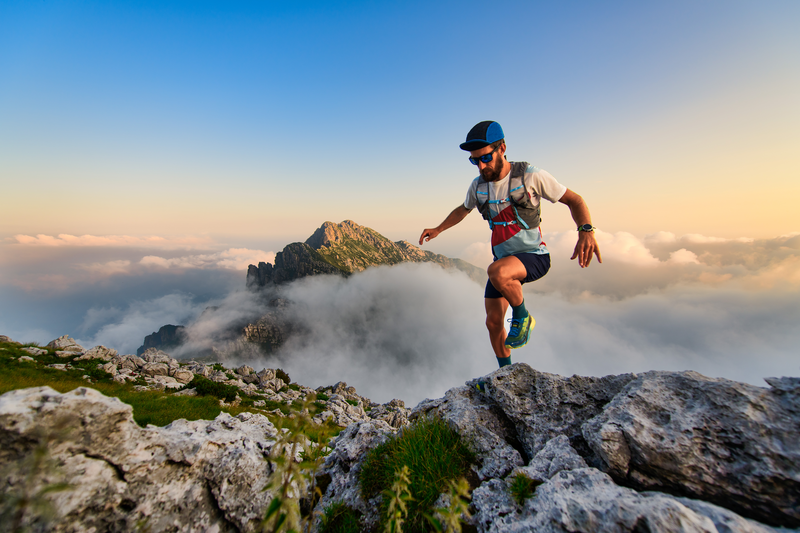
94% of researchers rate our articles as excellent or good
Learn more about the work of our research integrity team to safeguard the quality of each article we publish.
Find out more
ORIGINAL RESEARCH article
Front. Hortic. , 03 August 2023
Sec. Breeding and Genetics
Volume 2 - 2023 | https://doi.org/10.3389/fhort.2023.1190145
This article is part of the Research Topic Emerging Talents in Horticulture Breeding and Genetics 2022 View all 4 articles
One of the most important problems for the field-grown Ayaş tomato (Solanum lycopersicum Ayas population), which is preferred for consumption in Central Anatolia because of its aromatic taste, is that the shelf life is short because of insufficient fruit firmness. Therefore, a study was initiated to develop high-quality lines and variety candidates through mutation breeding in the current Ayaş population. In this study, the effective mutation dose (EMD50) was found to be 150 Gy for seeds using a Cobalt-60 gamma ray source. The main mutant population was generated by applying EMD50. During the study, mutant lines selected by following the classical mutation breeding stages were evaluated in terms of yield and quality traits (antioxidant content, total soluble solid amount, fruit firmness, Brix, etc.) starting from the M4 stage. One of our aims was to determine and improve the phytonutrient content of field-grown tomatoes. The fruits of selected lines at the M4 stage were extracted by liquid-liquid partition and accelerated solvent extraction techniques and analyzed by High Performance Liquid Chromatography (HPLC) in this study. It was found that 28 of the 29 mutant lines were significantly different from the control and mutant lines. Mutant lines 9-22, 8-90, 8-135, and 8-127 were determined to be the most promising for commercialization.
The tomato (Solanum lycopersicum, formerly Lycopersicon esculentum) is a member of the Solanaceae family that is grown and consumed all over the world and, thus, is an important commercial crop (Esengun et al., 2007). With a production capacity of 13.000.000 tons per year, Turkey is the fourth-largest producer in the world (TUIK, 2022). Besides their economic value, tomatoes make a valuable contribution to human health. This vegetable crop represents an excellent source of health-promoting compounds due to the perfect combination of minerals and antioxidants, including vitamins C and E (tocopherols), carotenoids (lycopene, b-carotene, lutein, etc.), and flavonoids (Hernández et al., 2008; Alothman et al., 2009; Balacheva et al., 2012; Ali et al., 2021). Dietary antioxidants have been consistently shown to be associated with a reduced risk of chronic disease (Marti et al., 2016). These antioxidants are understood to be bioactive substances that protect humans from the potential negative consequences of free radical-induced harm. (Salehi et al., 2019; Saini et al., 2020). Improvement in such phytonutrients can be achieved by several techniques, such as cultivar selection, environmental factors, agronomic practices, plant breeding (classical or biotechnological methods), etc. (Dorais et al., 2008; Tomlekova et al., 2009a; Tomlekova et al., 2009b; Fentik, 2017). Plant breeding is the strategy of modifying a plant’s genetics to achieve desired traits (Poehlman and Sleeper, 1995; Kaushik et al., 2015; Hanson, 2016; Natalini et al., 2021). It may be done using a variety of methods, from straightforward molecular procedures to selecting plants for production that have desirable traits. Mutation breeding is one of the most widely used techniques and a common approach for producing vegetable crops that are sustainable under varied agro-climatic conditions and have high nutrient content (Ahloowalia and Maluszynski, 2001; Quartey et al., 2012; Chaudhary et al., 2019; Yali and Mitiku, 2022; Datta, 2023).
Due to its importance in terms of human nutrition and the possibility of evaluating it in terms of consumption in different ways (dry, tomato paste, juice, fresh consumption, etc.), tomato emerges as a species that is studied intensively. Especially due to the changing climatic conditions and the agricultural production area decreasing day by day, it has become important to develop new varieties with high nutritional value for other breeding targets (tolerance to abiotic and biotic conditions, high yield per unit area, etc.) in all species. Today, tomato breeding studies are carried out using both classical breeding methods and biotechnological techniques (transgene, gene editing, etc.) at different technological levels (Vu et al., 2020). However, the mutation breeding method, which is included in the classical methods with the aim of expanding the shrinking gene pool in some conditions, is a method used in practice for tomato species (Levin et al., 2006; Laskar et al., 2018). Mutation breeding is used as a good tool (Chaudhary et al., 2019), especially in breaking the barriers in interspecies hybridization and creating genetic resources for today’s next generation sequence (NGS) studies (Tripodi, 2022).
Deliberately induced mutations can improve yield, quality, taste, size, and resistance to disease and can help plants adapt to diverse climates and conditions. Such mutations have been used to enhance the economic value of seed-propagated crops such as cereals and Solanaceae (Ahloowalia et al., 2004; Levin et al., 2006; Quartey et al., 2012). Though not widely understood by consumers, induced mutation provides an opportunity for plant breeders to create new varieties, including vegetable crops (lettuce, tomato, pepper, green bean, eggplant), field crops (wheat, barley, chickpea, rice, cotton), fruit crops (peach, cherry, lemon, mandarin, apple, banana), and ornamental crops (chrysanthemum, tulip, carnation). Mutants producing a high yield or increased vitamin C (ascorbic acid) or antioxidant capacity have been produced in bell and green pepper (Tomlekova et al., 2009a; Petrov et al., 2013) and tomato (Levin et al., 2006; Cebolla-Cornejo et al., 2013; Kornienko et al., 2014; Tomlekova et al., 2014) breeding programs. The economic value of a new variety can be assessed based on several parameters, such as yield, savings (e.g., water), and nutritional content.
High-performance liquid chromatography (HPLC) is the most widely used technique in food analysis for measuring vitamins, carbohydrates, additives (antioxidants, colorants, artificial sweeteners, etc.), proteins, amino acids, mycotoxins, triglycerides (TGs) in fats and oils, lipids, chiral compounds, and pigments (carotenoids, anthocyanines) (Chang et al., 1995; Tarrago-Trani et al., 2012; Silva et al., 2016; Colak et al., 2022).
The aim of this study was to investigate the vitamin C, carotenoids, and tocopherol content of M4-stage mutant field-grown tomato lines obtained previously. Tomato fruits were characterized in terms of physical traits (fruit firmness, fruit volume, peel thickness, fruit yield, cracking, shape, dry matter, and fruit color) and chemical properties (carotenoid, tocopherol, and vitamin C contents) in the framework of an agronomical analysis of mutant tomato lines and their control lines. The physical traits of M4, M5, and M6 stage mutants were identical. Tomato fruits at the M5 and M6 stages were similar to those at the M4 stage, so M4 stage mutants were selected for further analysis. For this purpose, liquid-liquid partitioning and accelerated solvent extraction techniques were used to extract phytonutrients analyzed by HPLC. Statistical analysis has also been applied to compare sets of means between control and mutant lines.
Field-grown tomato (Solanum lycopersicum Ayas population) seeds obtained from the Faculty of Agriculture under the University of Ankara (TR). In order to determine the effective mutation dose, 8 different doses of seed (0, 50, 100, 200, 300, 400, 500, and 600 Gy) were irradiated in 2010. The irradiated seeds were planted in viols with three replications and 30 seeds in each replication, and the plant height was measured on the 30th day after sowing (van Harten, 1998). The obtained data were evaluated with linear regression analysis, and the effective mutagen dose was determined. 3000 seeds were irradiated with 150 Gy (effective mutagen dose) using 60Co gamma rays at a fixed dose rate (0.654 kGy/h). Seeds were sown in nursery beds under controlled climatic conditions. Then, seedlings were transplanted to a 4,500-m2 field at Sarayköy (Ankara, TR) with an alternate system of plantation and a spacing of 1.5 m between rows and 1 m between plants. Each year, plantations were set up in the different field to protect the plants from soil-originating diseases and soil fatigue. Just before the transplantation, the soil was enriched with the animal manure (1 tone/1000 m2) and NPK (nitrogen:phosphorous:potassium - 18:18:18). This NPK application was also repeated after 1 and 2 months of growth using a drip irrigation system. In addition, plants were supported with supplements of commercial EC fertilizers of İGSAŞ brand calcium nitrate (Ca(NO3)2) and Agrosol Max brand magnesium sulphate (MgO 16% and SO3 32%) during the bloom period. It is obvious that environmental factors are also important, as are agronomic practices. At the time of harvest, the average temperature, daylight hours, and humidity were 23.3°C, 10.6 h, and 44.9%, respectively. Approximately 2500 varied tomato lines were obtained under these conditions. Initially, the trial was established with 50 plants in each line at M3 generation. As a result of positive and negative selection, observations were continued on 29 mutant lines selected at the M4 stage. For this purpose, plantations were established in M4, M5, and M6 generations with 100 plants in each line. The plantation was established according to a randomized plot design for each generation. After seasonal selection periods, 29 mutant lines and 1 control at the M4 were harvested by hand without plucking the fruit stalk in late August and early September. 75 tomato fruit samples from each mutant line were randomly selected at the same growth and ripeness stages for the phytonutrient analysis. The fruits were chopped to ensure each sample was representative and then stored at -86°C until extraction. For vitamin C, a further 75 randomly selected tomato fruits from each line were stored at -21°C until analysis. All chemicals were HPLC-grade and supplied by Merck Chemicals Ltd. (Poole, UK). Standard chemicals for vitamin C (L-ascorbic acid), lutein, lycopene, α-tocopherol, β- tocopherol, γ- tocopherol, δ- tocopherol were purchased from Sigma-Aldrich (St. Louis, USA). At M4 stage yield, fruit firmness, total soluble solid %, Brix analysis carried out on selected 16 mutant lines according to phytonutrient analysis and control. For these analysis 75 fruits were used for each line.
The method described by Abushita and his co-workers (Abushita et al., 1997) was used for the extraction of vitamin C, carotenoids, and tocopherols from tomato samples. However, some modifications were made, especially to the vitamin C extraction protocol. Carotenoids and tocopherol extractions were made with the liquid-liquid partition technique, whereas vitamin C extractions were made with accelerated solvent extraction. All extractions were made just before the injection. Extracts were kept cold and protected against daylight during the sample preparation steps and analysis. Sample preparation was done from each sample in duplicate, and each extract was injected in triplicate. After extraction, samples were immediately injected to eliminate the decomposition loss. Two separate HPLC systems were used in the tocopherol and carotenoid analyses. Tocopherols and carotenoids were extracted sequentially. To eliminate the storage time effect on the analysis results, the first system was dedicated to tocopherol analysis, and the second system was dedicated to carotenoid analysis.
A novel extraction method was utilized for the vitamin C content of mutant and control tomato lines. In the extraction of vitamin C with an accelerated solvent extraction system (ASE) (Dionex ASE 350 model), 5 g of frozen sample was weighed into a stainless steel 5 mL ASE cell and placed in the system. The samples were extracted with an aqueous solution of metaphosphoric acid (MPA) (3% w/v). It was carried out at ambient temperature under high pressure (1500 psi) of nitrogen gas. Each sample was kept 5 min at static time and was subjected to two cycle extractions. After collecting a known volume of extract in 90 seconds of purge time, it was filtered to remove the suspended fruit debris through a 0.45 µm pore-sized hydrophilic membrane filter and then vialled (using amber-colored vials) for further analysis. After two cycles of extraction with ASE and purification with sodium sulphate (NaSO4), the extract was immediately injected into HPLC to prevent the decomposition of L-ascorbic acid into dehydroascorbic acid. No other sample purification prior to chromatographic determination was necessary. The recovery of vitamin C extraction with ASE was validated with a synthetically prepared sample and was found to be 97%. In the analysis, a Waters HPLC system with a Waters 2695 Separations Module and a Waters 2996 Photodiode Array Detector controlled by Empower Pro® software was used. The detector recorded the signals between 190 and 400 nm. However, the peak of vitamin C was extracted and quantified at 243.8 nm. In the analysis, an EC 150/4.6 Nucleosil, 100-3 C18 Nautilus analytical column, and gradient elution with a flow rate of 0.7 mL/min were applied. 10 µL of sample was introduced into the column with the mobile phase of 1% (w/v) KH2PO4 in water and acetonitrile. Eluting conditions expressed as a proportion of 1% KH2PO4 in water are: 0.01-3 min: 99%, 10 min: 70%, 15 min: 60%, and 18-20 min: 99%. Standard L-ascorbic acid (vitamin C) solutions were prepared in 3% MPA and injected to a HPLC column under the same chromatographic conditions as in the samples (Gnayfeed et al., 2001).
A homogenized sample (2.5 g) was weighed into a crucible mortar and crushed with quartz sand. Then, 20 mL of methanol was gradually added with continuous crushing. The supernatant was carefully decanted. The residue was continuously crushed with the gradual addition of a mixture of methanol and dichloroethane (10:50, v/v) and was poured into the flask along with the previous one. Approximately 3-5 mL of deionized water was added to the flask and shaken. Then, the sample was gently transferred into the separator funnel for liquid-liquid partitioning. The higher solvent layer containing the carotenoids was separated, and residual water was removed through an anhydrous sodium sulfate (Na2SO4) column. The Na2SO4 column was then washed with 5 mL of dichloroethane. The solvent was evaporated under vacuum (100–150 mBar) at 40°C by a rotary evaporator (Büchi Rotavapor® R-210/R-215, Switzerland) until dryness was achieved. The residue was dissolved in 5 mL of acetone and filtered using a 0.45 µm-pore membrane filter to remove the suspended solids, then filled into the brown (amber-colored) vial for further analysis. A Varian ProStar HPLC system and UV detector controlled with Varian Workstation® software were used for the carotenoid analysis. 475, 465, and 456 nm wavelengths were used to extract and quantify the peaks of lycopene, lutein, and b-carotene from the sample chromatogram, respectively. In the analysis, Nucleodur C18 Isis, a 3, 150/4.6 mm analytical column, and gradient flow with a flow rate of 0.7 mL/min were applied. 10 µL of sample moved into the column with the mobile phases of deionized water and acetone. Eluting conditions expressed as a proportion of deionized water: 0.01-3 min: 20%, 8–12 min: 12%, 20–25 min: 7%, 28–30 min: 20% (Abushita et al., 2000).
The same extraction protocol for carotenoids was used for the tocopherol extractions. The extracted lipid fraction was saponified by refluxing with 5 mL of 30% (w/v) KOH in methanol and 20 mL of methanol for 35 min at the boiling point of methanol in the presence of 0.5 mg of L-ascorbic acid. After cooling, 15 mL of a 20% (w/v) NaCl solution was added, and the analogues of tocopherol were extracted twice with 40 mL of n-hexane in a separator funnel. The n-hexane fractions were washed twice with deionized water and dried over an anhydrous Na2SO4 column, and then the Na2SO4 column was washed with 5 mL of n-hexane. The solvent was evaporated under vacuum (100–150 mBar) at 40°C by a rotary evaporator until it reached dryness. The residue was dissolved in 5 mL of n-hexane and filtered with a 0.45 µm pore-sized hydrophobic membrane filter to remove the suspended solids, then filled into the brown vial. In the analysis, a Waters HPLC system with a Waters 2695 Separations Module and a Waters 2475 Multi-L Fluorescence Detector controlled with Empower Pro® software were used. The detector wavelength was adjusted to 295 and 320 nm for excitation and emission, respectively. In the analysis, Nucleosil 100 silica (5 mm), 250/4.6 mm analytical column, and isocratic elution with a flow rate of 0.9 mL/min were applied. 10 µL of sample was injected into the column with the mobile phases of n-hexane and absolute ethanol (500: 3.5; v:v). In the evaluation of the results, β-tocopherol in samples was quantified as γ-tocopherol equivalent and used as specified in further discussions (Abushita et al., 2000).
For the analysis, 75 fruits taken from each line at the same maturity and development period were passed through a blender. The fruit juice was filtered with coarse filter paper, the fruit juice taken from the filtrate was measured using a Krüss brand refractometer, and the Brix value was read. Experiments were set up with three replicates and nine samples for each replicate.
At the same development and maturity stage, 75 fruits were collected from each mutant line and control fruits. They were measured with a penetrometer with 5 replications and 15 fruits in each replication. The evaluation of the collected data was done according to Kader et al. (1978).
Seventy five fruits were passed through the blender for both the control and 16 selected mutant lines. Samples (5 g) were placed in 6 cm-diameter Pyrex Petri dishes with three replications and 10 samples for each replication. After measuring the wet weights of the samples in tared petri dishes, they were kept in ovens at 105 ± 1°C. Dry sample weights were determined for the samples that were completely dried after 24 h, and the total dry matter amount was calculated proportionally.
A randomized experimental plot design with three repetitions and 100 plants in each replicate was used to set up the yield experiments. Fruits were weighed after gradual collection over the same maturation time to compare the yield values of the mutant lines and the control.
ANOVA and Duncan’s MRT were used to evaluate the chromatographic results statistically in terms of variance analysis between groups. Experiments were set up in a completely randomized way. Data on vitamin C, carotenoids, and tocopherols, fruit firmness, brix, total soluble solids, and yield were evaluated by the ANOVA statistical program under MINITAB software, and differences in groups were determined with Duncan’s MRT program under MSTATC at the p = 0.01 level.
Shelf-life properties are the main concern for the tomato industry and consumers, even when the phytonutrient quality is high. Since both properties are desired, their improvement is essential in the mutation breeding process. For this purpose, field-grown tomato seeds were irradiated to generate the different mutant lines and then planted for selection. At the M4 stage, all genetically modified properties were stabilized and ready for physical and phytonutrient characterization studies. With this purpose, phytonutrient capacity analyses of mutant lines were investigated by HPLC, and the results were then statistically evaluated. In addition to chemical tests, yield, fruit hardness, brix content, and total soluble solid content of chosen mutant lines and control plants were assessed.
The extraction protocol of Abushita (2000) was applied to all stored mutant tomato lines for the analysis of α-, β-, γ-, δ-tocopherols. After extraction, samples were immediately injected to eliminate the decomposition loss. All chromatograms were quantified, and the extraction results and their statistical evaluations were presented in Table 1. Tocopherol (alpha) values of 28 samples were higher than those of the control. While α-tocopherol contents in mutant tomato lines ranged between under level of detection (LOD)-3.04 mg/100g, β-, γ-, and δ-tocopherol contents in mutant tomato lines ranged between under LOD-1.06 mg/100g and under LOD-0.10 mg/100g, respectively. However, the values of α-, β-, γ-, and δ-tocopherols in the control were 0.14, 0.55, 0.55, and 0.06 mg/100g, respectively (Table 1).
In the α-tocopherol analysis, 8-52 was found to have the highest content when compared with the other lines and control (0.14 mg/100 g), forming about 3.04 mg/100 g of the edible portion. In addition, statistically significant differences were determined. 9-31 and 8-35 mutant lines were the next higher lines, with phytonutrient capacities of 2.57 and 2.45 mg/100 g, respectively. β- tocopherol and γ- tocopherol analysis results were compared with the control (0.55 mg/100 g), mutant lines of 8-135 (1.06 mg/100 g), 8-159 (1.05 mg/100 g), and 8-50 (0.92 mg/100 g) were found to be the highest content mutant lines, respectively. Mutant lines were significantly different when compared with the control (p<0.01). However, these results are statistically insignificant when compared between lines. When δ- tocopherol results were evaluated statistically, the differences between the results revealed that either mutant lines or control line were insignificant (p>0.01). This result indicated that tomato lines with different characteristics (either positive or negative) were generated by radiation-induced mutation.
Mutant tomato lines and control were analyzed by HPLC to better understand the improvement in the carotenoid contents. In this regard, all lines stored at -80°C were taken to extraction. After extraction, samples were introduced to the HPLC system, and results were summarized in Table 1. As could be seen from Table 1, the lutein, β-carotene, and lycopene content values of the control were 0.29, 0.96, and 15.91 mg/100 g of edible portion, respectively. However, the lutein, β-carotene, and lycopene content values of mutant tomato lines were in the range of 0.11-0.47, 0.25-1.65, and 5.94-33.10 mg/100g of edible portion, respectively. The carotenoid values of mutant lines of 17 of 29, 8 of 29, and 14 of 29 were compared with the control and were found to be higher values for lutein, β-carotene, and lycopene, respectively. In Table 1, the highest lycopene content of 33.10 mg/100 g of edible portion was obtained at the 8-127 mutant line, which was more than twofold that of the control, whereas the control was 15.91 mg/100 g of edible portion. This mutant line also contained the highest content of β-carotene, which was about 1.65 mg/100g of edible portion. A significant difference was found at the mutant 8-127 line. The highest lycopene content was recorded in mutant lines 8-127 followed by 8-90 and 8-135. In contrast, the lowest lycopene content was recorded in mutant lines 8-13 (5.94 mg/100g of edible portion). Lutein results were evaluated statistically and the differences between the results in both mutant lines and control were found to be insignificant (Table 1). Petrov et al. (2013), Tomlekova et al. (2009b) and Tomlekova et al. (2021) obtained the same results on mutant pepper lines. On tomato mutant lines that were examined for β-carotene capacity, results showed the induced mutant lines have higher β-carotene content than control (Tomlekova et al., 2014).
Vitamin C, analyzed by HPLC is one of the important major phytonutrients in tomato. As it is well known, vitamin C is very sensitive to daylight and oxygen. Therefore, the vitamin C extraction and analysis period must be kept as short as possible. The result of the ASE method utilized for vitamin C extraction demonstrated that it had a faster extraction time than any other extraction technique and significantly more stable vitamin C in aqueous solution. The total elapsed time from the beginning to the extraction and end of the analysis was about 30 minutes, with a recovery rate of 97%. On the other hand, to differentiate and quantify the L-ascorbic acid and the dehydroascorbic acid, standard solutions of both acids were injected separately. But each peak was at the same retention time (3.41 min.) and both overlapped. In the calculation of total vitamin C content, L-ascorbic acid and dehydroascorbic acid were taken into account together and added to the other quantities (Llyod et al., 1987). Therefore, the peak extracted at 243.8 nm at the retention time of 3.41 min was assumed to be the total vitamin C content containing both acid quantities (Table 1). Vitamin C content in mutant tomato lines was found to range between under LOD and 37.34 mg/100 g, while in control it was 7.57 mg/100g of edible portion. On the other hand, the world average of vitamin C content in tomato is in the range of 10–20 mg/100 g of edible portion (Rucker et al., 2001). The result demonstrated that new varieties above control and the world average were generated by radiation-induced mutations. In Table 1, statistical significance with control was revealed by mutants, and it was found that 8–90 had the highest vitamin C content of 37.34 mg/100 g of edible portion, whereas control was 7.57 mg/100 g of edible portion. It was higher than the other mutant lines. It was also found that their statistical differences were significant. Mutant lines 8-86 and 8-208 were the next higher mutant lines, with 34.46 and 32.53 mg/100 g of edible portion, respectively. The differences between control and mutant lines were significant in terms of vitamin C content (Table 1). The tomato variety known as Co3 (MVD, 2023), which was created and registered through mutation breeding, has high values in terms of vitamin C content, according to an examination of the IAEA’s mutant variety database. As a result, it is clear that it is a useful tool because of the sensitivity of tomato to mutation to increase the nutritional content.
After calculation of the phytonutrient contents of mutant lines and controls, the values of control and highest content were summarized in Table 2. An improvement in the phytonutrient capacity was achieved through radiation-induced mutation presented in Table 2. In addition, the number of positive and negative variations was also determined and shown in Figure 1. In Figure 1, some of the 29 mutant lines had higher and some had lower variations than the control. Wang et al. (2022) reported that the expected vitamin C and lycopene content for a standard tomato variety should be 12.7 mg/100 g and 20.54 mg/100 g, respectively. In this study, it was determined that these values were 37.34 mg/100 g and 33.10 mg/100 g, respectively, in mutant lines developed after mutation breeding (Table 3). This was the nature of radiation-induced mutation breeding. Because radiation randomly interacts with genes and generates different varieties of mutant lines (Novak and Brunner, 1992; Tomlekova et al., 2014). 16 lines were chosen after the 29 lines' phytonutrient content was examined. Fruit hardness, yield, Brix, and the total soluble solids ratio were assessed in these 16 lines. According to Table 3, line 9–21 performed best in terms of fruit hardness and Brix, although its Brix content was lower than that of the control. Fruit yield and fruit firmness were distinguishing characteristics of Line 9–22. The highest yield values were seen on mutant lines 8-127 and 8-135 compared to all other lines and the control. No mutant line found exceeded the limit of the control sample for the amount of total soluble solid content.
Figure 1 Number of negative and positive deviation of phytonutrient contents from control sample at M4 stage.
Table 3 Yield, fruit firmness, brix and dry matter content results of 16 selected M4 stage mutants and control.
A total of 26 mutant varieties that have been reported in the online IAEA database (MVD, 2023) have been developed and registered, according to studies on tomato breeding by induced mutation. It becomes clear that traits like yield (cv. Lunch 1, S12, and Pusa Lal Meeruti, Bahar, Binatomato-13), transportation (fruit firmness) (cv. PKM1), total soluble solid (cv. Girón 50), fruit quality (cv. Magine), disease tolerance (cv. Ryugyoku and Kagyoku), drought tolerance (cv. Domi and Maybel), heath stress (cv. Summer King), Brix (cv. Giron 50 and Summer Star) (and earliness (cv. Lufanqie 7) are enhanced by mutation breeding (MVD, 2023; Sarsu et al., 2023), which is an efficient breeding technique in this regard.
Levin et al. (2006) reported that there are so many number of photomorphogenic mutants in tomato. These mutants have higher pigmentation capacity than wild types. Also all these mutants have higher level of carotenoids especially lycopene (Dono et al., 2022). In tomato breeding studies, it has been found that breeding materials with greater phytonutrient contents (such as lycopene and betacarotene) are better able to assess biotic and abiotic stress situations, and as a result, their yield value is higher (Cebolla-Cornejo et al., 2013; Hanson, 2016). The results of this research, whose conclusions we have reported, also show a similar parallelism with these investigations.
In this study, M4-stage mutant lines were analyzed for the determination of phytonutrient capacity by using an HPLC system with different types of detectors depending on the analyses (vitamin C, carotenoids, and tocopherols).The results showed that the induced mutation resulted in some variations in the phytonutrient capacity and other quality and yield parameters of mutant tomato lines. And also, an improvement was observed in the mutant lines when compared with the control. The average nutrient capacity was rather above the standard control. Eleven mutant lines were discovered to be significantly different from the control in terms of these eight phytonutrients, with the exception of lutein and delta tokoferol. The results showed that for the other quality characteristics, the eleven mutant lines performed better than the control and other selected lines. In mutation breeding studies, it is desired that all improvements in the physical and phytonutrient capacity of fruit be collected on a single genotype as much as possible. In this regard, it was considered that 9-22, 8-55, 8-135, and 8-127 were the most promising mutant lines to commercialize among the mutant lines. The findings of the research reported here and the studies on tomatoes so far demonstrate that mutation breeding is a successful tool, particularly for enhancing plant nutrients as well as yield metrics for tomatoes.
The original contributions presented in the study are included in the article/supplementary material. Further inquiries can be directed to the corresponding author.
KK: project leader; she did mutation breeding, sampling of the material, extraction stage, statistical analysis, and manuscript writing. EI: he worked on the extraction stage. DO: she worked on the extraction stage. FB: she worked on the extraction and HPLC analyses stages. EE: she worked on the HPLC stage. OK: he worked on the HPLC stage, data analysis of HPLC results, manuscript writing. MO: he was a coworker for Brix, fruit firmness, and total soluble solid analyses. All authors contributed to the article and approved the submitted version.
The authors wish to express their gratitude to both the Turkish Atomic Energy Authority (TENMAK-A2.H1.P14) and the International Atomic Energy Agency (IAEA-RER/05/017), which financially supported this work.
The authors declare that the research was conducted in the absence of any commercial or financial relationships that could be construed as a potential conflict of interest.
All claims expressed in this article are solely those of the authors and do not necessarily represent those of their affiliated organizations, or those of the publisher, the editors and the reviewers. Any product that may be evaluated in this article, or claim that may be made by its manufacturer, is not guaranteed or endorsed by the publisher.
Abushita A. A., Daood H. G., Biacs P. A. (2000). Change in carotenoids and antioxidant vitamins in tomato as a function of varietal and technological factors. J. Agric. Food Chem. 48, 2075–2081. doi: 10.1021/jf990715p
Abushita A. A., Hebshi E. A., Daood H. G., Biacs P. A. (1997). Determination of antioxidant vitamins in tomatoes. Food Chem. 60 (2), 207–212. doi: 10.1016/S0308-8146(96)00321-4
Ahloowalia B. S., Maluszynski M. (2001). Induced mutations – A new paradigm in plant breeding. Euphytica 118, 167–173. doi: 10.1023/A:1004162323428
Ahloowalia B. S., Maluszynski M., Nichterlein K. (2004). Global impact of mutation – derived varieties. Euphytica 135, 187–204. doi: 10.1023/B:EUPH.0000014914.85465.4f
Ali Y., Ibn Sina A. A., Khandker S. S., Neesa L., Tanvir M. V., Kabir A., et al. (2021). Nutritional composition and bioactive compounds in tomatoes and their impact on human health and disease: A review. Foods 10 (1), 45. doi: 10.3390/foods10010045
Alothman M., Bhat R., Karim A. A. (2009). Effects of radiation processing on phytochemicals and antioxidants in plant produce. Trends Food Sci. Tech. 20, 201–212. doi: 10.1016/j.tifs.2009.02.003
Balacheva E., Kartzeva T., Atanassova B., Tomlekova N. (2012). The diversity in tomato colours - potential for diversity in tomato nutritive quality. Acta Hortic. 960, 31–36. doi: 10.17660/ActaHortic.2012.960.2
Cebolla-Cornejo J., Roselló S., Nuez F. (2013). “Selection of tomato rich in nutritional terpenes,” in Natural Products. Eds. Ramawat K., Mérillon J. M. (Berlin, Heidelberg: Springer). doi: 10.1007/978-3-642-22144-6_127
Chang S. K. C., Holm E., Schwarz J., Rayas-Duarte P. (1995). Food. Anal. Chem. 67 (12), 127–153. doi: 10.1021/ac00108a008
Chaudhary J., Alisha A., Bhatt V., Chandanshive S., Kumar N., Mir Z., et al. (2019). Mutation breeding in tomato: advances, applicability and challenges. Plants 8 (5), 128. doi: 10.3390/plants8050128
Colak N., Kurt-Celebi A., Gruz J., Strnad M., Hayirlioglu-Ayaz S., Choung M. G., et al. (2022). The phenolics and antioxidant properties of black and purple versus white eggplant cultivars. Molecules 8), 2410. doi: 10.3390/molecules27082410
Datta S. K. (2023). Technology package for induced mutagenesis. Journal of biology and. Nature 15 (1), 70–88. doi: 10.56557/joban/2023/v15i18077
Dono G., Rambla J. L., Frusciante S., Fabene E., Gómez-Cadenas A., Granell A., et al. (2022). Pigment-related mutations greatly affect berry metabolome in San Marzano tomatoes. Horticulturae 8, 2, 120. doi: 10.3390/horticulturae8020120
Dorais M., Ehret D. L., Papadaopoulos A. (2008). Tomato (Solanum lycopersicum) health components: from the seed to the consumer. Phytochem. Rev. 7, 231–250. doi: 10.1007/s11101-007-9085-x
Esengun K., Erdal G., Gündüz O., Erdal H. (2007). An economic analysis and energy use in stake-tomato production in Tokat province of Turkey. Renew. Ener. 32, 1873–1881. doi: 10.1016/j.renene.2006.07.005
Fentik D. A. (2017). Review on genetics and breeding of tomato (Lycopersicon esculentum Mill). Adv. Crop Sci. Tech 5, 306. doi: 10.4172/2329-8863.1000306
Gnayfeed M. H., Daood H. G., Biacs P. A., Alcaraz C. F. (2001). Content of bioactive compounds in pungent spice red pepper (Paprika) as affected by ripening and genotype. J. Sci. Food. Agric. 81, 1580–1585. doi: 10.1002/jsfa.982
Hanson P. M. (2016). Genetic Improvement of Tomato (Solanum lycopersicum L.) for Phytonutrient Content at AVRDC - The World Vegetable Center. Ekin J. Crop Breed. Genet. 2 (2), 1–10.
Hernández M. H., Rodríguez E. R., Romero C. D. (2008). Chemical composition of tomato (Lycopersicon esculentum) from Tenerife, the Canary Islands. Food Chem. 106, 1046–1056. doi: 10.1016/j.foodchem.2007.07.025
Kader A. A., Morris L. L., Chen P. (1978). Evaluation of two objective methods and subjective rating scale for measuring tomato firmness. J. Amer. Soc Hortic. Sci. 103, 70–73. doi: 10.21273/JASHS.103.1.70
Kaushik P., Andújar I., Vilanova S., Plazas M., Gramazio P., Herraiz F. J., et al. (2015). Breeding vegetables with increased content in bioactive phenolic acids. Molecules 20, 18464–18481. doi: 10.3390/molecules201018464
Kornienko S., Ivchenko T., Gurin M., Volkova N. (2014). “Realization of genetic potential for mutant variability in tomato breeding,” in Mutagenesis: exploring genetic diversity of crops. Eds. Tomlekova N. B., Kozgar N., Wani M. R. (The Netherlands: Wageningen Academic Publisher) 57–76. doi: 10.3920/978-90-8686-796-7_3
Laskar R. A., Chaudhary C., Khan S., Chandra A. (2018). Induction of mutagenized tomato populations for investigation on agronomic traits and mutant phenotyping. J. Saudi Soc. Agric. Sci. 17 (1), 51–60. doi: 10.1016/j.jssas.2016.01.002
Levin I., Ric de Vos C. H., Tadmor Y., Bovy A., Lieberman M., Oren-Shamir M., et al. (2006). High pigment tomato mutants—more than just lycopene. Israel J. Plant Sci. 54, 179–190. doi: 10.1560/IJPS_54_3_179
Llyod L., Warner F. P., White C. A., Kennedy J. F. (1987). Quantitative reverse phase HPLC analysis of L-ascorbic acid (Vitamin C) and identification of its degradation products. Chromatographia 24, 371–376. doi: 10.1007/BF02688509
Marti R., Roselló S., Cebolla-Cornejo J. (2016). Tomato as a source of carotenoids and polyphenols targeted to cancer prevention. Cancers 8, 58. doi: 10.3390/cancers8060058
MVD (2023). Available at: https://nucleus.iaea.org/sites/mvd/SitePages/Search.aspx (Accessed 10.06.2023).
Natalini A., Acciarri N., Cardi T. (2021). Breeding for nutritional and organoleptic quality in vegetable crops: The case of tomato and cauliflower. Agriculture 11, 606. doi: 10.3390/agriculture11070606
Novak F. J., Brunner H. (1992). Plant breeding: induced mutation technology for crop improvement. IAEA Bull. 4, 25–33.
Petrov V., Denev I., Draganov M., Timin O., Panchev I., Tomlekova N. (2013). Molecular characterization of advanced mutants for early detection of high β-carotene content in pepper breeding programs. Sci. Agraires 66 (2), 303–310. doi: 10.7546/CR-2013-66-2-13101331-20
Poehlman J. M., Sleeper D. A. (1995). Breeding Field Crops. 4th Ed (New Delhi, Bangolore: Panima Publishing Corp.).
Quartey E. K., Amoatey H. M., Achel D. G., Klu G. Y. P., Mba R. A. (2012). Induced mutations for improved lycopene, total antioxidant properties and other quality factors in wild tomato (Solanum pimpinellifolium L.). Advance J. Food Sci. Technol. 4 (4), 182–188.
Rucker R. B., Suttie J. W., McCormick D. B., Machlin L. J. (2001). Handbook of Vitamins (New York: Marcel Dekker).
Saini R. K., Rengasamy K. R., Mahomoodally F. M., Keum Y. S. (2020). Protective effects of lycopene in cancer, cardiovascular, and neurodegenerative diseases: An update on epidemiological and mechanistic perspectives. Pharmacol. Res. 155, 104730. doi: 10.1016/j.phrs.2020.104730
Salehi B., Sharifi-Rad R., Sharopov F., Namiesnik J., Roointan A., Kamle M., et al. (2019). Beneficial effects and potential risks of tomato consumption for human health: An overview. Nutrition 62, 201–208. doi: 10.1016/j.nut.2019.01.012
Sarsu F., Penna S., Nikalje G. C. (2023). “Strategies for screening induced mutants for stress tolerance,” in Mutation Breeding for Sustainable Food Production and Climate Resilience. Eds. Penna S., Jain M. J. (Finland: Springer) 151–176. doi: 10.1007/978-981-16-9720-3
Silva R. M. G., Figueiredo C. C. M., Gomes A. C., De Mello Peixoto E. C. T., Ferreira P. C., Silva L. P. (2016). Mutagenicity, antioxidant activity and nutritional content of long-life tomato. Biosci. J. Uberlândia 32 (4), 999–1007. doi: 10.14393/BJ-v32n4a2016-32806
Tarrago-Trani M. T., Phillips K. M., Cotty M. (2012). Matrix-specific method validation for quantitative analysis of vitamin C in diverse foods. J. Food Comp. Analy. 26, 12–25. doi: 10.1016/j.jfca.2012.03.004
Tomlekova N. B., Panayotov N., Kozgar M. I., Wani M. R., Serdaris P., Balacheva E. (2014). “Inducing and exploring new mutant donors of tomato,” in Mutagenesis: exploring genetic diversity of crops. Eds. Tomlekova N. B., Kozgar N., Wani M. R. (The Netherlans: Wageningen Academic Publisher) 283–306. doi: 10.3920/978-90-8686-796-7_14
Tomlekova N., Spasova-Apostolova V., Pantchev I., Sarsu F. (2021). Mutation associated with orange fruit color increases concentrations of β-Carotene in a sweet pepper variety (Capsicum annuum L.). Foods 10, 6, 1225. doi: 10.3390/foods10061225
Tomlekova N., Todorova V., Petkova V., Yancheva S., Nikolova V., Panchev I., et al. (2009a). “Creation and evaluation of induced mutants for pepper breeding programmes,” in Proceedings of FAO/IAEA International Symposium on Induced Mutations in Plant. Ed. Shu Q. Y. (Rome: Food and Agriculture Organization of the United Nations), 187–190.
Tomlekova N., Timina O., Timin O. Y. (2009b). Achievements and perspectives of sweet pepper breeding towards high beta-carotene. Acta Hortic. 830 (830), 205–212. doi: 10.17660/ActaHortic.2009.830.28
Tripodi P. (2022). Next generation sequencing technologies to explore the diversity of germplasm resources: Achievements and trends in tomato. Comput. Struct. Biotechnol. J. 20-, 6250–6258. doi: 10.1016/j.csbj.2022.11.028
TUIK (2022). Available at: https://data.tuik.gov.tr/Bulten/Index?p=Bitkisel-Uretim-Istatistikleri-2022-45504 (Accessed 12.02.2023).
van Harten A. M. (1998). “The use of radiation for mutation induction,” in Mutation Breeding Theory and Practical Applications (United Kingdom: Cambridge University Press), 111–135.
Vu T. V., Swati D., Thi T. M., Chan H. J., Jae-Yean K. (2020). Precision genome engineering for the breeding of tomatoes: recent progress and future perspectives. Front. Genome Editing, 2, 612137. doi: 10.3389/fgeed.2020.612137
Wang C., Li M., Duan X., Abu-Izneid T., Rauf A., Khan Z., et al. (2022). Phytochemical and nutritional profiling of tomatoes; impact of processing on bioavailability - A comprehensive review. Food Rev. Int. doi: 10.1080/87559129.2022.2097692
Keywords: radiation, induced mutation, phytonutrient, tomato, HPLC
Citation: Kantoğlu KY, İç E, Özmen D, Bulut FŞ, Ergun E, Kantoğlu Ö and Özçoban M (2023) Gamma rays induced enhancement in the phytonutrient capacities of tomato (Solanum Lycopersicum L.). Front. Hortic. 2:1190145. doi: 10.3389/fhort.2023.1190145
Received: 20 March 2023; Accepted: 11 July 2023;
Published: 03 August 2023.
Edited by:
Aamir Raina, Aligarh Muslim University, IndiaReviewed by:
María Serrano, Miguel Hernández University of Elche, SpainCopyright © 2023 Kantoğlu, İç, Özmen, Bulut, Ergun, Kantoğlu and Özçoban. This is an open-access article distributed under the terms of the Creative Commons Attribution License (CC BY). The use, distribution or reproduction in other forums is permitted, provided the original author(s) and the copyright owner(s) are credited and that the original publication in this journal is cited, in accordance with accepted academic practice. No use, distribution or reproduction is permitted which does not comply with these terms.
*Correspondence: K. Yaprak Kantoğlu, a2Fkcml5ZXlhcHJhay5rYW50b2dsdUB0ZW5tYWsuZ292LnRy
Disclaimer: All claims expressed in this article are solely those of the authors and do not necessarily represent those of their affiliated organizations, or those of the publisher, the editors and the reviewers. Any product that may be evaluated in this article or claim that may be made by its manufacturer is not guaranteed or endorsed by the publisher.
Research integrity at Frontiers
Learn more about the work of our research integrity team to safeguard the quality of each article we publish.