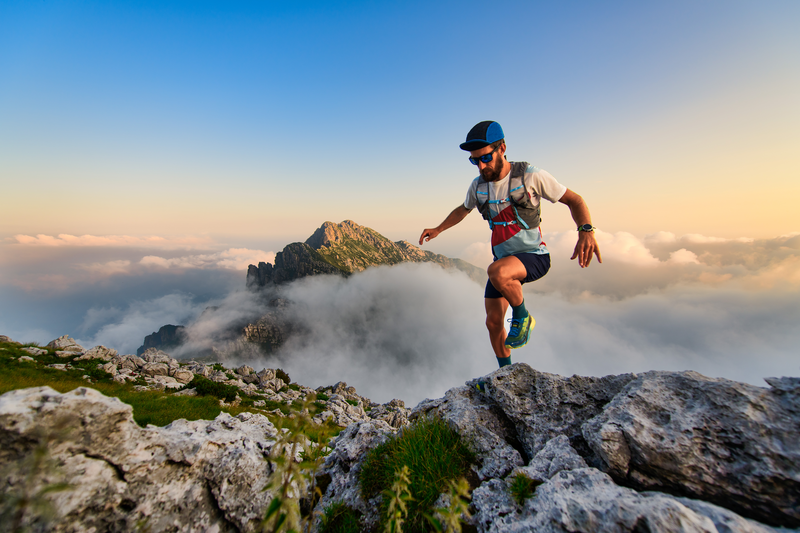
94% of researchers rate our articles as excellent or good
Learn more about the work of our research integrity team to safeguard the quality of each article we publish.
Find out more
PERSPECTIVE article
Front. Food. Sci. Technol. , 07 February 2025
Sec. Food Biotechnology
Volume 5 - 2025 | https://doi.org/10.3389/frfst.2025.1507933
This article is part of the Research Topic Superfoods Production, Acceptance, and Quality: From Farm to Fork View all articles
“Superfoods” is a marketing term used to describe nutrient-dense foods claimed to have health benefits due to their high concentrations of essential amino acids, vitamins, minerals, antioxidants, and other bioactive compounds. Recently, the need for healthy diets has led to increased demand for these functional foods, which have become popular among middle- and high-income groups in developed regions of the earth. Superfoods can represent a smart way to improve diets, particularly in relation to diet-related issues. However, the superfood production system, as well as the broader food production system, must be reconsidered to avoid overexploitation of soil, workers, and natural resources. This perspective explores sustainability of “superfoods” by proposing a new classification system, dividing them into “synthesizers” and “accumulators” based on their origin. The aim is to provide a framework for identifying and promoting superfoods that align with environmental and social sustainability goals, helping guide both consumers and producers toward more responsible choices in food systems. Analyzing the sources and characteristics of the main compounds found in superfoods reveals that most of them come from plants or bacteria, rather than animal origins. Since animal-based food production is one of the largest contributors to greenhouse gas emissions in the agro-food sector and a major driver of deforestation and water use, it is advisable to focus on plant organisms as the primary and direct sources of beneficial compounds. Nevertheless, great attention must be paid to vegetable crops that imply an overexploitation of soil and water and induce the degradation of entire ecosystems.
The term “superfood,” originally coined in the 60s to describe foods capable of fighting malnutrition (Jelliffe, 1966), has seen a significant change in meaning since the 2000s, becoming a target for marketing strategies. Nowadays, no specific scientific standards define what constitutes a superfood, but the term generally refers to a group of nutrient-dense foods and bioactive compounds with positive effects on human health.
In various religious traditions, there are narratives of foods with “miraculous effects”. A well-known example is manna, described in the Old Testament as food provided by God to men during the exodus from Egypt. Manna is actually the sugary sap of plants of the genus Fraxinus, in particular Fraxinus ornus (Włodarczyk, 2007). Foods such as honey, almonds and olives are mentioned in the Bible and appreciated for their nutritional properties, while the Quran contains accounts of miracles linked to food performed by prophets, and Hindu scriptures recount divine food in times of need.
Aside from the metaphysical aspects of recalling symbolic episodes or imitating the Divine, there are certainly traditions of foods that lie outside of sacred texts. For instance, traditional balsamic vinegar of Modena has been administered by the spoonful to children, as well as cooked must (made from grapes or figs), fruit compotes and preserves are other examples of foods enjoyed on special festive occasions (Montevecchi et al., 2010; Vasile Simone et al., 2013).
The variety of foods considered superfoods varies globally. In some cultures, local berries and fruits are preferred, while in others, fatty fish or whole grains are valued. This diversity shows that the definition of superfood fits the dietary needs of each culture.
In the most current sense, from a compositional perspective, a superfood is characterized by its “high nutritional density,” in terms of vitamins and other essential nutrients (e.g., fatty acids, amino acids), minerals, soluble fibers, amino acid analogues like L-theanine (Montevecchi, 2005), as well as bioactive compounds with antioxidant activity (Manach et al., 2004). Superfoods consumption is often associated with a reduction in the risk of chronic diseases, non-communicable diseases, and support for the immune and cognitive systems (Rasouli et al., 2017). In addition, many superfoods are minimally processed, thus maintaining nutritional quality and facilitating their integration into the daily diet.
Garlic (Allium sativum) and ginger (Zingiber officinale) are widely regarded as superfoods due to their health properties. Garlic, rich in allicin, has antithrombotic, antimicrobial and anti-inflammatory effects, contributing to reduced LDL cholesterol and blood pressure (Bongiorno et al., 2008). Ginger, containing gingerol, relieves pain and inflammation, improving motor skills (Mohd and Makpol, 2019). Red yeast rice (or red fermented rice), rich in monacolin K, is another superfood known for its effect in controlling LDL cholesterol (EFSA Panel et al., 2018).
In recent times, the pandemic has increased interest in foods capable of boosting immunity and protecting against pathogens. This list includes foods such as ginseng (Panax sp. pl.), eleuthero (Eleutherococcus senticosus, also known as Siberian ginseng or devil’s bush), echinacea (Echinacea sp. pl.), goji berries (Lycium barbarum), aloe (Aloe vera), turmeric (Curcuma longa), gingko (Ginko biloba), or individual molecules such as quercetin (a flavonoid found in many plants) and chloroquine (a synthetic derivative of quinine, a natural alkaloid originally extracted from the bark of the Andean plant Cinchona, known and used as a medication with antipyretic, antimalarial and analgesic properties). These foods are considered as potential panaceas in extreme situations. In this context, superfoods can represent not only a nutritional option, but also a way to address public health challenges.
The global superfoods market is projected to grow from a minimum estimated size of 155.2 billion dollars in 2022 to a maximum of 344.9 billion dollars by 2033, with a compound annual growth rate (CAGR) ranging between 4.0% and 10.2% during the forecast period, depending on the information source (Globenewswire.com, 2025; Grandviewresearch.com, 2025; Marketresearch.com, 2025; Market.us, 2025; Mordorintelligence.com, 2025).
Several countries dominate the global market, each excelling in the production of specific superfoods due to their agricultural traditions and favorable climates. The United States, within the largest market of North America, leads in cultivating and innovating with superfoods like kale, quinoa, and blueberries. Peru plays a critical role as a leading exporter of quinoa and Andean crops such as maca root. China, part of the fastest-growing market in Asia-Pacific, is the top producer of goji berries and spirulina, supplying most global exports. India is a major player in moringa and turmeric production, driven by its Ayurvedic heritage and government support for exports. Japan is renowned for matcha green tea, a growing favorite in Western markets.
Beyond economic projections, it is crucial to consider that the growing popularity of superfoods necessitates assessing their production impacts on environmental, economic, and social sustainability. This perspective paper aims to establish a novel classification system that distinguishes superfoods based on their origin—synthesizers or accumulators. By analyzing these categories, we provide insight into how superfoods can be produced and consumed responsibly, contributing to sustainable dietary practices and minimizing adverse impacts on natural resources and ecosystems.
Although superfoods are currently experiencing great popularity, it is crucial to reflect on the potential issues associated with their global consumption, as seen with other foods or raw materials. Growing demand can lead to unsustainable agricultural practices and, in turn, a reduction in biodiversity. Therefore, responsible consumption and the choice of local and sustainable superfoods can contribute not only to a healthy diet but also to a more resilient use of the planet’s resources.
A prominent example is the case of palm oil (Elaeis guineensis), whose growing demand has led to dramatic changes in Southeast Asian ecosystems, with severe consequences for biodiversity and human rights. Deforestation for its cultivation has triggered conflicts with indigenous communities and contributed to the climate crisis (Mukherjee and Sovacool, 2014; Ardiansyah, 2006). Similar situations can be observed in the Amazon, where deforestation for large-scale agriculture threatens biodiversity and the wellbeing of local populations (da Silveira Bueno et al., 2021). The production of cocoa and coffee is also widely associated with issues such as child labor and deforestation (Bertrand and de Buhr, 2019).
Overfishing undermines global food security. Fish such as bluefin tuna (Thunnus thynnus) and salmon (Salmo salar) have experienced drastic population declines due to growing demand (Knudsen et al., 2020). In addition, animal welfare issues are evident in luxury foods such as “civet coffee,” obtained through invasive practices on civets (Paradoxurus hermaphroditus) (Carder et al., 2016). Furthermore, there has long been strong opposition to the production of foie gras and caviar (Skippon, 2013). The rising global food demand, driven by population growth, is causing environmental and social concerns due to the corresponding increase in the consumption of animal-based products (meat, fish, and dairy), which is projected to grow by up to 8.5% over the next decade (OECD-FAO, 2023). Since livestock and other forms of animal farming contribute significantly to greenhouse gas emissions (Poore and Nemecek, 2018), the shifting to a sustainable plant-based protein production, performed with low-impact systems, is crucial for addressing this growing demand while mitigating environmental harmful effects.
Considering the points discussed, it is essential to classify superfoods based on principles of environmental, social, economic and ethical sustainability. It is urgent and necessary to avoid devastating aberrations such as deforestation to establish Lycium plantations for goji berry production, or the use of anabolic doping to achieve the “world record nutritional performance” in salmon. Moreover, it is crucial to limit the exploitation of natural resources for animal feed and farming, currently applied as the primary source of proteins production (Bryant, 2022). Gaining a deeper understanding of nutrient synthesizers and accumulators could support the food technology and production sectors in advancing a sustainable transition.
On the other hand, consumer’s perceptions of synthetic molecules with bioactive properties can vary greatly depending on several factors such as culture, knowledge, health concerns, and risk perception. Synthetic vitamin C consists solely of ascorbic acid, while natural vitamin C is obtained, for instance, from the rose hips of Rosa canina. This fruit contains a phytocomplex that, in addition to ascorbic acid, also includes bioflavonoids, enzymes, coenzymes and other factors, that enhance the effectiveness of vitamin C. Similarly, natural vitamin E that includes tocopherols and tocotrienols–and not just alpha-tocopherol–is more effective than its synthetic equivalent (Mindell, 1991).
A synthetic substance can cause a reaction in a person susceptible to certain chemicals, while the same substance of natural origin is often better tolerated, despite having an identical chemical structure. This may be due to more gradual absorption. An emblematic example is fructose, which is widely abundant in fruit without raising significant concerns, whereas fructose used as a sweetener is universally recognized as hepatotoxic, with effects similar to ethanol (Lustig, 2013). In general, natural compounds tend to cause fewer gastrointestinal issues, and toxic reactions are less common when consumed in doses higher than recommended.
The classification proposed in this prospective article allows superfoods to be divided into two categories based on their origins:
- organisms that synthesize nutrients and bioactive molecules;
- organisms that accumulate nutrients and bioactive molecules, synthesized by the former.
In this regard, Table 1 shows a list of the main essential nutrients.
Table 1. Breakdown of nutrients in the human diet according to source of origin: synthesizing organisms and accumulating organisms.
Vitamin A is essential for vision and cellular health and is mainly obtained through precursors such as beta-carotene, which is synthesized by plants, algae, and microorganisms. Animals, unable to synthesize vitamin A on their own, obtain it through their diet and store it in organs such as the liver (Rafeeq et al., 2020).
B vitamins include water-soluble compounds vital for cellular metabolism, though they do not necessarily share structural or functional characteristics (Said, 2015). Plants can synthesize most vitamins of the B group, although B12 is not synthesized by plants at all and must be obtained from other sources (LeBlanc et al., 2013). B vitamins are water-soluble and are generally not accumulated in large quantities in organisms, with only limited storage possible in animal tissues.
Humans cannot synthesize these vitamins and must obtain them from the diet or from the gut microbiota, which produces some B vitamins, such as B7 or small amounts of vitamin B12 (LeBlanc et al., 2013). However, these quantities are sometimes insufficient or are produced in distal regions of the intestine (large intestine) that do not allow for adequate absorption. In addition, there are several chemical forms of vitamin B12. Cyanocobalamin (an artifact formed during extraction with papain) and hydroxocobalamin (of microbial origin) are “active” forms, while green algae and blue algae (such as cyanobacterium Spirulina) may contain pseudo-vitamin B12, a form that is not bioavailable for humans (Watanabe and Bito, 2018). On the other hand, the multicellular alga known as Nori (Porphyra spp.) contains an active form of vitamin B12, which can be absorbed and used by the human body (Watanabe et al., 2013).
The intake of vitamin B12 is a critical concern for those following vegetarian, and especially vegan, diets (Watanabe et al., 2014). Therefore, an interesting nutritional challenge is finding non-animal alternatives without resorting to supplements. Tempeh (fermented soybeans) is a valuable alternative source of vitamins, particularly vitamin B12. While Rhizopus oligosporus, the mold conventionally used in tempeh fermentation, does not synthesize vitamin B12 itself, this vitamin is typically produced by non-pathogenic bacteria, such as Klebsiella pneumoniae and Citrobacter freundii, present during the fermentation process. In addition, Rhizopus oligosporus increases other B vitamins, including folic acid, niacin, riboflavin, and pyridoxine, making tempeh a nutritious food choice for those seeking to boost their vitamin intake (Kårlund et al., 2020).
Ruminants, such as cows, sheep and goats can exploit microbiota in their rumen for B vitamins synthesis (Jiang et al., 2022). In contrast, in monogastric animals, gut microbial production largely occurs in areas where absorption is ineffective. Similarly, in fish and birds, vitamins of the B group must also be obtained from the diet.
Vitamin C (ascorbic acid) is an essential molecule for humans (as well as some primates, guinea pigs, and some birds) because they have lost the ability to synthesize it (Carr and Maggini, 2017). In addition, this molecule is thermolabile, meaning its source should not require drastic thermal treatments to preserve its efficacy.
The plant kingdom is the primary source of vitamin C, which accumulates mainly in fruits, leaves, tubers, and seeds. Mammals, birds (chicken and turkey), and some fish (salmon and tuna) can instead synthesize it in the liver, while mollusks and crustaceans obtain it from the diet. Vitamin C is generally not accumulated in large quantities in organisms, but is used according to metabolic needs, with excess amounts excreted via urine.
In humans, vitamin D is mainly produced by the skin through exposure to sunlight (UV-B) from the precursor of vitamin D3 (7-dehydrocholesterol). However, vitamin D can only be accumulated in small amounts in organisms, mainly in the liver and adipose tissue (Bouillon et al., 2007).
Algae, fungi, and plants can produce vitamin D, in the form D2 (ergocalciferol) or in the form of ergosterol (precursor of D2). However, vitamin D3 (cholecalciferol) appears to be the most bioavailable and active form for humans, primarily found in animal sources.
Mammals and birds such as cow, sheep, chicken, and turkey can synthesize vitamin D when exposed to sunlight; however, supplementation may be required under conditions of low sun exposure. Fish such as salmon and tuna accumulate vitamin D through diet and light exposure, while mollusks and crustaceans do not produce vitamin D independently.
The vitamin E complex (which includes tocopherols and tocotrienols) is a potent antioxidant but is not autonomously produced by mammals, birds, or marine organisms (Brigelius-Flohé and Traber, 1999). Humans obtain it through diet, mainly from plant sources (such as olives and olive oil, seeds and their oils), as vitamin E concentrates near triglycerides, exerting an antioxidant action. Alpha-tocopherol is the most common and active form in terms of human nutritional needs.
Vitamin K must also be obtained through diet. It is essential for blood clotting and can also be synthesized by certain gut bacteria (Shearer and Newman, 2008). Plants are the main sources of vitamin K1 (phylloquinone). Vitamin K can be stored in small quantities in organisms, mainly in the liver.
Lutein and zeaxanthin are two carotenoids essential for humans, involved in the biochemistry of vision. Major dietary sources include green leafy vegetables, yellow and orange fruits and vegetables, and some grains (Johnson, 2014), as well as certain algae and bacteria (Landrum and Bone, 2001).
Mammals, birds, fish, mollusks, and crustaceans do not produce carotenoids independently but instead accumulate them from their diet.
Essential fatty acids are critical for human health and must be obtained through the diet (Calder, 2015). In addition to vegetable oils, some algae can synthesize omega-3 fatty acids, particularly eicosapentaenoic acid (EPA) and docosahexaenoic acid (DHA), while algae thriving in freshwater (typically warmer) have very limited unsaturated fatty acid production (Santunione et al., 2024).
The ability to synthesize these fatty acids is limited in mammals, birds, and marine organisms. Fatty fish such as salmon, cod, sardines, and herring accumulate omega-3 fatty acids due to their seaweed diet. An interesting potential source of essential fatty acids could be insect larvae, as long as these fatty acids are present in their diet (Hadj Saadoun et al., 2020).
Essential amino acids (EAAs) and semi-essential amino acids must be introduced through the diet (Hou and Wu, 2018). Plants can synthesize all EAAs, although some reserve proteins (e.g., in cereals and legumes) are deficient in certain EAAs for human needs. However, combining cereals and legumes, together with a varied diet, can easily meet the EAA requirements.
Certain insects are protein-rich and capable of synthesizing all EAAs (Montevecchi et al., 2021; Miron et al., 2023). Spirulina (Arthrospira platensis) and chlorella (Chlorella sp pl.) are rich in proteins that provide a wide range of AAE (Montevecchi et al., 2022).
The human gut microbiota can produce small amounts of EAAs such as lysine and methionine. Some microorganisms used in food production, such as yeast and lactic acid bacteria, can produce proteins containing EAAs. For instance, brewer’s yeast (Saccharomyces cerevisiae) can synthesize EAAs during the fermentation process.
Animal products contain the full range of AAEs. However, the thermal processing they undergo often leads to the degradation of specific EAAs, such as lysine (in both free and bound forms).
Plant fiber is divided into soluble and insoluble (cellulose, lignin). The main fractions of soluble fibers consumed in the diet include pectin, which help reduce cholesterol and regulate blood sugar levels by slowing the absorption of sugars and preventing spikes in blood glucose. In addition, beta-glucans are known for lowering cholesterol levels and promoting cardiovascular health. Inulin and fructooligosaccharides (FOS) are prebiotics that stimulate the growth of beneficial bacteria in the colon, improving gut health and boosting the immune system. Mucilages enhance bowel movement by hydrating the stool (Slavin, 2005).
Other sources of fiber include algae and fungi, which are known to support the immune system (Vijayabaskar and Vaseela, 2012). In addition, gut bacteria metabolize fiber into short-chain fatty acids, contributing to overall wellbeing (Louis and Flint, 2017).
Polyphenols are bioactive compounds synthesized as secondary metabolites by plants that represent their main source in the human diet (Scalbert et al., 2005; Tagliazucchi et al., 2010). Seaweed and mushrooms (shiitake and reishi) also contain beneficial polyphenols (Fernando et al., 2016; Muszynska et al., 2018).
Among the phytonutrients, glucosinolates are particularly interesting. When the cells of the Brassicaceae are damaged (for example, during chewing or processing), the enzyme myrosinase converts glucosinolates into isothiocyanates, bioactive compounds with cancer-preventive properties, particularly in the colon, breast, lungs, and prostate. They also exhibit anti-inflammatory effects, modulating inflammatory responses and reducing chronic inflammation, which is linked to various chronic diseases. In addition, they aid in detoxification by stimulating phase II enzyme activity in the liver, enhancing the elimination of toxic and carcinogenic substances, and provide cardiovascular protection by reducing inflammation and LDL oxidation (Halkier and Gershenzon, 2006; Herr and Büchler, 2010).
The gut microbiota is an ecosystem of microorganisms that both collaborate and compete, contributing to the overall health and wellbeing of the human host. Their balance can be modulated through specific dietary components known as probiotics, prebiotics, and postbiotics. Probiotics (yogurt, kefir and fermented foods such as kimchi and miso) introduce live bacteria (ferments) that help maintain and improve the balance of the intestinal flora.
Prebiotics are non-digestible substances that promote growth, activity, and a healthy balance of the intestinal flora, without introducing them directly (Gibson and Roberfroid, 1995). Some examples are soluble fibers, such as inulin, which is found in artichokes, cauliflower, asparagus, and Jerusalem artichokes. Other prebiotic sources include bananas, apples, and whole grains, which provide soluble fiber or its fractions with prebiotic activity. Garlic and onions contain fructooligosaccharides (FOS), known for their prebiotic effects.
Postbiotics are metabolic products of probiotic microorganisms and include organic acids, enzymes and peptides, polysaccharides, vitamins, and other bioactive molecules that can modulate immune responses and reduce intestinal inflammation (Salminen et al., 2021). Although postbiotics hold great promise, research in this area is still in its early stages.
Fortification of staple foods can improve nutrition by addressing deficiencies in vitamins, minerals, and antioxidants (Allen et al., 2006). Incorporation of superfoods such as berries, seeds, vegetables, algae, and insects, or individual substances such as vitamins and minerals into common products such as cereals, bread, baked goods, dried fruits, legumes, oils and seasonings, yoghurts, dairy products, as well as specially prepared snacks and bars, can make essential nutrients more accessible and help prevent malnutrition (Calvo et al., 2004). Fortification is particularly beneficial in regions where specific nutrient deficiencies, such as iron and vitamin A, are prevalent, contributing to improved public health outcomes (Ofori et al., 2022). However, fortification must be approached with caution to avoid harmful excesses of certain nutrients. Indeed, while food fortification and consumption of superfoods aim to address nutrient deficiencies, excessive intake of certain nutrients can pose significant health risks.
Excessive intake of vitamin A and related carotenoids, often through fortified foods or supplements, can lead to hypervitaminosis A, a condition associated with symptoms such as nausea, headaches, irritability, and dizziness. More severe effects can include decreased bone mineral density, bone fractures, liver enlargement, and even birth defects. Superfoods like liver and some fortified dairy products rich in vitamin A should be consumed in moderation, particularly by vulnerable populations such as pregnant women and young children (Wrzochal et al., 2019; National Institute of Health, 2022; Orkusz and Dobrzyńska, 2022).
While essential for bone health and immune function, excessive consumption of vitamin D can cause hypercalcemia, leading to kidney damage, cardiovascular issues, and calcification of soft tissues. This is a growing concern as vitamin D is increasingly added to fortified foods and supplements (Vieth, 2006; Vieth, 2007).
Over-fortification of iron in food can result in iron overload, particularly in individuals with genetic conditions. This can lead to liver damage, diabetes, and heart disease. Foods like fortified cereals and red meat should be consumed in moderation to avoid such risks (Liu et al., 2023).
Excessive consumption of omega-3s from supplements or fish oil-enriched foods can lead to increased bleeding risk, immune suppression, and gastrointestinal discomfort. This highlights the importance of balancing omega-3 intake from superfoods like salmon, flaxseeds, and fortified products (Javaid et al., 2024).
Considering all these factors, education on portion sizes, labeling transparency, and dietary guidelines are essential to mitigate the risks of superfood overconsumption and harmful nutrient excesses. Additionally, policymakers and manufacturers should consider population-specific needs when designing fortification programs to avoid unintended health consequences.
Most nutrients and bioactive molecules can be supplied by both plant-based and animal-based products. However, it is crucial to consider that both options must face environmental and social challenges. On the one hand, large-scale plant production can lead to the overexploitation of agricultural areas, causing negative impacts on biodiversity and soil health, as well as precarious working conditions for workers employed across many supply chains. On the other hand, animal-based products still require enormous land use for feed production and are often associated with intensive farming practices that raise serious concerns regarding environmental sustainability and animal welfare.
Drawing from the extensive scientific literature on the topic (Nelson et al., 2016; Djekic et al., 2014), a shift to plant-based nutrient sources should be considered a more sustainable choice to address global environmental and health challenges. Nevertheless, dietary choices are shaped by a combination of individual, cultural, social, and environmental factors, with affordability remaining a critical choice parameter. Unhealthy dietary patterns are more common among lower socioeconomic groups compared to wealthier ones (Swinburn et al., 2019).
The production of eco-friendly food that is both affordable and nutrient-rich represents a challenge for current agricultural and global food systems (Viroli et al., 2023). Moreover, in many cultures, animal-based foods are deeply embedded in diets, as well as traditional rituals. In this context, food can be viewed as an essential component of cultural evolution, a process that has been significantly accelerated by globalization. Consequently, cultural preferences and culinary traditions, which remain representative of specific regions, are undergoing a major transition influenced by changes in economic, environmental, and social landscapes. In the long term, this transition is going to pave the way for new food habits that reflect the ongoing transformation of society and the environment, striking a balance between sustainability, health, and respect for natural and human resources.
To analyze in more detail the sustainability parameters of superfoods, three products of non-animal origin and three products of animal origin were selected.
Regarding the environmental impact, Spirulina is highly sustainable, even compared to many terrestrial crops, as it requires minimal land and water resources (Amin et al., 2024). It can be grown in controlled environments (open ponds and bioreactors) with water recycling, allowing for good yields with a lower carbon footprint. It requires mixtures of inorganic salts that could be conveniently recovered from co-products collected from other agri-food chains through ultra- and nano-filtration processes.
The cultivation of Spirulina can also contribute to local economies, particularly in regions where traditional agriculture is difficult due to climatic or soil conditions. However, it is essential to scale production sustainably and ensure fair labor practices (Henrikson, 2010).
Goji berries are mainly grown in Asia and require considerable water resources, especially in arid regions where they are often grown. There is also concern about monoculture practices, which can lead to biodiversity loss and soil degradation (Wang et al., 2015).
Global demand for goji berries has led to intensive agriculture, often in economically vulnerable areas (Potterat, 2010). While the market provides income for local farmers, it also raises questions about fair wages, working conditions, and exploitation of local resources.
Quinoa is resilient to harsh climates and has low water needs, making it sustainable for growth in diverse environments (Gómez-Caravaca et al., 2014). However, increased global demand has led to shifts in land use, impacting traditional farming practices and potentially contributing to soil erosion in Peru and Bolivia.
Quinoa production has benefited Andean farmers economically, but rising demand has also led to higher prices, sometimes restricting access for local populations who traditionally relied on it as a staple food (Bazile et al., 2016).
Wild salmon populations are at risk from overfishing. On the other hand, salmon farming can have considerable environmental impacts, including water pollution, habitat degradation, and high feed requirements, even though it remains important for boosting local economies, especially in coastal areas (Naylor et al., 2009). In addition, the issues concerning overfishing and environmental degradation are affecting marine ecosystems and local fishing communities (Torrissen et al., 2011).
Fish oil extraction supports economic activity in coastal regions, but sustainability concerns are growing as overfishing disrupts marine food chains, impacting local communities reliant on fish stocks. Overfishing of species like anchovies and sardines has a severe impact on marine resources (Tacon and Metian, 2009). Alternative sources, such as algae-based oils, are being developed to reduce the ecological footprint. Indeed, scientific evidence is collecting promising results about their application as sustainable omega-3 source (Shahidi and Ambigaipalan, 2018).
Beef liver is a nutrient-dense food. However, it comes from beef production, which represents the most impactful animal production in terms of greenhouse gas emissions, deforestation, land use, and water usage (Eshel et al., 2014). Sustainable practices have yet to become widespread in this sector. Such practices must necessarily also include the necessary reduction in the size of animal farming.
Beef liver is one of the least sustainable options due to the high environmental costs associated with beef production, making it a poorer choice compared to other alternatives available in the market (Godfray et al., 2018).
The classification proposed in this article provides a valuable framework for addressing sustainability and animal welfare concerns within the context of global superfood consumption. Organisms that synthesize nutrients, such as plants, algae, and microorganisms offer highly sustainable food options, as they require fewer resources compared to organisms that accumulate nutrients, like animals. Adopting a plant-based superfood diet can help reduce the environmental impact associated with animal production, which leads to high greenhouse gas emissions, water consumption, and land overexploitation. However, it is necessary to practice agriculture in a sustainable way, and with a low environmental impact, preferring crops that can potentially be cultivated at different latitudes and in large areas of the globe, with reduced water and pesticide requirements.
In addition, growing awareness of animal welfare is encouraging consumers to seek more ethical alternatives. The classification proposed in this perspective article emphasizes the potential to meet nutritional needs without relying on animals, favoring the choice of nutrient-rich plant-based foods. This transition to a plant-based superfood diet not only promotes human health but contributes to a fairer and more responsible food system.
The original contributions presented in the study are included in the article/supplementary material, further inquiries can be directed to the corresponding author.
GS: Investigation, Validation, Writing–review and editing. GM: Conceptualization, Supervision, Writing–original draft.
The author(s) declare that no financial support was received for the research, authorship, and/or publication of this article.
The authors declare that the research was conducted in the absence of any commercial or financial relationships that could be construed as a potential conflict of interest.
The author(s) declare that no Generative AI was used in the creation of this manuscript.
All claims expressed in this article are solely those of the authors and do not necessarily represent those of their affiliated organizations, or those of the publisher, the editors and the reviewers. Any product that may be evaluated in this article, or claim that may be made by its manufacturer, is not guaranteed or endorsed by the publisher.
Abdel-Aal, E. S. M., Akhtar, H., Zaheer, K., and Ali, R. (2013). Dietary sources of lutein and zeaxanthin carotenoids and their role in eye health. Nutrients 5 (4), 1169–1185. doi:10.3390/nu5041169
Allen, L., de Benoist, B., Dary, O., and Hurrell, R. (2006). Guidelines on food fortification with micronutrients. World Health Organization. Available at: https://iris.who.int/bitstream/handle/10665/43412/9241594012_eng.pdf.
Amin, M., ul Haq, A., Shahid, A., Boopathy, R., and Syafiuddin, A. (2024). “Spirulina as a food of the future,” in Pharmaceutical and nutraceutical potential of cyanobacteria. Editors M. A. Mehmood, P. Verma, M. P. Shah, and M. J. Betenbaugh (Cham, Switzerland: Springer International Publishing). doi:10.1007/978-3-031-45523-0_3
Anastassakis, K. (2022). “Vit B5 (pantothenic acid),” in Androgenetic alopecia from A to Z: vol. 2 drugs, herbs, nutrition and supplements (Cham, Switzerland: Springer International Publishing.), 309–313. doi:10.1007/978-3-031-08057-9_31
Aravind, S. M., Wichienchot, S., Tsao, R., Ramakrishnan, S., and Chakkaravarthi, S. (2021). Role of dietary polyphenols on gut microbiota, their metabolites and health benefits. Food Res. Int. 142, 110189. doi:10.1016/j.foodres.2021.110189
Ardiansyah, F. (2006). “Realising sustainable oil palm development in Indonesia–challenges and opportunities,” in International oil palm conference (Indonesia: Bali). Available at: https://awsassets.panda.org/downloads/wwfpaperrealisingsustpalmoil.pdf.
Averianova, L. A., Balabanova, L. A., Son, O. M., and Podvolotskaya, A. B. (2020). Production of vitamin B2 (riboflavin) by microorganisms: an overview. Front. Bioeng. Biotechnol. 8, 570828. doi:10.3389/fbioe.2020.570828
Bacher, A., Eberhardt, S., Eisenreich, W., Fischer, M., Herz, S., Illarionov, B., et al. (2001). Biosynthesis of riboflavin. Vitam. Horm. 61, 1–49. doi:10.1016/S0083-6729(01)61001-X
Bacher, A., Eberhardt, S., Fischer, M., Kis, K., and Richter, G. (2000). Biosynthesis of vitamin B2 (riboflavin). Annu. Rev. Nutr. 20, 153–167. doi:10.1146/annurev.nutr.20.1.153
Bailey, L. B., and Caudill, M. A. (2012). “Folate,” in Present knowledge in nutrition. Editors J. W. ErdmanJr, I. A. Macdonald, and S. H. Zeisel tenth edition (Washington, DC, USA: Wiley-Blackwell), 321–342. doi:10.1002/9781119946045.ch21
Bazile, D., Jacobsen, S. E., and Verniau, A. (2016). The global expansion of quinoa: trends and limits. Front. Plant Sci. 7, 622. doi:10.3389/fpls.2016.00622
Benedik, E. (2022). Sources of vitamin D for humans. Int. J. Vitam. Nutr. Res. 92 (2), 118–125. doi:10.1024/0300-9831/a000733
Bertrand, W., and de Buhr, E. (2015). Trade, development and child labor: regulation and law in the case of child labor in the cocoa industry. Law Dev. Rev. 8 (2), 503–521. doi:10.1515/ldr-2015-0019
Bischoff, K. L. (2021). “Glucosinolates,” in Nutraceuticals. Efficacy, safety and toxicity. Editors R. C. Gupta,, and A. Srivastava second edition (Cambridge, MA, USA: Elsevier), 903–909. doi:10.1016/B978-0-12-821038-3.00053-7
Blanco-Pérez, F., Steigerwald, H., Schülke, S., Vieths, S., Toda, M., and Scheurer, S. (2021). The dietary fiber pectin: health benefits and potential for the treatment of allergies by modulation of gut microbiota. Curr. Allergy Asthma Rep. 21 (43), 43–19. doi:10.1007/s11882-021-01020-z
Bongiorno, P. B., Fratellone, P. M., and LoGiudice, P. (2008). Potential health benefits of garlic (Allium sativum): a narrative review. J. Complement. Integr. Med. 5 (1). doi:10.2202/1553-3840.1084
Booth, S. L. (2012). Vitamin K: food composition and dietary intakes. Food Nutr. Res. 56 (1), 5505. doi:10.3402/fnr.v56i0.5505
Booth, S. L., and Suttie, J. W. (1998). Dietary intake and adequacy of vitamin K. J. Nutr. 128 (5), 785–788. doi:10.1093/jn/128.5.785
Bouillon, R., Norman, A. W., and Lips, P. (2007). Vitamin D deficiency. N. Engl. J. Med. 357 (19), 1980–1982. doi:10.1056/NEJMc072359
Brigelius-Flohé, R., and Traber, M. G. (1999). Vitamin E: function and metabolism. FASEB J. 13 (10), 1145–1155. doi:10.1096/fasebj.13.10.1145
Bryant, C. J. (2022). Plant-based animal product alternatives are healthier and more environmentally sustainable than animal products. Future Foods 6, 100174. doi:10.1016/j.fufo.2022.100174
Calder, P. C. (2015). Functional roles of fatty acids and their effects on human health. J. Parenter. Enter. Nutr. 39 (1S), 18S–32S. doi:10.1177/0148607115595980
Calvo, M. S., Whiting, S. J., and Barton, C. N. (2004). Vitamin D fortification in the United States and Canada: current status and data needs. Am. J. Clin. Nutr. 80 (6), 1710S–6S. doi:10.1093/ajcn/80.6.1710S
Carder, G., Proctor, H., Schmidt-Burbach, J., and D’Cruze, N. (2016). The animal welfare implications of civet coffee tourism in Bali. Anim. Welf. 25 (2), 199–205. doi:10.7120/09627286.25.2.199
Cardwell, G., Bornman, J. F., James, A. P., and Black, L. J. (2018). A review of mushrooms as a potential source of dietary vitamin D. Nutrients 10 (10), 1498. doi:10.3390/nu10101498
Carr, A. C., and Maggini, S. (2017). Vitamin C and immune function. Nutrients 9 (11), 1211. doi:10.3390/nu9111211
Cazzonelli, C. I. (2011). Carotenoids in nature: insights from plants and beyond. Funct. Plant Biol. 38 (11), 833–847. doi:10.1071/FP11192
Costa-Perez, A., Nunez-Gomez, V., Baenas, N., Di Pede, G., Achour, M., Manach, C., et al. (2023). Systematic review on the metabolic interest of glucosinolates and their bioactive derivatives for human health. Nutrients 15 (6), 1424. doi:10.3390/nu15061424
da Silva, V. R., Russell, K. A., and Gregory, J. F. (2012). “Vitamin B6,” in Present knowledge in nutrition. Editors J. W. ErdmanJr, I. A. Macdonald, and S. H. Zeisel tenth edition (Washington, DC, USA: Wiley-Blackwell), 307–320. doi:10.1002/9781119946045.ch20
da Silveira Bueno, R., Marchetti, L., Cocozza, C., Marchetti, M., and Salbitano, F. (2021). Could cattle ranching and soybean cultivation be sustainable? A systematic review and a meta-analysis for the Amazon. IFOREST 14, 285–298. doi:10.3832/ifor3779-014
Di Pasquale, M. G. (2009). The essentials of essential fatty acids. J. Diet. Suppl. 6 (2), 143–161. doi:10.1080/19390210902861841
Djekic, I., Miocinovic, J., Tomasevic, I., Smigic, N., and Tomic, N. (2014). Environmental life-cycle assessment of various dairy products. J. Clean. Prod. 68, 64–72. doi:10.1016/j.jclepro.2013.12.054
EFSA Panel on Food Additives and Nutrient Sources added to Food, Younes, M., Aggett, P., Aguilar, F., Crebelli, R., Dusemund, B., Wright, M., et al. (2018). Scientific opinion on the safety of monacolins in red yeast rice. EFSA J. 16 (8), e05368. doi:10.2903/j.efsa.2018.5368
Eisenhauer, B., Natoli, S., Liew, G., and Flood, V. M. (2017). Lutein and zeaxanthin-Food sources, bioavailability and dietary variety in age-related macular degeneration protection. Nutrients 9 (2), 120. doi:10.3390/nu9020120
Eshel, G., Shepon, A., Makov, T., and Milo, R. (2014). Land, irrigation water, greenhouse gas, and reactive nitrogen burdens of meat, eggs, and dairy production in the United States. Proc. Natl. Acad. Sci. U. S. A. 111 (33), 11996–12001. doi:10.1073/pnas.1402183111
Estévez-Santiago, R., Beltrán-de-Miguel, B., and Olmedilla-Alonso, B. (2016). Assessment of dietary lutein, zeaxanthin and lycopene intakes and sources in the Spanish survey of dietary intake (2009–2010). Int. J. Food Sci. Nutr. 67 (3), 305–313. doi:10.3109/09637486.2016.1147020
Fernandez, M. L. (2001). “Pectin. Composition, chemistry, physicochemical properties, food applications, and physiological effects,” in Handbook of dietary fiber. Editor S. S. Cho (Boca Raton, FL, USA: CRC Press), 583–601. doi:10.1201/9780203904220-34
Fernando, I. S., Nah, J. W., and Jeon, Y. J. (2016). Potential anti-inflammatory natural products from marine algae. Environ. Toxicol. Pharmacol. 48, 22–30. doi:10.1016/j.etap.2016.09.023
Flamm, G., Glinsmann, W., Kritchevsky, D., Prosky, L., and Roberfroid, M. (2001). Inulin and oligofructose as dietary fiber: a review of the evidence. Crit. Rev. Food Sci. Nutr. 41 (5), 353–362. doi:10.1080/20014091091841
Gebauer, S. K., Psota, T. L., Harris, W. S., and Kris-Etherton, P. M. (2006). n-3 fatty acid dietary recommendations and food sources to achieve essentiality and cardiovascular benefits. Am. J. Clin. Nutr. 83 (6), 1526S–35S. doi:10.1093/ajcn/83.6.1526S
Gibson, G. R., and Roberfroid, M. B. (1995). Dietary modulation of the human colonic microbiota: introducing the concept of prebiotics. J. Nutr. 125 (6), 1401–1412. doi:10.1093/jn/125.6.1401
Globenewswire.com (2025). Global superfood market size. Available at: https://www.globenewswire.com/news-release/2024/07/25/2919158/0/en/Global-Superfood-Market-Size-To-Worth-USD-183-Billion-By-2033-l-CAGR-Of-4-66.html (Accessed January 13, 2025).
Godfray, H. C. J., Aveyard, P., Garnett, T., Hall, J. W., Key, T. J., Lorimer, J., et al. (2018). Meat consumption, health, and the environment. Science 361, eaam5324. doi:10.1126/science.aam5324
Gómez-Caravaca, A. M., Iafelice, G., Verardo, V., Marconi, E., and Caboni, M. F. (2014). Phenolic compounds and saponins in quinoa samples (Chenopodium quinoa Willd.) grown under different saline and non-saline irrigation regimens. J. Agric. Food Chem. 62 (9), 1994–2003. doi:10.1021/jf3002125
Grandviewresearch.com (2025). Superfoods market growth and trends. Available at: https://www.grandviewresearch.com/press-release/global-superfoods-market (Accessed January 13, 2025).
Granger, M., and Eck, P. (2018). Dietary vitamin C in human health. Adv. Food Nutr. Res. 83, 281–310. doi:10.1016/bs.afnr.2017.11.006
Hadj Saadoun, J., Montevecchi, G., Zanasi, L., Bortolini, S., Macavei, L. I., Masino, F., et al. (2020). Lipid profile and growth of black soldier flies (Hermetia illucens, Stratiomyidae) reared on by-products from different food chains. J. Sci. Food Agric. 100 (9), 3648–3657. doi:10.1002/jsfa.10397
Halkier, B. A., and Gershenzon, J. (2006). Biology and biochemistry of glucosinolates. Annu. Rev. Plant Biol. 57, 303–333. doi:10.1146/annurev.arplant.57.032905.105228
Henrikson, R. S. (2010). Earth Food. How this micro algae can transform your health and our planet. Richmond, CA: Ronore Enterprises, Inc. Available at: https://smartmicrofarms.com/PDF.cfm/SpirulinaWorldFood2021.pdf.
Herr, I., and Büchler, M. W. (2010). Dietary constituents of broccoli and other cruciferous vegetables: implications for prevention and therapy of cancer. Cancer Treat. Rev. 36 (5), 377–383. doi:10.1016/j.ctrv.2010.01.002
Hou, Y., and Wu, G. (2018). Nutritionally essential amino acids. Adv. Nutr. 9 (6), 849–851. doi:10.1093/advances/nmy054
Hughes, L. J., Black, L. J., Sherriff, J. L., Dunlop, E., Strobel, N., Lucas, R. M., et al. (2018). Vitamin D content of Australian native food plants and Australian-grown edible seaweed. Nutrients 10 (7), 876. doi:10.3390/nu10070876
Javaid, M., Kadhim, K., Bawamia, B., Cartlidge, T., Farag, M., and Alkhali, M. (2024). Bleeding Risk in patients receiving Omega-3 polyunsaturated fatty acids: a systematic review and meta-analysis of randomized clinical trials. J. Am. Heart Assoc. 13 (10), e032390. doi:10.1161/JAHA.123.032390
Jelliffe, D. B. (1966). “The assessment of the nutritional status of the community (with special reference to field surveys in developing regions of the world),” in WHO monograph series No. 53 (Geneva: World Health Organization), 271. Available at: http://www.ernaehrungsdenkwerkstatt.de/fileadmin/user_upload/EDWText/TextElemente/PHN-Texte/WHO_FAO_Report/Jelliffe_Assessment_Buch_WHO_MONO_53_assessment_part1.pdf.
Jiang, Q., Lin, L., Xie, F., Jin, W., Zhu, W., Wang, M., et al. (2022). Metagenomic insights into the microbe-mediated B and K2 vitamin biosynthesis in the gastrointestinal microbiome of ruminants. Microbiome 10 (1), 109. doi:10.1186/s40168-022-01298-9
Johnson, E. J. (2014). Role of lutein and zeaxanthin in visual and cognitive function throughout the lifespan. Nutr. Rev. 72 (9), 605–612. doi:10.1111/nure.12133
Jungert, A., Spinneker, A., Nagel, A., and Neuhäuser-Berthold, M. (2014). Dietary intake and main food sources of vitamin D as a function of age, sex, vitamin D status, body composition, and income in an elderly German cohort. Food Nutr. Res. 58 (1), 23632. doi:10.3402/fnr.v58.23632
Kalyani Nair, K., Kharb, S., and Thompkinson, D. K. (2010). Inulin dietary fiber with functional and health attributes—a review. Food Rev. Int. 26 (2), 189–203. doi:10.1080/87559121003590664
Kårlund, A., Gómez-Gallego, C., Korhonen, J., Palo-Oja, O. M., El-Nezami, H., and Kolehmainen, M. (2020). Harnessing microbes for sustainable development: food fermentation as a tool for improving the nutritional quality of alternative protein sources. Nutrients 12 (4), 1020. doi:10.3390/nu12041020
Khan, U. M., Sevindik, M., Zarrabi, A., Nami, M., Ozdemir, B., Kaplan, D. N., et al. (2021). Lycopene: food sources, biological activities, and human health benefits. Oxid. Med. Cell Longev. 2021 (1), 2713511. doi:10.1155/2021/2713511
Knudsen, E. E., MacDonald, D. D., and Steward, C. R. (2020). “Setting the stage for a sustainable Pacific salmon fisheries strategy,” in Sustainable fisheries management. Editors E. E. Knudsen,, and D. D. MacDonald (Boca Raton, FL, USA: CRC Press), 3–13. doi:10.1201/9780429104411
Lam, K. L., and Cheung, P. C. K. (2013). Non-digestible long chain beta-glucans as novel prebiotics. Bioact. Carbohydr. Diet. Fibre 2 (1), 45–64. doi:10.1016/j.bcdf.2013.09.001
Landrum, J. T., and Bone, R. A. (2001). Lutein, zeaxanthin, and the macular pigment. Arch. Biochem. Biophys. 385 (1), 28–40. doi:10.1006/abbi.2000.2171
LeBlanc, J. G., Milani, C., De Giori, G. S., Sesma, F., Van Sinderen, D., and Ventura, M. (2013). Bacteria as vitamin suppliers to their host: a gut microbiota perspective. Curr. Opin. Biotechnol. 24 (2), 160–168. doi:10.1016/j.copbio.2012.08.005
Lipsky, J. J. (1994). Nutritional sources of vitamin K. Mayo Clin. Proc. 69 (5), 462–466. doi:10.1016/S0025-6196(12)61643-7
Liu, Y., Li, G., Lu, F., Guo, Z., Cai, S., and Huo, T. (2023). Excess iron intake induced liver injury: the role of gut-liver axis and therapeutic potential. Biomed. Pharmacother. 168, 115728. doi:10.1016/j.biopha.2023.115728
Louis, P., and Flint, H. J. (2017). Formation of propionate and butyrate by the human colonic microbiota. Environ. Microbiol. 19 (1), 29–41. doi:10.1111/1462-2920.13589
Lustig, R. H. (2013). Fructose: it’s “alcohol without the buzz”. Adv. Nutr. 4 (2), 226–235. doi:10.3945/an.112.002998
Manach, C., Scalbert, A., Morand, C., Rémésy, C., and Jiménez, L. (2004). Polyphenols: food sources and bioavailability. Am. J. Clin. Nutr. 79 (5), 727–747. doi:10.1093/ajcn/79.5.727
Marketresearch.com (2025). Global superfoods market - 2023-2030. Available at: https://www.marketresearch.com/DataM-Intelligence-4Market-Research-LLP-v4207/Global-uperfoods-34671824 (Accessed January 13, 2025).
Market.us (2025). Rep. Overv. Available at: https://market.us/report/superfoods-market (Accessed January 13, 2025).
Marques de Brito, B., Campos, V. D. M., Neves, F. J., Ramos, L. R., and Tomita, L. Y. (2023). Vitamin B12 sources in non-animal foods: a systematic review. Crit. Rev. Food Sci. Nutr. 63 (26), 7853–7867. doi:10.1080/10408398.2022.2053057
Meléndez Granados, J. S. (2020). Carotenoids in bacteria: general scheme on the diversity of the biosynthetic pathways. Repos. Inst. Séneca. Available at: http://hdl.handle.net/1992/49031.
Miron, L., Montevecchi, G., Bruggeman, G., Macavei, L. I., Maistrello, L., Antonelli, A., et al. (2023). Functional properties and essential amino acid composition of proteins extracted from black soldier fly larvae reared on canteen leftovers. Innov. Food Sci. Emerg. Technol. 87, 103407. doi:10.1016/j.ifset.2023.103407
Mohd, S. N. F. N., and Makpol, S. (2019). Ginger (Zingiber officinale Roscoe) in the prevention of ageing and degenerative diseases: review of current evidence. Evid. Based Complement. Altern. Med. 2019 (1), 5054395. doi:10.1155/2019/5054395
Montevecchi, G., Licciardello, F., Masino, F., Miron, L. T., and Antonelli, A. (2021). Fortification of wheat flour with black soldier fly prepupae. Evaluation of technological and nutritional parameters of the intermediate doughs and final baked products. Innov. Food Sci. Emerg. Technol. 69, 102666. doi:10.1016/j.ifset.2021.102666
Montevecchi, G., Masino, F., Chinnici, F., and Antonelli, A. (2010). Occurrence and evolution of amino acids during grape must cooking. Food Chem. 121 (1), 69–77. doi:10.1016/j.foodchem.2009.12.005
Montevecchi, G., Santunione, G., Licciardello, F., Köker, Ö., Masino, F., and Antonelli, A. (2022). Enrichment of wheat flour with Spirulina. Evaluation of thermal damage to essential amino acids during bread preparation. Food Res. Int. 157, 111357. doi:10.1016/j.foodres.2022.111357
Mordorintelligence.com (2025). Superfood market size and share analysis - growth trends and forecasts (2025 - 2030). Available at: https://www.mordorintelligence.com/industry-reports/superfoods-market (Accessed January 13, 2025).
Mukherjee, I., and Sovacool, B. K. (2014). Palm oil-based biofuels and sustainability in southeast Asia: a review of Indonesia, Malaysia, and Thailand. Renew. Sustain Energy Rev. 37, 1–12. doi:10.1016/j.rser.2014.05.001
Murphy, S. P., Subar, A. F., and Block, G. (1990). Vitamin E intakes and sources in the United States. Am. J. Clin. Nutr. 52 (2), 361–367. doi:10.1093/ajcn/52.2.361
Muszynska, B., Grzywacz-Kisielewska, A., Kala, K., and Gdula-Argasinska, J. (2018). Anti-inflammatory properties of edible mushrooms: a review. Food Chem. 243, 373–381. doi:10.1016/j.foodchem.2017.09.149
National Institute of Health (2022). Vitamin a and carotenoids. Fact Sheet Consumers, 1–3. Available at: https://ods.od.nih.gov/pdf/factsheets/VitaminA-Consumer.pdf.
Naylor, R. L., Hardy, R. W., Bureau, D. P., Chiu, A., Elliott, M., Farrell, A. P., et al. (2009). Feeding aquaculture in an era of finite resources. Proc. Natl. Acad. Sci. U. S. A. 106 (36), 15103–15110. doi:10.1073/pnas.0905235106
Nelson, M. E., Hamm, M. W., Hu, F. B., Abrams, S. A., and Griffin, T. S. (2016). Alignment of healthy dietary patterns and environmental sustainability: a systematic review. Adv. Nutr. 7 (6), 1005–1025. doi:10.3945/an.116.012567
Ofori, K. F., Antoniello, S., English, M. M., and Aryee, A. N. (2022). Improving nutrition through biofortification–A systematic review. Front. Nutr. 9, 1043655. doi:10.3389/fnut.2022.1043655
Orkusz, A., and Dobrzyńska, D. (2022). Can you trust a box diet? Eng. Sci. Technol. 1 (38), 124–133. doi:10.15611/nit.2022.38.08
Penberthy, W. T., and Kirkland, J. B. (2020). “Niacin,” in Present knowledge in nutrition. Editors B. P. Marriott, D. F. Birt, V. A. Stallings, and A. A. Yates eleventh edition (Cambridge, MA, USA: Elsevier Inc.), 209–224. doi:10.1002/9781119946045.ch19
Poore, J., and Nemecek, T. (2018). Reducing food’s environmental impacts through producers and consumers. Science 360 (6392), 987–992. doi:10.1126/science.aaq0216
Potterat, O. (2010). Goji (Lycium barbarum and Lycium chinense): phytochemistry, pharmacology and safety in the perspective of traditional uses and recent popularity. Planta Med. 76 (1), 7–19. doi:10.1055/s-0029-1186218
Putnam, E. E., and Goodman, A. L. (2020). B vitamin acquisition by gut commensal bacteria. PLoS Pathog. 16 (1), e1008208. doi:10.1371/journal.ppat.1008208
Rafeeq, H., Ahmad, S., Tareen, M. B. K., Shahzad, K. A., Bashir, A., Jabeen, R., et al. (2020). Biochemistry of fat soluble vitamins, sources, biochemical functions and toxicity. Haya Saudi J. Life Sci. 5 (6), 188–196. doi:10.36348/sjls.2020.v05i09.007
Rasouli, H., Farzaei, M. H., and Khodarahmi, R. (2017). Polyphenols and their benefits: a review. Int. J. Food Prop. 20 (Suppl. 2), 1–42. doi:10.1080/10942912.2017.1354017
Reeds, P. J. (2000). Dispensable and indispensable amino acids for humans. J. Nutr. 130 (7), 1835S–40S. doi:10.1093/jn/130.7.1835S
Revuelta, J. L., Ledesma-Amaro, R., and Jiménez, A. (2016). ““Industrial production of vitamin B2 by microbial fermentation” (chapter 2),” in Industrial biotechnology of vitamins, biopigments, and antioxidants. Editors E. J. Vandamme,, and J. L. Revuelta (Weinheim, Germany: WileyVCH Verlag GmbH and Co), 15–40. doi:10.1002/9783527681754.ch2
Said, H. M. (2015). Water-soluble vitamins. Nutr. Prim. Care Provid. 111, 30–37. doi:10.1159/000362294
Salminen, S., Collado, M. C., Endo, A., Hill, C., Lebeer, S., Quigley, E. M., et al. (2021). Reply to: postbiotics - when simplification fails to clarify. Nat. Rev. Gastroenterol. Hepatol. 18 (11), 827–828. doi:10.1038/s41575-021-00522-5
Santunione, G., Masino, F., Montevecchi, G., and Sgarbi, E. (2024). UV-B light (radiation) affects the metabolism of pigments and fatty acids in green algae Edaphochlorella mirabilis and Klebsormidium flaccidum in vitro. Algal Res. 83, 103736. doi:10.1016/j.algal.2024.103736
Scalbert, A., Johnson, I. T., and Saltmarsh, M. (2005). Polyphenols: antioxidants and beyond. Am. J. Clin. Nutr. 81 (1), 215S–7S. doi:10.1093/ajcn/81.1.215S
Shahidi, F., and Ambigaipalan, P. (2018). Omega-3 polyunsaturated fatty acids and their health benefits. Annu. Rev. Food Sci. Technol. 9 (1), 345–381. doi:10.1146/annurev-food-111317-095850
Shahidi, F., Pinaffi-Langley, A. C. C., Fuentes, J., Speisky, H., and de Camargo, A. C. (2021). Vitamin E as an essential micronutrient for human health: common, novel, and unexplored dietary sources. Free Radic. Biol. Med. 176, 312–321. doi:10.1016/j.freeradbiomed.2021.09.025
Shearer, M. J., and Newman, P. (2008). Metabolism and cell biology of vitamin K. Thromb. Haemost. 100 (04), 530–547. doi:10.1160/TH08-03-0147
Singh, J. (2021). “Vitamin B9 in dark green vegetables: deficiency disorders, bio-availability, and fortification issues,” in B-complex vitamins-sources, intakes and novel applications. Editor J. G. LeBlanc (Rijeka, Croazia: IntechOpen). doi:10.5772/intechopen.100318
Singh, P., and Goyal, G. K. (2008). Dietary lycopene: its properties and anticarcinogenic effects. Compr. Rev. Food Sci. Food Saf. 7 (3), 255–270. doi:10.1111/j.1541-4337.2008.00044.x
Skippon, W. (2013). The animal health and welfare consequences of foie gras production. Can. Vet. J. 54 (4), 403–404.
Slavin, J. L. (2005). Dietary fiber and body weight. Nutrition 21 (3), 411–418. doi:10.1016/j.nut.2004.08.018
Stabler, S. P. (2020). “Vitamin B12,” in Present knowledge in nutrition. Editors B. P. Marriott, D. F. Birt, V. A. Stallings, and A. A. Yates eleventh edition (Cambridge, MA, USA: Elsevier Inc.), 257–271. doi:10.1016/B978-0-323-66162-1.00015-9
Swinburn, B. A., Kraak, V. I., Allender, S., Atkins, V. J., Baker, P. I., Bogard, J. R., et al. (2019). The global syndemic of obesity, undernutrition, and climate change: the Lancet Commission report. Lancet 393 (10173), 791–846. doi:10.1016/S0140-6736(18)32822-8
Tacon, A. G. J., and Metian, M. (2009). Fishing for feed or fishing for food: increasing global competition for small pelagic forage fish. Ambio 38 (6), 294–302. doi:10.1579/08-a-574.1
Tagliazucchi, D., Verzelloni, E., Bertolini, D., and Conte, A. (2010). In vitro bio-accessibility and antioxidant activity of grape polyphenols. Food Chem. 120 (2), 599–606. doi:10.1016/j.foodchem.2009.10.030
Torrissen, O., Olsen, R. E., Toresen, R., Hemre, G. I., Tacon, A. G., Asche, F., et al. (2011). Atlantic salmon (Salmo salar): the “super-chicken” of the sea? Rev. Fish. Sci. 19 (3), 257–278. doi:10.1080/10641262.2011.597890
Vasile Simone, G., Montevecchi, G., Masino, F., Matrella, V., Imazio, S. A., Antonelli, A., et al. (2013). Ampelographic and chemical characterization of Reggio Emilia and Modena (northern Italy) grapes for two traditional seasonings: “saba” and “agresto”. J. Sci. Food Agric. 93 (14), 3502–3511. doi:10.1002/jsfa.6296
Vieth, R. (2006). What is the optimal vitamin D status for health? Prog. Biophys. Mol. Biol. 92 (1), 26–32. doi:10.1016/j.pbiomolbio.2006.02.003
Vieth, R. (2007). Vitamin D toxicity, policy, and science. J. Bone Min. Res. 22 (S2), V64–V68. doi:10.1359/jbmr.07s221
Vijayabaskar, P., and Vaseela, N. (2012). In vitro antioxidant properties of sulfated polysaccharide from brown marine algae Sargassum tenerrimum. Asian Pac J. Trop. Dis. 2, S890–S896. doi:10.1016/S2222-1808(12)60287-4
Viroli, G., Kalmpourtzidou, A., and Cena, H. (2023). Exploring benefits and barriers of plant-based diets: health, environmental impact, food accessibility and acceptability. Nutrients 15 (22), 4723. doi:10.3390/nu15224723
Vlassopoulou, M., Yannakoulia, M., Pletsa, V., Zervakis, G. I., and Kyriacou, A. (2021). Effects of fungal beta-glucans on health–a systematic review of randomized controlled trials. Food Funct. 12 (8), 3366–3380. doi:10.1039/D1FO00122A
Wang, X., Qi, Y., and Zheng, H. (2022). Dietary polyphenol, gut microbiota, and health benefits. Antioxidants 11 (6), 1212. doi:10.3390/antiox11061212
Wang, X., Zhang, B., and Zhao, Q. (2015). Ecological risk assessment of Lycium barbarum cultivation in China. Environ. Sci. Pollut. Res. 22 (23), 18524–18532. doi:10.3390/ijerph192316186
Watanabe, F., and Bito, T. (2018). Vitamin B12 sources and microbial interaction. Exp. Biol. Med. 243 (2), 148–158. doi:10.1177/1535370217746612
Watanabe, F., Yabuta, Y., Bito, T., and Teng, F. (2014). Vitamin B₁₂-containing plant food sources for vegetarians. Nutrients 6 (5), 1861–1873. doi:10.3390/nu6051861
Watanabe, F., Yabuta, Y., Tanioka, Y., and Bito, T. (2013). Biologically active vitamin B12 compounds in foods for preventing deficiency among vegetarians and elderly subjects. J. Agric. Food Chem. 61 (28), 6769–6775. doi:10.1021/jf401545z
Wertz, P. W. (2009). Essential fatty acids and dietary stress. Toxicol. Ind. Health 25 (4-5), 279–283. doi:10.1177/0748233709103035
Wolak, N., Zawrotniak, M., Gogol, M., Kozik, A., and Rapala-Kozik, M. (2017). Vitamins B1, B2, B3 and B9–occurrence, biosynthesis pathways and functions in human nutrition. Mini Rev. Med. Chem. 17 (12), 1075–1111. doi:10.2174/1389557516666160725095729
Wrzochal, A., Gładyś-Jakubczyk, A., and Suliga, E. (2019). Evaluation of diet in preschool-age children with Down syndrome – preliminary examination. Med. Stud. 35, 128–138. doi:10.5114/ms.2019.86332
Keywords: nutritional sustainability, functional foods, environmental impact, plant-based diets, agroecological practices
Citation: Santunione G and Montevecchi G (2025) Superfoods: exploring sustainability perspectives between nutrient synthesizers and accumulators. Front. Food. Sci. Technol. 5:1507933. doi: 10.3389/frfst.2025.1507933
Received: 08 October 2024; Accepted: 16 January 2025;
Published: 07 February 2025.
Edited by:
Wahyudi David, Bakrie University, IndonesiaReviewed by:
Javier Carballo, University of Vigo, SpainCopyright © 2025 Santunione and Montevecchi. This is an open-access article distributed under the terms of the Creative Commons Attribution License (CC BY). The use, distribution or reproduction in other forums is permitted, provided the original author(s) and the copyright owner(s) are credited and that the original publication in this journal is cited, in accordance with accepted academic practice. No use, distribution or reproduction is permitted which does not comply with these terms.
*Correspondence: G. Montevecchi, Z2l1c2VwcGUubW9udGV2ZWNjaGlAdW5pbW9yZS5pdA==
Disclaimer: All claims expressed in this article are solely those of the authors and do not necessarily represent those of their affiliated organizations, or those of the publisher, the editors and the reviewers. Any product that may be evaluated in this article or claim that may be made by its manufacturer is not guaranteed or endorsed by the publisher.
Research integrity at Frontiers
Learn more about the work of our research integrity team to safeguard the quality of each article we publish.