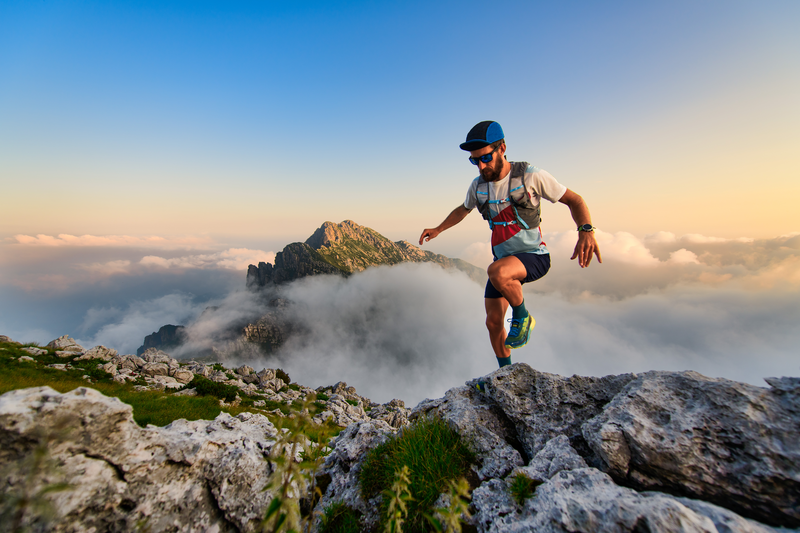
94% of researchers rate our articles as excellent or good
Learn more about the work of our research integrity team to safeguard the quality of each article we publish.
Find out more
ORIGINAL RESEARCH article
Front. Genet.
Sec. Genetics of Common and Rare Diseases
Volume 16 - 2025 | doi: 10.3389/fgene.2025.1522406
The final, formatted version of the article will be published soon.
You have multiple emails registered with Frontiers:
Please enter your email address:
If you already have an account, please login
You don't have a Frontiers account ? You can register here
As the development of molecular diagnostic methods, a large number of clinically relevant or disease-related copy number variations (CNVs) could be detected, and the demand for genetic counselling and clinical treatment is also increasing. For patients with pathogenic or likely pathogenic CNVs, preimplantation genetic testing (PGT) could provide a feasible path to prevent the inheritance of the genetic disorder in the offspring. In this study, we included a couple with 1q21.1 recurrent microduplication to conduct molecular diagnosis and PGT clinical application. The optical genome mapping (OGM) successfully verified the orientation and location of the microduplication, which further proved OGM as a promising approach for chromosomal anomalies detection with high resolutions. In PGT application, linkage-analysis-based PGT and high resolution PGT-A were simultaneously conducted for the pedigree and all the embryos. The results were consistent between linkage analysis and high resolution aneuploid analysis in the targeted region. One embryo that was absent of paternal 1q21.1q21.2 duplication was selected for further transplantation. This successful clinical practice in this study shed light for future molecular diagnosis and PGT application in tandem microduplications.
Keywords: PGT application for 1q21.1 recurrent microduplication Preimplantation Genetic Testing, Microduplication, next generation sequencing, Optical genome mapping, Marsala
Received: 04 Nov 2024; Accepted: 17 Feb 2025.
Copyright: © 2025 Peng, Chen, Zhou, Yang, Li, Keqie, Zhao, Wang, Hu, Liu, Ren and Chen. This is an open-access article distributed under the terms of the Creative Commons Attribution License (CC BY). The use, distribution or reproduction in other forums is permitted, provided the original author(s) or licensor are credited and that the original publication in this journal is cited, in accordance with accepted academic practice. No use, distribution or reproduction is permitted which does not comply with these terms.
* Correspondence:
Cuiting Peng, West China Second University Hospital, Sichuan University, Chengdu, China
Xinlian Chen, West China Second University Hospital, Sichuan University, Chengdu, China
Disclaimer: All claims expressed in this article are solely those of the authors and do not necessarily represent those of their affiliated organizations, or those of the publisher, the editors and the reviewers. Any product that may be evaluated in this article or claim that may be made by its manufacturer is not guaranteed or endorsed by the publisher.
Research integrity at Frontiers
Learn more about the work of our research integrity team to safeguard the quality of each article we publish.