- 1National Key Laboratory of Crop Biology, National Research Center for Apple Engineering and Technology, College of Horticulture Science and Engineering, Shandong Agricultural University, Taian, China
- 2College of Agriculture, Yunnan University, Kunming, China
The multicopy suppressor of IRA (MSI) is a subfamily of WD40 repeat proteins, which is widely involved in plant growth and development. In order to explore the function of MdMSI members in abiotic stress, we identified eight MSI gene family members from the Malus × domestica reference genome. They were distributed on six chromosomes, and they had similar secondary and tertiary structures. We found a variety of regulatory elements in response to hormones and abiotic stress in MdMSI promoters. Through qRT-PCR analysis, it was revealed that MdMSIs were expressed in all tissues, especially in roots. The analysis results also revealed that the expression of MdMSIs was induced in varying degrees under salt, drought stress, and ABA treatments. Furthermore, we obtained the overexpression of MdMSI1-1 transgenic apple calli and Arabidopsis. The overexpression of MdMSI1-1 in calli and Arabidopsis played a negative regulatory role in salt stress response. Our work laid a foundation for further verifying the function of MSI genes under abiotic stress in apples.
Introduction
WD40 repeat protein is generally composed of 4–10 corresponding WD40 domains, of which the WD40 domain is composed of about 40 conserved amino acids, and its N-terminal is the glycine–histidine dimer peptide. WD40 protein is the most studied in eukaryotes and plays a variety of important functions, such as embryogenesis and gamete formation, flower development, and flowering process (Jiang et al., 2009; Jiang et al., 2011; Pazhouhandeh et al., 2011). Some members can respond to abiotic stress and hormone induction (Cheng et al., 2014; Qi et al., 2014; Liu et al., 2018).
The multicopy suppressor of IRA (MSI) is a kind of WD40 repeat protein, which has similar and specific functions in different plants. MSI1 is screened for the first time in Saccharomyces cerevisiae and is determined to negatively regulate the RAS-mediated cAMP pathway (Ruggieri et al., 1989). In previous studies, the MSI family is widely reported in plant reproductive development, such as male and female gamete development, endosperm development, regulating flowering, and low-temperature vernalization (Kaya et al., 2001; Rossi et al., 2001; Guitton and Berger 2005; Pazhouhandeh et al., 2011; Derkacheva et al., 2013). In addition, MSI genes play an important role in participating in some abiotic stresses during plant growth and development (Jeon and Kim 2011; Kenzior and Folk 2015; Cui et al., 2022). There are five members of this family in Arabidopsis (Hennig et al., 2005), among which AtMSI1 plays a negative regulatory role in salt and drought stress (Alexandre et al., 2009; Mehdi et al., 2016). AtMSI4 plays an important role in controlling of flowering and salt stress (Kenzior and Folk 2015). Other studies have shown that AtMSI4/FVE plays a role in cold resistance (Kim et al., 2004). At present, there are few studies on AtMSI2 and AtMSI3, and it is uncertain about what kind of function they play in Arabidopsis (Shen et al., 2019). Moreover, GmFVE can interact with GmNFYA and play a negative regulatory role in salt stress (Lu et al., 2021). Some members of the MSIL family in longan have a variety of abiotic stress response elements. It is considered that some members of the MSIL gene family may participate in a variety of abiotic stresses (Shen et al., 2019).
The apple is one of the fruits with the largest planting area in the world (An et al., 2016; Wang et al., 2019). With the increasing deterioration of the global environment, plants will encounter more and more complex stresses in the growth process. Salt and drought stress have great effects on plant growth and development (Manickavelu et al., 2006; Jaleel et al., 2009). Salt stress will cause ion and osmotic stress to plants, and drought stress will reduce the plant photosynthetic rate and photosynthetic electron transfer rate (Tian et al., 2015; Sequera-Mutiozabal et al., 2016).
In apples, the MSI gene family has not been systematically investigated. In this study, the whole genome identification, systematic prediction and analysis of gene and protein levels, tissue expression, and abiotic stress changes of the MdMSI gene family were carried out. In addition, we further verified the salt intolerant function of MdMSI-1 by genetic transformation of apple calli and Arabidopsis. It provides a theoretical basis for studying the mechanism of the MdMSI gene family in the process of abiotic stress in apples.
Materials and Methods
Plant Materials and Growth Conditions
The test plant materials were obtained from 12-year-old “Royal Gala” apple (Malus domestica Borkh. “Royal Gala”) trees cultivated at the Shandong Agricultural University experimental station and from 1-month-old self-rooted apple seedlings [Malus hupehensis (Pamp.) Rehd. “pinyiensis.”] cultivated in the laboratory of molecular biology of fruit trees of the Academy of Horticultural Science and Engineering, Shandong Agricultural University. After taking the roots, stems, leaves, flowers (initial flowering), peels, and pulps of “Royal Gala,” the samples were quickly added in liquid nitrogen and timely transferred to an ultra-low temperature refrigerator for storage for subsequent test operations.
The apple shoot cultures, derived from “Royal Gala,” were stored at 24°C on MS solid medium (containing 0.8% agar, 0.2 mg/L NAA, 0.5 mg/L 6-BA, and 0.1 mg/L GA3) under a photoperiod (8/16 h dark/light) for 25 days. To obtain self-rooted plantlets, the 4-week-old shoot cultures were transferred to a root-inducing MS solid medium containing 0.2 mg/L IAA. For gene expression analysis, 25-day-old self-rooted apple seedlings were treated with 150 mM NaCl, 10% PEG 6000, and 100 μM ABA. Apple seedlings were sampled at different time points after treating for 0, 1, 3, 6, and 12 h and were quickly placed in liquid nitrogen and stored in an ultra-low temperature refrigerator for subsequent experiments (Yamaguchi-Shinozaki and Shinozaki 1994).
“Orin” apple calli were grown on MS solid medium supplemented with 0.8% agar, 0.4 mg/L 6-BA, and 1.5 mg/L 2, 4-dichlorophenoxyacetic acid (2,4-D) at 25°C in the dark. After 15 days of growth, calli (WT and MdMSI1-1-OX) were transferred to the MS solid medium supplemented with 0 or 100 mM NaCl and placed in the dark for 16 days. Arabidopsis thaliana (Columbia) seeds were germinated on the MS solid medium at 22°C in a photoperiod (16 h/8 h, light/dark). The 3-day-old Arabidopsis seedlings (WT and MdMSI1-1-OE) were transferred to the MS solid medium that supplied with 0 or 100 mM NaCl for 14 days. After treatment, we measured the MDA content of calli following the study by (Ma et al., 2017) and the relative electronic conductivity level of Arabidopsis following the study by (Hu et al., 2013).
Construction of the MdMSI1-1 Expression Plasmid
The full-length cDNA of MdMSI1-1 was cloned into the PRI-101 (35S promoter, GFP) vector to obtain its overexpression plasmid. The primer pairs MdMSI1-1-F (5′-ATGGGCAAAGAC -3′)/−R and (5′- AGGCTTTGCCGGTTC -3′) were used to amplify the full-length MdMSI1-1.
Genetic Transformation
Apple calli were infected by Agrobacterium-mediated transformation to obtain MdMSI1-1 overexpression transgenic apple calli (MdMSI1-1-OX) (An et al., 2016). The transgenic Arabidopsis (MdMSI1-1-OE) were obtained by using the floral dip transformation method (Yang et al., 2021).
Identification and Basic Properties of MSI Family Members in the Apple
The apple “GDDH13” reference genome is from the Apple Genome and Epigenome database (GDDH13_1-1, https://iris.angers.inra.fr/gddh13/) (Daccord et al., 2017). Arabidopsis MSI proteins are from the NCBI (https://www.ncbi.nlm.nih.gov/). The apple MSIs were searched by using AtMSI sequences (BLASTp). In addition, apple MSIs were obtained by the HMM method with the E-value (10e−5) (http://pfam.xfam.org/) (Finn et al., 2014). The domains (CAF1C_H4-bd, WD40) were analyzed and confirmed by online software SMART (http://smart.embl-heidelberg.de/). The members of apple MSIs were finally obtained. The number of amino acids, molecular weight, isoelectric point, and other physical and chemical properties of MdMSI gene family members were analyzed by using ProtParam in online software EXPASY (https://web.expasy.org/protparam/). The subcellular localization of MdMSIs was predicted by online software WoLF PSORT (https://www.genscript.com/wolf-psort.html) (Horton et al., 2007).
Domain Analysis and Multi-Sequence Alignment of MdMSIs
MdMSI protein sequence alignment was performed by online software Clustal Omega (https://www.ebi.ac.uk/Tools/msa/clustalo/) and visualized by Jalview (Jalview 2.11.1.4).
Phylogenetic Tree and Conserved Motif Analysis of MdMSIs
MdMSI protein sequences were downloaded from the GDDH13 website, AtMSIs from the TAIR (http://www.Arabidopsis.org/), and OsMSIs from the Phytozome (https://phytozome.jgi.doe.gov/pz/portal.html). All MSI protein sequences of the apple, Arabidopsis, and rice were constructed using a phylogenetic tree by software Mega7 (Kumar et al., 2016). The phylogenetic tree was constructed by using the NJ method (Bootstrap set to 1,000), and 10 conserved motifs of MdMSIs and AtMSIs were analyzed by online software MEME (http://meme-suite.org/) (Bailey et al., 2006).
MdMSI Protein Structure Prediction and Protein Interaction Analysis
The secondary structures of MdMSI proteins were predicted by online software SOPMA (https://npsa-prabi.ibcp.fr/cgi-bin/npsa_automat.pl?page=npsa_sopma.html). The tertiary structures of MdMSI proteins were predicted by homology modeling of online software Phyre2 (http://www.sbg.bio.ic.ac.uk/phyre2/html/page.cgi? id=index) (Kelley et al., 2015).
The interaction of AtMSI member-related proteins was predicted by online software STRING (https://stringdb.org) (Szklarczyk et al., 2019), and then, the related homologous apple MSI members were matched.
Chromosome Mapping and Gene Structure Analysis of MSIs in Apples
The chromosome position of apple MSI family members was visually analyzed by online software MG2C (http://mg2c.iask.in/mg2c_v2.1/). Using gene annotation information, the gene structure of MSI family members was analyzed by online software GSDS 2.0 (http://gsds.gao-lab.org/) (Hu et al., 2015).
Promoter Analysis of MdMSIs
The promoter region of about 2000 bp upstream of the apple MSI gene was submitted to the online software PlantCARE (http://bioinformatics.psb.ugent.be/webtools/plantcare/html/) (Lescot et al., 2002) for cis-acting element analysis.
Quantitative Real-Time PCR Analysis
RNA was extracted from the apple material using the RNA plant plus Kit (Tiangen, Beijing), cDNA was obtained by reverse transcription of the extracted RNA using the primescript RT reagent kit a with gDNA eraser (Takara, Dalian), qRT-PCR was performed on an iCycler iQ5 system (Bio-RAD) instrument, and data on relative gene expressions were analyzed by 2−ΔΔCT methods. The quantitative primer design for the MSI genes in the apple was performed by using online software Primer3Plus (http://primer3plus.com/cgi-bin/dev/primer3plus.cgi) (Untergasser et al., 2007), as shown in Supplementary Table S1.
Statistical Analysis
We performed data significance analysis by DPS software. According to the Tukey-Kramer test, it indicated that different lowercase letters represent significant differences. Each experiment was repeated at least three times.
Results
Identification and Basic Information of MdMSIs
After stringent dereplication screening using the online software SMART and Pfam with AtMSIs as the query sequence, eight MdMSI members were finally identified from the “Golden Delicious” apple genome sequence, which were sequentially named MdMSI1-1 (MD02G1228600), MdMSI1-2 (MD07G1083900), MdMSI2 (MD15G1206400), MdMSI3-1 (MD05G1121700), MdMSI3-2 (MD10G1124300), MdMSI4-1 (MD02G1079600), MdMSI4-2 (MD09G1193500), and MdMSI4-3 (MD15G1207100) based on their homology to Arabidopsis MSI family genes. Using the genome annotation file and protein analysis website, the gene length, coding sequence, amino acid number, molecular weight, isoelectric point (pI), and subcellular localization of MdMSI genes were statistically analyzed. It was found that the length of MdMSI genes ranged from 2,673 to 5,972 bp: the shortest was MdMSI3-2 and the longest was MdMSI4-2. The length of the coding sequence ranged from 1,080 to 1,557 bp: the shortest was MdMSI3-2 and the longest was MdMSI4-1. The number range of amino acids was 359–518, the molecular weight was 40,171.82–57,353.08 Da, the isoelectric point was 4.60–6.08, and the pI was less than 7. By using online software WoLF PSORT, the subcellular localization of MdMSIs was predicted to be all in the nucleus (Table 1).
Protein Domain Analysis and Multi-Sequence Alignment of MdMSIs
In the process of protein domain query by online software SMART, it was found that the eight MSI proteins of apple and the five MSI proteins of Arabidopsis contain two typical domains: one was the CAF1C_H4-bd domain, and the other was a typical WD40 domain (Figure 1). The MSI protein sequences of Arabidopsis and apple were compared by online software Clustal Omega and visualized by using the software tool Jalview 2.11.1.4. Arabidopsis and apple MSI proteins had only one highly conserved CAF1C_H4-bd domain. Another WD40 domain was composed of about 40 amino acids.
Evolutionary Tree and Motif Analysis of MdMSIs
The phylogenetic tree was constructed by using apple, Arabidopsis, and rice MSIs, and the apple MSI was grouped according to the classification of Arabidopsis MSI. MSI were divided into three categories, MdMSI1-1 and MdMSI1-2 were divided into one category (I); MdMSI2, MdMSI3-1, and MdMSI3-2 were divided into one category (II); and MdMSI4-1, MdMSI4-2, and MdMSI4-3 were divided into one category (III) (Figure 2).
Using the online software MEME, we predicted 10 motifs in the MdMSI and AtMSI proteins (Figure 3). The motif distribution of MSI proteins was similar. All MSI proteins contain motifs 1, 5, and 6. However, there are also differences in the composition of MSI protein motifs in different groups of the evolutionary tree (Figure 2). For example, motif 7 was detected only in members of (III) (MdMSI4-1, MdMSI4-2, and MdMSI4-3 in apple and AtMSI4 and AtMSI5 in Arabidopsis).
Protein Structure Prediction of MdMSIs
The secondary structure of MdMSI protein was compared. The largest proportion of the secondary structure of eight proteins was random coil, followed by α-helix, and the smallest proportion was β-turn. Moreover, these proteins had roughly similar proportions of the four secondary structure elements (Table 2). The online software Phyre2 was used for the homology model to predict MdMSI families, and the results showed that the tertiary structure of the protein-conserved region of the MdMSI family was very consistent (Figure 4). The special structure was often related to its function, indicating that their function was very likely to be similar.
Construction of MdMSI Protein Interaction Protein Network
By using online software STRING, the protein network and potential function of the MdMSI protein interaction were predicted. MdMSI1-1 and MdMSI1-2 correspond to AtMSI1; MdMSI2 corresponds to AtMSI2; MdMSI3-1 and MdMSI3-2 correspond to AtMSI3; and MdMSI4-1, MdMSI4-2, and MdMSI4-3 correspond to AtFVE (Figure 5). According to the predicted results of the Arabidopsis MSI protein interaction network, AtMSI was associated with FIE protein, SWN protein, VRN2 protein, RNR1 protein, PCNA1 protein, and PCNA2 protein. In addition to interacting with reproductive development-related proteins, such as the FIE protein, SWN protein, and VRN2 protein, it was also associated with stress-related proteins, such as chloroplast posttranscriptional regulation-related protein RNR1 and PCNA1/2 protein interaction, which played an important role in DNA damage response. It showed that the MSI protein was regulated by multiple protein interactions.
Chromosome Location and Gene Structure Analysis of MSIs in Apple
Chromosome location and gene structure analysis of MdMSI genes showed that they were unevenly distributed on six chromosomes, of which only one gene was distributed on chr05, chr07, chr09, and chr10, which were MdMSI3-1, MdMSI1-2, MdMSI4-2, and MdMSI3-2 respectively. There were two genes on chr02 and chr15, respectively, MdMSI1-1 and MdMSI4-1, MdMSI2 and MdMSI4-3 (Figure 6A). The online software GSDS 2.0 was used to visually analyze the gene structure. The number of exons of the gene family was 6–15, and the number of introns was 5–14. It was very interesting that MdMSI1-1 and MdMSI1-2, MdMSI3-1, and MdMSI3-2 corresponding to AtMSI1 and AtMSI3, respectively, had six exons and five introns (Figure 6B). According to the analysis of the aforementioned apple MSI phylogenetic tree, it was speculated that the two groups of genes were likely to have similar functions. In addition, the three genes corresponding to AtMSI4 also had the same exon and intron, and their numbers are 15 and 14, respectively.
Cis-Element Analysis of MdMSI
The promoter of MSI genes in apples was analyzed (Table 3). It was detected that there were a large number of cis-acting elements in response to hormones and stress, such as the ABRE element in response to abscisic acid, CGTCA of jasmonic acid, ERE of ethylene, P-box and GARE motif of gibberellin, TCA of salicylic acid, and TGA of auxin, and were in response to hypoxia, MBS in drought, TC-rich repeats of defense and stress, etc. In conclusion, it was speculated that apple MSI may play an important role in abiotic stress.
Expression of MdMSI Genes in Different Tissues
In order to explore the temporal and spatial expression of MdMSIs in apple, they were analyzed by qRT PCR in roots, stems, leaves, flowers, peels, and pulps. MdMSIs were expressed in all tested tissues (Figure 7). MdMSI1-1, MdMSI2, MdMSI3-1, and MdMSI4-1 were the most expressed in roots. The expression of MdMSI1-1 in roots was about two times higher than that in other tissues. Moreover, MdMSI1-2, MdMSI3-2, and MdMSI4-3 were highly expressed in stems and peels. They all had a low expression level in the leaf.
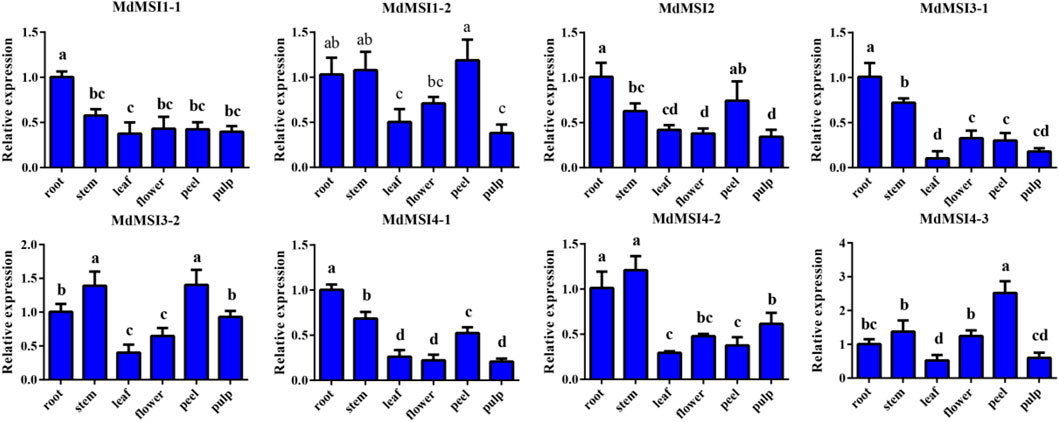
FIGURE 7. Relative expression levels of MdMSIs in different tissues. Different lowercase letters represent a significant difference (p < 0.05).
Expression Analysis of MdMSIs Under Abiotic Stress and Abscisic Acid
In order to study the response of apple MSI genes to abiotic stress, the seedlings of apple were treated with salt and drought. After salt stress, the relative expression levels of the other seven genes reached the maximum at 12 h, except MdMSI3-2 (Figure 8A). In addition, it was very interesting that the relative expression of the MdMSI gene family decreased first and then increased after salt stress treatment, except MdMSI3-1 and MdMSI4-1. After drought treatment, except MdMSI3-2 and MdMSI4-2, the overall expression of the other six genes in the MdMSI gene family showed an upward trend (Figure 8B). In addition, many cis-elements related to hormones were found in the analysis of the apple MSI promoter. Among them, the number and range of ABA were the most extensive in the MdMSI gene family. Therefore, the seedlings of apple were also treated with ABA. The expressions of MdMSI1-1, MdMSI1-2, MdMSI3-1, MdMSI3-2, MdMSI4-2, and MdMSI4-3 were similar, and they first showed an upward trend, peaked at 6 h, and then decreased (Figure 8C). The expression of MdMSI4-1 reached the highest levels at 3 h.
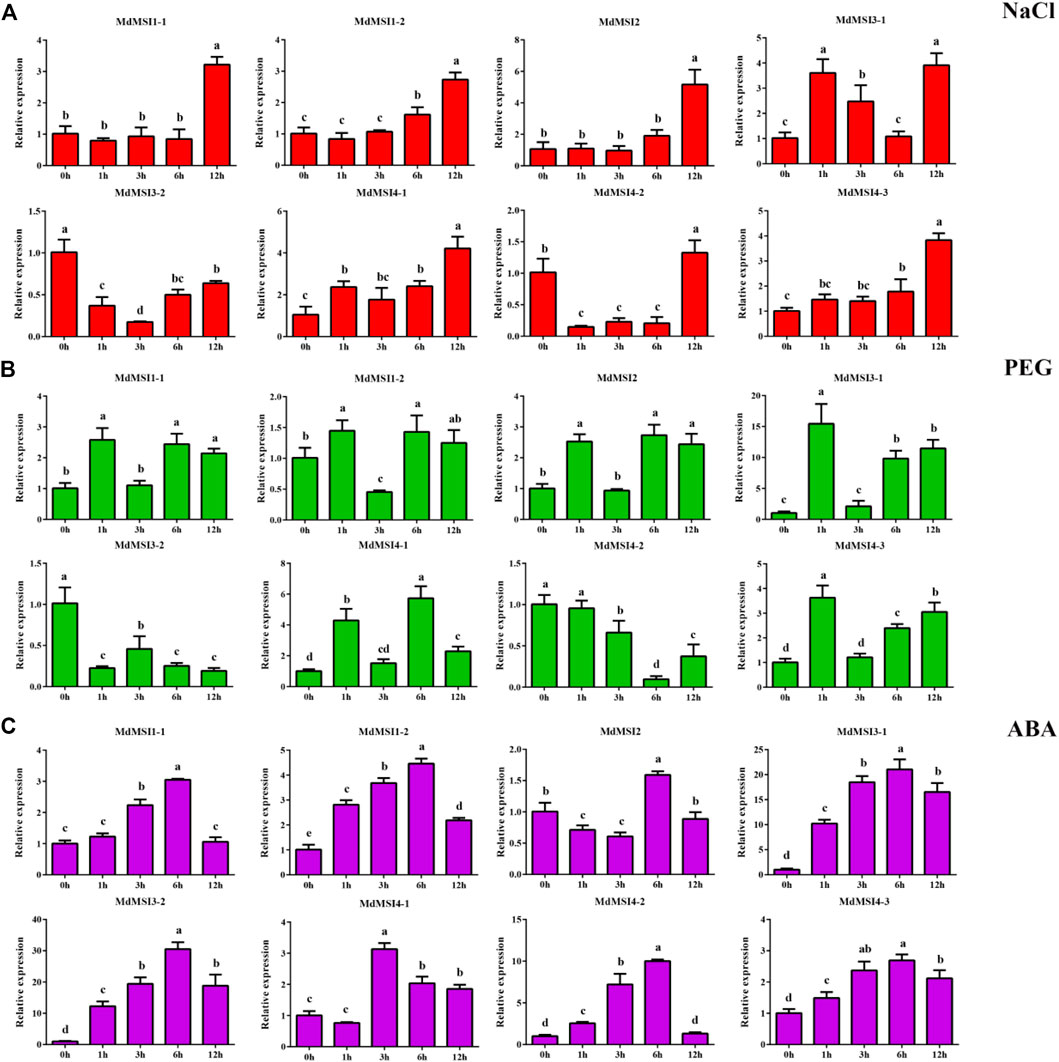
FIGURE 8. Expression analysis of MdMSI genes under different stress conditions. Expression of MdMSIs under (A) NaCl, (B) PEG, and (C) ABA conditions. Different lowercase letters represent a significant difference (p < 0.05).
MdMSI1-1 Overexpression Increases Sensitivity to Salt Stress in Transgenic Apple Calli and Arabidopsis
Among the MdMSIs promoters, a large number of cis-elements related to hormones and abiotic stress were identified in MdMSIs promoters, indicating that the family genes may be involved in abiotic stress response (Table 3). There were a lot of abiotic stress-related elements in the MdMSI1-1 promoter sequence, and its expression level was significantly affected by salt stress. Therefore, we predicted that it was very likely to participate in salt stress. We obtained three transgenic apple callus lines of overexpressed MdMSI1-1 (MdMSI1-1-OX1, MdMSI1-1-OX2, and MdMSI1-1-OX3) (Figure 9D). Under the control condition, there was no significant difference in fresh weight and MDA between WT and MdMSI1-1-OX, but under the salt treatment condition, the fresh weight of MdMSI1-1-OX was significantly lower than WT, and MDA was significantly higher than WT (Figures 9A–C). The results showed that MdMSI1-1 negatively regulated salt stress in apple.
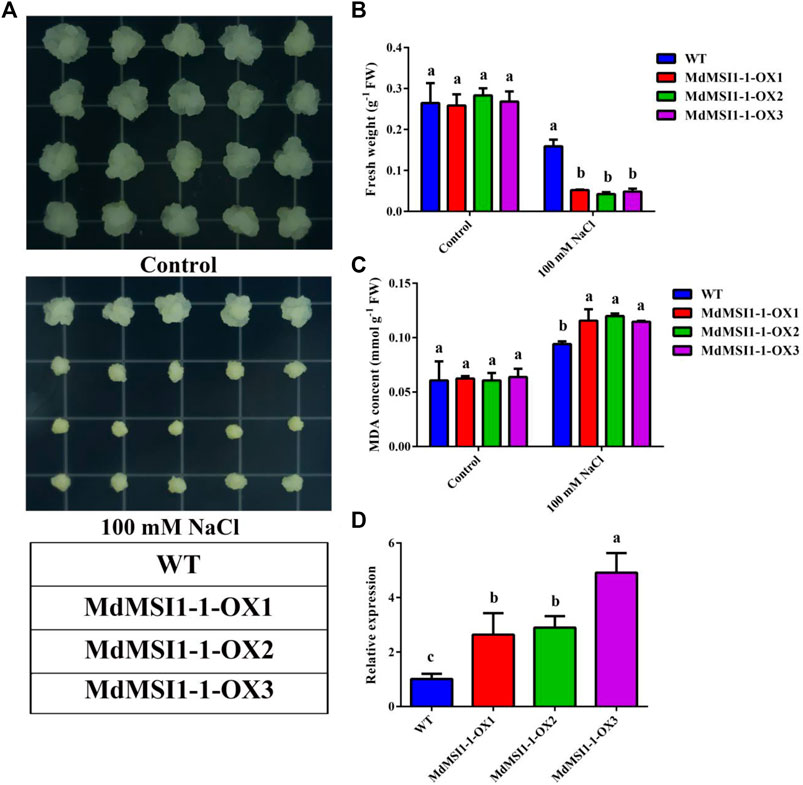
FIGURE 9. MdMSI1-1 increased sensitivity to salt stress in apple calli. (A) The phenotypes of 16-day-old WT and MdMSI1-1-OX calli in MS, or MS + 100 mM NaCl, respectively. (B) fresh weight and (C) MDA content in apple calli after treatment (MS, or MS + 100 mM NaCl). (D) The expression level of MdMSI1-1 in WT and MdMSI1-1-OX calli. Different lowercase letters represent a significant difference (p < 0.05).
In order to further verify the function of MdMSI1-1 in salt stress, we obtained three MdMSI1-1 overexpressions in Arabidopsis (OE1, OE2, and OE3) (Figure 10B). We treated 3-day-old Arabidopsis seedlings (WT and MdMSI1-1-OE) with 100 mM NaCl and found that MdMSI1-1-OE had lighter fresh weight and shorter primary root length than WT (Figures 10A,C,D). Moreover, we detected the relative electronic conductivity in Arabidopsis (Figure 10E). The relative electronic conductivity of MdMSI1-1-OE was higher than that of WT. These results showed that MdMSI1-1 increased the sensitivity of apple calli and Arabidopsis under salt stress.
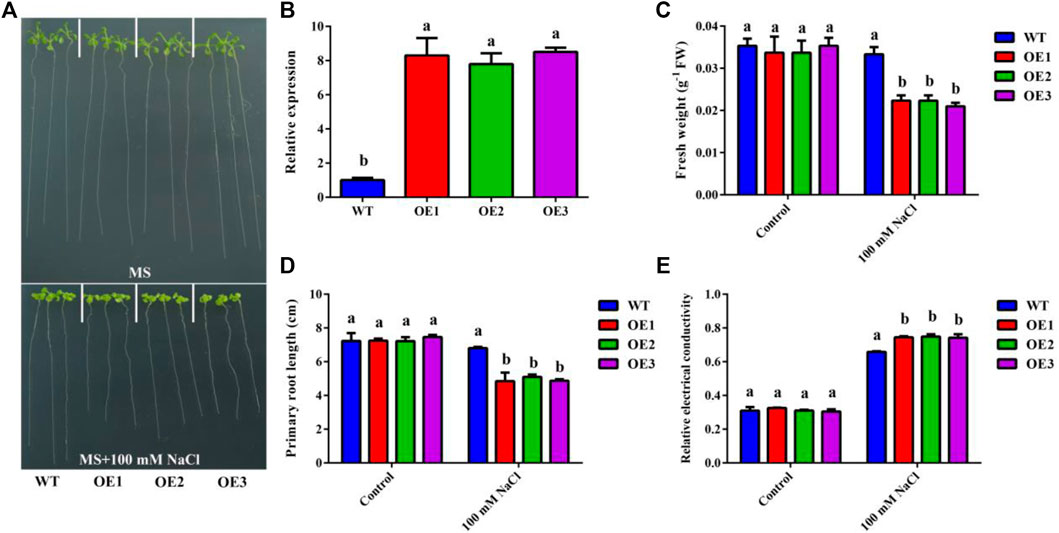
FIGURE 10. Ectopic expression of MdMSI1-1 negatively regulated salt tolerance in Arabidopsis. (A) The phenotypes of Arabidopsis seedlings (WT, and MdMSI1-1-OE) treated with MS, or MS + 100 mM NaCl treatment. (B) Expression of MdMSI1-1in Arabidopsis (WT, and MdMSI1-1-OE). (C) fresh weight, (D) primary root length, and (E) relative electronic conductivity in WT and transgenic Arabidopsis after treatment (MS, or MS + 100 mM NaCl). Different lowercase letters represent a significant difference (p < 0.05).
Discussion
WD40 repeat proteins widely exist in eukaryotes (Janda et al., 1996), and its function has been reported in many species, such as Arabidopsis (Xiong et al., 2005; Chen et al., 2008; Biedermann and Hellmann 2010; Lee et al., 2010; Chen and Brandizzi 2012), rice (Raghuvanshi et al., 2001), Petunia hybrid (de Vetten et al., 1997), corn (Carey et al., 2004), and pomegranate (Ben-Simhon et al., 2011). The IRA multi copy inhibitor (MSI) belongs to the subfamily of the WD40 repeat protein family. In this study, MSI in apple was comprehensively characterized, followed by strict screening and identification, and finally, eight MdMSI members were screened (Table 1). There are five MSI genes in Arabidopsis (Ruggieri et al., 1989) and six in longan (Shen et al., 2019), thus making it clear that there are differences in the number of MSI family members among different species. The number of MSI family members in apple is more than that in Arabidopsis, rice, and longan, indicating a more complex genome in apple.
Through domain analysis and multi-sequence alignment of MSI protein in Arabidopsis and apple, it was found that they all have a CAF1C_H4-bd-conserved domain and WD40 domain, indicating that they are highly conservative (Figure 1). CAF1C_H4-bd is a subunit of the CAF-1 complex, and CAF-1 is mainly involved in chromatin formation during DNA replication and damage repair (Winkler et al., 2012). Therefore, it was initially believed that MSI genes play an important role in apple growth and stress. A large number of studies have shown that CAF-1 exists conservatively in various species (Yu et al., 2015). Previous studies have shown that MSI1 is also involved in the formation of the CAF-1 complex and MSI1-RBR1 complex (Ach et al., 1997; Exner et al., 2006; Jullien et al., 2008; Kaya et al., 2001). Furthermore, MdMSIs were predicted to be located in the nucleus (Table 1). Based on phylogenetic analysis and genetic evolution analysis of Arabidopsis, we divided the MdMSI proteins into three classes (Figure 2) (Hennig et al., 2005). The same class of proteins has similar secondary and tertiary structures, indicating that the same class of proteins may have the same function (Table 2 and Figure 4).
Previous studies have shown that MSI has been widely reported in reproduction, such as endosperm formation, regulation of flowering process, and pollen development (Bouveret et al., 2006; Pazhouhandeh et al., 2011; Gao et al., 2010; Rodrigues et al., 2010; Dumbliauskas et al., 2011; Mozgová et al., 2010). In addition, the MSI family also plays an important role in abiotic stress, especially salt and drought stress (Mehdi et al., 2016; Kenzior and Folk 2015; Kim et al., 2004; Lu et al., 2021). However, MdMSIs on abiotic stress have not been described in detail. Tissue expression analysis showed that all MdMSI genes were constitutively expressed in the tissues examined, with relatively highly expressed levels in roots, indicating that MdMSIs may play an important role in abiotic stress (Figure 7). Similarly, we showed that MdMSIs responded to salt, drought stress, and ABA by qRT-PCR (Figure 8). Plants face some abiotic stresses through a large number of transcriptional reactions in stress. However, transcriptional regulation is largely controlled by chromatin (Aditya and Aryadeep, 2017), and the MSI gene family is a part of protein complexes involved in the chromatin assembly. Moreover, analysis of the MdMSI promoters revealed the presence of a large number of cis-acting elements in this family that respond to hormones with adversity, such as ABA. ABA is an important regulator of growth inhibition and plays an irreplaceable role in regulating various stresses (Cutler et al., 2010; Nakashima and Yamaguchi-Shinozaki 2013; Ye et al., 2012). qRT-PCR and promoter analysis further confirmed that apple MSIs may play an irreplaceable role in plants facing abiotic stress. In addition, the expression of MdMSI1-1 was the highest in roots (Figure 7), and MdMSI1-1 could be significantly induced under salt stress (Figure 8A), indicating that it may be involved in salt stress response because roots are the first plant organs to suffer salt stress (Xing et al., 2021). Here, we obtained transgenic apple calli and ectopical MdMSI1-1-OE Arabidopsis. Overexpressing MdMSI1-1 negatively regulated salt tolerance (Figures 9, 10). It is reported that GmNFYA interacts with GmFVE to jointly regulate salt stress (Lu et al., 2021), and AtMSI1 has a negative regulatory effect on drought stress (Alexandre et al., 2009).
Conclusion
In conclusion, eight MdMSI genes were identified in the apple. We studied the function of MdMSI genes in apple growth and development by bioinformatics, gene expression, and functional analysis. Functional characterization showed that MdMSIs may play an important role in salt stress. It will provide a basis for future studies to comprehensively and comparatively analyze the functional characteristics of MSI and to deeply investigate aspects of abiotic stress in the apple.
Data Availability Statement
The original contributions presented in the study are included in the article/Supplementary Material, further inquiries can be directed to the corresponding authors.
Author Contributions
Data curation, DW; Formal analysis, XuW, CZ, KY, XiW, and JC; Funding acquisition, CY; Investigation, DL and CY; Resources, CY; Visualization, DW and XuW; Writing—original draft, DW.
Funding
This research was supported by the National Natural Science Foundation of China (31772288, 32172538) and the National Key R&D Program of China (2018YFD1000100).
Conflict of Interest
The authors declare that the research was conducted in the absence of any commercial or financial relationships that could be construed as a potential conflict of interest.
Publisher’s Note
All claims expressed in this article are solely those of the authors and do not necessarily represent those of their affiliated organizations, or those of the publisher, the editors, and the reviewers. Any product that may be evaluated in this article, or claim that may be made by its manufacturer, is not guaranteed or endorsed by the publisher.
Supplementary Material
The Supplementary Material for this article can be found online at: https://www.frontiersin.org/articles/10.3389/fgene.2022.846321/full#supplementary-material
Supplementary Table S1 | Primers for quantitative real-time PCR.
References
Ach, R. A., Taranto, P., and Gruissem, W. (1997). A Conserved Family of WD-40 Proteins Binds to the Retinoblastoma Protein in Both Plants and Animals. Plant Cell 9 (9), 1595–1606. doi:10.1105/tpc.9.9.1595
Aditya, B., and Aryadeep, R. (2017) Epigenetic Regulation during Salinity and Drought Stress in Plants: Histone Modifications and DNA Methylation. Plant Gene.
Alexandre, C., Möller-Steinbach, Y., Schönrock, N., Gruissem, W., and Hennig, L. (2009). Arabidopsis MSI1 Is Required for Negative Regulation of the Response to Drought Stress. Mol. Plant 2 (4), 675–687. doi:10.1093/mp/ssp012
An, J.-P., Li, H.-H., Song, L.-Q., Su, L., Liu, X., You, C.-X., et al. (2016). The Molecular Cloning and Functional Characterization of MdMYC2, a bHLH Transcription Factor in Apple. Plant Physiol. Biochem. 108, 24–31. doi:10.1016/j.plaphy.2016.06.032
Bailey, T. L., Williams, N., Misleh, C., and Li, W. W. (2006). MEME: Discovering and Analyzing DNA and Protein Sequence Motifs. Nucleic Acids Res. 34, W369–W373. doi:10.1093/nar/gkl198
Ben-Simhon, Z., Judeinstein, S., Nadler-Hassar, T., Trainin, T., Bar-Ya’akov, I., Borochov-Neori, H., et al. (2011). A Pomegranate (Punica Granatum L.) WD40-Repeat Gene Is a Functional Homologue of Arabidopsis TTG1 and Is Involved in the Regulation of Anthocyanin Biosynthesis during Pomegranate Fruit Development. Planta 234 (5), 865–881. doi:10.1007/s00425-011-1438-4
Biedermann, S., and Hellmann, H. (2010). The DDB1a Interacting Proteins ATCSA-1 and DDB2 Are Critical Factors for UV-B Tolerance and Genomic Integrity in Arabidopsis thaliana. Plant J. 62 (3), 404–415. doi:10.1111/j.1365-313X.2010.04157.x
Bouveret, R., Schönrock, N., Gruissem, W., and Hennig, L. (2006). Regulation of Flowering Time byArabidopsis MSI1. Development 133 (9), 1693–1702. doi:10.1242/dev.02340
Carey, C. C., Strahle, J. T., Selinger, D. A., and Chandler, V. L. (2004). Mutations in the Pale Aleurone Color1 Regulatory Gene of the Zea mays Anthocyanin Pathway Have Distinct Phenotypes Relative to the Functionally Similar TRANSPARENT TESTA GLABRA1 Gene in Arabidopsis thaliana [W]. Plant Cell 16 (2), 450–464. doi:10.1105/tpc.018796
Chen, Y., and Brandizzi, F. (2012). AtIRE1A/AtIRE1B and AGB1 Independently Control Two Essential Unfolded Protein Response Pathways in Arabidopsis. Plant J. 69 (2), 266–277. doi:10.1111/j.1365-313X.2011.04788.x
Chen, Z.-H., Jenkins, G. I., and Nimmo, H. G. (2008). Identification of an F-Box Protein that Negatively Regulates Pi Starvation Responses. Plant Cel Physiol 49 (12), 1902–1906. doi:10.1093/pcp/pcn157
Cheng, Y., Zhu, W., Chen, Y., Ito, S., Asami, T., and Wang, X. (2014) Brassinosteroids Control Root Epidermal Cell Fate via Direct Regulation of a MYB-bHLH-WD40 Complex by GSK3-like Kinases, 3, 2525. doi:10.7554/eLife.02525
Cui, B., Huang, M., Guo, C., Li, R., and Wang, Y. (2022). Cloning and Expression Analysis of DnMSI1 Gene in Orchid Species Dendrobium Nobile Lindl. Plant Signaling Behav. 10, 2021649. doi:10.1080/15592324.2021.2021649
Cutler, S. R., Rodriguez, P. L., Finkelstein, R. R., and Abrams, S. R. (2010). Abscisic Acid: Emergence of a Core Signaling Network. Annu. Rev. Plant Biol. 61, 651–679. doi:10.1146/annurev-arplant-042809-112122
Daccord, N., Celton, J.-M., Linsmith, G., Becker, C., Choisne, N., Schijlen, E., et al. (2017). High-quality De Novo Assembly of the Apple Genome and Methylome Dynamics of Early Fruit Development. Nat. Genet. 49 (7), 1099–1106. doi:10.1038/ng.3886
de Vetten, N., Quattrocchio, F., Mol, J., and Koes, R. (1997). The An11 Locus Controlling Flower Pigmentation in Petunia Encodes a Novel WD-Repeat Protein Conserved in Yeast, Plants, and Animals. Genes Dev. 11 (11), 1422–1434. doi:10.1101/gad.11.11.1422
Derkacheva, M., Steinbach, Y., Wildhaber, T., Mozgová, I., Mahrez, W., Nanni, P., et al. (2013). Arabidopsis MSI1 Connects LHP1 to PRC2 Complexes. Embo j 32 (14), 2073–2085. doi:10.1038/emboj.2013.145
Dumbliauskas, E., Lechner, E., Jaciubek, M., Berr, A., Pazhouhandeh, M., Alioua, M., et al. (2011). The Arabidopsis CUL4-DDB1 Complex Interacts with MSI1 and Is Required to maintainMEDEAparental Imprinting. Embo j 30 (4), 731–743. doi:10.1038/emboj.2010.359
Exner, V., Taranto, P., Schönrock, N., Gruissem, W., and Hennig, L. (2006). Chromatin Assembly Factor CAF-1 Is Required for Cellular Differentiation during Plant Development. Development 133 (21), 4163–4172. doi:10.1242/dev.02599
Finn, R. D., Bateman, A., Clements, J., Coggill, P., Eberhardt, R. Y., Eddy, S. R., et al. (2014). Pfam: the Protein Families Database. Nucl. Acids Res. 42 (Database issue), D222–D230. doi:10.1093/nar/gkt1223
Gao, Y., Hong-Liang, X. U., Ya-Xuan, L. I., and Ying-Kao, H. U. (2010) Cloning and Sequence Analysis of FIE and MSI1 Genes from Nicotiana Tabacum. Acta Botanica Boreali-Occidentalia Sinica.
Guitton, A.-E., and Berger, F. (2005). Loss of Function of MULTICOPY SUPPRESSOR of IRA 1 Produces Nonviable Parthenogenetic Embryos in Arabidopsis. Curr. Biol. 15 (8), 750–754. doi:10.1016/j.cub.2005.02.066
Hennig, L., Bouveret, R., and Gruissem, W. (2005). MSI1-like Proteins: an Escort Service for Chromatin Assembly and Remodeling Complexes. Trends Cel Biol. 15 (6), 295–302. doi:10.1016/j.tcb.2005.04.004
Horton, P., Park, K.-J., Obayashi, T., Fujita, N., Harada, H., Adams-Collier, C. J., et al. (2007). WoLF PSORT: Protein Localization Predictor. Nucleic Acids Res. 35 (Web Server issue), W585–W587. doi:10.1093/nar/gkm259
Hu, B., Jin, J., Guo, A.-Y., Zhang, H., Luo, J., and Gao, G. (2015). GSDS 2.0: an Upgraded Gene Feature Visualization Server. Bioinformatics 31 (8), 1296–1297. doi:10.1093/bioinformatics/btu817
Hu, W., Huang, C., Deng, X., Zhou, S., Chen, L., Li, Y., et al. (2013). TaASR1, a Transcription Factor Gene in Wheat, Confers Drought Stress Tolerance in Transgenic Tobacco. Plant Cel Environ 36 (8), 1449–1464. doi:10.1111/pce.12074
Jaleel, C. A., Manivannan, P., Wahid, A., Farooq, M., Al-Juburi, H. J., Somasundaram, R., et al. (2009). Drought Stress in Plants: A Review on Morphological Characteristics and Pigments Composition. Int. J. Agric. Biol. 11 (1), 100
Janda, L., Tichý, P., Spízek, J., and Petrícek, M. (1996). A Deduced Thermomonospora Curvata Protein Containing Serine/threonine Protein Kinase and WD-Repeat Domains. J. Bacteriol. 178 (5), 1487–1489. doi:10.1128/jb.178.5.1487-1489.1996
Jeon, J., and Kim, J. (2011). FVE, an Arabidopsis Homologue of the Retinoblastoma-Associated Protein that Regulates Flowering Time and Cold Response, Binds to Chromatin as a Large Multiprotein Complex. Mol. Cell 32 (3), 227–234. doi:10.1007/s10059-011-1022-6
Jiang, D., Gu, X., and He, Y. (2009). Establishment of the Winter-Annual Growth Habit via FRIGIDA-Mediated Histone Methylation at FLOWERING LOCUS C in Arabidopsis. Plant Cell 21 (6), 1733–1746. doi:10.1105/tpc.109.067967
Jiang, D., Kong, N. C., Gu, X., Li, Z., and He, Y. (2011). Arabidopsis COMPASS-like Complexes Mediate Histone H3 Lysine-4 Trimethylation to Control floral Transition and Plant Development. Plos Genet. 7 (3), e1001330. doi:10.1371/journal.pgen.1001330
Jullien, P. E., Mosquna, A., Ingouff, M., Sakata, T., Ohad, N., and Berger, F. (2008). Retinoblastoma and its Binding Partner MSI1 Control Imprinting in Arabidopsis. Plos Biol. 6 (8), e194. doi:10.1371/journal.pbio.0060194
Kaya, H., Shibahara, K.-i., Taoka, K.-i., Iwabuchi, M., Stillman, B., and Araki, T. (2001). FASCIATA Genes for Chromatin Assembly Factor-1 in Arabidopsis Maintain the Cellular Organization of Apical Meristems. Cell 104 (1), 131–142. doi:10.1016/s0092-8674(01)00197-0
Kelley, L. A., Mezulis, S., Yates, C. M., Wass, M. N., and Sternberg, M. J. E. (2015). The Phyre2 Web portal for Protein Modeling, Prediction and Analysis. Nat. Protoc. 10 (6), 845–858. doi:10.1038/nprot.2015.053
Kenzior, A., and Folk, W. R. (2015). Arabidopsis thaliana MSI4/FVE Associates with Members of a Novel Family of Plant Specific PWWP/RRM Domain Proteins. Plant Mol. Biol. 87 (4-5), 329–339. doi:10.1007/s11103-014-0280-z
Kim, H.-J., Hyun, Y., Park, J.-Y., Park, M.-J., Park, M.-K., Kim, M. D., et al. (2004). A Genetic Link between Cold Responses and Flowering Time through FVE in Arabidopsis thaliana. Nat. Genet. 36 (2), 167–171. doi:10.1038/ng1298
Kumar, S., Stecher, G., and Tamura, K. (2016). MEGA7: Molecular Evolutionary Genetics Analysis Version 7.0 for Bigger Datasets. Mol. Biol. Evol. 33 (7), 1870–1874. doi:10.1093/molbev/msw054
Lee, I., Ambaru, B., Thakkar, P., Marcotte, E. M., and Rhee, S. Y. (2010). Rational Association of Genes with Traits Using a Genome-Scale Gene Network for Arabidopsis thaliana. Nat. Biotechnol. 28 (2), 149–156. doi:10.1038/nbt.1603
Lescot, M., Déhais, P., Thijs, G., Marchal, K., Moreau, Y., Van de Peer, Y., et al. (2002). PlantCARE, a Database of Plant Cis-Acting Regulatory Elements and a portal to Tools for In Silico Analysis of Promoter Sequences. Nucleic Acids Res. 30 (1), 325–327. doi:10.1093/nar/30.1.325
Liu, W. C., Zheng, S. Q., Yu, Z. D., Gao, X., Shen, R., and Lu, Y. T. (2018). WD 40‐ REPEAT 5a Represses Root Meristem Growth by Suppressing Auxin Synthesis through Changes of Nitric Oxide Accumulation in Arabidopsis. Plant J. 93 (5), 883–893. doi:10.1111/tpj.13816
Lu, L., Wei, W., Tao, J. J., Lu, X., Bian, X. H., Hu, Y., et al. (2021). Nuclear Factor Y Subunit GmNFYA Competes with GmHDA13 for Interaction with GmFVE to Positively Regulate Salt Tolerance in Soybean. Plant Biotechnol. J. 19 (11), 2362–2379. doi:10.1111/pbi.13668
Ma, Q.-J., Sun, M.-H., Lu, J., Liu, Y.-J., You, C.-X., and Hao, Y.-J. (2017). An Apple CIPK Protein Kinase Targets a Novel Residue of AREB Transcription Factor for ABA-dependent Phosphorylation. Plant Cel Environ. 40 (10), 2207–2219. doi:10.1111/pce.13013
Manickavelu, A., Nadarajan, N., Ganesh, S. K., Gnanamalar, R. P., and Babu, R. C. (2006). Drought Tolerance in rice: Morphological and Molecular Genetic Consideration. Plant Growth Regul. 50 (2-3), 121–138. doi:10.1007/s10725-006-9109-3
Mehdi, S., Derkacheva, M., Ramström, M., Kralemann, L., Bergquist, J., and Hennig, L. (2016). The WD40 Domain Protein MSI1 Functions in a Histone Deacetylase Complex to Fine-Tune Abscisic Acid Signaling. Plant Cell 28 (1), 42–54. doi:10.1105/tpc.15.00763
Mozgová, I., Mokroš, P., and Fajkus, J. (2010). Dysfunction of Chromatin Assembly Factor 1 Induces Shortening of Telomeres and Loss of 45S rDNA inArabidopsis Thaliana. Plant Cell 22 (8), 2768–2780. doi:10.1105/tpc.110.076182
Nakashima, K., and Yamaguchi-Shinozaki, K. (2013). ABA Signaling in Stress-Response and Seed Development. Plant Cel Rep 32 (7), 959–970. doi:10.1007/s00299-013-1418-1
Pazhouhandeh, M., Molinier, J., Berr, A., and Genschik, P. (2011). MSI4/FVE Interacts with CUL4-DDB1 and a PRC2-like Complex to Control Epigenetic Regulation of Flowering Time in Arabidopsis. Proc. Natl. Acad. Sci. 108 (8), 3430–3435. doi:10.1073/pnas.1018242108
Qi, T., Huang, H., Wu, D., Yan, J., Qi, Y., Song, S., et al. (2014). Arabidopsis DELLA and JAZ Proteins Bind the WD-Repeat/bHLH/MYB Complex to Modulate Gibberellin and Jasmonate Signaling Synergy. Plant Cell 26 (3), 1118–1133. doi:10.1105/tpc.113.121731
Raghuvanshi, S., Kelkar, A., Khurana, J. P., and Tyagi, A. K. (2001). Isolation and Molecular Characterization of the COP 1 Gene Homolog from Rice, Oryza Sativa L. Subsp. Indica Var. Pusa Basmati 1. DNA Res. 8 (2), 73–79. doi:10.1093/dnares/8.2.73
Rodrigues, J. C. M., Okada, T., Johnson, S. D., and Koltunow, A. M. (2010). A MULTICOPY SUPPRESSOR of IRA1 (MSI1) Homologue Is Not Associated with the Switch to Autonomous Seed Development in Apomictic (Asexual) Hieracium Plants. Plant Sci. 179 (6), 590–597. doi:10.1016/j.plantsci.2010.05.005
Rossi, V., Varotto, S., Locatelli, S., Lanzanova, C., Lauria, M., Zanotti, E., et al. (2001). The maize WD-Repeat Gene ZmRbAp1 Encodes a Member of the MSI/RbAp Sub-family and Is Differentially Expressed during Endosperm Development. Mol. Gen. Genomics 265 (4), 576–584. doi:10.1007/s004380100461
Ruggieri, R., Tanaka, K., Nakafuku, M., Kaziro, Y., Toh-e, A., and Matsumoto, K. (1989). MSI1, a Negative Regulator of the RAS-cAMP Pathway in Saccharomyces cerevisiae. Proc. Natl. Acad. Sci. 86 (22), 8778–8782. doi:10.1073/pnas.86.22.8778
Sequera-Mutiozabal, M., Tiburcio, A. F., and Alcázar, R. (2016) Drought Stress Tolerance in Relation to Polyamine Metabolism in Plants. Springer International Publishing.
Shen, X., Chen, X., Xiaoping, X. U., Huo, W., Xiaofei, L. I., Jiang, M., et al. (2019) Genome-wide Identification and Expression Analysis of RNA Methylation Related Genes during Somatic Embryogenesis in Dimocarpus Longan Lour. Chinese Journal of Tropical Crops.
Szklarczyk, D., Gable, A. L., Lyon, D., Junge, A., Wyder, S., Huerta-Cepas, J., et al. (2019). STRING V11: Protein-Protein Association Networks with Increased Coverage, Supporting Functional Discovery in Genome-wide Experimental Datasets. Nucleic Acids Res. 47 (D1), D607–d613. doi:10.1093/nar/gky1131
Tian, M., Lou, L., Liu, L., Yu, F., Zhao, Q., Zhang, H., et al. (2015). The RING finger E3 Ligase STRF1 Is Involved in Membrane Trafficking and Modulates Salt-Stress Response inArabidopsis Thaliana. Plant J. 82 (1), 81–92. doi:10.1111/tpj.12797
Untergasser, A., Nijveen, H., Rao, X., Bisseling, T., Geurts, R., and Leunissen, J. A. M. (2007). Primer3Plus, an Enhanced Web Interface to Primer3. Nucleic Acids Res. 35 (Web Server issue), W71–W74. doi:10.1093/nar/gkm306
Wang, Y., Li, W., Xu, X., Qiu, C., Wu, T., Wei, Q., et al. (2019) Progress of Apple Rootstock Breeding and its Use. Horticultural Plant Journal. (5), 9.
Winkler, D. D., Zhou, H., Dar, M. A., Zhang, Z., and Luger, K. (2012). Yeast CAF-1 Assembles Histone (H3-H4)2 Tetramers Prior to DNA Deposition. Nucleic Acids Res. 40 (20), 10139–10149. doi:10.1093/nar/gks812
Xing, L., Zhu, M., Luan, M., Zhang, M., Jin, L., Liu, Y., et al. (2021) miR169q and NUCLEAR FACTOR YA8 Enhance Salt Tolerance by Activating PEROXIDASE1 Expression in Response to ROS, 188, 608, 623. doi:10.1093/plphys/kiab498
Xiong, Y., Contento, A. L., and Bassham, D. C. (2005). AtATG18a Is Required for the Formation of Autophagosomes during Nutrient Stress and Senescence in Arabidopsis thaliana. Plant J. 42 (4), 535–546. doi:10.1111/j.1365-313X.2005.02397.x
Yamaguchi-Shinozaki, K., and Shinozaki, K. (1994). A Novel Cis-Acting Element in an Arabidopsis Gene Is Involved in Responsiveness to Drought, Low-Temperature, or High-Salt Stress. Plant Cell 6 (2), 251–264. doi:10.1105/tpc.6.2.251
Yang, K., Li, C.-Y., An, J.-P., Wang, D.-R., Wang, X., Wang, C.-K., et al. (2021). The C2H2-type Zinc finger Transcription Factor MdZAT10 Negatively Regulates Drought Tolerance in Apple. Plant Physiol. Biochem. 167, 390–399. doi:10.1016/j.plaphy.2021.08.014
Ye, N., Jia, L., and Zhang, J. (2012). ABA Signal in rice under Stress Conditions. Rice 5 (1), 1. doi:10.1186/1939-8433-5-1
Keywords: apple, MSI, expression pattern, salt stress, phylogenetic tree
Citation: Wang D, Wang X, Zhang C, Yang K, Wang X, Cui J, Liu D and You C (2022) Genome-wide Identification, Expression, and Functional Analysis of MdMSI Genes in Apples (Malus domestica Borkh.). Front. Genet. 13:846321. doi: 10.3389/fgene.2022.846321
Received: 31 December 2021; Accepted: 04 February 2022;
Published: 03 March 2022.
Edited by:
Zefeng Yang, Yangzhou University, ChinaReviewed by:
Muhammad Waseem, Hainan University, ChinaBowen Liang, Hebei Agricultural University, China
Copyright © 2022 Wang, Wang, Zhang, Yang, Wang, Cui, Liu and You. This is an open-access article distributed under the terms of the Creative Commons Attribution License (CC BY). The use, distribution or reproduction in other forums is permitted, provided the original author(s) and the copyright owner(s) are credited and that the original publication in this journal is cited, in accordance with accepted academic practice. No use, distribution or reproduction is permitted which does not comply with these terms.
*Correspondence: Dandan Liu, liudandan@ynu.edu.cn; Chunxiang You, youchunxiang@sdau.edu.cn