- Department of Pharmaceutical Science, College of Pharmacy, Princess Nourah bint Abdulrahman University, Riyadh, Saudi Arabia
Human SULT2B1gene is responsible for expressing SULT2B1a and SULT2B1b enzymes, which are phase II metabolizing enzymes known as pregnenolone and cholesterol sulfotransferase (SULT), respectively. They are expressed in several tissues and contribute to steroids and hydroxysteroids homeostasis. Genetic variation of the SULT2B1 is reported to be associated with various pathological conditions, including autosomal recessive ichthyosis, cardiovascular disease, and different types of cancers. Understanding the pathological impact of SULT2B1 genetic polymorphisms in the human body is crucial to incorporating these findings in evaluating clinical conditions or improving therapeutic efficacy. Therefore, this paper summarized the most relevant reported studies concerning SULT2B1 expression, tissue distribution, substrates, and reported genetic polymorphisms and their mechanisms in enzyme activity and pathological conditions.
1 Introduction
Human cytosolic Sulfotransferases (SULTs) are a family of phase II metabolizing enzymes that play a significant role in regulating several endogenous pathways involving synthesis, inactivation, and excretion of various endogenous compounds, including catecholamines, estrogen, thyroid hormones, bile acids, and hydroxysteroids (Liu and Klaassen, 1996; Falany, 1997; Strott, 2002). Cytosolic SULTs facilitate the transfer of the sulfonate group from a 3′-phosphoadenosine 5′-phosphosulfate (PAPS) molecule to an acceptor molecule containing amine or hydroxy group (Falany and Rohn-Glowacki, 2013). Most resulting metabolites become biologically inactive and eliminated via biliary or urinary excretion (Glatt et al., 2001; Strott, 2002).
Human cytosolic SUTLs contain thirteen enzymes categorized into four gene families named SULT1, SULT2, SULT4, and SULT6 (Allali-Hassani et al., 2007; Frank et al., 2011). Enzymes of the SULT2 family have been reported to exhibit the highest affinity in sulfating steroids and hydroxysteroid substrates (Glatt et al., 2001). In the SULT2 family, three isoforms have been identified: SULT2A1, SULT2B1a, and SULT2B1b, each exhibiting extinct affinity to a specific substrate and expressed in particular tissues, which influence their physiological role and involvement in different diseases (Her et al., 1996; Falany J. L. et al., 2006; Riches et al., 2009). SULT2A1, for instance, has a higher affinity toward dehydroepiandrosterone (DHEA), while SULT2B1a and SULT2B1b toward pregnenolone and cholesterol, respectively (Javitt et al., 2001; Falany and Rohn-Glowacki, 2013).
The role of SULT2B1 enzymes in sulfating endogenous substrates, especially steroids and hydroxysteroids, and their expression in hormonal-responsive tissues influence their roles in regulating the homeostasis of sex hormones (Higashi et al., 2004; Yanai et al., 2004; Falany C. N. et al., 2006; Koizumi et al., 2010; Salman et al., 2011; Yang et al., 2019). Therefore, interindividual variation in the SULT2B1 gene may also impact physiological functions and pathological conditions. Thus, this paper aims to comprehensively review recently reported findings related to SULT2B1 gene expression, tissue distribution, substrate specificity, and the impact of genetic polymorphism in enzyme activity and expression level and their role in pathological conditions.
2 Expression of SULT2b1 isoforms and tissue distribution
Human SULT2B1 cDNA was first cloned and characterized from the placenta, and its localization is mapped to the human chromosome 19 (Her et al., 1998). SULT2B1 gene expression generates two mRNA isoforms, SULT2B1a and SULT2B1b, because of the different first exon used (Ji et al., 2007; Falany and Rohn-Glowacki, 2013). Therefore, the expressed isoenzymes (SULT2B1a and SULT2B1b) differ in the N-terminus region, and that influences their substrate specificity toward pregnenolone and cholesterol, respectively (Fuda et al., 2002; Ji et al., 2007; Falany and Rohn-Glowacki, 2013). The 25-residue of the N-terminus region of SULT2B1b was also identified as a part of allosteric binding site in in silico screening and molecular dynamic studies (Cook and Leyh, 2022). Moreover, compared to all reported SULT enzymes, SULT2B1a and SULT2B1b have unique extended carboxyl terminals rich with proline and serine residues, which were reported to be involved in enzymatic activity, thermostability, and subcellular localization (Lee et al., 2003; He and Falany, 2006).
Human SULT2B1a and SULT2B1b mRNA expression have been detected in several tissues like the prostate, placenta, small intestine, colon, trachea, lung, brain, and skin (Javitt et al., 2001). However, SULT2B1b mRNA is reported to be expressed several folds greater than SULT2B1a and in widespread tissues (Geese and Raftogianis, 2001; Javitt et al., 2001; Higashi et al., 2004; Yanai et al., 2004). Furthermore, only SULT2B1b protein has been detected in immunoblot analysis of human tissues and cell lines such as platelet, lung, skin, placenta, endometrium, and prostate (He et al., 2004; Higashi et al., 2004; Yanai et al., 2004; He et al., 2005; Koizumi et al., 2010; Zimmer et al., 2018). Besides normal tissues, SULT2B1b protein has also been detected in human cancerous tissues and cell lines involving prostate adenocarcinoma (LNCaP) and breast cancer cell lines (MCF-7, T47D) (Falany C. N. et al., 2006; He and Falany, 2007). Thus, most reported literature focuses on SULT2B1b enzyme.
3 SULT2B1 substrate specificity
As mentioned earlier, SULT2B1 enzymes exhibit activity toward hydroxysteroids, including cholesterol, pregnenolone, and DHEA (Javitt et al., 2001). Cholesterol plays crucial roles in the body, such as being the precursor for all steroid hormones, facilitating nutrient transportation, and activating cellular processes (Schade et al., 2020). SULT2B1b converts cholesterol into cholesterol sulfate, which is biologically active by itself (Javitt et al., 2001; Park J. H. et al., 2023). Cholesterol is also involved in the synthesis of pregnenolone, an initial precursor for the synthesis of steroid hormones, including DHEA (Falany and Rohn-Glowacki, 2013). Even though pregnenolone and DHEA are metabolized by several SULTs, with SULT2A1 exhibiting the highest activity, especially toward DHEA, SULT2B1b is reported to play a vital role in sulfating pregnenolone and DHEA due to its selective expression in important tissues like lung, skin, and reproductive tissues where SULT2A1 expression is low or not detectable (He et al., 2004; He et al., 2005). Therefore, it has been suggested that SULT2B1b could be involved in regulating DHEA, which is an essential precursor for reproductive hormones (Falany and Rohn-Glowacki, 2013). Furthermore, DHEA and pregnenolone, as well as their sulfated metabolites, act as neuromodulators, which are produced and released in the nervous system (brain) where SULT2B1b is expressed in higher levels (Vallee et al., 2001; Sanchez-Guijo et al., 2016; Vallee, 2016; Raciti et al., 2023).
SULT2B1b has also been reported to play an essential role in sulfating and regulating cholesterol-oxygenated derivatives (oxysterols), including 5α,6α-epoxycholesterol (5α,6α-EC), 5β,6β-epoxycholesterol (5 β,6 β -EC), 7-ketocholesterol (KC), 24-hydroxycholesterol (24HC), and 25-hydroxycholesterol (25HC) (Fuda et al., 2007; Cook et al., 2009; Ren and Ning, 2014). Oxysterols mediate several biological activities like sterol biosynthesis and act as signaling elements in several cellular processes (Vaya et al., 2011; Luu et al., 2016). Oxysterol sulfation by SUL2B1b may influence their biological activities and elimination (Fuda et al., 2007; Cook et al., 2009). For instance, SULT2B1b is reported to be able to convert KC, which is involved in atherosclerosis and retinal macular degeneration, into a sulfated metabolite, which prevents its cytotoxic effect (Fuda et al., 2007). Another study has also proven that inducing SULT2B1b expression in 293T cells mitigates the cytotoxic effect of KC by generating sulfated metabolite (Fuda et al., 2007; Bai et al., 2011). Furthermore, SULT2B1b was proposed to influence intracellular lipid homeostasis by catalyzing 25HC to its sulfated derivative (25HC-S), which is a main regulator of serum and hepatic lipid metabolism through its effects on the nuclear liver oxysterol receptor (LXR) and sterol regulatory element-binding proteins (SREBPs) (Bai et al., 2011; Bai et al., 2012).
In addition to endogenous substrates, SUlT2B1 enzymes were reported to exhibit minimal sulfating activity toward exogenous substrates and xenobiotics, like raloxifene, 4-n-nonylphenol, 3-OH-tibolone, p-nitrophenol, bisphenol A, 4-n-octylphenol, diethylstilbestrol, and 17-α-ethynylestradiol (Pai et al., 2002; Falany et al., 2004; Falany J. L. et al., 2006). SULT2B1b sulfating activity was also reported to be inhibited by endogenous cholesterol metabolite 7-dehydrocholesterol and antiandrogens like cyproterone, abiraterone, galeterone, and danazol (Yip et al., 2018; Cook and Leyh, 2022).
4 Effect of SULT2B1 genetic variation and enzyme activity
According to the NCBI dbSNP database, a total of 19,975 single-nucleotide polymorphisms (SNPs) have been reported in the human SULT2B1 gene, including 540 missense variants (NCBI, n.d.). Several studies have been conducted to study the effect of genetic variations, especially missense variants since they could lead to changes in the expressed amino acid and influence the function of the expressed enzymes. The first study was conducted on African American and Caucasian subjects and showed that SULT2B1 genetic variations affect the expressed enzyme activities (Ji et al., 2007). The reported variants were eight coding missense variants in both SULT2B1b (Leu51Ser, Asp191Asn, Arg230His, and Peo345Leu) and SULT2B1a (Leu36Ser, Asp176Asn, Arg215His, and Pro330Leu) enzymes were expressed in COS-1 cells, and their activities were evaluated using DHEA as a substrate (Ji et al., 2007). The study showed that compared to the wild-type SULT2B1b and SULT2B1a, the tested allozymes displayed significant differential sulfating activities ranging from 76%–98% and 64%–88%, respectively (Ji et al., 2007). Another study examining the effect of ten missense SULT2B1b coding SNPs using cholesterol as a substrate showed a complete loss of the enzyme activity in three SULT2B1b allozymes (Gly72Val, Arg147His, and Gly276Val), while the rest of the allozymes (Pro69Ala, Thr73Met, Asp191Asn, Arg230His, Ser244Thr, Arg274Gln, and Pro345Leu) showed significant differential activities toward cholesterol between 0.5% and 54.6% of the wildtype activity (Table 1) (Alherz et al., 2018). Similar results were also reported for the same ten allozymes when their activities were evaluated using other endogenous substrates like DHEA and pregnenolone as substrates (Alherz et al., 2019). Furthermore, a study of 12 coding missense variations of SULT2B1a enzyme using pregnenolone as a substrate has reported no detectable activity of SULT2B1a-Arg132His, while the rest of the tested allozymes showed significant differential sulfating activity 0.5%–103.4% of the wildtype activity (Alatwi, 2022).
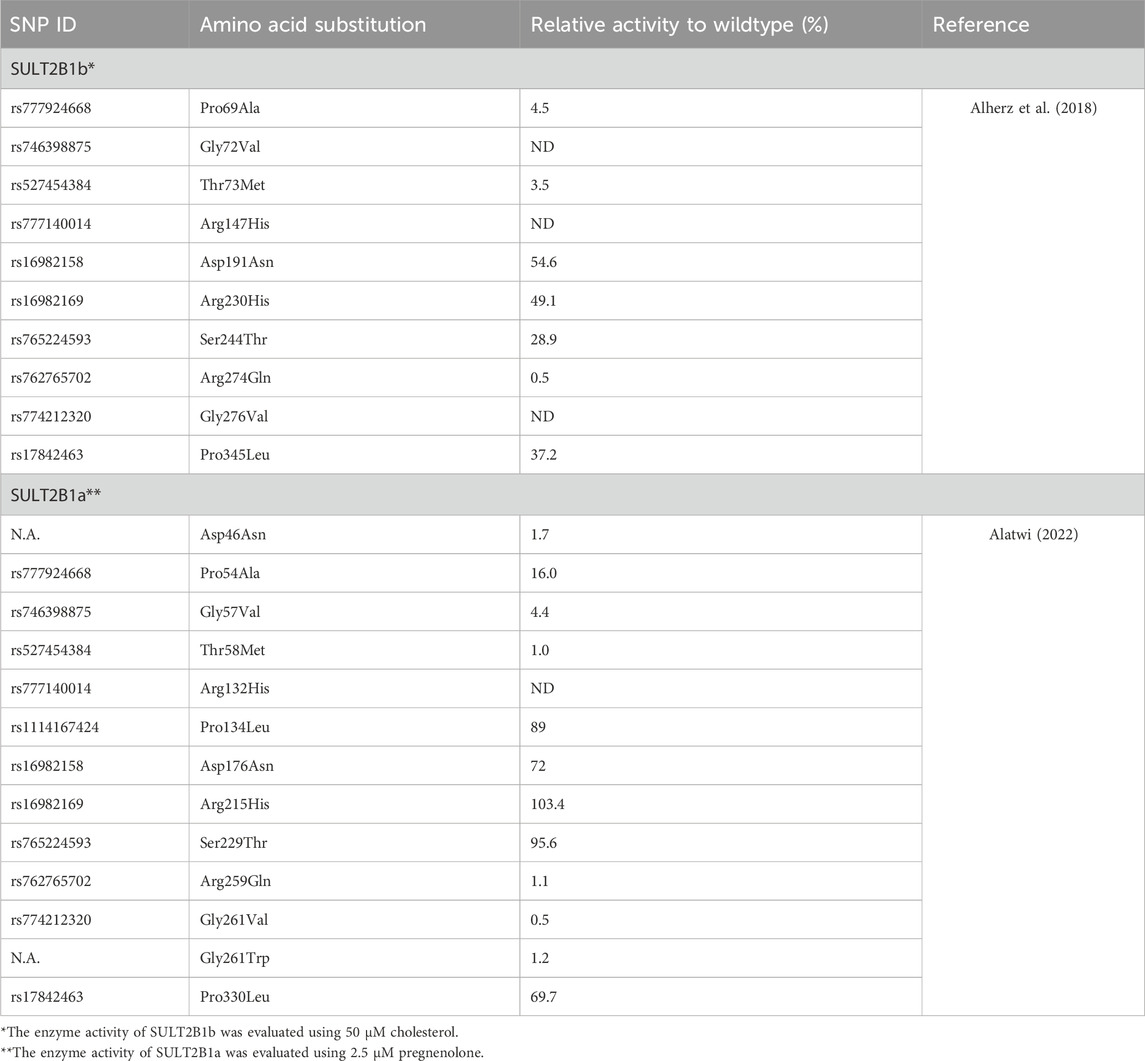
Table 1. Effect of single nucleotide polymorphisms on the enzymatic activity of SULT2B1a and SULT2B1b allozymes.
5 SULT2B1 genetic variations and diseases
As mentioned earlier, SULT2B1 is involved in metabolizing endogenous substrates such as steroids and hydroxysteroids and is highly expressed in hormone-responsive tissues, which suggests its critical role in physiological and pathophysiological conditions (Geese and Raftogianis, 2001; Higashi et al., 2004; Yanai et al., 2004; He et al., 2005; Koizumi et al., 2010; Salman et al., 2011). For instance, numerous studies reported the association of genetic variation or change in the expression of SULT2B1 in various diseases and cancer types (Table 2) (He and Falany, 2007; Seo et al., 2013; Yang et al., 2013; Hu et al., 2015; Chen et al., 2016; Heinz et al., 2017; Youssefian et al., 2019; Fozia et al., 2021).
5.1 Skin-related disorder
Congenital ichthyosis is a skin disorder characterized by dry, scaling skin due to an imbalance in cholesterol sulfation and desulfation in the skin (Strott and Higashi, 2003). Four missense SNPs from exon coding SULT2B1b, p.Glu78Lys, p.Arg100Trp, p.Ala140Val, p.Arg274Gln, and p.Met304Ile were reported to be associated with the pathogenesis of autosomal recessive ichthyosis (Heinz et al., 2017; Youssefian et al., 2019; Fioretti et al., 2020; Fozia et al., 2021). One study reported that the change of glutamic acid to Lysine in SULT2B1bGlu78Lys caused a reduction in the cholesterol sulfating capacity of the enzyme, leading to the imbalance between cholesterol and cholesterol sulfate in the skin (Heinz et al., 2017). On the other hand, in SULT2B1bAla140Val, the change of alanine in location 140 into valine was proposed to disrupt the binding of SULT2B1b with the cofactor PAPS and affects the protein stability, which influences the activity of the enzyme in sulfating cholesterol efficiently (Fozia et al., 2021).
5.2 Cardiovascular disorder
A recent study has reported a possible link between the level of SULT2B1b in lymphocytes and acute myocardial infarction in patients with low levels of low-density lipoprotein (LDL) (Zhang Y. et al., 2020). The analysis of lymphocytes of those patients reveals a high expression level of SULT2B1b mRNA and protein, which is associated with high levels of cholesterol, inflammatory mediators like tumor necrosis factor-alpha (TNFα), and interferon-g (IFN- γ) in lymphocytes (Zhang Y. et al., 2020). Cholesterol accumulation in lymphocytes was reported to be promoted by SULT2B1b inhibition of LXR (Zhang Y. et al., 2020). LXR is activated by oxysterols such as 7 KC and 5 β,6 β -EC, and sulfation of oxysterols by SULT2B1b converts them into sulfated metabolites, which then act as antagonists of LXR (Song et al., 2001; Zhang Y. et al., 2020). Sulfated oxysterols may also play a role in atherosclerosis by promoting de novo cholesterol synthesis and apoptosis in several cell types, including macrophages (Song et al., 2001). Additionally, a recent study has reported that SULT2B1 expression is elevated with the progression of atherosclerosis (Pan et al., 2024). In fact, the knockdown of SULT2B1 in animal models was suggested to promote atherosclerosis remission and reduce inflammatory mediator levels (Pan et al., 2024). The study explains that lowering the expression of SULT2B1 in macrophages leads to a reduction of 25HC-S production, which then increases the expression of LXR, suppressing the activation of macrophages via nuclear factor κB (NF-κB) and attenuating inflammation accordingly (Pan et al., 2024). It was also reported that SULT2B1b knockdown upregulates miR148a-3p and inhibits IκB kinase β (IKKβ)\ NF-κB signaling pathway in macrophage, which reduces inflammation (Yin et al., 2021; Zhang J. et al., 2023). On the other hand, overexpression of SULT2B1 in mice (under high cholesterol diet) inhibits LXR, which reduces the high-density lipoprotein (HDL) levels and, as a consequence, reduces the beneficial effects of HDL in lowering cholesterol levels through activation of reverse cholesterol transport (Nishida et al., 2024). Finally, genotyping of monocytes in coronary artery disease patients has shown that the SULT2B1 genetic variant in the promoter region (rs2665580), especially with the GG genotype, is associated with a high expression level of SULT2B1, which corresponds with increased inflammatory factors and unstable coronary plaques (Pan et al., 2024).
5.3 Cancer-related to reproductive Organs
SULT2B1 intron variants (rs12460535, rs2665582, and rs10426628) were found to be correlated with prostate cancer progression and overall survival rate (Levesque et al., 2014). The study suggested that SULT2B1 (rs10426628) lowers the risk of prostate cancer progression by reducing the circulating steroid hormones via forming less active sulfated metabolites (Levesque et al., 2014). In vivo and in vitro studies demonstrated that inhibiting SULT2B1b enzyme expression in prostate cancer cells could promote prostate cancer proliferation in response to DHEA treatment (He and Falany, 2007; Seo et al., 2013). In fact, data from the Protein Atlas shows that the low expression level of SULT2B1 is significantly (p = 0.044) and inversely correlated with the 5-year survival rate (Yang et al., 2019). These findings were confirmed in a clinical study using isolated human prostate cancer tissue samples, which reported that SULT2B1b enzyme is expressed at a very low level in advanced metastatic prostate cancer compared to normal prostate, suggesting SULT2B1b expression may offer a protective effect by reducing the availability of active steroid hormone precursors like DHEA (He and Falany, 2007; Seo et al., 2013). Furthermore, in castration-resistant prostate cancer, androgen biosynthesis from adrenal DHEA is mediated by the action of aldo-keto reductase (AKR)1C3, which promotes cancer growth and invasion by activating androgen receptors (Park et al., 2020). SULT2B1b depletion was reported to promote AKR1C3 expression, which activates extracellular-signal-regulated kinase 1/2 (ERK1/2) tumor cell survival signal, activates androgen receptors, and induces epithelial-to-mesenchymal (EMT)-like changes that promote cancer progression and invasiveness (Park et al., 2020). Interestingly, another study has reported contradictory results, demonstrating that SULT2B1b knockdown increases TNFα expression in prostate cancer, promoting TNF-mediated apoptosis (Vickman et al., 2019). Similarly, another in vitro study has shown that SULT2B1b knockdown reduces prostate cancer cell growth and viability and promotes cell death (Vickman et al., 2016).
On the other hand, in breast cancer, SULT2B1 expression was reported to be upregulated in both estrogen receptor a (ERa) positive and negative breast cancer tissues, with a higher level in ER-positive tumors (Bieche et al., 2004; Tozlu et al., 2006; Low et al., 2010; Hevir et al., 2011). Similarly, SULT2B1 is also reported to be expressed at a high level in endometrial cancer, cervical cancer, and ovarian cancer, which negatively impacts prognosis (Dumas et al., 2008; Low et al., 2010; Hevir et al., 2011; Zhang Y. et al., 2023; Gao et al., 2024). Downregulation of SULT2B1 in ovarian cancer cell lines reduces cell proliferation, migration, and invasion by binding to annexin A9 (ANXA9) and regulates its expression (Gao et al., 2024). ANXA9 is a calcium-dependent phospholipid-binding protein that has been reported to promote different cancer development and chemotherapy resistance (Boudhraa et al., 2016; Kou et al., 2021; Zhang et al., 2021; Zhou et al., 2021). Furthermore, inhibiting the expression of SULT2B1 with small molecules like verteporfin was reported to inhibit cervical cancer cell proliferation, migration, and invasion and promote cell apoptosis (Yin and Chen, 2020).
5.4 Gastrointestinal cancers
Genetic analysis of esophageal squamous cell carcinoma (ESCC) reveals that the SULT2B1 rs4149455 intronic variant and rs1052131 synonymous variant correlate with reduced cancer risks (Hyland et al., 2013). A clinical study on patient samples of ESCC demonstrated that SULT2B1 expression level is reduced or even abolished in ESCC tissues compared to matched adjacent normal epithelial cells (Li et al., 2021). The study suggested that reduced expression of SULT2B1 upregulates Per1 gene expression, a circadian clock gene that is involved in tumor initiation and malignant progression (Li et al., 2021). Functional analysis proves that SULT2B1 overexpression in vitro reduces tumor cell proliferation and retard tumor growth in vivo, while SULT2B1b knockdown promotes ESCC progression (Yue et al., 2017; Li et al., 2021). Another study proposed different mechanisms, which suggest that SULT2B1enzymes promote the development of ESCC by sulfating DHEA, leading to a reduced pool of sex hormones such as estradiol (Hyland et al., 2013). Estradiol treatment of ESCC cell lines inhibited the viability and migration ability of cancerous cells (Wang et al., 2020). A clinical study has also shown that low estradiol levels increase the incidence of developing ESCC (Wang et al., 2011).
In normal gastric epithelial cells, SULT2B1 expression was reported to promote their repair after damage and play a protective role in preventing gastric carcinogenesis induced by 3-methylcholanthrene (a carcinogenic agent) (Hong et al., 2019). However, elevated expression of SULT2B1b mRNA and protein in gastric cancer tissue was reported to promote tumor angiogenesis, lower the survival rate, and affect prognosis negatively (Chen et al., 2016). The SULT2B1 expression level was also correlated with gastric cancer stage and proposed to be used as an independent biomarker for gastric cancer prognosis (Chen et al., 2016).
In colorectal cancer, SULT2B1b expression was reported to be upregulated, which could promote disease progression and lower disease-specific survival and disease-free rates; thus, it was proposed to be used as a prognostic biomarker and a potential therapeutic target (Hu et al., 2015; Zhang Z. Y. et al., 2020; Tatsuguchi et al., 2022b; Che et al., 2024). In vitro study SULT2B1b knockdown suppresses colorectal cancer cell growth and migration, suggesting the vital role of SULT2B1b in colorectal cancer cell proliferation and invasion (Hu et al., 2015; Che et al., 2024). The study also showed that SULT2B1 facilitates lipid metabolism and promotes colon cancer cell metastasis by interacting directly with stearoyl-CoA desaturase (SCD1), which is involved in lipid metabolism (Che et al., 2024). Furthermore, another study has reported that SULT2B1 is highly expressed in chemoresistance colon cancer and radio-resistance tissues, which promotes cell proliferation and chemoresistance in colon cancer through its involvement in oncogenic signaling involving the OLR1/c-MYC/SULT2B1 axis (Zhao et al., 2021; Huang et al., 2022). Oxidized low-density lipoprotein receptor 1 (OLR1) promotes SULT2B1 expression by increasing c-MYC expression while knockdown the ORL1 downregulating c-MYC expression, which results in reducing SULT2B1 level as well as reduced glycolytic metabolism leading to decreased cancer cell growth and chemoresistance in colon cancer (Zhao et al., 2021; Huang et al., 2022). Furthermore, SULT2B1b was proposed to increase cancer cell resistance to immunotherapy by promoting the production of cholesterol sulfate (Tatsuguchi et al., 2022b). For instance, T cells treated with cholesterol sulfate showed a reduction in the immune response by reducing T-cell receptor signaling through disturbing T-cell microvilli function (Park J. S. et al., 2023). Furthermore, cholesterol sulfate inhibits dedicator of cytokinesis protein 2 (DOCK2), a Rac activator crucial for lymphocyte activation and migration, decreasing CD8+ T cell infiltration into colon cancer tissues (Sakurai et al., 2018; Tatsuguchi et al., 2022b; Morino et al., 2023; Wang et al., 2023). In vivo, inhibition of SULT2B1b with 3b-hydroxy-5-cholenoic acid promotes CD8+ T cell infiltration to cholesterol-sulfate-producing tumor and sensitizes the tumor to immunotherapy (Tatsuguchi et al., 2022a).
Similarly, in vitro and in vivo studies in hepatocellular carcinoma showed that SULT2B1b is overexpressed in cancer tissues and associated with cell proliferation and migration (Yang et al., 2013; Wang et al., 2019). The knockdown of SULT2B1b in vitro suppresses tumor cell growth and promotes apoptosis and cell cycle arrest in Hepa1-6 cells by increasing the pro-apoptotic factor (FAS) expression, and downregulating the anti-apoptotic factor BCL-2, cyclinB1, and MYC, promoting cancer cell death (Yang et al., 2013). SULT2B1b overexpression may also promote tumor growth by indirectly inhibiting the LXR by sulfated oxysterols, which prevents the antiproliferative, anti-inflammatory, and lipid-regulating activities of LXR (Uppal et al., 2007; Zhang et al., 2012). Wang et al. have also reported that the SULT2B1-cholesterol sulfate-DOCK2 axis plays a vital role in inhibiting CD8+ T cell infiltration to the tumor microenvironment of hepatocellular carcinoma, which may affect immunotherapy efficacy (Wang et al., 2023). Thus, it was suggested to target the SULT2B1-cholesterol sulfate-DOCK2 axis to improve immunotherapy efficacy (Seimiya et al., 2023; Wang et al., 2023).
5.5 Urinary tract cancer
SULT2B1 was also reported to be expressed at a high level in kidney cancer compared to normal tissues, and that correlated with poor prognosis (Li et al., 2019). Overexpression of SULT2B1 promotes cancer cell proliferation by reducing tumor infiltration with lymphocytes as well as reducing the expression level of macrophages, neutrophils, B cells, CD4+ cells, CD8+ cells, and dendritic cells (Li et al., 2019). On the other hand, SULT2B1 knockdown inhibits cancer cell proliferation and reduces invasion and migration (Li et al., 2019).
6 Conclusion and future directions
SULT2B1b is a phase II metabolizing enzyme that plays a key role in regulating the homeostasis of several steroids, hydroxysteroids, and oxysterols in various body tissues, as well as molecular signaling pathways. SULT2B1 displays interindividual genetic variability in different ethnic groups (Glatt and Meinl, 2004). The altered activity or expression level of SULT2B1 due to coding or non-coding SNPs could have clinical consequences influencing disease susceptibility, drug response, and normal physiological function (Daniels and Kadlubar, 2013; Mueller et al., 2015). In fact, SULT2B1 genetic variation has been proven to be involved in various disease conditions like autosomal recessive ichthyosis, cardiovascular disease, and various malignancies. Animal studies, in vitro studies, and clinical data reported that aberrant expression of SULT2B1b has been associated with different cancer progression and tumor growth. An increased expression of SULT2B1 in colorectal, breast, endometrial, and liver cancers has been reported to promote tumor growth and poor prognosis. In contrast, reduced expression in ESCC and prostate cancer promotes tumor growth and negatively impacts survival rates (Hu et al., 2015). Furthermore, SULT2B1 expression level has been suggested to be used as an oncogenic marker for colon cancer stage and prognosis.
To date, studies conducted to study the association between SULT2B1 genetic variation and disease susceptibility or drug response are limited or have contradictory results. Thus, more genome-wide association studies and functional studies on SULT2B1 will help predict individual suitability to different diseases and drug responses.
Author contributions
FA: Writing–review and editing, Writing–original draft.
Funding
The author(s) declare that no financial support was received for the research, authorship, and/or publication of this article.
Conflict of interest
The author declares that the research was conducted in the absence of any commercial or financial relationships that could be construed as a potential conflict of interest.
Publisher’s note
All claims expressed in this article are solely those of the authors and do not necessarily represent those of their affiliated organizations, or those of the publisher, the editors and the reviewers. Any product that may be evaluated in this article, or claim that may be made by its manufacturer, is not guaranteed or endorsed by the publisher.
References
Alatwi, E. (2022). Functional genomic studies on the single nucleotide genetic polymorphisms of the human cytosolic sulfotransferase 2B1a (hSULT2B1a) [doctoral dissertation]. Toledo (OH)]: University of Toledo.
Alherz, F. A., Abunnaja, M. S., El Daibani, A. A., Bairam, A. F., Rasool, M. I., Kurogi, K., et al. (2018). On the role of genetic polymorphisms in the sulfation of cholesterol by human cytosolic sulphotransferase SULT2B1b. J. Biochem. 164 (3), 215–221. doi:10.1093/jb/mvy042
Alherz, F. A., El Daibani, A. A., Abunnaja, M. S., Bairam, A. F., Rasool, M. I., Sakakibara, Y., et al. (2019). Effect of SULT2B1 genetic polymorphisms on the sulfation of dehydroepiandrosterone and pregnenolone by SULT2B1b allozymes. Mol. Cell. Endocrinol. 496, 110535. doi:10.1016/j.mce.2019.110535
Allali-Hassani, A., Pan, P. W., Dombrovski, L., Najmanovich, R., Tempel, W., Dong, A., et al. (2007). Structural and chemical profiling of the human cytosolic sulfotransferases. PLoS Biol. 5 (5), e97. doi:10.1371/journal.pbio.0050097
Authors Anonyms (2024). National center for biotechnology information. Available at: https://www.ncbi.nlm.nih.gov/variation/view/(Accessed January 1, 2024).
Bai, Q., Xu, L., Kakiyama, G., Runge-Morris, M. A., Hylemon, P. B., Yin, L., et al. (2011). Sulfation of 25-hydroxycholesterol by SULT2B1b decreases cellular lipids via the LXR/SREBP-1c signaling pathway in human aortic endothelial cells. Atherosclerosis 214 (2), 350–356. doi:10.1016/j.atherosclerosis.2010.11.021
Bai, Q., Zhang, X., Xu, L., Kakiyama, G., Heuman, D., Sanyal, A., et al. (2012). Oxysterol sulfation by cytosolic sulfotransferase suppresses liver X receptor/sterol regulatory element binding protein-1c signaling pathway and reduces serum and hepatic lipids in mouse models of nonalcoholic fatty liver disease. Metabolism 61 (6), 836–845. doi:10.1016/j.metabol.2011.11.014
Bieche, I., Girault, I., Urbain, E., Tozlu, S., and Lidereau, R. (2004). Relationship between intratumoral expression of genes coding for xenobiotic-metabolizing enzymes and benefit from adjuvant tamoxifen in estrogen receptor alpha-positive postmenopausal breast carcinoma. Breast Cancer Res. 6 (3), R252–R263. doi:10.1186/bcr784
Boudhraa, Z., Bouchon, B., Viallard, C., D'Incan, M., and Degoul, F. (2016). Annexin A1 localization and its relevance to cancer. Clin. Sci. (Lond) 130 (4), 205–220. doi:10.1042/CS20150415
Che, G., Wang, W., Wang, J., He, C., Yin, J., Chen, Z., et al. (2024). Sulfotransferase SULT2B1 facilitates colon cancer metastasis by promoting SCD1-mediated lipid metabolism. Clin. Transl. Med. 14 (2), e1587. doi:10.1002/ctm2.1587
Chen, W., Zhou, H., Ye, L., and Zhan, B. (2016). Overexpression of SULT2B1b promotes angiogenesis in human gastric cancer. Cell. Physiol. Biochem. 38 (3), 1040–1054. doi:10.1159/000443055
Cook, I., and Leyh, T. S. (2022). The N-terminus of human sulfotransferase 2B1b─a sterol-sensing allosteric site. Biochemistry 61 (10), 843–855. doi:10.1021/acs.biochem.1c00740
Cook, I. T., Duniec-Dmuchowski, Z., Kocarek, T. A., Runge-Morris, M., and Falany, C. N. (2009). 24-hydroxycholesterol sulfation by human cytosolic sulfotransferases: formation of monosulfates and disulfates, molecular modeling, sulfatase sensitivity, and inhibition of liver x receptor activation. Drug Metab. Dispos. 37 (10), 2069–2078. doi:10.1124/dmd.108.025759
Daniels, J., and Kadlubar, S. (2013). Sulfotransferase genetic variation: from cancer risk to treatment response. Drug Metab. Rev. 45 (4), 415–422. doi:10.3109/03602532.2013.835621
Dumas, N. A., He, D., Frost, A. R., and Falany, C. N. (2008). Sulfotransferase 2B1b in human breast: differences in subcellular localization in African American and Caucasian women. J. Steroid Biochem. Mol. Biol. 111 (3-5), 171–177. doi:10.1016/j.jsbmb.2008.05.006
Falany, C. N. (1997). Enzymology of human cytosolic sulfotransferases. FASEB J. 11 (4), 206–216. doi:10.1096/fasebj.11.4.9068609
Falany, C. N., He, D., Dumas, N., Frost, A. R., and Falany, J. L. (2006a). Human cytosolic sulfotransferase 2B1: isoform expression, tissue specificity and subcellular localization. J. Steroid Biochem. Mol. Biol. 102 (1-5), 214–221. doi:10.1016/j.jsbmb.2006.09.011
Falany, C. N., and Rohn-Glowacki, K. J. (2013). SULT2B1: unique properties and characteristics of a hydroxysteroid sulfotransferase family. Drug Metab. Rev. 45 (4), 388–400. doi:10.3109/03602532.2013.835609
Falany, J. L., Macrina, N., and Falany, C. N. (2004). Sulfation of tibolone and tibolone metabolites by expressed human cytosolic sulfotransferases. J. Steroid Biochem. Mol. Biol. 88 (4-5), 383–391. doi:10.1016/j.jsbmb.2004.01.005
Falany, J. L., Pilloff, D. E., Leyh, T. S., and Falany, C. N. (2006b). Sulfation of raloxifene and 4-hydroxytamoxifen by human cytosolic sulfotransferases. Drug Metab. Dispos. 34 (3), 361–368. doi:10.1124/dmd.105.006551
Fioretti, T., Auricchio, L., Piccirillo, A., Vitiello, G., Ambrosio, A., Cattaneo, F., et al. (2020). Multi-gene next-generation sequencing for molecular diagnosis of autosomal recessive congenital ichthyosis: a genotype-phenotype study of four Italian patients. Diagn. (Basel) 10 (12), 995. doi:10.3390/diagnostics10120995
Fozia, F., Nazli, R., Alam Khan, S., Bari, A., Nasir, A., Ullah, R., et al. (2021). Novel homozygous mutations in the genes TGM1, SULT2B1, SPINK5 and FLG in four families underlying congenital ichthyosis. Genes. (Basel) 12 (3), 373. doi:10.3390/genes12030373
Frank, J. G., Michael, C., and Robert, H. T. (2011). “Drug metabolism,” in Goodman and gilman's: the pharmacological basis of therapeutics. Editors L. B. Laurence, A. C. Bruce, and C. K. Björn 12 th edn (New York: McGraw-Hill), 123–144.
Fuda, H., Javitt, N. B., Mitamura, K., Ikegawa, S., and Strott, C. A. (2007). Oxysterols are substrates for cholesterol sulfotransferase. J. Lipid Res. 48 (6), 1343–1352. doi:10.1194/jlr.M700018-JLR200
Fuda, H., Lee, Y. C., Shimizu, C., Javitt, N. B., and Strott, C. A. (2002). Mutational analysis of human hydroxysteroid sulfotransferase SULT2B1 isoforms reveals that exon 1B of the SULT2B1 gene produces cholesterol sulfotransferase, whereas exon 1A yields pregnenolone sulfotransferase. J. Biol. Chem. 277 (39), 36161–36166. doi:10.1074/jbc.M207165200
Gao, H., Xia, M., and Ruan, H. (2024). Knockdown of sulfotransferase 2B1 suppresses cell migration, invasion and promotes apoptosis in ovarian carcinoma cells via targeting annexin A9. J. Obstet. Gynaecol. Res. 50 (8), 1334–1344. doi:10.1111/jog.15969
Geese, W. J., and Raftogianis, R. B. (2001). Biochemical characterization and tissue distribution of human SULT2B1. Biochem. Biophys. Res. Commun. 288 (1), 280–289. doi:10.1006/bbrc.2001.5746
Glatt, H., Boeing, H., Engelke, C. E., Ma, L., Kuhlow, A., Pabel, U., et al. (2001). Human cytosolic sulfotransferases: genetics, characteristics, toxicological aspects. Mutat. Res. 482 (1-2), 27–40. doi:10.1016/s0027-5107(01)00207-x
Glatt, H., and Meinl, W. (2004). Pharmacogenetics of soluble sulfotransferases (SULTs). Naunyn Schmiedeb. Arch. Pharmacol. 369 (1), 55–68. doi:10.1007/s00210-003-0826-0
He, D., and Falany, C. N. (2006). Characterization of proline-serine-rich carboxyl terminus in human sulfotransferase 2B1b: immunogenicity, subcellular localization, kinetic properties, and phosphorylation. Drug Metab. Dispos. 34 (10), 1749–1755. doi:10.1124/dmd.106.011114
He, D., and Falany, C. N. (2007). Inhibition of SULT2B1b expression alters effects of 3beta-hydroxysteroids on cell proliferation and steroid hormone receptor expression in human LNCaP prostate cancer cells. Prostate 67 (12), 1318–1329. doi:10.1002/pros.20615
He, D., Frost, A. R., and Falany, C. N. (2005). Identification and immunohistochemical localization of Sulfotransferase 2B1b (SULT2B1b) in human lung. Biochim. Biophys. Acta 1724 (1-2), 119–126. doi:10.1016/j.bbagen.2005.03.018
He, D., Meloche, C. A., Dumas, N. A., Frost, A. R., and Falany, C. N. (2004). Different subcellular localization of sulfotransferase 2B1b in human placenta and prostate. Biochem. J. 379 (Pt 3), 533–540. doi:10.1042/BJ20031524
Heinz, L., Kim, G. J., Marrakchi, S., Christiansen, J., Turki, H., Rauschendorf, M. A., et al. (2017). Mutations in SULT2B1 cause autosomal-recessive congenital ichthyosis in humans. Am. J. Hum. Genet. 100 (6), 926–939. doi:10.1016/j.ajhg.2017.05.007
Her, C., Szumlanski, C., Aksoy, I. A., and Weinshilboum, R. M. (1996). Human jejunal estrogen sulfotransferase and dehydroepiandrosterone sulfotransferase: immunochemical characterization of individual variation. Drug Metab. Dispos. 24 (12), 1328–1335.
Her, C., Wood, T. C., Eichler, E. E., Mohrenweiser, H. W., Ramagli, L. S., Siciliano, M. J., et al. (1998). Human hydroxysteroid sulfotransferase SULT2B1: two enzymes encoded by a single chromosome 19 gene. Genomics 53 (3), 284–295. doi:10.1006/geno.1998.5518
Hevir, N., Sinkovec, J., and Rizner, T. L. (2011). Disturbed expression of phase I and phase II estrogen-metabolizing enzymes in endometrial cancer: lower levels of CYP1B1 and increased expression of S-COMT. Mol. Cell. Endocrinol. 331 (1), 158–167. doi:10.1016/j.mce.2010.09.011
Higashi, Y., Fuda, H., Yanai, H., Lee, Y., Fukushige, T., Kanzaki, T., et al. (2004). Expression of cholesterol sulfotransferase (SULT2B1b) in human skin and primary cultures of human epidermal keratinocytes. J. Investig. Dermatol 122 (5), 1207–1213. doi:10.1111/j.0022-202X.2004.22416.x
Hong, W., Guo, F., Yang, M., Xu, D., Zhuang, Z., Niu, B., et al. (2019). Hydroxysteroid sulfotransferase 2B1 affects gastric epithelial function and carcinogenesis induced by a carcinogenic agent. Lipids Health Dis. 18 (1), 203. doi:10.1186/s12944-019-1149-6
Hu, L., Yang, G. Z., Zhang, Y., Feng, D., Zhai, Y. X., Gong, H., et al. (2015). Overexpression of SULT2B1b is an independent prognostic indicator and promotes cell growth and invasion in colorectal carcinoma. Lab. Investig. 95 (9), 1005–1018. doi:10.1038/labinvest.2015.84
Huang, J., Zhou, M., Zhang, H., Fang, Y., Chen, G., Wen, J., et al. (2022). Characterization of the mechanism of Scutellaria baicalensis on reversing radio-resistance in colorectal cancer. Transl. Oncol. 24, 101488. doi:10.1016/j.tranon.2022.101488
Hyland, P. L., Freedman, N. D., Hu, N., Tang, Z. Z., Wang, L., Wang, C., et al. (2013). Genetic variants in sex hormone metabolic pathway genes and risk of esophageal squamous cell carcinoma. Carcinogenesis 34 (5), 1062–1068. doi:10.1093/carcin/bgt030
Javitt, N. B., Lee, Y. C., Shimizu, C., Fuda, H., and Strott, C. A. (2001). Cholesterol and hydroxycholesterol sulfotransferases: identification, distinction from dehydroepiandrosterone sulfotransferase, and differential tissue expression. Endocrinology 142 (7), 2978–2984. doi:10.1210/endo.142.7.8244
Ji, Y., Moon, I., Zlatkovic, J., Salavaggione, O. E., Thomae, B. A., Eckloff, B. W., et al. (2007). Human hydroxysteroid sulfotransferase SULT2B1 pharmacogenomics: gene sequence variation and functional genomics. J. Pharmacol. Exp. Ther. 322 (2), 529–540. doi:10.1124/jpet.107.122895
Koizumi, M., Momoeda, M., Hiroi, H., Hosokawa, Y., Tsutsumi, R., Osuga, Y., et al. (2010). Expression and regulation of cholesterol sulfotransferase (SULT2B1b) in human endometrium. Fertil. Steril. 93 (5), 1538–1544. doi:10.1016/j.fertnstert.2009.01.075
Kou, X., Ding, H., Li, L., and Chao, H. (2021). Hsa-miR-105-1 regulates cisplatin-resistance in ovarian carcinoma cells by targeting ANXA9. Anal. Cell. Pathol. (Amst) 2021, 6662486. doi:10.1155/2021/6662486
Koutros, S., Andreotti, G., Berndt, S. I., Hughes Barry, K., Lubin, J. H., Hoppin, J. A., et al. (2011). Xenobiotic-metabolizing gene variants, pesticide use, and the risk of prostate cancer. Pharmacogenet Genomics 21 (10), 615–623. doi:10.1097/FPC.0b013e3283493a57
Lee, K. A., Fuda, H., Lee, Y. C., Negishi, M., Strott, C. A., and Pedersen, L. C. (2003). Crystal structure of human cholesterol sulfotransferase (SULT2B1b) in the presence of pregnenolone and 3'-phosphoadenosine 5'-phosphate. Rationale for specificity differences between prototypical SULT2A1 and the SULT2BG1 isoforms. J. Biol. Chem. 278 (45), 44593–44599. doi:10.1074/jbc.M308312200
Levesque, E., Laverdiere, I., Audet-Walsh, E., Caron, P., Rouleau, M., Fradet, Y., et al. (2014). Steroidogenic germline polymorphism predictors of prostate cancer progression in the estradiol pathway. Clin. Cancer Res. 20 (11), 2971–2983. doi:10.1158/1078-0432.CCR-13-2567
Li, S., Xie, L., Du, M., Xu, K., Zhu, L., Chu, H., et al. (2018). Association study of genetic variants in estrogen metabolic pathway genes and colorectal cancer risk and survival. Arch. Toxicol. 92 (6), 1991–1999. doi:10.1007/s00204-018-2195-y
Li, Y. Q., Ding, Q., Xiong, Z. Q., Wen, H., and Feng, C. C. (2019). Overexpression of steroid sulfotransferase genes is associated with worsened prognosis and with immune exclusion in clear cell-renal cell carcinoma. Aging-Us 11 (20), 9209–9219. doi:10.18632/aging.102392
Li, Z., Li, M. Y., Wang, L. L., Li, L., Chen, Q. Y., Zhu, Y. H., et al. (2021). The promoter hypermethylation of SULT2B1 accelerates esophagus tumorigenesis via downregulated PER1. Thorac. Cancer 12 (24), 3370–3379. doi:10.1111/1759-7714.14211
Liu, L., and Klaassen, C. D. (1996). Regulation of hepatic sulfotransferases by steroidal chemicals in rats. Drug Metab. Dispos. 24 (8), 854–858.
Low, Y. L., Li, Y., Humphreys, K., Thalamuthu, A., Li, Y., Darabi, H., et al. (2010). Multi-variant pathway association analysis reveals the importance of genetic determinants of estrogen metabolism in breast and endometrial cancer susceptibility. PLoS Genet. 6 (7), e1001012. doi:10.1371/journal.pgen.1001012
Luu, W., Sharpe, L. J., Capell-Hattam, I., Gelissen, I. C., and Brown, A. J. (2016). Oxysterols: old tale, new twists. Annu. Rev. Pharmacol. Toxicol. 56, 447–467. doi:10.1146/annurev-pharmtox-010715-103233
Morino, K., Kunimura, K., Sugiura, Y., Izumi, Y., Matsubara, K., Akiyoshi, S., et al. (2023). Cholesterol sulfate limits neutrophil recruitment and gut inflammation during mucosal injury. Front. Immunol. 14, 1131146. doi:10.3389/fimmu.2023.1131146
Mueller, J. W., Gilligan, L. C., Idkowiak, J., Arlt, W., and Foster, P. A. (2015). The regulation of steroid action by sulfation and desulfation. Endocr. Rev. 36 (5), 526–563. doi:10.1210/er.2015-1036
Nishida, T., Ayaori, M., Arakawa, J., Suenaga, Y., Shiotani, K., Uto-Kondo, H., et al. (2024). Liver-specific Lr inhibition represses reverse cholesterol transport in cholesterol-fed mice. Atherosclerosis, 117578. doi:10.1016/j.atherosclerosis.2024.117578
Pai, T. G., Sugahara, T., Suiko, M., Sakakibara, Y., Xu, F., and Liu, M. C. (2002). Differential xenoestrogen-sulfating activities of the human cytosolic sulfotransferases: molecular cloning, expression, and purification of human SULT2B1a and SULT2B1b sulfotransferases. Biochim. Biophys. Acta 1573 (2), 165–170. doi:10.1016/s0304-4165(02)00416-6
Pan, H., Wu, T., Huang, K., Guo, Z., Liang, H., Lyu, P., et al. (2024). Reducing SULT2B1 promotes the interaction of LncRNAgga3-204 with SMAD4 to inhibit the macrophage inflammatory response and delay atherosclerosis progression. Transl. Res. 268, 13–27. doi:10.1016/j.trsl.2024.01.004
Park, J. H., Lee, J., Lee, G. R., Kwon, M., Lee, H. I., Kim, N., et al. (2023a). Cholesterol sulfate inhibits osteoclast differentiation and survival by regulating the AMPK-Sirt1-NF-κB pathway. J. Cell. Physiol. 238 (9), 2063–2075. doi:10.1002/jcp.31064
Park, J. S., Chung, I. J., Kim, H. R., and Jun, C. D. (2023b). The immunosuppressive potential of cholesterol sulfate through T cell microvilli disruption. Immune Netw. 23 (3), e29. doi:10.4110/in.2023.23.e29
Park, S., Song, C. S., Lin, C. L., Jiang, S., Osmulski, P. A., Wang, C. M., et al. (2020). Inhibitory interplay of SULT2B1b sulfotransferase with AKR1C3 aldo-keto reductase in prostate cancer. Endocrinology 161 (2), bqz042. doi:10.1210/endocr/bqz042
Raciti, L., Formica, C., Raciti, G., Quartarone, A., and Calabro, R. S. (2023). Gender and neurosteroids: implications for brain function, neuroplasticity and rehabilitation. Int. J. Mol. Sci. 24 (5), 4758. doi:10.3390/ijms24054758
Ren, S., and Ning, Y. (2014). Sulfation of 25-hydroxycholesterol regulates lipid metabolism, inflammatory responses, and cell proliferation. Am. J. Physiol. Endocrinol. Metab. 306 (2), E123–E130. doi:10.1152/ajpendo.00552.2013
Riches, Z., Stanley, E. L., Bloomer, J. C., and Coughtrie, M. W. (2009). Quantitative evaluation of the expression and activity of five major sulfotransferases (SULTs) in human tissues: the SULT pie. Drug Metab. Dispos. 37 (11), 2255–2261. doi:10.1124/dmd.109.028399
Sakurai, T., Uruno, T., Sugiura, Y., Tatsuguchi, T., Yamamura, K., Ushijima, M., et al. (2018). Cholesterol sulfate is a DOCK2 inhibitor that mediates tissue-specific immune evasion in the eye. Sci. Signal 11 (541), eaao4874. doi:10.1126/scisignal.aao4874
Salman, E. D., Faye-Petersen, O., and Falany, C. N. (2011). Hydroxysteroid sulfotransferase 2B1b expression and localization in normal human brain. Horm. Mol. Biol. Clin. Investig. 8 (1), 445–454. doi:10.1515/HMBCI.2011.117
Sanchez-Guijo, A., Neunzig, J., Gerber, A., Oji, V., Hartmann, M. F., Schuppe, H. C., et al. (2016). Role of steroid sulfatase in steroid homeostasis and characterization of the sulfated steroid pathway: evidence from steroid sulfatase deficiency. Mol. Cell. Endocrinol. 437, 142–153. doi:10.1016/j.mce.2016.08.019
Schade, D. S., Shey, L., and Eaton, R. P. (2020). Cholesterol review: a metabolically important molecule. Endocr. Pract. 26 (12), 1514–1523. doi:10.4158/EP-2020-0347
Seimiya, T., Otsuka, M., and Fujishiro, M. (2023). Overcoming T-cell exhaustion: new therapeutic targets in HCC immunotherapy. Hepatology 78 (4), 1009–1011. doi:10.1097/HEP.0000000000000039
Seo, Y. K., Mirkheshti, N., Song, C. S., Kim, S., Dodds, S., Ahn, S. C., et al. (2013). SULT2B1b sulfotransferase: induction by vitamin D receptor and reduced expression in prostate cancer. Mol. Endocrinol. 27 (6), 925–939. doi:10.1210/me.2012-1369
Song, C., Hiipakka, R. A., and Liao, S. (2001). Auto-oxidized cholesterol sulfates are antagonistic ligands of liver X receptors: implications for the development and treatment of atherosclerosis. Steroids 66 (6), 473–479. doi:10.1016/s0039-128x(00)00239-7
Strott, C. A. (2002). Sulfonation and molecular action. Endocr. Rev. 23 (5), 703–732. doi:10.1210/er.2001-0040
Strott, C. A., and Higashi, Y. (2003). Cholesterol sulfate in human physiology: what's it all about? J. Lipid Res. 44 (7), 1268–1278. doi:10.1194/jlr.R300005-JLR200
Tatsuguchi, T., Uruno, T., Sugiura, Y., Oisaki, K., Takaya, D., Sakata, D., et al. (2022a). Pharmacological intervention of cholesterol sulfate-mediated T cell exclusion promotes antitumor immunity. Biochem. Biophys. Res. Commun. 609, 183–188. doi:10.1016/j.bbrc.2022.04.035
Tatsuguchi, T., Uruno, T., Sugiura, Y., Sakata, D., Izumi, Y., Sakurai, T., et al. (2022b). Cancer-derived cholesterol sulfate is a key mediator to prevent tumor infiltration by effector T cells. Int. Immunol. 34 (5), 277–289. doi:10.1093/intimm/dxac002
Tozlu, S., Girault, I., Vacher, S., Vendrell, J., Andrieu, C., Spyratos, F., et al. (2006). Identification of novel genes that co-cluster with estrogen receptor alpha in breast tumor biopsy specimens, using a large-scale real-time reverse transcription-PCR approach. Endocr. Relat. Cancer 13 (4), 1109–1120. doi:10.1677/erc.1.01120
Uppal, H., Saini, S. P., Moschetta, A., Mu, Y., Zhou, J., Gong, H., et al. (2007). Activation of LXRs prevents bile acid toxicity and cholestasis in female mice. Hepatology 45 (2), 422–432. doi:10.1002/hep.21494
Vallee, M. (2016). Neurosteroids and potential therapeutics: focus on pregnenolone. J. Steroid Biochem. Mol. Biol. 160, 78–87. doi:10.1016/j.jsbmb.2015.09.030
Vallee, M., Mayo, W., and Le Moal, M. (2001). Role of pregnenolone, dehydroepiandrosterone and their sulfate esters on learning and memory in cognitive aging. Brain Res. Brain Res. Rev. 37 (1-3), 301–312. doi:10.1016/s0165-0173(01)00135-7
Vaya, J., Szuchman, A., Tavori, H., and Aluf, Y. (2011). Oxysterols formation as a reflection of biochemical pathways: summary of in vitro and in vivo studies. Chem. Phys. Lipids 164 (6), 438–442. doi:10.1016/j.chemphyslip.2011.03.005
Vickman, R. E., Crist, S. A., Kerian, K., Eberlin, L., Cooks, R. G., Burcham, G. N., et al. (2016). Cholesterol sulfonation enzyme, SULT2B1b, modulates AR and cell growth properties in prostate cancer. Mol. Cancer Res. 14 (9), 776–786. doi:10.1158/1541-7786.MCR-16-0137
Vickman, R. E., Yang, J., Lanman, N. A., Cresswell, G. M., Zheng, F., Zhang, C., et al. (2019). Cholesterol sulfotransferase SULT2B1b modulates sensitivity to death receptor ligand TNFα in castration-resistant prostate cancer. Mol. Cancer Res. 17 (6), 1253–1263. doi:10.1158/1541-7786.MCR-18-1054
Wang, C., Wang, P., Liu, J. C., Zhao, Z. A., Guo, R., Li, Y., et al. (2020). Interaction of estradiol and endoplasmic reticulum stress in the development of esophageal carcinoma. Front. Endocrinol. (Lausanne) 11, 410. doi:10.3389/fendo.2020.00410
Wang, Q. M., Qi, Y. J., Jiang, Q., Ma, Y. F., and Wang, L. D. (2011). Relevance of serum estradiol and estrogen receptor beta expression from a high-incidence area for esophageal squamous cell carcinoma in China. Med. Oncol. 28 (1), 188–193. doi:10.1007/s12032-010-9457-8
Wang, S., Wang, R., Xu, N., Wei, X., Yang, Y., Lian, Z., et al. (2023). SULT2B1-CS-DOCK2 axis regulates effector T-cell exhaustion in HCC microenvironment. Hepatology 78 (4), 1064–1078. doi:10.1097/HEP.0000000000000025
Wang, Z. Y., Zhu, Z., Wang, H. F., Qin, B., Liu, J., Yao, X. H., et al. (2019). Downregulation of circDYNC1H1 exhibits inhibitor effect on cell proliferation and migration in hepatocellular carcinoma through miR-140-5p. J. Cell. Physiol. 234 (10), 17775–17785. doi:10.1002/jcp.28403
Yanai, H., Javitt, N. B., Higashi, Y., Fuda, H., and Strott, C. A. (2004). Expression of cholesterol sulfotransferase (SULT2B1b) in human platelets. Circulation 109 (1), 92–96. doi:10.1161/01.CIR.0000108925.95658.8D
Yang, J., Broman, M. M., Cooper, P. O., Lanman, N. A., Strand, D. W., Morrissey, C., et al. (2019). Distinct expression patterns of SULT2B1b in human prostate epithelium. Prostate 79 (11), 1256–1266. doi:10.1002/pros.23829
Yang, X., Xu, Y., Guo, F., Ning, Y., Zhi, X., Yin, L., et al. (2013). Hydroxysteroid sulfotransferase SULT2B1b promotes hepatocellular carcinoma cells proliferation in vitro and in vivo. PLoS One 8 (4), e60853. doi:10.1371/journal.pone.0060853
Yin, L., and Chen, G. (2020). Verteporfin promotes the apoptosis and inhibits the proliferation, migration, and invasion of cervical cancer cells by downregulating SULT2B1 expression. Med. Sci. Monit. 26, e926780. doi:10.12659/MSM.926780
Yin, M., Lu, J., Guo, Z., Zhang, Y., Liu, J., Wu, T., et al. (2021). Reduced SULT2B1b expression alleviates ox-LDL-induced inflammation by upregulating miR-148-3P via inhibiting the IKKβ/NF-κB pathway in macrophages. Aging (Albany NY) 13 (3), 3428–3442. doi:10.18632/aging.202273
Yip, C. K. Y., Bansal, S., Wong, S. Y., and Lau, A. J. (2018). Identification of galeterone and abiraterone as inhibitors of dehydroepiandrosterone sulfonation catalyzed by human hepatic cytosol, SULT2A1, SULT2B1b, and SULT1E1. Drug Metab. Dispos. 46 (4), 470–482. doi:10.1124/dmd.117.078980
Youssefian, L., Vahidnezhad, H., Saeidian, A. H., Touati, A., Sotoudeh, S., Mahmoudi, H., et al. (2019). Autosomal recessive congenital ichthyosis: genomic landscape and phenotypic spectrum in a cohort of 125 consanguineous families. Hum. Mutat. 40 (3), 288–298. doi:10.1002/humu.23695
Yue, Y., Song, M., Qiao, Y., Li, P., Yuan, Y., Lian, J., et al. (2017). Gene function analysis and underlying mechanism of esophagus cancer based on microarray gene expression profiling. Oncotarget 8 (62), 105222–105237. doi:10.18632/oncotarget.22160
Zhang, J., Zhang, R., Li, W., Ma, X. C., Qiu, F., and Sun, C. P. (2023a). IκB kinase β (IKKβ): structure, transduction mechanism, biological function, and discovery of its inhibitors. Int. J. Biol. Sci. 19 (13), 4181–4203. doi:10.7150/ijbs.85158
Zhang, T. T., Yu, S. Y., and Zhao, S. P. (2021). ANXA9 as a novel prognostic biomarker associated with immune infiltrates in gastric cancer. Peerj 9, e12605. doi:10.7717/peerj.12605
Zhang, X., Bai, Q., Xu, L., Kakiyama, G., Pandak, W. M., Zhang, Z., et al. (2012). Cytosolic sulfotransferase 2B1b promotes hepatocyte proliferation gene expression in vivo and in vitro. Am. J. Physiol. Gastrointest. Liver Physiol. 303 (3), G344–G355. doi:10.1152/ajpgi.00403.2011
Zhang, Y., Dong, K., Jia, X., Du, S., Wang, D., Wang, L., et al. (2023b). A novel extrachromosomal circular DNA related genes signature for overall survival prediction in patients with ovarian cancer. BMC Med. Genomics 16 (1), 140. doi:10.1186/s12920-023-01576-x
Zhang, Y., Guo, Z., Wu, T., Liu, J., Zhang, B., Lai, W., et al. (2020a). SULT2B1b inhibits reverse cholesterol transport and promotes cholesterol accumulation and inflammation in lymphocytes from AMI patients with low LDL-C levels. Clin. Sci. (Lond) 134 (2), 273–287. doi:10.1042/CS20190459
Zhang, Z. Y., Yao, Q. Z., Liu, H. Y., Guo, Q. N., Qiu, P. J., Chen, J. P., et al. (2020b). Metabolic reprogramming-associated genes predict overall survival for rectal cancer. J. Cell. Mol. Med. 24 (10), 5842–5849. doi:10.1111/jcmm.15254
Zhao, T., Li, Y., Shen, K., Wang, Q., and Zhang, J. (2021). Knockdown of OLR1 weakens glycolytic metabolism to repress colon cancer cell proliferation and chemoresistance by downregulating SULT2B1 via c-MYC. Cell. Death Dis. 13 (1), 4. doi:10.1038/s41419-021-04174-w
Zhou, Y., Qiu, C. Y., Wang, T., Tao, L. S., Zhang, Z. G., and Yao, J. (2021). High expression of annexin A9 promotes cell proliferation and migration in gastric cancer via the TGF-β signaling pathway. J. Environ. Pathology Toxicol. Oncol. 40 (3), 87–94. doi:10.1615/jenvironpatholtoxicoloncol.2021038527
Keywords: cholesterol sulfotransferase, SULT2B1, autosomal recessive ichthyosis, polymorphism, pregnenolone sulfotransferase, colon cancer
Citation: Alherz FA (2024) Human sulfotransferase SULT2B1 physiological role and the impact of genetic polymorphism on enzyme activity and pathological conditions. Front. Genet. 15:1464243. doi: 10.3389/fgene.2024.1464243
Received: 13 July 2024; Accepted: 22 August 2024;
Published: 30 August 2024.
Edited by:
Otito Frances Iwuchukwu, Fairleigh Dickinson University, United StatesReviewed by:
Myeongjin Yi, National Institute of Environmental Health Sciences (NIH), United StatesCopyright © 2024 Alherz. This is an open-access article distributed under the terms of the Creative Commons Attribution License (CC BY). The use, distribution or reproduction in other forums is permitted, provided the original author(s) and the copyright owner(s) are credited and that the original publication in this journal is cited, in accordance with accepted academic practice. No use, distribution or reproduction is permitted which does not comply with these terms.
*Correspondence: Fatemah A. Alherz, RmFhbGhlcnpAcG51LmVkdS5zYQ==