- 1Department of Horticultural Sciences, Faculty of Agricultural Science and Engineering, College of Agriculture and Natural Resources, University of Tehran, Karaj, Iran
- 2Photosynthesis Laboratory, Department of Horticulture, University of Tehran, Tehran, Iran
- 3Department of Horticultural Science, North Carolina State University, Raleigh, NC, United States
- 4Department of Plant and Environmental Sciences, Copenhagen Plant Science Centre, University of Copenhagen, Frederiksberg C, Denmark
- 5Department of Horticultural Science, INRES-Institute of Crop Science and Resource Conservation, University of Bonn, Bonn, Germany
Saffron quality is determined by the content of three apocarotenoids, including crocin, picrocrocin, and safranal, giving saffron culinary, industrial, and medical importance. The level of these secondary metabolites is also affected by environmental factors such as light, which play a pivotal role in regulating plants' signaling pathways. In this study, we investigated the effects of different ratios of blue (B) to red (R) and white (W) light on physiological, biochemical, and molecular responses of saffron in the flowering stage. Flowers' morphological properties were improved in plants grown under monochromatic B light. The highest content of total carotenoids, anthocyanins, and flavonoids was detected in plants grown under a high proportion of B light. The highest crocin content, especially the trans-crocetin ester isomer, was recorded in monochromatic B light-grown plants. However, the highest picrocrocin content was in both monochromatic R- and B light-grown plants. The highest safranal content was detected in plants grown under a high proportion of R light. Transcriptome analysis of secondary metabolism pathways showed that the transcript level of the genes was highly correlated with the content of the target metabolites. Monochromatic B light upregulated the expression of genes involved in crocin production (CsCCD2, CsALDH31l, and CsUGT2). However, the expression of CsUGT709G1, which is involved in picrocrocin and safranal pathways, was upregulated in plants grown under a high ratio of R light and W lights. In conclusion, monochromatic B light enhances the flowering rate, crocin (trans-crocetin ester), and picrocrocin content, which increases the quantity and quality of saffron products under controlled conditions.
Introduction
Known as the Red Gold, the dried stigmas of saffron are the most expensive culinary additive globally. They are highly valued in the textile dyeing industry, cosmetics, and, most importantly, the pharmaceutical industry (Moratalla-López et al., 2019). Saffron is also used in disease treatments such as Alzheimer's, impotence, diabetes, fatty liver, and cancer (Mzabri et al., 2019; Roshanravan and Ghaffari, 2021). Saffron petals can be used as an antidepressant, antibacterial, antispasmodic, immunomodulatory, and antitussive (Serrano-Díaz et al., 2012; Hosseini et al., 2018; Mzabri et al., 2019; Roshanravan and Ghaffari, 2021). During the last few years, it has been suggested to use saffron petals as an essential by-product of saffron for its antioxidants such as anthocyanins and flavonoids. The value and price of saffron are determined by crocin, picrocrocin, and safranal, which provide stigma's color, taste, and aroma. The essential secondary metabolite in saffron is crocin, a crocetin ester (CE) derived from carotenoids. Crocetin ester has two isomers, trans and cis, from which the trans isomer is pharmacologically more valuable than cis isomer (Moratalla-López et al., 2019; García-Rodríguez et al., 2021). The bitter taste of saffron is derived from the glycoside terpenoid compound known as picrocrocin (Tahri et al., 2015). Safranal is also an important essential oil, which results in the unique aroma of stigma and is used in perfume manufacturing. The amount of this volatile substance in fresh flowers is negligible, but its value soars in the drying process of stigma during post-harvest (Rezaee and Hosseinzadeh, 2013; García-Rodríguez et al., 2021). Accordingly, the post-harvest conditions of drying and storage are determined by the amount of this substance (Moratalla-López et al., 2019). The mentioned three substances are the most important secondary metabolites in saffron plants. As a result, efforts have been made to increase these three apocarotenoids, both naturally and artificially (Mir et al., 2010). Saffron is also rich in other secondary metabolites, such as different types of anthocyanins and flavonoids in other parts of the flower.
Environmental conditions are fundamental in producing and storing apocarotenoids (Felemban et al., 2019). With the rapid growth of controlled-environment agriculture (CEA), saffron producers have recently shown interest in the indoor production of saffron. This is due to ease of change in environmental cues due to high-tech instrumental devices in CEA. Reduction of labor work, increased flowering and yield, facilitating dormancy removal, accelerating forcing, and flowering in synchronous flowering (which is of great importance in mechanization), and high quality of saffron stigma are among the advantages of saffron production in controlled-environment systems (Molina et al., 2004, 2005b, 2010; Renau-Morata et al., 2012; Poggi et al., 2017; García-Rodríguez et al., 2021; Moradi et al., 2021). Among all environmental factors, light plays a regulatory role in many processes such as photosynthesis, photo-morphogenesis, and the production of secondary metabolites (Kozai et al., 2016). Light quality, in particular, has been shown to regulate antioxidant capacity, pigment accumulation, and photosynthesis performance (Ouzounis et al., 2014; Hosseini et al., 2019; Naznin et al., 2019; Aalifar et al., 2020a). Despite many studies on many other environmental factors, the effect of light on the production of secondary metabolites of saffron has mostly slipped the scientific attention. To date, just one study has mentioned the positive effect of far-red (FR) light on the crocin production of saffron stigma (Kajikawa et al., 2018).
At the molecular level, a large body of research has been performed on key genes involved in the production of crocin, picrocrocin, and safranal. Recent studies have identified carotenoid cleavage dioxygenase (CsCCD2), Aldehyde dehydrogenases (CsALDH31l), and UDP-glycosyltransferases (CsUGT2 or UGT74AD21) as central genes for predicting crocin levels (Ahrazem et al., 2019; Diretto et al., 2019). The expression analysis of the CsUGT709G1 gene has also been proposed to estimate picrocrocin production (Diretto et al., 2019; López et al., 2021). The gene encoding the enzyme that converts picrocrocin to safranal has not yet been identified in saffron, and CsUGT709G1 gene has been suggested for estimating safranal production at the molecular level (Diretto et al., 2019).
The present study aims to shed light on the effect of different ratios of B to R light as the two main spectra for providing energy for the photosynthetic process together with white (W) light as the control on the morphological traits of flower, as well as production of secondary metabolites at the biochemical and molecular levels.
Materials and methods
Plant materials and growth conditions
Saffron (Crocus sativus L.) corms were provided from a traditional saffron production region (Nishabur, Khorasan-Razavi Province, Iran). Corms were harvested in late June then equal-sized daughter corms weighing between 10–12 g were selected. After dipping into a fungicide solution (0.1% Prochloraz) and dried for 1–2 h, corms were incubated in the darkroom for flower initiation at 25°C and 85 ± 2% relative humidity for 90 days as described before (Molina et al., 2005a; Moradi et al., 2021). Corms were then planted in pots filled with medium-size perlite as the culture medium. The rest of the experiment was carried out in a closed LED-equipped growth chamber with a 10 m2 floor area and 2.5 m height.
Corms were fertilized every other day (i.e., ≈ three times a week) with Hoagland nutrient solution (Hoagland and Arnon, 1938) using a drip irrigation system (Hoagland and Arnon, 1938). Corms were incubated at 17°C under different light conditions provided by blue (B, 400–500 nm), red (R, 600–700 nm), combinations of B and R light including 100%B (monochromatic B light), 75%B (75%B + 25%R), 50%B (50%B + 50%R), 25%B (25%B + 75%R), 0% (monochromatic R light), and white (W) provided by LED modules (24 W, OPPEL, China) at a photosynthetic photon flux density (PPFD) of 150 ± 10 μmol m−2 s−1. Following this, the temperature was set to 15/6 °C (light/dark) with an 11/13 h photoperiod for induction of corms since previous studies showed that the highest sink capacity of corms occurs in this condition (Miyagawa et al., 2015; Kajikawa et al., 2018).
The light intensity and spectrum were monitored using Fluorpen FP 100-MAX (Photon Systems Instruments, Drasov, Czech Republic) and Sekonic light meter (Sekonic C-7000), respectively. The lighting conditions and the relative spectra of each light treatment are shown in Figure 1.
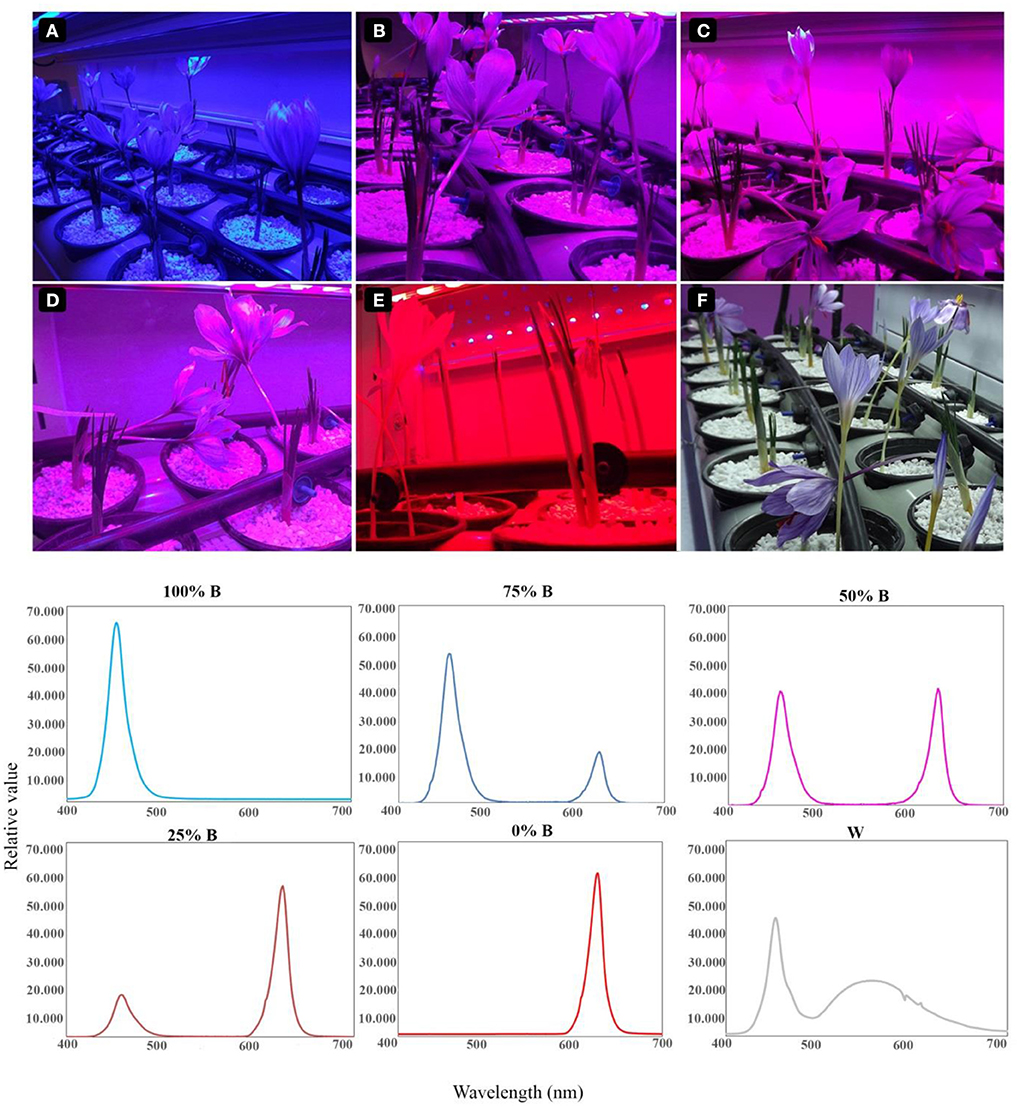
Figure 1. Lighting conditions for the growth of saffron plants. Six lighting treatments are shown based on the percentage of blue (B) to red (R) light including: (A) 100%B (monochromatic B), (B) 75%B (75%B + 25% R), (C) 50%B (50%B + 50%R), (D) 25%B (25%B + 75%R), (E) 0% B (monochromatic R) as well as (F) White (W).
Morphological properties of the saffron flower
Morphological characteristics of flowers such as flower number per corm, flower fresh weight (FW), flower dry weight (DW), flower diameter, stigma height, FW and DW were measured at flowering time. Flowering was observed 18–24 days after light regimes, and fresh weight of flower and stigma samples were recorded immediately after harvesting. For analyzing their DW, flowers and stigmas were oven-dried at 70°C for 48 h and 72 min, respectively.
Pigments measurement
The effect of light conditions on some antioxidant pigments (i.e., carotenoids, anthocyanins, and flavonoids) of saffron flowers was assessed. For assaying total carotenoid content, 100 mg powder of petal tissue was homogenized with 10 mL of 80% acetone and then incubated at 4°C in darkness for 24 h. The solution was centrifuged (SIGMA 1-14K, Darmstadt, Germany) at 6,000 rpm for 10min at 25°C, and the absorbance of the supernatant was spectrophotometrically recorded at 470 nm (PowerWaveTM XSMicroplate Reader, BioTek Instruments, Inc. Vermont, USA).
The total anthocyanins content was assayed by making methanol-formic acid (98:2, v/v) extraction solution. Subsequently, 25 ml of this solution were added to vials containing 500 mg powder of saffron petals to extract total anthocyanin. The vials were then subjected to an ultrasonic bath for 2 min and centrifuged (SIGMA 1-14K, Darmstadt, Germany) at 2500 rpm for 5 min at 25°C. Finally, anthocyanins were quantified based on calibration curves of malvidin-3-glucoside (Serrano-Díaz et al., 2012).
For assaying total flavonoids content, 500 mg of petals were homogenized with 25 mL of ethanol-H2O-1 M HCl (70:29:1, v/v/v) solution, then shacked (Sigma Darmstadt, Germany) for 1 h in dark conditions and centrifuged (SIGMA 1-14K, Darmstadt, Germany) at 2000 rpm for 20 min. Total flavonoids were calculated via calibration curves of kaempferol-3-glucoside (Serrano-Díaz et al., 2012).
Determination of secondary metabolites in saffron stigma
According to ISO 3632 (ISO 3632-2:2010(en), 2022), the aqueous extract was prepared using stigma parts of saffron plants. For this purpose, 900 mL of Milli-Q water were added to 0.5 g powdered stigma, and the solution was stirred by a magnetic stirrer at 1,000 rpm for one hour in dark conditions before adjusting to 1 L by Milli-Q water. Finally, the water solution was filtered by a hydrophilic polytetrafluoroethylene filter (PTFE) with a 0.45 μm pore size (Sigma-Aldrich, Darmstadt, Germany). For the identification and qualification of cis and trans-crocetin esters, safranal and picrocrocin, the analysis was carried out according to pervious study (García-Rodríguez et al., 2014). Accordingly, the values were identified by injecting 20 μl of the aqueous saffron extract into a high-performance liquid chromatography with diode-array detection (HPLC-DAD) instrument (Agilent 1200, California, USA). For this purpose, cis and trans-crocetin esters at 440 nm, picrocrocine at 250 nm and safranal at 330 nm was analyzed by the HPLC-DAD method with use of their standards. Their quantification was based on calibration curves according to a protocol defined by García-Rodríguez et al. (2014).
Total RNA extraction and RT-QPCR analysis
Stigma samples of saffron plants grown under different light treatments were collected at flowering time, immediately frozen in liquid nitrogen, and stored in a −80°C freezer. Samples were grounded to a fine powder and lyophilized (Beta 1-8 LSC, CHRIST Co, Osterode am Harz, Germany). Total RNA was isolated with TRIzol from 200 mg of stigma powder, according to Wang et al. (2012). The RNA integrity was checked by electrophoresis on 1.5% agarose gel. The RNA concentration and purity were determined on a NanoDrop spectrophotometer (Thermo, Nanodrop 2000, Wilmington, USA). Total RNA samples were reverse transcripted into cDNA using ProtoScript ® II First Strand cDNA Synthesis Kit #E6560 by using Oligo (dT)18 Primer (New England Biolabs, Hitchin, UK).
For analyzing gene expression of target genes in the pathway of saffron secondary metabolites, primers of CsCCD2, CsALDH31l, CsUGT2 (UGT74AD21), and CsUGT709G1 were designed using Primer3plus (Untergasser et al., 2012). The primers for quantitative real-time (qRT)-PCR are listed in Table 1. To choose the best reference gene, the stability of gene expression was assessed for 18S rRNA, ubiquitin, and tubulin genes, and based on this tubulin was used as the reference gene. Reactions were set up in a final volume of 15 μl HOT FIREPol® EvaGreen® qPCR Mix Plus (Solis BioDyne, Tartu, Estonia) according to the manufacturer's instructions. Assays were carried out with LightCycler® (Roche, Mannheim, Germany).
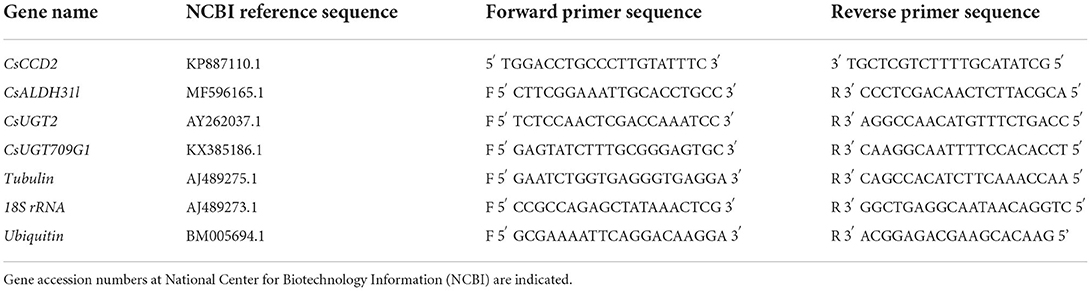
Table 1. Primer sequences for genes involved in crocin, picrocrocin, and safranal pathways and for reference genes.
Three biological replicates and two technical replicates were assessed. The relative gene expressions were calculated by the 2−ct method (Livak and Schmittgen, 2001).
Statistical analysis
In the present study, 20 plants were grown under each light treatments. Six replicate plants in each light treatment were randomly selected and used for the subsequent morphological, HPLC, and pigments measurement. For molecular analysis three biological and two technical replicates were used. Individual plants were considered as independent replicates. All the treatments were conducted in an environment controlled room. The data were subjected to analysis of variance (ANOVA) and means were separated using Duncan's multiple range tests at a significance level of 0.01 using SAS software version 9.4 (v. 9.4, SAS Institute Inc., Cary, NC, USA).
Results
Blue light accelerates flowering and increases flower number and stigma weight
In the current research, flowering occurred 18–22 days after the beginning of light exposure. The number of days to flowering was significantly different among treatments. The earliest flowering was recorded in plants grown under treatments with a high proportion of B light (i.e., 100, 75, and 25%B). The earliest flowering time was recorded in 100% B light-grown plants, almost 18% earlier than those grown under W light as control. Increase in B light proportion in the whole spectrum, resulted in elevation in the number of flowers per corm, flower FW and DW, as well as stigma FW and DW (Table 2). The highest number of flowers per corm (3.01) was observed in B light which was 4.3 times higher than plants grown under R light. The highest flower FW and DW were also observed in 100%B light-grown plants, with the former being 1.6 and the latter 3 times heavier compared to plants grown under W light. Furthermore, the highest stigma FW and DW were recorded in plants grown under B light, while their lowest weights were recorded in plants grown under W light. The thickest flower diameter was recorded in plants grown under a high ratio of B to R light (i.e., 100, 75, and 25%B).
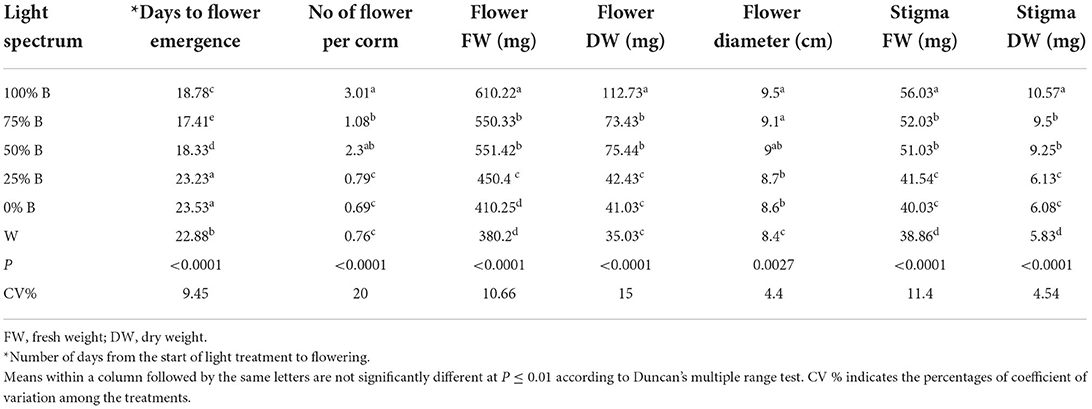
Table 2. Saffron flower properties under light recipes with different ratios of blue (B) to red (R) light including: 100%B (monochromatic B), 75%B (75%B + 25%R), 50%B (50%B + 50%R), 25%B (25%B + 75%R), 0%B (monochromatic R) as well as white (W), with 150 ± 10 μmol m−2 s−1 PPFD.
Blue light promotes the accumulation of antioxidant pigments in the saffron petal
The effects of different ratios of B to R and W light on the accumulation of antioxidant pigments in the petals of saffron flowers were analyzed. The carotenoid concentration showed a downward trend by increasing the ratio of R light (Figure 2A). The concentration of carotenoids in petals of plants grown under 100%B light was nearly 1.5 times higher than their concentrations in plants grown under 25%B and W light. Besides, no significant differences were noted between the carotenoid concentrations of petals that emerged under 75%B and 50%B lights, both of which ranked second among all.
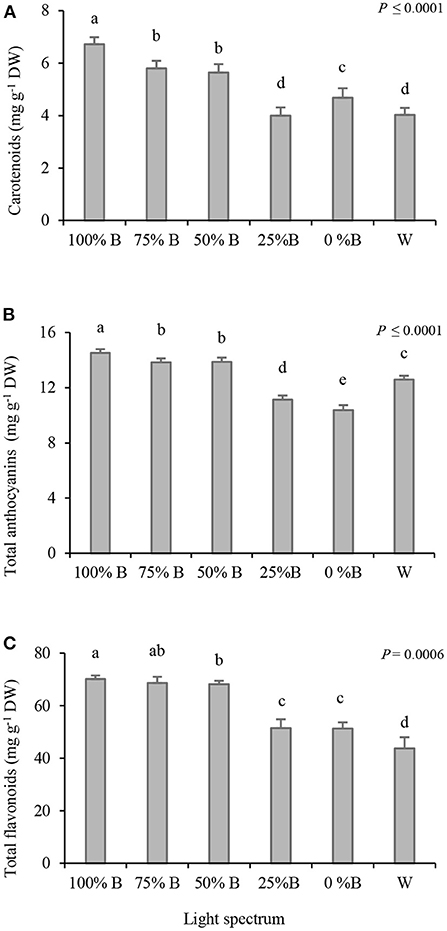
Figure 2. Carotenoids (A), anthocyanins (B), and flavonoids (C) content in the petals of saffron plants grown under light regimens with different ratios of blue (B) to red (R) light including: 100%B (monochromatic B), 75%B (75%B + 25%R), 50%B (50%B + 50%R), 25%B (25%B + 75%R), 0% B (monochromatic R) as well as white (W), with 150 ± 10 μmol m−2 s−1 PPFD. Different letters indicate that the value is significantly different at P ≤ 0.01 according to Duncan's multiple range test. Bars represent mean value of six replicate plants ± standard error (P ≤ 0.01). DW, dry weight.
Total anthocyanins showed an upward trend by increasing the B light proportion and the highest concentration was recorded in the flowers grown under the monochromatic B light (Figure 2B). Similar to the results of total carotenoids, no significant differences were recorded in the amount of anthocyanins between 75%B and 50%B treatments. Plants grown under a low ratio of B to R light (25%B and 0%B), showed the most negligible anthocyanins content.
The highest amount of flavonoids (~ 70 mg g−1 DW) was recorded in the petals of plants grown under 100%B and 75%B light treatments; while those grown under 50%B light ranked second (Figure 2C). Plants grown under W light showed the lowest flavonoid content (~ 44 mg g−1 DW). The concentration of flavonoids in 100%B light was almost 1.5 times higher than those grown under W light.
Red light promotes safranal content while both blue and red light promotes picrocrocin
According to HPLC-DAD analysis, increasing the ratio of B light led to an increase in the trans-CE content, while a downward trend was observed in cis-CE under the same conditions (Figures 3A–C). The highest amount of cis-CE was recorded in 25% B-grown plants, which was ~6 times higher than its content in plants grown under the 100% B light treatment. The highest content of total crocetin ester was recorded in the stigma of plants grown under 100%B light. The 25%B and 0%B light treatments did not show a significant difference (Figure 3C). Except for 50%B light, the total crocetin ester enhanced by increasing the ratio of B light, leading to the highest crocetin ester content under 100%B light (Figure 3C). The results of total crocetin ester percentage show the high percentage in the stigmas grown under high proportion of B light (100% B, 75%B and 50% B), also the lowest percentage was recorded in stigma grown under 25%B and 0%B (Figure 3D). Both monochromatic B and R lights (100%B and 0%B) resulted in a higher picrocrocin content than its amount under combinational light spectra (Figure 3E). An upward trend was observed in safranal content by decreasing the ratio of B to R light (Figure 3F). The highest safranal content was recorded in 0%B light which was two times higher than the safranal content of plants grown under 75%B light.
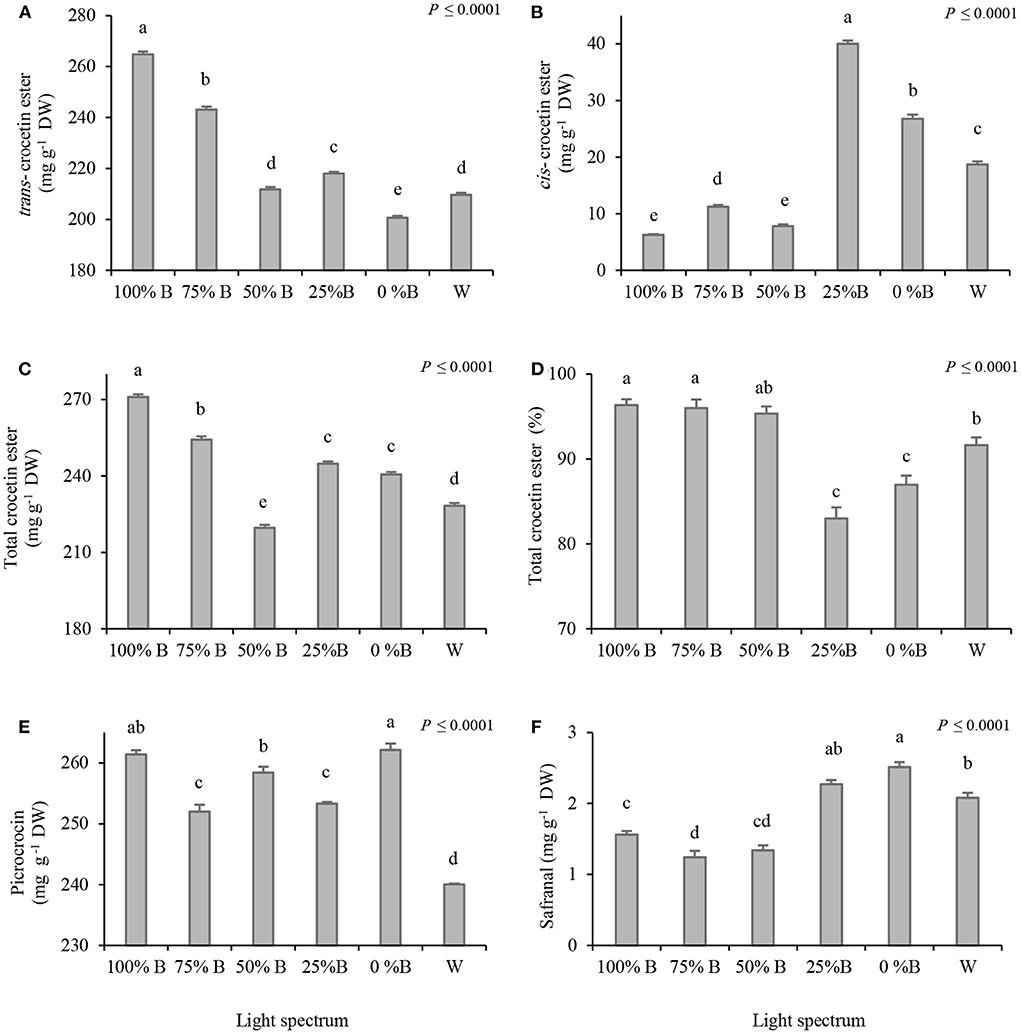
Figure 3. Crocin [trans-crocetin ester (A), cis-crocetin ester (B), total crocetin ester (C), percentage of trans-crocetin ester (D)], picrocrocin (E) and safranal (F) content (mg per g dry stigma) by HPLC-DAD analysis in Saffron plants grown under light recipes with different ratios of blue (B) to red (R) light including: 100%B (monochromatic B), 75%B (75%B + 25% R), 50%B (50%B+ 50%R), 25%B (25%B +75%R), 0% B (monochromatic R) as well as white (W), with 150 ± 10 μmol m−2 s−1 PPFD. Different letters indicate that the value is significantly different at P ≤ 0.01 according to Duncan's multiple range test. Bars represent mean value of six replicate plants ± standard error (P ≤ 0.01). DW, dry weight.
Gene expression results
Critical genes in crocin biosynthesis
Expression patterns of three critical genes for crocin biosynthesis (i.e., CsCCD2, CsALDH31l, and CsUGT2) in saffron stigmas were analyzed in response to different light regimes (Figure 4). The CsCCD2 was upregulated under lighting with a high proportion of B light (i.e., 100 and 75%B) together with monochromatic R (i.e., 0%B) (Figure 4A). Besides, the highest expression of CsALDH31l was detected in plants grown under 100%B light, which was six times higher than in plants grown under W light. Plants grown under 0%B conditions exhibited 3.5 fold increase in the expression of CsALDH31l compared to those grown under W light (Figure 4B). The expression of CsUGT2, which is the last gene in crocin biosynthesis, was upregulated by both monochromatic B and R light, with its highest expression recorded under B. The lowest CsUGT2 expression was detected in W light-grown plants (Figure 4C).
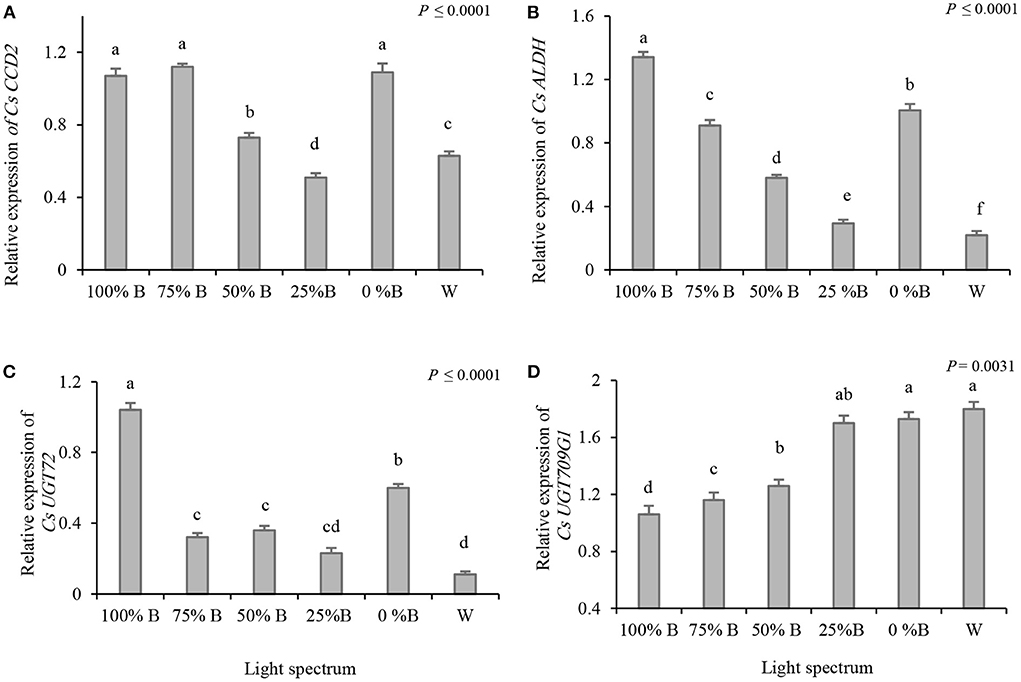
Figure 4. Relative gene expression of Cs CCD2 (A), CsALDH31l (B), CsUGT2 (C) and CsUGT709G1 (D) in the stigma of plants grown under light regimens with different ratios of blue (B) to red (R) light including: 100%B (monochromatic B), 75%B (75%B + 25% R), 50%B (50%B + 50%R), 25%B (25%B + 75%R), 0% B (monochromatic R) as well as white (W), with 150 ± 10 μmol m−2 s−1 PPFD. Different letters indicate that the value is significantly different at P ≤ 0.01 according to Duncan's multiple range test. Bars represent mean value of three replicate plants ± standard error (P ≤ 0.01).
Blue light decreases the expression of CsUGT709G1, encoding a key enzyme in the biosynthesis of picrocrocin and safranal
Since the gene responsible for the final step of safranal biosynthesis has not been identified so far, the changes in the expression of genes critical for picrocrocin biosynthesis (i.e., CsUGT709G1) were analyzed to reveal the regulatory role of light in the biosynthesis of these secondary metabolites. The results showed that the expression of CsUGT709G1 was upregulated by decreasing the B light proportion. The expression of CsUGT709G1 increased in plants grown under W, 25%B, and 0%B light treatments, with no significant differences among them, thereby indicating an 80% increase compared to plants grown under 100%B lights (Figure 4D).
To gain a general perspective on the relation among a: gene expression, b: metabolic content, c: photosynthesis performance, and d: carotenoid content (as the basic compound of the studied secondary metabolites), a correlation analysis was also performed (Figure 5). The results showed that trans-CE content was highly correlated with the expression of CsCCD2 (R2 = 0.67), CsALDH31l (R2 = 0.89) and CsUGT2 (R2 = 0.83). However, cis-CE and the expression of CCD2 (R2 = 0.07), CsALDH31l (R2 = −0.55) and CsUGT2 (R2 = −0.50) showed the least correlation. Carotenoid content and trans-CE were highly correlated (R2 = 0.962), while carotenoid content and cis-CE were found to be negatively correlated (R2 = −0.85). PIABS and Fv/Fm (derived from the results published in our previous study; Moradi et al., 2021) showed a high positive correlation with trans-CE (R2 = 0.77 for PIABS and 0.61 for Fv/Fm).
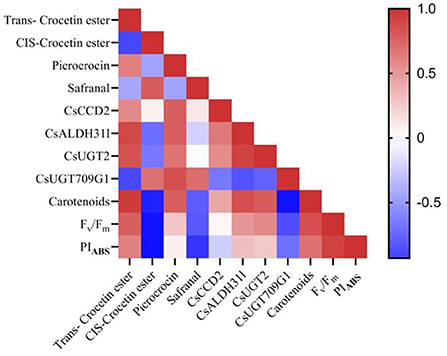
Figure 5. Correlation between gene expression of critical genes in crocin, picrocrocin and safranal biosynthesis and crocin (cis and trans crocetin ester), picrocrocin and safranal content in the stigma of saffron plants grown under light regimens with different ratios of blue (B) to red (R) light including 100%B (monochromatic B), 75%B (75%B + 25% R), 50%B (50%B+ 50%R), 25%B (25%B +75%R), 0% B (monochromatic R) as well as white (W), with 150 ± 10 μmol m−2 s−1 PPFD.
In addition, the correlation between CsUGT709G1 expression with relation to picrocrocin and safranal content was calculated (Figure 5). Results showed a positive correlation between CsUGT709G1 expression and picrocrocin content (R2 = 0.85) and also between CsUGT709G1 expression and safranal content (R2 = 0.71). Accordingly, this shows that expression CsUGT709G1 is relevant for predicting the production of safranal. The carotenoid content and picrocrocin were also highly correlated (R2 = 0.78); While parameters indicating photosynthetic functionality (i.e., Fv/Fm and PIABS) were negatively correlated with safranal, there was no correlation between these photosynthetic parameters and picrocrocin.
Discussion
Light as the energy source is among the most critical environmental factors affecting plant growth and yield. It also plays a signaling role, regulating physiological processes such as photomorphogenesis and the production of secondary metabolites. Among the photosynthetically-active light spectra (i.e., 380–720 nm), B and R lights are exquisitely absorbed by plants leading to the high photosynthesis efficiency of these spectra (Kozai et al., 2016; Zhong et al., 2021). Taking this into account, this study investigated the different ratios of B to R together with W light as a control on saffron flower induction, morphological properties, and secondary metabolites production.
Blue light improves harvestable floral parts of saffron
The present study results indicate the positive effect of a high B to R light ratio on all morphological properties of flower organs. The highest flower number, FW, DW, and earliest flower emergence were recorded in monochromatic B light-grown plants. This positive effect of B light on flowering may be transduced by B light receptor cryptochromes (CRY) on plants' flowering. It has been reported in previous studies that CRY initiated the flowering by controlling the CRY2/COP1 complex (Fankhauser and Ulm, 2011; Jeong et al., 2014; Lopez et al., 2020). CONSTANS (CO) is a zinc-finger transcription factor that triggers plants to flower. The coat protein, COP1, influences the dehydration of CO. Therefore, B light increases the CO accumulation by controlling the CRY2/COP1 complex and provokes flowering in plants (Fankhauser and Ulm, 2011; Jeong et al., 2014). It has also been reported that R light decreases plants flowering via R light photoreceptor phytochrome B (PHYB) that increases CO dehydration (Yu et al., 2010). Another possibility is that B light increases plants' flowering via CRY1 that antagonistically affects PHYB, thereby decreasing CO dehydration (Yu et al., 2010). It was also suggested that promoting flower initiation under B light was observed in the low activity of CRY1 and high CRY2 in Arabidopsis plants (Kong and Zheng, 2022). The present study results are consistent with those reporting the highest flower number in B light-grown plants (Yu et al., 2010; Fankhauser and Ulm, 2011; Jeong et al., 2014; Lopez et al., 2020). It was suggested to measure the effect of light spectra on the expression of genes related to CO and CRY1 to understand how light affects flowering by modulating their transcript level.
Pigments content increased under a high ratio of B to R light
Carotenoids are essential pigments that play an important role in photosynthesis (Thoma et al., 2020) and have antioxidant properties protecting cells from harmful radicals (Felemban et al., 2019). Since animal cells cannot produce carotenoids, plants are the only source of carotenoids for human health, highlighting the importance of carotenoid-rich crops (Thoma et al., 2020). The highest content of carotenoids was detected in plants grown under B light. A higher concentration of carotenoids under the high B to R light ratio was also reported in lettuce, basil, spinach, kale, and pepper plants (Hosseini et al., 2019; Naznin et al., 2019). It has been reported that monochromatic B light promotes the biosynthesis and accumulation of carotenoids in watermelon seedlings (Moosavi-Nezhad et al., 2021) and carnation (Aalifar et al., 2020a). Zhong et al. (2021) suggested that the positive effect of B light on carotenoid concentration in common duckweed plants may be due to the role of B light on carotenoid biosynthesis according to Kyoto Encyclopedia of Genes and Genomes(KEGG) pathway enrichment analysis (Zhong et al., 2021).
Anthocyanins help the reproduction of plants by attracting pollinators and protecting plants in different ways from biotic and abiotic stress (Thoma et al., 2020). It has been suggested that anthocyanin accumulation is affected by light receptors such as UV-B photoreceptor, UVR8, and B light photoreceptor, CRY (Coffey et al., 2017; Meng et al., 2019; Thoma et al., 2020). The current study shows the positive effect of B light on anthocyanin accumulation in saffron flowers, taking into account that the least amount of this pigment was recorded in flowers of plants grown under 0%B light. The maximum anthocyanin concentration in lettuce was also reported in the treatment with the highest daily integral of B light (Nicole et al., 2016; Meng et al., 2020). Similarly, the positive effect of monochromatic B light was observed on the accumulation of phenolic compounds and total anthocyanins in basil plants (Vaštakaite et al., 2018). Also, another study reported the most negligible anthocyanin in 0%B light treatment, which is in agreements with the results of the present study (Lobiuc et al., 2017).
Flavonoids, mainly known as flower pigments, have antioxidant properties by scavenging free radicals and increasing tolerance toward biotic and abiotic stresses. Flavonoids are also UV filters, protecting the plants in UV-rich environments such as crop production under supplemental UV-B or artificial B lights (Thoma et al., 2020; Chen et al., 2021). The maximum value of flavonoids was reported in lettuce plants grown under a 1:1 ratio of B and R light, and the lowest content was observed in W light treatment (Długosz-Grochowska et al., 2016). Another study suggested that flavonoid accumulation in leafy greens occurred in the near-UV spectra. The maximum content was reported in monochromatic B light, and the lowest was recorded in 0% B light. They also indicated that light spectra differentially affect the accumulation of flavonoids in various species (Thoma et al., 2020). A positive effect of B light on increasing flavonol index was also reported in kale (Brazaityte et al., 2021). Similarly, the results of the current study shows that the maximum concentration of flavonoids is obtained in plants that were grown under a high proportion of B light (i.e., 100%B and 75%B) and the lowest content of flavonoids is produced in the W light-grown plants.
Saffron secondary metabolites increased under monochromatic B and R lights
The HPLC-DAD analysis showed that while increasing the ratio of B light led to the highest content of trans-CE, a high concentration of safranal was detected in plants grown under the low B:R ratio (e.g., monochromatic R light). Interestingly, a high amount of picrocrocin was recorded in both monochromatic B and R light-grown plants. All of these three metabolites are members of apocarotenoids family (García-Rodríguez et al., 2021). The production and accumulation of apocarotenoids were species-dependent and regulated by environmental factors (Ahrazem et al., 2016). A large body of research has focused on the effects of environmental factors on the production of apocarotenoids. For example, it was reported that plants' apocarotenoid production is temperature-dependent (Ahrazem et al., 2016, 2019). Some studies suggested that crocin production in saffron is regulated by cold in autumn and dark conditions (Ahrazem et al., 2016). However, there are few studies on the effect of light on saffron secondary metabolites. In a study on the effect of the R:FR ratio on crocin production, a positive effect of FR was reported (Kajikawa et al., 2018). In the current study, a positive effect of B light was detected in crocin production in saffron plants. These apocarotenoids are generally derived from carotenoids via oxidative cleavage enzyme (Felemban et al., 2019). Light quality also affects other members of the apocarotenoid family. The positive role of B light on increasing the production and accumulation of another member of the apocarotenoid family, i.e., strigolactone, was reported in Chrysanthemum (Dierck et al., 2017). The production of abscisic acid (ABA), an important member of the apocarotenoids family, is also regulated by the R:FR ratio (Dierck et al., 2017; Thoma et al., 2020). Also, the maximum content of carotenoids was reported in lettuce and watermelon grown under B light (Thoma et al., 2020; Moosavi-Nezhad et al., 2021). The positive effect of monochromic R and B light on the carotenoid accumulation in vegetables has also been widely reported (Bian et al., 2015; Moradi et al., 2021).
Light spectra-regulated gene expression of secondary metabolites
To find the molecular basis of the effect of different B to R light ratios on the production of secondary metabolites, the relative expression of four critical genes in the crocin, picrocrocin, and safranal biosynthesis pathways were investigated. The highest expression of the CsCCD2 gene was recorded in B light-grown plants. It has been suggested that the molecular level of the carotenoids was regulated by environmental signals, especially light signals, by increasing the transcription of genes that are important in the carotenoid biosynthesis pathway (Fuentes et al., 2012). The CCD genes family are crucial genes in carotenoid and apocarotenoid biosynthesis (Zhou et al., 2019). It was suggested that NtCCDs of tobacco plants were upregulated in response to hormone treatments and abiotic stresses such as high light stress (Zhou et al., 2019).
The differential expression of CCD genes under UV radiation in achiote plants (Bixa orellana) was also reported, showing that the expression of CCD was upregulated in UV-C compared to UV-B radiation (Sankari et al., 2017). These results show the effect of light on regulating CCD gene expression. The results of the present study are consistent with the previous ones and show the effect of light quality on CCD gene expression.
The ALDH gene is also crucial in the apocarotenoid pathway, mainly in crocin biosynthesis (Ahrazem et al., 2016; López et al., 2021). Significant upregulation was observed in the CsALDH31l gene expression under B light treatment in the current study. Furthermore, it has been reported that the expression of ALDH was upregulated under UV-B radiation in achiote plants (Sankari et al., 2017).
Correlation analysis to gain higher perspective in the results
In addition to the investigation of the effects of B to R light ratio on gene expression, the correlation between gene expression, secondary metabolites, carotenoid content, and photosynthetic parameters was analyzed. The results showed a high correlation between trans-CE content and the expression of CsCCD2, CsALDH31l, and CsUGT2, so their relation to crocin content suggests that they can be used as central genes for detecting crocin production under different conditions. Analyzing the expression of CsCCD2, CsALDH31l, and CsUGT2 genes was also previously suggested for detecting crocin production (Ahrazem et al., 2016, 2019; Sharma et al., 2018; Diretto et al., 2019). Furthermore, the high correlation between carotenoid content in flowers and trans-CE indicated the importance of carotenoid pigment in improving the antioxidant value of plants. The result is in agreement with previous reports about the positive effect of carotenoids on protecting plants from various stresses (Felemban et al., 2019; Aalifar et al., 2020b). Also, a high correlation was found among the expression of the CsUGT709G1 gene, picrocrocin, and safranal content. These results make the expression of this gene (CsUGT709G1) a potential candidate for the prediction of picrocrocin and safranal production. These results are in agreement with the previous report, suggesting that this gene plays a crucial role in picrocrocin and safranal biosynthesis (Diretto et al., 2019).
The correlation analysis also showed a high negative correlation between safranal and the photosynthetic parameters such as Fv/Fm and PIABS. Both PIABS and Fv/Fm are essential parameters that represent PSII functionality. These parameters are susceptible to abiotic stress and can be used for evaluating photosynthesis performance (Mathur et al., 2013; Bayat et al., 2018; Estaji et al., 2019). In our previous report, the highest value of PIABS was found in saffron plants grown under monochromatic B light, and the lowest value of PIABS was detected under the low proportions of B light (Moradi et al., 2021). Correspondingly, another report suggested that the production and accumulation of some apocarotenoids increased under stress conditions (Felemban et al., 2019). Therefore, the increasing amount of safranal can be expected under high ratios of R light and a corresponding decreasing value in the photosynthetic parameters (Fv/Fm, PIABS).
Conclusion
The positive effect of B light on flower number per corm, flower and stigma FW and DW was detected for saffron. The highest content of crocin and trans-CE was recorded in B light-grown plants. A high content of picrocrocin was recorded in both monochromatic B and R light. However, a high amount of safranal was only observed in plants grown under a high ratio of R light. A high correlation between the content of secondary metabolites and target genes in the secondary metabolites pathway was observed in all light treatments. The expressions of CsCCD2, CsALDH31l, and CsUGT2 in the crocin pathway were upregulated under B light. In contrast, the expression of the CsUGT709G1 gene in the picrocrocin and safranal pathway was upregulated under the high ratio of R light treatments. Petals of saffron have received attention in recent years due to the high content of antioxidant compounds, and our results showed a positive effect of a high proportion of B light on these compounds. B light improves the quality of saffron in stigma tissue and other by-products of saffron plants.
Data availability statement
The original contributions presented in the study are included in the article/supplementary material, further inquiries can be directed to the corresponding author/s.
Author contributions
Investigation, data curation, formal analysis: SM. Visualization, writing—original draft: SM and MM-N. Methodology, supervision, and project administration: MK and SA. Funding acquisition: MK, SA, and NG. Conceptualization: SS, MK, and SA. Validation: MK, SA, CP, SS, and NG. Writing—review and editing: MM-N, MK, SA, SS, CP, and NG. All authors have read and agreed to the published version of the manuscript.
Acknowledgments
We thank Iran's National Science Foundation and the University of Tehran for their financial support. The valuable work of Saeid Eilkhani during the construction and installation of growth chambers and hydroponic irrigation systems is greatly acknowledged.
Conflict of interest
The authors declare that the research was conducted in the absence of any commercial or financial relationships that could be construed as a potential conflict of interest.
Publisher's note
All claims expressed in this article are solely those of the authors and do not necessarily represent those of their affiliated organizations, or those of the publisher, the editors and the reviewers. Any product that may be evaluated in this article, or claim that may be made by its manufacturer, is not guaranteed or endorsed by the publisher.
References
Aalifar, M., Aliniaeifard, S., Arab, M., Mehrjerdi, M. Z., Serek, M. (2020a). Blue light postpones senescence of carnation flowers through regulation of ethylene and abscisic acid pathway-related genes. Plant Physiol. Biochem. 151, 103–112. doi: 10.1016/j.plaphy.2020.03.018
Aalifar, M., Aliniaeifard, S., Arab, M., Zare Mehrjerdi, M., Dianati Daylami, S., Serek, M., et al. (2020b). Blue light improves vase life of carnation cut flowers through its effect on the antioxidant defense system. Front. Plant Sci. 11, 511. doi: 10.3389/FPLS.2020.00511
Ahrazem, O., Argandoña, J., Fiore, A., Rujas, A., Rubio-Moraga, Á., Castillo, R., et al. (2019). Multi-species transcriptome analyses for the regulation of crocins biosynthesis in Crocus. BMC Genom. 20, 1–15. doi: 10.1186/S12864-019-5666-5/FIGURES/5
Ahrazem, O., Rubio-Moraga, A., Argandoña-Picazo, J., Castillo, R., Gómez-Gómez, L. (2016). Intron retention and rhythmic diel pattern regulation of carotenoid cleavage dioxygenase 2 during crocetin biosynthesis in saffron. Plant Mol. Biol. 91, 355–374. doi: 10.1007/S11103-016-0473-8
Bayat, L., Arab, M., Aliniaeifard, S., Seif, M., Lastochkina, O., Li, T. (2018). Effects of growth under different light spectra on the subsequent high light tolerance in rose plants. AoB Plants 10, ply052. doi: 10.1093/AOBPLA/PLY052
Bian, Z. H., Yang, Q. C., Liu, W. K. (2015). Effects of light quality on the accumulation of phytochemicals in vegetables produced in controlled environments: a review. J. Sci. Food Agric. 95, 869–877. doi: 10.1002/JSFA.6789
Brazaityte, A., Miliauskiene, J., Vaštakaite-Kairiene, V., Sutuliene, R., LauŽike, K., Duchovskis, P., et al. (2021). Effect of different ratios of blue and red LED light on brassicaceae microgreens under a controlled environment. Plants 10, 801. doi: 10.3390/PLANTS10040801
Chen, Y., Fanourakis, D., Tsaniklidis, G., Aliniaeifard, S., Yang, Q., Li, T. (2021). Low UVA intensity during cultivation improves the lettuce shelf-life, an effect that is not sustained at higher intensity. Postharvest Biol. Technol. 172, 111376. doi: 10.1016/J.POSTHARVBIO.2020.111376
Coffey, A., Prinsen, E., Jansen, M. A. K., Conway, J. (2017). The UVB photoreceptor UVR8 mediates accumulation of UV-absorbing pigments, but not changes in plant morphology, under outdoor conditions. Plant Cell Environ. 40, 2250–2260. doi: 10.1111/PCE.13025
Dierck, R., Dhooghe, E., van Huylenbroeck, J., van der Straeten, D., de Keyser, E. (2017). Light quality regulates plant architecture in different genotypes of Chrysanthemum morifolium Ramat. Sci. Horticult. 218, 177–186. doi: 10.1016/J.SCIENTA.2017.02.016
Diretto, G., Ahrazem, O., Rubio-Moraga, Á., Fiore, A., Sevi, F., Argandoña, J., et al. (2019). UGT709G1: a novel uridine diphosphate glycosyltransferase involved in the biosynthesis of picrocrocin, the precursor of safranal in saffron (Crocus sativus). New Phytol. 224, 725–740. doi: 10.1111/NPH.16079
Długosz-Grochowska, O., Kołton, A., Wojciechowska, R. (2016). Modifying folate and polyphenol concentrations in Lamb's lettuce by the use of LED supplemental lighting during cultivation in greenhouses. J. Funct. Foods 26, 228–237. doi: 10.1016/J.JFF.2016.07.020
Estaji, A., Kalaji, H. M., Karimi, H. R., Roosta, H. R., Moosavi-Nezhad, S. M. (2019). How glycine betaine induces tolerance of cucumber plants to salinity stress? Photosynthetica 57, 753–761. doi: 10.32615/ps.2019.053
Fankhauser, C., Ulm, R. (2011). Light-regulated interactions with SPA proteins underlie cryptochrome-mediated gene expression. Genes Dev. 25, 1004. doi: 10.1101/GAD.2053911
Felemban, A., Braguy, J., Zurbriggen, M. D., Al-Babili, S. (2019). Apocarotenoids involved in plant development and stress response. Front. Plant Sci. 10, 1168. doi: 10.3389/FPLS.2019.01168/BIBTEX
Fuentes, P., Pizarro, L., Moreno, J. C., Handford, M., Rodriguez-Concepcion, M., Stange, C. (2012). Light-dependent changes in plastid differentiation influence carotenoid gene expression and accumulation in carrot roots. Plant Mol. Biol. 79, 47–59. doi: 10.1007/S11103-012-9893-2
García-Rodríguez, M., Serrano-Díaz, J., Tarantilis, P. A., López-Córcoles, H., Carmona, M., Alonso, G. L. (2014). Determination of saffron quality by high-performance liquid chromatography. J. Agric. Food Chem. 62, 8068–8074. doi: 10.1021/JF5019356
García-Rodríguez, M. V., Moratalla-López, N., López-Córcoles, H., Alonso, G. L. (2021). Saffron quality obtained under different forcing condition, considering varios vegetative stages of corms. Sci. Horticult. 277, 109811. doi: 10.1016/J.SCIENTA.2020.109811
Hoagland, D. R., Arnon, D. I. (1938). The Water-Culture Method for Growing Plants Without Soil. University of California, California Agricultural Experiment Station, 347, 32. Available online at: https://www.cabdirect.org/cabdirect/abstract/19500302257 (accessed June 20, 2021).
Hosseini, A., Razavi, B. M., Hosseinzadeh, H. (2018). Saffron (Crocus sativus) petal as a new pharmacological target: a review. Iran. J. Basic Med. Sci. 21, 1091. doi: 10.22038/IJBMS.2018.31243.7529
Hosseini, A., Zare Mehrjerdi, M., Aliniaeifard, S., Seif, M. (2019). Photosynthetic and growth responses of green and purple basil plants under different spectral compositions. Physiol. Mol. Biol. Plants 25, 741–752. doi: 10.1007/s12298-019-00647-7
ISO 3632-2:2010(en) (2022). Spices—Saffron (Crocus sativus L.)—Part 2: Test methods. Available online at: https://www.iso.org/obp/ui/#iso:std:iso:3632:-2:ed-2:v1:en (accessed January 9, 2022).
Jeong, S. W., Hogewoning, S. W., van Ieperen, W. (2014). Responses of supplemental blue light on flowering and stem extension growth of cut chrysanthemum. Sci. Horticult. 165, 69–74. doi: 10.1016/J.SCIENTA.2013.11.006
Kajikawa, N., Uno, Y., Kuroki, S., Miyagawa, S., Yamashita, Y., Hamaguchi, Y., et al. (2018). Effect of far-red light on saffron (Crocus sativus L.) growth and crocin yields. Environ. Control Biol. 56, 51–57. doi: 10.2525/ecb.56.51
Kong, Y., Zheng, Y. (2022). Low-activity cryptochrome 1 plays a role in promoting stem elongation and flower initiation of mature Arabidopsis under blue light associated with low phytochrome activity. Can. J. Plant Sci. 2022, 1–5. doi: 10.1139/CJPS-2021-0122
Kozai, T., Fujiwara, K., Runkle, E. S. (2016). LED Lighting for Urban Agriculture. Singapore: Springer, 1–454. doi: 10.1007/978-981-10-1848-0
Livak, K. J., Schmittgen, T. D. (2001). Analysis of relative gene expression data using real-time quantitative PCR and the 2(-Delta Delta C(T)) method. Methods 25, 402–408. doi: 10.1006/METH.2001.1262
Lobiuc, A., Vasilache, V., Pintilie, O., Stoleru, T., Burducea, M., Oroian, M., et al. (2017). Blue and red LED illumination improves growth and bioactive compounds contents in acyanic and cyanic Ocimum basilicum L. Microgreens. Mol. 22, 2111. doi: 10.3390/MOLECULES22122111
López, A. J., Frusciante, S., Niza, E., Ahrazem, O., Rubio-Moraga, Á., Diretto, G., et al. (2021). A new glycosyltransferase enzyme from family 91, UGT91P3, is responsible for the final glucosylation step of crocins in saffron (Crocus sativus L.). Int. J. Mol. Sci. 22, 8815. doi: 10.3390/IJMS22168815
Lopez, R. G., Meng, Q., Runkle, E. S. (2020). Blue radiation signals and saturates photoperiodic flowering of several long-day plants at crop-specific photon flux densities. Sci. Horticult. 271, 109470. doi: 10.1016/J.SCIENTA.2020.109470
Mathur, S., Mehta, P., Jajoo, A. (2013). Effects of dual stress (high salt and high temperature) on the photochemical efficiency of wheat leaves (Triticum aestivum). Physiol. Mol. Biol. Plants 19, 179–188. doi: 10.1007/s12298-012-0151-5
Meng, Q., Boldt, J., Runkle, E. S. (2020). Blue radiation interacts with green radiation to influence growth and predominantly controls quality attributes of lettuce. J. Am. Soc. Horticult. Sci. 145, 75–87. doi: 10.21273/JASHS04759-19
Meng, Q., Kelly, N., Runkle, E. S. (2019). Substituting green or far-red radiation for blue radiation induces shade avoidance and promotes growth in lettuce and kale. Environ. Exp. Bot. 162, 383–391. doi: 10.1016/J.ENVEXPBOT.2019.03.016
Mir, J. I., Ahmed, N., Wani, S. H., Rashid, R., Mir, H., Sheikh, M. A. (2010). In vitro development of microcorms and stigma like structures in saffron (Crocus sativus L.). Physiol. Mol. Biol. Plants 16, 369–373. doi: 10.1007/S12298-010-0044-4
Miyagawa, S., Itoh, H., Uno, Y., Kuroki, S., Miyaji, D., Kitaaji, H., et al. (2015). Effect of plant shoot number and air temperature on saffron (Crocus sativus L.) corm production and total crocin content. Shokubutsu Kankyo Kogaku 27, 204–212. doi: 10.2525/shita.27.204
Molina, R., Renau-Morata, B., Nebauer, S. G., García-Luis, A., Guardiola, J. L. (2010). Greenhouse saffron culture—temperature effects on flower emergence and vegetative growth of the plants. Acta Horticult. 850, 91–94. doi: 10.17660/actahortic.2010.850.12
Molina, R., Valero, M., Navarro, Y., García-Luis, A., Guardiola, J. L. (2004). The effect of time of corm lifting and duration of incubation at inductive temperature on flowering in the saffron plant (Crocus sativus L.). Sci. Horticult. 103, 79–91. doi: 10.1016/j.scienta.2004.04.008
Molina, R., Valero, M., Navarro, Y., García-Luis, A., Guardiola, J. L. (2005a). Low temperature storage of corms extends the flowering season of saffron (Crocus sativus L.). J. Horticult. Sci. Biotechnol. 80, 319–326. doi: 10.1080/14620316.2005.11511937
Molina, R., Valero, M., Navarro, Y., Guardiola, J. L., García-Luis, A. (2005b). Temperature effects on flower formation in saffron (Crocus sativus L.). Sci. Horticult. 103, 361–379. doi: 10.1016/j.scienta.2004.06.005
Moosavi-Nezhad, M., Salehi, R., Aliniaeifard, S., Tsaniklidis, G., Woltering, E. J., Fanourakis, D., et al. (2021). Blue light improves photosynthetic performance during healing and acclimatization of grafted watermelon seedlings. Int. J. Mol. Sci. 22, 8043. doi: 10.3390/IJMS22158043
Moradi, S., Kafi, M., Aliniaeifard, S., Salami, S. A., Shokrpour, M., Pedersen, C., et al. (2021). Blue light improves photosynthetic performance and biomass partitioning toward harvestable organs in saffron (Crocus sativus L.). Cells 10, 1994. doi: 10.3390/CELLS10081994
Moratalla-López, N., Bagur, M. J., Lorenzo, C., Martínez-Navarro, M. E., Rosario Salinas, M., Alonso, G. L. (2019). Bioactivity and bioavailability of the major metabolites of Crocus sativus L. flower. Molecules. 24, 2827. doi: 10.3390/MOLECULES24152827
Mzabri, I., Addi, M., Berrichi, A. (2019). Traditional and modern uses of saffron (Crocus sativus). Cosmetics. 6, 63. doi: 10.3390/COSMETICS6040063
Naznin, M. T., Lefsrud, M., Gravel, V., Azad, M. O. K. (2019). Blue light added with red LEDs enhance growth characteristics, pigments content, and antioxidant capacity in lettuce, Spinach, Kale, Basil, and sweet pepper in a controlled environment. Plants 8, 93. doi: 10.3390/plants8040093
Nicole, C. C. S., Charalambous, F., Martinakos, S., van de Voort, S., Li, Z., Verhoog, M., et al. (2016). Lettuce growth and quality optimization in a plant factory. Acta Horticult. 1134, 231–238. doi: 10.17660/ACTAHORTIC.2016.1134.31
Ouzounis, T., Fretté, X., Rosenqvist, E., Ottosen, C. O. (2014). Spectral effects of supplementary lighting on the secondary metabolites in roses, chrysanthemums, and campanulas. J. Plant Physiol. 171, 1491–1499. doi: 10.1016/j.jplph.2014.06.012
Poggi, L. M., Portela, J. A., Pontin, M. A., Moratalla-López, N., Alonso, G. L. (2017). New proposal for handling of saffron flowering (Crocus sativus L.) under environmentally controlled conditions. Acta Horticult. 1184, 245–252. doi: 10.17660/ActaHortic.2017.1184.34
Renau-Morata, B., Nebauer, S. G., Sánchez, M., Molina, R.v. (2012). Effect of corm size, water stress and cultivation conditions on photosynthesis and biomass partitioning during the vegetative growth of saffron (Crocus sativus L.). Indus. Crops Prod. 39, 40–46. doi: 10.1016/j.indcrop.2012.02.009
Rezaee, R., Hosseinzadeh, H. (2013). Safranal: from an aromatic natural product to a rewarding pharmacological agent. Iran. J. Basic Med. Sci. 16, 12–26. doi: 10.22038/IJBMS.2013.244
Roshanravan, N., Ghaffari, S. (2021). The therapeutic potential of Crocus sativus Linn.: a comprehensive narrative review of clinical trials. Phytotherapy Res. 36, 98–111. doi: 10.1002/PTR.7286
Sankari, M., Hridya, H., Sneha, P., George Priya Doss, C., Ramamoorthy, S. (2017). Effect of UV radiation and its implications on carotenoid pathway in Bixa orellana L. J. Photochem. Photobiol. B 176, 136–144. doi: 10.1016/J.JPHOTOBIOL.2017.10.002
Serrano-Díaz, J., Sánchez, A. M., Maggi, L., Martínez-Tomé, M., García-Diz, L., Murcia, M. A., et al. (2012). Increasing the applications of Crocus sativus flowers as natural antioxidants. J. Food Sci. 77, C1162–C1168. doi: 10.1111/J.1750-3841.2012.02926.X
Sharma, M., Kaul, S., Dhar, M. K. (2018). Transcript profiling of carotenoid/apocarotenoid biosynthesis genes during corm development of saffron (Crocus sativus L.). Protoplasma 256, 249–260. doi: 10.1007/S00709-018-1296-Z
Tahri, K., Tiebe, C., Bougrini, M., Saidi, T., el Alami El Hassani, N., el Bari, N., et al. (2015). Characterization and discrimination of saffron by multisensory systems, SPME-GC-MS and UV-Vis spectrophotometry. Anal. Methods 7, 10328–10338. doi: 10.1039/C5AY01986A
Thoma, F., Somborn-Schulz, A., Schlehuber, D., Keuter, V., Deerberg, G. (2020). Effects of light on secondary metabolites in selected leafy greens: a review. Front. Plant Sci. 11, 497. doi: 10.3389/FPLS.2020.00497/BIBTEX
Untergasser, A., Cutcutache, I., Koressaar, T., Ye, J., Faircloth, B. C., Remm, M., et al. (2012). Primer3—new capabilities and interfaces. Nucleic Acids Res. 40, e115. doi: 10.1093/NAR/GKS596
Vaštakaite, V., Viršile, A., Brazaityte, A., Samuoliene, G., Miliauskiene, J., Jankauskiene, J., et al. (2018). Pulsed LED light increases the phytochemical level of basil microgreens. Acta Horticult. 1227, 579–584. doi: 10.17660/ACTAHORTIC.2018.1227.73
Wang, G., Zhang, X., Wang, F., Song, R. (2012). Isolation of high quality RNA from cereal seeds containing high levels of starch. Phytochem. Anal. 23, 159–163. doi: 10.1002/PCA.1337
Yu, X., Liu, H., Klejnot, J., Lin, C. (2010). The cryptochrome blue light receptors. Arabidopsis Book 8, e0135. doi: 10.1199/tab.0135
Zhong, Y., Wang, L., Ma, Z., Du, X. (2021). Physiological responses and transcriptome analysis of Spirodela polyrhiza under red, blue, and white light. Planta 255, 1–15. doi: 10.1007/S00425-021-03764-4
Keywords: light spectrum, secondary metabolites, controlled-environment agriculture (CEA), antioxidant pigments, gene expression
Citation: Moradi S, Kafi M, Aliniaeifard S, Moosavi-Nezhad M, Pedersen C, Gruda NS and Salami SA (2022) Monochromatic blue light enhances crocin and picrocrocin content by upregulating the expression of underlying biosynthetic pathway genes in saffron (Crocus sativus L.). Front. Hortic. 1:960423. doi: 10.3389/fhort.2022.960423
Received: 02 June 2022; Accepted: 18 July 2022;
Published: 18 August 2022.
Edited by:
Manuel Jamilena, University of Almeria, SpainReviewed by:
Alberto López, University of Castilla-La Mancha, SpainElena Rakosy-Tican, Babeş-Bolyai University, Romania
Copyright © 2022 Moradi, Kafi, Aliniaeifard, Moosavi-Nezhad, Pedersen, Gruda and Salami. This is an open-access article distributed under the terms of the Creative Commons Attribution License (CC BY). The use, distribution or reproduction in other forums is permitted, provided the original author(s) and the copyright owner(s) are credited and that the original publication in this journal is cited, in accordance with accepted academic practice. No use, distribution or reproduction is permitted which does not comply with these terms.
*Correspondence: Mohsen Kafi, bWthZmkmI3gwMDA0MDt1dC5hYy5pcg==; Sasan Aliniaeifard, YWxpbmlhZWlmYXJkJiN4MDAwNDA7dXQuYWMuaXI=