- 1Co-Innovation Center for Sustainable Forestry in Southern China, Key Laboratory of Forest Genetics and Biotechnology, College of Biology and the Environment, Nanjing Forestry University, Ministry of Education, Nanjing, China
- 2State Key Laboratory of Tea Plant Biology and Utilization, Anhui Agricultural University, Hefei, China
- 3School of Life Sciences, Anhui Agricultural University, Hefei, China
- 4State Key Laboratory of Subtropical Silviculture, Zhejiang A&F University, Hangzhou, China
- 5Zhejiang Provincial Key Laboratory of Resources Protection and Innovation of Traditional Chinese Medicine, Zhejiang A&F University, Hangzhou, China
Superoxide dismutase (SOD) is an important enzyme that serves as the first line of defense in the plant antioxidant system and removes reactive oxygen species (ROS) under adverse conditions. The SOD protein family is widely distributed in the plant kingdom and plays a significant role in plant growth and development. However, the comprehensive analysis of the SOD gene family has not been conducted in Cucurbitaceae. Subsequently, 43 SOD genes were identified from Cucurbitaceae species [Citrullus lanatus (watermelon), Cucurbita pepo (zucchini), Cucumis sativus (cucumber), Lagenaria siceraria (bottle gourd), Cucumis melo (melon)]. According to evolutionary analysis, SOD genes were divided into eight subfamilies (I, II, III, IV, V, VI, VII, VIII). The gene structure analysis exhibited that the SOD gene family had comparatively preserved exon/intron assembly and motif as well. Phylogenetic and structural analysis revealed the functional divergence of Cucurbitaceae SOD gene family. Furthermore, microRNAs 6 miRNAs were predicted targeting 3 LsiSOD genes. Gene ontology annotation outcomes confirm the role of LsiSODs under different stress stimuli, cellular oxidant detoxification processes, metal ion binding activities, SOD activity, and different cellular components. Promoter regions of the SOD family revealed that most cis-elements were involved in plant development, stress response, and plant hormones. Evaluation of the gene expression showed that most SOD genes were expressed in different tissues (root, flower, fruit, stem, and leaf). Finally, the expression profiles of eight LsiSOD genes analyzed by qRT-PCR suggested that these genetic reserves responded to drought, saline, heat, and cold stress. These findings laid the foundation for further study of the role of the SOD gene family in Cucurbitaceae. Also, they provided the potential for its use in the genetic improvement of Cucurbitaceae.
Introduction
In natural conditions, plants are often susceptible to drought, salt, extreme temperatures, heavy metals, and other stresses that have a significant impact on the growth, development, and production of plants (Mittler and Blumwald, 2010; Cramer et al., 2011). When a plant is stressed, it adjusts its homeostatic apparatus by producing more reactive oxygen species (ROS) in its cells and ROS, toxic-free radicals produced under stress by plant cells that can oxidize the protein, destroy the cell membrane, and cause DNA damage (Lee et al., 2007; Karuppanapandian et al., 2011; Feng et al., 2015). These stresses inevitably accompany the development of ROS, including hydroxyl radicals (OH), superoxide anion radicals (O2−), peroxide radicals (HOO−), hydrogen peroxide (H2O2), and singlet oxygen (1O2), which cause damage to the cell membrane, peroxidization and deterioration of macromolecules, and ultimately lead to the death of cells. ROS are also considered signaling molecules in different organisms and can affect various physiological processes in plants, For example, some prominent active oxygen scavengers can resist environmental stresses by regulating the expression of enzyme reaction family genes such as superoxide dismutase (SOD), catalase (CAT), peroxidase (POD), glutathione peroxidase (GPX), and peroxidase (PrxR) (Mittler et al., 2004; Ahmad et al., 2010; Bafana et al., 2011; Filiz and Tombuloğlu, 2015). Mainly, ROS are formed in the apoplast, mitochondria, plasma membrane, chloroplast, peroxisomes, endoplasmic reticulum, and cell walls (Mittler, 2017; Hasanuzzaman et al., 2020). Therefore, to manage ROS noxiousness, plants have established well-organized and composite antioxidant defense systems, including numerous non-enzymatic and enzymatic antioxidants.
SOD is a type of metal enzyme that is first found in bovine red blood cells and then characterized in bacteria, vertebrates, and higher plants (Mann and Keilin, 1938; Rabinowitch and Sklan, 1980; Tepperman and Dunsmuir, 1990; Kim et al., 1996; Zelko et al., 2002). Researchers of different studies have found that SODs can catalyze superoxide O2 to disproportionate into O2 and H2O2 (McCord and Fridovich, 1969; Tepperman and Dunsmuir, 1990). SODs are detected in plants in roots, fruits, leaves, and seeds, which provide necessary protection for cells against oxidative stress (Tepperman and Dunsmuir, 1990). As per the binding pattern of metallic cofactors that cooperate with vigorous sites, SOD genes are categorized further into four groups: 1) iron FeSODs, 2) copper/zinc Cu/ZnSODs, 3) manganese MnSODs, and 4) nickel NiSODs (Mittler et al., 2004; Feng et al., 2016; Yan et al., 2016; Su et al., 2021). The different subtypes of SODs have almost comparable functions. However, they have different metallic cofactors and amino acid sequencing, in vitro subcellular location and crystal structure, and different hydrogen peroxide sensitivity (Abreu and Cabelli, 2010; Yan et al., 2016). These SODs are distributed in cell compartments individually and play an essential role in oxidative stress (Alscher et al., 2002). Among the SODs, Cu/ZnSODs are predominantly distributed in the chloroplast, extracellular space, and cytoplasm, and are present in certain bacteria and all eukaryotes, while MnSODs mostly present in plant mitochondria (Pilon et al., 2011; Feng et al., 2015; Song et al., 2018; Wang et al., 2018; Hu et al., 2019; Verma et al., 2019; Lu et al., 2020; Silva et al., 2020). MnSODs in the plant genome play a role in eliminating ROS from mitochondria (Møller, 2001). FeSODs are primarily distributed in protozoa, prokaryotes, cytoplasms, and plant chloroplasts, while NiSODs are found in Streptomyces and also in some cyanobacteria, but not in plants (Youn et al., 1996; Wuerges et al., 2004; Miller, 2012).
Recent studies have shown that SODs can protect plants from abiotic stress such as heat, drought, cold, abscisic acid, salt, and ethylene (Wang et al., 2004; Pilon et al., 2011; Asensio et al., 2012; Feng et al., 2015). Several studies have shown that the SOD genes can be induced and transcribed in different plants under various stress conditions, such as heat, drought, cold, salt, osmotic stress, oxidative stress, and hormonal signal transduction (Wang et al., 2004; Pilon et al., 2011; Feng et al., 2015; Feng et al., 2016; Yan et al., 2016). SOD gene family under different hormones and abiotic stress conditions in rapeseed (Su et al., 2021), Salvia miltiorrhiza (Han et al., 2020), Zostera marina (Zang et al., 2020), and Hordeum vulgare (Zhang et al., 2021) were recently published articles. Furthermore, diverse forms of SOD genes display different expression patterns under different stress conditions. In tomatoes, for example, SlSOD1 is the only significantly upregulated gene in the nine SlSOD genes, while SlSOD2, SlSOD5, SlSOD6, and SlSOD8 are regulated by salt stress. However, the expression levels of four “SlSOD2, SlSOD5, SlSOD6, and SlSOD8” genes are found high during imposed drought environment (Feng et al., 2016). Furthermore, the expression patterns of the same type of SOD gene were different under stress. For example, the studies found that there was no change in the expression of MnSODs in Arabidopsis under oxidative stress, and the researchers found that there was a significant change in the expression of MnSODs in peas, wheat, and Zostera marina under salt stress (Gómez et al., 1999; Wu et al., 1999; Baek and Skinner, 2003; Zang et al., 2020). These results suggest that different SOD genes have different expression patterns under different environmental stresses. Furthermore, researchers have also discovered that alternative splicing and miRNAs may be involved in the regulation of SOD expression (Srivastava et al., 2009; Lu et al., 2011). Until now, the SOD gene family has been described in many plant species, including Arabidopsis, Sorghum, Musa acuminata, Phaseolus vulgaris, and Populus (Kliebenstein et al., 1998; Zelko et al., 2002; Ballal and Manna, 2009; Fernández-Ocaña et al., 2011; Lin and Lai, 2013; Molina-Rueda et al., 2013; Filiz et al., 2014; Cui et al., 2015; Feng et al., 2015; Feng et al., 2016; Ho et al., 2017; Verma et al., 2019). Cucurbitaceae is a significant economic and nutritional crop in the world and SOD gene exists as a superfamily. The phylogeny line, genome circulation, gene assembly, preserved patterns, and expression profiles of these genes in different tissues have been thoroughly analyzed, laying the foundation for functional identification of SOD genes in Cucurbitaceae.
Materials and Methods
Retrieval of SOD Gene Family in Five Cucurbitaceae Species
To study the SOD gene family of cucurbit plants, the whole genome of individual species of Cucurbitaceae was searched by the Blastp search method and Arabidopsis sequence of SOD used as the query (Kliebenstein et al., 1998). Genomic, protein, and CDS (coding DNA sequence) sequences of SOD gene family have been identified and downloaded from the Cucurbitaceae database (CuGenDB) (http://cucurbitgenetics.org/) (Zheng et al., 2019) Subsequently, we used two methods to identify SOD genes in five Cucurbitaceae species, i.e., BLASTP (protein blast) and the hidden Markov model (HMM). For BLASTP, we used eight A. thaliana SODs (AT1G08830.1/AtCSD1, AT2G28190.1/AtCSD2, AT5G18100.1/AtCSD3, AT4G25100.1/AtFSD1, AT5G51100.1/AtFSD2, AT5G23310.1/AtFSD3, AT3G10920.1/AtMSD1, and AT3G56350.1/At00MSD2) amino acid sequences as a query with an e-value set to 1e−5. The amino acid sequences of eight AtSODs were obtained from the TAIR Arabidopsis genome database (http://www.arabidopsis.org/). The database web resources SMART (http://smart.embl-heidelberg.de/) (Schultz et al., 1998) and the Pfam protein domain database (http://pfam.xfam.org/) were used to scan certain amino acid sequences conserved domain SOD_Cu (PF00080.21) and SOD_Fe_C (PF02777.19). The ProtParam software (http://web.expasy.org/ProtParam) (Gasteiger et al., 2005) was used to evaluate the physiochemical properties (molecular weight, amino acid length, and isoelectric point) of each SOD protein.
Phylogenetic Analyses of SOD Gene Family
All SOD full-length protein sequences of the five Cucurbitaceae species [Citrullus lanatus (watermelon), Cucurbita pepo (zucchini), Cucumis sativus (cucumber), Lagenaria siceraria (bottle gourd), Cucumis melo (melon)] including seven other plant species were aligned using clustalX software with default parameters (1,000 bootstraps, pairwise deletion) (Thompson et al., 1997). A phylogenetic tree was constructed with a maximum likelihood method (MLM) by using the online version of the IQ-TREE software (http://iqtree.cibiv.univie.ac.at). Finally, the phylogenetic tree was visualized through online iTOL software (https://itol.embl.de/) (Letunic and Bork, 2019; Manzoor et al., 2021a).
Chromosomal Distribution of SOD Genes
The chromosomal localizations of Cucurbitaceae were obtained from CuGenDB database (http://cucurbitgenetics.org/). For chromosome mapping of SOD gene, map inspect tool (http://mapinspect.software.com) was used. The aforementioned description is used to map the distribution of SOD gene throughout the Cucurbitaceae family individual species used in this study (Hu et al., 2016).
Intron/Exon Structure and Conserved Motif Analysis of Protein
The genome sequence and the coding sequence of the SOD gene were downloaded from the genome of the individual species of the Cucurbitaceae family from CuGenDB database. The Gene Structure and Display Server (GSDS) (http://gsds.cbi.pku.edu.cn/) (Hu et al., 2015) was used to evaluate intron distribution dynamics and the splicing mechanism of each SOD gene. The Multiple Expectation Maximization for Motif Elicitation (MEME) software (Bailey et al., 2009) identified the conserved SOD protein motif (http://meme-suite.org/tools/meme). Finally, the subcellular localization was predicted using WoLF PSORT (http://wolfpsort.org) (Horton et al., 2007).
Prediction of the SOD Cis-Regulatory Elements in the Promoter
For the prediction of regulatory elements on the promoter regions of CuSODs, 1,500 kb upstream DNA sequence of each SOD gene was collected from all SOD genes using the online web server PlantCARE (http:/bioinformatics.psb.ugent.be/webtools/PlantCARE/html/) (Higo et al., 1999; Lescot et al., 2002).
Gene Duplications and Collinearity Relationship Analysis
Multiple collinearity scan toolkit (MCScanX) was used for collinearity analysis with BLASTP (E < 1e−5) against five Cucurbitaceae species (Wang et al., 2012; Qiao et al., 2018). Different modes of duplications (WGD/segmental, dispersed, proximal, and tandem duplications) among five Cucurbitaceae species (Citrullus lanatus, Cucurbita pepo, Cucumis sativus, Lagenaria siceraria, Cucumis melo) were used. Gene duplications and collinearity relationships were visualized by using TBtools (Chen et al., 2020) and circos software.
Gene Ontology Annotation and MicroRNA Target Site Analysis
We used the CELLO v.2.5 software functional annotation platform to determine the Cucurbitaceae SOD gene’s functional Classification. CELLO (http://cello.life.nctu.edu.tw/site) platform connects the genes with GO terms through hierarchical vocabularies (Conesa and Götz, 2008). Functional enrichment analysis of SOD genes was performed using DAVID online tools (DAVID 6.8; http://david.ncifcrf.gov/) (Huang et al., 2009). The GO terms were classified into three categories: biological process (BP), cellular component (CC), and molecular function (MF). The upregulated SOD genes and downregulated SOD genes were entered separately and p <0.01 was considered to indicate a statistically significant difference.
To determine the miRNA-mediated posttranscriptional regulation of SODs, we searched the 5′ and 3′ untranslated regions (UTRs), and the coding regions, of the SODs for target sites of Lagenaria siceraria miRNAs obtained from various databases and published articles on the psRNA Target server using default parameters (Dai et al., 2018).
Plant Materials and Abiotic Stresses
The L. siceraria plants were planted in the growth chamber; seeds (variety: Winall 808) were provided by The National Engineering Laboratory of Crop Resistance Breeding, School of Life Sciences, Anhui Agricultural University, Hefei, China. To examine the behavior of the SOD genes under abiotic stress, seedlings of 2-week-old plants were carefully watered and grown under 24 h/18 h light and 16 h/8 h dark conditions in a growth chamber before different stress inductions. However, seedlings were later transferred to the growth chamber at 50°C under normal lighting conditions during the heat treatment. The plant was treated for salt and drought resistance on the Murashige and Skoog (MS) liquid media containing 300 mM of PEG-6000. For the cold treatment, seedlings in the growth chamber were transferred to 4°C under light conditions (Hu et al., 2016). After treatment, the leaves were collected at 0, 4, 8, 16, and 24 h, and instantly frozen in liquid nitrogen immediately, and the sample was obtained to determine the transcription level of the SOD gene family in treated plant seedlings. Finally, all samples were instantly frozen in liquid nitrogen and stored at −80°C until further use.
RNA Isolation and Quantitative Real-Time PCR Reaction
RNA extraction reagent kit (Trizol) was purchased from Hua and Maike Biotechnology Co. Ltd., Beijing, China. Total RNA was extracted following the manufacturer’s instructions. The RNA reverse transcription kit (TaKaRa Company, Japan) and Fluorescent Quantitative Reagent SYBR Green Master (Roche, United States) were used, and qRT-PCR was performed using a previously described method (Zhou et al., 2014). Gene-specific primers of LsiSODs and Lsi-actin for the qRT-PCR system were designed by using GenScript server (www.genscript.com) and synthesized by Sangon Biotech (Shanghai) (Supplementary Table S1).
Actin gene of L. siceraria was used as an endogenous control to detect the relative expression of LsiSOD genes based on previous studies (Zhang et al., 2018). Primers used are given in Supplementary Table S1. qRT-PCR reactions were performed in biological triplicates. The relative expression level was calculated by 2−∆Ct method and statistical analyses were measured using Microsoft Office 2010.
Results
Genome-Wide Characterization of SOD Genes in Five Cucurbitaceae Species
In total, 43 SODs were identified in five Cucurbitaceae species from CuGenDB, and 8–10 genes were retrieved for individual species such as Citrullus lanatus (8 SODs), Cucurbita pepo (10 SODs), Cucumis sativus (9 SODs), Lagenaria siceraria (8 SODs), and Cucumis melo (8 SODs) (Supplementary Table S2). The detailed information of Cucurbitaceae species (molecular weight, starting and ending point, and theoretical pI) of the protein are listed in Table 1, and all protein sequences are shown in Supplementary Table S10.
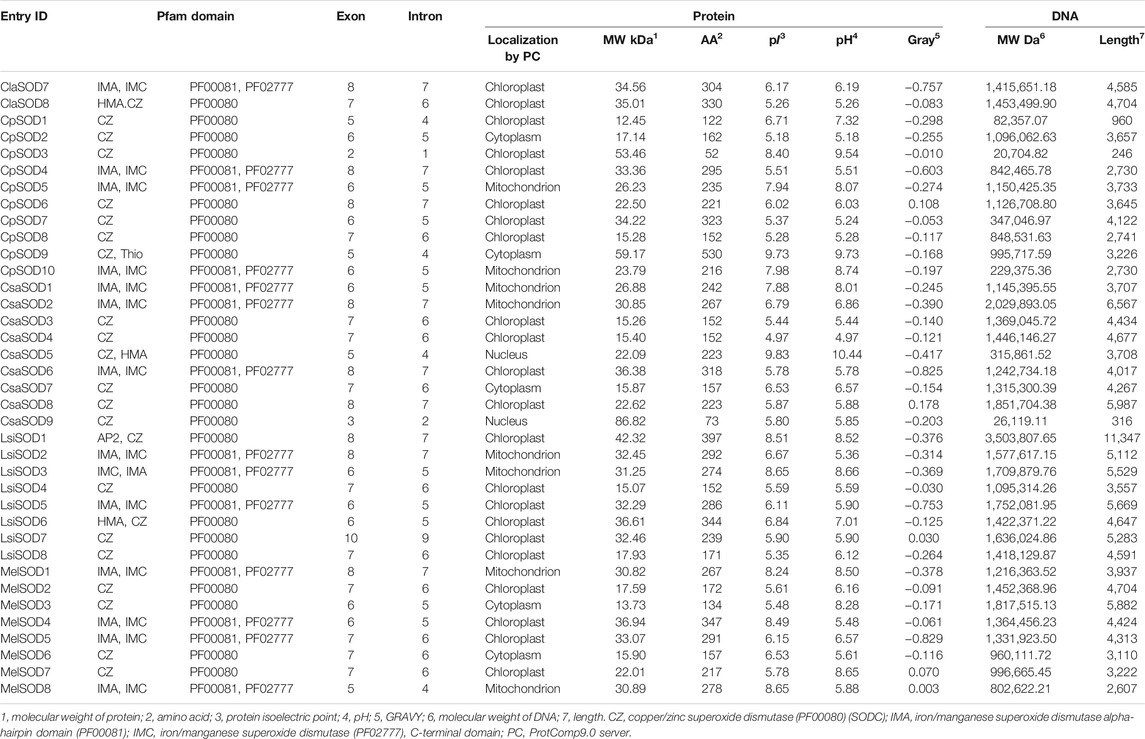
TABLE 1. Physio-chemical characteristics of 43 SOD identified genes and sequence information in five species of Cucurbitaceae family.
In Citrullus lanatus, we detected eight SOD genes, containing five copper/zinc SODs and three iron/manganese SODs. The molecular weight, length, and pI of SOD protein were 15.12–35.01 kDa, 152–330 amino acids, and 5.31–7.95, respectively: five Cu/ZnSODs (ClaSOD1, ClaSOD2, ClaSOD3, ClaSOD6, ClaSOD8) were acidic in nature and one MnSOD (ClaSOD5) was basic, and in the two FeSODs, ClaSOD7 was acidic and ClaSOD4 was slightly basic. Subcellular localization prediction results showed that six SODs (ClaSOD1, ClaSOD2, ClaSOD3, ClaSOD6, ClaSOD7, ClaSOD8) of Cu/Zn SODs were in the chloroplast, and Cla-SOD4 and Cla-SOD5 were localized to the mitochondria.
In Cucurbita pepo, ten SOD genes were detected, including seven Cu/ZnSODs and three Fe/Mn-SODs; the molecular weight, length, and pI values of SOD proteins were within the ranges of 15.28–59.17 kDa, 52–530 amino acids, and 5.18–9.73, respectively. The subcellular localization prediction showed that Cu/ZnSODs CpSOD1, CpSOD3, CpSOD4, CpSOD6, and CpSOD7 were localized to the chloroplast, and two Cu/ZnSODs, CpSOD2 and CpSOD9, were localized to the cytoplasm. Furthermore, CpSOD5 and CpSOD10 were localized to the mitochondrion.
In Cucumis sativus, nine SODs were retrieved—six were Cu/ZnSODs and three were Fe/MnSODs. The molecular weight, length, and pI values of SOD proteins were within the range of 15.26–86.82, while amino acids range from 73 to 318 and 4.97–9.83 kDa, respectively. CsaSOD2, CsaSOD3, CsaSOD4, CsaSOD6, CsaSOD7, CsaSOD8, and CsaSOD9 were acidic and CsaSOD1 and CsaSOD5 were basic. In the prediction of subcellular localization, CsaSOD1 and CsaSOD2 were localized to the mitochondrion. CsaSOD3, CsaSOD4, CsaSOD6, and CsaSOD8 are localized to the chloroplast; CsaSOD5 and CsaSOD9 are in the nucleus; and CsaSOD7 is localized to the cytoplasm.
In Lagenaria siceraria, eight SODs were detected collectively, including five copper/zinc SODs and three iron/manganese SODs. The molecular weight, length, and pI of SOD protein were 15.07–42.32 kDa, 152–397 amino acids, and 5.35–8.65, respectively. LsiSOD1 and LsiSOD3 of Cu/Zn-SODs are basic and LsiSOD6 is slightly basic; LsiSOD2, LsiSOD4, LsiSOD5, LsiSOD7, and LsiSOD9 are acidic. Subcellular localizations of LsiSOD1, LsiSOD4, LsiSOD5, LsiSOD6, LsiSOD7, and LsiSOD8 are found in the chloroplast while LsiSOD2 and LsiSOD3 are in the mitochondrion.
In Cucumis melo, a total of eight SODs were identified, including four Cu/ZnSODs and four Fe/MnSODs, and the SOD protein’s molecular weight, length, and pI values were observed within the ranges of 15.90–36.95 kDa, 134–347 amino acids, and 5.16–8.49, respectively; three SODs such as MelSOD1, MelSOD4, and MelSOD8 are basic, and MelSOD2, MelSOD3, MelSOD5, MelSOD6, and MelSOD7 are acidic. The prediction of subcellular localizations showed that MelSOD1 and MelSOD8 are in the mitochondrion, and MelSOD3 and MelSOD7 are in the cytoplasm. MelSOD2, MelSOD4, and MelSOD5 are in the chloroplast (Table 1).
Structural Phylogenetic Analysis of SOD Genes Family in Five Cucurbitaceae Species
To define the evolutionary relationship of SODs in different plant species, we constructed a phylogenetic tree of SODs based on the full length of protein sequences and divided them into eight groups. A total of 106 SOD proteins were obtained from 13 plant species including Arabidopsis thaliana, Lagenaria siceraria, Citrullus lanatus, Cucurbita pepo, Cucumis sativa, Cucumis melo, Gossypium arboretum, Solanum lycopersicum, Zea mays, Solanum tuberosum, Sorghum bicolor, Populus trichocarpa, and Oryza sativa. Three methods, maximum likelihood (ML), minimal evolution (ME), and maximum parsimony (MP), yielded nearly identical phylogenetic trees; therefore, only NJ tree was used for further analyses (Kumar et al., 2016) (Figure 1; Supplementary Figure S2). Based on the phylogenetic tree, the SOD genes were divided into five subgroups according to Arabidopsis (Silva et al., 2020). However, three unique clades in a phylogenetic tree may have independent evolutionary trajectories from other clades. It is also suggested that these clades may have individual evolution that is different from Arabidopsis (Nijhawan et al., 2008; Wei et al., 2012; Manzoor et al., 2021a). According to the bootstrap values quoted on the nodes, topography, and sequence similarities, all identified SODs from the Cucurbitaceae species were categorized into eight subfamilies (I–VIII). SOD protein sequences from all Cucurbitaceae species contributed in all subfamilies (Figure 1). These results suggested that there may have been gene loss or gain events that occurred throughout the evolutionary process. The gain and loss of specific SOD gene members caused functional divergence.
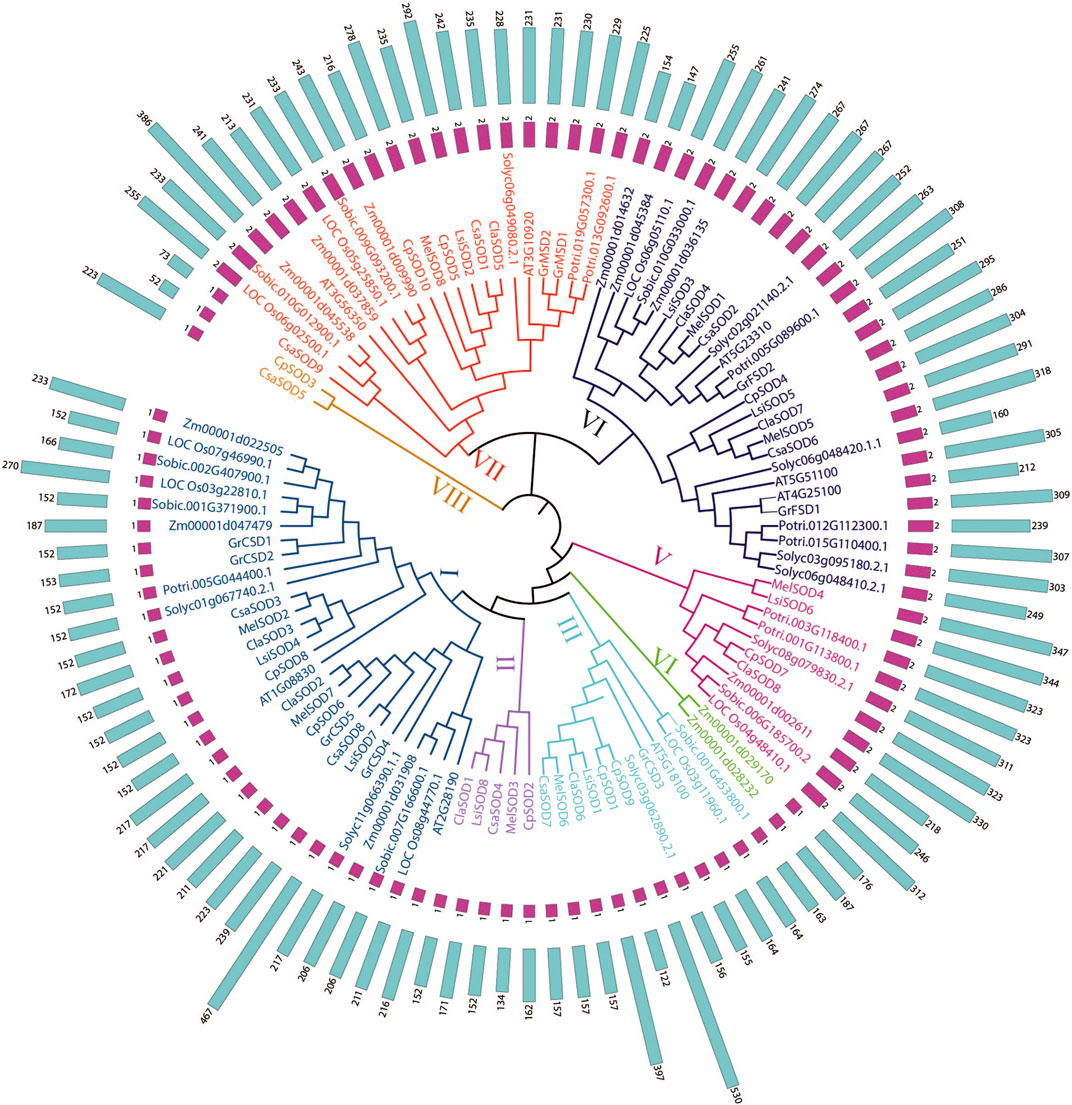
FIGURE 1. Phylogenetic tree of 106 SOD proteins from Lagenaria siceraria and other plants including 13 plant species—Arabidopsis thaliana, Lagenaria siceraria, Citrullus lanatus, Cucurbita pepo, Cucumis sativa, Cucumis melo, Gossypium arboretum, Solanum lycopersicum, Zea mays, Solanum tuberosum, Sorghum bicolor, Populus trichocarpa, and Oryza sativa. Protein sequences were aligned using ClustalW2 sequence alignment program and the phylogenetic tree was constructed by software MEGA7.
Conserved Motif Analysis of SOD Gene Family in Five Cucurbitaceae Species
The conserved motif analysis of the SOD family supported the classification and evolutionary relationships of the five Cucurbitaceae species SOD genes. In total, 20 motifs were detected from 43 SOD genes in Citrullus lanatus, Cucurbita pepo, Cucumis sativus, Lagenaria siceraria, and Cucumis melo. All SOD genes contained at least two motifs except CpSOD3 and CsaSOD5, which contain only one motif (Figure 2B): Cu/ZnSOD and Fe/MnSODs. Besides, FeSODs and MnSODs were clustered in the same group and belonged to a similar subcluster, while the Cu/ZnSOD was clustered in a different group. A similar cluster distribution was detected in the SOD proteins of each species, indicating that these SOD genes are highly conserved in different plants (Figure 2A). In Cucurbitaceae species, 5–9 exons were detected in SOD genes by comparing the genomic DNA and CDS sequences using the GSDS 2.0 utility (Figure 2C). However, differences were observed in the size and number of exons/introns in Cucurbitaceae. In the organization of exons/introns, a high degree of conservation has been observed, which is consistent with the high degree of similarity found by multiple alignments between protein sequences, which gives high similarities between them. The evolutionary analysis suggested that structural gene diversity is the primary source for the evolution of multigene families (Xu et al., 2012; Manzoor et al., 2021b).
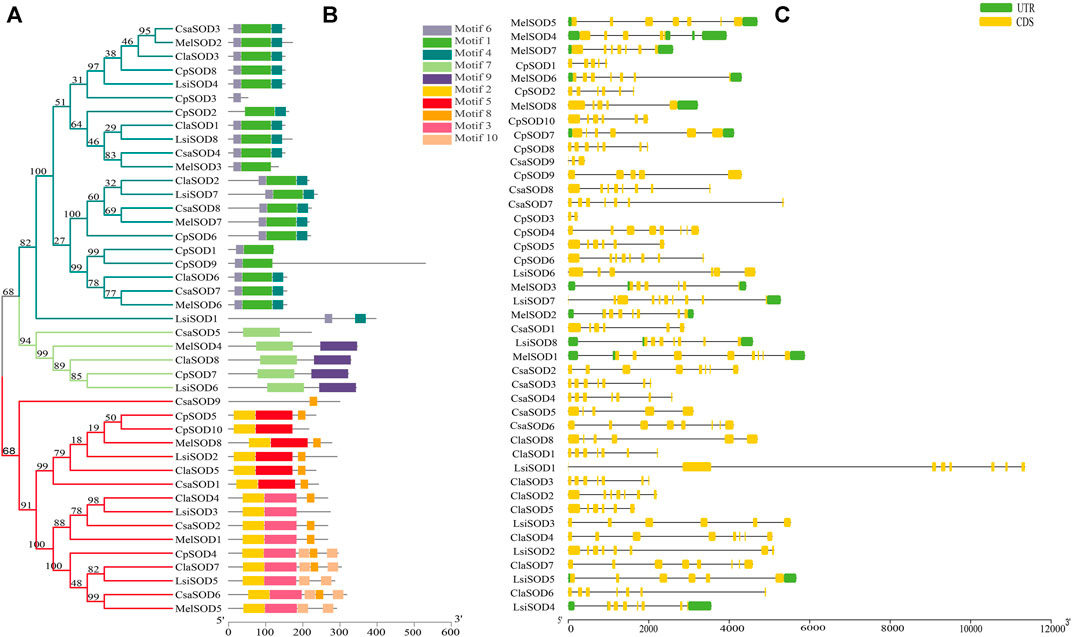
FIGURE 2. (A) The protein structures of SODs based on the presence of conserved motifs were arranged corresponding to the phylogenetic tree. (B) All conserved motifs of the SOD proteins were identified by the MEME program. Different motifs are highlighted with different colored boxes with numbers 1 to 10. (C) Gene structures of SODs exons are shown as yellow boxes, introns are shown as thin black lines, and UTRs are shown as blue boxes.
C. lanatus contains 6–8 exons and C. pepo contains 3–9 exons; interestingly, CpSOD3 contains 2 exons with 1 intron. C. sativus contains 5–8 exons while CsaSOD9 has 3 exons and 2 introns. Both CpSOD3 and CsaSOD9 genes are the smallest genes among all 43 SOD genes identified in five Cucurbitaceae species. C. melo consists of 5–8 exons in which MelSOD8 has 5 exons and 4 introns. L. siceraria contains 6–10 exons; interestingly, LsiSOD1 and LsiSOD2 comprise 8/7 exons/introns. On the other hand, LsiSOD3, LsiSOD5, and LsiSOD6 comprise 6/5 exons/introns that have the upstream and downstream sequence. LsiSOD4 contains exons/introns (7/6) with upstream and downstream sequences and LsiSOD7 contains 10 exons with the only downstream sequence. The remaining LsiSOD8 contains 7 exons and 6 introns with both upstream and downstream sequences.
Chromosomal Distribution and Promoter Analysis of SOD Gene Family
The chromosomadal distribution of the SOD gene family of the Cucurbitaceae species was determined, as shown in Figure 3 (Supplementary Table S3), and detailed data are given in Supplementary Table S4. C. lanatus chromosome 2 contains two genes, and chromosome 3 contains three genes, while chromosomes 4, 7, and 10 contain only one gene. In C. pepo, chromosome 0 contains three genes; 1, 2, 5, 11, and 20 contain only one gene while chromosome 8 contains 2 genes. In C. sativus, chromosome 1 contains three genes, and chromosomes 4 and 6 contain two genes each, while only one gene was found on chromosomes 2 and 3. In L. siceraria, chromosomes 1, 2, 10, and 11 contain one gene, while chromosomes 6 and 7 contain two genes, respectively. C. melo contains two genes on chromosome 2, while chromosomes 5, 6, 7, 8, 11, and 12 contain only one SOD gene.
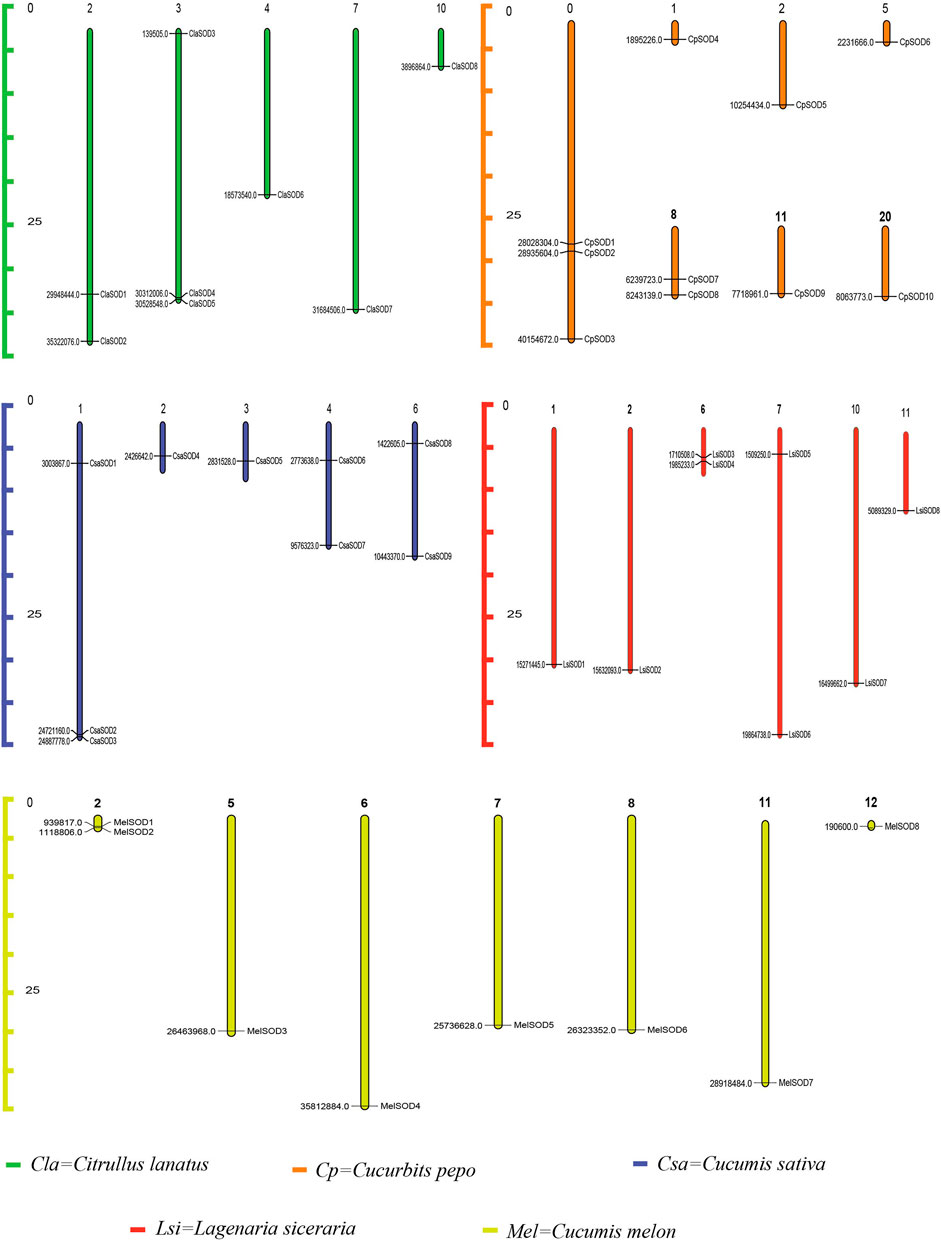
FIGURE 3. Chromosomal locations of 43 SOD genes in the genome of five species of the Cucurbitaceae family. Watermelon: Cla; melon: Mel; cucumber: Csa; zucchini: Cp; bottle gourd: Lsi. The chromosome numbers are indicated at the top of chromosomes, and the size of the chromosome is represented with a vertical scale. The gene number is located on each chromosome and the left scale is in megabases (Mb).
To clearly understand the function and regulation of SOD proteins, cis-elements in the promoter sequence of SOD genes in Cucurbitaceae were retrieved. The 1,500-bp upstream region of the start codon of each SOD gene was analyzed by using the PlantCARE database. According to the obtained results, the cis-elements can be divided into three classes: stress related, light related, and hormone response elements (Figure 4A). Five cis-elements of stress response were determined, including LTR, TC rich, MBS, ARE, and box-w1; these elements reflected the response of plants to drought, low temperature, anaerobic induction, stress defense, and fungal inducer. Four hormone-sensitive cis-elements (SA, MeJA, GA, and ethylene) were identified, which are associated with ABA, SA, ethylene, and MeJA responses (Figure 4B). On the promoter region of SOD gene family of Cucurbitaceae, a considerable number of phyto-response cis-elements were detected (Supplementary Table S4). The results suggested that the cis-elements of SOD promoter had a positive response to abiotic stress and regulation mechanism of plant growth and development.
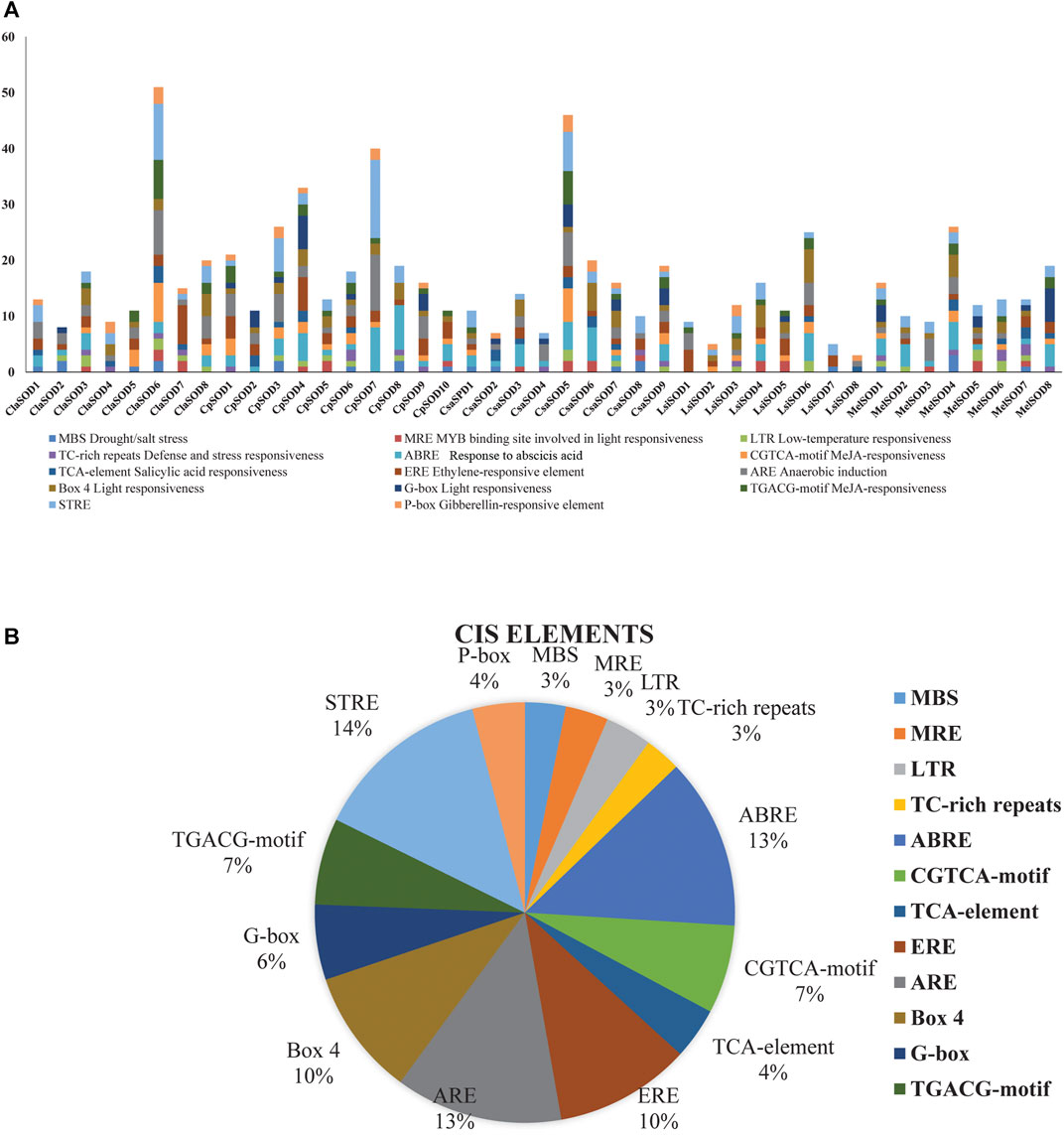
FIGURE 4. Analysis of cis-elements in putative promoters of SOD gene family of five species of Cucurbitaceae family. 1,500 kb upstream of the transcription initiation site, sequences of SOD genes were retrieved and analyzed by PlantCARE. (A) Different stress-responsive and hormone-associated elements are identified, and their numbers are plotted on the graph. Various elements in each promoter are coded in different colors according to the legend at the top. (B) The size of the pie charts showed the percentage of promoter element in each category.
Gene Duplication Events and Collinearity Relationships in Five Cucurbitaceae Genomes
To further understand the evolution and new function of genes, gene duplication events of the SOD gene family were identified in five Cucurbitaceae species (Citrullus lanatus, Cucurbita pepo, Cucumis sativus, Lagenaria siceraria, Cucumis melo). We analyzed three modes of gene duplications in all SOD gene families, including transposed duplication (TRD), dispersed duplication (DSD), and whole-genome duplication (WGD). We identified many kinds of gene duplications and their contributions to the expansion of the SOD genes. Interestingly, 25 pairs of the duplicated gene were identified in five Cucurbitaceae species, with a maximal number of duplicated gene pairs derived from dispersed duplications (15 genes pair out of 25), followed by transposed duplications (8 genes pair out of 25) and whole-genome duplications (2 genes pair out of 25) showing that the expression of the SOD gene family was mainly associated with WGD, TRD, and DSD events (Supplementary Table S5) (Figure 5). These results indicate that DSDs play a vital role in SOD gene expansion in Cucumis sativus, Lagenaria siceraria, and Cucumis melo. WGDs might contribute to the expansion of the SOD gene family (Supplementary Table S5). Our study showed that duplication events play an important role in SOD gene expansion, and TRDs and WGD occurred at high frequency in Cucurbitaceae species (Figure 6).
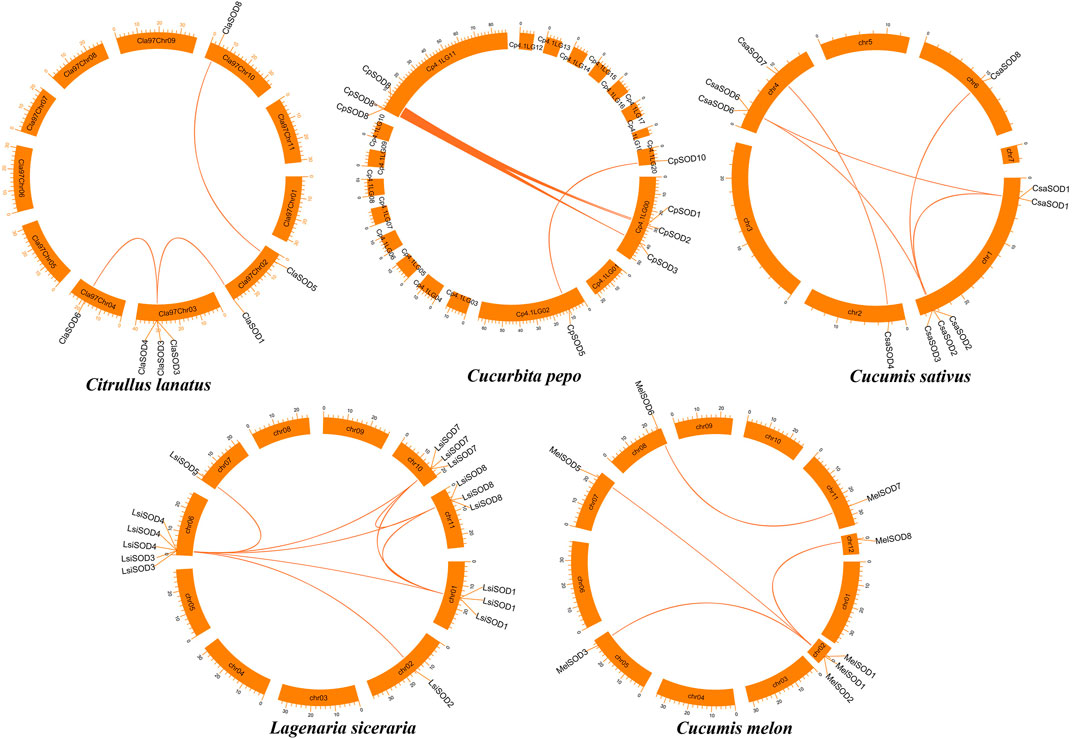
FIGURE 5. Chromosomal localization and duplication events of five Cucurbitaceae genomes and gene pairs are joined with a colored line.
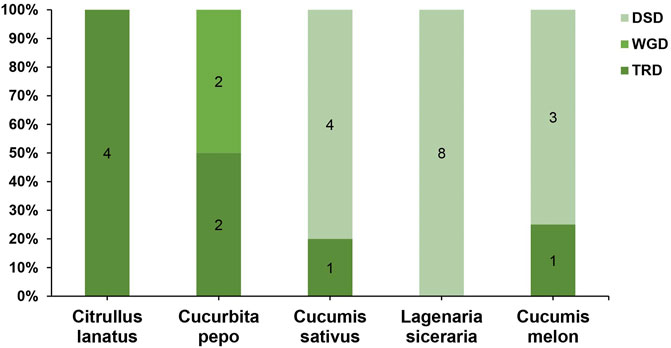
FIGURE 6. Comparison of gene duplication events in five Cucurbitaceae genomes. WGD, whole-genome duplicates; TRD, transposed duplicates; DSD, dispersed duplicates.
We further analyzed the collinearity relationships of SOD genes between five Cucurbitaceae species (Citrullus lanatus, Cucurbita pepo, Cucumis sativus, Lagenaria siceraria, Cucumis melo) as these five plants belong to the Cucurbitaceae family and shared a similar antique (Supplementary Table S6) (Figure 7). A total of 35 collinear gene pairs were found between the five Cucurbitaceae genomes, including 5 orthologous gene pairs between Arabidopsis and Cucurbita pepo, 4 orthologous gene pairs between Arabidopsis and Lagenaria siceraria, 4 orthologous gene pairs between Arabidopsis and Cucumis melo, 8 orthologous gene pairs between Citrullus lanatus and Cucumis sativus, 7 orthologous gene pairs between Citrullus lanatus and Lagenaria siceraria, and 7 orthologous gene pairs between Cucurbita pepo and Lagenaria siceraria, suggesting a close relationship among the five Cucurbitaceae genomes. The results show that the genetic relationship between SOD gene pairs in C. lanatus, C. pepo, C. sativus, C. melo, and L. siceraria is close. No pairs of collinear SODs are shared between Arabidopsis and five Cucurbitaceae genomes, indicating the long distance phylogenetic relationship between two species.
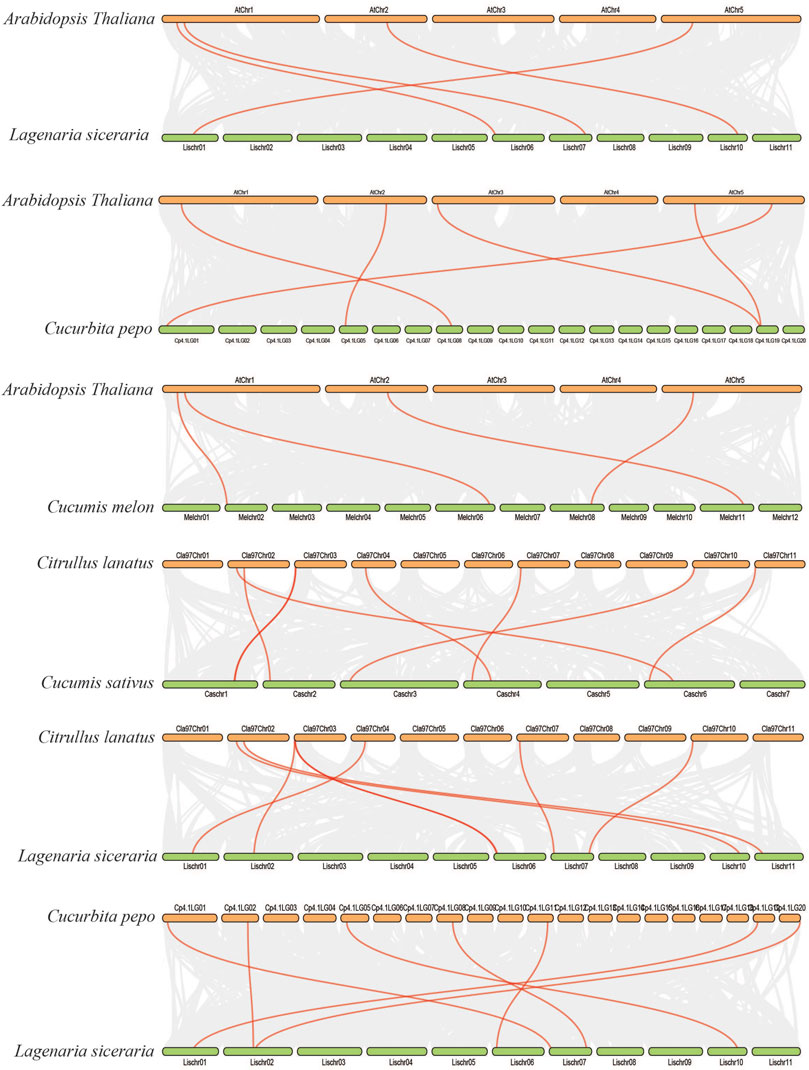
FIGURE 7. Collinearity relationships in five Cucurbitaceae genomes and Arabidopsis thaliana; different line color represents synteny relationships in the five Cucurbitaceae genomes.
Gene Ontology Annotation Study of SOD Gene Family
Functional enrichment analysis of SOD genes was performed using DAVID. The SOD genes were categorized into three functional groups—biological processes, molecular functions, and cellular components—that are characteristics of genes or gene products, which enable us to understand the diverse molecular functions of proteins (Altschul et al., 1990). These results may be related to protein sequence similarities caused by genomic events (8, Supplementary Table S7). Evaluation of the biological processes mediated by SODs indicated that the same percentage (∼27%) of proteins was involved in oxidoreductase activity and ion binding. Among the SOD proteins, ∼14.58% of members showed potential involvement in protein binding, enzyme binding, and DNA binding, respectively, while nucleic acid binding transcription factor activity involvement is ∼2.08% during the 8 LsiSOD genes of Lagenaria siceraria life cycle (Figure 8). At the same time, in SOD genes of five Cucurbitaceae species, “Cellular Components” includes 17.40% response to stress and reproduction, respectively. Meanwhile, 15.83% of genes were involved in aging, and 10.73% of genes were involved in homeostatic process and transport, respectively (Figure 8). Furthermore, the SOD genes of 8 LsiSOD genes of Lagenaria siceraria were involved in the molecular functions, 11.9% of the genes were involved in intracellular, cell, cytoplasm, organelle, and mitochondrion while 10.51% of the genes were involved in plastid and 5.65% were involved in extracellular region, cytosol, nucleus, and extracellular space, respectively (Figure 8). The results corroborated the putative SOD promoter analysis (Jagadeeswaran et al., 2009; Jia et al., 2009; Guan et al., 2013; Manzoor et al., 2021a). Furthermore, analysis of the molecular function annotations revealed that all of the LsiSOD protein functions were enriched in SOD activity and metal ion binding.
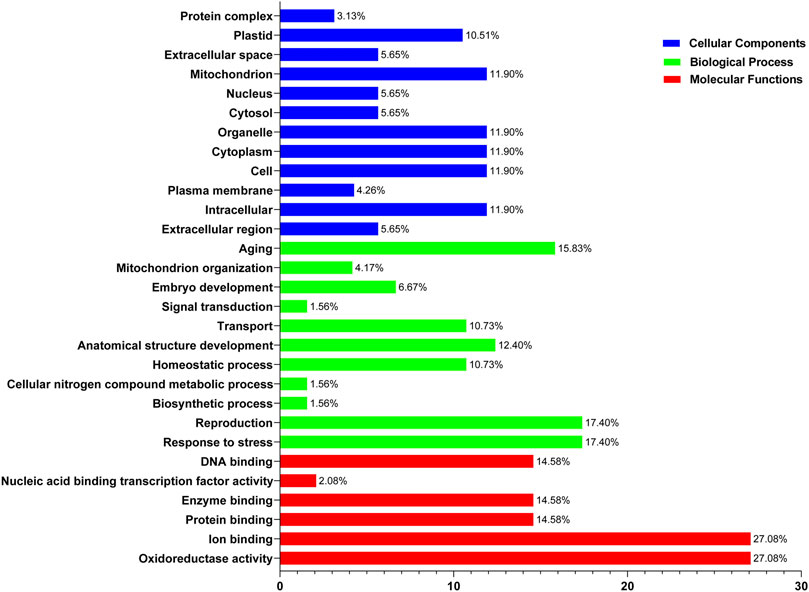
FIGURE 8. Gene Ontology (GO) annotation results of SOD genes of five species in the Cucurbitaceae family. GO analysis of SOD protein sequences is analyzed for their involvement or function in three important categories: biological process, molecular function, and cellular component.
Genome-Wide Analysis of miRNA Targeting LsiSOD Genes
MicroRNAs (miRNAs) are endogenous microRNAs that play an important role in plant growth/development and stress responses. Thus, for a deep understanding of miRNA-mediated post-transcriptional regulation of LsiSOD, we identified 6 miRNAs (Lsi-MIR164a, Lsi-MIR164b, Lsi-MIR164c, Lsi-MIR164f, Lsi-miRN1740, and Lsi-miRN1741) with different classes of miRNA families (Figure 9; Supplementary Table S8). The analysis indicated that 3 LsiSOD genes (LsiSOD3, LsiSOD6, and LsiSOD7) were targeted on different miRNA. The expression profiles of these miRNAs and their targets are needed to detect and verify in further experiments to determine their biological functions in bottle gourd. The regulation of SOD genes’ expression by miRNA needs to be studied further.
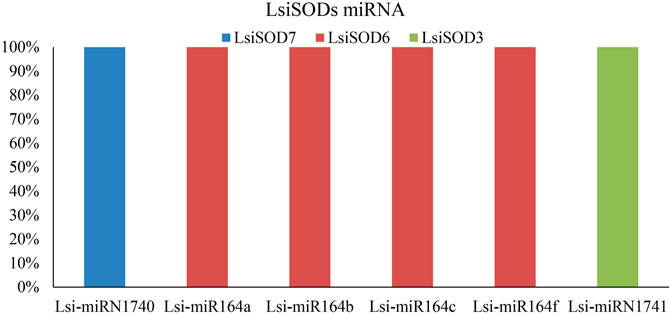
FIGURE 9. SODs targeted by miRNAs of Lagenaria siceraria (bottle gourd). The RNA sequence of each complementary site from 5′ to 3′ and the predicted miRNA sequence from 3′ to 5′ are shown in the expanded regions.
Tissue-Specific Expression of LsiSOD Genes
A strong link between gene expression and function has been suggested and that the SOD gene family is primarily involved in plant growth, development, and stress responses. To determine the biological functions in Lagenaria siceraria, the expression profiles of the 8 LsiSOD genes were analyzed in tissues (root, flower, fruit, stem, and leaf) using RNA-seq data downloaded from CuGenDB (http://cucurbitgenetics.org/) (Zheng et al., 2019). These results indicated that LsiSOD genes have tissue-specific expression patterns. However, LsiSOD4, LsiSOD5, and LsiSOD6 exhibited low transcript abundance in root and flower while their highest expression was detected in stems and leaves. This suggested that they play an important role in the development of plants. In addition, LsiSOD1, LsiSOD2, and LsiSOD3 are highly expressed in all parts of plants especially in the early stages of plant development (Figure 10). All LsiSOD genes were widely expressed and showed tissue-specific expression patterns while LsiSOD8 shows less expression in all different developmental parts.
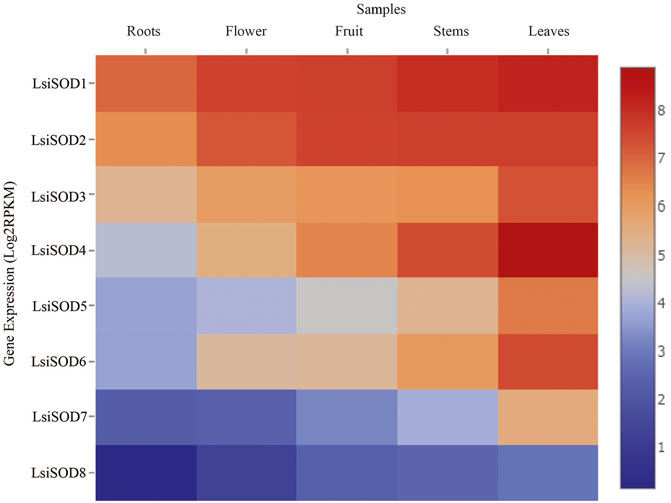
FIGURE 10. Relative expression profiles of SOD genes of five species in Cucurbitaceae family during different developmental stages. The FPKMs calculated by RNA-seq data are shown as a heat map. The color scale is shown at the side of the heat map reflecting the higher and lower relative abundance in each tissue.
Gene Expression Analysis of LsiSOD Gene Under Abiotic Stresses
To understand the role of the SODs, qRT-PCR was used to analyze the expression patterns of the SOD gene in response to stress from heat, cold, drought, and NaCl. Significant differences were observed in the expression levels of the LsiSOD genes under different treatments, and complexity was detected in their expression patterns (Figure 11). The 8 LsiSOD genes were induced by heat treatment, and their expression profiles were significantly enhanced. Among them, at 4 h of treatment one gene LsiSOD7 (2.62-fold), at 8 h of treatment two genes LsiSOD3 (20.35-fold) and LsiSOD4 (14.62-fold), at 16 h of treatment two genes LsiSOD2 (13.22-fold) and LsiSOD3 (16.41-fold), and at 24 h 2 genes LsiSOD2 (18.24-fold) and LsiSOD3 (24.16-fold) reached the highest expression level respectively. Interestingly, the transcription of LsiSOD3 and LsiSOD4 was gradually induced to 8 h, then decreased at 16 h, and finally reached a high level at 24 h, as described in Figure 11. Besides, in the expression of LsiSOD7, a dramatic increase was observed, which reached the maximum level at 4 h and decreased significantly at 8 h, then increased gradually with the treatment (Figure 11A). During cold treatment at 4 h, many LsiSOD genes were downregulated, LsiSOD3 (1.17-fold) remained unchanged, LsiSOD7 (1.27-fold) slightly increased, and then LsiSOD3 (7.27-fold) increased significantly at 8 h (Figure 11B). After 8 h, except for the increased expression of LsiSOD2 (1.71-fold) at 16 h, the other LsiSOD genes were significantly downregulated at 16 h and continued to decline at 24 h as compared with 0 h. After 8 h, the expression of LsiSOD7 (4.53-fold) and LsiSOD8 (4.39-fold) was progressively induced, and the induction peak appeared at 16 and 24 h, respectively. Under the PEG stress treatment, almost all LsiSOD gene expressions increased significantly at 4 h except LsiSOD1, and then the level of transcription decreased. At the same time, LsiSOD1 decreased somewhat at 4 h, and its transcription remained unchanged (Figure 11C). At 4 h of treatment with PEG, the expression of LsiSOD8 (4.27-fold) was maximum, whereas the expression of LsiSOD8 (1.36-fold) was highest during 8 h of treatment, while at 12 h of treatment LsiSOD4 (2.17-fold) shows maximum expression. During NaCl stress treatment, mostly the expression pattern of all LsiSOD genes increased tremendously at 8 h, decreased at 16 h, and finally increased at 24 h (Figure 11D). At 4 h of treatment, expression of LsiSOD3 increased (3.71-fold), and at 8 h of treatment with NaCl, expression of LsiSOD2 increased (8.59-fold), while at 12 h of treatment, the expression of LsiSOD8 increased (3.01-fold) and the expression of LsiSOD4 increased (4.53-fold). It should be worth mentioning that LsiSOD2 transcription level increased significantly in 24 h, which was significantly higher than other LsiSOD genes.
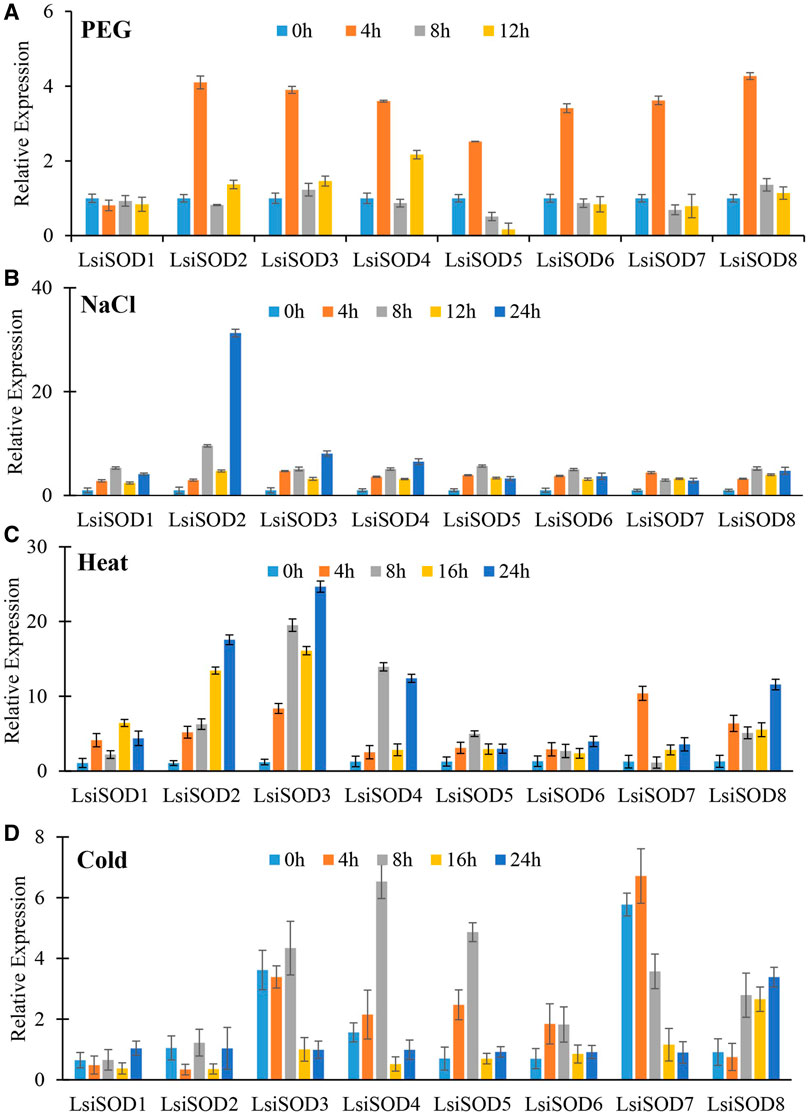
FIGURE 11. qRT-PCR study of SOD genes of bottle gourd in response to different abiotic stresses including (A) heat, (B) cold, (C) PEG, and (D) NaCl at different time points (0, 4, 8, 16, 24 h). Error bars indicate ±SD based on three biological replicates.
Discussion
Environmental pressure poses a considerable challenge to crop production. Superoxide dismutase activity plays an important role in plant resistance to different stresses, such as salt, drought, and metal toxicity (Alscher et al., 2002; Schützendübel and Polle, 2002; Fernández-Ocaña et al., 2011; Atkinson et al., 2013; Lin and Lai, 2013; Wang et al., 2018). SOD gene family is widely distributed among many plant species, including Arabidopsis (Kliebenstein et al., 1998), rice (Dehury et al., 2013), longan (Lin and Lai, 2013), banana (Molina-Rueda et al., 2013), poplar (Gosavi et al., 2014), sorghum (Nath et al., 2014), cotton (Feng et al., 2015), and tomato (Filiz and Tombuloğlu, 2015; Zhang et al., 2016; Wang et al., 2016; Wang et al., 2017). Our study provides a systematic and comprehensive whole-genome evolutionary analysis of SOD members obtained from five Cucurbitaceae genomes while the focus of our study is on Lagenaria siceraria, which is one of the excellent vegetables that contain all the essential ingredients necessary for human health and quality of life (Division and Jambheshwar, 2011). SODs are identified as a crucial enzyme involved in many oxidation processes and protect the plant against ROS (Alscher et al., 2002). Therefore, we systematically analyzed the SOD gene family of Cucurbitaceae and determined the gene expression patterns in L. siceraria under different abiotic stresses (drought, heat, salt, and cold).
A phylogenetic study was conducted on SOD proteins in Lagenaria siceraria and four other cucurbit plants, namely, Citrullus lanatus, Cucurbita pepo, Cucumis sativus, and Cucumis melo. SODs could be classified into eight groups with good statistical support in line with previous studies (Alscher et al., 2002; Feng et al., 2015; Filiz and Tombuloğlu, 2015; Feng et al., 2016; Wang et al., 2017; Verma et al., 2019). SOD genes are localized to chloroplasts, and mitochondria were classified into the same subgroups. Furthermore, nearly all LsiSODs are systematically grouped with at least one member of all plant species under consideration, highlighting the functional conservation of LsiSODs with other plant species SODs (Figure 1).
In this study, SOD gene families were found in Cucurbitaceae genome, including Citrullus lanatus, Cucurbita pepo, Cucumis sativus, Lagenaria siceraria, and Cucumis melo. There are considerable differences in the size of genomes and the number of SODs in these plant organisms, but there is no substantial dependence on the size of genomes. The number of amino acids of SOD proteins ranged from 52 to 530 kDa, showing a significant variation. The difference in the number of SOD genes in different plant species may be due to gene duplication. In addition, the difference between clades might be related to different functions and diversity of exons/introns and conserved motif structure. Intron and exon variations play a major role in the evolution of different genes. The variation in introns/exons and motif structure of SOD genes suggests a high level of complexity between Cucurbitaceae species.
We further investigated the diversity between SODs by analyzing their subcellular localization as localization plays a vital role in their functions. The cytoplasm (34%), chloroplast (33%), mitochondria (22%), and nucleus (11%) SOD gene localizations were predicted in five Cucurbitaceae species (Supplementary Table S8), and further study is needed to confirm those localizations. Increasing evidence points to an important role of miRNAs in stress tolerance. Several studies have reported that miRNAs regulate the expression of stress-responsive protein-coding genes at post-transcriptional level, showing a reverse correlation between the miRNA and the target expression (Sunkar et al., 2007; Lomakina, 2018; Manzoor et al., 2020). These miRNAs resulted from computational predictions and deep sequencing, and they are involved in some biological processes reported in plants, including responses to environmental stresses and regulating cell growth, development, and metabolism (Qiu et al., 2007; Li et al., 2012). In the present study, we identified 3 miRNAs targeting 8 LsiSOD genes (Figure 9; Supplementary Table S8). In Lagenaria siceraria, the miR164 family comprises 3 members that generate four mature products, miR164a/b/c and miR164f, which could target at least three LsiSOD genes. The miR164 family is a highly conserved miRNA that has been found in many plant species. miRNA164a thereby increased the tolerance of the plant to the abiotic stress or increasing the biomass, vigor, or yield of the plant. The miRNA miR164 plays a central role during the development of serrated leaf margins in Arabidopsis. In this study, transcripts of three miRNA families were identified because miRNA expression is highly regulated under different abiotic stress conditions (Figure 9). Further studies are needed to determine the role of SODs in Lagenaria siceraria.
Gene duplications are an essential mechanism for creating genetic novelty in all plants, which could help organisms to adapt to environmental change (Alvarez-Buylla et al., 2000; Kondrashov, 2012; Manzoor et al., 2020). There are different kinds of gene duplication, including dispersed duplication (DSD), transposed duplication (TRD), and whole-genome duplication (WGD), which differentially contribute to the expansion of plant-specific genes (Qiao et al., 2018, Qiao et al., 2019). Gene replication can cause variation in the number of SOD genes in different plant species, and involves tandem and segmented replication, which plays an important role in SOD gene diversity, expansion, and duplication (Filiz and Tombuloğlu, 2015; Wang et al., 2016; Zhang et al., 2016; Wang et al., 2017). Thus, tandem repetition is likely to play a vital function in the amplification of the LsiSODs, for example, the two neighboring genes LsiSOD3 and LsiSOD6 on Chr6 (Figure 3). Characterization of the gene structure describes that the number of introns from the SOD gene family differed between the observed Cucurbitaceae species (Figure 2); in addition, the number of introns in L. siceraria was 6–8. Previous studies have shown that the plant’s SOD gene has a strongly conserved intron pattern and that seven introns are present in most cytoplasmic and chloroplast SODs (Fink and Scandalios, 2002). In our study, only the LsiSOD members were predicted to contain seven introns, as shown in Figure 2.
Analysis of the cis-elements in SOD gene promoters resulted in the detection of three major types of cis-elements associated with light, abiotic stress, and hormone response as well as cis-elements related to developmental processes and tissue-specific expression. A relatively large number of light-responsive cis-elements were detected in SOD gene promoters, suggesting that SODs might participate in the abiotic response. Some studies have shown that SOD genes are involved in the response to abiotic stress in different plants like Dendrobium catenatum, Pennisetum glaucum, maize, and Arabidopsis (Divya et al., 2019; Huang et al., 2020; Kliebenstein et al., 2020; Liu et al., 2021). In addition, a series of cis-elements related to abiotic stress responses were identified in SOD gene promoters, such as MBS, ERE, TC-rich repeats, ARE, ABRE, and Box-4, which may regulate gene expression under various stresses. Most of the SOD genes in Arabidopsis, banana, rice, tomato, poplar, cotton, and other different plants can be induced in response to various abiotic stresses such as heat, cold, drought, and salinity (Kliebenstein et al., 1998; Lee et al., 2007; Dehury et al., 2013; Nath et al., 2014; Feng et al., 2016; Wang et al., 2016; Zhang et al., 2016; Wang et al., 2017; Verma et al., 2019). GO annotation analysis further verified these results (Supplementary Table S7). In addition, some researchers have reported similar findings in different crops and found that SOD gene plays an important role under different stress conditions. GO annotation results confirmed the LsiSOD’s role in response to different stress stimuli, cellular oxidant detoxification processes, metal ion binding activities, SOD activity, and different cellular components. These results can promote our understanding of LsiSOD genes under different environmental conditions. We also found protein similarities (Supplementary Table S9) by using protein–protein interactions (PPIs) handle a wide range of biological processes, including metabolic and developmental control and cell-to-cell interactions (Mittler, 2017). Regarding the possible role of SODs from Cucurbitaceae, the expression profile of SODs in different tissues was analyzed based on sequence evidence from RNA-seq data. Data analysis suggests that the gene family of SOD was expressed in all tissues; some SODs had tissue-specific expression patterns (Figure 10).
Expression Patterns of SOD Gene Family of Lagenaria siceraria Under Heat, Cold, Drought, and Salt Stress
Excess ROS resulting from abiotic stress can pose threats to L. siceraria yield. The SODs play an active role in ROS removal from plants caused by various abiotic stresses (Gill and Tuteja, 2010). However, LsiSODs’ specific response to heat, cold, drought, and salt is not well understood. Therefore, the analyses of qRT-PCR provide important clues for understanding the possible role of LsiSODs under various stresses. Based on the evaluation of cis-elements of LsiSOD gene promoter, three main cis-element types related to light, abiotic stress, and hormone response, as well as cis-elements related to development process, were determined. The cis-elements in LsiSODs promoter include TC-rich motif, LTR motif, MBS motif, ARE motif, and ABRE motif, which is the evidence of abiotic stress response (Supplementary Table S4). These motifs were previously observed in different plant species to cope with abiotic stresses, such as bananas, tomatoes, Brassica, tobacco, and millet (Oppenheim et al., 1996; Kurepa et al., 1997; Kliebenstein et al., 1998; Pilon et al., 2011; Feng et al., 2015; Wang et al., 2018; Hu et al., 2019; Verma et al., 2019). In this study, the expression level of eight LsiSOD genes also changed significantly under different stresses, indicating that these genes play an important regulatory role in stress response and may have a certain functional relationship. Almost all LsiSOD genes were upregulated during heat treatment, and some displays have similar expression (Figure 11C).
Under cold stress, the expression level of all LsiSOD genes is unregulated significantly, and their patterns of expression are different from each other, as shown in Figure 11D, which means that LsiSOD genes under cold stress may have functional diversity. Similar expression patterns were observed in LsiSOD1 with MaCSD1B and MaCSD2B (Feng et al., 2015). In PEG treatment, almost all Lsi-SOD1 genes have identical expression patterns, reach the highest level at 4 h, and then decline, as shown in Figure 11A, meaning that the function of the LsiSOD gene is related to drought.
The expression of most of the LsiSOD genes changed during NaCl treatment; LsiSOD2 is the only one of the eight LsiSOD genes with a significant increase, as shown in Figure 11B, indicating that LsiSOD2 plays an active role in detoxification of ROS during salt stress. Similar results were observed during salt stress in tomato (Feng et al., 2016). SlSOD1 and LsiSOD2 were grouped in the same subgroup, showing approximately high amino acid sequence homology. Altogether, we conclude that the LsiSOD gene plays a specific role in ROS removal induced by abiotic stress, which enhances plant adaptability to stress. In addition, some LsiSOD genes were correlated to abiotic stress exhibiting different expression patterns. For example, cold treatment reduced the expression of LsiSOD1, heat treatment and NaCl treatment increased the expression of LsiSOD1, while under PEG stress, the expression level remained the same without significant change, as shown in Figure 11, which means that the function of LsiSOD1 in different signaling pathways was different. It needs to be further explored to elucidate the role of the LsiSOD gene in L. siceraria under various abiotic stresses.
Conclusion
In this study, we identified SOD genes from the Cucurbitaceae family and analyzed their genomic structure, GO annotation analysis (molecular processes, biological functions, and cellular components), miRNA, gene duplication events (TD, PD, DSD, WGD, and TD), conserved motif patterns, phylogenetic relationships, mode of gene duplications, subcellular localization, and RNA-seq data analysis. qRT-PCR was used to evaluate the LsiSOD gene regulatory response under a variety of abiotic stresses, such as heat, cold, PEG, and NaCl. This research provided insight and further functional identification of the Cucurbitaceae family of SOD genes and laid the framework for understanding the molecular mechanism of the SOD gene in response to stress and plant growth. Genome-wide study of SOD genes provides insights into the evolutionary history and has laid a foundation for gene role, functional characteristics, and molecular mechanism in the plant development process and stress response (Mistry et al., 2021).
Data Availability Statement
The datasets presented in this study can be found in online repositories. The names of the repository/repositories and accession number(s) can be found below: CuGenDB, PRJNA387615.
Author Contributions
SR performed the main experiments. SR and AR prepared the article draft. MAM helped in bioinformatic tools and methodology. LL, WS, MWR, and DL reviewed the article.
Funding
This research was supported by the National Natural Science Foundation of China (NSFC) (31570650) and the Priority Academic Program Development of Jiangsu Higher Education Institutions.
Conflict of Interest
The authors declare that the research was conducted in the absence of any commercial or financial relationships that could be construed as a potential conflict of interest.
Publisher’s Note
All claims expressed in this article are solely those of the authors and do not necessarily represent those of their affiliated organizations, or those of the publisher, the editors, and the reviewers. Any product that may be evaluated in this article, or claim that may be made by its manufacturer, is not guaranteed or endorsed by the publisher.
Acknowledgments
Thank full for this grant from National Natural Science Foundation of China.
Supplementary Material
The Supplementary Material for this article can be found online at: https://www.frontiersin.org/articles/10.3389/fgene.2021.784878/full#supplementary-material
References
Abreu, I. A., and Cabelli, D. E. (2010). Superoxide Dismutases-A Review of the Metal-Associated Mechanistic Variations. Biochim. Biophys. Acta (Bba) - Proteins Proteomics 1804, 263–274. doi:10.1016/j.bbapap.2009.11.005
Ahmad, P., Umar, S., and Sharma, S. (2010). Mechanism of Free Radical Scavenging and Role of Phytohormones in Plants under Abiotic Stresses. Plant Adaptation and Phytoremediation, 99–118. doi:10.1007/978-90-481-9370-7_5
Alscher, R. G., Erturk, N., and Heath, L. S. (2002). Role of Superoxide Dismutases (SODs) in Controlling Oxidative Stress in Plants. J. Exp. Bot. 53, 1331–1341. doi:10.1093/jexbot/53.372.1331
Altschul, S. F., Gish, W., Miller, W., Myers, E. W., and Lipman, D. J. (1990). Basic Local Alignment Search Tool. J. Mol. Biol. 215, 403–410. doi:10.1016/S0022-2836(05)80360-2
Alvarez-Buylla, E. R., Pelaz, S., Liljegren, S. J., Gold, S. E., Burgeff, C., Ditta, G. S., et al. (2000). An Ancestral MADS-Box Gene Duplication Occurred before the Divergence of Plants and Animals. Proc. Natl. Acad. Sci. 97, 5328–5333. doi:10.1073/pnas.97.10.5328
Asensio, A. C., Gil-Monreal, M., Pires, L., Gogorcena, Y., Aparicio-Tejo, P. M., and Moran, J. F. (2012). Two Fe-Superoxide Dismutase Families Respond Differently to Stress and Senescence in Legumes. J. Plant Physiol. 169, 1253–1260. doi:10.1016/j.jplph.2012.04.019
Atkinson, N. J., Lilley, C. J., and Urwin, P. E. (2013). Identification of Genes Involved in the Response of Arabidopsis to Simultaneous Biotic and Abiotic Stresses. Plant Physiol. 162, 2028–2041. doi:10.1104/pp.113.222372
Baek, K.-H., and Skinner, D. Z. (2003). Alteration of Antioxidant Enzyme Gene Expression during Cold Acclimation of Near-Isogenic Wheat Lines. Plant Sci. 165, 1221–1227. doi:10.1016/S0168-9452(03)00329-7
Bafana, A., Dutt, S., Kumar, S., and Ahuja, P. S. (2011). Superoxide Dismutase: An Industrial Perspective. Crit. Rev. Biotechnol. 31, 65–76. doi:10.3109/07388551.2010.490937
Bailey, T. L., Boden, M., Buske, F. A., Frith, M., Grant, C. E., Clementi, L., et al. (2009). MEME Suite: Tools for Motif Discovery and Searching. Nucleic Acids Res. 37, W202–W208. doi:10.1093/nar/gkp335
Ballal, A., and Manna, A. C. (2009). Regulation of Superoxide Dismutase ( Sod ) Genes by SarA in Staphylococcus aureus. J. Bacteriol. 191, 3301–3310. doi:10.1128/JB.01496-08
Chen, C., Chen, H., Zhang, Y., Thomas, H. R., Frank, M. H., He, Y., et al. (2020). TBtools: An Integrative Toolkit Developed for Interactive Analyses of Big Biological Data. Mol. Plant 13, 1194–1202. doi:10.1016/j.molp.2020.06.009
Conesa, A., and Götz, S. (2008). Blast2GO: A Comprehensive Suite for Functional Analysis in Plant Genomics. Int. J. Plant Genomics 2008, 1–12. doi:10.1155/2008/619832
Cramer, G. R., Urano, K., Delrot, S., Pezzotti, M., and Shinozaki, K. (2011). Effects of Abiotic Stress on Plants: a Systems Biology perspective.Pdf (Application/pdf Object), BMC Plant Biol., 11, 1–14. Available at: http://www.biomedcentral.com/content/pdf/1471-2229-11-163.pdf.doi:10.1186/1471-2229-11-163
Cui, L., Huang, Q., Yan, B., Wang, Y., Qian, Z., Pan, J., et al. (2015). Molecular Cloning and Expression Analysis of a Cu/Zn SOD Gene (BcCSD1) from Brassica Campestris Ssp. Chinensis. Food Chem. 186, 306–311. doi:10.1016/j.foodchem.2014.07.121
Dai, X., Zhuang, Z., and Zhao, P. X. (2018). PsRNATarget: A Plant Small RNA Target Analysis Server (2017 Release). Nucleic Acids Res. 46, W49–W54. doi:10.1093/nar/gky316
Dehury, B., Sarma, K., Sarmah, R., Sahu, J., Sahoo, S., Sahu, M., et al. (2013). In Silico analyses of Superoxide Dismutases (SODs) of rice (Oryza Sativa L). J. Plant Biochem. Biotechnol. 22, 150–156. doi:10.1007/s13562-012-0121-6
Division, P., and Jambheshwar, G. (2011). Is Bottle Gourd a Natural Guard. Int. Res. J. Pharm. 2, 13–17.
Divya, K., Kavi Kishor, P. B., Bhatnagar-Mathur, P., Singam, P., Sharma, K. K., Vadez, V., et al. (2019). Isolation and Functional Characterization of Three Abiotic Stress-Inducible (Apx, Dhn and Hsc70) Promoters from Pearl Millet (Pennisetum Glaucum L). Mol. Biol. Rep. 46, 6039–6052. doi:10.1007/s11033-019-05039-4
Feng, K., Yu, J., Cheng, Y., Ruan, M., Wang, R., Ye, Q., et al. (2016). The SOD Gene Family in Tomato: Identification, Phylogenetic Relationships, and Expression Patterns. Front. Plant Sci. 7, 1–12. doi:10.3389/fpls.2016.01279
Feng, X., Lai, Z., Lin, Y., Lai, G., and Lian, C. (2015). Genome-wide Identification and Characterization of the Superoxide Dismutase Gene Family in Musa Acuminata Cv. Tianbaojiao (AAA Group). BMC Genomics 16, 1–16. doi:10.1186/s12864-015-2046-7
Fernández-Ocaña, A., Chaki, M., Luque, F., Gómez-Rodríguez, M. V., Carreras, A., Valderrama, R., et al. (2011). Functional Analysis of Superoxide Dismutases (SODs) in sunflower under Biotic and Abiotic Stress Conditions. Identification of Two New Genes of Mitochondrial Mn-SOD. J. Plant Physiol. 168, 1303–1308. doi:10.1016/j.jplph.2011.01.020
Fink, R. C., and Scandalios, J. G. (2002). Molecular Evolution and Structure-Function Relationships of the Superoxide Dismutase Gene Families in Angiosperms and Their Relationship to Other Eukaryotic and Prokaryotic Superoxide Dismutases. Arch. Biochem. Biophys. 399, 19–36. doi:10.1006/abbi.2001.2739
Fi̇li̇z, E., Koç, İ., and Tombuloğlu, H. (2014). Genome-wide Identification and Analysis of Growth Regulating Factor Genes in Brachypodium Distachyon: In Silico Approaches. Turk J. Biol. 38, 296–306. doi:10.3906/biy-1308-57
Fi̇li̇z, E., and Tombuloğlu, H. (2015). Genome-wide Distribution of Superoxide Dismutase (SOD) Gene Families in Sorghum Bicolor. Turk J. Biol. 39, 49–59. doi:10.3906/biy-1403-9
Gasteiger, E., Hoogland, C., Gattiker, A., Duvaud, S. e., Wilkins, M. R., Appel, R. D., et al. (2005). Protein Identification and Analysis Tools on the ExPASy Server. The Proteomics Protoc. Handbook, 571–607. doi:10.1385/1-59259-890-0:571
Gill, S. S., and Tuteja, N. (2010). Reactive Oxygen Species and Antioxidant Machinery in Abiotic Stress Tolerance in Crop Plants. Plant Physiol. Biochem. 48, 909–930. doi:10.1016/j.plaphy.2010.08.016
Gómez, J. M., Hernández, J. A., Jiménez, A., Del Río, L. A., and Sevilla, F. (1999). Differential Response of Antioxidative Enzymes of Chloroplasts and Mitochondria to Long-Term NaCl Stress of Pea Plants. Free Radic. Res. 31, 11–18. doi:10.1080/10715769900301261
Gosavi, G. U., Jadhav, A. S., Kale, A. A., Gadakh, S. R., Pawar, B. D., and Chimote, V. P. (2014). Effect of Heat Stress on Proline, Chlorophyll Content, Heat Shock Proteins and Antioxidant Enzyme Activity in Sorghum (Sorghum Bicolor) at Seedlings Stage. Indian J. Biotechnol. 13, 356–363.
Guan, Q., Lu, X., Zeng, H., Zhang, Y., and Zhu, J. (2013). Heat Stress Induction ofmiR398triggers a Regulatory Loop that Is Critical for Thermotolerance in Arabidopsis. Plant J. 74, 840–851. doi:10.1111/tpj.12169
Han, L.-m., Hua, W.-p., Cao, X.-y., Yan, J.-a., Chen, C., and Wang, Z.-z. (2020). Genome-wide Identification and Expression Analysis of the Superoxide Dismutase (SOD) Gene Family in Salvia Miltiorrhiza. Gene 742, 144603. doi:10.1016/j.gene.2020.144603
Hasanuzzaman, M., Bhuyan, M. H. M. B., Zulfiqar, F., Raza, A., Mahmud, J., Fujita, M., et al. (2020). Reactive Oxygen Species and Antioxidant Defense in Plants under Abiotic Stress : Revisiting the Crucial Role of a Universal Defense Regulator. Antioxidants 9, 681. doi:10.3390/antiox9080681
Higo, K., Ugawa, Y., Iwamoto, M., and Korenaga, T. (1999). Plant Cis-Acting Regulatory DNA Elements (PLACE) Database: 1999. Nucleic Acids Res. 27, 297–300. doi:10.1093/nar/27.1.297
Ho, K.-H., Chen, P.-H., Hsi, E., Shih, C.-M., Chang, W.-C., Cheng, C.-H., et al. (2017). Identification of IGF-1-Enhanced Cytokine Expressions Targeted by miR-181d in Glioblastomas via an Integrative miRNA/mRNA Regulatory Network Analysis. Sci. Rep. 7, 1–13. doi:10.1038/s41598-017-00826-0
Horton, P., Park, K.-J., Obayashi, T., Fujita, N., Harada, H., Adams-Collier, C. J., et al. (2007). WoLF PSORT: Protein Localization Predictor. Nucleic Acids Res. 35, W585–W587. doi:10.1093/nar/gkm259
Hu, B., Jin, J., Guo, A.-Y., Zhang, H., Luo, J., and Gao, G. (2015). GSDS 2.0: An Upgraded Gene Feature Visualization Server. Bioinformatics 31, 1296–1297. doi:10.1093/bioinformatics/btu817
Hu, L., Yang, Y., Jiang, L., and Liu, S. (2016). The Catalase Gene Family in Cucumber: Genome-wide Identification and Organization. Genet. Mol. Biol. 39, 408–415. doi:10.1590/1678-4685-gmb-2015-0192
Hu, X., Hao, C., Cheng, Z.-M., and Zhong, Y. (2019). Genome-wide Identification, Characterization, and Expression Analysis of the grapevine Superoxide Dismutase (SOD) Family. Int. J. Genomics 2019, 1–13. doi:10.1155/2019/7350414
Huang, D. W., Sherman, B. T., and Lempicki, R. A. (2009). Bioinformatics Enrichment Tools: Paths toward the Comprehensive Functional Analysis of Large Gene Lists. Nucleic Acids Res. 37, 1–13. doi:10.1093/nar/gkn923
Huang, H., Wang, H., Tong, Y., and Wang, Y. (2020). Insights into the Superoxide Dismutase Gene Family and its Roles in Dendrobium Catenatum under Abiotic Stresses. Plants 9 (11), 1452. doi:10.3390/plants9111452
Jagadeeswaran, G., Saini, A., and Sunkar, R. (2009). Biotic and Abiotic Stress Down-Regulate miR398 Expression in Arabidopsis. Planta 229, 1009–1014. doi:10.1007/s00425-009-0889-3
Jia, X., Wang, W.-X., Ren, L., Chen, Q.-J., Mendu, V., Willcut, B., et al. (2009). Differential and Dynamic Regulation of miR398 in Response to ABA and Salt Stress in Populus Tremula and Arabidopsis thaliana. Plant Mol. Biol. 71, 51–59. doi:10.1007/s11103-009-9508-8
Karuppanapandian, T., Wang, H. W., Prabakaran, N., Jeyalakshmi, K., Kwon, M., Manoharan, K., et al. (2011). 2,4-dichlorophenoxyacetic Acid-Induced Leaf Senescence in Mung Bean (Vigna Radiata L. Wilczek) and Senescence Inhibition by Co-treatment with Silver Nanoparticles. Plant Physiol. Biochem. 49, 168–177. doi:10.1016/j.plaphy.2010.11.007
Kim, E.-J., Kim, H.-P., Hah, Y. C., and Roe, J.-H. (1996). Differential Expression of Superoxide Dismutases Containing Ni and Fe/Zn in Streptomyces Coelicolor. Eur. J. Biochem. 241, 178–185. doi:10.1111/j.1432-1033.1996.0178t.x
Kliebenstein, D. J., Monde, R.-A., and Last, R. L. (1998). Superoxide Dismutase in Arabidopsis: An eclectic Enzyme Family with Disparate Regulation and Protein Localization. Plant Physiol. 118, 637–650. doi:10.1104/pp.118.2.637
Kliebenstein, D. J., Monde, R., and Last, R. L. (2020). Superoxide Dismutase in Arabidopsis : An Eclectic Enzyme Family with Disparate Regulation and Protein Localization 1. Plant Physiol. 118, 637–650. doi:10.1104/pp.118.2.637
Kondrashov, F. A. (2012). Gene Duplication as a Mechanism of Genomic Adaptation to a Changing Environment. Proc. R. Soc. B. 279, 5048–5057. doi:10.1098/rspb.2012.1108
Kumar, S., Stecher, G., and Tamura, K. (2016). MEGA7: Molecular Evolutionary Genetics Analysis Version 7.0 for Bigger Datasets. Mol. Biol. Evol. 33, 1870–1874. doi:10.1093/molbev/msw054
Kurepa, J., Van Montagu, M., and Inzé, D. (1997). Expression of sodCp and sodB Genes in Nicotiana Tabacum: Effects of Light and Copper Excess. J. Exp. Bot. 48, 2007–2014. doi:10.1093/jexbot/48.317.2007
Lee, S.-H., Ahsan, N., Lee, K.-W., Kim, D.-H., Lee, D.-G., Kwak, S.-S., et al. (2007). Simultaneous Overexpression of Both CuZn Superoxide Dismutase and Ascorbate Peroxidase in Transgenic Tall Fescue Plants Confers Increased Tolerance to a Wide Range of Abiotic Stresses. J. Plant Physiol. 164, 1626–1638. doi:10.1016/j.jplph.2007.01.003
Lescot, M., Déhais, P., Thijs, G., Marchal, K., Moreau, Y., Van De Peer, Y., et al. (2002). PlantCARE, a Database of Plant Cis-Acting Regulatory Elements and a portal to Tools for In Silico Analysis of Promoter Sequences. Nucleic Acids Res. 30, 325–327. doi:10.1093/nar/30.1.325
Letunic, I., and Bork, P. (2019). Interactive Tree of Life (iTOL) V4: Recent Updates and New Developments. Nucleic Acids Res. 47, W256–W259. doi:10.1093/nar/gkz239
Li, Q., Jin, X., and Zhu, Y.-X. (2012). Identification and Analyses of miRNA Genes in Allotetraploid Gossypium Hirsutum Fiber Cells Based on the Sequenced Diploid G. Raimondii Genome. J. Genet. Genomics 39, 351–360. doi:10.1016/j.jgg.2012.04.008
Lin, Y.-L., and Lai, Z.-X. (2013). Superoxide Dismutase Multigene Family in Longan Somatic Embryos: A Comparison of CuZn-SOD, Fe-SOD, and Mn-SOD Gene Structure, Splicing, Phylogeny, and Expression. Mol. Breed. 32, 595–615. doi:10.1007/s11032-013-9892-2
Liu, J., Xu, L., Shang, J., Hu, X., Yu, H., Wu, H., et al. (2021). Gene Family Reveals Important Roles in Drought and Salt Responses. Genet. Mol. Biol. 44, 20210035. doi:10.1590/1678-4685-gmb-2021-0035
Lomakina, N. (2018). Forming Long-Term Competitiveness of a mineral Sector as Part of Economy Innovative Development Strategy. E3s Web Conf. 56, 04010–04148. doi:10.1051/e3sconf/20185604010
Lu, W., Duanmu, H., Qiao, Y., Jin, X., Yu, Y., Yu, L., et al. (2020). Genome-wide Identification and Characterization of the Soybean SOD Family during Alkaline Stress. PeerJ 8, e8457–19. doi:10.7717/peerj.8457
Lu, Y., Feng, Z., Bian, L., Xie, H., and Liang, J. (2011). miR398 Regulation in rice of the Responses to Abiotic and Biotic Stresses Depends on CSD1 and CSD2 Expression. Funct. Plant Biol. 38, 44. doi:10.1071/fp10178
Mann, T., and Keilin, D. (1938). Haemocuprein and Hepatocuprein, Copper-Protein Compounds of Blood and Liver in Mammals. Proc. R. Soc. Lond. Ser. B - Biol. Sci. 126, 303–315. doi:10.1098/rspb.1938.0058
Manzoor, M. A., Cheng, X., Li, G., Su, X., Abdullah, M., and Cai, Y. (2020). Gene Structure, Evolution and Expression Analysis of the P-ATPase Gene Family in Chinese Pear (Pyrus Bretschneideri). Comput. Biol. Chem. 88, 107346. doi:10.1016/j.compbiolchem.2020.107346
Manzoor, M. A., Li, G., Abdullah, M., Han, W., Wenlong, H., Yang, Z., et al. (2021a). Genome-wide Investigation and Comparative Analysis of MATE Gene Family in Rosaceae Species and Their Regulatory Role in Abiotic Stress Responses in Chinese Pear ( Pyrus Bretschneideri ). Physiologia Plantarum 173, 1163–1178. doi:10.1111/ppl.13511
Manzoor, M. A., Manzoor, M. M., Li, G., Abdullah, M., Han, W., Wenlong, H., et al. (2021b). Genome-wide Identification and Characterization of bZIP Transcription Factors and Their Expression Profile under Abiotic Stresses in Chinese Pear (Pyrus Bretschneideri). BMC Plant Biol. 21, 1–16. doi:10.1186/s12870-021-03191-3
McCord, J. M., and Fridovich, I. (1969). Superoxide Dismutase. An Enzymic Function for Erythrocuprein (Hemocuprein). J. Biol. Chem. 244, 6049–6055.
Miller, A.-F. (2012). Superoxide Dismutases: Ancient Enzymes and New Insights. FEBS Lett. 586, 585–595. doi:10.1016/j.febslet.2011.10.048
Mistry, J., Chuguransky, S., Williams, L., Qureshi, M., Salazar, G. A., Sonnhammer, E. L. L., et al. (2021). Pfam: The Protein Families Database in 2021. Nucleic Acids Res. 49, D412–D419. doi:10.1093/nar/gkaa913
Mittler, R., and Blumwald, E. (2010). Genetic Engineering for Modern Agriculture: Challenges and Perspectives. Annu. Rev. Plant Biol. 61, 443–462. doi:10.1146/annurev-arplant-042809-112116
Mittler, R., Vanderauwera, S., Gollery, M., and Van Breusegem, F. (2004). Reactive Oxygen Gene Network of Plants. Trends Plant Sci. 9, 490–498. doi:10.1016/j.tplants.2004.08.009
Molina-Rueda, J. J., Tsai, C. J., and Kirby, E. G. (2013). The Populus Superoxide Dismutase Gene Family and its Responses to Drought Stress in Transgenic Poplar Overexpressing a Pine Cytosolic Glutamine Synthetase (GS1a). PLoS One 8, e56421. doi:10.1371/journal.pone.0056421
Møller, I. M. (2001). PLANT MITOCHONDRIA and OXIDATIVE STRESS: Electron Transport, NADPH Turnover, and Metabolism of Reactive Oxygen Species. Annu. Rev. Plant Physiol. Plant Mol. Biol. 52, 561–591. doi:10.1146/annurev.arplant.52.1.561
Nath, K., Kumar, S., Poudyal, R. S., Yang, Y. N., Timilsina, R., Park, Y. S., et al. (2014). Developmental Stage-dependent Differential Gene Expression of Superoxide Dismutase Isoenzymes and Their Localization and Physical Interaction Network in rice (Oryza Sativa L). Genes Genom 36, 45–55. doi:10.1007/s13258-013-0138-9
Nijhawan, A., Jain, M., Tyagi, A. K., and Khurana, J. P. (2008). Genomic Survey and Gene Expression Analysis of the Basic Leucine Zipper Transcription Factor Family in rice. Plant Physiol. 146, 323–324. doi:10.1104/pp.107.112821
Oppenheim, A. V., Willsky, A. S., and Hamid, S. (1996). Signals and Systems. 2nd Edition, 18, 957. doi:10.1105/tpc.106.041673.1
Pilon, M., Ravet, K., and Tapken, W. (2011). The Biogenesis and Physiological Function of Chloroplast Superoxide Dismutases. Biochim. Biophys. Acta - Bioenerg. 1807, 989–998. doi:10.1016/j.bbabio.2010.11.002
Qiao, X., Li, Q., Yin, H., Qi, K., Li, L., Wang, R., et al. (2019). Gene Duplication and Evolution in Recurring Polyploidization-Diploidization Cycles in Plants. Genome Biol. 20, 1–23. doi:10.1186/s13059-019-1650-2
Qiao, X., Yin, H., Li, L., Wang, R., Wu, J., Wu, J., et al. (2018). Different Modes of Gene Duplication Show Divergent Evolutionary Patterns and Contribute Differently to the Expansion of Gene Families Involved in Important Fruit Traits in Pear (Pyrus Bretschneideri). Front. Plant Sci. 9, 1–16. doi:10.3389/fpls.2018.00161
Qiu, C. X., Xie, F. L., Zhu, Y. Y., Guo, K., Huang, S. Q., Nie, L., et al. (2007). Computational Identification of microRNAs and Their Targets in Gossypium Hirsutum Expressed Sequence Tags. Gene 395, 49–61. doi:10.1016/j.gene.2007.01.034
Rabinowitch, H. D., and Sklan, D. (1980). Superoxide Dismutase: A Possible Protective Agent against Sunscald in Tomatoes (Lycopersicon Esculentum mill). Planta 148, 162–167. doi:10.1007/BF00386417
Schultz, J., Milpetz, F., Bork, P., and Ponting, C. P. (1998). SMART, a Simple Modular Architecture Research Tool: Identification of Signaling Domains. Proc. Natl. Acad. Sci. 95, 5857–5864. doi:10.1073/pnas.95.11.5857
Schützendübel, A., and Polle, A. (2002). Plant Responses to Abiotic Stresses: Heavy Metal-Induced Oxidative Stress and protection by Mycorrhization. J. Exp. Bot. 53, 1351–1365. doi:10.1093/jxb/53.372.1351
Silva, S. A. F., Silva, F. L. B., Ribas, A. F., de Souza, S. G. H., and dos Santos, T. B. (2020). Genome-wide In Silico Analysis of SOD Genes in Common Bean (Phaseolus vulgaris L). J. Crop Sci. Biotechnol. 23, 241–251. doi:10.1007/s12892-020-00030-z
Song, J., Zeng, L., Chen, R., Wang, Y., and Zhou, Y. (2018). In Silico identification and Expression Analysis of Superoxide Dismutase (SOD) Gene Family in Medicago Truncatula. 3 Biotech. 8, 1–12. doi:10.1007/s13205-018-1373-1
Srivastava, V., Srivastava, M. K., Chibani, K., Nilsson, R., Rouhier, N., Melzer, M., et al. (2009). Alternative Splicing Studies of the Reactive Oxygen Species Gene Network in Populus Reveal Two Isoforms of High-Isoelectric-Point Superoxide Dismutase. Plant Physiol. 149, 1848–1859. doi:10.1104/pp.108.133371
Su, W., Raza, A., Gao, A., Jia, Z., Zhang, Y., Hussain, M. A., et al. (2021). Genome-wide Analysis and Expression Profile of Superoxide Dismutase (Sod) Gene Family in Rapeseed (brassica Napus l.) under Different Hormones and Abiotic Stress Conditions. Antioxidants 10, 1182. doi:10.3390/antiox10081182
Sunkar, R., Chinnusamy, V., Zhu, J., and Zhu, J.-K. (2007). Small RNAs as Big Players in Plant Abiotic Stress Responses and Nutrient Deprivation. Trends Plant Sci. 12, 301–309. doi:10.1016/j.tplants.2007.05.001
Tepperman, J. M., and Dunsmuir, P. (1990). Transformed Plants with Elevated Levels of Chloroplastic SOD Are Not More Resistant to Superoxide Toxicity. Plant Mol. Biol. 14, 501–511. doi:10.1007/BF00027496
Thompson, J., Gibson, T. J., Plewniak, F., Jeanmougin, F., and Higgins, D. G. (1997). The CLUSTAL_X Windows Interface: Flexible Strategies for Multiple Sequence Alignment Aided by Quality Analysis Tools. Nucleic Acids Res. 25, 4876–4882. doi:10.1093/nar/25.24.4876
Verma, D., Lakhanpal, N., and Singh, K. (2019). Genome-wide Identification and Characterization of Abiotic-Stress Responsive SOD (Superoxide Dismutase) Gene Family in Brassica Juncea and B. Rapa. BMC Genomics 20, 1–18. doi:10.1186/s12864-019-5593-5
Wang, B., Lüttge, U., and Ratajczak, R. (2004). Specific Regulation of SOD Isoforms by NaCl and Osmotic Stress in Leaves of the C3 Halophyte Suaeda Salsa L. J. Plant Physiol. 161, 285–293. doi:10.1078/0176-1617-01123
Wang, T., Song, H., Zhang, B., Lu, Q., Liu, Z., Zhang, S., et al. (2018). Genome-wide Identification, Characterization, and Expression Analysis of Superoxide Dismutase (SOD) Genes in Foxtail Millet (Setaria Italica L). 3 Biotech. 8, 1–11. doi:10.1007/s13205-018-1502-x
Wang, W., Xia, M., Chen, J., Deng, F., Yuan, R., Zhang, X., et al. (2016). Genome-wide Analysis of Superoxide Dismutase Gene Family in Gossypium Raimondii and G. Arboreum. Plant Gene 6, 18–29. doi:10.1016/j.plgene.2016.02.002
Wang, W., Zhang, X., Deng, F., Yuan, R., and Shen, F. (2017). Genome-wide Characterization and Expression Analyses of Superoxide Dismutase (SOD) Genes in Gossypium Hirsutum. BMC Genomics 18, 1–25. doi:10.1186/s12864-017-3768-5
Wang, Y., Tang, H., Debarry, J. D., Tan, X., Li, J., Wang, X., et al. (2012). MCScanX: A Toolkit for Detection and Evolutionary Analysis of Gene Synteny and Collinearity. Nucleic Acids Res. 40, e49. doi:10.1093/nar/gkr1293
Wei, K., Chen, J., Wang, Y., Chen, Y., Chen, S., Lin, Y., et al. (2012). Genome-wide Analysis of bZIP-Encoding Genes in maize. DNA Res. 19, 463–476. doi:10.1093/dnares/dss026
Wu, G., Wilen, R. W., Robertson, A. J., and Gusta, L. V. (1999). Isolation, Chromosomal Localization, and Differential Expression of Mitochondrial Manganese Superoxide Dismutase and Chloroplastic Copper/Zinc Superoxide Dismutase Genes in Wheat1. Plant Physiol. 120, 513–520. doi:10.1104/pp.120.2.513
Wuerges, J., Lee, J.-W., Yim, Y.-I., Yim, H.-S., Kang, S.-O., and Djinovic Carugo, K. (2004). Crystal Structure of Nickel-Containing Superoxide Dismutase Reveals Another Type of Active Site. Proc. Natl. Acad. Sci. U. S. A. 101, 8569–8574. doi:10.1073/pnas.0308514101
Xu, G., Guo, C., Shan, H., and Kong, H. (2012). Divergence of Duplicate Genes in Exon-Intron Structure. Proc. Natl. Acad. Sci. 109, 1187–1192. doi:10.1073/pnas.1109047109
Yan, J.-J., Zhang, L., Wang, R.-Q., Xie, B., Li, X., Chen, R.-L., et al. (2016). The Sequence Characteristics and Expression Models Reveal Superoxide Dismutase Involved in Cold Response and Fruiting Body Development in Volvariella Volvacea. Int. J. Mol. Sci. 17, 34. doi:10.3390/ijms17010034
Youn, H.-D., Kim, E.-J., Roe, J.-H., Hah, Y. C., and Kang, S.-O. (1996). A Novel Nickel-Containing Superoxide Dismutase from Streptomyces Spp. Biochem. J. 318, 889–896. doi:10.1042/bj3180889
Zang, Y., Chen, J., Li, R., Shang, S., and Tang, X. (2020). Genome-wide Analysis of the Superoxide Dismutase (SOD) Gene Family in Zostera marina and Expression Profile Analysis under Temperature Stress. PeerJ 8, e9063–18. doi:10.7717/peerj.9063
Zelko, I. N., Mariani, T. J., and Folz, R. J. (2002). Superoxide Dismutase Multigene Family: A Comparison of the CuZn-SOD (SOD1), Mn-SOD (SOD2), and EC-SOD (SOD3) Gene Structures, Evolution, and Expression. Free Radic. Biol. Med. 33, 337–349. doi:10.1016/S0891-5849(02)00905-X
Zhang, C., Zheng, H., Wu, X., Xu, H., Han, K., Peng, J., et al. (2018). Genome-wide Identification of New Reference Genes for RT-qPCR Normalization in CGMMV-Infected Lagenaria Siceraria. PeerJ 6, e5642. doi:10.7717/peerj.5642
Zhang, J., Li, B., Yang, Y., Hu, W., Chen, F., Xie, L., et al. (2016). Genome-Wide Characterization and Expression Profiles of the Superoxide Dismutase Gene Family in Gossypium. Int. J. Genomics 2016, 1–11. doi:10.1155/2016/8740901
Zhang, X., Zhang, L., Chen, Y., Wang, S., Fang, Y., Zhang, X., et al. (2021). Genome-wide Identification of the SOD Gene Family and Expression Analysis under Drought and Salt Stress in Barley. Plant Growth Regul. 94, 49–60. doi:10.1007/s10725-021-00695-8
Zheng, Y., Wu, S., Bai, Y., Sun, H., Jiao, C., Guo, S., et al. (2019). Cucurbit Genomics Database (CuGenDB): A central portal for Comparative and Functional Genomics of Cucurbit Crops. Nucleic Acids Res. 47, D1128–D1136. doi:10.1093/nar/gky944
Keywords: LsiSOD genes, phylogenetic analysis, stress response, duplication events, expression pattern
Citation: Rehman S, Rashid A, Manzoor MA, Li L, Sun W, Riaz MW, Li D and Zhuge Q (2022) Genome-Wide Evolution and Comparative Analysis of Superoxide Dismutase Gene Family in Cucurbitaceae and Expression Analysis of Lagenaria siceraria Under Multiple Abiotic Stresses. Front. Genet. 12:784878. doi: 10.3389/fgene.2021.784878
Received: 28 September 2021; Accepted: 30 December 2021;
Published: 08 February 2022.
Edited by:
Reyazul Rouf Mir, Sher-e-Kashmir University of Agricultural Sciences and Technology, IndiaReviewed by:
Ali Raza, Fujian Agriculture and Forestry University, ChinaIrfan Ali Sabir, Shanghai Jiao Tong University, China
Haidong Yan, University of Georgia, United States
Copyright © 2022 Rehman, Rashid, Manzoor, Li, Sun, Riaz, Li and Zhuge. This is an open-access article distributed under the terms of the Creative Commons Attribution License (CC BY). The use, distribution or reproduction in other forums is permitted, provided the original author(s) and the copyright owner(s) are credited and that the original publication in this journal is cited, in accordance with accepted academic practice. No use, distribution or reproduction is permitted which does not comply with these terms.
*Correspondence: Qiang Zhuge, qzhuge@njfu.edu.cn