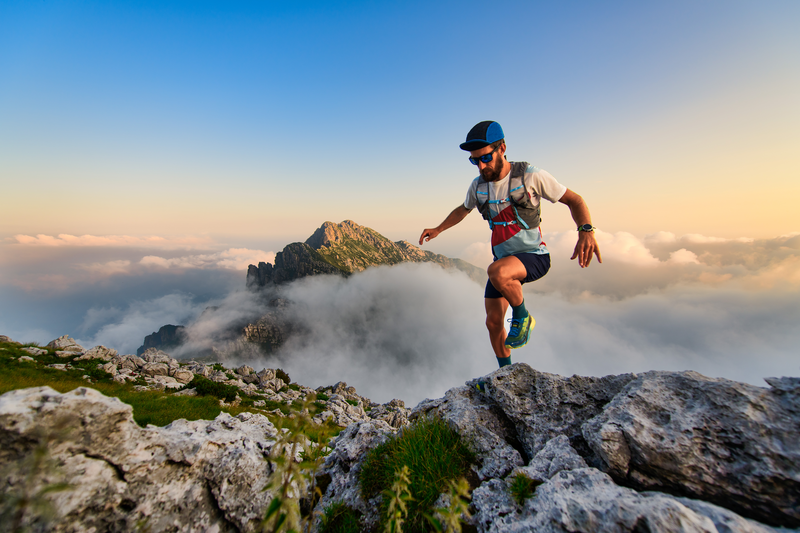
94% of researchers rate our articles as excellent or good
Learn more about the work of our research integrity team to safeguard the quality of each article we publish.
Find out more
ORIGINAL RESEARCH article
Front. Hortic. , 13 March 2023
Sec. Sustainable Pest and Disease Management
Volume 2 - 2023 | https://doi.org/10.3389/fhort.2023.1082204
This article is part of the Research Topic Epidemiology and Management of Pome Fruit Diseases View all 9 articles
A devastating outcome of fire blight in apple trees is the infection of rootstock, which leads to canker development. Fire blight cankers are infected zones of dead bark on perennial branches, trunk, or rootstock that develop after fire blight pathogen Erwinia amylovora invades wood. Cankers can girdle the trunk, branches and rootstock leading to tree death and production losses, especially significant in high-density orchards. An accurate diagnosis of trunk and rootstock blight is a top challenge for apple growers because fire blight cankers can be visually misdiagnosed with cankers caused by less frequent Oomycete and fungal pathogens (e.g., Phytophthora spp., Botryosphaeria dothidea, B. obtusa, Valsa mali). In addition, detecting E. amylovora in apple rootstocks is essential because this pathogen also causes asymptomatic infections. Accurate fire blight diagnosis is necessary to inform the complete removal of infected trees from the orchard and help replanting efforts while preventing further pathogen dissemination. To determine apple tree losses caused by fire blight rootstock infections, PCR was used to detect E. amylovora in symptomatic and asymptomatic rootstocks for two years. Rootstock canker incidence and tree death were rated in selected infection foci on seven commercial apple orchards in New York. Each infection focus consisted of central rootstock-blighted tree and the nearest surrounding edge trees showing no rootstock blight. E. amylovora strains collected from these seven orchards and other orchards in New York were characterized. In the first year, most of the orchards showed E. amylovora detection rates of 10.7 – 45.3% in asymptomatic rootstocks on the edge trees immediately surrounding visibly infected i.e. rootstock-blighted central trees. One year later, 20.8 – 56.3% cankered rootstocks were detected on the edge trees and from zero to 35.4% dead edge trees were recorded. However, the PCR from sampled edge rootstocks one year later showed no pathogen detections. E. amylovora rootstock strains showed slight variability in enzymatic activity, copper sensitivity, virulence, and exopolysaccharide production. These results elucidate the role and importance of rootstock infections for apple tree survival, the presence of latent fire blight infections, and the valuable utility of molecular detection methods to assist apple tree removal after epidemics.
Fire blight, a bacterial disease of pome fruit trees, is becoming more damaging due to warmer and wetter weather patterns in spring (Menzel et al., 2006; Keenan et al., 2014; Ault et al., 2015; Griffiths, 2021). Particularly, the Northeastern United States (U.S.) has experienced an increase in 0.09°C in temperature and 10-mm in precipitation per decade, with a 70% rise in precipitation as heavy rain events (Groisman et al., 2013; Kunkel et al., 2013). In the last 6 years, an alarming trend was recorded in the Northeastern and Mid-Atlantic U.S. of more years with springs characterized by an extremely warm and wet weather that facilitates continuous blocks of 3 – 7 days with high fire blight infection potential according to developed prediction models, often reaching very high values in just a few days, and allowing 3 to 9 fire blight infection events during apple bloom (Philion and Trapman, 2011; Cornell University, 2016; RIMpro-Erwinia, 2016). It is predicted that more fire blight epidemics in the Mid-Atlantic, Northeastern, and Northwestern U.S. are poised to occur with the increasingly wetter and warmer climate (Frumhoff et al., 2007; Hayhoe et al., 2007; CBC News, 2014; CBC News, 2016; Robbins, 2019). For example, it is highly probable that protracting epidemics like the ones in Washington state from 2017 to 2019, could repeat. Fire blight removal in this state during 2017 alone cost pome fruit growers $9 million on 21,450 ha, while 78.5 ha of pear and 121 ha of apple were removed (DuPont 2021, personal communication). Cost of removal by pruning ranged from $66.7 to $2,135 per ha. The fire blight outbreaks continued in 2018 and 2019, with cumulative effect of losses (DuPont 2021, personal communication). In New York state (henceforth “NY”), sudden fire blight epidemics in 2000 and 2008 (Douglas, 2022) led to 50% apple tree losses in young, recently planted orchards (Breth 2008). In 2016, severe regional fire blight epidemic in northern NY caused more than $16 million in damage (Aćimović and Meredith, 2017). High- and medium-density apple orchards have lost 2,500 trees per farm on average, due to fire blight cankers developing on apple tree central leaders and rootstocks (Figure 1). Tree losses continued in 2017 because of latent (asymptomatic) fire blight infections of rootstocks (Aćimović and Meredith, 2017).
Figure 1 Different expressions of fire blight cankers on the main apple tree stem. Cankers on apple tree central leader (A) and trunk (B) both initiated from shoot blight. (C) Infected crown suckers on a rootstock with a canker. Red arrows point to crown suckers and blue arrows point to root suckers. (D) Rootstock girdled with a canker. Fire blight canker on the trunk and rootstock (E) with Erwinia amylovora ooze on the canker margin (F).
The life cycle of fire blight pathogen, Erwinia amylovora (Burrill; Winslow et al., 1920) starts by survival in apple wood bark around fire blight canker edges and below the visible canker necrosis (Santander et al., 2019, Santander et al., 2022b). In spring, E. amylovora emerges on the surface of cankers and can be disseminated by insects, rain, or wind to flowers and shoots. Under favorable warm and wet weather conditions during bloom: (I) ≥18.3°C average daily temperature; (II) 198 degree hours >18.3°C accumulated from first open flowers; and (III) ≥ 0.25 mm rain or heavy wetting dew or ≥0.25 mm rain the previous day, which facilitate E. amylovora population growth on flower stigma and washing of these colonies to nectar glands, flower and shoot infections establish and lead to blossom and shoot blight (Turechek and Biggs, 2015). New infections of succulent tissue allow the bacterium to move via xylem and parenchyma and invade wood tissues to develop fire blight cankers. E. amylovora residual cells can asymptomatically migrate far beyond the visible symptoms in the tree canopy (Steiner, 2000) and reach the tree rootstock (Momol et al., 1998; Vanneste and Eden-Green, 2000). This systemic cell transfer occurs via xylem of the central leader and trunk to the graft union (Momol et al., 1998; Norelli et al., 2003a). An alternative pathway for the pathogen to invade the rootstock are infections of rootstock suckers growing around the base of the tree. Crown suckers emerge in the area immediately surrounding the rootstock trunk base (Figure 1C, red arrows), while the root suckers develop from roots further away from the trunk (Figure 1C, blue arrow) (Utah State University - Extension, 2020; Utah State University, 2022).
Depending on the level of the rootstock’s genetic fire blight susceptibility, infections with E. amylovora can express as development of cankers on the bark, often referred to as rootstock blight, which may girdle the rootstock stem or trunk base and kill the tree (Figure 2) or remain asymptomatic as latent infections (Norelli et al., 2001; Aldwinckle et al., 2004; Johnson and Temple, 2016). There are differences in rootstock susceptibility to fire blight. Very susceptible to fire blight are Malling series M.26 and M.9 and its subclones (Nic29, T337, Pajam 2), tolerant or moderately resistant to fire blight are M.7, and Budagovskij series B.9 and B.118, and fire blight resistant are Geneva series G.11, G.41, G.202, G.214, G. 890, G.935, G.969 and similar (Wertheim, 1998; Norelli et al., 2001; Norelli et al., 2003b; Aldwinckle et al., 2004; WSU, 2022).
Figure 2 (A) Fire blight canker on apple rootstock with an exposed canker margin. (B) Dead apple tree from rootstock girdling by a fire blight canker (Photo by Wallis A. E. 2016, Cornell Cooperative Extension).
In high density apple orchards, which hold between 1,200 – 5,000 trees/ha, fire blight cankers have much higher chances to quickly develop on the tree stem and rootstock due to smaller tree sizes, narrow i.e., columnar tree training systems, and short bearing limbs. These traits allow E. amylovora to quickly migrate from infected flowers and shoots to the tree stem (Aćimović et al., 2021) and cause high incidence of cankers on the central leader and rootstock. Rootstock cankers are often overlooked by growers as they are close to or below the soil line and difficult to discern from healthy bark, sometimes due to soil particles splash- or dust-covering the rootstock. Additionally, asymptomatic rootstock infections by E. amylovora are difficult to detect using classic or molecular methods as there are no clues where the samples for analysis should be collected. They often lead to surprise tree collapse and death in the current and the year following an outbreak, especially after successful aerial symptom removal by summer and winter pruning. Even if cankers are visible on the rootstock, they are particularly hard to associate by growers with the previous blight removal from the tree canopy because they often express with time delay in relation to blossom and shoot blight. This time delay leads growers to think the cause is different because they believe that fire blight was successfully and completely removed by pruning. Despite the many aspects of rootstock blight being unclear, there are limited studies recording the extent of rootstock blight damage and detecting and managing the asymptomatic infections in high density orchards (Momol et al., 1998; Norelli et al., 2001; Aldwinckle et al., 2004; Johnson and Temple, 2016).
Simultaneously with fire blight damage rising, high-density farms have caught the national attention with the syndrome of bearing apple trees abruptly dying during the summer, which was named Rapid Apple Decline (RAD) or Sudden Apple Death (SAD) (Stokstad, 2019). Necrosis and wood decay expanding from bark or vascular cambium into the heartwood were observed mostly on the rootstock wood, below the graft union of RAD/SAD affected apple trees (Singh et al., 2019). This work indicated that rootstock is the starting point of this syndrome. Diagnosing the cause(s) of rapid apple decline, that is markedly different in expression from fire blight, quickly became important and studies are underway to determine potential causes of this syndrome (Liu et al., 2018; Singh et al., 2019; Gruber, 2022). Tree decline has led the tree fruit experts to analyze weather data and postulate that extremely cold winter followed by drought in 2015 – 2016 contributed to weakening of the trees and making them more susceptible to a variety of stresses (Singh et al., 2019). However, by far, fire blight has historically been the top cause of apple tree death in comparison to other causes and was ranked as the number one IPM Research and Extension Priority for 7 consecutive years (2014-2020) by the Tree Fruit IPM Working Group at the Northeastern IPM Center (Tree Fruit IPM Working Group, 2020).
Diagnosing that trunk and rootstock cankers are caused by E. amylovora is a top challenge for apple growers because visually they can be misdiagnosed with cankers caused by the less frequent Oomycete and fungal pathogens like Phytophthora spp., Botryosphaeria dothidea, B. obtusa, Sclerotium delphinii, and Valsa mali which cause Phytophthora crown, collar and root rot; white rot of fruit and wood canker; black rot of fruit and apple wood canker; Southern blight of apple; and Valsa canker, respectively (Aycock 1966; Sutton et al., 2014; Peter, 2020; Yeonghyeon et al., 2022). What is more alarming is that most growers do not see the economic impact of fire blight cankers until apple trees start to decline and die because they are obscured by tree canopy, are large in numbers, and hard to see. It is impossible to detect all cankers on a single tree by a human eye (Aćimović et al., 2014). Due to a large number of tall, narrow trees per acre, more fire blight cankers are likely to be overlooked in high-density orchards and carryover into the next growing season perpetuating fire blight infections (Aćimović et al., 2014; Aćimović et al., 2021). The result is a recurring problem for growers to reliably determine which apple trees are infected and must be removed, signifying the practical importance of detecting E. amylovora as the cause of rootstock cankers.
With the rising impact of trunk and rootstock cankers causing tree top and whole tree death in high-density orchards, a need for rapid but cheap molecular detection for E. amylovora, bypassing pathogen culturing, increased so that the positive diagnostic result can inform tree removal and help orchard insurance claims (Courtney, 2020; USDA, 2020a; USDA, 2020b). In this work, the first goal was to use PCR test to detect E. amylovora in apple rootstocks on commercial farms affected by fire blight and deliver the results to growers to timely remove whole trees with both latent infections and visible canker(s). The second goal was to determine if E. amylovora can be detected in the trees with healthy looking, canker-free rootstocks, that are closest to the central tree with visible rootstock canker(s), thus revealing asymptomatic infections. The central trees with rootstock canker(s) were considered in need of being removed, while the trees surrounding the central tree were considered as the edge trees with potential latent rootstock infections. Both tree types comprised one infection focus. Besides canker(s) on the rootstock, the central trees often had fire blight strikes in the crown and sometimes were completely blighted or dead. The results of PCR detection on the edge trees would reveal the probability for latent E. amylovora infections and their spatial distribution. The above-described infection foci were purposely chosen because they are positioned on the rim of large groups of severely infected or dead trees and are the most difficult to decide for removal by growers. Only the dead or the trees with severely cankered rootstock, trunk, and/or central leader in these large groups are removed in fire blight eradication or replanting efforts. Therefore, knowledge of E. amylovora asymptomatic infections in the foci edge trees would serve as valuable information to growers by showing the need to expand tree removal extent beyond dead and the trees with cankered rootstocks. This would improve the efficacy of efforts to eradicate E. amylovora from the orchards timely and thoroughly.
In 2016, NEWA’s online Cougarblight model and the PC model MaryBlyt 7.1 for Windows predicted extremely conducive weather for fire blight infections for the last 10 days of May affecting northern NY. Depending on cultivar, apple bloom started from 7 – 10 May. While trees were at the end of bloom and beginning of petal fall, according to Maryblyt disease model (Turechek and Biggs, 2015) severe fire blight infections occurred on flowers and intensively growing shoots and were triggered by light rain and hail events on 22, 29 and 30 May (Supplementary Figure 1). In Cougarblight model (Smith, 2000; Smith and Pusey, 2011), transition from Low to Caution and then to High infection risk occurred in only three days, reaching Extreme risk from infection on 23 May and continuing until the infection events on 29 and 30 May (Supplementary Figures 2 and 3). Several apple cultivars were at the end of bloom allowing enough open flowers to sustain and grow E. amylovora populations on stigma and allow inoculum to disseminate to the shoots. The key weather conditions facilitating E. amylovora populations growth on flowers were sudden rise of average daily temperatures to 19.6°C on 22 May and 22.7°C and 24.1°C on 29 and 30 May, respectively. At the same time relative air humidity ranged from 96 to 98% on 29 and 30 May, allowing prolonged flower, fruitlet, and leaf wetness from dew, which were followed by a few hailstorms in June. First blossom blight and shoot blight were visible on June 6 indicating that the infections initiated on the 22 May were simultaneous infections of any remaining flowers and of intensively growing shoots where the latter were approximately 1.5 months away from terminal bud set when they become ontogenically more resistant to fire blight. In the affected orchards, preventive applications of streptomycin on 21 or 22 May and on 28, 29 or 30 May were not applied or were applied just after the first blossom blight symptoms were visible. Invasion of wood by E. amylovora from infected flowers and shoots led to development of fire blight cankers on bearing branches, central leaders, trunks, and rootstock via trunk xylem or suckers (Figure 1). The blossom and shoot blight incidence ranged from 30 to 100% depending on the location and site, with 40 to 90% crop loss. Many orchards had 35 to 65% severely infected and dead trees (Figure 2) and were completely removed and replanted.
After the 2016 fire blight epidemic, seven commercial farm sites were selected on three locations in Northeastern NY and a natural experiment lasting for two years was established. On each farm the most affected orchards were selected (Table 1) and approximately 50 apple tree rootstocks per farm were sampled to detect E. amylovora by PCR. The total number of rootstocks sampled and analyzed with PCR was 361 on 1 November 2016 and 361 on 16 November 2017. Rootstock sampling was carefully conducted in the pattern of an infection foci (Figure 3), which were purposedly selected so that each focus consisted of one central tree with a visible canker(s) on the rootstock and the nearest apple trees with no visible cankers on the rootstock (4 – 8 trees per focus, depending on whether the focus was inside or at the edge of an orchard, as per Figure 3). The trees around the central cankered tree were defined as edge trees. Except for farm G (Table 1), which had only 3 selected infection foci (28 central and edge trees), between 6 – 8 infection foci per farm were selected (Table 1).
Table 1 Commercial orchards sampled for PCR detection of asymptomatic rootstock infections by Erwinia amylovora from 2016 until 2018, and whose rootstocks were also rated in 2018 and 2018 for canker presence and tree death.
Figure 3 Infection foci sampling patterns for (A) complete fire blight infection focus, inside the orchard plots, consisting of one central and eight nearest surrounding trees (maximum number of trees per focus), and (B, C) for partial fire blight infection foci on the orchard side(s) or corners, consisting of one central and five or four nearest surrounding trees (C shows the minimum number of trees per focus). All trees in the infection focus were sampled on rootstock and analyzed for E. amylovora detection with PCR. Around 50 rootstock samples per each of seven farm sites were collected, with 3 – 6 foci per site selected and sampled. The central trees in the infection foci (orange background) had visible fire blight canker(s) on the rootstock, often with fire blight strikes in the crown, and sometimes completely blighted or dead. The central trees would be assumed as in need to be removed by farm staff. The edge trees surrounding the central tree (green background) had no visible canker(s) on the rootstock at the time of first sampling in November 2016.
Rootstock bark and xylem sampling was performed using a surface sterilized 2.5-cm-wide wood chisel. Using a hammer, the chisel was inserted perpendicularly into the rootstock stem, pressing it through the bark and into the xylem, down to a 5-6 mm depth, to cut out four square-shaped tissue pieces on four cardinally oriented sides around the rootstock radius (N, S, E, W). Each piece was 2.5 × 2.5 cm in size and separated from the stem using the chisel. The four carved-out bark samples from one tree were placed in a plastic resealable 6 × 8-inch Ziplock bag, thus making a one-tree composite sample, labelled, and stored in a portable rotomolded camping cooler with frozen icepacks, securing sample transportation at 4°C. Samples were stored in a refrigerator at 4°C until homogenization described below, DNA extraction and PCR.
By following the same apple trees from November 2016 sampling, in October 2017 it was found hat none of the PCR positive and dead trees from November 2016 were removed by farm staff allowing sampling of the same tree rootstocks again in November 2017, to repeat PCR detection for E. amylovora presence in rootstocks. The tissue collection was performed using the same tools and procedure as described above with the only modification of offsetting the four collected sampling pieces of rootstock to the right side of the previous sampling points (NE, SE, NW, SW).
Rootstock bark samples were processed similarly to Santander et al. (2019) and Santander et al. (2022a), with some modifications. Briefly, samples were weighed using a scale and placed in a resealable plastic bag containing 50 mL of ice-cold antioxidant maceration buffer (AMB) per gram of sample (EPPO, 2022). Plant tissues from one tree were then homogenized by smashing them with a hammer over the bag, on a horizontal surface. The macerate bags were incubated on ice for 10-15 min and two 0.8-mL aliquots of the bark tissue homogenates were transferred to two microcentrifuge tubes, one of them containing 0.8 mL of 40% (w/v) sterile glycerol to cryopreserve samples at -80°C until use. The other aliquot was centrifuged at 15,000 g for 10 min, the supernatant discarded, and the pellets stored at -20°C until use. For the molecular analysis of samples, the tubes without glycerol stored at -20°C were thawed at room temperature, centrifuged at 15,000 g for 10 min, the supernatant discarded, and the pellet’s DNA extracted with the DNeasy Plant Mini Kit (Qiagen, Frederick, MD) following the manufacturer’s instructions.
E. amylovora detection was carried out by PCR in 25 μL reactions, using 5 μL sample DNA and a master mix containing 1X DreamTaq Green Polymerase Buffer (Thermo Fisher Scientific, Waltham, MA), 0.2 mM dNTPs, 0.4 mM of G1-F and G2-R primers (Taylor et al., 2001; EPPO, 2022) and 1U of DreamTaq Green Polymerase (Thermo Fisher Scientific, Waltham, MA). The employed thermal cycling were the ones recommended by the (EPPO, 2013; EPPO, 2022). The presence of the 187 bp amplicon was determined electrophoretically by running 10 μL of sample in 1.5% agarose gels stained with SYBR Safe (Thermo Fisher Scientific, Carlsbad, CA). Finally, to confirm the presence of the pathogen, a random selection of cryopreserved samples with glycerol that were positive by PCR were plated on Sucrose Nutrient Agar (SNA) (Billing et al., 1961) and CCT media (Ishimaru and Klos, 1984) after serial dilutions in PBS. After 40-72 h of incubation at 28°C, E. amylovora-like colonies were identified by colony PCR, using the primers and thermal conditions described above.
After the analysis, edge tree rootstocks on each orchard site were grouped in percentages of PCR detection positive for E. amylovora presence (latent or asymptomatic infections confirmed) or PCR detection negative for E. amylovora absence (latent or asymptomatic infection absent).
Rootstock cankers on the edge trees with asymptomatic E. amylovora infections detected in November 2016 took time to develop. To determine canker development and their impact on the tree status, roughly one year after the first PCR detection, i.e., on 22 October 2017, the edge tree rootstocks were rated for canker incidence and the overall tree health status (tree alive or dead). After one more year, a repeated rating of both parameters was conducted on 22 September 2018. Both parameters were expressed as percent of edge trees with or without rootstocks canker(s), and the percent of dead or alive edge trees.
Finally, tree removal status in all infection foci was followed in October 2017 and September 2018 and expressed as number and percent of PCR positive rootstock, non removed trees, PCR positive rootstock, removed trees, and dead trees. In September 2018, we recorded the number and percent of removed dead trees and and remaining dead trees in each orchard.
The biochemical profile of a selection of E. amylovora isolates from rootstocks was characterized using API 20E strips (Biomérieux, France). The presence of plasmid pEA29 was assessed by PCR, according to Bereswill et al. (1992). The susceptibility of the isolates to streptomycin was tested on LB amended with the antibiotic at 25-100 μg/mL, using as control the streptomycin-resistant strain Ea88-100 (Loper et al., 1991). The production of amylovoran and levan by the E. amylovora isolates was tested by the cetylpyrimidinium chloride (CPC) (Bellemann et al., 1994) and the levansucrase buffer methods (Bereswill and Geider, 1997), respectively, normalizing data to the A600 nm of the analyzed cultures, as reported previously (Santander et al., 2014).
The pathogenicity of the isolates was confirmed using pear ‘Conference’ slices, as described elsewhere (EPPO, 2022). Differences in virulence among the isolates were assessed using ‘Bartlett’ pear leaves. Briefly, the third, fourth and fifth youngest leaves of new shoots of pear trees were collected, rinsed with tap water and surface disinfected with cotton ball soaked in 70% ethanol. Afterwards, the leaves were cut transversally, 2.5 cm below the tip, and immersed in an E. amylovora suspension at 108 CFU/mL in PBS for 30 seconds. Afterwards, the inoculated leaves were incubated individually in 90-mm diameter Petri dishes containing a sterile filter paper of the same size as the plate, soaked in sterile distilled water. Plates sealed with parafilm were incubated at 28°C and differences in virulence were assessed using size of the necrosed areas, which were measured by image analysis (Santander et al., 2018).
Comparisons of the average EPS production and virulence values between E. amylovora isolates from rootstock strains, other NY state isolates, and the American and European reference strains ATCC 49946 (Norelli et al., 1987) and CFBP 1430 (Paulin and Samson, 1973), respectively, were performed by one-way ANOVA or Kruskal-Wallis tests, depending on the normality of the residuals, assessed by D’Agostino, Anderson-Darling, Shapiro-Wilk and Kolmogorov-Smirnov tests, using GraphPad Prism 9 for macOS.
Some E. amylovora isolates showed partial or total growth inhibition on a differential medium for E. amylovora containing copper sulfate (Ordax et al., 2012). This medium is a modified King’s B (KB) medium (King et al., 1954) amended with 1.5 mM CuSO4. To test the effect of copper on E. amylovora isolates growth, differences in the number of colonies on KB and KB plus 1.5-5 mM CuSO4 were calculated. Briefly, E. amylovora suspensions were prepared at 107 CFU/mL in PBS, and serial tenfold dilutions spread plated in parallel on KB, KB + 1.5 mM CuSO4, KB + 2.5 mM CuSO4, KB + 3.5 mM CuSO4 and KB + 5 mM CuSO4. Plates were incubated at 28°C for 40-72 h and the colonies in the dilutions containing between 30 and 300 colonies counted. The percentage of growth enhancement or inhibition by copper was calculated as:
Where KB are the colonies counted on KB medium without copper, and KBCu is the number of colonies counted on KB amended with CuSO4 at the assayed concentration, taking into consideration the dilution factors.
Two groups of data are presented for the same monitored infection foci. The first group of data in the subtitle 3.1 below and Figure 4 shows the pathogen detection and disease rating parameters that were monitored only on the edge trees in the infection foci: PCR detection of E. amylovora on rootstock (positive; negative), rootstock canker incidence, healthy rootstock percent, dead and alive tree percentages. The second group of data in the subtitle 3.2 below and Figure 5 presents the action by farm staff in terms of the whole tree removal based on the PCR detection data in rootstocks from Figure 4A and encompasses all the trees in the infection foci, both central and the edge trees.
Figure 4 Two-year results of (A) PCR detection of Erwinia amylovora in apple rootstocks [+, detection positive, E. amylovora present, and −, detection negative i.e., E. amylovora not present], and of (B) two ratings of rootstock canker incidence (purple) and tree death on edge trees in infection foci on seven commercial farm sites across two years (blue). Trees were infected with fire blight after natural infections (Supplement Figures 1, 2 and 3). Colour bars indicate highest to lowest number of all sampled trees/pale blown bars/and of edge trees only/orange bars/(N), or percent edge tree: rootstock canker incidence/purple bars/, healthy rootstock/green bars/, dead trees/blue bars/and alive trees/green bars/, relative to the number of edge trees (N).
Figure 5 Two-year results of (A) tree removal status in infection foci on October 2017, roughly one year after rootstock sampling and PCR detection of E. amylovora in November 2016, and (B) tree removal status in infection foci on September 2018, roughly one year after rootstock sampling and PCR detection of E. amylovora in November 2017. Note: PCR positive detections of E. amylovora in rootstocks in Figure 4A (red bars) consist of different numbers of PCR+ non-removed and dead trees in Figure 5A. Bold and underlined denote farms with almost all or all dead trees removed. ‘New’ denotes additional tree deaths in comparison to October 2017. Colour bars indicate highest to lowest number of all sampled trees (pale blown bars).
In November 2016, E. amylovora was detected in 10.7 – 45.3% of the sampled edge tree rootstocks with no fire blight cankers (Figure 4A), thus exposing high levels of asymptomatic or latent infections. The average detection rate was 27.4% on all orchard sites. In October 2017, roughly a year after, 20.8 – 56.3% of the edge rootstocks developed cankers (Figure 4B), with an all-orchard sites average canker incidence of 34.7%. At the same time from zero to 35.4% of the edge trees died, with an all-orchard sites average of 13.5% (Figure 4B).
Unexpectedly, in November 2017 E. amylovora was not detected in any of the same edge tree rootstocks sampled in November 2016 (Figure 4A). In September 2018, roughly a one year after the second PCR testing, the percent of cankers on the edge rootstocks developed between November 2016 and October 2017 has remained the same (Figure 4B). The percent of the dead edge trees in September 2018 remained the same as in October 2017 (Figure 4B), except for the orchard site E which had an increase of dead trees from 25.4 to 27% (Figure 4B, bold font).
The rating of canker incidence on the edge tree rootstocks in October 2017 showed roughly a similar pattern to the PCR positive detections from November 2016 (Figures 4A, B). The dominant trend was a higher rootstock canker incidence (Figure 4B) in comparison to the PCR positive E. amylovora detections (Figure 4A). Namely, on five out of seven orchard sites, i.e., A, C, D, F and G, there was a 28.5%, 13.9%, 13.7%, 6.5%, and 10.1% higher incidence of cankers, respectively, in comparison to E. amylovora positive PCR detections from November 2016 (Figures 4A, B). Only two sites, i.e., B and E, had a 19% and 2.7% lower percent of cankers in comparison to the percent of positive pathogen detections, respectively (Figures 4A, B). However, the canker incidence rating in October 2017 diverged in pattern from the result of no positive PCR detections of E. amylovora in November 2017.
In October 2017, one year after the first PCR detection, it was determined that the majority of the PCR positive trees and dead or cankered central trees from November 2016 were still not removed by the farm staff (Figure 5A). Therefore, on all the orchard sites 7.1 – 47.2% of the trees could have served as reservoirs of E. amylovora and thus inoculum sources for infections in spring and summer 2018.
On 22 September 2018, farm staff acted upon the PCR detection information delivered to them from the current work. They removed all PCR positive trees (Figure 5B) in comparison to 22 October 2017 (Figure 5A), with only four farms (orchards B, C, F, G) removing almost all dead trees (bold and underlined font in Figure 5B). The farms with orchards A, D and E still had 6, 4 and 10 old dead trees to remove, respectively (Figure 5A, B), in addition to some new dead trees (Figure 5B). This highlights a requirement for multiple rounds of tree removal due to slow canker development and variable time of tree death onset. On some farms (orchards B, C, D, E) several new tree deaths were detected, with likely more latent rootstock infections expressing (Figure 5B).
Five E. amylovora isolates from rootstocks on the 7 monitored farms were analyzed and their phenotypes compared with 9 isolates from other orchards in NY state, originating from other plant organs than rootstocks, as well as with the reference strains ATCC 49946, CFBP 1430, and Ea88-100. The biochemical profiles obtained with API 20E strips coincided with the typical profiles of E. amylovora and did not differ significantly from the profiles obtained with other E. amylovora isolates from NY state and reference strains included in the analysis (Table 2). All the E. amylovora isolates fermented glucose by the butylene glycol pathway (Voges-Proskauer test), and acidified the medium during the fermentation of glucose, mannose, sorbitol, and sucrose. Only one of the rootstock isolates and none of the other isolates from NY orchards showed arginine dihydrolase activity. None of the tested E. amylovora rootstock isolates were able to hydrolyze gelatin, and only 3 out of 9 of the NY State isolates showed this activity. None of the rootstock isolates produced acid as a subproduct of inositol fermentation, and only one of the other NY state isolates showed this phenotype. Acid production from arabinose fermentation was observed in 3 out of 5 rootstock isolates and in 2 out of 9 NY state isolates.
Table 2 Phenotypical characterization of E. amylovora rootstock isolates and comparison to other New York state isolates and reference strains.
All the analyzed strains contained the pEA29 plasmid, were pathogenic on pear slices and were susceptible to all the assayed streptomycin concentrations (Table 2).
The analyzed E. amylovora isolates, both from rootstocks and other NY state isolates, also showed variability in levan and amylovoran production, although no significant differences were observed between rootstock and other NY state isolates of the pathogen, or with the reference strains (Kruskal Wallis test, P > 0.05) (Figures 6A, B). The virulence of E. amylovora rootstock isolates on pear leaves (Figures 6C, D) was comparable to that of other NY state isolates (Figure 6C). All assayed E. amylovora isolates were more virulent than the reference strains ATCC 49946 and CFBP 1430 (One-Way ANOVA, P = 0.0014).
Figure 6 Phenotypic characterization of E. amylovora rootstock isolates and comparison with reference strains and other New York isolates. (A) Amylovoran production; (B) levan production; (C) virulence on pear ‘Bartlett’ leaves and (D) example of different degrees of necrosis in pear leaves; (E) effect of copper sulfate on E. amylovora’s growth on King’s B agar. Boxes and whiskers represent interquartile ranges of data, maximum and minimum values and the median. For the reference strains, represented data show values obtained in 3 experimental repeats. The data grouped as “Rootstock isolates” and “Other NY State isolates” contain average values of an experiment performed in triplicate, for a total of 5 and 9 representative isolates, respectively. Different letters indicate statistically significant differences between the compared groups (P < 0.05); ns indicate non-significant differences between the compared groups.
Regarding the effects of copper on E. amylovora’s growth (Figure 6E), the American and European E. amylovora reference strains ATCC 49946 and CFBP 1430 showed an improvement of culturability of around 33% and 17%, respectively, when exposed to 1.5 mM CuSO4. The exposure to 2.5 mM CuSO4 only had a slight positive effect (ca. 3% growth enhancement) in the European strain CFBP 1430. Higher copper concentrations partially or totally impaired the growth on KB agar of both reference strains. The effects of the different tested copper concentrations on the remaining E. amylovora isolates from rootstocks and other sources were similar to those described for the reference strains, although copper-induced growth was evident only in 36% and 55% of the isolates at 1.5 mM and 2.5 mM, respectively. At 3.5 mM CuSO4, 93% of the isolates grew less in the presence of copper. This means that most of the tested isolates experienced more difficulties to grow in the presence of copper, regardless of the used concentration. Growth inhibition was more evident at the highest copper concentrations, with 3.5 mM CuSO4 reducing colony formation between a 14.7% and a 100%; and 5 mM CuSO4 reducing growth around 100% in all the assayed strains (Figure 6E). Two strains, one isolated from rootstocks and one isolated from other plant organs in orchards in NY state, showed high susceptibility to copper, even at the concentration used in differential media.
Apple rootstock infections and cankers from fire blight are economically important but poorly investigated part of E. amylovora life cycle. After natural epidemic in 2016, multiple infection foci in seven orchards planted on either M.9 or M.26 rootstock were selected. Each focus consisted of one central tree with visible rootstock canker(s) and the closest surrounding trees to it with visually healthy-looking rootstocks, or the edge trees. Such foci are usually positioned at the rim(s) of the large groups of trees dead from fire blight and present a problem for removal of infected trees due to latent E. amylovora infections in their rootstock and subsequent delayed tree death. Around 30% of latent E. amylovora infections were detected in the edge tree rootstocks in the fall of the epidemic year. One year later, fire blight cankers on the edge tree rootstocks (35%) expressed with delay, during the growing season following an epidemic. These rootstock cankers led to death of up to 35% of the edge trees. This and a few new tree deaths at the end of the experiment indicated that the zone of tree removal should be expanded beyond the borders of already dead groups of trees first killed in the epidemic, and should be conducted in multiple cycles. Such zone expansion, which should include the edge trees around the rootstock cankered trees, would ensure removal of the most or all the infected apple trees in less returns to the orchard.
As reported by Russo et al. (2007), in the last 10 years planting of high-density apple orchards in the U.S. still continues to strongly depend on the widely available M.9 rootstock. M.26 rootstock was also used in high-density systems but is mostly reserved for less vigorous cultivars. In stool beds, M.9 and M.26 rootstock “mother plants” are much more productive in growing rootstock liners, i.e., the individual shoots that will become rootstock plants, in comparison to the less liner-productive Geneva series rootstocks. Thus, for faster profitability, nurseries can grow larger quantities of M.9 and M.26 rootstocks in a short time and use them for grafting scions i.e. producing apple saplings. However, M.9 and M.26 are extremely susceptible to fire blight and in years with severe natural fire blight infection pressure, apple tree mortality greater than 50% and 60% is often recorded when orchards are planted on M.9 and M.26 rootstocks, respectively (Ferree et al., 2002; Norelli et al., 2003a; Robinson et al., 2007). In contrast, the resistant Geneva series rootstocks, G.16 and G.30, were found to have 70% less rootstock blight-related tree mortality in comparison to M.26 and M.9 in both natural and inoculated field trials (Norelli et al., 2003a). The fire blight susceptibility of M.9 and M.26 rootstocks reduces apple orchard profitability and disrupts production continuity in high-density plantings where initial costs for establishment are substantial. Higher capacity of production of fire blight-resistant rootstocks and thus their wider use are both necessary to reduce grower reliance on M.9 and M.26.
E. amylovora was not detected with PCR in the edge tree rootstocks in fall 2017. The positive PCR detection of E. amylovora in asymptomatic rootstocks in fall 2016 was significantly offset in time from the detection of cankers on rootstocks and tree mortality in fall 2017. In fall 2017, the concentration of E. amylovora in rootstocks might have declined below the limit of detection of PCR (EPPO, 2013; Ham et al., 2022). The 10.1% – 28.5% higher rootstock canker incidence in fall 2017, in comparison to the precent of E. amylovora detections with PCR in fall 2016, aligns well with the faster death of susceptible host wood from cankers and lower linked incidence of E. amylovora-positive cankers on their branches (Santander et al., 2022b). Viability digital PCR in this work showed that more E. amylovora cells die in cankers on highly susceptible pome fruit tree hosts due to large extent of damage on branches and faster tissue death, thus causing lower detection rates of E. amylovora-positive cankers. Santander et al. (2022b) also demonstrated that in fall, E. amylovora canker populations on highly susceptible Asian pear cultivar ‘Shinko’ declined to 105 – 106 CFU/g of canker in fall and down to non-detectable levels in winter. Thus, the high susceptibility of wood tissues to fire blight could lead to faster wood death and decline in E. amylovora concentrations in cankers to the levels below the detection limit of PCR. Fire blight rootstocks M.26 and M.9 in the current study, are rated as very susceptible to fire blight (Wertheim, 1998; Norelli et al., 2001; Aldwinckle et al., 2004; WSU, 2022). Their woody tissues probably responded in a similar way as highly susceptible Asian pear wood tissues (Santander et al., 2022b). High susceptibility of rootstock tissues and high canker incidence detected in the current study could explain how E. amylovora concentrations in rootstock samples collected in the current study declined in fall 2017 below the limit of detection of PCR used (Taylor et al., 2001). PCR can have a detection limit of E. amylovora in plant DNA from apple shoot extracts of 105 – 106 CFU/ml with A/B primers (Bereswill et al., 1992) or 3.7 × 105 CFU/ml with RS24580-205 primers (Ham et al., 2022). The G1/G2 primers used in the current study (Taylor et al., 2001) and FER1-F/rgER2R primers (Obradović et al., 2007) have a detection limit of E. amylovora in plant DNA of 103 – 105 CFU/ml. The PEANT1/2 primers (Stöger et al., 2006) have a detection limit of 104 – 106 CFU/ml (Ham et al., 2022). However, in contrast to shoot extracts, suberized and lignified tissues of bark and xylem from apple rootstocks were used in the current study. Such tissues could significantly impact E. amylovora DNA extraction efficiency and raise the limit of detection of PCR, i.e. lower its sensitivity (Santander et al., 2019; Santander et al., 2022a). Thus, high fire blight susceptibility of M.9 and M.26 rootstocks and sampled lignified tissues could have led to very low E. amylovora concentrations in sampled rootstocks in fall 2017 and no pathogen detection with PCR used in this study.
One year after the 2016 epidemic, in fall 2017, 7.1 – 47.2% of the PCR positive rootstock trees in fall 2016 and dead or cankered central trees recorded in fall 2017, were not removed from the orchards. This demonstrated that on average 22% of rootstock infected trees remained in the orchards, developed cankers, and continued to decline in the year after epidemic. This made it difficult for growers to associate this tree collapse with continued fire blight activity because of the long time for symptom expression on rootstock after the trees were freed of crown symptoms by pruning. The infection foci selected were hypothesized as the most difficult cases to deal with in epidemiological and tree removal respects, because they were largely positioned at the rim(s) of the large groups of fire blight dead trees, i.e., just outside the border of these groups. The focus of the current study was on these foci to determine whether the extent of tree removal needs to be larger in surface area, i.e., expanded beyond the edge of the large groups of dead trees first killed in the epidemic. With 27% latent infections detected in fall 2016, 35% cankered rootstocks and 13.5% dead trees in fall 2017, along with new tree deaths in fall 2018, the area of tree removal should be expanded to just outside of the border of large groups of dead trees. This expanded zone should include the edge trees around the rootstock cankered trees in the infection foci that were examined in the current study. Such practice could prevent continuing tree decline and reduce the need for multiple cycles of tree removal.
The biochemical profiles of the E. amylovora isolates from rootstocks and other plant organs from apple tree orchards in NY state coincided with those described for E. amylovora (EPPO, 2022). The API 20E results were, in general, homogeneous, with slight variability in the metabolism of inositol, gelatin hydrolysis and arginine dihydrolase. These results are similar to those described by other authors (Donat et al., 2007; Végh et al., 2017). The isolates analyzed in the current work also showed diverse EPS production and virulence. Despite E. amylovora’s genome homogeneity (Mann et al., 2013), variability in the virulence and EPS production among E. amylovora strains is usually reported (Puławska and Sobiczewski, 2012; Popović et al., 2020; Mendes et al., 2022). A link between EPS and virulence has also been established (Maes et al., 2001). The most of the phenotypic diversity observed among strains is probably due to different plasmid and genomic island content (Mann et al., 2013).
Although copper has been used as a selective and differential compound for the isolation of E. amylovora on different media (Bereswill et al., 1998; Ordax et al., 2012), and reported as beneficial for the recovery of stressed cells when amended to King’s B medium (Ordax et al., 2012), less than half of the strains analyzed showed improved growth in the presence of copper. The different results in the current study compared to those of Ordax et al. (2012) might be due to the use of a reduced number of strains to characterize the medium, including the European type strain CFBP 1430, which was one of the strains showing growth enhancement in the presence of copper, in the assays of the current study. Additionally, in the current study, two of the analyzed strains showed high susceptibility to copper and were unable to grow on KB amended with the recommended copper concentration. Hence, although the use of copper-amended media may still be useful for the easy differentiation of E. amylovora colonies during isolations, the use of other selective/differential media in parallel is recommended to improve the success of isolation.
This study is the first work that demonstrates high presence (up to 45%) of E. amylovora latent infections in the edge tree rootstocks during the fall season of the epidemic year. The latent infections expressed as rootstock cankers in the following growing season, i.e. with delay, and the incidence of cankers on the edge tree rootstocks (up to 56%) was higher than the percent of latent infections detected by PCR in an epidemic year. As these cankers expressed with significant delay, PCR detections were negative indicating on the likely decline of E. amylovora concentrations in rootstocks to the levels below the PCR detection limit. Up to 35% of the rootstock-cankered edge trees died with a few new tree deaths two years after the first PCR detection. The data of the current study indicates that tree removal due to latent E. amylovora infections of rootstock should be expanded beyond the borders of the dead trees killed early in the epidemic. In conclusion, the recommended removal of trees with latent E. amylovora infections based on the current study would ensure a more complete elimination of E. amylovora-infected apple trees in the orchards and help improve disease management and replanting efforts.
The raw data supporting the conclusions of this article will be made available by the authors, without undue reservation.
SGA originated the project ideas and questions in consultation with apple growers, designed the field experiments, wrote project proposals, and secured funding, provided the necessary laboratory equipment and materials, supervised, and coordinated the work, mentored ŽMP, RDS and CLM, created rootstock graphs and tables, and drafted, reviewed, and edited the manuscript. RDS optimized processing of rootstock samples for PCR, optimized PCR extraction conditions, co-mentored ŽMP, conducted all the assays for characterization of E. amylovora strains, and co-drafted, reviewed, and edited the manuscript. CLM performed infection foci selection, field tree and rootstock labelling, and rootstock sampling, rated canker symptoms and tree health status, recorded data in paper and electronic files, and reviewed and edited the manuscript. ŽMP processed rootstock samples, performed DNA extractions, contributed to the optimization of PCR conditions, conducted PCR detection assays, and reviewed and edited the manuscript. All authors contributed to the article and approved the submitted version.
This work was supported by the New York State Farm Viability Institute competitive grants program 2017, through a 2-year grant award 81927/A001-FVI 17-006 to SGA for the project “Development of effective spray program for post-infection fire blight management in apples and cost-benefit analysis of its key components”, by the New York State Department of Agriculture and Markets Apple Research and Development Program 2018-2019 for the grant award NYS ESDC ARDP 86698-11419 to SGA for the project “Continued lab detection of fire blight bacterium Erwinia amylovora in susceptible apple rootstocks in NY orchards and Hudson Valley Research Laboratory efficacy trials”, and by the Cornell Cooperative Extension Jefferson County’s Northern New York Agricultural Development Program 2017, through the grant award 84896/A001 NNY Acimovic Apple ID: 2018-023C, to SGA for the project “Continued Lab Detection of Fire Blight Bacterium Erwinia amylovora in Susceptible Apple Rootstocks in Commercial Orchards Affected by the 2016 Epidemic in Northern NY”.
The authors thank Dr. David A. Rosenberger, Professor Emeritus at Cornell University’s Hudson Valley Research Laboratory, for valuable advice. The authors are thankful to the Cornell Cooperative Extension Agents in the Champlain Lake Valley, Anna Wallis and Michael Basedow, for helping in communication with apple growers and owners of the farms affected with the fire blight epidemic in 2016. Finally, the authors are grateful to the apple growers for giving them access to their farms for establishing rootstock canker experiments in their orchards and helping us manage them from 2016 – 2018.
The authors declare that the research was conducted in the absence of any commercial or financial relationships that could be construed as a potential conflict of interest.
All claims expressed in this article are solely those of the authors and do not necessarily represent those of their affiliated organizations, or those of the publisher, the editors and the reviewers. Any product that may be evaluated in this article, or claim that may be made by its manufacturer, is not guaranteed or endorsed by the publisher.
The Supplementary Material for this article can be found online at: https://www.frontiersin.org/articles/10.3389/fhort.2023.1082204/full#supplementary-material
Aćimović S. G., Balaž J. S., Aćimović D.Đ., Reeb P. D. (2014). High magnitude of fire blight symptom development and canker formation from July onwards on two apple cultivars under severe natural infections. J. Plant Pathol. 96, 159–168. doi: 10.4454/JPP.V96I1.035
Aćimović S. G., Meredith C. L. (2017). Evaluation of newer biologicals and the SAR-activator candidate regalia in fire blight control applied by spraying or trunk injection. Fruit Q. 25, 25–29. Available at: https://www.researchgate.net/publication/323152886_Evaluation_of_newer_biologicals_and_the_SAR-activator_candidate_Regalia_in_fire_blight_control_applied_by_spraying_or_trunk_injection.
Aćimović S. G., Meredith C. L., Santander R. D., Khodadadi F. (2021). Proof of concept for shoot blight and fire blight canker management with postinfection spray applications of prohexadione-calcium and acibenzolar-S-Methyl in apple. Plant Dis. 105, 4095–4105. doi: 10.1094/PDIS-08-20-1744-RE
Aldwinckle H. S., LoGiudice N., Robinson T. L., Holleran H. T., Fazio G., Johnson W. C., et al. (2004). Resistance of apple rootstocks to fire blight infection caused by internal movement of Erwinia amylovora from scion infections. Acta Hort 663, 229–233. doi: 10.17660/ActaHortic.2004.663.36
Ault T. R., Schwartz M. D., Zurita-Milla R., Weltzin J. F., Betancourt J. L. (2015). Trends and natural variability of spring onset in the coterminous United States as evaluated by a new gridded dataset of spring indices. J. Clim. 28, 8363–8378. doi: 10.1175/JCLI-D-14-00736.1
Aycock R. (1966). Stem Rot and other diseases by Sclerotium rolfsii or the status of the Rolf's fungus after 70 years. In North Carolina Agricultural Experiment Station, Tech. Bul. No. 174, 202.
Bellemann P., Bereswill S., Berger S., Geider K. (1994). Visualization of capsule formation by Erwinia amylovora and assays to determine amylovoran synthesis. Int. J. Biol. Macromol. 16, 290–296. doi: 10.1016/0141-8130(94)90058-2
Bereswill S., Geider K. (1997). Characterization of the rcsB gene from Erwinia amylovora and its influence on exoploysaccharide synthesis and virulence of the fire blight pathogen. J. Bacteriol. 179, 1354–1361. doi: 10.1128/jb.179.4.1354-1361.1997
Bereswill S., Jock S., Bellemann P., Geider K. (1998). Identification of Erwinia amylovora by growth morphology on agar containing copper sulfate and by capsule staining with lectin. Plant Dis. 82, 158–164. doi: 10.1094/PDIS.1998.82.2.158
Bereswill S., Pahl A., Bellemann P., Zeller W., Geider K. (1992). Sensitive and species-specific detection of Erwinia amylovora by polymerase chain reaction analysis. Appl. Environ. Microbiol. 58, 3522–3526. doi: 10.1128/aem.58.11.3522-3526.1992
Billing E., Baker L., Crosse J. E., Garrett C. M. E. (1961). Characteristics of English isolates of Erwinia amylovora (Burrill) Winslow et al. J. Appl. Bacteriol. 24, 195–211. doi: 10.1111/j.1365-2672.1961.tb00253.x
CBC News (2014) Fire blight hits 90% of apple orchards in Nova Scotia. CBC news (CBC). Available at: https://www.cbc.ca/news/canada/nova-scotia/apple-fire-blight-hits-90-of-nova-scotia-orchards-1.2754597 (Accessed February 11, 2019).
CBC News. (2016). Help on the way for Nova Scotia fruit farmers devastated by fire blight. CBC news (CBC). Available at: https://www.cbc.ca/news/canada/nova-scotia/fire-blight-funding-ns-apple-pear-growers-1.3421282 (Accessed February 11, 2019).
Cornell University. (2016). NEWA - Network for Environment and Weather Applications. Available at: https://newa.cornell.edu/crop-and-pest-management (Accessed September 19, 2022).
Courtney R.. (2020). Expanded coverage for trees. In: Good fruit grow. Available at: https://www.goodfruit.com/expanded-coverage-for-trees/ (Accessed September 22, 2022).
Donat V., Biosca E. G., Peñalver J., López M. M. (2007). Exploring diversity among Spanish strains of Erwinia amylovora and possible infection sources. J. Appl. Microbiol. 103, 1639–1649. doi: 10.1111/j.1365-2672.2007.03399.x
Douglas S. M. (2022). Fire blight. PP0299 503R, Department of Plant Pathology and Ecology, The Connecticut Agricultural Experiment Station. 5, 1–8. Available at: https://portal.ct.gov/CAES/Fact-Sheets/Plant-Pathology/Fire-Blight.
Ferree D. C., Schmid J. C., Bishop B. L. (2002). Survival of apple rootstocks to natural infections of fire blight. HortTechnology 12, 239–241. doi: 10.21273/HORTTECH.12.2.239
Frumhoff P. C., McCarthy J. J., Melillo J. M., Moser S. C., Wuebbles D. J. (2007). Confronting climate change in the US northeast. Science, Impacts, and Solutions. Synthesis report of the Northeast. Climate Impacts Assessment (NECIA). A report of the Northeast Climate Impacts Assessment, Union of Concerned Scientists. Cambridge, MA., 47–61. Available at: https://www.ucsusa.org/sites/default/files/2019-09/confronting-climate-change-in-the-u-s-northeast.pdf.
Griffiths H. M. (2021). Fire blight a concern for NY orchards. Lanc. Farming. Available at: https://www.lancasterfarming.com/fire-blight-a-concern-for-ny-orchards/article_7f632389-1d5d-53d8-8be3-a5fd1664f695.html.
Groisman P. Y., Knight R. W., Zolina O. G. (2013). Recent trends in regional and global intense precipitation patterns. Clim. Vulnerability 5, 25–55. doi: 10.1016/B978-0-12-384703-4.00501-3
Gruber P. (2022). Cause of rapid apple decline remains mysterious. Lanc. Farming. Available at: https://www.lancasterfarming.com/farming-news/news/cause-of-rapid-apple-decline-remains-mysterious/article_89b09b1e-8507-11ec-9890-e325193f8221.html#:~:text=Rapid%20apple%20decline%20appears%20as,like%20drought%20or%20harsh%20winter.
Ham H., Kim K., Yang S., Kong H. G., Lee M.-H., Jin Y. J., et al. (2022). Discrimination and detection of Erwinia amylovora and Erwinia pyrifoliae with a single primer set. Plant Pathol. J. 38, 194–202. doi: 10.5423/PPJ.OA.03.2022.0027
Hayhoe K., Wake C. P., Huntington T. G., Luo L., Schwartz M. D., Sheffield J., et al. (2007). Past and future changes in climate and hydrological indicators in the US northeast. Clim. Dyn. 28, 27. doi: 10.1007/s00382-006-0187-8
Ishimaru C., Klos E. J. (1984). New medium for detecting Erwinia amylovora and its use in epidemiological studies. Phytopathology 74, 1342–1345. doi: 10.1094/Phyto-74-1342
Johnson K., Temple T. N. (2016). Comparison of methods of acibenzolar-S-Methyl application for post-infection fire blight suppression in pear and apple. Plant Dis 100, 1125–1131. doi: 10.1094/PDIS-09-15-1062-RE
Keenan T. F., Gray J., Friedl M. A., Toomey M., Bohrer G., Hollinger D. Y., et al. (2014). Net carbon uptake has increased through warming-induced changes in temperate forest phenology. Nat. Clim. Change 4, 598–604. doi: 10.1038/nclimate2253
King E. O., Ward M., Raney D. E. (1954). Two simple media for the demonstration of pyocyanin and fluorescein. J. Lab. Clin. Med. 44, 301–307.
Kunkel K., Stevens L., Stevens S., Sun L., Janssen E., Wuebbles D., et al. (2013). Regional climate trends and scenarios for the US national climate assessment. part 1. climate of the northeast US NOAA technical report NESDIS 142-1. National Oceanic and Atmospheric Administration, National Environmental Satellite, Data, and Information Service, Washington, D.C. 87 pp. Available at: https://scenarios.globalchange.gov/sites/default/files/NOAA_NESDIS_Tech_Report_142-1-Climate_of_the_Northeast_U.S_1.pdf.
Liu H., Wu L., Nikolaeva E., Peter K., Liu Z., Mollov D., et al. (2018). Characterization of a new apple luteovirus identified by high-throughput sequencing. Virol. J. 15, 85. doi: 10.1186/s12985-018-0998-3
Loper J. E., Henkels M. D., Roberts R. G., Grove G. G., Willet M. J., Smith T. J. (1991). Evaluation of streptomycin, oxytetracycline, and copper resistance of Erwinia amylovora isolated from pear orchards in Washington state. Plant Dis. 75, 287–290. doi: 10.1094/PD-75-0287
Maes M., Orye K., Bobev S., Devreese B., van Beeumen J., de Bruyn A., et al. (2001). Influence of amylovoran production on virulence of Erwinia amylovora and a different amylovoran structure in E. amylovora isolates from Rubus. Eur. J. Plant Pathol. 107, 839–844. doi: 10.1023/A:1012215201253
Mann R. A., Smits T. H. M., Bühlmann A., Blom J., Goesmann A., Frey J. E., et al. (2013). Comparative genomics of 12 strains of Erwinia amylovora identifies a pan-genome with a large conserved core. PloS One 8, e55644. doi: 10.1371/journal.pone.0055644
Mendes R. J., Amaro C., Luz J. P., Tavares F., Santos C. (2022). Variability within a clonal population of Erwinia amylovora disclosed by phenotypic analysis. PeerJ 10, e13695. doi: 10.7717/peerj.13695
Menzel A., Sparks T. H., Estrella N., Koch E., Aasa A., Ahas R., et al. (2006). European Phenological response to climate change matches the warming pattern. Glob. Change Biol. 12, 1969–1976. doi: 10.1111/j.1365-2486.2006.01193.x
Momol M. T., Norelli J. L., Piccioni D. E., Momol E. A., Gustafson H. L., Cummins J. N., et al. (1998). Internal movement of Erwinia amylovora through symptomless apple scion tissues into the rootstock. Plant Dis. 82, 646–650. doi: 10.1094/PDIS.1998.82.6.646
Norelli J. L., Aldwinckle H. (2001). Resistance of 'Geneva' apple rootstocks to Erwinia amylovora when grown as potted plants and orchard trees. in. IX Int. Workshop Fire Blight 590, 359–362. doi: 10.17660/ActaHortic.2002.590.53
Norelli J. L., Aldwinckle H. S., Beer S. V., Lamb R. C. (1987). The effects of virulence of Erwinia amylovora on the evaluation of fire blight resistance in Malus. Phytopathology 77, 1551–1555. doi: 10.1094/Phyto-77-1551
Norelli J. L., Holleran H. T., Johnson W. C., Robinson T. L., Aldwinckle H. S. (2003a). Resistance of Geneva and other apple rootstocks to Erwinia amylovora. Plant Dis. 87, 26–32. doi: 10.1094/PDIS.2003.87.1.26
Norelli J. L., Jones A. L., Aldwinckle H. S. (2003b). Fire blight management in the twenty-first century: using new technologies that enhance host resistance in apple. Plant Dis. 87, 756–765. doi: 10.1094/PDIS.2003.87.7.756
Obradović D., Balaž J., Kevrešan S. (2007). Detection of Erwinia amylovora by novel chromosomal polymerase chain reaction primers. Microbiology 76, 748–756. doi: 10.1134/S002626170706015X
Ordax M., Biosca E. G., López M. M., Marco-Noales E. (2012). Improved recovery of Erwinia amylovora-stressed cells from pome fruit on RESC, a simple, rapid and differential medium. Trees – Struct. Funct. 26, 83–93. doi: 10.1007/s00468-011-0614-2
Paulin J. P., Samson R. (1973). Feu bacterien en france. II. caracteres des souches d’Erwinia amylovora (Burril) Winslow et al. 1920, isolees du foyer franco-belge. Ann. Phytopathol. 5, 389–397.
Peter K. A. (2020). Apple disease - southern blight. Penn State Ext. Available at: https://extension.psu.edu/apple-disease-southern-blight.
Philion V., Trapman M. (2011). Description and preliminary validation of RIMpro-Erwinia, a new model for fire blight forecast. Acta Hortic. 896, 307–318. doi: 10.17660/ActaHortic.2011.896.43
Popović T., Jelušić A., Živković L., Živković N., Iličić R., Stanisavljević R., et al. (2020). Identification, genetic characterization and virulence of Serbian Erwinia amylovora isolates. Eur. J. Plant Pathol. 157, 857–872. doi: 10.1007/s10658-020-02046-1
Puławska J., Sobiczewski P. (2012). Phenotypic and genetic diversity of Erwinia amylovora: the causal agent of fire blight. Trees 26, 3–12. doi: 10.1007/s00468-011-0643-x
RIMpro-Erwinia (2016). Available at: https://rimpro.eu (Accessed September 19, 2022).
Robbins J. (2019). Fire blight spreads northward, threatening apple orchards. N. Y. Times. Issue of Dec. 3, 2019, Section D, Page 1. Available at: https://www.nytimes.com/2019/12/02/science/fire-blight-spreads-northward-threatening-apple-orchards.html.
Robinson T., Anderson L., Autio W., Barritt B., Cline J., Cowgill W., et al. (2007). A multi-location comparison of “Geneva® 16”, “Geneva® 41” and “M.9“apple rootstocks in North America. Acta Hortic. 732, 59–65. doi: 10.17660/ActaHortic.2007.732.4
Russo N. L., Robinson T. L., Fazio G., Aldwinckle H. S. (2007). Field evaluation of 64 apple rootstocks for orchard performance and fire blight resistance. HortScience 42, 1517–1525. doi: 10.21273/HORTSCI.42.7.1517
Santander R. D., Figàs-Segura À., Biosca E. G. (2018). Erwinia amylovora catalases KatA and KatG are virulence factors and delay the starvation-induced viable but non-culturable (VBNC) response. Mol. Plant Pathol. 19, 922–934. doi: 10.1111/mpp.12577
Santander R. D., Gašić K., Aćimović S. G. (2022a). “Selective quantification of Erwinia amylovora live cells in pome fruit tree cankers by viability digital PCR,” in Plant pathology: Method and protocols methods in molecular biology. Ed. Luchi N. (New York, NY: Springer US), 231–249. doi: 10.1007/978-1-0716-2517-0_14
Santander R. D., Khodadadi F., Meredith C. L., Rađenović Ž., Clements J., Aćimović S. G. (2022b). Fire blight resistance, irrigation and conducive wet weather improve Erwinia amylovora winter survival in cankers. Front. Microbiol. 13: 1009364. doi: 10.3389/fmicb.2022.1009364
Santander R. D., Meredith C. L., Aćimović S. G. (2019). Development of a viability digital PCR protocol for the selective detection and quantification of live Erwinia amylovora cells in cankers. Sci. Rep. 9, 11530. doi: 10.1038/s41598-019-47976-x
Santander R. D., Monte-Serrano M., Rodríguez-Herva J. J., López-Solanilla E., Rodríguez-Palenzuela P., Biosca E. G. (2014). Exploring new roles for the rpoS gene in the survival and virulence of the fire blight pathogen Erwinia amylovora. FEMS Microbiol. Ecol. 90, 895–907. doi: 10.1111/1574-6941.12444
Singh J., Silva K. J. P., Fuchs M., Khan A. (2019). Potential role of weather, soil and plant microbial communities in rapid decline of apple trees. PloS One 14, e0213293. doi: 10.1371/journal.pone.0213293
Smith T. J. (2000) Cougar blight fire blight infection risk assessment model (Washington State University). Available at: http://treefruit.wsu.edu/crop-protection/disease-management/fire-blight/cougar-blight-model/#:~:text=The%20Cougar%20Blight%20Model%20estimates,events%20based%20on%20blossom%20wetting (Accessed October 27, 2022).
Smith T. J., Pusey P. L. (2011). CougarBlight 2010, a significant update of the CougarBlight fire blight infection risk model. Acta Hort 896, 331–336. doi: 10.17660/ActaHortic.2011.896.45
Steiner P. W. (2000). The biology and epidemiology of fire blight. University of Maryland, pg. 6. Available at: http://extension.cropsciences.illinois.edu/fruitveg/pdfs/biology_fire_blight.pdf.
Stöger A., Schaffer J., Ruppitsch W. (2006). A rapid and sensitive method for direct detection of Erwinia amylovora in symptomatic and asymptomatic plant tissues by polymerase chain reaction. J. Phytopathol. 154, 469–473. doi: 10.1111/j.1439-0434.2006.01130.x
Stokstad E. (2019). Something is rapidly killing young apple trees in North American orchards. Scientists are stumped. Science News. [Accessed September 15, 2022]. doi: 10.1126/science.aax4195
Sutton T. B., Aldwinckle H. S., Agnello A. M., Walgenbach J. F. (2014). Compendium of apple and pear diseases and pests. 2nd Edition. The American Phytopathological Society, St. Paul, MN.
Taylor R. K., Guilford P. J., Clark R. G., Hale C. N., Forster R. L. S. (2001). Detection of Erwinia amylovora in plant material using novel polymerase chain reaction (PCR) primers. N. Z. J. Crop Hortic. Sci. 29, 35–43. doi: 10.1080/01140671.2001.9514158
Tree Fruit IPM Working Group (2020) Ranking of research and extension priorities: 8-year comparison. Available at: https://www.northeastipm.org/.
Turechek W. W., Biggs A. R. (2015). Maryblyt v. 7.1 for windows: An improved fire blight forecasting program for apples and pears. Plant Health Prog. 16, 16–22. doi: 10.1094/PHP-RS-14-0046
USDA (2020a) APPLE TREE FIRE BLIGHT ENDORSEMENT, common crop insurance policy basic provisions. Available at: https://www.rma.usda.gov/-/media/RMA/Policies/Apple-Tree/Apple-Tree-Crop-Provisions-21-APT/Apple-Tree-Fire-Blight-Endorsement-21-APT-B.ashx.
USDA (2020b) Federal crop insurance corporation - APPLE TREE CROP PROVISIONS. Available at: https://rma.usda.gov/-/media/RMA/Policies/Apple-Tree/Apple-Tree-Crop-Provisions-21-APT.ashx.
Utah State University (2022). Pest management options: Crown and root suckers. Intermountain Tree Fruit Production Guide for Utah, Colorado, Idaho, Montana. Available at: https://intermountainfruit.org/pest-management/crown_and_root_suckers. (Accessed September 19, 2022).
Utah State University - Extension (2020) Managing suckers around fruit trees. Available at: https://extension.usu.edu/productionhort/research/managing-suckers-around-fruit-trees (Accessed September 19, 2022).
Vanneste J. L., Eden-Green S. (2000). “Migration of Erwinia amylovora in host plant tissues,” in Fire blight: the disease and its causative agent, Erwinia amylovora (Hamilton, New Zealand: CABI), 73–83.
Végh A., Hevesi M., Pájtli É., Palkovics L. (2017). Characterization of Erwinia amylovora strains from Hungary. Eur. J. Plant Pathol. 147, 455–461. doi: 10.1007/s10658-016-1001-z
Wertheim S. J. (1998). Rootstock guide: Apple, pear, cherry, European plum (The Netherlands: Fruit Research Station Wilhelminadorp).
Winslow C.-E. A., Broadhurst J., Buchanan R. E., Krumwiede C., Rogers L. A., Smith G. H. (1920). The families and genera of the bacteria final report of the committee of the society of American bacteriologists on characterization and classification of bacterial types. J. Bacteriol. 5, 191–229. doi: 10.1128/jb.5.3.191-229.1920
WSU (2022). Rootstocks for apple. WSU Tree Fruit. Available at: http://treefruit.wsu.edu/web-article/apple-rootstocks/. [Accessed October 4, 2022].
Yeonghyeon G., Yin H., Jin D., Zheng R., Lee J.-M. (2022) Computer vision-based deep learning for fire blight recognition. third international symposium on fire blight of rosaceous plants (ISFB), 5-9 Sep 2022, Dresden, Germany. Available at: https://3rd-fireblightsymposium.julius-kuehn.de/ (Accessed September 5, 2022).
Keywords: rootstock blight, fire blight losses, apple tree death, PCR detection, Erwinia amylovora, strain characterization
Citation: Aćimović SG, Santander RD, Meredith CL and Pavlović ŽM (2023) Fire blight rootstock infections causing apple tree death: A case study in high-density apple orchards with Erwinia amylovora strain characterization. Front. Hortic. 2:1082204. doi: 10.3389/fhort.2023.1082204
Received: 27 October 2022; Accepted: 30 January 2023;
Published: 13 March 2023.
Edited by:
Marcel Wenneker, Wageningen University and Research, NetherlandsReviewed by:
Assunta Bertaccini, University of Bologna, ItalyCopyright © 2023 Aćimović, Santander, Meredith and Pavlović. This is an open-access article distributed under the terms of the Creative Commons Attribution License (CC BY). The use, distribution or reproduction in other forums is permitted, provided the original author(s) and the copyright owner(s) are credited and that the original publication in this journal is cited, in accordance with accepted academic practice. No use, distribution or reproduction is permitted which does not comply with these terms.
*Correspondence: Srđan G. Aćimović, YWNpbW92aWNAdnQuZWR1
Disclaimer: All claims expressed in this article are solely those of the authors and do not necessarily represent those of their affiliated organizations, or those of the publisher, the editors and the reviewers. Any product that may be evaluated in this article or claim that may be made by its manufacturer is not guaranteed or endorsed by the publisher.
Research integrity at Frontiers
Learn more about the work of our research integrity team to safeguard the quality of each article we publish.