- 1International Centre of Insect Physiology and Ecology (icipe), Nairobi, Kenya
- 2Groupement d'intérêt économique (GIE), Ziguinchor, Senegal
- 3USAID's Bureau for Humanitarian Assistance (BHA) Technical and Program Quality (TPQ), Washington, DC, United States
Introduction: Horticulture is an important sub-sector for many African countries. Tephritid fruit flies continue to cause serious direct and indirect damage to the sub-sector. The male annihilation technique (MAT) using methyl eugenol (ME) is among the most revered techniques in fruit fly management. However, ME-based lures are only available to some farmers in Africa. Several basils of the genus Ocimum produce ME.
Methods: In laboratory experiments, we used a dual choice olfactometer to assess the attraction of adult oriental fruit fly Bactrocera dorsalis to intact plants, 100 µL commercial methyl eugenol (ME) (positive control), 10 mg/mL of O. tenuiflorum aqueous extract (Otae), and a negative control (blank). Large arena experiments were conducted to compare the responses of four fruit fly species of economic importance in Africa to Otae-baited traps.
Results: In dual choice olfactometer assay, more B. dorsalis males (78.6%) than females (23.8%) were attracted to Otae. Most B. dorsalis males were attracted to the positive control (90.5%) and 10 mg/mL Otae (88.5%) compared to the whole plant (84.0%). In large arena experiments, Otae attracted the most B. dorsalis males (47.7%), followed by Zeugodacus cucurbitae males (25.1%), while males of Ceratitis species were the least attracted. The analysis of headspace volatiles from aerial plants, Otae and essential oil using gas chromatography-mass spectrometry detected 97 compounds. The intact plant without flowers, intact plant with flowers, Otae, and the O. tenuiflorum essential oil contributed 76, 62, 33 and 28 compounds, respectively. ME comprised the top three most abundant compounds, especially in the essential oil.
Discussion: Our study confirms the potential of O. tenuiflorum as a source of ME for tephritid fruit fly integrated management in Africa. Otae, a readily available alternative to commercial ME, can be used to develop fruit fly-baited traps, particularly for smallholder farmers.
Introduction
Horticulture is an important sub-sector for many African countries, contributing significantly to rural livelihoods by providing nutritious food, increased farm profits, and various ecosystem services (FAO, 2017). Despite of the ongoing improvement in the nutritional security, economies, and livelihoods of African countries, the sub-sector is highly constrained by the infestation of insect pests and resource inputs. Tephritid fruit flies, particularly the alien invasive, the oriental fruit fly B. dorsalis (Hendel) and Z. cucurbitae (Coquillett), as well as the native C. cosyra (Walker) and C. capitata (Wiedemann) continue to cause serious damage to the horticultural sub-sector in many countries across the globe, resulting in considerable economic losses (Ndlela et al., 2022). B. dorsalis was first detected in the continent during 2003 (Lux et al., 2003). It is the most damaging fruit fly, which has impeded mango production across Africa (Vargas et al., 2015; Mutamiswa et al., 2021) through direct yield losses in range from 30 to 100% (Nankinga et al., 2014; Ndlela et al., 2022). Furthermore, the pest causes massive indirect economic losses because of rejection of pest-infested fruits due to stringent quarantine restrictions imposed by lucrative global markets on exports from Africa (Ekesi et al., 2016).
The main control option for tephritid fruit fly pests is the use of synthetic chemical insecticides. This approach is not economically accessible to the low-income farmers, sustainable or effective because of the concealed nature of the pest and the development of resistance in pest populations (Couso-Ferrer et al., 2011). Furthermore, chemical pesticides can negatively affect co-habiting beneficial ecosystem service providers such as natural enemies and pollinators. Moreover, the injudicious application of these pesticides seriously compromises human, animal and environmental health. For example, methyl bromide, widely used to disinfect infected fruits, is known to deplete the ozone layer (Ristaino and Thomas, 1997). Therefore, there is a critical need for environmentally friendly, accessible and adaptable integrated pest management (IPM) strategies to limit the use of chemical pesticides against tephritid fruit flies.
IPM strategy includes multiple tactical and ecological prevention, monitoring and control processes, based on understanding the behavior, biology and ecology of fruit flies. IPM strategies suppress the fruit fly population in an environmentally friendly and cost-effective manner that generates higher yields of pest- and chemical-free fruits (Midingoyi et al., 2019; Niassy et al., 2022). Pest monitoring is the first step in IPM and relies on trapping techniques using lures or baits. The male annihilation technique (MAT) uses the para-pheromone methyl eugenol (ME), a natural compound occurring in a variety of plant species to attract the males of B. dorsalis (Vargas et al., 2000; Shelly et al., 2004; Shelly et al., 2014). ME can effectively attract male fruit flies within a 36-meter radius, especially B. dorsalis, and serves as a precursor compound for sex pheromone synthesis (Manoukis et al., 2015). ME feeding by males of the B. dorsalis complex enhances their mating competitiveness (Shelly et al., 2010; Haq et al., 2016). Its application in Lynfield or locally constructed traps decreases the number of male fruit flies in orchards, thus minimizing mating and subsequent production of viable eggs by females (Leblanc et al., 2011; Ndlela et al., 2016; Ullah et al., 2017). MAT using ME is compatible with other IPM methods, such as orchard sanitation, protein baits, biopesticides and parasitoids (Niassy et al., 2022).
Moreover, ME can be in an attract-and-kill strategy using soft-killing chemical insecticides or any other killing agents (Vargas et al., 2005; Vargas et al., 2010; Faye et al., 2021) or use in autodissemination devices with biopesticide to lure-and-infect. ME significantly reduces fruit fly populations and fruit infestations, as compared with areas with no interventions (Ballo et al., 2020). However, ME-based lures are unavailable to most farmers because of import procedures and associated prohibitive ME costs in African countries (Niassy et al., 2022).
The synthesizing of ME is expensive and highly toxic, as it requires the use of chemicals for the methylation process (Asnawati et al., 2015). To the best of our knowledge, there is no local producer of ME in Africa. For instance, in Kenya, the importing cost of 1 L ME is USD 80/kg, enough to produce 300 units of lure, with 12 units required for a hectare. If ME could be produced locally, then MAT kit would cost much less. When MAT is integrated with other management practices such as protein bait sprays, parasitoid releases and the use of augmentorium, it can result in the reduction of mango rejection by 54.5%, cost of insecticide per acre by 46.3% less and increased net income by 22.4% to mango farmers (Kibira et al., 2015). Most mango (Mangifera indica L.) producers are smallholders who may not afford the cost of imported ME to conduct area-wide monitoring of fruit fly populations. Therefore, identifying a plant base that produce ME could provide the resource-constrained fruit farmers with affordable alternative and enable them to sustainably manage the fruit flies to enhance the quality and yield of mango.
Several aromatic plants have been reported to produce ME, including basils of the genus Ocimum (Lamiaceae family), which are widespread in Asia, Africa, Central America and South America (Pandey et al., 2014; Srivastava et al., 2018). Some of these basil plants include O. tenuiflorum L., O. basilicum L., O. gratissimum L., O. kilimandscharicum Gürke, and O. africanum Sims, which are annul and perennial herbs cultivated for many uses, including cooking, traditional medicine and other (economic) benefits. Ocimum species contain a wide range of bioactive compounds with anti-cancer and anti-inflammatory properties and antimicrobial activities against various fungi and bacteria (Khair-ul-Bariyah et al., 2012). Furthermore, members of Ocimum genus contain ME in their chemical profiles (Dharmadasa et al., 2015; Singh et al., 2020). Formulations using these properties have been proposed in the health and nutrition industry (Kothari et al., 2006; Lam et al., 2018; Piras et al., 2018; Manjudevi et al., 2022). However, there are many varieties within the different species of the Ocimum genus, with different physical appearances and aromas, because of their extensive cultivation and use worldwide, which could mislead in their identification. While basil plants can produce ME, the comparative volatile headspaces of various stages of the plant, aqueous formulation, and essential oils, and the behavioral response of fruit fly species to these are poorly investigated. To better harness the potential of basil in attracting fruit fly, the botanical and molecular identity of this plant species, and the compounds responsible for the attraction to fruit flies, need to be elucidated. Additionally, the behavioral response to volatile headspace between male and female fruit flies across species that cause serious damage to fruits is not well understood in most cases. Thus, this study aimed to determine the relative responses of four fruit fly species of economic importance to aqueous solutions of O. tenuiflorum and to generate data that could form the basis for future studies on ME extraction from the plant.
Materials and methods
Study sites and cultivation of O. tenuiflorum
O. tenuiflorum, which has been reported to occur in Kenya, Malawi and Zambia (Datiles and Acevedo-Rodríguez, 2014) and Senegal (Author’s observation), was sourced from the wild, cultivated and maintained in greenhouses at the International Centre of Insect Physiology and Ecology (icipe), Nairobi, Kenya (01°13′26″S 36°53′48″E, 1,600 m above sea level). Plants were raised from seeds in a well-drained, rich, loam soil nursery bed (1 m by 1 m width). Three-week-old seedlings from the nursery bed were transplanted in 4 L plastic pots (Kenpoly Ltd., Nairobi, Kenya) containing farmyard manure and maintained at 12L:12D (light:dark) photo phase. Plants were watered daily using a sprinkler hose.
Plant collection and identification
Morphological identification
This study was conducted between February and December 2022 at icipe. The aerial parts (stem, leaves and inflorescence) of O. tenuiflorum were harvested during the active blooming period in February 2022. The plant was identified using plant morphological identification keys at the University of Nairobi, Kenya, and voucher specimens of the plants were deposited at the herbarium of the University of Nairobi.
Molecular identification
Leaf samples were surface sterilized using 3% bleach, rinsed three times with distilled water, then blot dried on 90 mm Whatman filter paper. Genomic DNA was extracted from individual leaf samples using the Isolate II Plant DNA Kit (Bioline, London, UK) following the manufacturer’s instructions. The resultant DNA was eluted in a final 50 μL volume, and quality and quantity checks were performed using the Nanodrop 2000/2000c Spectrophotometer (Thermo Fischer Scientific, Wilmington, USA). The maturase K (matK) gene region was targeted through polymerase chain reaction (PCR) using 3F_Kim 5’ CGTACAGTACTTTTGTGTTTACGAG 3’ and 1R_Kim 5’ ACCCAGTCCATCTGGAAATCTTGGTTC 3’ (Ki-Joong Kim, unpublished) primers, while the chloroplast large subunit of ribulose biphosphate carboxylase (rbcL) gene region was amplified using rbcL-Fw 5’ATGTCACCACAAACAGAAACTAAAGC 3’ (Fay et al., 1998) and rbcL-z895R 5’ACCATGATTCTTCTGCCTATCAATAACTGC 3’ (Crane et al., 1995) markers.
The PCR tests were carried out in a total reaction volume of 20 µL containing 5X My Taq reaction buffer (5 mM dNTPs, 15 mM MgCl2, stabilizers and enhancers), 0.5 pmol µL-1 of each primer, 0.5 mM MgCl2, 0.0625 U µL-1 My Taq DNA polymerase (Bioline) and 15 ng µL-1 of DNA template. These reactions were set up in the Nexus Mastercycler gradient thermocycler (Eppendorf, Hamburger, Germany). The following cycling conditions were used: initial denaturation for 2 min at 95°C, followed by 40 cycles of 30 sec at 95°C, 30 s annealing (49°C for matK 3F/1R Kim and 55.7°C for rbcL) and 1 min at 72°C, then a final elongation step of 10 min at 72°C. The gene fragment targeted for matK was 889 bp, while for rbcL, it was 900 bp.
The amplified PCR products were resolved through a 1.2% agarose gel. DNA bands on the gel were analyzed and documented using KETA GL imaging system trans-illuminator (Wealtec, Meadowvale Way Sparks, USA). Successfully amplified products were excised and purified using Isolate II PCR and Gel Kit (Bioline), following the manufacturer’s instructions. The purified samples were shipped to Macrogen (Meibergreef, Amsterdam, the Netherlands) for bi-directional sequencing.
The successful sequences were assembled and edited using Geneious Version 8 (Dotmatics, Boston, USA) (Kearse et al., 2012). The primer sequences were identified and removed from the consensus sequences generated from both the forward and reverse reads. For conclusive identification of the species from both markers, similarity searches were conducted by querying the consensus sequences via Basic Local Alignment Search Tool (BLAST) at the GenBank database, hosted by the National Centre of Biotechnology Information (NCBI) (Maryland, United States). The BLAST algorithm finds regions of local similarity between sequences, in which consensus sequences were compared with reference sequences in the GenBank database, in addition to the Barcode of Life Database (BOLD) (Ratnasingham and Hebert, 2007).
Extraction of essential oil of O. tenuiflorum
The dried aerial parts (stem, leaves and inflorescence) of O. tenuiflorum were extracted by hydro-distillation, using the Clevenger apparatus (Shiva Scientific Glass Pvt. Ltd., New Delhi, India). Approximately 350 g of the dried plant materials was put into a 6 L round-bottom glass flask, and water was added at the ratio of 1:3 (plant sample:water) (Ochola et al., 2022). The flask was then fitted with the Clevenger apparatus and a double pocket condenser, and then hydro-distilled for 4 h. The crude essential oil was collected over a water layer in hexane. The organic and aqueous layers were then left to separate in a separating funnel. The hexane extract was then dried with anhydrous sodium sulfate to remove traces of water, filtered, and the solvent removed by distillation under reduced pressure. The essential oil yield was determined and stored in amber-colored vials at 4°C until subsequent use.
Preparation of O. tenuiflorum aqueous extract (Otae)
Leaves (50 g) were harvested from one-month-old O. tenuiflorum. The levels of essential oils are high after one to two months of transplanting of O. tenuiflorum (Sims et al., 2014). The harvested leaves were washed with distilled water to remove surface dirt, and an O. tenuiflorum aqueous extract (Otae) was prepared as follows: 5 g of fresh leaves was blended into a paste, using a domestic blender, with 100 mL distilled water, transferred into a clean 500 mL volumetric flask, and the volume topped up to the mark with distilled water. The Otae was shaken by hand for 30 s, sonicated at 20 kHz for 10 min, filtered through a 31.5-um nylon mesh to remove large particles, before centrifuging at 4,200 rpm for 10 min and the supernatants stored as crude Otae (10 mg/mL fresh leaves equivalent) for subsequent bioassays.
Source and rearing of fruit flies
B. dorsalis, Z. cucurbitae, C. capitata and C. cosyra were obtained from laboratory colonies kept at icipe and reared at 26 ± 2 °C, 50-60% relative humidity (RH), and a photoperiod of 12:12 h (L:D), following procedures described by Ekesi and Mohamed (2011). Briefly, adult fruit flies were fed on an artificial diet containing a mixture of finely ground sugar (Mumias Sugar Company, Nairobi, Kenya) and enzymatic yeast hydrolysate (USB Corporation, Cleveland, OH, USA) in a ratio of 3:1 sugar:hydrolysate. They were provided with mango domes (oviposition substrate) and water ad libitum in glass Petri dishes (90 × 15 mm) with pumice granules to prevent drowning. Colonies were supplemented with flies from the wild after every three months to reduce inbreeding and loss of genetic vigor.
Response of B. dorsalis to intact O. tenuiflorum plants in dual choice olfactometer assays
Bioassays were carried out using a dual choice olfactometer assay (30 × 30 × 100 cm), as previously described by Nyasembe et al. (2012), with some modifications. Briefly, activated charcoal-filtered and humidified medical air from a compressed air tank was passed over the samples, using a push-pull Gast pump (Gast Manufacturing, Benton Harbor, USA) at an airflow rate of 700 mL/min split equally into two. One half was passed through an enclosed airtight oven bag (Reynolds, Richmond, USA), containing the test arm comprised of either (a) an O. tenuiflorum intact plant, (b) 100 µL methyl eugenol impregnated on a 2 cm2 Whatman filter paper No.1, or (c) 250 mL (1, 5 and 10 mg/mL leaf equivalent) of Otae, while the other half was passed through an enclosed empty oven bag (control). At a rate of 700 mL/min, a vacuum line driven by a fan removed air from the center of the olfactometer.
For each bioassay, a group of 10 B. dorsalis males (10-15-day old) was placed in a releasing vial and kept for 10 min for acclimatization. Thereafter, the males were released through a hole at the center of the bottom of the wind tunnel and were allowed 20 min to make a choice. The base of the wind tunnel was marked from 0-50 cm on either side of the insect release point to allow for scoring to be noted. The insects that moved beyond 30 cm from the center on either side of the olfactometer were considered to have made a choice; hence, their numbers were recorded for further analysis. The same procedure was repeated with B. dorsalis females. The bioassay experiments were replicated 20 times, using different batches of fruit flies. After two successfully conducted bioassays, the air inlets were changed to avoid positional bias, and the interior of the olfactometer was cleaned using acetone and allowed to dry by passing air through both arms.
Behavioral experiments included: (i) responses of B. dorsalis females to negative control (blank); (ii) responses of B. dorsalis females to positive control (commercial ME) versus negative control; (iii) responses of B. dorsalis females to O. tenuiflorum intact plant headspace volatiles versus negative control; (iv) responses of B. dorsalis females to 100 µL methyl eugenol impregnated on a 2 cm2 Whatman filter versus negative control; (v) responses of B. dorsalis females to 250 mL (10 mg/mL) Otae versus negative control; (vi) responses of B. dorsalis males to similar controls and treatments described in i-iv; and (vii) responses of B. dorsalis males to 250 mL (1, 5, 10 mg/mL) of Otae versus negative control.
Response of B. dorsalis, B. curcubitae, C. capitata and C. cosyra to O. tenuiflorum aqueous extract (Otae) baited traps in room experiment
This experiment was carried out in an enclosed room measuring 3 × 1.5 m and fitted with a large, clear glass sealed window. A photoperiod of 12:12 h (L:D) cycle was maintained in the room, illuminated using four cool daylight fluorescent tubes (Osram L 58W/765, Munich, Germany) hung from the ceiling (0.5 m from the floor). The room conditions were similar to those used to rear fruit flies.
Four fruit fly traps, made from 500 mL clear water bottles (modified to eliminate visual cues), were baited with 250 mL (10 mg/mL) Otae and were hung 2 m from the floor. This was followed by the release of a specific number of a particular species of fruit flies in behavioral room experiments. The experiment was conducted separately and data collected included: (i) responses of B. dorsalis to 250 mL (10 mg/mL) Otae; (ii) responses of B. dorsalis to 100 µL ME; (iii) responses of B. dorsalis, B. curcubitae, C. capitata and C. cosyra to 250 mL (10 mg/mL) Otae; (iv) responses of B. dorsalis, B. curcubitae, C. capitata and C. cosyra to 100 µL of ME. Each experiment examined two hundred 10-15-day-old flies, with the ratio of males and females being 1:1. For control, 2 fruit fly traps with no baits were used.
Analysis of O. tenuiflorum aqueous extract (Otae) headspace volatiles
Otae (250 mL) was transferred into a 1 L volatile collection glass jar (Agricultural Research Service (ARS), Gainesville, USA). Activated charcoal-filtered and humidified air was passed over the Otae sample using a push-pull Gast pump at a 340 mL/min flow rate. The headspace volatiles were trapped onto pre-cleaned with GC-grade dichloromethane (DCM) HayeSep-Q® traps (30 mg, Analytical Research System, Gainesville, FL, USA) at a flow rate of 170 mL/min, using a Vacuubrand CVC2 vacuum pump (Vacuubrand, Wertheim, Germany) for 12 h. The HayeSep-Q traps were then eluted with 200 μL DCM into 2 mL clear glass vials, each containing 250 μL conical point glass inserts (Supelco, Bellefonte, USA) and immediately analyzed using GC-MS. All headspace volatile organic compounds were trapped in triplicates, using different batches of prepared Otae.
Analysis of O. tenuiflorum headspace volatiles
Aerial parts of 1-month-old plants without visible flowers or blooms were used. The aerial parts of intact plants were carefully sealed within an airtight oven bag and volatiles were collected, as described above.
Coupled gas chromatography-mass spectrometry (GC-MS) analysis
Volatile organic compounds (VOCs) were collected from intact O. tenuiflorum plants, either with or without flowers, and Otae and essential oils were identified by GC-MS on an HP 7890A series gas chromatograph, linked to an HP 5975C mass spectrometer operated in an electron impact ionization mode (70 eV). The instrument was fitted with an HP-5MS capillary column (30 m × 0.25 mm i.d., 0.25 μm film thickness: J and W Scientific, Folsom, USA). Helium was used as the carrier gas at a 1.2 mL/min flow rate. From each sample, 1 µL was injected in splitless mode, at an inlet temperature of 270°C. The oven temperature was programed from 35°C to 285°C, with an initial temperature being maintained for 5 min, with a ramp of 10°C/min to 285°C, and the held at this temperature for the duration of 50 min analysis time. The solvent DCM, used for elution, was similarly analyzed. The mass selective detector was maintained at an ion source temperature of 230°C, and a quadrupole temperature at 180°C. Fragment ions were analyzed over a mass range of 40-550 m/z. VOCs were identified by using their retention times and mass fragmentation spectra against authentic standards (where available). Others were tentatively identified by using matches from three published mass spectral libraries: Adams.1 (Diablo Analytical, Inc., Antioch, USA), Chemecol.l (Agilent, Santa Clara, CA, USA) and National Institute of Standards and Technology (NIST, Diablo Analytical, Inc., Antioch, USA) 05,08 and 11). A blend of alkanes (C6-C32) was injected and used to calculate the retention index, based on the equation of Van Den Dool and Kratz (1963):
where:
RI = retention index;
x = the name of the target compound;
n0 = n-alkane CnoH2no+2 directly eluting before x;
n1 = Cn1H2n1 + 2 directly eluting after x; and
RT = retention time.
Components identified in the blank volatile collections and elution solvent were excluded from the analysis. Serial dilutions of the authentic standard methyl eugenol (0.1-100 ng/μL) (≥ 98% Sigma-Aldrich, Munich, Germany) were analyzed by GC-MS to generate a linear calibration curve (peak area vs. concentration). The linear equation generated y=2000000x–169256; R2 = 0.9996 was used for the external quantification of the different VOCs.
Data analysis
Dataset on the percentages of insects that chose either arm of the olfactometer that contained either test treatments were tested if it was normally distributed (Shapiro-Wilks test: P>0.5) and analyzed using analysis of variance (ANOVA). Treatment included: intact plant headspace volatiles, Otae and the commercial ME, or the negative control (blank). In this analysis, the explanatory variables were B. dorsalis sexes, treatments and interaction of sexes and treatments while the response variable was percentages of B. dorsalis attracted to the treatments. Furthermore, dataset on the percentage of B. dorsalis, Z cucurbitae, C. capitata and C. cosyra that responded to commercial ME and Otae was normally distributed according to the Shapiro-Wilks test (P>0.5) and statistical difference among the four fruit fly species, between treatments (commercial ME and Otae) and interaction of fruit fly species and treatments were determined using ANOVA. Here, percentages of 330 fruit flies attracted to the treatments was used as response variable. Mean separations were performed for explanatory variable showing significance difference using the Tukey’s HSD (honestly significant difference) test. All statistical analyses were done using R software version 4.0.2 (R Core Team, 2020). The averages of the volatile release rates (ηg/plant/hr) of the compounds were calculated and are summarized in tabular form.
Results
Morphological and molecular characterization of O. tenuiflorum
Molecular and morphological identifications confirmed the tested specimen as O. tenuiflorum. The specimen had an erect plant height of 30-60 cm (12-24 inches), with hairy stems and many branches (Figure 1). A BLAST on NCBI indicated similarity values of 99%.
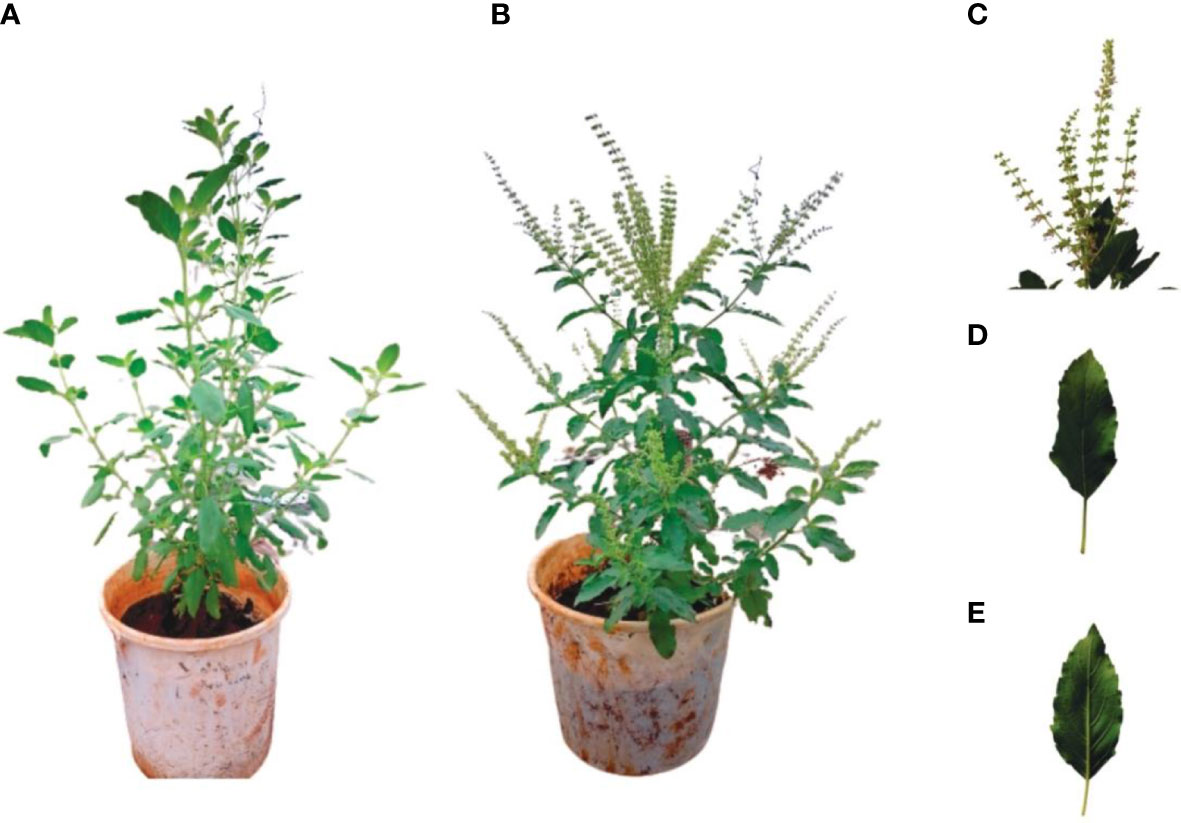
Figure 1 Some morphological characteristics of candidate Ocimum sp.; aerial shoot before (A) and (B) flowering, inflorescence (C), and upper (D) and lower (E) part of leaf.
Response of B. dorsalis to O. tenuiflorum intact plant headspace volatiles, ME and the aqueous extract (Otae)
Wind tunnel behavioral assays indicated that the responses of B. dorsalis to the different treatments (intact plant headspace volatiles, Otae and the two controls (positive control (commercial ME) and negative control (blank)) varied significantly between males and females (F = 384.48, df = 1, 158, P<0.001). Males of B. dorsalis (78.6 ± 1.7) were highly attracted to Otae as compared with females (23.8 ± 2.2%) were attracted to the treatments of Otae.
The highest percentage of males of B. dorsalis were attracted by positive control (ME 100 µL) (90.5 ± 3.1%) and 10 mg/mL Otae (88.5 ± 2.3%), compared with those attracted by the whole plant (84.0 ± 2.3) (Figure 2). On the other hand, more female B. dorsalis were attracted to the control (47.5 ± 1.7%) than to the test treatments (23.5-24.5%).
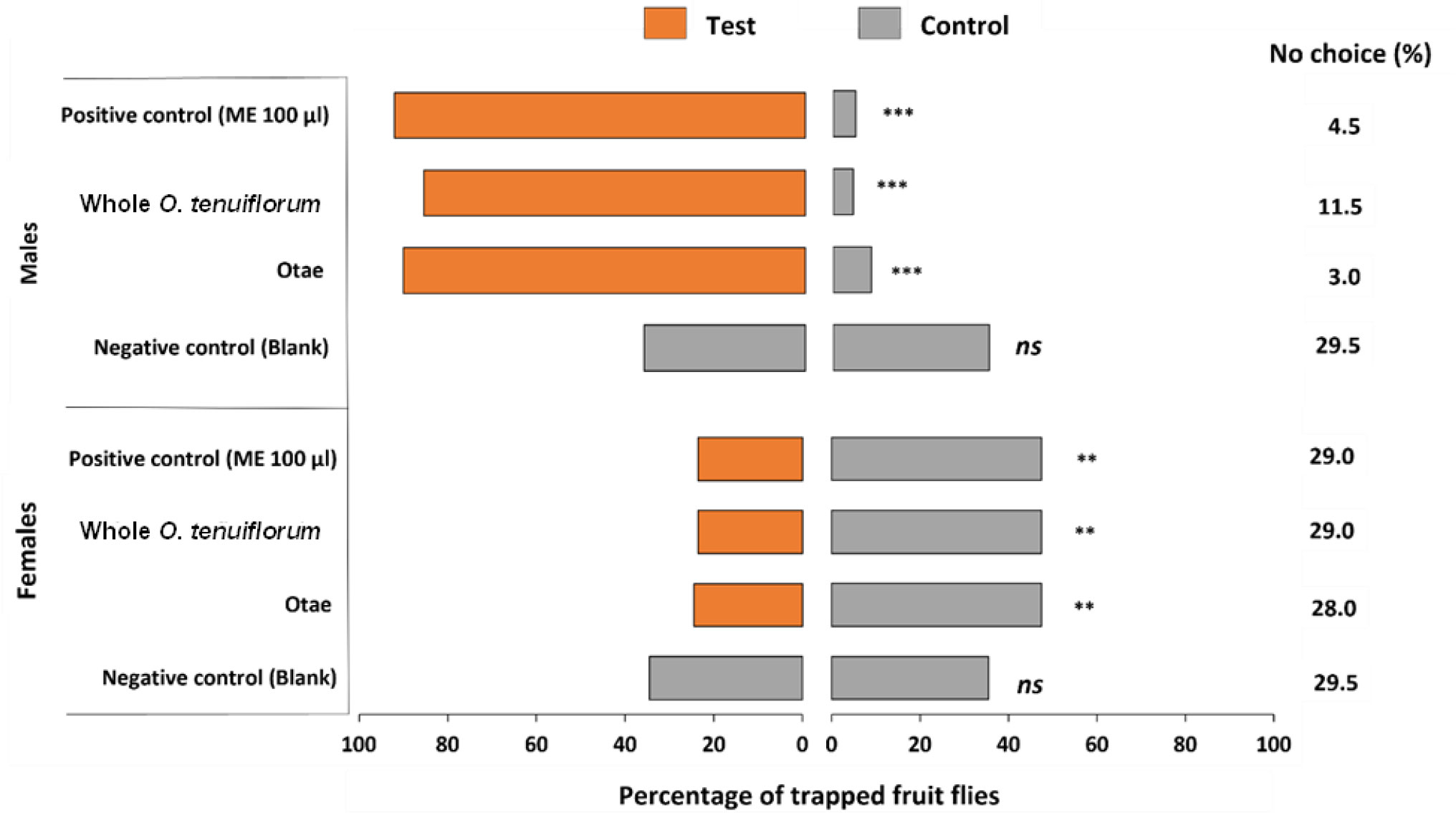
Figure 2 Effectiveness of basil plants in attracting males and females of B. dorsalis. Otae: O. tenuiflorum aqueous extract. ME: Methyl eugenol. The fruit flies that remain nonresponsive were included as “no choice”group. ns is not significant at P > 0.05, ** is significant at P ≤ 0.01, *** is significant at P ≤ 0.001.
Although a concentration of 10 mg/mL of Otae was equally effective as a positive control (ME 100 µL) in attracting male B. dorsalis flies, the attraction of males of B. dorsalis to the Otae was dose-dependent (Table 1).
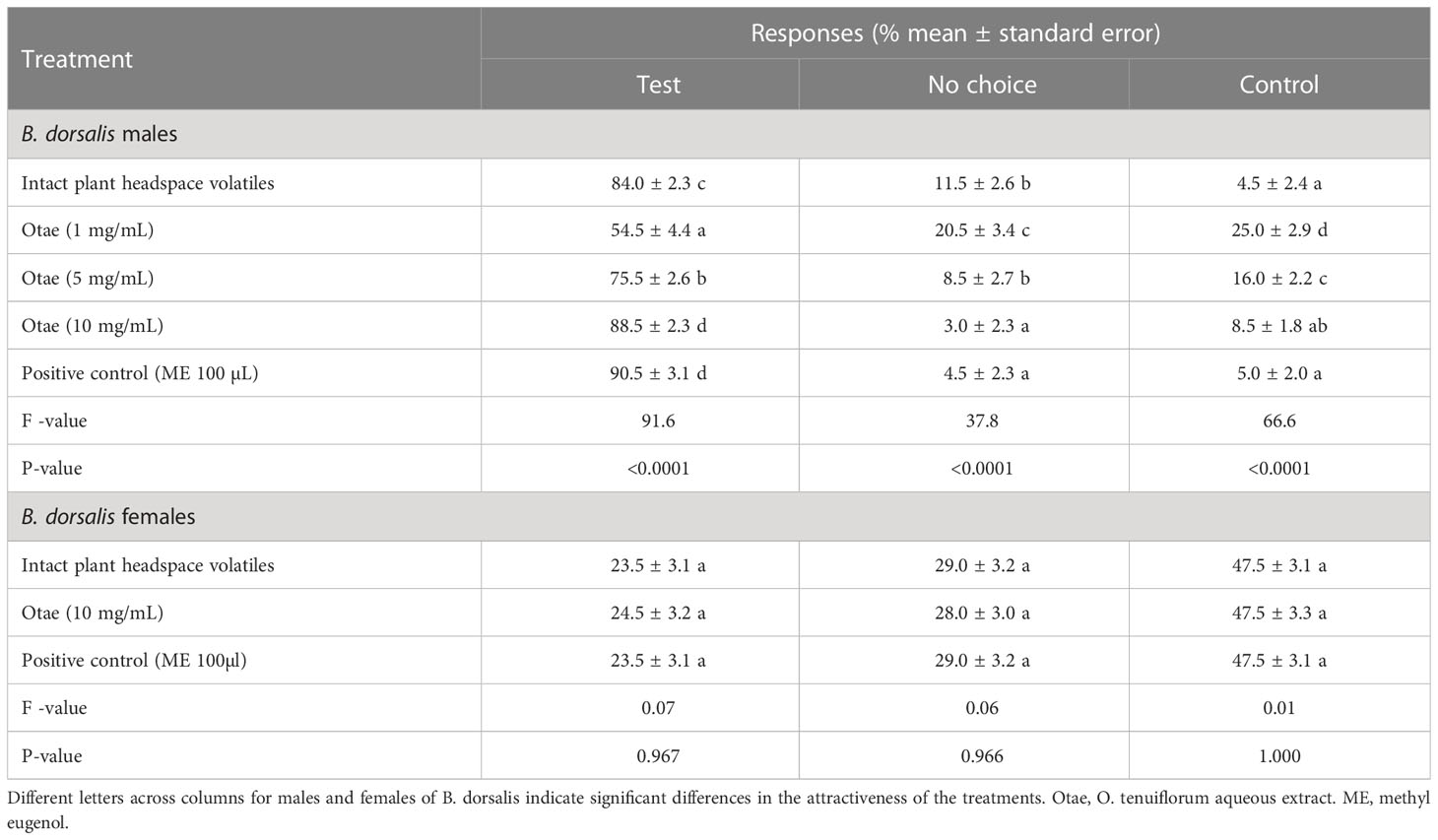
Table 1 Responses of males and females of B. dorsalis to intact plant headspace volatiles, Otae and positive control (ME 100 µL).
Response of four fruit fly species to O. tenuiflorum aqueous extract (Otae) and the positive control methyl eugenol (ME)
The males of the four fruit fly species were more attracted to the treatments than the females (F = 134.36, df = 1, 154, P<0.001). Fruit flies caught in Otae (10 mg/ml) and ME (100 µl) baited traps differed significantly for males (F = 13.88, df = 3,75, P<0.001), but not for females (F = 1.74, df = 3,75, P=0.16). Otae attracted the highest number of B. dorsalis male flies (47.7 ± 6.5%), followed by Z. cucurbitae (25.1 ± 5.9%), while the least attracted were C. capitata (18.4 ± 3.8%) and C. cosyra (18.2 ± 4.2%) (Table 2). No fruit flies were recorded in unbaited traps.
Coupled gas chromatography-mass spectrometry (GC-MS) analysis of O. tenuiflorum volatile organic compounds
Across the three headspaces of VOCs (intact plant without flowers, intact plant with flowers, and Otae) and the essential oil analyzed, 97 peaks were detected, 55 authenticated with commercial standards, and 39 tentatively identified based on fragmentation pattern database matches and/or retention index (RI) values (Table 3). Of the 97 peaks detected, the intact plant without flowers, intact plant with flowers, Otae and the O. tenuiflorum essential oil, contributed 76, 62, 33 and 28 compounds, respectively.
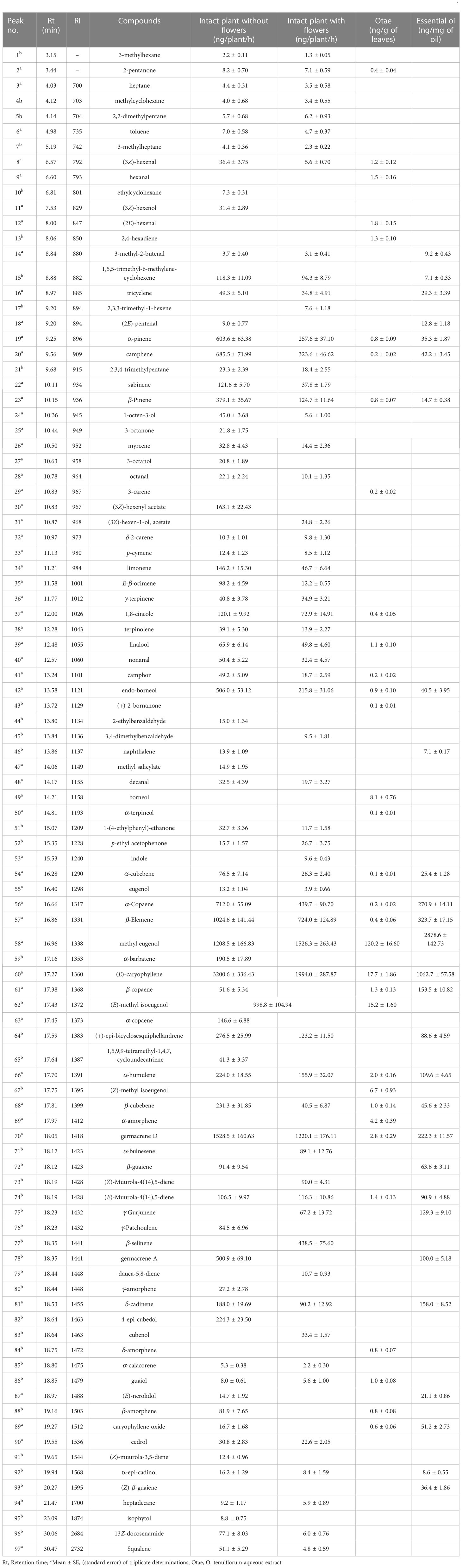
Table 3 Composition of volatile organic compounds (VOCs) in O. tenuiflorum analyzed by Gas Chromatography coupled to Mass Spectrometry (GC-MS)*.
Among the most abundant VOCs in intact plant without flowers were (E)-caryophyllene, followed by germacrene D and ME, (E)-methyl isoeugenol, α-pinene, camphene, α-copaene and β-elemene. In an intact plant with flowers, the most abundant compounds were (E)-caryophyllene, methyl eugenol, germacrene D, α-pinene, camphene, copaene, β-Elemene and β-selinene. ME, (E)-caryophyllene and (E)-methyl isoeugenol were the most abundant compounds in Otae, while methyl eugenol and (E)-caryophyllene were the two most abundant compounds in essential oil.
Discussion
It has been documented that 150 Ocimum species are distributed throughout the tropical and temperate regions of the world (Pandey et al., 2014). Recently, a vast range of subspecies has been identified, which is attributable to extended cultivation and inter- and intra-specific cross-hybridization at different geospatial scales, leading to variations in chemical and biological makeup (Srivastava et al., 2018; Tangpao et al., 2022). The increasing demand for essential oils from these Ocimum species for various pharmaceutical and pest management interventions could be the main driver for the diversity (Pandey et al., 2014). The vast global diversity of Ocimum species and sub-species necessitates accurate identification. The morphological features are comparable to the description of Dharmadasa et al. (2015). The molecular characterization indicated that the plant specimen of interest in this study is O. tenuiflorum, which was confirmed by molecular markers.
The current study presents the findings of an evaluation of the effects of odors emanating from an intact O. tenuiflorum plant and its aqueous extracts on both male and female B. dorsalis fruit flies in a dual choice wind tunnel, and room experiments with other fruit fly species namely, Z. cucurbitae, C. capitata and C. cosyra.
B. dorsalis males responded more strongly to the odor sources from the O. tenuiflorum plant (intact plant, Otae) and to the positive control (commercial ME) than females did. A similar pattern was recorded for the other fruit fly species Z. cucurbitae, C. capitata and C. cosyra, where six times as many male fruit flies were attracted than females. Numerous studies have shown that fruit fly males in the genus Rhagoletis, Ceratitis, Bactrocera/Dacus and Anastrepha exhibit an extraordinary liking for volatiles from certain plant species, such as O. sanctum L. (Nishida et al., 2000; Nishida and Tan, 2016). The male fruit flies congregate in leks and feed voraciously on these plant volatiles. They selectively sequester some of the secondary metabolites in their rectal pheromone glands, for production of sex pheromones and enhance their defense system (Tan and Nishida, 2007). On the other hand, plants, particularly those in rainforests, benefit from their pollination services (Nishida and Tan, 2016).
In all our experimental set-ups used in this study, the attractiveness of Otae was comparable with that of the positive control (commercial ME). This result corroborates previous studies that found water extract to be superior to other organic solvents in extracting the volatiles for attracting fruit flies. For instance, Prokopy et al. (1997) found that the water extract of ripe C. arabica fruit had a more alluring aroma to the fruit flies than the fruit’s extracts collected with methanol, methylene chloride, or hexane after 24 h. Although our findings give an insight into the mechanisms, we recommend a thorough investigation be undertaken of various extracting solvents to optimize O. tenuiflorum extracts for application in fruit fly-baited traps.
Otae attracted more males of B. dorsalis, as compared with Z. cucurbitae, C. capitata, and C. cosyra, in the room experiment. This observation may be partly attributed to the physical and physiological advantages of B. dorsalis over Z. cucurbitae and other native species, such as flight capability, large body size and wing length (Drew et al., 2005; Drew and Romig, 2007) and thus competitively displaces the latter from aggressively competing for food sources and habitat (Ekesi et al., 2006; Rwomushana et al., 2009). The genus Bactrocera is native to Asia, where O. tenuiflorum originated, indicating possible coevolution, and this may account for the strong responses of B. dorsalis and Z. cucurbitae. Zheng et al. (2012) reported that odorant receptor co-receptor Orco in B. dorsalis upregulated by ME in the antenna of male flies and silencing Orco through the ingestion of dsRNA can reduce the attractive effects of ME. More research is needed to elucidate the mechanisms by which B. dorsalis and other fruit fly species respond to ME.
The abundance of the chemical composition of essential oils in basil plants may vary according to plant species and to the accessions, cultivation, growing location and extraction methods (Abduelrahman et al., 2009; Avetisyan et al., 2017; Tangpao et al., 2022). For instance, Raina and Misra (2018) reported a chemical divergence among seventeen accessions, representing five Ocimum species, namely O. tenuiflorum, O. basilicum, O. gratissimum, O. americanum L. and O. citriodorum. In our study, a GC-MS analysis of O. tenuiflorum plant volatiles showed the presence of 97 different organic compounds distributed across the plant, with flowers, Otae, and the essential oil. We recorded the highest number of organic compounds in an intact plant without flowers (76), followed by an intact plant with flowers (62), then leave extracts (33) and essential oil (28). The results agree with those in a study by da Silva et al. (1999), reporting that essential oils are more concentrated in leaves than in flowers. Surprisingly, ME was one of the most prevalent compounds and among the top three in each category analyzed.
ME has been used as an attractant in the early detection, monitoring, and control of different fruit flies (Wee et al., 2002; Roger et al., 2010). The fact that the odors of the O. tenuiflorum intact plant and its aqueous extract contain ME partly explains why the male fruit fly under investigation found these odor sources to be alluring. The attractiveness of O. tenuiflorum aqueous extract to B. dorsalis was dose dependent. So, the results of the study validate the use of Otae by smallholder farmers to drown male fruit flies.
Male fruit flies aggressively seek volatile compounds such as ME and their metabolic derivatives released by plants and use them for the manufacture of sex pheromone in addition to enhanced female attraction and improved male mating success (Tan and Nishida, 2012). Furthermore, a sesquiterpene hydrocarbon, β-caryophyllene, germacrene D and I-methyl isoeugenol were also detected among the predominant compounds, similar to the previous studies on the essential oil of the same plant species collected in Sri Lanka (Dharmadasa et al., 2015). β-caryophyllene has been reported to be present in the rectal glands of wild B. correcta males (Wee et al., 2002; Tokushima et al., 2010; Nishida and Tan, 2016) and was found to be more attractive to this species than ME. Future studies on the role of individual semiochemicals from O. tenuiflorum in fruit fly attractant and their use in baited traps are warranted. Since O. tenuiflorum occurs naturally, it can be tamed and used for ME extraction as an attractant for fruit flies. The optimization of extraction techniques may offer new prospects for obtaining ME from organic sources, in contrast to the current synthetic industrial processes that have adverse effects on human and environmental health. While there are no reviews that directly compare the production costs of synthetic ME and natural ME, we extrapolate that cultivation of O. tenuiflorum, and extraction of ME may reduce production costs and thereby make ME readily available and accessible to millions of low-income farmers in Africa and elsewhere.
Conclusion
The study confirms that significantly higher proportions of males of B. dorsalis are attracted to O. tenuiflorum. This is attributed to the fact that the plant has a high methyl eugenol content, which is equally effective as the commonly used commercial ME. The attraction of native species C. capitata and C. cosyra could be attributable to some other compounds present in the extracts. The purification of extracts would result in a greater specificity of the ME product. We recommend a detailed study on cost-benefit analysis on the production and use of the naturally occurring ME with an extrapolated mass production for scaling among low, and perhaps, middle income farmers that are often struggling with management of the devastating fruit flies.
Data availability statement
The datasets generated for this study can be accessed from the corresponding author upon reasonable request.
Author contributions
SNi, BB, SM, and SE: conceived and designed the project. XC: conducted the extraction of volatiles from the plants and conducted experiments. JB: performed morphological identification. FK and LO: conducted the molecular identification of the plant. EO, SNi, and XC: performed the data analysis. SNi, XC, and EO: wrote the original draft. SNd, SE, SM, NO, DK, YTB, and TD reviewed the manuscript. SS, SE, and SM: supervised the project. SM: provided financial resources. All authors contributed to the article and approved the submitted version.
Funding
The authors gratefully acknowledge the financial support for this research provided by the following organizations and agencies: the International Development Research Centre (IDRC) and the Australian Centre for International Agricultural Research (ACIAR) for the project “Alien invasive fruit flies in Southern Africa: Implementation of a sustainable IPM programme to combat their menaces (grant number 109040)”; the Swedish International Development Cooperation Agency (Sida); the Swiss Agency for Development and Cooperation (SDC); the Australian Centre for International Agricultural Research (ACIAR); the Federal Democratic Republic of Ethiopia; and the Government of the Republic of Kenya. The views expressed herein do not necessarily reflect the official opinion of the donors.
Acknowledgments
This research received financial support from the Norwegian Agency for Development Cooperation (NORAD), the section for research, innovation, and higher education, for the project “Combatting Arthropod Pests for better Health, Food and Climate Resilience" (grant number RAF-3058 KEN-18/0005) and the International Atomic Energy Agency (IAEA) CRP code: D41027, Contract Number: 23181. Also the authors gratefully acknowledge the financial support for this research provided by the following organizations and agencies: the Swedish International Development Cooperation Agency (Sida); the Swiss Agency for Development and Cooperation (SDC); the Australian Centre for International Agricultural Research (ACIAR); the Federal Democratic Republic of Ethiopia; and the Government of the Republic of Kenya. The views expressed herein do not necessarily reflect the official opinion of the donors. We acknowledge Mr. Patrick Mutiso, Chief Technologist/Curator of the University of Nairobi Herbarium, for assisting in the morphological identification of the plant under study.
Conflict of interest
The authors declare that the research was conducted in the absence of any commercial or financial relationships that could be construed as a potential conflict of interest.
Publisher’s note
All claims expressed in this article are solely those of the authors and do not necessarily represent those of their affiliated organizations, or those of the publisher, the editors and the reviewers. Any product that may be evaluated in this article, or claim that may be made by its manufacturer, is not guaranteed or endorsed by the publisher.
References
Abduelrahman A. H. N., Elhussein S. A., Osman N. A., Nour A. H. (2009). Morphological variability and chemical composition of essential oils from nineteen varieties of basil (Ocimum basilicum l.) growing in Sudan. Int. J. Sci. Technol. Res. 1 (1), 1–10. doi: 10.3923/ijct.2009.1.10
Asnawati D., Sudarma I. M., Yuanita E., Arlina B. F., Hamdiani S., Kamali S. R. (2015). Methylation of eugenol using dimethyl carbonate and bentonite as catalyst. Indones. J. Chem. 15 (3), 256–262. doi: 10.22146/ijc.21193
Avetisyan A., Markosian A., Petrosyan M., Sahakyan N., Babayan A., Aloyan S., et al. (2017). Chemical composition and some biological activities of the essential oils from basil Ocimum different cultivars. BMC complement. Altern. Med. 17, 1–8. doi: 10.1186/s12906-017-1587-5
Ballo S., Demissie G., Tefera T., Mohamed S. A., Khamis F. M., Niassy S., et al. (2020). “Use of para-pheromone methyl eugenol for suppression of the mango fruit fly, Bactrocera dorsalis (Hendel) (Diptera: tephritidae) in southern Ethiopia,” in Sustainable management of invasive pests in Africa (Cham, Switzerland: Springer International Publishing), 203–217.
Couso-Ferrer F., Arouri R., Beroiz B., Perera N., Cervera A., Navarro-Llopis V., et al. (2011). Cross-resistance to insecticides in a malathion-resistant strain of Ceratitis capitata (Diptera: tephritidae). J. Econ. Entomol. 104, 1349–1356. doi: 10.1603/EC11082
Crane P. R., Friis E. M., Pedersen K. R. (1995). The origin and early diversification of angiosperms. Nature 374, 27–33. doi: 10.1038/374027a0
da Silva M. H. L., Andrade E. H. A., Zoghbi M. D. G. B., Luz A. I. R., da Silva J. D., Maia J. G. S. (1999). The essential oils of Lantana camara l. occurring in north Brazil. Flavour Fragr. J. 14 (4), 208–210. doi: 10.1002/(SICI)1099-1026(199907/08)14:4<208::AID-FFJ811>3.0.CO;2-F
Datiles D., Acevedo-Rodríguez P. (2014). Ocimum tenuiflorum (holy basil) (CABI Compendium). doi: 10.1079/cabicompendium.110287
Dharmadasa R. M., Siriwardhane D. A. S., Samarasinghe K., Rangana S. H. C. S., Nugaliyadda L., Gunawardane I., et al. (2015). Screening of two Ocimum tenuiflorum l. (Lamiaceae) morphotypes for their morphological characters, essential oil composition and fruit fly attractant ability. World. J. Agric. Res. 3, 1–4. doi: 10.12691/wjar-3-1-1
Drew R. A. I., Romig M. C. (2007). Records of dacine fruit flies and new species of Dacus (Diptera: tephritidae) in Bhutan. Raffles Bull. Zool. 55, 1–21.
Drew R. A. I., Tsuruta K., White I. M. (2005). A new species of pest fruit fly (Diptera: tephritidae: dacinae) from Sri Lanka and Africa. Afr. Entomol. 13, 149–154.
Ekesi S., Mohamed S. A. (2011). “Mass rearing and quality control parameters for tephritid fruit flies of economic importance in Africa,” in Wide spectra of quality control. Ed. Akyar I. (Rijeka, Croatia: InTech), 387–410.
Ekesi S., Mohamed S. A., De Meyer M. (2016). Fruit fly research and development in Africa-towards a sustainable strategy to improve horticulture (Cham, Switzerland: Springer International Publishing), 778.
Ekesi S., Nderitu P. W., Rwomushana I. (2006). Field infestation, life history and demographic parameters of the fruit fly Bactrocera invadens (Diptera: tephritidae) in Africa. Bull. Entomol. Res. 96, 379–386. doi: 10.1079/BER2006442
FAO (2017). The future of food and agriculture-trends and challenges (Rome, Italy: Food and Agricultural Organization (FAO), 1–180.
Fay M. F., Olmstead R. G., Richardson J. E., Santiago E., Prance G. T., Chase M. W. (1998). Molecular data support the inclusion of Duckeodendron cestroides in solanaceae. Kew Bull. 53 (1), 203–212. doi: 10.2307/4110460
Faye P. D., Bal A. B., Ndiaye N. M., Diop F., Sangaré Y. K., Haddad C., et al. (2021). Field efficacy of Metarhizium acridum (Hypocreales: clavicipitaceae) in the control of Bactrocera dorsalis (Diptera: tephritidae) in citrus orchards in Senegal. Int. J. Trop. Insect Sci. 41 (2), 1185–1195. doi: 10.1007/s42690-020-00306-5
Haq I. U., Vreysen M. J., Schutze M., Hendrichs J., Shelly T. (2016). Effects of methyl eugenol feeding on mating compatibility of Asian population of Bactrocera dorsalis (Diptera: tephritidae) with African population and with B. carambolae. J. Econ. Entomol. 109 (1), 148–153. doi: 10.1093/jee/tov274
Kearse M., Moir R., Wilson A., Stones-Havas S., Cheung M., Sturrock S., et al. (2012). Geneious basic: an integrated and extendable desktop software platform for the organization and analysis of sequence data. Bioinformatics 28 (12), 1647–1649. doi: 10.1093/bioinformatics/bts199
Khair-ul-Bariyah S., Ahmed D., Aujla M. I. (2012). Ocimum basilicum: A review on phytochemical and pharmacological studies. Pak. J. Chem. 2 (2), 78–85. doi: 10.15228/2012.v02i02p05
Kibira M., Affognon H., Njehia B., Muriithi B., Mohamed S., Ekesi S. (2015). Economic evaluation of integrated management of fruit fly in mango production in embu county, Kenya. Afr. J. Agric. Resour. Econ. 10, 343–353.
Kothari S. K., Bhattacharya A. K., Darokar M. P., Khanuja S. P. S. (2006). Antimicrobial activity of essential oil of methyl eugenol rich Ocimum tenuiflorum LF (syn. O. sanctum l.). Indian Drugs 43 (5), 410–415. doi: 10.1016/j.chroma.2004.03.019
Lam S. N., Neda G. D., Rabeta M. S. (2018). The anticancer effect of Ocimum tenuiflorum leaves. Food Res. 2 (2), 154–162. doi: 10.26656/fr.2017.2(2).251
Leblanc L., Vargas R. I., MacKey B., Putoa R., Piñero J. C. (2011). Evaluation of cue-lure and methyl eugenol solid lure and insecticide dispensers for fruit fly (Diptera: tephritidae) monitoring and control in Tahiti. Fla. Entomol. 94 (3), 510–516. doi: 10.1653/024.094.0315
Lux A., Luxová M., Abe J., Tanimoto E., Hattori T., Inanaga S. (2003). The dynamics of silicon deposition in the sorghum root endodermis. New Phytol. 158 (3), 437–441. doi: 10.1046/j.1469-81372003.00764x
Manjudevi M., Thirugnanasampandan R., Vishnupriya B. (2022). In vitro Propagation of Ocimum sanctum linn., Ocimum canum sims., Ocimum tenuiflorum linn., evaluation of antioxidant, MMP-9 down regulation of eugenol and camphor. S. Afr. J. Bot 5 (12), 1–10. doi: 10.1016/j.sajb.2022.01.006
Manoukis N. C., Siderhurst M., Jang E. B. (2015). Field estimates of attraction of Ceratitis capitata to trimedlure and Bactrocera dorsalis (Diptera: tephritidae) to methyl eugenol in varying environments. Environ. Entomol. 44 (3), 695–703. doi: 10.1093/ee/nvv020
Midingoyi S. K. G., Kassie M., Muriithi B., Diiro G., Ekesi S. (2019). Do farmers and the environment benefit from adopting integrated pest management practices? evidence from Kenya. J. Agric. Econ. 70, 452–470. doi: 10.1111/1477-9552.12306
Mutamiswa R., Nyamukondiwa C., Chikowore G., Chidawanyika F. (2021). Overview of oriental fruit fly, Bactrocera dorsalis (Hendel) (Diptera: tephritidae) in Africa: from invasion, bio-ecology to sustainable management. Crop Prot. 141, 105492. doi: 10.1016/j.cropro.2020.105492
Nankinga C. M., Isabirye B. E., Muyinza H., Rwomushana I., Stevenson P. C., Mayamba A., et al. (2014). Fruit fly infestation in mango: a threat to the horticultural sector in Uganda. Uganda J. Agric. Sci. 15, 1–14.
Ndlela S., Mohamed S., Ekesi S., Ndegwa P. N., Ongamo G. O. (2016). Male Annihilation technique using methyl eugenol for field suppression of Bactrocera dorsalis (Hendel) (Diptera: tephritidae) on mango in Kenya. Afr. Entomol. 24 (2), 437–447. doi: 10.4001/003.024.0437
Ndlela S., Niassy S., Mohamed S. A. (2022). Important alien and potential native invasive insect pests of key fruit trees in Sub-Saharan Africa: advances in sustainable pre- and post-harvest management approaches. CABI Agric. Biosci. 3, 7. doi: 10.1186/s43170-022-00074-x
Niassy S., Murithii B., Omuse E. R., Kimathi E., Tonnang H., Ndlela S., et al. (2022). Insight on fruit fly IPM technology uptake and barriers to scaling in Africa. Sustainability 14 (5), 2954. doi: 10.3390/su14052954
Nishida R., Shelly T. E., Kaneshiro K. Y. (2000). Roles of semiochemicals in mating systems: a comparison between oriental fruit fly and medfly (Penang (Malaysia: Fifth international symposium on fruit flies of economic importance), 631–637. Abstract retrieved from Abstracts in International Atomic Energy Agency database.
Nishida R., Tan K. (2016). “Search for new fruit fly attractants from plants: a review,” in Proceedings of the 9th International Symposium on Fruit Flies of Economic Importance. Eds. Sabater-Muñoz B., Vera T., Pera R., Orankanok W.(Bangkok, Thailand: International Fruit Fly Steering Committee), 249–262.
Nyasembe V. O., Teal P. E., Mukabana W. R., Tumlinson J. H., Torto B. (2012). Behavioural response of the malaria vector Anopheles gambiae to host plant volatiles and synthetic blends. Parasit Vectors. 5, 234. doi: 10.1186/1756-3305-5-234
Ochola J. B., Mutero C. M., Marubu R. M., Haller B. F., Hassanali A., Lwande W. (2022). Mosquitoes larvicidal activity of Ocimum kilimandscharicum oil formulation under laboratory and field-simulated conditions. Insects 13, 203. doi: 10.3390/insects13020203
Pandey A. K., Singh P., Tripathi N. N. (2014). Chemistry and bioactivities of essential oils of some Ocimum species: an overview. Asian Pac. J. Trop. Biomed. 4 (9), 682–694. doi: 10.12980/APJTB.4.2014C77
Piras A., Gonçalves M. J., Alves J., Falconieri D., Porcedda S., Maxia A., et al. (2018). Ocimum tenuiflorum L. and Ocimum basilicum l., two spices of lamiaceae family with bioactive essential oils. Ind. Crops. Prod. 113, 89–97. doi: 10.1016/j.indcrop.2018.01.024
Prokopy R. J., Phillips T. W., Vargas R. I., Jang E. B. (1997). Defining sources of coffee plant odor attractive to Ceratitis capitata flies. J. Chem. Ecol. 23, 1577–1587. doi: 10.1023/B:JOEC.0000006423.53109.15
Raina A. P., Misra R. C. (2018). Chemo-divergence in essential oil composition among germplasm collection of five Ocimum species from eastern coastal plains of India. J. Essent. Oil Res. 30 (2), 47–55. doi: 10.1080/10412905.2017.1371087
Ratnasingham S., Hebert P. D. (2007). BOLD: the barcode of life data system. Mol. Ecol. Notes. 7 (3), 355–364. doi: 10.1111/j.1471-8286.2007.01678.x
R Core Team (2020). R: a language and environment for statistical computing (Vienna: R Foundation for Statistical Computing).
Ristaino J. B., Thomas W. (1997). Agriculture, methyl bromide, and the ozone hole: can we fill the gaps? Plant Dis. 81 (9), 964–977. doi: 10.1094/PDIS.1997.81.9.964
Roger I. V., Shelly T. E., Leblanc L., Piñero J. C. (2010). Chapter twenty-three-recent advances in methyl eugenol and cue-lure technologies for fruit fly detection, monitoring, and control in Hawaii. Vitam. Horm. 83, 575–595. doi: 10.1016/S0083-6729(10)83023-7
Rwomushana I., Ekesi S., Ogol C. K. P. O., Gordon I. (2009). Mechanisms contributing to the competitive success of the invasive fruit fly Bactrocera invadens over the indigenous mango fruit fly, Ceratitis cosyra: the role of temperature and resource pre-emption. Entomol. Exp. Appl. 133, 27–37. doi: 10.1111/j.1570-7458.2009.00897x
Shelly T. E., Edu J., McInnis D. (2010). Pre-release consumption of methyl eugenol increases the mating competitiveness of sterile males of the oriental fruit fly, Bactrocera dorsalis, in large field enclosures. J. Insect Sci. 10 (1), 8. doi: 10.1673/031.010.0801
Shelly T., Epsky N., Jang E. B., Reyes-Flores J., Vargas R. (2014). Trapping and the detection, control, and regulation of tephritid fruit flies: lures, area-wide programs, and trade implications (Dordrecht, German: Springer Science+Business Media), 1–15.
Shelly T. E., McInnis D. O., Pahio E., Edu J. (2004). Aromatherapy in the Mediterranean fruit fly (Diptera: tephritidae): sterile males exposed to ginger root oil in prerelease storage boxes display increased mating competitiveness in field-cage trials. J. Econ. Entomol. 97 (3), 846–853. doi: 10.1093/jee/97.3.846
Sims C. A., Juliani H. R., Mentreddy S. R., Simon J. E. (2014). Essential oils in holy basil (Ocimum tenuiflorum l.) as influenced by planting dates and harvest times in north Alabama. J. Med. Act. Plants 2 (3), 33–41. doi: 10.1046/j.1469-8137.2003.00764x
Singh S., Lal R. K., Singh B. (2020). “Genetic variability in ocimum l. germplasm: medicinal and economic potential for value addition and product development,” in Botanical leads for drug discovery (Taipei, Singapore: Springer), 243–253. doi: 10.1007/978-981-15-5917-4_11
Srivastava A., Gupta A. K., Sarkar S., Lal R. K., Yadav A., Gupta P., et al. (2018). Genetic and chemotypic variability in basil (Ocimum basilicum l.) germplasm towards future exploitation. Ind. Crops Prod. 112, 815–820. doi: 10.1016/j.indcrop.2018.01.009
Tan K. H., Nishida R. (2007). Zingerone in the floral synomone of Bulbophyllum baileyi (Orchidaceae) attracts Bactrocera fruit flies during pollination. Biochem. Syst. Ecol. 35 (2), 334–341. doi: 10.1016/j.bse.2007.01.013
Tan K. H., Nishida R. (2012). Methyl eugenol: its occurrence, distribution, and role in nature, especially in relation to insect behavior and pollination. J. Insect Sci. 12 (1), 56. doi: 10.1673/031.012.5601
Tangpao T., Charoimek N., Teerakitchotikan P., Leksawasdi N., Jantanasakulwong K., Rachtanapun P., et al. (2022). Volatile organic compounds from basil essential oils: plant taxonomy, biological activities, and their applications in tropical fruit productions. Hortic 8 (2), 144. doi: 10.3390/horticulturae8020144
Tokushima I., Orankanok W., Tan K. H., Ono H., Nishida R. (2010). Accumulation of phenylpropanoid and sesquiterpenoid volatiles in male rectal pheromonal glands of the guava fruit fly, Bactrocera correcta. J. Chem. Ecol. 36, 1327–1334. doi: 10.1007/s10886-010-9874-3
Ullah F., Khan M. W., Maula F., Younus M., Badshah H. (2017). Impact of close proximity of traps baited with various attractants on fruit fly catch. J. Entomol. Zool. Stud. 5 (6), 1843–1845.
Van Den Dool H., Kratz P. D. (1963). A generalization of the retention index system including linear temperature programmed gas–liquid partition chromatography. J. Chromatogr. A. 11, 463–471. doi: 10.1016/S0021-9673(01)80947-X
Vargas R. I., Piñero J. C., Leblanc L. (2015). An overview of pest species of Bactrocera fruit flies (Diptera: tephritidae) and the integration of biopesticides with other biological approaches for their management with a focus on the pacific region. Insects 6 (2), 297–318. doi: 10.3390/insects6020297
Vargas R. I., Shelly T. E., Leblanc L., Pinero J. C. (2010). Recent advances in methyl eugenol and cue-lure technologies for fruit fly detection, monitoring, and control in Hawaii. Vitam. Horm. 83, 575–595. doi: 10.1016/s0083-6729(10)83023-7
Vargas R. I., Stark J. D., Kido M. H., Ketter H. M., Whitehand L. C. (2000). Methyl eugenol and cue-lure traps for suppression of male oriental fruit flies and melon flies (Diptera: tephritidae) in Hawaii: effects of lure mixtures and weathering. J. Econ. Entomol. 93 (1), 81–87. doi: 10.1603/0022-0493-93.1.81
Vargas R. I., Stark J. D., Mackey B., Bull R. (2005). Weathering trials of amulet cue-lure and amulet methyl eugenol “attract-and-kill” stations with male melon flies and oriental fruit flies (Diptera: tephritidae) in Hawaii. J. Econ. Entomol. 98 (5), 1551–1559. doi: 10.1603/0022-0493-98.5.1551
Wee S. L., Hee A. K. W., Tan K. H. (2002). Comparative sensitivity to and consumption of methyl eugenol in three Bactrocera dorsalis (Diptera: tephritidae) complex sibling species. Chemoecology 12, 193–197. doi: 10.1007/PL00012668
Keywords: basil (Ocimum tenuiflorum), fruit fly, mango, integrated pest management (IPM), Bactrocera
Citation: Niassy S, Mohamed SA, Cheseto X, Omuse ER, Ochola JB, Khamis FM, Badji B, Ndlela S, Ombura L, Okun NL, Kupesa DM, Dubois T, Belayneh YT, Subramanian S and Ekesi S (2023) Response of some mango-infesting fruit flies to aqueous solutions of the basil plant Ocimum tenuiflorum L. Front. Hortic. 2:1139525. doi: 10.3389/fhort.2023.1139525
Received: 07 January 2023; Accepted: 17 April 2023;
Published: 10 May 2023.
Edited by:
Mathias Twizeyimana, AgBiome Inc, United StatesReviewed by:
Mojtaba Hosseini, Ferdowsi University of Mashhad, IranMondjonnesso Gomina, Université de Kara, Togo
Copyright © 2023 Niassy, Mohamed, Cheseto, Omuse, Ochola, Khamis, Badji, Ndlela, Ombura, Okun, Kupesa, Dubois, Belayneh, Subramanian and Ekesi. This is an open-access article distributed under the terms of the Creative Commons Attribution License (CC BY). The use, distribution or reproduction in other forums is permitted, provided the original author(s) and the copyright owner(s) are credited and that the original publication in this journal is cited, in accordance with accepted academic practice. No use, distribution or reproduction is permitted which does not comply with these terms.
*Correspondence: Saliou Niassy, c25pYXNzeUBpY2lwZS5vcmc=