- 1Groupe de Recherche en Physiologie végétale (GRPV), Earth and Life Institute – Agronomy (ELI-A), Université catholique de Louvain, Louvain-la-Neuve, Belgium
- 2Instituto de Investigaciones Agropecuarias (INIA – La Cruz), La Cruz, Chile
Introduction: Soil salinization is a major global problem. Tomato (Solanum lycopersicum) is one of the main crops produced in the world and is cultivated in areas affected by salinity. However, it is considered as a glycophyte species and is sensitive to salt stress. Solanum chilense is a wild tomato native to the Atacama Desert and is therefore adapted to harsh environmental conditions, including salinity. It is considered as a promising source of genes for tomato improvement. However, the physiology of abiotic stress resistance remains largely unknown in S. chilense and no studies have investigated the resistance of S. lycopersicum x S. chilense interspecific hybrids and the potential value of their use in harsh environments.
Methods: In this study, we compared the salt (0 vs. 100 mM NaCl) resistance of S. lycopersicum, S. chilense and their hybrids at vegetative and reproductive stages.
Results: Overall, hybrids showed an intermediate behavior between their parents and a higher salt resistance than S. lycopersicum. Their physiological behavior toward salt stress was sometimes closed to S. lycopersicum and sometimes closed to S. chilense. Their sodium accumulation was intermediate between parents, with a sodium concentration closer to S. lycopersicum than S. chilense in roots, but with an aerial concentration closer to S. chilense than to S. lycopersicum. In inflorescences and fruits, the sodium concentration of hybrids was closer to S. lycopersicum than to S. chilense. Despite a decrease in instantaneous transpiration, the photosynthetic nitrogen use efficiency of hybrids was not decreased by salt stress: our results suggest a greater tolerance to the osmotic phase of salt stress in hybrids compared to the parents. Regarding the reproductive stage, inflorescence production and fruit quality were not affected by salt in hybrids.
Discussion: This study highlights the potential use of hybrids in improving tomato for salt stress resistance.
1 Introduction
Soil salinization is a growing constraint in agriculture and tomato, as a glycophyte species, is not spared (Yadav et al., 2020). Global map of salt affected soils estimates that more than 833 Mha of soils are salt-affected (8.7% of terrestrial lands, representing 20 to 50% of irrigated soils) (FAO, 2021). Tomato is cultivated in arid and semi-arid areas where soil salinization may represent a tremendous constraint (FAOStats, 2022). Tomato is the main horticultural crop in the world (250 M tons produced in 2020) and its glycophyte status induces major yield losses in saline areas (decrease of tomato yield by in average 50% for an electrical conductivity of 5 dS.m-1) (Campos et al., 2006; Assaha et al., 2017; FAOStats, 2022). In contrast to most of its wild relatives adapted to saline coastal regions, cultivated tomato probably lost its salt resistance properties during domestication (Guo et al., 2022). Indeed, as for several other crop species, improvement of quality traits induced a loss of abiotic stress resistance traits (Van Deynze et al., 2007; Rothan et al., 2019; Wang et al., 2020; Rawat et al., 2022). Despite its large distribution, cultivated tomato has a very low genetic diversity (Van Deynze et al., 2007; Tamburino et al., 2020). To struggle against abiotic stresses, increasing genetic diversity through the use of wild relative species constitutes a promising option (Rick and Chetelat, 1995; Zsögön et al., 2017; Rawat et al., 2022). Tomato could be improved with wild relatives via different strategies. The most common use of wild species is the selection of introgression lines (Chetelat et al., 2019). Wild species could also be domesticated de novo (Zsögön et al., 2017; Li et al., 2018). Moreover, a strategy closed to introgression is the “rewilding” of crops, by a more holistic action than introgression of single genes (Rawat et al., 2022). In addition, wild relatives or hybrids could also be used as rootstocks for grafting (Singh et al., 2020).
Solanum chilense is a wild halophyte species in the Solanum sect. Lycopersicon clade (Peralta et al., 2008). Its important genetic diversity makes S. chilense a promising source of genes for tomato improvement (Igic et al., 2007; Böndel et al., 2015; Tamburino et al., 2020). This species has indeed a wide distribution in a huge range of stressful environments, such as high altitude, salty and drought-affected soils, as well as areas prone to high or low temperatures (Moyle, 2008; Chetelat et al., 2009; Ramírez-Ojeda et al., 2021b). Solanum chilense is one of the most diverse species in terms of habitats (different soils and climates) among the Solanum sect. Lycopersicon clade (Ramírez-Ojeda et al., 2021a; Ramírez-Ojeda et al., 2021b). It has already been used to improve tomato resistance to biotic stresses such as Tomato Yellow Leaf Curl Virus (Ji et al., 2009) or Cucumber Mosaic Virus (Stamova and Chetelat, 2000) but also to improve fruit qualities, especially anthocyanin content (Schreiber et al., 2012). The drought, heat, cold and salinity resistance of this species has been investigated (Martínez et al., 2014; Nosenko et al., 2016; Tapia et al., 2016; Gharbi et al., 2018; Blanchard-Gros et al., 2021). However, breeding for abiotic stress resistance by crossing S. lycopersicum and S. chilense has never been attempted.
Crossing between species of the Solanum sect. Lycopersicon clade is possible despite several interspecific reproductive barriers. In this clade, species are divided into “red-fruited” species (which comprises self-compatible species only) and “green-fruited” species (self-compatible and self-incompatible species) (Baek et al., 2015). Hybridization can be spontaneous between red-fruited species: it is the case of crossings in Galapagos islands between the invasive S. pimpinellifolium and the native S. cheesmaniae (Gibson et al., 2021). However, among green- and red-fruited species, interspecific reproductive barriers strongly hamper hybridization. Indeed, species in the tomato clade are dependent on the « SI x SC rule »: hybridization between self-incompatible and self-compatible species is only possible when the self-compatible species is used as female parent (Lewis and Crowe, 1958; Tovar-Méndez et al., 2014; Baek et al., 2015). Self-compatible pollen tubes are rejected in pistils of self-incompatible species (Tovar-Méndez et al., 2014; Baek et al., 2015). As S. lycopersicum is self-compatible and S. chilense is self-incompatible, hybridization can only be achieved in the direction S. lycopersicum x S. chilense. Nevertheless, the phenotype of F1 hybrids can be superior to that of the parents for one or more traits due to heterosis or hybrid vigor (Shivaprasad et al., 2012; Kumar et al., 2020).
To the best of our knowledge, the resistance to salinity of hybrids between S. lycopersicum and S. chilense was not described until now, although there is an obvious interest to assess the salinity resistance of such hybrids. In this study, S. lycopersicum (used as female parent) and S. chilense (used as male parent) were crossed and F1 interspecific hybrids were obtained. Salinity resistance of S. lycopersicum, S. chilense and their hybrids was quantified in plants exposed to100 mM NaCl for 150 days and growth, reproductive and physiological parameters were monitored.
2 Material and methods
2.1 Plant material and growth conditions
Seeds of S. lycopersicum L. cv Ailsa Craig (accession LA2838A) and of S. chilense Dunal (accession LA4107) were obtained from the Tomato Genetics Resource Center (TGRC, University of California, Davis, CA, USA) and INIA-La Cruz (La Cruz, Chile), respectively. Hybrids were obtained by crossing emasculated flowers of S. lycopersicum with pollen from one single plant of S. chilense. Flowers were emasculated in stage 15 of Brukhin et al. (2003) scale and pollinated at anthesis. After sowing of the obtained seeds, the hybrid status of the progeny was confirmed through the use of CAPS markers on leaf DNA according to Pérez de Castro et al. (2013) (Figure S1). Primers sequences, restriction enzymes and length of fragments are given in Table S1. For the sake of clarity, the term “plant groups” will be used to describe S. lycopersicum, S. chilense and the hybrids S. lycopersicum x S. chilense.
After 6 days pre-germination in Petri dishes (25°C, 12h photoperiod), seeds of S. chilense were sown in peat compost (DCM, Amsterdam, The Netherlands) in a temperate greenhouse (16h, 24 ± 1.5°C, 63 ± 8%/8h, 21 ± 0.8°C, 67 ± 5% day/night photoperiod, temperature and RH). LED LumiGrow lights (650 W, red-blue) to obtain a minimum mean light intensity in the middle of the day of 181.33 ± 63.42 µmol.m-2.s-1 provided additional lighting. Seeds of hybrids and of S. lycopersicum were sown in the same peat compost and in the same greenhouse respectively 8 and 12 days after the sowing of S. chilense to obtain plants at the same developmental stage. When the second-leaf stage was reached, plants were individually transplanted in 2.5 L pots filled with perlite/vermiculite (50% v/v) in the same greenhouse. Plants were watered three times a week with modified Hoagland solution (5 mM KNO3, 5.5 mM Ca(NO3)2, 1 mM NH4H2PO4, 0.5 mM MgSO4, 25 µM KCl, 10 µM H3BO4, 1 µM MnSO4, 0.25 µM CuSO4, 1 µM ZnSO4, 10 µM (NH4)6Mo7O and 1.87 g.L-1 Fe-EDTA, pH 5.5-6). After four days of acclimation, plants were randomly divided into 2 groups (10 to 15 plants per group) receiving 0 or 100 mM NaCl (respectively 0.86 and 10.82 mS cm-1). NaCl was added to the Hoagland solution. Plants were watered three times a week with either Hoagland solution (control plants) or Hoagland solution containing NaCl (salt-treated plants), volume depending on the physiological stage of the plant.
2.2 Growth measurements
During 85 days of stress, numbers of leaves, inflorescences and ramifications were estimated weekly on the main stem of 10 plants per treatment. At 85 and 150 DASt (days after stress imposition), fresh (FW) and dry (DW) weights of the different organs (leaves, stems and roots) of respectively 3 and 5 plants was estimated before and after 72 hours at 70°C. The area of the sixth youngest leaf from the top of 3 plants per treatment was measured at 85 DASt using ImageJ (v1.53a) on the basis of pictures (Lumix, Panasonic DMC SZ-10). After measuring the DW of the same leaves, specific leaf area (SLA) was calculated as the ratio between leaf area and DW of the same leaf (cm2.g-1).
2.3 Flower development and fruit production
Flowering time of initial and sympodial segments was assessed by counting the number of leaves respectively below the first inflorescence and between the following inflorescences on 10 plants per treatment. The number of flower buds and flowers at anthesis per inflorescence was determined on the second inflorescence, enabling to determine the percentage of bud development in an inflorescence.
Ten developed flowers per treatment were harvested on the second inflorescence of the main stem and stored in FAA (5 mL formaldehyde 35%, 5 mL glacial acetic acid, 90 mL ethanol 70% v/v) to evaluate the number of floral organs and the length of sepals, petals, stamens and pistil. Pictures were taken under binocular magnifier (Leica,-MZ75, Moticam 10.0 MP) and organs were then measured using ImageJ (v1.53a). The style exertion was also monitored for S. chilense and the hybrids (subtraction of the length of the stamens to the length of ovary and style).
The number of pollen grains was estimated on two anthers of ten flowers per treatment according to Ayenan et al. (2020) using ImageJ (v1.53a) with a pollen size of 5-800 pixel² and a circularity of 0.3-1.0; pictures were taken under a microscope connected with a camera (Polyvar Reichert-Jung, sCMEX-6, Euromex). Pollen viability was assessed on two anthers of ten flowers at anthesis per treatment using Alexander’s dye (Alexander, 1969). Pollen was considered viable when a red coloration appeared, while it was considered non-viable when its coloration was blue (a minimum of 100 pollen grains per anther was counted, in 2 anthers per flower). Stigma receptivity was assessed on 10 flowers per treatment by detecting peroxidase activity on the stigma surface, as described by Dafni and Maués (1998). Reddish-brown color developed on the surface was scored as 0% (no color = no receptive stigma) or 100% (reddish-brown color = receptive stigma).
Flowers of the self-compatible S. lycopersicum were self-pollinated and flowers of the self-incompatible S. chilense and hybrids were hand-pollinated with pollen of other plants from the same treatment. The fruit set per inflorescence was calculated as the ratio between the number of fruits and the number of pollinated flowers. Fruits were collected at maturity stage. The number of seeds per fruit, the fruit fresh weight (FWf) and the equatorial diameter of the fruit were measured on 10 fruits per treatment. Sugar concentration and pH of the juice of those fruits were analyzed respectively by refractometry in degrees Brix (Eclipse, Bellingham + Stanley, Tunbridge Wells, UK) and by pH paper indicator (Dosatest pH test strips pH 3.6 - 6.1, VWR). Fruit FW (FWfr) was measured on 5 fruits per treatment and the DW of the same fruits (DWfr) was measured after 3 days at 70°C: water content (WCfr, in %) was then calculated using the formula 100*(FWfr-DWfr)/FWfr.
2.4 Water status of vegetative parts and photosynthesis parameters
Water content (WC) of the vegetative organs (leaves, stems, roots) harvested at 85 DASt and 150 DASt on respectively 3 and 5 plants per treatment was calculated using the formula WC = ((FW-DW)/FW)*100. Leaf and root osmotic potentials (ψs) were quantified on the extracted sap from respectively the 5th youngest leaf and the first secondary root (counting from the root collar) of 3 plants per treatment harvested at 85 DASt, using a vapor pressure osmometer (Wescor 5500, Oceanside, CA, USA), as previously described (Lutts et al., 1999).
Photosynthetic and gas-exchange parameters were quantified at 28 and 70 DASt, on the 5th youngest leaf of 6 plants per treatment. The instantaneous CO2 assimilation rate under ambient conditions (400 ppm CO2) (A), the instantaneous transpiration rate (E), the intercellular CO2 concentration (Ci) and the stomatal conductance (gs) were quantified using an infrared gas analyzer (ADC BioScientific LCI-SD System Serial 33413, Hoddesdon, UK). Gas exchanges were measured using a Parkinson leaf cuvette on intact leaves for 1 min (20 records min−1) with an air flow of 300 mL min−1. Instantaneous water use efficiency (WUEi) was calculated as the A/E ratio. Chlorophyll fluorescence parameters (maximum quantum yield of photosystem II (Fv/Fm), non-photochemical quenching (NPQ), photochemical quenching (qP), efficiency of photosystem II (ΦPSII)) were analyzed using a fluorimeter (FMS II, Hansatech Instruments, Norfolf, UK) according to Mathieu et al. (2014). The chlorophyll content index (CCI) was estimated using a chlorophyllometer (Opti-Sciences, CCM-200, Hudson, USA) and measurements were performed in triplicates on each leaf. Net photosynthesis was corrected by CCI to get photosynthetic nitrogen use efficiency (PNUE) according to Paponov and Engels (2003). At 28 and 70 DASt, the abaxial stomatal density (in number of stomata per mm2) was measured on the same leaf than the one used for photosynthetic and gas-exchange parameters measurements for 3 plants per treatment by using polish nail method as described by Sun et al. (2014). The area of the same leaf was measured using ImageJ (v1.53a) from pictures (Lumix, Panasonic DMC SZ-10).
2.5 Biochemical parameters
At 85 DASt, the 6th youngest leaf and inflorescences of 3 plants per treatment were harvested and grounded separately in liquid nitrogen. Total soluble sugars were quantified in leaves and inflorescences from 100 mg of fresh material using anthrone method (Yemm and Willis, 1954) after extraction with ethanol 70% (v/v). The absorbance was read at 625 nm using a UV-1800 spectrophotometer (Shimadzu,’s-Herlogenbosch, the Netherlands) and soluble sugars quantification was determined using a standard curve of glucose.
Proline was extracted from 200 mg of leaves in 3% (w/v) sulfosalicylic acid with 30 min incubation at 70°C (Bates et al., 1973). Two mL of ninhydrin solution (1.25 g of ninhydrin in 30 mL glacial acetic acid and 20 mL of H3PO4 6M) were added to 2 mL of filtrate (Whatman, 11 µm) then water-bathed (1 h at 100°C). Reaction was stopped on ice then 2 mL toluene were added. Proline was quantified based to a standard curve. Absorbance was read at 520 nm after strong vortex of the samples.
Malondialdehyde (MDA) was quantified according to Heath and Packer (1968) method by thiobarbituric acid reaction from 250 mg leaves and inflorescences. Absorbance was read at 532 nm and 600 nm (non-specific values, subtracted to 532 nm values).
2.6 Mineral quantification
Sodium (Na+) and potassium (K+) were quantified on roots, stems and leaves at 85 DASt (3 plants per treatment) and 150 DASt (5 plants per treatment) and on inflorescences, pericarps and seeds harvested all along the experiment. Fifty to 100 mg DW were weighted and digested in 4 mL of hot 68% (v/v) HNO3. After complete evaporation, minerals were dissolved in aqua regia (HCl 37%: HNO3 68% 3:1), filtered (Whatman, 11 µm) and quantified by flame atomic absorption spectrophotometry (ICE 3300, Thermo Scientific, Waltham, MA) using standards (Spectracer-CPACHEM; accredited through ISO/IEC17025).
2.7 Statistical treatments
The experiment was performed twice and gave similar results. We present the results of one repetition. All statistical treatments were analyzed using RStudio (R Core Team, 2018). Normality distribution and homoscedasticity were verified using respectively Shapiro-Wilk and Levene’s test and data were transformed when required. Two-ways analysis of variance (ANOVA II) was used to compare species, NaCl treatment and their interactions. Data were represented by the means ± standard deviation. Statistical results are presented in Table S2. Principal component analysis (PCA) followed by vector projection was realized on the whole dataset using the package FactoMineR (Lê et al., 2008).
3 Results
To understand the impact of NaCl on plant growth and physiology of S. lycopersicum, S. chilense and their hybrids, differences among treatments were visualized using PCA (Figure 1). The PCA shows that 23.2% of the variance was explained by axis 1 and 21.0% by axis 2 while axis 3 only explained 9% of the variance. Plants firstly grouped according to the salt treatment and within each treatment according to the plant groups (Figure 1A). NaCl-stressed and control plants of the three plant groups were discriminated according to axis 1 which was mainly explained by stem K+ concentrations, ψs of leaves and roots and root Na+ concentration (Figure 1B). Axis 2 discriminated the plant groups mainly according to reproduction and flower morphology parameters (length of sepals, style exertion and number of inflorescences on the main stem) (Figure 1B). Hybrids exhibited an intermediate behaviour between the parents according to those parameters. However, according to the PCA, they were closer to S. chilense than to S. lycopersicum, at least under control conditions (Figure 1A).
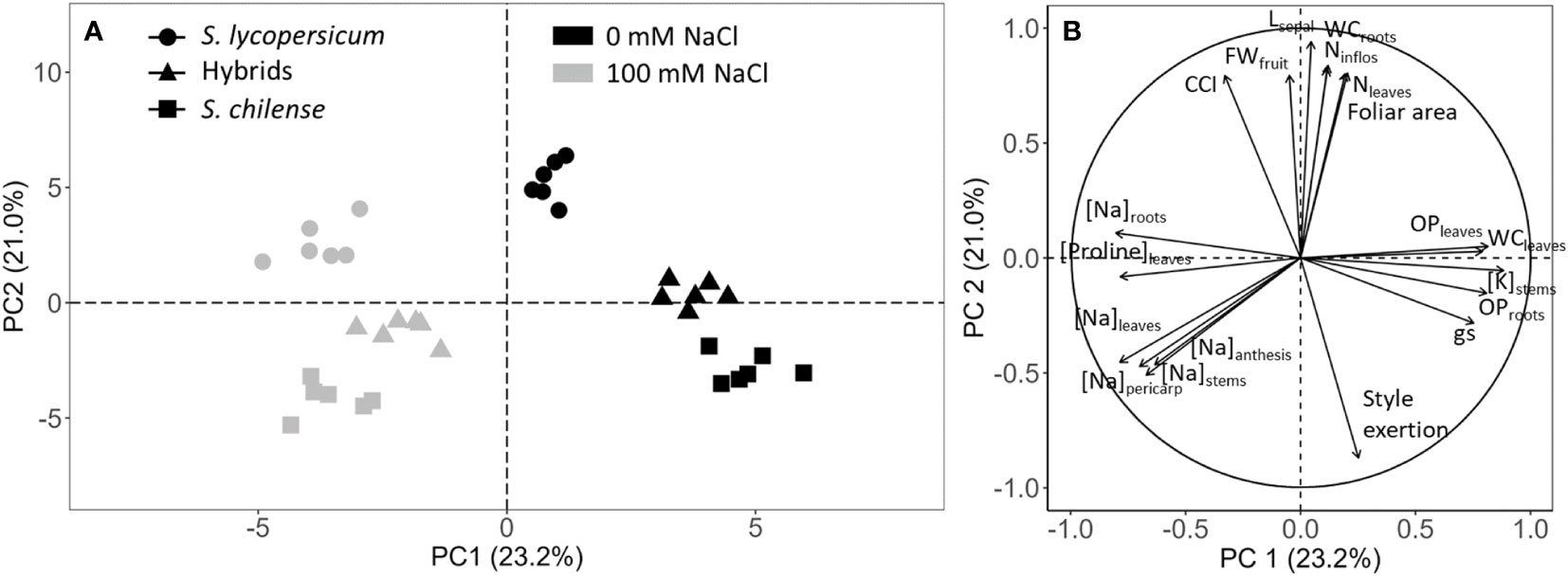
Figure 1 Principal component analysis (PCA) of vegetative and reproductive growth, physiological parameters and mineral concentrations in response to salt stress (0 mM and 100 mM NaCl) in S. lycopersicum, S. chilense and their hybrids. (A) Individual graph. (B) Variable graph of PCA (only variables with cos²>0.6 are shown). PC1 and 2, Principal component 1 and 2; CCI, chlorophyll content index; FWfruit, fruit fresh weight; Lsepal, sepal length; [Na] and [K], concentration of sodium and potassium in the different organs (roots, stems, leaves, flowers in anthesis, pericarp); Nleaves, number of leaves on the main stem; Ninflos, number of inflorescences on the main stem; OP, osmotic potential in leaves or roots; [Proline], proline concentration in the leaves; WC, water content of leaves and roots.
3.1 Growth measurements
Solanum lycopersicum produced more biomass than the two other plant groups. At 85 DASt, the plant DW was higher for S. lycopersicum than for the two other plant groups while at 150 DASt, hybrids had an intermediate DW between their two parents, especially at 100 mM NaCl (Figure 2A, Table S2). At 85 DASt, the stems represented the biggest part of the plant DW (44.5% ± 8.6%), followed by leaves (41.3% ± 7.3%) and roots (2.2% ± 4.6%) (Figures 2B–D). Although the proportion of root DW was similar between plant groups, stems were more developed in hybrids and S. chilense while leaves were more developed in S. lycopersicum, mainly at 150 DASt (Figures 2B–D; Table S2). Solanum lycopersicum produced more leaves than hybrids and S. chilense while S. chilense produced much more ramifications than the two other plant groups at 85 DASt (Figures 2E, F; Table S2).
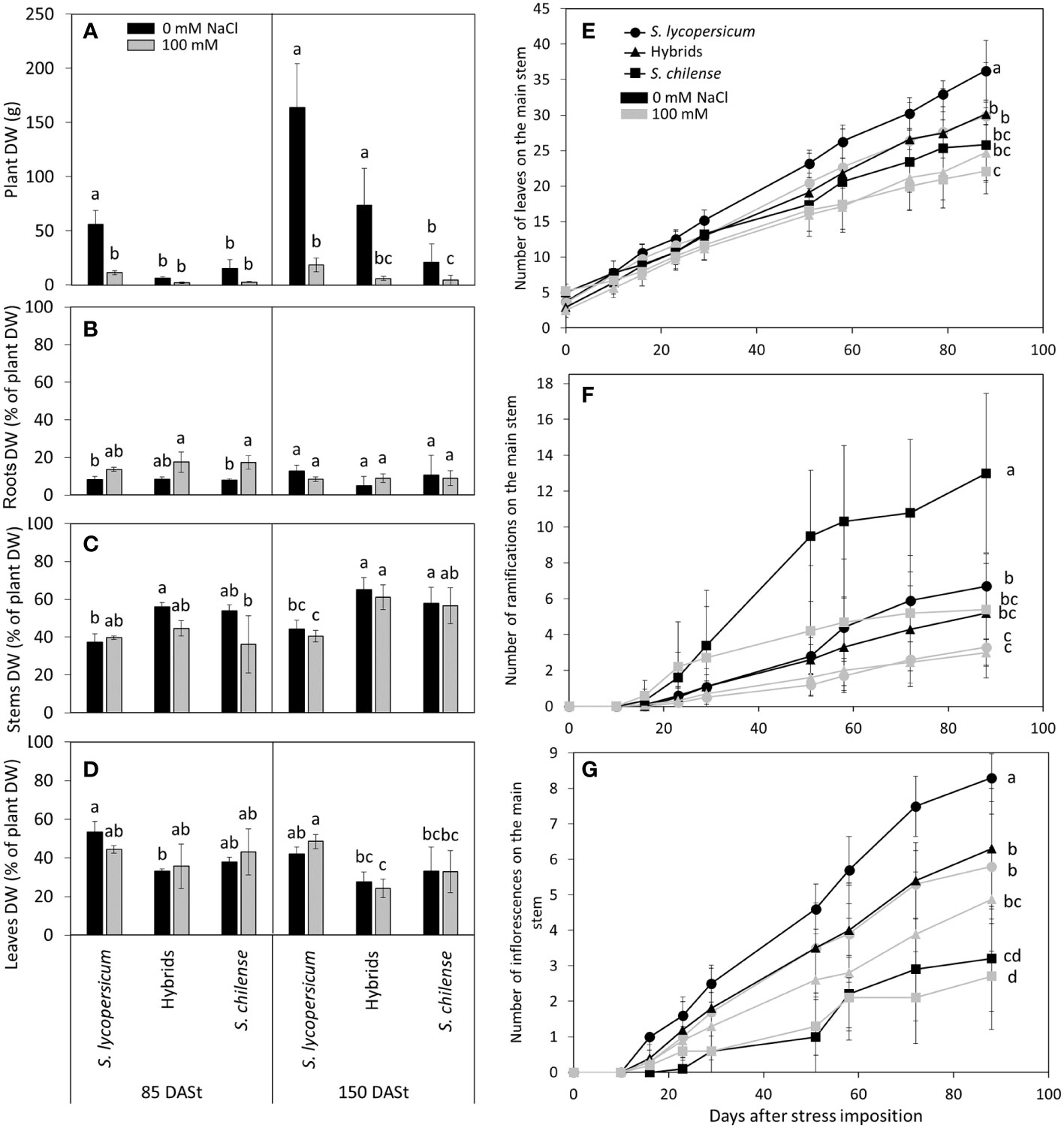
Figure 2 Effects of salt stress (0 mM and 100 mM NaCl) on Solanum lycopersicum, Solanum chilense and their hybrids. (A) Plant dry weight (DW) at 85 and 150 days after stress imposition (DASt) and (B–D) percentage of the DW represented by (B) the roots, (C) the stems and (D) the leaves. (E–G) Evolution of the number of (E) leaves, (F) ramifications and (G) inflorescences on the main stem up to 85 days after stress imposition. Data are means ± standard deviation, different letters indicate significant difference at p < 0.05 for a same date.
Although NaCl decreased plant DW as early as 85 DASt only in S. lycopersicum, it decreased it in all three plant groups at 150 DASt (Figure 2A; Table S2). Nevertheless, NaCl had only a slight effect on the DW proportions of the different organs (Figures 2B–D; Table S2). The number of leaves on the main stem decreased with salinity only in S. lycopersicum at 85 DASt (Figure 2E; Table S2) and the number of ramifications decreased with salinity in both parents but not in the hybrids (Figure 2F, Table S2).
3.2 Flowers development and morphology
The flowering time of the initial segment was similar among plant groups while the flowering time of the sympodial segments was slightly higher in S. chilense than in the other plant groups (Table 1, Table S2). As a result, S. lycopersicum produced more inflorescences on the main stem than S. chilense while the hybrids had an intermediate behavior (Figure 2G, Table S2). Within an inflorescence, S. chilense and hybrids produced more floral buds and had a higher percentage of floral buds reaching anthesis than S. lycopersicum (Tables 1, S2). Salt did not affect flowering time nor anthesis percentage (Tables 1, S2) but decreased inflorescence production in S. lycopersicum only (Figure 2G, Table S2).
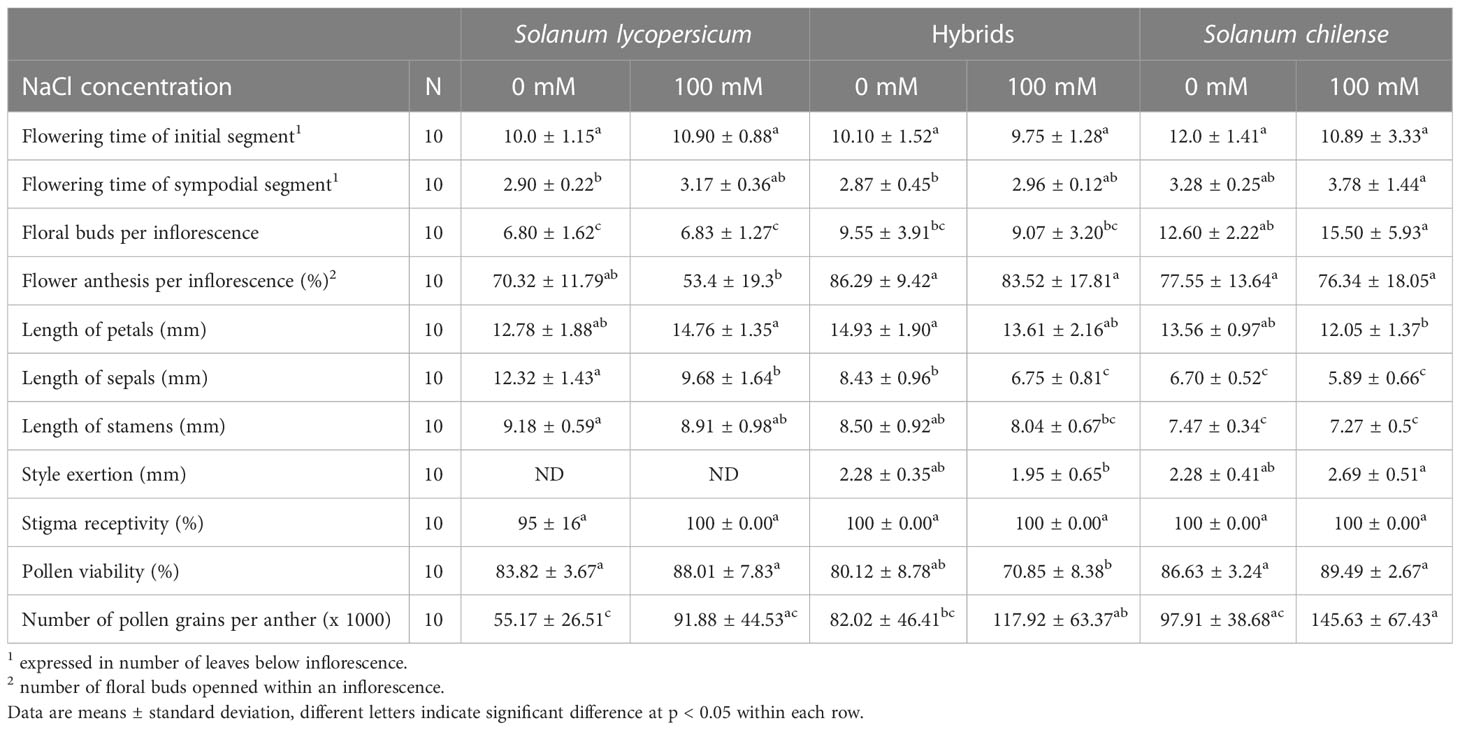
Table 1 Effects of salt stress on flowering parameters and flower morphology and fertility of Solanum lycopersicum, Solanum chilense and their hybrids grown at 0 and 100 mM NaCl.
The flower morphology of the hybrids was intermediate between those of the parents (Figure S2C) Despite similar petal length between the three plant groups, sepal and stamen length of the hybrids were intermediate to those of S. lycopersicum and S. chilense. Hybrids presented a style exertion like Solanum chilense (Tables 1, S2). The only effect of NaCl on flower morphology was recorded on sepal length, which decreased in S. lycopersicum and hybrids but not in S. chilense (Tables 1, S2).
Regarding flower fertility parameters, stigma receptivity was affected neither by plant groups nor by salt stress (Tables 1, S2). The number of pollen grains per anther and pollen viability were affected by the plant group (Table S2) although no significant differences were observed under control conditions (Table 1). Salt did not alter pollen viability (Tables 1, S2) but tended to increase the number of pollen grains per anther (Table S2) although the difference was not significant inside a same plant group (Table 1).
3.3 Fruit production
The fruit set per inflorescence was slightly higher in the hybrids than in the parents while the seed set was 9 and 3 times lower in the hybrids than in S. lycopersicum and S. chilense, respectively (Tables 2, S2). The general appearance of hybrid fruits was closer to that of S. chilense than to S. lycopersicum, although this was not the case for all parameters (Figure S2A). Indeed, fruit FW, DW and diameter were intermediate in hybrids compared to parents but were closer to S. chilense than to S. lycopersicum (Tables 2, S2). Similarly, fruit sugar content was twice as high in hybrids than in S. lycopersicum but was slightly lower than in S. chilense (Tables 2, S2). However, hybrid and S. lycopersicum fruits had a higher WC and were more acidic than S. chilense fruits (Tables 2, S2).
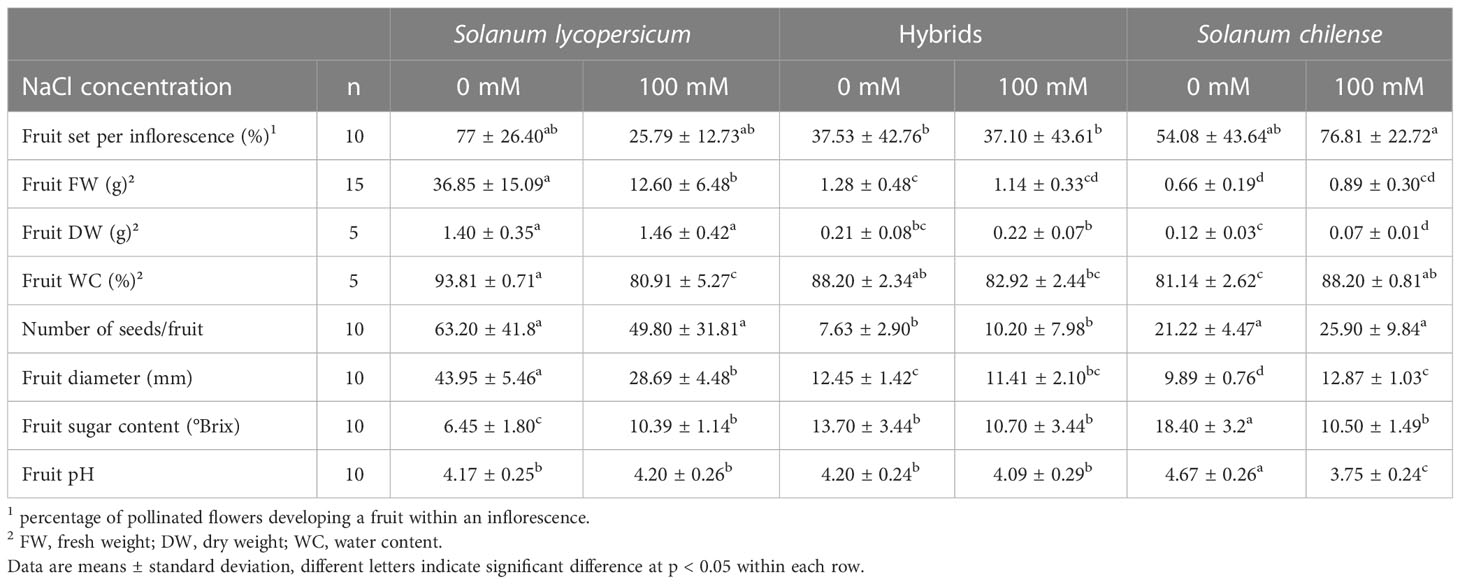
Table 2 Effects of salt stress on fructification parameters of Solanum lycopersicum, Solanum chilense and their hybrids grown at 0 and 100 mM NaCl.
Salt did not affect neither the fruit set per inflorescence nor the seed set in any of the 3 plant groups (Tables 2, S2). However, it affected fruit quality in parents but not in hybrids (Tables 2, S2). Indeed, salt decreased fruit FW in S. lycopersicum, fruit DW in S. chilense and fruit equatorial diameter in both species (Tables 2, S2). It also decreased fruit WC in S. lycopersicum but increased it in S. chilense. Salinity decreased fruit pH and sugar content in S. chilense and increased fruit sugar content in S. lycopersicum (Tables 2, S2).
3.4 Water status and photosynthesis parameters
Water content was similar among plant groups for a given organ and salt did not affect plant WC except for the stems of S. lycopersicum at 85 DASt (Tables 3, S2). Leaf ψs was intermediate in hybrids compared to parents and root ψs was lower in hybrids and S. chilense compared to S. lycopersicum under control conditions (Figures 3B, C, Table S2). NaCl decreased leaf ψs in S. lycopersicum and S. chilense but not in hybrids, while it decreased root ψs in all plant groups (Figures 3B, C, Table S2).
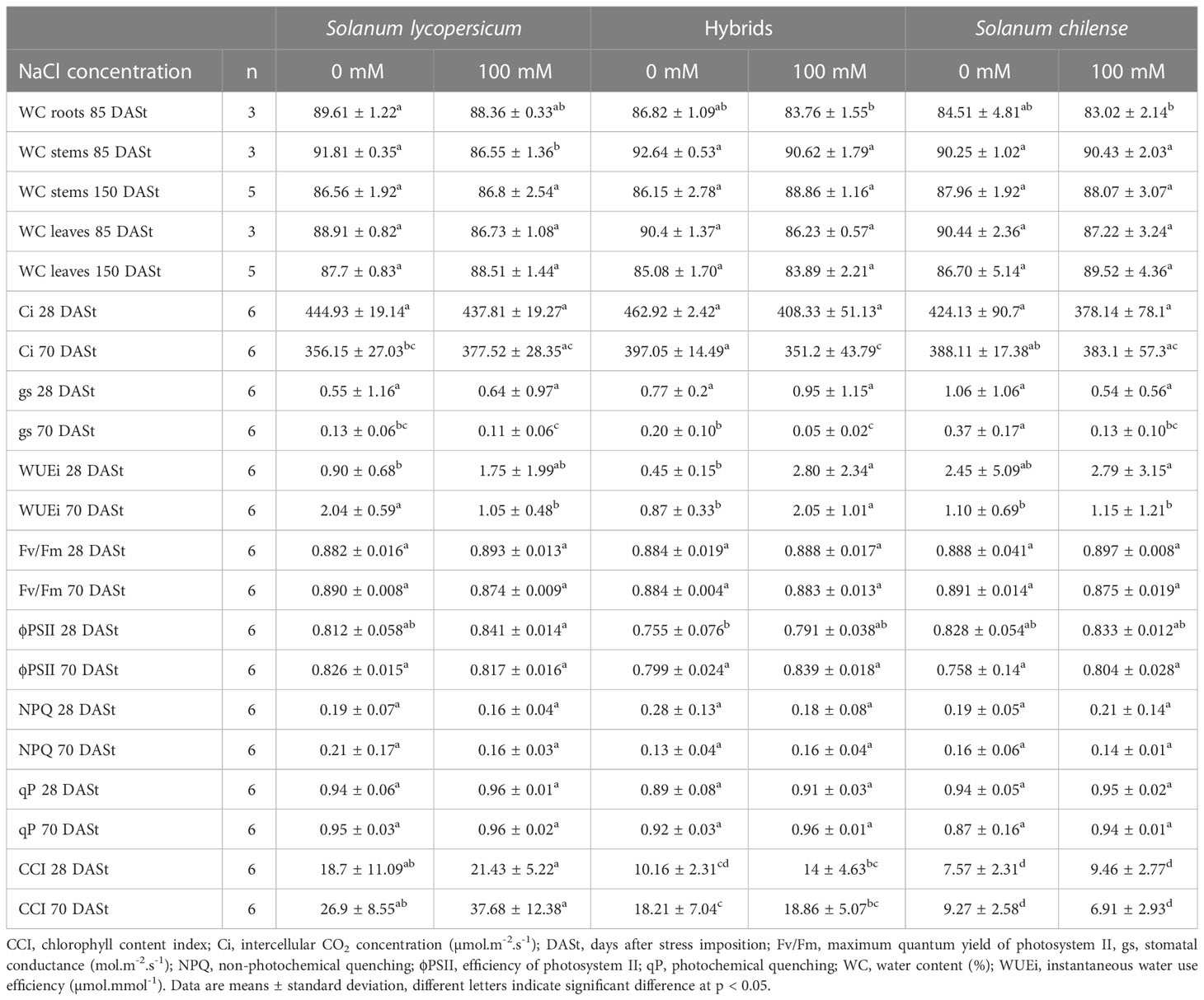
Table 3 Effects of salt stress on photosynthesis and water status parameters of Solanum lycopersicum, Solanum chilense and their hybrids grown at 0 and 100 mM NaCl.
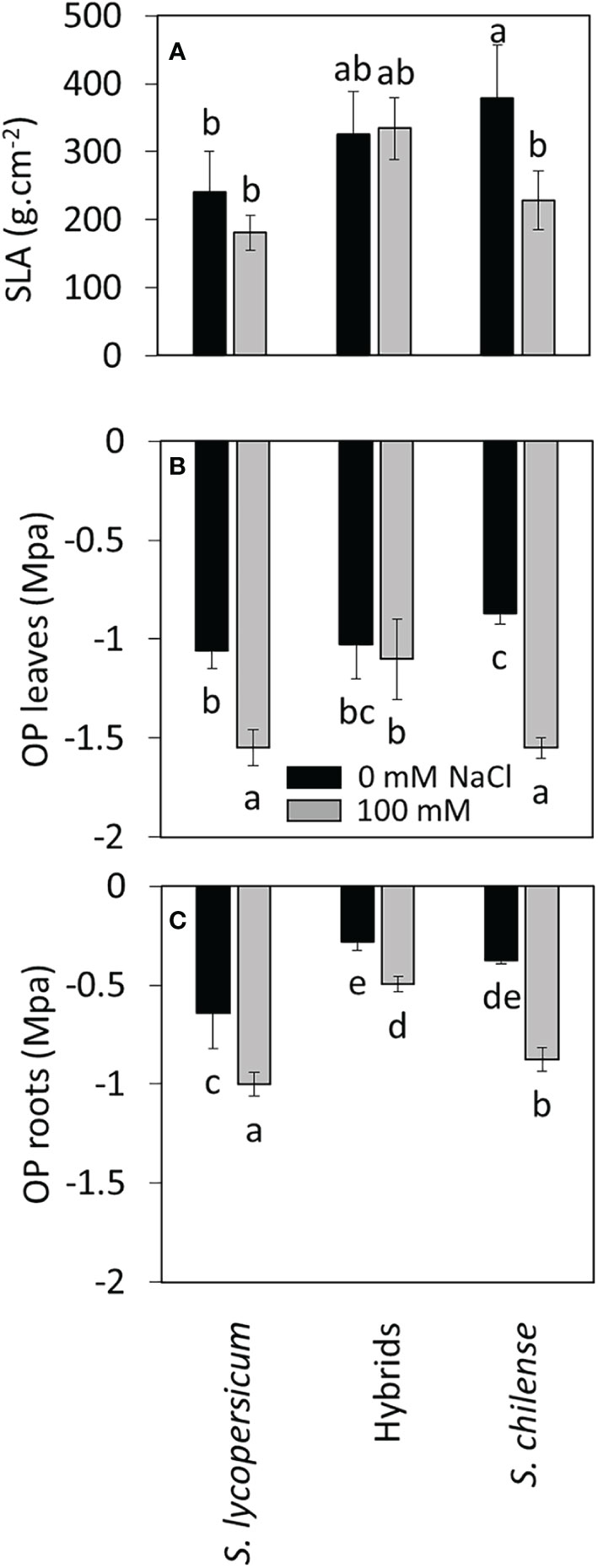
Figure 3 Effects of salt stress (0 mM and 100 mM NaCl) on specific leaf area and osmotic potential of Solanum lycopersicum, Solanum chilense and their hybrids 85 days after stress imposition. (A) Specific leaf area (SLA) of the 6th youngest leaf. (B, C) Osmotic potential (OP) of (B) the 5th youngest leaf and (C) the first secondary root. Data are means ± standard deviation, different letters indicate significant difference at p < 0.05.
Under control conditions, the leaf area of the first mature leaf was the highest in S. lycopersicum, followed by the hybrids and the smallest in S. chilense (Figure 4A, Table S2). As a result, the specific leaf area (SLA) of hybrids was intermediate between their parents under control conditions (Figure 3A, Table S2). Salt decreased the leaf area of S. lycopersicum at 70 DASt (Figure 4B, Table S2) and the SLA of S. chilense at 85 DASt (Figure 3C, Table S2) without affecting these parameters in the hybrids. Stomatal density differed among plant groups but was not affected by salinity (Figure 4B, Table S2): it was intermediate in hybrids compared to parents at 28 DASt, but closer to that of S. lycopersicum at 70 DASt (Figure 4B, Table S2).
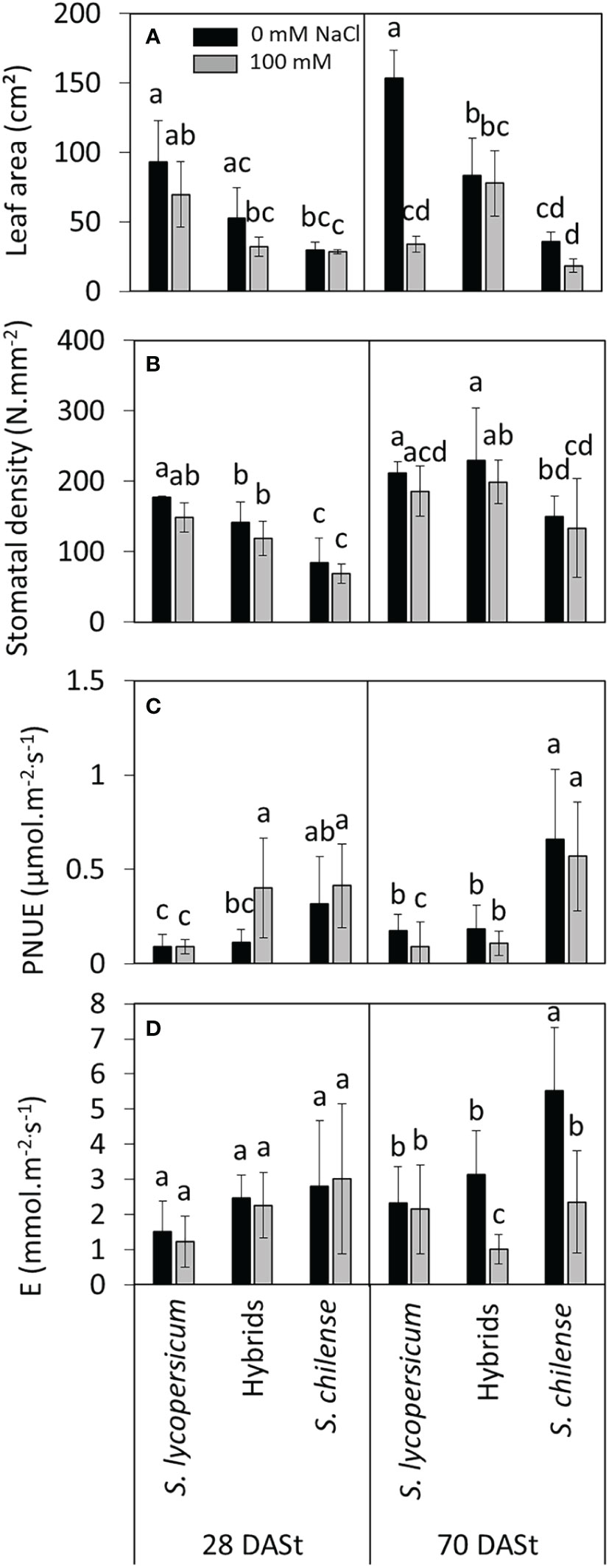
Figure 4 Effects of salt stress (0 mM and 100 mM NaCl) on leaf parameters of Solanum lycopersicum, Solanum chilense and their hybrids at 28 and 70 days after stress imposition (DASt). (A) Leaf area, (B) abaxial stomatal density (number/mm²), (C) photosynthetic nitrogen use efficiency (PNUE) and (D) instantaneous transpiration of the 5th youngest leaf. Data are means ± standard deviation, different letters indicate significant difference at p < 0.05 for a same date.
The photosynthetic nitrogen use efficiency (PNUE) was the highest in S. chilense under control condition (Figure 4C, Table S2) and salt increased PNUE of the hybrids at 28 DASt and decreased PNUE of S. lycopersicum at 70 DASt (Figure 4C, Table S2). Stomatal conductance (gs), intercellular CO2 concentration (Ci) and instantaneous transpiration (E) were only affected at 70 DASt (Table 3, Figure 4D, Table S2): gs was the highest in S. chilense under control condition and decreased with salt in hybrids and S. chilense; Ci was the lowest in S. lycopersicum and decreased with salt in hybrids; E was the highest in S. chilense and decreased with salt in both S. chilense and hybrids. Instantaneous water use efficiency (WUEi) was the highest in S. lycopersicum under control condition at 70 DASt but decreased with salt in the latter while it increased with salt in hybrids (Tables 3, S2). All chlorophyll fluorescence-related parameters (Fv/Fm, NPQ, qP) were similar between plant groups and remained unaffected by NaCl, except ϕPSII at 28 DASt which was slightly lower in hybrids than in parents (Tables 3, S2).
3.5 Biochemical parameters
Soluble sugars were more than 2.5 times more concentrated in inflorescences than in leaves (Table 4, t78.6 = 12.32, p < 0.001). Under control condition, the leaf soluble sugars concentration was similar between plant groups. However, as far as inflorescences were concerned, hybrids and S. chilense had higher soluble sugars concentrations than S. lycopersicum (Tables 4, S2). NaCl affected soluble sugar concentration only in leaves, where it increased mostly in S. chilense (Tables 4, S2). Proline concentration in leaves was low under control condition, especially in S. chilense and it increased with salt by 8.2, 3.3 and 161.5 times in S. lycopersicum, hybrids and S. chilense, respectively (Tables 4, S2). The MDA concentration was 1.5 times higher in inflorescences than in leaves (t70 = -5.071, p < 0.001). The MDA concentration in leaves was similar between plant groups while its concentration in inflorescences was slightly higher in S. lycopersicum and hybrids than in S. chilense (Tables 4, S2). Salt slightly increased MDA concentrations in the leaves (Tables 4, S2).
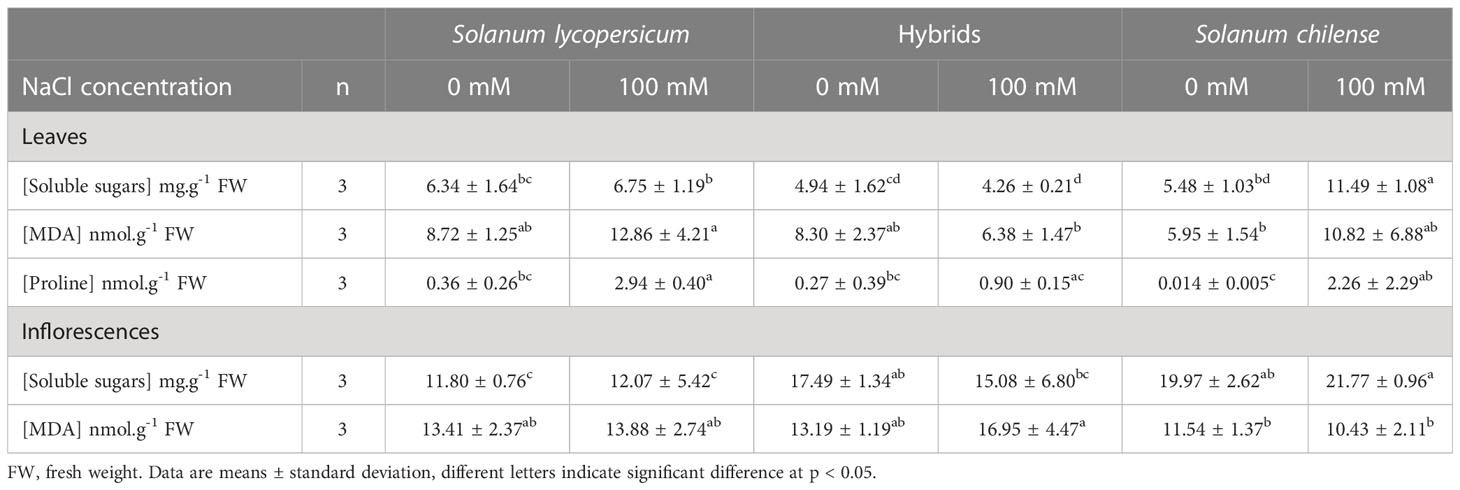
Table 4 Effects of salt stress on biochemical parameters of Solanum lycopersicum, Solanum chilense and their hybrids grown at 0 and 100 mM NaCl.
3.6 Mineral quantification
3.6.1 Sodium
The Na+ concentration was similar among plant groups in control conditions. Considering the whole plant, under salinity condition at 85 DASt, the Na+ concentration was the lowest in S. lycopersicum and the highest in hybrids (Figure 5A, Table S2). However, at 150 DASt, the difference between plant groups was not significant anymore (Figure 5A, Table S2). At 85 DASt, the increase of Na+ concentration between control and salted conditions was the highest in hybrids (Figures 5B–D) while at 150 DASt, this relative increase was lower (Figures 5B–D). Such increase in Na+ concentration was clearly lower in S. lycopersicum and S. chilense. As a result, at 85 DASt, the hybrids had a Na+ concentration under salinity close to S. lycopersicum in roots and close to S. chilense in stems and leaves (Figures 5B–D, Table S2). In contrast, at 150 DASt, the Na+ concentration was similar in stressed plants of all three plant groups in stems and leaves but lower in roots of S. chilense (Figures 5B–D, Table S2). The root/shoot ratio of Na+ quantity differed among plant groups at both 85 and 150 DASt while salt affected it only at 150 DASt (Figure 5E, Table S2). Under control condition, the root/shoot ratio of Na+ quantity was the highest in S. lycopersicum and the lowest in S. chilense. In hybrids, the ratio was intermediate between parents at 85 DASt and close to S. chilense at 150 DASt (Figure 5E). Comparing only stressed plants, the root/shoot ratio of Na+ was similar in the three plant groups at 85 DASt (F = 1.685, p = 0.263) and at 150 DASt, it was intermediate in hybrids between S. lycopersicum (highest) and S. chilense (lowest)(F = 6.914, p = 0.011), although such difference was not observed for post-hoc test comparing both salt conditions (Figure 5E).
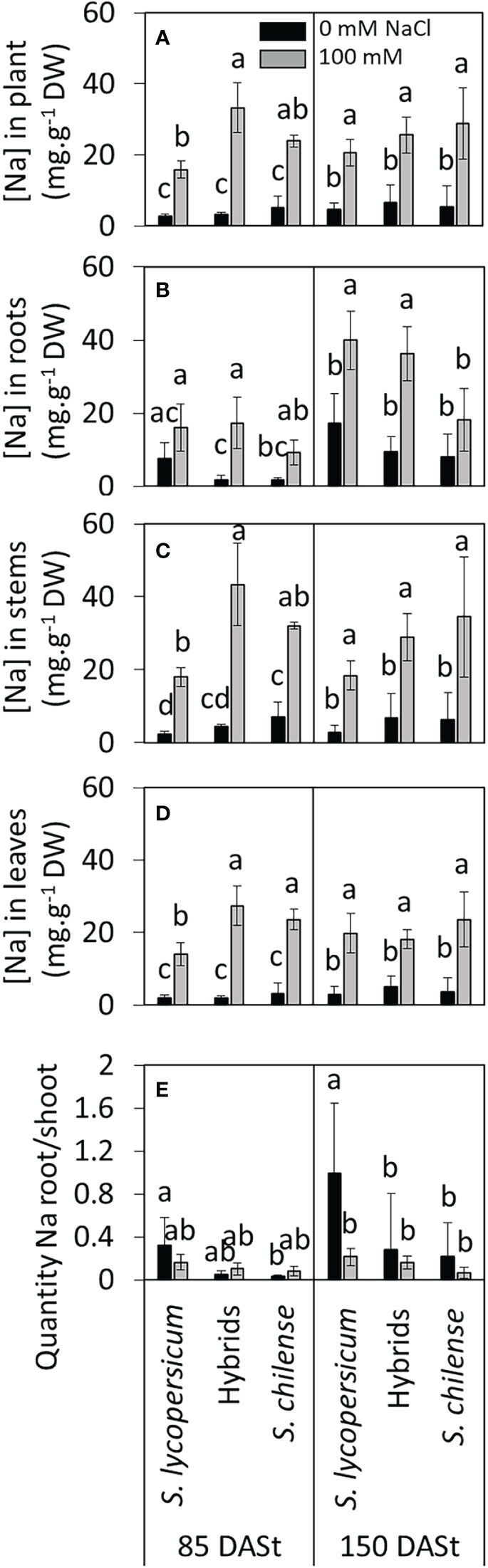
Figure 5 Effects of salt stress (0 mM and 100 mM NaCl) on Na+ accumulation in Solanum lycopersicum, Solanum chilense and their hybrids at 85 and 150 days after stress imposition (DASt). (A–D) Concentration of sodium in (A) the whole plant, (B) the roots, (C) the stems and (D) the leaves; (E) ratio of quantities of Na+ between roots and shoots. Data are means ± standard deviation, different letters indicate significant difference at p < 0.05 for a same date.
As far as reproductive organs are concerned, Na+ concentration in inflorescences of stressed plants was lower than in vegetative organs (t27.255 = -4.509, p < 0.001). It was higher in S. chilense than in S. lycopersicum and hybrids (Figure 6A, Table S2). In the fruits, the Na+ concentration was higher in pericarp than in the seeds (Figures 6B, C, F = 39.955, p < 0.001). In salt-treated plants, the Na+ concentration in pericarp was higher in S. chilense than in the other plant groups (Figures 6A, B, Table S2) while seed Na+ concentration was similar among the three plant groups (Figure 6C, Table S2).
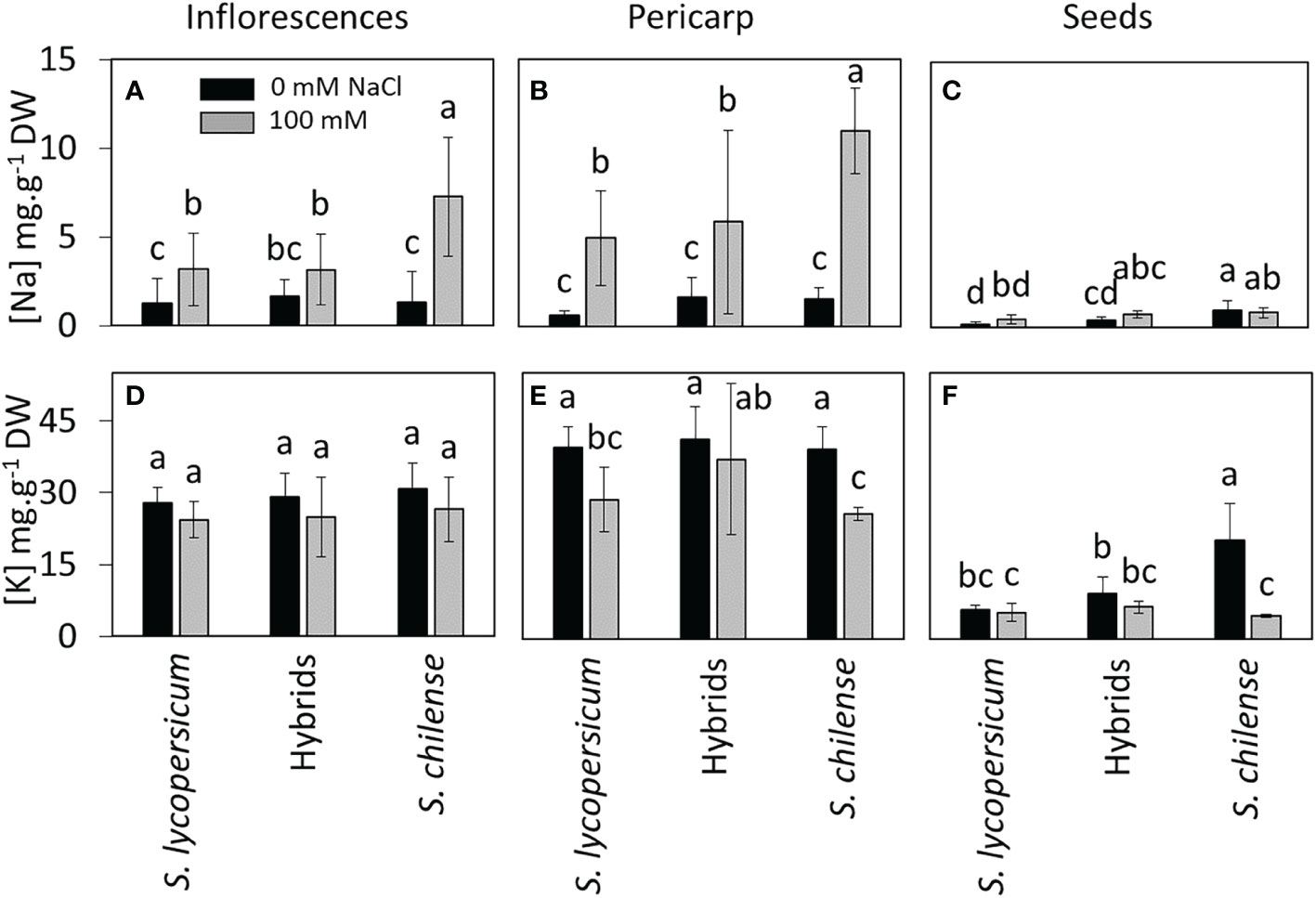
Figure 6 Effects of salt stress (0 mM and 100 mM NaCl) on Na+ and K+ concentrations in the reproductive organs of Solanum lycopersicum, Solanum chilense and their hybrids. Concentration of (A–C) sodium and (D–F) potassium in (A, D) inflorescences, (B, E) fruit pericarp and (C, F) seeds. Data are means ± standard deviation, different letters indicate significant difference at p < 0.05.
3.6.2 Potassium
In control conditions, the K+ concentration in the plant at 85 DASt was higher in hybrids than in S. lycopersicum while such a difference was not recorded at 150 DASt (Figure 7A, Table S2). Salinity decreased K+ concentration in S. chilense at 85 and 150 DASt, in hybrids at 85 DASt but did not affect it in S. lycopersicum (Figure 7A, Table S2). The response of each plants group differed depending on the organ and the considered date (Figures 7B–D). In roots, K+ concentration was higher in hybrids compared to the two other plant groups at 85 DASt under control condition and salt had only a limited impact (Figure 7B, Table S2). In stems, the highest K+ concentration was observed in hybrids at 85 DASt and in S. chilense at 150 DASt and the lowest in S. lycopersicum under control condition while K+ concentration was similar among plant groups under salinity (Figure 7C, Table S2). In leaves, K+ concentration was neither affected by plant groups nor by NaCl (Figure 7D; Table S2).
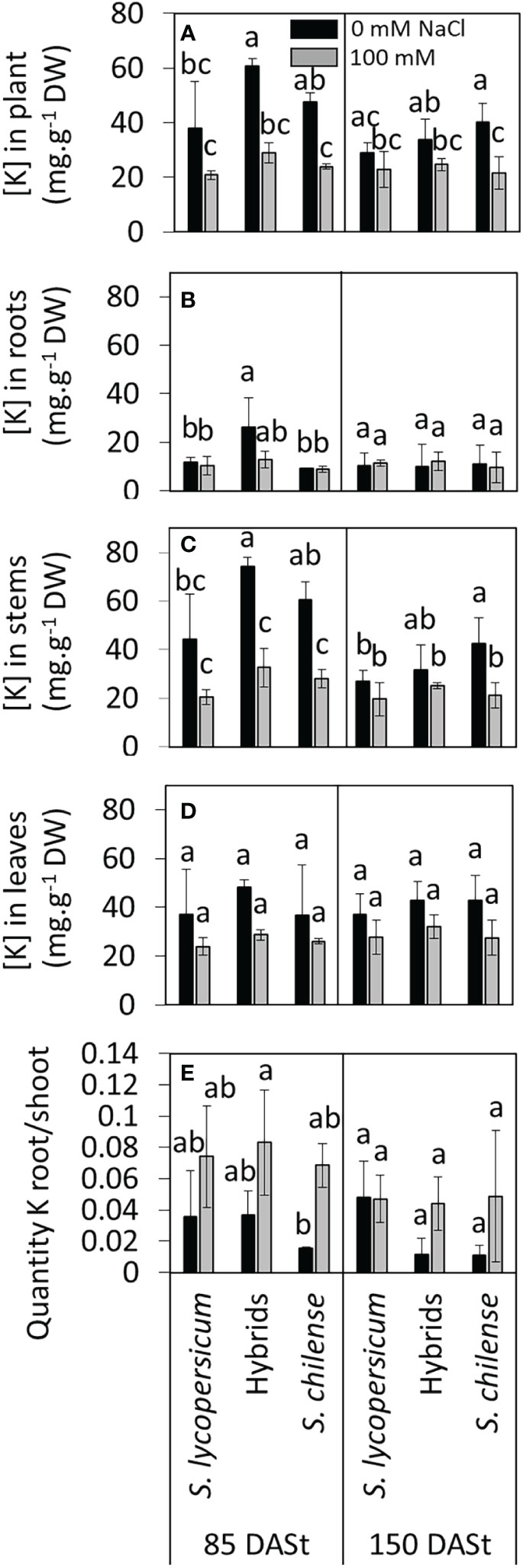
Figure 7 Effects of salt stress (0 mM and 100 mM NaCl) on K+ accumulation in Solanum lycopersicum, Solanum chilense and their hybrids at 85 and 150 days after stress imposition (DASt). (A–D) Concentration of potassium in (A) the whole plant, (B) the roots, (C) the stems and (D) the leaves; (E) ratio of quantities of K+ between roots and shoots. Data are means ± standard deviation, different letters indicate significant difference at p < 0.05 for a same date.
The root/shoot K+ ratio was similar whatever the plant groups and slightly increased with salt, mainly in hybrids and S. chilense at 85 DASt (Figure 7E; Table S2).
In control conditions, K+ concentration was slightly lower in reproductive than in vegetative organs (t26.789 = -3.928, p < 0.001) and was not affected by plant groups and salt treatment (Figure 6D, Table S2). In fruits, K+ concentration was higher in pericarp than in seeds (Figures 6E–F, F = 211.34, p < 0.001). In pericarp, salinity decreased K+ concentration in the two parent species but not in hybrids (Figure 6E, Table S2). In seeds, S. chilense had a higher K+ concentration than S. lycopersicum and hybrids and salt decreased K+ concentration in S. chilense only (Figure 6F, Table S2).
4 Discussion
4.1 Hybrids exhibited an intermediate phenotype between parents
Figure 8 summarizes the response of the three plant groups to salinity. We observed that hybrids globally exhibited an intermediate phenotype between S. lycopersicum and S. chilense. Similar results were previously reported for interspecific crosses within the tomato clade or between other species (Baek et al., 2016; Ghani et al., 2020). Ghani et al. (2020) crossed S. lycopersicum with three wild Solanum species (S. pimpinellifolium, S. arcanum and S. pennellii) and observed that most of the hybrids presented morphological parameters intermediate between parents, such as plant height, leaf length, number of fruits and fruit DW. However, this is not the case for all interspecific crosses: hybrids may show better or worse fitness compared to the parents (Hooftman et al., 2005; Rhode and Cruzan, 2005). In our study, even if an intermediate phenotype was globally observed, it was not the case for all recorded parameters. Morphologically, the general appearance of the hybrids was closer to S. lycopersicum than to the wild parent, especially regarding the vegetative parts. Indeed, the hybrids do not have the bushy appearance of S. chilense which has a high number of ramifications in the main stem and a decumbent profile (Peralta et al., 2008). Instead, the hybrids had a stem width and a leaf shape more similar to S. lycopersicum (Figure S2). Grafting is a technique more and more used in production and hybrids present thus a better morphological compatibility to S. lycopersicum than S. chilense. Thus, the use of hybrids, if they present a good resistance to salinity, could be an alternative to the grafting of thin wild tomato species (Singh et al., 2020). In terms of flower morphology, hybrids presented style exertion as observed in S. chilense. Style exertion is described as a marker of self-incompatibility (Rick et al., 1977; Chetelat et al., 2009) and S. lycopersicum x S. chilense hybrids are self-incompatible as their S. chilense parent (Martin, 1961). This is a feature already observed in others hybrids between self-compatible and self-incompatible species, as it has also been observed for hybrids between S. lycopersicum and S. peruvianum (McGuire and Rick, 1954) or Petunia hybrids (Ai et al., 1991). The fruits of the hybrids were also morphologically more similar to those of S. chilense than to S. lycopersicum: they remained green and smaller than the large red fruits of S. lycopersicum (Figure S2). In addition, the fruits of the hybrids contained fewer seeds than the parents despite the fact that flower fertility was similar in hybrids comparatively to parents, suggesting a reproductive barrier or inbreeding depression in the hybrids. The production of fruits with few seeds can be observed in self-compatible species x self-incompatible species crosses (Baek et al., 2016).
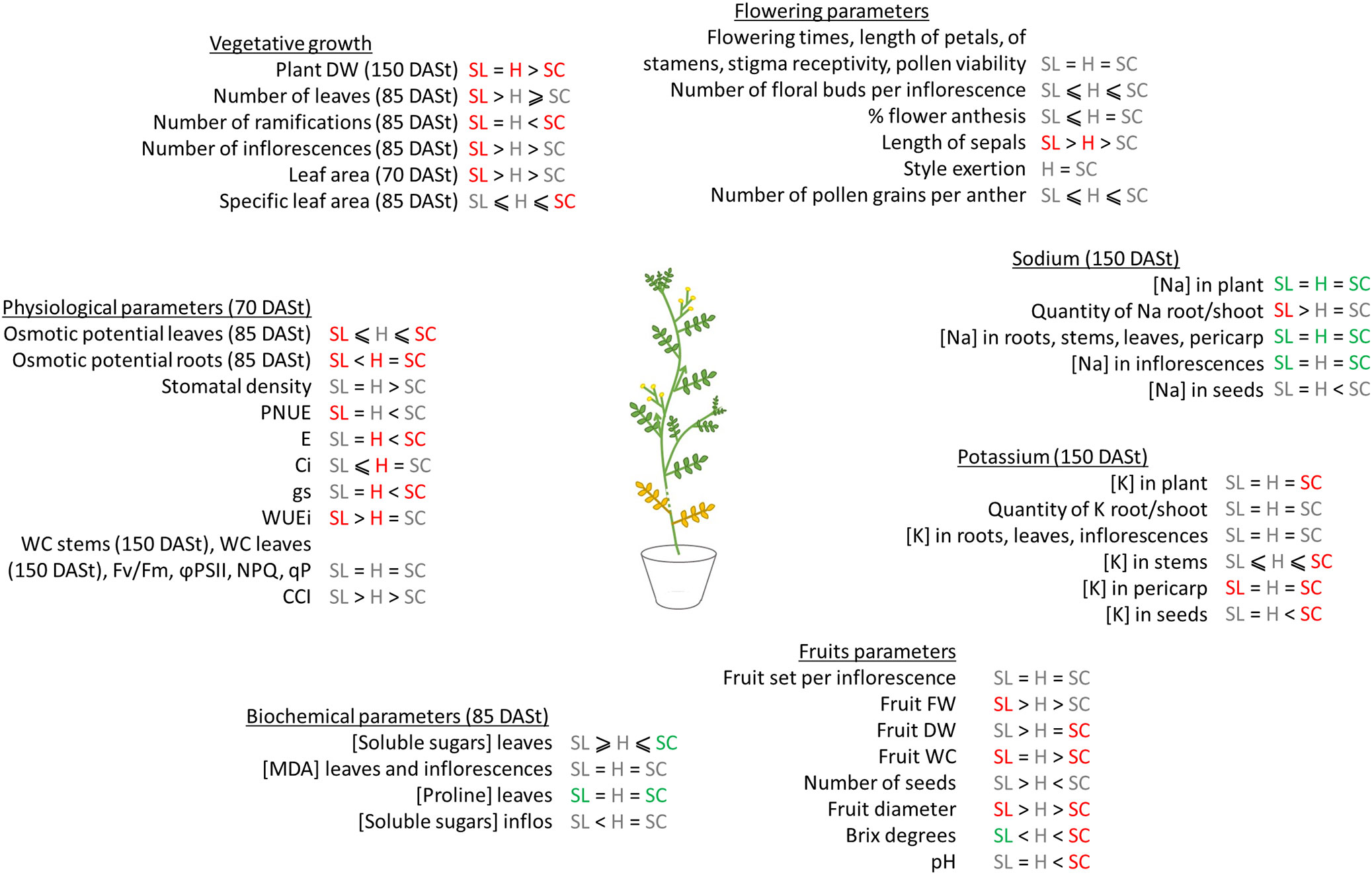
Figure 8 Summary graph of the main results of the study. SL, Solanum lycopersicum, H, hybrids, SC, Solanum chilense. The symbols represent the differences between the three groups of plants at 0 mM NaCl (=, equal; >, bigger; <, smaller; ≥ bigger or equal; ≤, smaller or equal) and colors represent the impact of NaCl on each group of plants (red, decrease with NaCl; green, increase with NaCl, grey, no modification with NaCl).
4.2 Tolerance to salt stress in hybrids
We observed that salinity decreased plant growth in the 3 plant groups although such decrease was only observed at 150 DASt in hybrids and S. chilense while plant growth decrease was already visible at 85 DASt in S. lycopersicum. This clearly suggests that hybrids and S. chilense were more salt resistant than S. lycopersicum. Solanum lycopersicum is described as a glycophyte species and S. chilense, as a halophyte species (Martínez et al., 2014; Gharbi et al., 2018). Since hybrids had an intermediate phenotype between parents and some characteristics were closer to S. lycopersicum and others to S. chilense, we could therefore suspect that the physiological behavior of hybrids could be sometimes closer to halophyte behavior and sometimes closer to glycophyte behavior (Cano et al., 1996; Uwimana et al., 2012; Aycan et al., 2021). In addition, interspecific hybrids may have better fitness for some traits than their parents (Rhode and Cruzan, 2005; Kumar et al., 2020).
As far as osmotic phase of salt stress is concerned, some accessions of S. chilense were reported to increase leaf succulence in response to salt stress, which is a characteristic enabling plant to cope with arid conditions (Martínez et al., 2014). In our study, salinity did not increase the leaf WC of S. chilense but decreased the SLA without affecting leaf area, which could indicate succulence in this species. However, our results suggested that hybrids could have a higher constitutive leaf succulence than the parents, since salinity decreased neither SLA nor leaf osmotic potential of hybrids in contrast to their parents. Moreover, instantaneous water use efficiency (WUEi) increased with salinity in hybrids in contrast to their parents. Increase of WUEi is considered as a salt tolerance mechanism, improving the capacity of the plant to limit water loss despite salinity toxic effects (Martínez et al., 2003; Yan et al., 2015; Tarin et al., 2020). Together, these results suggested a greater tolerance to osmotic phase in hybrids compared to the parents.
Individual leaf area was not significantly decreased by salt in hybrids, in contrast to S. lycopersicum. A decrease in leaf area can be considered as an avoidance mechanism by reducing evapotranspiration and by extension the amount of Na+ in the shoots (Munns and Tester, 2008). It can also be considered a symptom of salt stress, which may reflect a decrease in cell expansion, common in osmotic stress, but a measurement of mesophyll cell diameter would be necessary to confirm this symptom (Tenhaken, 2015). However, instantaneous transpiration (E) was importantly decreased by salt in hybrids 70 DASt as observed for S. chilense, in contrast to S. lycopersicum. This decrease in E was not due to a regulation of the number of stomata but to a decrease in stomatal conductance (gs). Plants close their stomata to avoid transpiration and water loss (Tapia et al., 2016; Hedrich and Shabala, 2018). In control conditions, S. chilense was the species with the lowest stomatal density but with the highest gs. It could mean that this species would have stomata able to open efficiently, maybe because of bigger stomata than the other plant groups. Indeed, stomatal conductance is not always positively correlated with stomatal density, but may also be influenced by the mean stomatal size (Morales-Navarro et al., 2018). Stomatal conductance and density in hybrids were closer to S. lycopersicum than to S. chilense in control conditions although hybrids behave more like S. chilense under salinity regarding transpiration regulation.
Solanum chilense and hybrids were able to maintain their photosynthetic nitrogen use efficiency (PNUE) under salt stress, despite the decrease in transpiration. In contrast, the glycophyte S. lycopersicum was unable to maintain PNUE values in salt stress conditions. In terms of tolerance strategies, the stability of PNUE of hybrids under salt stress would enable them to synthesize proteins and osmoprotectants (Shabala, 2013; Slama et al., 2015). However, we observed no increase of soluble sugars nor proline concentrations in hybrids with salt stress. This could be related to the fact that salt did not decrease leaf osmotic potential in hybrids and that osmotic adjustment was not needed. Osmotic adjustment is performed with a high metabolic cost (Shabala, 2013). It is possible that hybrids directed their metabolism toward limiting salt stress-induced water loss rather than osmotic adjustment. In contrast, osmotic adjustment was observed in leaves of both parents; salt respectively increased sugar and proline concentrations in the leaves of S. chilense and S. lycopersicum. It was previously reported that proline contributed more to osmotic adjustment in S. lycopersicum than in S. chilense (Tapia et al., 2016; Gharbi et al., 2017). Moreover, under salt stress, ions (K+, Na+) were shown to be the main contributors to osmotic adjustment in both species, mainly in S. chilense (Gharbi et al., 2017). In contrast to leaves, salt decreased root osmotic potential in the three plant groups, suggesting that osmotic adjustment was at least required in roots of the hybrids. It is likely that K+ and Na+ ions contribute to this osmotic adjustment as observed previously for S. lycopersicum and S. chilense (Gharbi et al., 2017). However, since Na+ is highly toxic for cytosolic enzymes, including in halophytes, it has to be sequestered in vacuoles to display an efficient contribution to the whole cell osmotic adjustment (Flowers et al., 2015).
Despite an increase of Na+ concentration in the three plant groups under salinity, its distribution and concentration in the plants were different between plant groups and between the two dates of measurements. Surprisingly, hybrids accumulated more Na+ under salt stress than the parents at 85 DASt while the Na+ concentration was similar between plant groups at 150 DASt. Na+ concentration increased with time in S. lycopersicum under salinity but not in S. chilense and hybrids, suggesting that S. lycopersicum limited the Na+ accumulation on a short-term basis but could not maintain this strategy on a long-term basis. In contrast the two other plant groups behave as typical includers and accumulated Na+ since the beginning. It has been shown that at a very short exposure to NaCl (7 days of 125 mM NaCl), S. lycopersicum was able to retain a majority of Na+ in its roots parts, avoiding exposure of aerial parts to Na+ while S. chilense mainly accumulated Na+ in the shoots (Gharbi et al., 2016). Solanum lycopersicum has thus been described as an excluder species and S. chilense, as an includer species (Gharbi et al., 2016). An excluder strategy enables the plant to protect its photosynthetic apparatus from the Na+ toxicity. However, to adjust osmotically, plants require the use of osmotic compounds, and notably osmoprotectants that could be energetically costly (Shabala, 2013; Flowers et al., 2015). An includer strategy enables the plant to use minerals instead of compatible osmolytes for osmotic adjustment, thereby reducing the cost in energy (Shabala, 2013; Almeida et al., 2014). Hybrids seemed to have an intermediate accumulator behavior compared to their parents as they accumulated more Na+ in the roots than S. chilense (at 150 DASt) and in the shoots than S. lycopersicum (at 85 DASt) under salt stress, explaining the higher total Na+ accumulation. Pérez-Alfocea et al. (1994) and Ali et al. (2021) showed that the salt-tolerant species Solanum pennellii also accumulated more Na+ than S. lycopersicum in shoots and that their hybrids had intermediate Na+ concentration in stems and leaves (Pérez-Alfocea et al., 1994; Ali et al., 2021). This suggests that halophyte wild species can maintain metabolism despite Na+ accumulation and could be related to a more efficient Na+ vacuolar compartmentation at the cell level.
A decrease of K+ concentration is frequently observed in response to NaCl stress and often results from a lack of specificity of some transporters for Na+ and K+ (Ghanem et al., 2009; Gharbi et al., 2017). On the contrary, an increase in K+ concentration has already been observed in S. chilense during a short-term exposure to salinity (Gharbi et al., 2017). However, in our experiment, salt decreased K+ concentration with a stronger decrease in S. chilense than in S. lycopersicum and an intermediate decrease in the hybrids at the whole plant level; this decrease was mostly due to a decrease in K+ concentrations in the stems. This suggests that the impact of salt on K+ concentration in S. chilense may depend on the stress duration. Partly in accordance with our results, Pérez-Alfocea et al. (1994) did not observe a decrease in K+ concentration in stems and leaves of S. lycopersicum but observed a decrease in those of S. pennellii and hybrids. Similarly, Albaladejo et al. (2017) observed a greater reduction in K+ concentration in S. pennellii leaves than in S. lycopersicum. It has been discussed that halophytes can substitute K+ for small amounts of Na+ up to a certain concentration at which it becomes toxic for plants (Albaladejo et al., 2017; de Souza Mateus et al., 2019). Salinity also increased the K+ leaves/stems ratio in S. chilense compared to control conditions by 3.3, suggesting that this species could use the mobile properties of K+ to direct it in leaves, where K+ is the most needed for photosynthesis and osmoregulation (Kanai et al., 2011). K+ concentration in leaves was indeed not affected by salinity whatever the plant groups in our study.
At the reproductive level, salinity decreased inflorescence production in S. lycopersicum but not in S. chilense and had an intermediate impact in hybrids. Moreover, it affected neither flower anthesis nor flower fertility, and had only a slight effect on the number of pollen grains per anther in S. chilense. Such a high number of pollen grains could be a way to compensate a potential loss of pollen viability often induced by salinity (Ghanem et al., 2009; de Storme and Geelen, 2014). Moreover, Alexander staining could overestimate the rate of pollen viability because it only distinguish aborted from non-aborted pollen grains (Dafni and Firmage, 2000). Compensation between pollen grain production and pollen viability was previously reported in S. chilense under salt stress (Bigot et al., 2022). The decrease of flower production and flower fertility under stress is often explained by a decrease in sugar allocation to the reproductive structures (Ghanem et al., 2009). Here, we observed that the soluble sugar concentration was higher in the inflorescences of S. chilense and hybrids than in those of S. lycopersicum and that it was not affected by salt, maybe explaining the maintenance of flower fertility. The Na+ accumulation in inflorescence was also lower than in the vegetative organs although it was higher in S. chilense than in S. lycopersicum and hybrids. Na+ is a toxic ion for plants, which commonly exclude toxic ions from reproductive structures (Munns and Tester, 2008; Baby et al., 2016; Romero-Aranda et al., 2020). It has been shown that Na+ repartition inside the flower differed between S. lycopersicum and S. chilense, (Bigot et al., 2022) but such analysis was not yet performed for hybrids. Salinity also decreased the length of sepals in S. lycopersicum and hybrids, as previously reported for S. lycopersicum (Bigot et al., 2022). Such decrease could limit the photosynthesis rate of flower and fruit (Herrera, 2005) as green reproductive organs play a role in fruit growth (Hetherington et al., 1998; Smillie et al., 1999). However, this decrease could not explain alone the decrease of fruit weight and size observed in S. lycopersicum since salt did not affect those parameters in hybrids. Hybrids had better yield-related parameters under salt stress than S. lycopersicum. Indeed, salt did not affect fruit parameters in hybrids while it decreased them in S. lycopersicum and mostly increased them in S. chilense as previously observed (Bigot et al., 2022, Martinez et al., 2012).
Salt also affected fruit quality. Water content and sugar content of hybrid fruits were not modified by salt. In contrast, salt decreased fruit WC and increased fruit sugar content in S. lycopersicum; maintaining a low ψs in fruits of S. lycopersicum by sugars is required for fruit growth (Bolarín et al., 2001; Balibrea et al., 2006; Bonarota et al., 2022). In S. chilense, salt increased fruit WC but decreased sugar content, suggesting the other osmoprotectants than sugars may be involved in osmotic adjustment. In contrast to S. chilense, the wild tomato species S. chmielewskii and S. cheesmaniae accumulated more soluble sugars in ripe fruits than S. lycopersicum under salinity, as well as their hybrids (Balibrea et al., 2006). In our study, pericarp of S. chilense accumulated large amounts of Na+, suggesting that S. chilense was able to use Na+ for osmotic adjustment in fruits. However, hybrids did not accumulate as much Na+ in pericarp than S. chilense but accumulated more K+, suggesting that K+ could assume a similar function. It was indeed shown that the salt-tolerant S. pimpinellifollium was able to use K+ and Na+ as osmotic compounds in fruits whereas S. lycopersicum was limited in its ability to use Na+ as osmotic compound (Bolarín et al., 2001). Whatever the Na+ accumulation in the fruits, the Na+ content in the seeds was limited in the three plant groups, showing that the plants protected the next generation. This is a common feature in plants that exclude toxic ions from reproductive structures (Munns and Tester, 2008; Baby et al., 2016; Romero-Aranda et al., 2020).
In summary, the hybrids maintained their overall growth under salt stress, with an intermediate behavior between the parents. They accumulated intermediate concentrations of ions between the parents, but are more salt tolerant than their glycophyte parent both at the vegetative and reproductive stages. Their architecture is more similar to that of S. lycopersicum, making them suitable for grafting as salt-tolerant rootstocks. However, their self-incompatibility and post-zygotic barriers make them difficult to cross. Further studies on their compatibility and salinity tolerance are needed to see their potential for the improvement of cultivated tomato.
Data availability statement
The original contributions presented in the study are included in the article/Supplementary Material. Further inquiries can be directed to the corresponding author.
Author contributions
SB, MQ, J-PM and SL contributed to conception and design of the study. SB, CL, CR performed the experiments and analyzed the data. SB performed the statistical analysis. SB and MQ wrote the first draft of the manuscript. CL, CR, J-PM, and SL wrote sections of the manuscript. All authors contributed to manuscript revision, read, and approved the submitted version
Funding
This research was funded by the Belgian “Fonds National de la Recherche” Scientifique (FRS-FNRS), grant number CDR J.0136.19, and by the WBI/Chili project number 17.
Acknowledgments
The authors are grateful to Brigitte Van Pee and Marie-Eve Renard for technical support.
Conflict of interest
The authors declare that the research was conducted in the absence of any commercial or financial relationships that could be construed as a potential conflict of interest.
Publisher’s note
All claims expressed in this article are solely those of the authors and do not necessarily represent those of their affiliated organizations, or those of the publisher, the editors and the reviewers. Any product that may be evaluated in this article, or claim that may be made by its manufacturer, is not guaranteed or endorsed by the publisher.
Supplementary material
The Supplementary Material for this article can be found online at: https://www.frontiersin.org/articles/10.3389/fhort.2023.1130702/full#supplementary-material
References
Ai Y., Kron E., Kao T. (1991). S-alleles are retained and expressed in a self-compatible cultivar of Petunia hybrida. Molec. Gen. Genet. 230, 353–358. doi: 10.1007/BF00280291
Albaladejo I., Meco V., Plasencia F., Flores F. B., Bolarin M. C., Egea I. (2017). Unravelling the strategies used by the wild tomato species Solanum pennellii to confront salt stress: From leaf anatomical adaptations to molecular responses. Environ. Exp. Bot. 135, 1–12. doi: 10.1016/j.envexpbot.2016.12.003
Alexander M. P. (1969). Differential staining of aborted and nonaborted pollen. Stain Technol. 44, 117–122. doi: 10.3109/10520296909063335
Ali A. A. M., Romdhane W. B., Tarroum M., Al-Dakhil M., Al-Doss A., Alsadon A. A., et al. (2021). Analysis of salinity tolerance in tomato introgression lines based on morpho-physiological and molecular traits. Plants 10, 2594. doi: 10.3390/plants10122594
Almeida P., de Boer G.-J., de Boer A. H. (2014). Differences in shoot na+ accumulation between two tomato species are due to differences in ion affinity of HKT1;2. J. Plant Physiol. 171, 438–447. doi: 10.1016/j.jplph.2013.12.001
Assaha D. V. M., Ueda A., Saneoka H., Al-Yahyai R., Yaish M. W. (2017). The role of na+ and k+ transporters in salt stress adaptation in glycophytes. Front. Physiol. 8. doi: 10.3389/fphys.2017.00509
Aycan M., Baslam M., Ozdemir B., Asiloglu R., Mitsui T., Yildiz M. (2021). Direct contribution of the maternal genotype on the transgenerational salinity tolerance in wheat (Triticum aestivum l.). Environ. Exp. Bot. 192, 104648. doi: 10.1016/j.envexpbot.2021.104648
Ayenan M. A. T., Danquah A., Ampomah-Dwamena C., Hanson P., Asante I. K., Danquah E. Y. (2020). Optimizing pollencounter for high throughput phenotyping of pollen quality in tomatoes. MethodsX 7, 100977. doi: 10.1016/j.mex.2020.100977
Baby T., Collins C., Tyerman S. D., Gilliham M. (2016). Salinity negatively affects pollen tube growth and fruit set in grapevines and is not mitigated by silicon. Am. J. Enol. Vitic. 67, 218–228. doi: 10.5344/ajev.2015.15004
Baek Y. S., Covey P. A., Petersen J. J., Chetelat R. T., McClure B., Bedinger P. A. (2015). Testing the SI × SC rule: Pollen-pistil interactions in interspecific crosses between members of the tomato clade (Solanum section Lycopersicon, solanaceae). Am. J. Bot. 102, 302–311. doi: 10.3732/ajb.1400484
Baek Y. S., Royer S. M., Broz A. K., Covey P. A., López-Casado G., Nuñez R., et al. (2016). Interspecific reproductive barriers between sympatric populations of wild tomato species ( Solanum section lycopersicon ). Am. J. Bot. 103, 1964–1978. doi: 10.3732/ajb.1600356
Balibrea M. E., Martínez-Andújar C., Cuartero J., Bolarín M. C., Pérez-Alfocea F. (2006). The high fruit soluble sugar content in wild lycopersicon species and their hybrids with cultivars depends on sucrose import during ripening rather than on sucrose metabolism. Funct. Plant Biol. 33, 279. doi: 10.1071/FP05134
Bates L. S., Waldren R. P., Teare I. D. (1973). Rapid determination of free proline for water-stress studies. Plant Soil 39, 205–207. doi: 10.1007/BF00018060
Bigot S., Pongrac P., Šala M., van Elteren J. T., Martínez J.-P., Lutts S., et al. (2022). The halophyte species Solanum chilense dun. maintains its reproduction despite sodium accumulation in its floral organs. Plants 11, 672. doi: 10.3390/plants11050672
Blanchard-Gros R., Bigot S., Martinez J.-P., Lutts S., Guerriero G., Quinet M. (2021). Comparison of drought and heat resistance strategies among six populations of Solanum chilense and two cultivars of Solanum lycopersicum. Plants 10, 1720. doi: 10.3390/plants10081720
Bolarín M. C., Estañ M. T., Caro M., Romero-Aranda R., Cuartero J. (2001). Relationship between tomato fruit growth and fruit osmotic potential under salinity. Plant Sci. 160, 1153–1159. doi: 10.1016/S0168-9452(01)00360-0
Bonarota M.-S., Kosma D. K., Barrios-Masias F. H. (2022). Salt tolerance mechanisms in the Lycopersicon clade and their trade-offs. AoB Plants 14, plab072. doi: 10.1093/aobpla/plab072
Böndel K. B., Lainer H., Nosenko T., Mboup M., Tellier A., Stephan W. (2015). North–south colonization associated with local adaptation of the wild tomato species Solanum chilense. Mol. Biol. Evol. 32, 2932–2943. doi: 10.1093/molbev/msv166
Brukhin V., Hernould M., Gonzalez N., Chevalier C., Mouras A. (2003). Flower development schedule in tomato Lycopersicon esculentum cv. sweet cherry. Sex Plant Reprod. 15, 311–320. doi: 10.1007/s00497-003-0167-7
Campos C. A. B., Fernandes P. D., Gheyi H. R., Blanco F. F., Gonçalves C. B., Campos S. A. F. (2006). Yield and fruit quality of industrial tomato under saline irrigation. Sci. Agric. (Piracicaba Braz.) 63, 146–152. doi: 10.1590/S0103-90162006000200006
Cano E. A., Pérez-Alfocea F., Moreno V., Bolarín M. C. (1996). Responses to NaCl stress of cultivated and wild tomato species and their hybrids in callus cultures. Plant Cell Rep. 15, 791–794. doi: 10.1007/BF00232231
Chetelat R. T., Pertuzé R. A., Faúndez L., Graham E. B., Jones C. M. (2009). Distribution, ecology and reproductive biology of wild tomatoes and related nightshades from the atacama desert region of northern Chile. Euphytica 167, 77–93. doi: 10.1007/s10681-008-9863-6
Chetelat R. T., Qin X., Tan M., Burkart-Waco D., Moritama Y., Huo X., et al. (2019). Introgression lines of Solanum sitiens , a wild nightshade of the atacama desert, in the genome of cultivated tomato. Plant J. 100, 836–850. doi: 10.1111/tpj.14460
Dafni A., Firmage D. (2000). Pollen viability and longevity: Practical, ecological and evolutionary implications. Plant Syst. Evol. 222, 113–132. doi: 10.1007/BF00984098
Dafni A., Maués M. M. (1998). A rapid and simple procedure to determine stigma receptivity. Sex Plant Reprod. 11, 177–180. doi: 10.1007/s004970050138
de Souza Mateus N., Victor de Oliveira Ferreira E., Arthur Junior J. C., Domec J.-C., Jordan-Meille L., Leonardo de Moraes Gonçalves J., et al. (2019). The ideal percentage of K substitution by Na in eucalyptus seedlings: Evidences from leaf carbon isotopic composition, leaf gas exchanges and plant growth. Plant Physiol. Biochem. 137, 102–112. doi: 10.1016/j.plaphy.2019.02.006
de Storme N., Geelen D. (2014). The impact of environmental stress on male reproductive development in plants: biological processes and molecular mechanisms: Environmental impact on male gamete development. Plant Cell Environ. 37, 1–18. doi: 10.1111/pce.12142
FAO (2021). Global map of salt-affected soils. v1.0. Available online: https://www.fao.org/soils-portal/data-hub/soil-maps-and-databases/global-map-of-salt-affected-soils/en/ (accessed on 25 September 2021)
FAOStats (2022). Tomato yields and cultivated area of tomato in the world. Food Agric. Organization. Available online: https://www.fao.org/faostat/en/#data/QCL (accessed on 18 November 2022)
Flowers T. J., Munns R., Colmer T. D. (2015). Sodium chloride toxicity and the cellular basis of salt tolerance in halophytes. Ann. Bot. 115, 419–431. doi: 10.1093/aob/mcu217
Ghanem M. E., van Elteren J., Albacete A., Quinet M., Martínez-Andújar C., Kinet J.-M., et al. (2009). Impact of salinity on early reproductive physiology of tomato (Solanum lycopersicum) in relation to a heterogeneous distribution of toxic ions in flower organs. Funct. Plant Biol. 36, 125. doi: 10.1071/FP08256
Ghani M. A., Abbas M. M., Amjad M., Ziaf K., Ali B., Shaheen T., et al. (2020). Production and characterisation of tomato derived from interspecific hybridisation between cultivated tomato and its wild relatives. J. Hortic. Sci. Biotechnol. 95, 506–520. doi: 10.1080/14620316.2019.1689182
Gharbi E., Lutts S., Dailly H., Quinet M. (2018). Comparison between the impacts of two different modes of salicylic acid application on tomato (Solanum lycopersicum) responses to salinity. Plant Signal. Behav. 13, e1469361. doi: 10.1080/15592324.2018.1469361
Gharbi E., Martínez J.-P., Benahmed H., Fauconnier M.-L., Lutts S., Quinet M. (2016). Salicylic acid differently impacts ethylene and polyamine synthesis in the glycophyte Solanum lycopersicum and the wild-related halophyte Solanum chilense exposed to mild salt stress. Physiol. Plant 158, 152–167. doi: 10.1111/ppl.12458
Gharbi E., Martínez J.-P., Benahmed H., Lepoint G., Vanpee B., Quinet M., et al. (2017). Inhibition of ethylene synthesis reduces salt-tolerance in tomato wild relative species Solanum chilense. J. Plant Physiol. 210, 24–37. doi: 10.1016/j.jplph.2016.12.001
Gibson M. J., Torres M., de L., Brandvain Y., Moyle L. C. (2021). Introgression shapes fruit color convergence in invasive galápagos tomato. eLife 10, e64165. doi: 10.7554/eLife.64165
Guo M., Wang X.-S., Guo H.-D., Bai S.-Y., Khan A., Wang X.-M., et al. (2022). Tomato salt tolerance mechanisms and their potential applications for fighting salinity: A review. Front. Plant Sci. 13. doi: 10.3389/fpls.2022.949541
Heath R., Packer L. (1968). Photoperoxidation in isolated chloroplasts. i. kinetics and stoichiometry of fatty acid peroxidation. Arch. Biochem. Biophys. 125, 189–198. doi: 10.1016/0003-9861(68)90654-1
Hedrich R., Shabala S. (2018). Stomata in a saline world. Curr. Opin. Plant Biol. 46, 87–95. doi: 10.1016/j.pbi.2018.07.015
Herrera C. M. (2005). Post-floral perianth functionality: contribution of persistent sepals to seed development in Helleborus foetidus (Ranunculaceae). Am. J. Bot. 92, 1486–1491. doi: 10.3732/ajb.92.9.1486
Hetherington S. E., Smillie R. M., Davies W. J. (1998). Photosynthetic activities of vegetative and fruiting tissues of tomato. J. Exp. Bot. 49, 1173–1181. doi: 10.1093/jxb/49.324.1173
Hooftman D. A. P., Oostermeijer J. G. B., Jacobs M. M. J., Den Nijs H. C. M. (2005). Demographic vital rates determine the performance advantage of crop-wild hybrids in lettuce: Demographic vital rates of hybrids of lettuce. J. Appl. Ecol. 42, 1086–1095. doi: 10.1111/j.1365-2664.2005.01086.x
Igic B., Smith W. A., Robertson K. A., Schaal B. A., Kohn J. R. (2007). Studies of self-incompatibility in wild tomatoes: I. s-allele diversity in Solanum chilense dun. (Solanaceae). Heredity 99, 553–561. doi: 10.1038/sj.hdy.6801035
Ji Y., Scott J. W., Schuster D. J., Maxwell D. P. (2009). Molecular mapping of Ty-4, a new tomato yellow leaf curl virus resistance locus on chromosome 3 of tomato. J. Amer. Soc Hortic. Sci. 134, 281–288. doi: 10.21273/JASHS.134.2.281
Kanai S., Moghaieb R. E., El-Shemy H. A., Panigrahi R., Mohapatra P. K., Ito J., et al. (2011). Potassium deficiency affects water status and photosynthetic rate of the vegetative sink in green house tomato prior to its effects on source activity. Plant Sci. 180, 368–374. doi: 10.1016/j.plantsci.2010.10.011
Kumar A., Sharma V., Jain B. T., Kaushik P. (2020). Heterosis breeding in eggplant (Solanum melongena l.): Gains and provocations. Plants 9, 403. doi: 10.3390/plants9030403
Lê S., Josse J., Husson F. (2008). FactoMineR: An R package for multivariate analysis. J. Stat. Soft. 25, i01. doi: 10.18637/jss.v025.i01
Lewis D., Crowe L. K. (1958). Unilateral interspecific incompatibility in flowering plants. Heredity 12, 233–256. doi: 10.1038/hdy.1958.26
Li T., Yang X., Yu Y., Si X., Zhai X., Zhang H., et al. (2018). Domestication of wild tomato is accelerated by genome editing. Nat. Biotechnol. 36, 1160–1163. doi: 10.1038/nbt.4273
Lutts S., Majerus V., Kinet J.-M. (1999). NaCl Effects on proline metabolism in rice (Oryza sativa) seedlings. Physiol. Plant 105, 450–458. doi: 10.1034/j.1399-3054.1999.105309.x
Martin F. W. (1961). The inheritance of self-incompatibility in hybrids of Lycopersicon esculentum mill x L. chilense dun. Genetics 46, 1443–1454. doi: 10.1093/genetics/46.11.1443
Martínez J.-P., Antúnez A., Pertuzé R., Acosta M. D. P., Palma X., Fuentes L., et al. (2012). Effects of Saline Water on Water Status, Yield and Fruit Quality of Wild (Solanum chilense) and Domesticated (Solanum lycopersicum Var. Cerasiforme) Tomatoes. Exp. Agric. 48, 573–586.
Martínez J. P., Antúnez A., Araya H., Pertuzé R., Fuentes L., Lizana X. C., et al. (2014). Salt stress differently affects growth, water status and antioxidant enzyme activities in Solanum lycopersicum and its wild relative Solanum chilense. Aust. J. Bot. 62, 359. doi: 10.1071/BT14102
Martínez J. P., Ledent J. F., Bajji M., Kinet J. M., Lutts S. (2003). Effect of water stress on growth, na+ and k+ accumulation and water use efficiency in relation to osmotic adjustment in two populations of Atriplex halimus l. Plant Growth Regul. 41, 63–73. doi: 10.1023/A:1027359613325
Mathieu A.-S., Lutts S., Vandoorne B., Descamps C., Périlleux C., Dielen V., et al. (2014). High temperatures limit plant growth but hasten flowering in root chicory (Cichorium intybus) independently of vernalisation. J. Plant Physiol. 171, 109–118. doi: 10.1016/j.jplph.2013.09.011
McGuire D. C., Rick C. M. (1954). Self-incompatibility in species of Lycopersicon sect. eriopersicon and hybrids with L. esculentum. Hilgardia 23, 101–124. doi: 10.3733/hilg.v23n04p101
Morales-Navarro S., Pérez-Díaz R., Ortega A., de Marcos A., Mena M., Fenoll C., et al. (2018). Overexpression of a SDD1-like gene from wild tomato decreases stomatal density and enhances dehydration avoidance in arabidopsis and cultivated tomato. Front. Plant Sci. 9. doi: 10.3389/fpls.2018.00940
Moyle L. C. (2008). Ecological and evolutionary genomics in the wild tomatoes (Solanum sect. lycopersicon). Evolution 62, 2995–3013. doi: 10.1111/j.1558-5646.2008.00487.x
Munns R., Tester M. (2008). Mechanisms of salinity tolerance. Annu. Rev. Plant Biol. 59, 651–681. doi: 10.1146/annurev.arplant.59.032607.092911
Nosenko T., Böndel K. B., Kumpfmüller G., Stephan W. (2016). Adaptation to low temperatures in the wild tomato species Solanum chilense. Mol. Ecol. 25, 2853–2869. doi: 10.1111/mec.13637
Paponov I. A., Engels C. (2003). Effect of nitrogen supply on leaf traits related to photosynthesis during grain filling in two maize genotypes with different n efficiency. Z. Pflanzenernähr. Bodenk. 166, 756–763. doi: 10.1002/jpln.200320339
Peralta I. E., Spooner D. M., Knapp S. (2008). Taxonomy of wild tomatoes and their relatives (Solanum sect. lycopersicoides, sect. juglandifolia, sect. lycopersicon; solanaceae). Syst. Bot. Monogr. 84, 1–186. doi: 10.2307/25027972
Pérez-Alfocea F., Guerrier G., Estan M. T., Bolarin M. (1994). Comparative salt responses at cell and whole-plant levels of cultivated and wild tomato species and their hybrid. J. Hortic. Sci. 69, 639–644. doi: 10.1080/14620316.1994.11516495
Pérez de Castro A., Julián O., Díez M. J. (2013). Genetic control and mapping of Solanum chilense LA1932, LA1960 and LA1971-derived resistance to tomato yellow leaf curl disease. Euphytica 190, 203–214. doi: 10.1007/s10681-012-0792-z
R Core Team (2018). R: A language and environment for statistical computing. R Foundation for Statistical Comuting, Vienna, Austria. URL: https://www.R-project.org/
Ramírez-Ojeda G., Peralta I. E., Rodríguez-Guzmán E., Chávez-Servia J. L., Sahagún-Castellanos J., Rodríguez-Pérez J. E. (2021a). Climatic diversity and ecological descriptors of wild tomato species (Solanum sect. lycopersicon) and close related species (Solanum sect. juglandifolia y sect. lycopersicoides) in Latin America. Plants 10, 855. doi: 10.3390/plants10050855
Ramírez-Ojeda G., Peralta I. E., Rodríguez-Guzmán E., Sahagún-Castellanos J., Chávez-Servia J. L., Medina-Hinostroza T. C., et al. (2021b). Edaphoclimatic descriptors of wild tomato species (Solanum sect. lycopersicon) and closely related species (Solanum sect. juglandifolia and sect. lycopersicoides) in south America. Front. Genet. 12. doi: 10.3389/fgene.2021.748979
Rawat N., Wungrampha S., Singla-Pareek S. L., Yu M., Shabala S., Pareek A. (2022). Rewilding staple crops for the lost halophytism: Toward sustainability and profitability of agricultural production systems. Mol. Plant 15, 45–64. doi: 10.1016/j.molp.2021.12.003
Rhode J. M., Cruzan M. B. (2005). Contributions of heterosis and epistasis to hybrid fitness. Am. Nat. 166, E124–E139. doi: 10.1086/491798
Rick C. M., Chetelat R. T. (1995). Utilization of related wild species for tomato improvement. Acta Hortic. 412, 21–38. doi: 10.17660/ActaHortic.1995.412.1
Rick C. M., Fobes J. F., Holle M. (1977). Genetic variation in Lycopersicon pimpinellifolium: Evidence of evolutionary change in mating systems. Pl. Syst. Evol. 127, 139–170. doi: 10.1007/BF00984147
Romero-Aranda M. R., González-Fernández P., Pérez-Tienda J. R., López-Diaz M. R., Espinosa J., Granum E., et al. (2020). Na+ transporter HKT1;2 reduces flower na+ content and considerably mitigates the decline in tomato fruit yields under saline conditions. Plant Physiol. Biochem. 154, 341–352. doi: 10.1016/j.plaphy.2020.05.012
Rothan C., Diouf I., Causse M. (2019). Trait discovery and editing in tomato. Plant J. 97, 73–90. doi: 10.1111/tpj.14152
Schreiber G., Reuveni M., Evenor D., Oren-Shamir M., Ovadia R., Sapir-Mir M., et al. (2012). ANTHOCYANIN1 from Solanum chilense is more efficient in accumulating anthocyanin metabolites than its Solanum lycopersicum counterpart in association with the ANTHOCYANIN FRUIT phenotype of tomato. Theor. Appl. Genet. 124, 295–307. doi: 10.1007/s00122-011-1705-6
Shabala S. (2013). Learning from halophytes: physiological basis and strategies to improve abiotic stress tolerance in crops. Ann. Bot. 112, 1209–1221. doi: 10.1093/aob/mct205
Shivaprasad P. V., Dunn R. M., Santos B. A., Bassett A., Baulcombe D. C. (2012). Extraordinary transgressive phenotypes of hybrid tomato are influenced by epigenetics and small silencing RNAs: RNA silencing influences transgressive hybrid phenotypes. EMBO J. 31, 257–266. doi: 10.1038/emboj.2011.458
Singh H., Kumar P., Kumar A., Kyriacou M. C., Colla G., Rouphael Y. (2020). Grafting tomato as a tool to improve salt tolerance. Agronomy 10, 263. doi: 10.3390/agronomy10020263
Slama I., Abdelly C., Bouchereau A., Flowers T., Savouré A. (2015). Diversity, distribution and roles of osmoprotective compounds accumulated in halophytes under abiotic stress. Ann. Bot. 115, 433–447. doi: 10.1093/aob/mcu239
Smillie R. M., Hetherington S. E., Davies W. J. (1999). Photosynthetic activity of the calyx, green shoulder, pericarp, and locular parenchyma of tomato fruit. J. Exp. Bot. 50, 707–718. doi: 10.1093/jxb/50.334.707
Stamova B. S., Chetelat R. T. (2000). Inheritance and genetic mapping of cucumber mosaic virus resistance introgressed from Lycopersicon chilense into tomato. Theor. Appl. Genet. 101, 527–537. doi: 10.1007/s001220051512
Sun Y., Yan F., Cui X., Liu F. (2014). Plasticity in stomatal size and density of potato leaves under different irrigation and phosphorus regimes. J. Plant Physiol. 171, 1248–1255. doi: 10.1016/j.jplph.2014.06.002
Tamburino R., Sannino L., Cafasso D., Cantarella C., Orrù L., Cardi T., et al. (2020). Cultivated tomato (Solanum lycopersicum l.) suffered a severe cytoplasmic bottleneck during domestication: Implications from chloroplast genomes. Plants 9, 1443. doi: 10.3390/plants9111443
Tapia G., Méndez J., Inostroza L. (2016). Different combinations of morpho-physiological traits are responsible for tolerance to drought in wild tomatoes Solanum chilense and Solanum peruvianum. Plant Biol. 18, 406–416. doi: 10.1111/plb.12409
Tarin T., Nolan R. H., Medlyn B. E., Cleverly J., Eamus D. (2020). Water-use efficiency in a semi-arid woodland with high rainfall variability. Glob. Change Biol. 26, 496–508. doi: 10.1111/gcb.14866
Tenhaken R. (2015). Cell wall remodeling under abiotic stress. Front. Plant Sci. 5. doi: 10.3389/fpls.2014.00771
Tovar-Méndez A., Kumar A., Kondo K., Ashford A., Baek Y. S., Welch L., et al. (2014). Restoring pistil-side self-incompatibility factors recapitulates an interspecific reproductive barrier between tomato species. Plant J. 77, 727–736. doi: 10.1111/tpj.12424
Uwimana B., Smulders M. J. M., Hooftman D. A. P., Hartman Y., van Tienderen P. H., Jansen J., et al. (2012). Hybridization between crops and wild relatives: the contribution of cultivated lettuce to the vigour of crop–wild hybrids under drought, salinity and nutrient deficiency conditions. Theor. Appl. Genet. 125, 1097–1111. doi: 10.1007/s00122-012-1897-4
Van Deynze A., Stoffel K., Buell C. R., Kozik A., Liu J., van der Knaap E., et al. (2007). Diversity in conserved genes in tomato. BMC Genom. 8, 465. doi: 10.1186/1471-2164-8-465
Wang Z., Hong Y., Zhu G., Li Y., Niu Q., Yao J., et al. (2020). Loss of salt tolerance during tomato domestication conferred by variation in a Na+/K+ transporter. EMBO J. 39, e103256. doi: 10.15252/embj.2019103256
Yadav S., Modi P., Dave A., Vijapura A., Patel D., Patel M. (2020). “Effect of abiotic stress on crops,” in Sustainable crop production. Eds. Hasanuzzaman M., Carvalho Minhoto Teixeira Filho M., Fujita M., Assis Rodrigues Nogueira T. (London, UK: IntechOpen). doi: 10.5772/intechopen.88434
Yan J., Zhu C., Liu W., Luo F., Mi J., Ren Y., et al. (2015). High photosynthetic rate and water use efficiency of Miscanthus lutarioriparius characterize an energy crop in the semiarid temperate region. Glob. Change Biol. Bioenergy 7, 207–218. doi: 10.1111/gcbb.12118
Yemm E. W., Willis A. J. (1954). The estimation of carbohydrates in plant extracts by anthrone. Biochem. J. 57, 508–514. doi: 10.1042/bj0570508
Keywords: salinity, tomato, interspecific crosses, reproduction, wild relative
Citation: Bigot S, Leclef C, Rosales C, Martínez J-P, Lutts S and Quinet M (2023) Comparison of the salt resistance of Solanum lycopersicum x Solanum chilense hybrids and their parents. Front. Hortic. 2:1130702. doi: 10.3389/fhort.2023.1130702
Received: 23 December 2022; Accepted: 14 February 2023;
Published: 27 February 2023.
Edited by:
Andrea Mazzucato, University of Tuscia, ItalyReviewed by:
Anna Mensuali, Sant’Anna School of Advanced Studies, ItalyLaxman R H, Indian Institute of Horticultural Research (ICAR), India
Copyright © 2023 Bigot, Leclef, Rosales, Martínez, Lutts and Quinet. This is an open-access article distributed under the terms of the Creative Commons Attribution License (CC BY). The use, distribution or reproduction in other forums is permitted, provided the original author(s) and the copyright owner(s) are credited and that the original publication in this journal is cited, in accordance with accepted academic practice. No use, distribution or reproduction is permitted which does not comply with these terms.
*Correspondence: Muriel Quinet, bXVyaWVsLnF1aW5ldEB1Y2xvdXZhaW4uYmU=