higher NOS activity in wounded potato tuber tissues. However, treatment of wounded potato tuber discs with higher concentrations (25-200 mM) of NOS inhibitor (L-NAME) significantly inhibited NOS activity 1 d after wounding (Table 2B). Treatment of NO scavenger (PTIO) did not cause reduction of NOS activity, while combined treatment of PTIO+SNAP resulted in increased NOS activity 3 d after wounding (Table 2C). Results of this study suggest that generation of NO through catalysis of NOS plays some role in WH processes of potato tubers and align with previous reports. Enhanced NO content and improved resistance against Rhizoctonia solani with SNAP treatment was previously reported in kidney bean (Keshavarz-Tohid et al., 2016). Elimination of NOS activity by application of L-NAME combined with 24-Epibrassinolide was found in strawberry plant (Fragaria × ananassa Duch) tissues (Kaya et al., 2020). However, elimination or reduction of NOS activity did not completely inhibit endogenous NO (Kaya et al., 2020). Collectively, these results suggest that there could be alternative mechanisms involved in NO biosynthesis in wounded plant tissues. Future investigation on dose dependent and biphasic response of NO donors on NOS activity and production of NO after wounding will improve our understanding on modulatory role of NO in WH processes of potato tubers.
3.5 Activity of PAL during WH of potato tubers
The activity of PAL in tuber tissue sample was determined 3 d after wounding to find a potential alignment with SPP accumulation results and to examine the effects of NO related chemical treatments on biosynthesis of SPP. After 3 d of wounding, PAL activity was reduced with treatment of NO scavenger (PTIO 1 mM), while a higher concentration of PTIO (5 mM) treatment did not result in further reduction in PAL activity (Table 3). The contrasting trend between complete inhibition of SPP accumulation (Figure 1) and moderate PAL activity (Table 3) with higher concentration of PTIO treatment (5 mM) was unexpected. Similarly, decreased activity of PAL with NO donor (SNP and SNAP) treatments does not align. As biochemical response of wounded tissues to NO related chemicals is very specific to dose or concentrations of the chemicals, further studies with different NO donor treatments are required to clarify our findings. Additionally, it is also important to determine metabolic profile associated with phenylpropanoid pathways to better align PAL activity and SPP formation in wounded potato tuber tissues. While the results of the PAL activity with NO related chemical treatments are not conclusive in our study, role of PAL in WH processes of potato has been highlighted in numerous reports. For instance, increased PAL-transcript abundance was found in SNP treated and wounded potato leaflets (París et al., 2007). Enhanced production of endogenous NO and higher gene expression (StPAL) and activity of PAL enzyme were observed in wounded and melatonin treated potato tuber tissues (Wang et al., 2023). Increase in wound-induced PAL activity, especially in younger potato tubers and with ABA treatment was also reported (Lulai et al., 2008; Kumar et al., 2010). Future study with frequent and successive (between 0 h- 4 d after wounding) analysis of PAL activity along with determination of NO concentration is needed for understanding the role of PAL in NO related modulation of WH of potato tubers.
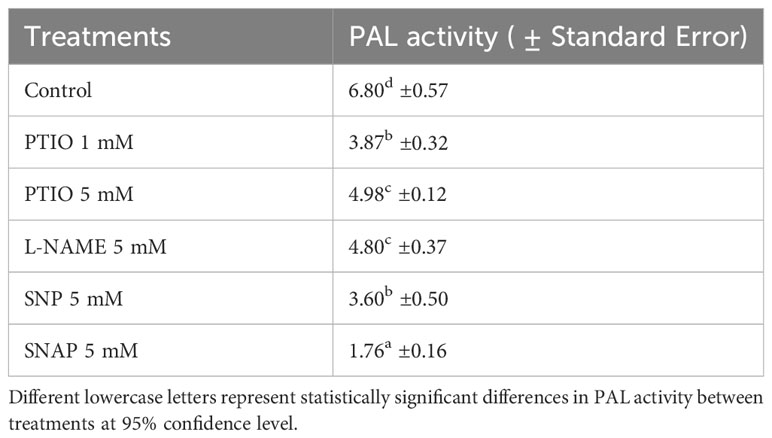
Table 3 Activity of PAL (Unit g-1 FW) in wounded and NO scavenger (PTIO), NOS inhibitor (L-NAME), and NO donors (SNP and SNAP) treated tuber discs at 0 h and assayed 3 d after wounding.
4 Conclusion
This is the first known research suggesting that NO is an important biological modulator in WH processes of potato tubers. The complete inhibition of SPP accumulation 3 d after wounding by an NO scavenger implies the putative role of endogenous NO in formation of closing layer during WH of potato tuber tissues. The positive correlation and alignment between NOS activity and generation of NO detected in cell free extracts also provides insights on the NOS-dependent biosynthesis of NO in wounded potato tubers. The dose and timing of exogenous application of NO donors can be further optimized to accelerate WH responses of different potato cultivars for successful long-term storage or for cut potato seed tubers. The impact of exogenous application of NO on other critical physiological processes for successful postharvest storage (e.g., tuber dormancy and sprout growth) and performance of cut seed tubers should also be examined prior to adopting such applications by the potato industry. Overall, our results are important for developing novel approaches to hasten suberization and reduce wound related losses.
Data availability statement
The raw data supporting the conclusions of this article will be made available by the authors, without undue reservation.
Author contributions
MD: Conceptualization, Data curation, Formal analysis, Investigation, Methodology, Project administration, Software, Supervision, Validation, Writing – original draft, Writing – review & editing. DS: Conceptualization, Data curation, Formal analysis, Investigation, Methodology, Software, Supervision, Validation, Writing – original draft, Writing – review & editing. EL: Conceptualization, Data curation, Formal analysis, Investigation, Methodology, Project administration, Software, Supervision, Validation, Writing – original draft, Writing – review & editing.
Funding
The author(s) declare financial support was received for the research, authorship, and/or publication of this article. This research was funded through United States Department of Agriculture (USDA) In-House Appropriated Project 3060-21430-009-000-D.
Conflict of interest
The authors declare that the research was conducted in the absence of any commercial or financial relationships that could be construed as a potential conflict of interest.
Publisher’s note
All claims expressed in this article are solely those of the authors and do not necessarily represent those of their affiliated organizations, or those of the publisher, the editors and the reviewers. Any product that may be evaluated in this article, or claim that may be made by its manufacturer, is not guaranteed or endorsed by the publisher.
Author disclaimer
USDA is an equal opportunity provider and employer. Mention of trade names in this publication is solely for the purpose of providing specific information and does not imply recommendation or endorsement by the USDA.
References
Arasimowicz M., Floryszak-Wieczorek J., Milczarek G., Jelonek T. (2009). Nitric oxide, induced by wounding, mediates redox regulation in pelargonium leaves. Plant Biol. 11 (5), 650–663. doi: 10.1111/j.1438-8677.2008.00164.x
Beligni M. V., Lamattina L. (2002). Nitric oxide interferes with plant photo-oxidative stress by detoxifying reactive oxygen species. Plant Cell Environ. 25 (6), 737–748. doi: 10.1046/j.1365-3040.2002.00857.x
Belz R. G., Duke S. O. (2022). Modelling biphasic hormetic dose responses to predict sub-NOAEL effects using plant biology as an example. Curr. Opin. Toxicol. 29, 36–42. doi: 10.1016/j.cotox.2022.01.003
Böhm F. M. L. Z., Ferrarese M. D. L. L., Zanardo D. I. L., Magalhaes J. R., Ferrarese-Filho O. (2010). Nitric oxide affecting root growth, lignification and related enzymes in soybean seedlings. Acta Physiologiae Plantarum 32, 1039–1046. doi: 10.1007/s11738-010-0494-x
Calabrese E. J., Agathokleous E. (2023). Nitric oxide, hormesis and plant biology. Sci. Total Environ. 866, 161299. doi: 10.1016/j.scitotenv.2022.161299
Calabrese E. J., Agathokleous E., Dhawan G., Kapoor R., Dhawan V., Manes P. K., et al. (2023). Nitric oxide and hormesis. Nitric. Oxide 133, 1–17. doi: 10.1016/j.niox.2023.02.001
Chaki M., Valderrama R., Fernandez-Ocana A. M., Carreras A., Gomez-Rodriguez M. V., Pedrajas J. R., et al. (2011). Mechanical wounding induces a nitrosative stress by down-regulation of GSNO reductase and an increase in S-nitrosothiols in sunflower (Helianthus annuus) seedlings. J. Exp. Bot. 62 (6), 1803–1813. doi: 10.1093/jxb/erq358
D’Alessandro S., Posocco B., Costa A., Zahariou G., Schiavo F. L., Carbonera D., et al. (2013). Limits in the use of cPTIO as nitric oxide scavenger and EPR probe in plant cells and seedlings. Front. Plant Sci. 4. doi: 10.3389/fpls.2013.00340
Floryszak-Wieczorek J., Milczarek G., Arasimowicz M., Ciszewski A. (2006). Do nitric oxide donors mimic endogenous NO-related response in plants? Planta 224, 1363–1372. doi: 10.1007/s00425-006-0321-1
Garcês H., Durzan D., Pedroso M. C. (2001). Mechanical stress elicits nitric oxide formation and DNA fragmentation in Arabidopsis thaliana. Ann. Bot. 87 (5), 567–574. doi: 10.1006/anbo.2000.1356
Gupta K. J., Hancock J. T., Petrivalsky M., Kolbert Z., Lindermayr C., Durner J., et al. (2020). Recommendations on terminology and experimental best practice associated with plant nitric oxide research. New Phytol. 225 (5), 1828–1834. doi: 10.1111/nph.16157
Huang X., Stettmaier K., Michel C., Hutzler P., Mueller M. J., Durner J. (2004). Nitric oxide is induced by wounding and influences jasmonic acid signaling in Arabidopsis thaliana. Planta 218, 938–946. doi: 10.1007/s00425-003-1178-1
Hussain A., Shah F., Ali F., Yun B. W. (2022). Role of nitric oxide in plant senescence. Front. Plant Sci. 13. doi: 10.3389/fpls.2022.851631
Ikeuchi M., Rymen B., Sugimoto K. (2020). How do plants transduce wound signals to induce tissue repair and organ regeneration? Curr. Opin. Plant Biol. 57, 72–77. doi: 10.1016/j.pbi.2020.06.007
Kaya C., Ashraf M., AlYemeni M. N., Ahmad P. (2020). Nitrate reductase rather than nitric oxide synthase activity is involved in 24-epibrassinolide-induced nitric oxide synthesis to improve tolerance to iron deficiency in strawberry (Fragaria× annassa) by up-regulating the ascorbate-glutathione cycle. Plant Physiol. Biochem. 151, 486–499. doi: 10.1016/j.plaphy.2020.04.002
Keshavarz-Tohid V., Taheri P., Taghavi S. M., Tarighi S. (2016). The role of nitric oxide in basal and induced resistance in relation with hydrogen peroxide and antioxidant enzymes. J. Plant Physiol. 199, 29–38. doi: 10.1016/j.jplph.2016.05.005
Kolupaev Y. E., Yemets A. I., Yastreb T. O., Blume Y. B. (2023). The role of nitric oxide and hydrogen sulfide in regulation of redox homeostasis at extreme temperatures in plants. Front. Plant Sci. 14. doi: 10.3389/fpls.2023.1128439
Krasylenko Y. A., Yemets A. I., Blume Y. B. (2019). Nitric oxide synthase inhibitor L-NAME affects Arabidopsis root growth, morphology, and microtubule organization. Cell Biol. Int. 43 (9), 1049–1055. doi: 10.1002/cbin.10880
Kumar G. M., Lulai E. C., Suttle J. C., Knowles N. R. (2010). Age-induced loss of wound-healing ability in potato tubers is partly regulated by ABA. Planta 232, 1433–1445. doi: 10.1007/s00425-010-1269-8
Lazalt A. M., Beligni M. V., Lamattina L. (1997). Nitric oxide preserves the level of chlorophyll in potato leaves infected by Phytophthora infestans. Eur. J. Plant Pathol. 103, 643–651. doi: 10.1023/A:1008604410875
León J., Costa-Broseta Á. (2020). Present knowledge and controversies, deficiencies, and misconceptions on nitric oxide synthesis, sensing, and signaling in plants. Plant Cell Environ. 43 (1), 1–15. doi: 10.1111/pce.13617
Liu S. L., Yang R. J., Pan Y. Z., Wang M. H., Zhao Y., Wu M. X., et al. (2015). Exogenous NO depletes Cd-induced toxicity by eliminating oxidative damage, re-establishing ATPase activity, and maintaining stress-related hormone equilibrium in white clover plants. Environ. Sci. pollut. Res. 22, 16843–16856. doi: 10.1007/s11356-015-4888-6
Lulai E. C., Campbell L. G., Fugate K. K., McCue K. F. (2016). Biological differences that distinguish the 2 major stages of wound healing in potato tubers. Plant Signal. Behav. 11 (12), e1256531. doi: 10.1080/15592324.2016.1256531
Lulai E. C., Corsini D. L. (1998). Differential deposition of suberin phenolic and aliphatic domains and their roles in resistance to infection during potato tuber (Solanum tuberosumL.) wound-healing. Physiol. Mol. Plant Pathol. 53 (4), 209–222. doi: 10.1006/pmpp.1998.0179
Lulai E. C., Suttle J. C., Pederson S. M. (2008). Regulatory involvement of abscisic acid in potato tuber wound-healing. J. Exp. Bot. 59 (6), 1175–1186. doi: 10.1093/jxb/ern019
Marvasi M. (2017). Potential use and perspectives of nitric oxide donors in agriculture. J. Sci. Food Agric. 97 (4), 1065–1072. doi: 10.1002/jsfa.8117
Neill S. (2005). NO way to die: nitric oxide, programmed cell death and xylogenesis. New Phytol. 165 (1), 5–7. doi: 10.1111/j.1469-8137.2004.01267.x
Neill S., Bright J., Desikan R., Hancock J., Harrison J., Wilson I. (2008). Nitric oxide evolution and perception. J. Exp. Bot. 59 (1), 25–35. doi: 10.1093/jxb/erm218
Noritake T., Kawakita K., Doke N. (1996). Nitric oxide induces phytoalexin accumulation in potato tuber tissues. Plant Cell Physiol. 37 (1), 113–116. doi: 10.1093/oxfordjournals.pcp.a028908
Orozco-Cárdenas M. L., Ryan C. A. (2002). Nitric oxide negatively modulates wound signaling in tomato plants. Plant Physiol. 130 (1), 487–493. doi: 10.1104/pp.008375
París R., Lamattina L., Casalongué C. A. (2007). Nitric oxide promotes the wound-healing response of potato leaflets. Plant Physiol. Biochem. 45 (1), 80–86. doi: 10.1016/j.plaphy.2006.12.001
Rasul S., Wendehenne D., Jeandroz S. (2012). Study of oligogalacturonides-triggered nitric oxide (NO) production provokes new questioning about the origin of NO biosynthesis in plants. Plant Signal. Behav. 7 (8), 1031–1033. doi: 10.4161/psb.20658
Sanz L., Albertos P., Mateos I., Sánchez-Vicente I., Lechón T., Fernández-Marcos M., et al. (2015). Nitric oxide (NO) and phytohormones crosstalk during early plant development. J. Exp. Bot. 66 (10), 2857–2868. doi: 10.1093/jxb/erv213
Senthil Kumar R., Shen C. H., Wu P. Y., Suresh Kumar S., Hua M. S., Yeh K. W. (2016). Nitric oxide participates in plant flowering repression by ascorbate. Sci. Rep. 6 (1), 35246. doi: 10.1038/srep35246
Shi H. T., Li R. J., Cai W., Liu W., Fu Z. W., Lu Y. T. (2012). In vivo role of nitric oxide in plant response to abiotic and biotic stress. Plant Signal. Behav. 7 (3), 437–439. doi: 10.4161/psb.19219
Si T., Wang X., Wu L., Zhao C., Zhang L., Huang M., et al. (2017). Nitric oxide and hydrogen peroxide mediate wounding-induced freezing tolerance through modifications in photosystem and antioxidant system in wheat. Front. Plant Sci. 8. doi: 10.3389/fpls.2017.01284
Signorelli S., Considine M. J. (2018). Nitric oxide enables germination by a four-pronged attack on ABA-induced seed dormancy. Front. Plant Sci. 9. doi: 10.3389/fpls.2018.00296
Simontacchi M., Galatro A., Ramos-Artuso F., Santa-María G. E. (2015). Plant survival in a changing environment: the role of nitric oxide in plant responses to abiotic stress. Front. Plant Sci. 6. doi: 10.3389/fpls.2015.00977
Singh N., Bhatla S. C. (2017). Signaling through reactive oxygen and nitrogen species is differentially modulated in sunflower seedling root and cotyledon in response to various nitric oxide donors and scavengers. Plant Signaling Behav. 12 (9), e1365214. doi: 10.1080/15592324.2017.1365214
Sun L. R., Yue C. M., Hao F. S. (2019). Update on roles of nitric oxide in regulating stomatal closure. Plant Signal. Behav. 14 (10), e1649569. doi: 10.1080/15592324.2019.1649569
Sun Y., Miao F., Wang Y., Liu H., Wang X., Wang H., et al. (2023). L-Arginine alleviates the reduction in photosynthesis and antioxidant activity induced by drought stress in maize seedlings. Antioxidants 12 (2), 482. doi: 10.3390/antiox12020482
Tamás L., Demecsová L., Zelinová V. (2018). L-NAME decreases the amount of nitric oxide and enhances the toxicity of cadmium via superoxide generation in barley root tip. J. Plant Physiol. 224, 68–74. doi: 10.1016/j.jplph.2018.03.007
Thornton M., Bohl W. (1995). Preventing potato bruise damage (Moscow, Idaho: University of Idaho Cooperative Extension System). Available at: https://idahopotato.com/uploads/media/intl-best-practices-PreventingPotatoBruiseDamage.pdf.
Wang Q., Han Y., Yang R., Zhang X., Zhu Y., Oyom W., et al. (2023). Melatonin facilitates the deposition of suberin polyphenolic and lignin at wounds of potato tubers by mediating nitric oxide and reactive oxygen species. Postharvest Biol. Technol. 198, 112270. doi: 10.1016/j.postharvbio.2023.112270
Wani K. I., Naeem M., Castroverde C. D. M., Kalaji H. M., Albaqami M., Aftab T. (2021). Molecular mechanisms of nitric oxide (NO) signaling and reactive oxygen species (ROS) homeostasis during abiotic stresses in plants. Int. J. Mol. Sci. 22 (17), 9656. doi: 10.3390/ijms22179656
Yin J., Bai S., Wu F., Lu G., Yang H. (2012). Effect of nitric oxide on the activity of phenylalanine ammonia-lyase and antioxidative response in sweet potato root in relation to wound-healing. Postharvest Biol. Technol. 74, 125–131. doi: 10.1016/j.postharvbio.2012.06.011
Keywords: biological modulator, nitric oxide biosynthesis, suberization, suberin polyphenolics, wound healing, postharvest loss
Citation: Dogramaci M, Sarkar D and Lulai EC (2024) Modulatory role of nitric oxide in wound healing of potato tubers. Front. Hortic. 3:1345461. doi: 10.3389/fhort.2024.1345461
Received: 27 November 2023; Accepted: 10 January 2024;
Published: 22 January 2024.
Edited by:
Natasha Damiana Spadafora, University of Calabria, ItalyReviewed by:
María Serrano, Miguel Hernández University of Elche, SpainMichail Michailidis, Aristotle University of Thessaloniki, Greece
Copyright © 2024 Dogramaci, Sarkar and Lulai. This is an open-access article distributed under the terms of the Creative Commons Attribution License (CC BY). The use, distribution or reproduction in other forums is permitted, provided the original author(s) and the copyright owner(s) are credited and that the original publication in this journal is cited, in accordance with accepted academic practice. No use, distribution or reproduction is permitted which does not comply with these terms.
*Correspondence: Munevver Dogramaci, bXVuZXZ2ZXIuZG9ncmFtYWNpQHVzZGEuZ292