- 1Department of Food Science and Technology, Federal University of Santa Catarina, Florianopolis, Santa Catarina, Brazil
- 2Department of Chemical and Food Engineering, Federal University of Santa Catarina, Florianopolis, Santa Catarina, Brazil
- 3Ministério da Agricultura e Pecuária, Laboratório Federal de Defesa Agropecuária, Setor Laboratorial Avançado de São José (SLAV/SC/LFDA/RS), São José, Santa Catarina, Brazil
Over the years, there has been an increase in demand for plant-based foods as alternatives. In line with this, this work explores the production and in vitro digestion of a fermented plant-based beverage (FPBB) produced with pea and rice proteins and 0% (FPBB-C), 5% (FPBB-5), and 10% (FPBB-10) uvaia pulp through lactic fermentation with Lacticaseibacillus rhamnosus GG. The in vitro gastrointestinal digestion process was conducted to assess the bioaccessibility of L. rhamnosus GG, total phenolic content (TPC), and antioxidant activity before and after simulating the gastrointestinal conditions. After 48 h of digestion, highly viable L. rhamnosus GG cells remained throughout the gastrointestinal system. FPBB-C (106.89%) and FPBB-5 (109.38%) exhibited higher survival rates than FPBB-10 (102.20%), indicating that these beverages have a higher prebiotic action potential. Compared with the non-digested samples, after 48 h of digestion, all samples exhibited a significant increase in TPC. The same behavior occurs for the antioxidant activity of FPBB-C, FPBB-5, and FPBB-10 by DPPH (4.06, 3.96, and 8.44 mg TEAC mL−1), ABTS (10.28, 11.06, 11.97 mg TEAC mL−1), and FRAP method (917.02, 863.87, and 1983.23 mg TEAC mL−1). Thirteen compounds were identified and quantified in uvaia pulp by HPLC-DAD-ESI-MS, particularly epigallocatechin gallate, quercetin-3-rhamnose, and quercetin-3-glucoside. Isorhamnetin was the main phenolic compound detected in the colon, assumably due to the conversion of quercetin-3-glucoside by the probiotic cells. In conclusion, as all counts were above 9 log CFU g−1, the FPBB formulations containing pea, rice protein, and uvaia pulp become a promising vehicle for carrying L. rhamnosus GG.
1 Introduction
Globally, a growing interest in healthy lifestyles drives the market for functional foods (Camelo-Silva et al., 2024; Galanakis, 2021; Sorita et al., 2022). In this context, plant-based beverages such as cereals, nuts, and legumes fermented with probiotics have been explored to produce non-dairy beverages, emerging as a significant segment within functional foods (Popova et al., 2023). Due to their health-promoting properties, the growth preference for plant-based diets is not limited to vegetarians, lactose intolerants, or milk protein allergies but also to other consumers, including flexitarians (individuals centered on a plant-based diet with occasional consumption of animal products) (Sheen et al., 2023). This trend has driven the non-dairy alternatives market to US$29.18 billion in 2023, with a forecasted annual growth of 12.6% by 2030. This growth conducts research and innovation in the sector to meet the growing consumer demand (Grand View Research, 2024).
The most consumed vegetable proteins and their derived products are from wheat and soy. However, both have allergenic potential, increasing the search for other vegetable matrices with reduced hypoallergenic characteristics (Reese et al., 2023). Cereals such as sorghum, corn, and legumes, like beans and lentils, have been widely employed as alternatives for creating probiotic carriers due to their low allergenicity and reasonable amino acid composition (Popova et al., 2023; Sheen et al., 2023; Reese et al., 2023; Zhang et al., 2023). Those protein-rich foods serve as rich sources of macro- and micronutrients, promoting the growth and enhancing the viability of probiotic microorganisms. Pea and rice proteins are also excellent substitutes for allergenic proteins and maintain their availability and adequate nutritional values. Combining pea and rice protein ensures the provision of adequate amounts of all essential amino acids in the human diet (Lu et al., 2020). These proteins are the current trend in the plant-based food products market, urging researchers to conduct frequent investigations (Lu et al., 2020; Manus et al., 2021).
Also, adding fruit pulp to fermented plant-based beverages (FPBB) can be an interesting alternative to enhance the sensory and nutritional properties. Fruit pulps are commonly added to beverages; however, native Brazilian fruits is still little investigated (Bianchini et al., 2020). Brazil has several biomes, including the Atlantic Forest, which produces nutritious unconventional fruits, such as uvaia (Eugenia pyriformis), with little explored economic potential (Sganzerla et al., 2021). The uvaia belongs to the Myrtaceae family, presenting a rounded, flattened, and pyriform shape with a soft or firm texture and coloring between yellow and orange tones; its aroma is pleasant with sweet and acidic notes (Bianchini et al., 2020; Spricigo et al., 2023; Rodrigues et al., 2021). Regarding nutritional aspects, uvaia has been recognized for its high nutritional value and bioactive compounds (including gallic, chlorogenic, p-coumaric, and ferulic acids, among others) (Farias et al., 2021). It is a fruit contains a high vitamin C and A content (Farias et al., 2021), as well as amino acids, alanine, arginine, asparagine, choline, glutamine, isoleucine, leucine, phenylalanine, proline, serine, threonine, tyrosine, and valine (Spricigo et al., 2023). Due to this wide range of beneficial compounds, an in vivo study proved that the ingestion of uvaia juice by female rats demonstrated a decrease in oxidative damage, improving antioxidant efficiency and its beneficial potential for health (Lopes et al., 2018). Another in vivo study also demonstrated the anti-inflammatory and antioxidant activities of uvaia pulp juice in male Wistar rats (Ramirez et al., 2012). Considering those high health benefits, uvaia pulp seems to be an interesting and innovative food product to enrich the sensory and functional properties of pea and rice FPBB.
Fermentation is a primary step in producing FPBB. During the lactic acid bacteria (LAB) fermentation process, pea and rice proteins enriched with uvaia pulp can produce diverse secondary metabolites that can exert prebiotic actions, positively altering other nutritional and bioactive profiles of beverages through enzymatic and microbial activities (Masiá et al., 2021; Cuvas-Limón et al., 2022). Certain LABs can produce exopolysaccharides, which improve the stability of beverages, enabling the replacement of thickeners. Furthermore, when using probiotic microorganisms, it is possible to create a functional beverage through acidification and to generate antimicrobial compounds that compete with undesirable microorganisms (Alcorta et al., 2021). L. rhamnosus GG has been employed to prepare diverse beverages, such as soy, oat, and coconut (Masiá et al., 2021), almonds and inulin (Muncey and Hekmat, 2021), wheat bread loaves (Sigüenza-Andrés et al., 2023), and others. A recent study focused on developing and accessing in vitro protein digestibility and physicochemical properties of a functional beverage enriched with rice-pea proteins (Manus et al., 2021). The authors observed a decrease in viscosity and a reduction in high molecular weight peptides associated with an increase in low molecular weight peptides, leading to a notable improvement in digestibility. However, no study was found in the literature data regarding producing rice and pea protein-based fermented beverages enriched with uvaia pulp.
Nevertheless, for bioactive compounds and the probiotic culture in FPBB to exert their biological activity and provide health benefits, they must be released from the food matrix and remain stable under gastrointestinal conditions (Camelo-Silva et al., 2024; Tang et al., 2023). Hence, assessing the bioactivity of fortified food products after undergoing gastrointestinal digestion is crucial. Accordingly, the objective of the present study was to formulate a fermented plant-based beverage using pea and rice proteins through a lactic fermentation process with L. rhamnosus GG enriched with uvaia pulp and to analyze its probiotic and prebiotic effects. For this, the survival of L. rhamnosus GG, total phenolic compounds, antioxidant activity, and chemical composition (by HPLC-ESI-MS/MS) were evaluated before and during the in vitro gastrointestinal digestion.
2 Material and methods
2.1 Materials
For the fermented drink preparation, isolated rice protein (80 g proteins 100 g−1, VeganWay, Florianópolis, SC, Brazil), isolated pea protein (80 g proteins 100 g−1, VeganWay, Florianópolis, SC, Brazil), freeze-dried probiotic culture composed by L. rhamnosus LGG® (Lr) (Chr. Hansen, Hønsholm, Denmark) and uvaia pulp (Eugenia pyriformis) (89.4% moisture, 1.0% protein, 0.25% fat, 9, 05% carbohydrates and 0.30% ash) were used. Man, Rogosa, Sharpe broth (MRS), and MRS agar (Kasvi, São José dos Pinhais, PR, Brazil) were employed for microbiological analyses. For the in vitro gastrointestinal simulation, the reagents used were α-amylase enzymes, pepsin from porcine gastric mucosa, porcine pancreatin, and bovine bile salts, all acquired from Sigma-Aldrich (São Paulo, SP, Brazil). BacTiter-Glo™ Viability Kit (Promega, Madison, WI, United States) was used for the viability of probiotic bacteria. Folin-Ciocalteu reagent was used in the total phenolic content (TPC) assay. For the antioxidant activities by the capture of DPPH and ABTS radicals, DPPH (2,2-diphenyl-1-picrylhydrazyl) and ABTS (2,2-AZINO-BIS(3-ethylbenzene-thiazoline-6-sulfonic acid) diammonium salt) radicals were used, respectively. TPTZ (2,4,6-Tris (2-pyridyl)-s-triazine) and ferric chloride were utilized for the antioxidant capacity by iron reduction FRAP assay. Gallic acid was used as the standard reference for TPC, while Trolox (6- Hydroxy-2,5,7,8-tetramethylchroman-2-carboxylic acid) was used as the standard reference for all antioxidant activity assays. All TPC and antioxidant activity reagents were purchased from Sigma-Aldrich (São Paulo, SP, Brazil). All chemicals used were of analytical grade.
2.2 Methods
2.2.1 Fermented plant-based beverage (FPBB) preparation
The probiotic FPBB was prepared following the methodology described by Deziderio et al. (2023), with modifications. First, 1.83% rice protein, 1.83% pea protein, 10% sucrose, 0.32% soy oil, and 0.2% diluted soy lecithin was diluted in distilled water and homogenized at 13.000 rpm (Ultra-Turrax, IKA™ T25 digital) for 3 min. Then, the freeze-dried probiotic culture composed of 109 Colony Forming Units (CFU) per gram of L. rhamnosus LGG® (Chr. Hansen, Hønsholm, Denmark) was inoculated, starting the fermentation stage. The fermentation was carried out at 37°C ± 2°C until it reached a pH of 4.8. After that, the fermentation stage was stopped by cooling the medium to 4°C ± 1°C (in a refrigerator, Consul, Brazil). Uvaia pulp was added in the formulations after the fermentation process. Three fermented beverage formulations were prepared: i) without addition of uvaia pulp - control sample (FPBB-C), and with the addition of ii) 5% (FPBB-5), and iii) 10% (FPBB-10) of pasteurized uvaia pulp (80°C for 3 min), respectively. The samples were kept refrigerated (4°C ± 1°C) until analysis. The samples were prepared in triplicate.
2.2.2 In vitro gastrointestinal simulation
The in vitro gastrointestinal simulation was performed according to the INFOGEST protocol (Verruck et al., 2020; Brodkorb et al., 2019), with modifications. The protocol simulates digestion in the mouth, stomach, small intestine, and colon sequentially, as presented in Figure 1.
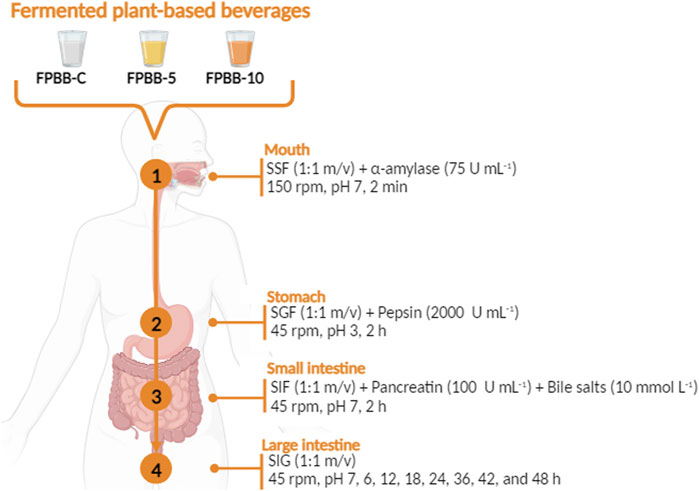
Figure 1. Flowchart of in vitro gastrointestinal digestion protocol employed to evaluate the bioaccessibility of L. rhamnosus GG, TPC, and antioxidant activity of the fermented plant-based beverages (FPBB-C, FPBB-5, and FPBB-10). FPBB-C: Fermented beverage without uvaia pulp; FPBB-5: Fermented beverage enriched with 5% uvaia pulp; FPBB-10: Fermented beverage enriched with 10% uvaia pulp).
The simulated fluid compositions (SSF - simulated salivary fluid, SGF - simulated gastric fluid, SIF - simulated intestinal fluid, and SFL - simulated large intestine fluid) employed in this protocol are described in Table 1.
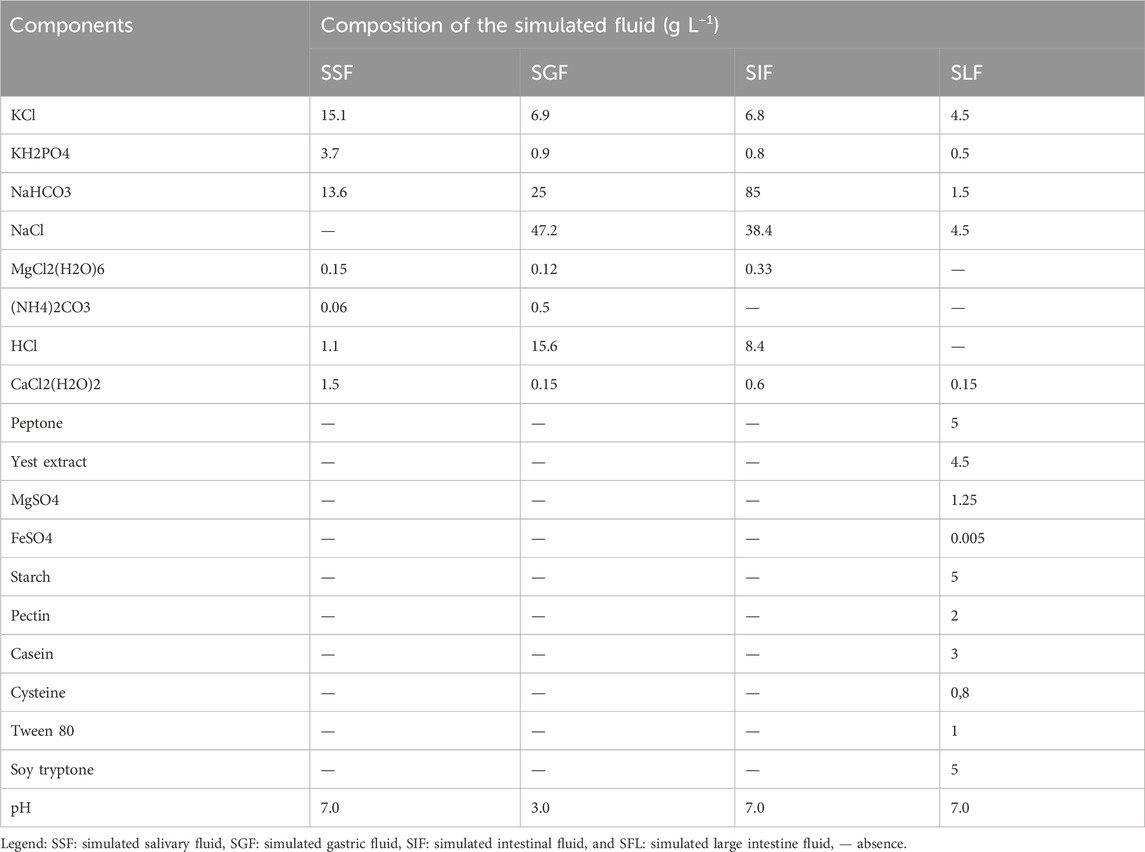
Table 1. Composition of simulated fluids (SSF, SGF, SIF, and SFL) employed during the in vitro gastrointestinal simulation.
For the first gastrointestinal step (mouth simulation), 5 g of each sample (FPBB-C, FPBB-5, and FPBB-10) were initially homogenized with SSF at the proportion of 1:1 (m/v). Then, human salivary α-amylase solution (75 U mL−1) was added, and the mixture was incubated in a water bath at 37°C at 150 rpm for 2 min.
After that, to simulate the stomach phase, the oral bolus was mixed with SGF 1:1 (v/v) (composition presented in Table 1) and porcine pepsin (2000 U mL−1), and the pH was adjusted with HCl (1 mol L−1) until pH 3.0 (gastric bolus). Then, the mixture was incubated at 37°C with gently stirring (100 rpm) for 2 h.
Subsequently, the small intestine digestion was simulated. For this, the gastric bolus was mixed with SIF 1:1 (v/v) (with the composition described in Table 1), pancreatin (100 U mL−1, based on trypsin activity), and bile salts (10 m mol L−1), previously heated in a water bath at 37°C. Then, the pH was adjusted to 7.0 by adding NaOH (1 mol L−1). The solution was incubated at 37°C under a stirring rate of 100 rpm for 2 h.
After passing through the small intestine, the fermented plant-based beverage reached the large intestine stage. For this step, the experiment was adapted to simulate the activity of the intestinal microbiota according to the protocol described by Verruck et al. (2020). The large intestine simulation was performed by adding SLF (Table 1) to the small intestine bolus at a ratio of 1:1 (v/v). Then, the pH was adjusted to 7.0 with NaOH solution (1 mol L−1) throughout the large intestine condition assay. During this step, the temperature was maintained at 37°C ± 1°C, and the starting rate was maintained at 45 rpm to simulate the intensity of peristaltic movements. The samples were collected after 6, 12, 18, 24, 36, 42 and 48 h.
Before and during the simulation, the enzyme solutions were kept cool and added gradually at each stage of digestion. After each gastrointestinal step, the remaining phenolic content was extracted using an 80% acetone solution with 0.01% HCl before conducting the TPC and AA assays. Quantification of L. rhamnosus GG, TPC, antioxidant activity (by DPPH, ABTS, and FRAP methods), and chemical characterization by LC-ESI-MS/MS were performed before the gastrointestinal simulation, during the oral, gastric, small intestine phase, and during the large intestine phase, as depicted in Figure 1. Each step of the gastrointestinal tract was simulated in triplicate.
2.2.3 Viability of Lacticaseibacillus rhamnosus GG cells during in vitro gastrointestinal simulation
The number of viable L. rhamnosus GG cells at the end of each stage of the gastrointestinal simulation was evaluated using the bioluminescence method, following the protocol described by Camelo-Silva et al. (Camelo-Silva et al., 2024). Briefly, 100 µL of each sample resulting from the gastrointestinal simulation stage were mixed with 50 µL of the BacTiter-Glo™ reagent and subjected to luminescence measurement in a microplate reader (GloMax®Explorer System model GM3500, Promega, United States). Each gastrointestinal stage’s viable L. rhamnosus GG cell counts were obtained by correlating the sample absorbances with a calibration standard curve (R2 = 0.9925) constructed by correlating luminescence values and plate counts. Plate counts were obtained using the pour plate method, which consists of performing serial dilutions (saline solution, 0.85 g 100 mL−1) of the pure culture of L. rhamnosus GG, followed by incubation in Man, Rogosa, and Sharp (MRS) agar at 37°C ± 1°C for 48 h in a bacteriological oven. Results were expressed as a log of forming units of colonies per gram (log UFC g−1). The analysis was carried out in triplicate, including the propagation of probiotic bacteria used to construct the curve.
2.2.4 Survival rate of Lacticaseibacillus rhamnosus GG
After simulated gastrointestinal conditions, the survival rate (%) of L. rhamnosus GG was evaluated according to Equation 1 (Verruck et al., 2015).
Where N1 represents the total viable cell count of L. rhamnosus GG after each stage of the gastrointestinal simulation, and N0 represents the viable cell count before exposure to gastrointestinal simulation.
2.2.5 Total phenolic content (TPC)
TPC of the digested and non-digested FPBB were evaluated according to the Folin-Ciocalteu colorimetric method described by Singleton and Rossi (Singleton and Rossi, 1965). First, 100 μL of each sample was mixed with 0.5 mL of Folin-Ciocalteu, 7 mL of distilled water, and 1.5 mL of 20% w/v sodium carbonate solution. Then, the solutions were kept in the dark for 2 h at room temperature (24°C). After that, the absorbances of the samples were read at 765 nm (UV-Vis mini-1240 Tokyo, Japan) using distilled water as a blank. Gallic acid was used as the reference standard, and the results were expressed in mg gallic acid equivalent (GAE) per mL (mg GAE mL−1) from triplicate measurements.
2.2.6 Released fraction of phenolic compounds
The released fraction (%) of phenolic content from each stage of the gastrointestinal simulation (mouth, stomach, small intestine, and large intestine) was determined by applying Equation 2, as described by Arend et al. (Arend et al., 2022).
Where: Nx represents the TPC quantified after each stage of simulated gastrointestinal conditions (mg g−1), and Ni represents the initial TPC quantified before the simulation.
2.2.7 Antioxidant activity (AA) by DPPH, ABTS, and FRAP
The DPPH and ABTS radical scavenging assays were conducted as described by Rufino et al. (Rufino et al., 2007). The DPPH assay was performed by reacting 0.1 mL of each diluted sample with 3.9 mL of the DPPH radical (2,2-difenil-1-picrilhidrazil, 0.06 mM DPPH solution). The samples were homogenized and kept in the dark for 30 min. Then, the sample absorbances were measured in a spectrophotometer (UV-Vis mini-1240 Tokyo, Japan) at 515 nm. Methyl alcohol was used as a blank. For ABTS (Rufino et al.), 30 µL of each diluted sample with 3 mL of the ABTS radical (2,2-azino-bis(3-ethylbenzo-thiazoline-6-sulfonic acid) diammonium salt). The samples were homogenized and kept in the dark at room temperature (24°C) for 6 min. After that, absorbances were measured (UV-Vis mini-1240 Tokyo, Japan) at 734 nm. Ethyl alcohol was used as a blank. Finally, in the FRAP (Ferric ion Reducing Antioxidant Power) method (Rufino et al.), an aliquot of 90 µL of each diluted sample was homogenized with 2.7 mL of FRAP reagent (25 mL of 0.3 M acetate buffer, 2.5 mL of TPTZ solution (2,4,6-Tris (2-pyridyl)-s-triazine) at 10 mM and 2.5 mL of 20 mM aqueous ferric chloride solution). The solution was kept in the dark for 30 min, and then the absorbances were measured (UV-Vis mini-1240 Tokyo, Japan) at 595 nm. FRAP reactive was used as a blank.
For all antioxidant methods, Trolox was used as a standard reference (DPPH - R2 = 0.9861, ABTS - R2 = 0.9944, and FRAP - R2 = 0.9962), and all the results were expressed in μM Trolox Equivalent (TE) mL−1 of sample from triplicates measurements.
2.2.8 Identification and quantification of phenolic compounds by LC-ESI-MS/MS
Phenolic compounds were analyzed by liquid chromatography – electrospray ionization – tandem mass spectrometry (LC-ESI-MS/MS) to identify and quantify individual compounds. The LC-ESI-MS/MS system employed an Agilent 1290 series liquid chromatograph coupled with a hybrid quadrupole linear ion trap mass spectrometer QTRAP® 5500 equipped with an electrospray ionization source (ESI). Phenolic compound identification and quantification were realized by comparing the sample’s peak area with commercial analytical standards using retention time, precursor ion, major fragment (quantification ion), and secondary fragment (confirmation ion). Multiple reaction monitoring (MRM) transitions mode, calibration curves, and detection and quantification limits for the 33 standards of phenolic compounds tested can be found in Supplementary Table S1 (Supplementary Material).
The column used for chromatographic separation was a Zorbax Eclipse Plus C18 (3.0 mm × 100 mm, 3.5 µm) from Agilent. The mobile phase was composed of water (A) and methanol (B), both with 0.1% of formic acid. A gradient mode was employed, where the following proportions of B were used: 2% for 0–3 min, 20% for 3–10 min, 90% for 10–11 min, and 2% for 11–13 min. The flow rate was seated at 0.3 mL min−1, and a 2 min equilibration time was used between runs. The volume injection was 5 µL. Data acquisition and analysis were performed using the software Analyst and MultiQuant, respectively, from Sciex.
2.2.9 Statistical analysis
The results from this work were expressed as mean ± standard deviation from triplicate measurements. One-way analysis of variance (ANOVA) and Tukey’s test were used to determine significant differences (p < 0.05) between the results. All data analysis was performed using STATISTICA software version 13.3 (TIBCO Software Inc., Palo Alto, CA).
3 Results and discussion
3.1 Lacticaseibacillus rhamnosus GG viability during the gastrointestinal simulation
The survival of probiotic microorganisms during their exposure to severe gastrointestinal environments plays a critical role in fulfilling their functional properties (Castro-López et al., 2023). Given the sensitivity of probiotics to the human gastrointestinal tract, monitoring the probiotic cells sublethally throughout the gastrointestinal tract is essential for comprehending the biological performance of new functional foods, such as FPBB.
The viable cell counts and the survival rate of L. rhamnosus GG cells from FPBB formulations (FPBB-C, FPBB-5, and FPBB-10) after the exposure to oral, gastric, and intestinal (ileum and colon) simulations are shown in Supplementary Table S2 (Supplementary Material) and Figure 2, respectively.
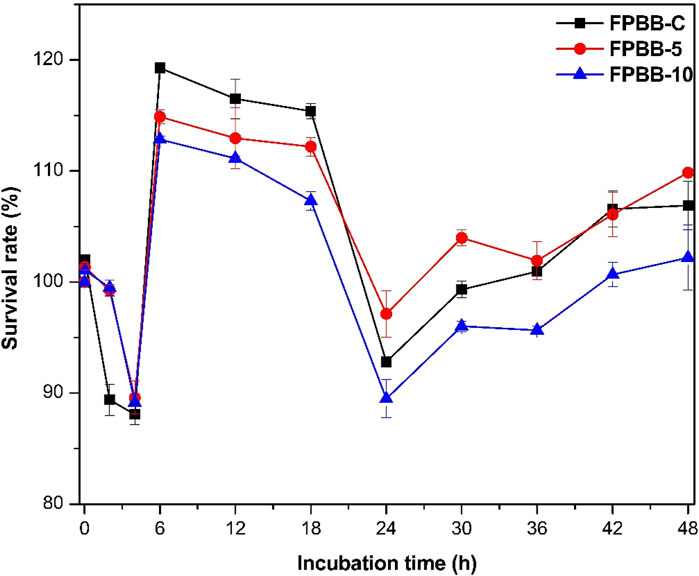
Figure 2. Survival rate of L. rhamnosus GG after exposure to simulated gastrointestinal fluids. (FPBB-C: Fermented beverage without uvaia pulp; FPBB-5: Fermented beverage enriched with 5% uvaia pulp; FPBB-10: Fermented beverage enriched with 10% uvaia pulp). Incubation time refers to the stages of the gastrointestinal system. Lines are used for better visualization.
According to Prestes et al. (2021)1, probiotic products should have at least 6 log CFU g−1 of viable cells to confer health benefits. Accordingly, all FPBB samples (Supplementary Table S2) exhibited initial viable cell counts higher than 9.30 log UFC g−1, surpassing the recommended threshold for potential health benefits and consequently classifying the three fermented beverages as probiotic products.
After exposure to mouth conditions, no statistical differences (p < 0.05) were observed in viable L. rhamnosus GG cell count for all beverages (FPBB-C: 9.49, FPBB-5: 9.57, and FPBB-10: 9.55 UFC g−1) compared to the initial (non-digested) samples. During the oral phase, the liquid and low-viscosity nature of the vegetable beverage results in brief contact with the simulated salivary fluid (SSF), which does not adversely affect the integrity of L. rhamnosus GG cells.
In the stomach simulation, the control beverage (FPBB-C) showed a statistical decrease (8.19 log UFC g−1) in L. rhamnosus GG cells count, while the count of the formulations enriched with uvaia pulp (stomach: FPBB- 5: 9.37 and FPBB-10: 9.40 log UFC g−1) remained equal (p < 0.05). In this gastrointestinal simulation stage, the beverages presented survival rates ranging from 89.35% to 99.47% (for FPBB-C and FPBB-10, respectively, Figure 2). It is important to highlight that food matrices may protect probiotic microorganisms through the gastrointestinal passage (Tang et al., 2023). The protective mechanisms provided by uvaia pulp in FPBB-5 and FPBB-10 formulations for L. rhamnosus GG during the gastric phase are varied and synergistic. Firstly, the high survival rates of the FPBB formulations during gastric passage (SGF) can be partly attributed to the formation of insoluble complexes between proteins (from peas and rice) and polysaccharides (such as pectin from uvaia pulp) (Spricigo et al., 2023). These complexes form a gel-like matrix around the probiotic cells, creating a protective barrier that shields L. rhamnosus GG from pepsin and gastric fluids. This barrier helps maintain the high viability of the probiotic culture. Polysaccharides also contribute to the controlled release of probiotics into the intestine by delaying exposure to harsh gastric conditions (Mohamadzadeh et al., 2024). Phenolic compounds, including flavonoids and phenolic acids (Table 2; Section 3.4), and non-phenolic compounds like vitamins C and A (identified in uvaia pulp (Silva et al., 2022)), play a crucial role as potent antioxidants. They neutralize free radicals and reduce oxidative stress caused by the stomach’s acidic environment and digestive processes (Mohamadzadeh et al., 2024; Wu C. et al., 2023; Ma et al., 2022). These compounds also help interrupt lipid peroxidation, further protecting the probiotic cells. Combining these bioactive components in uvaia pulp works synergistically to enhance the protection of L. rhamnosus GG, ensuring greater survival and efficacy of the probiotics.
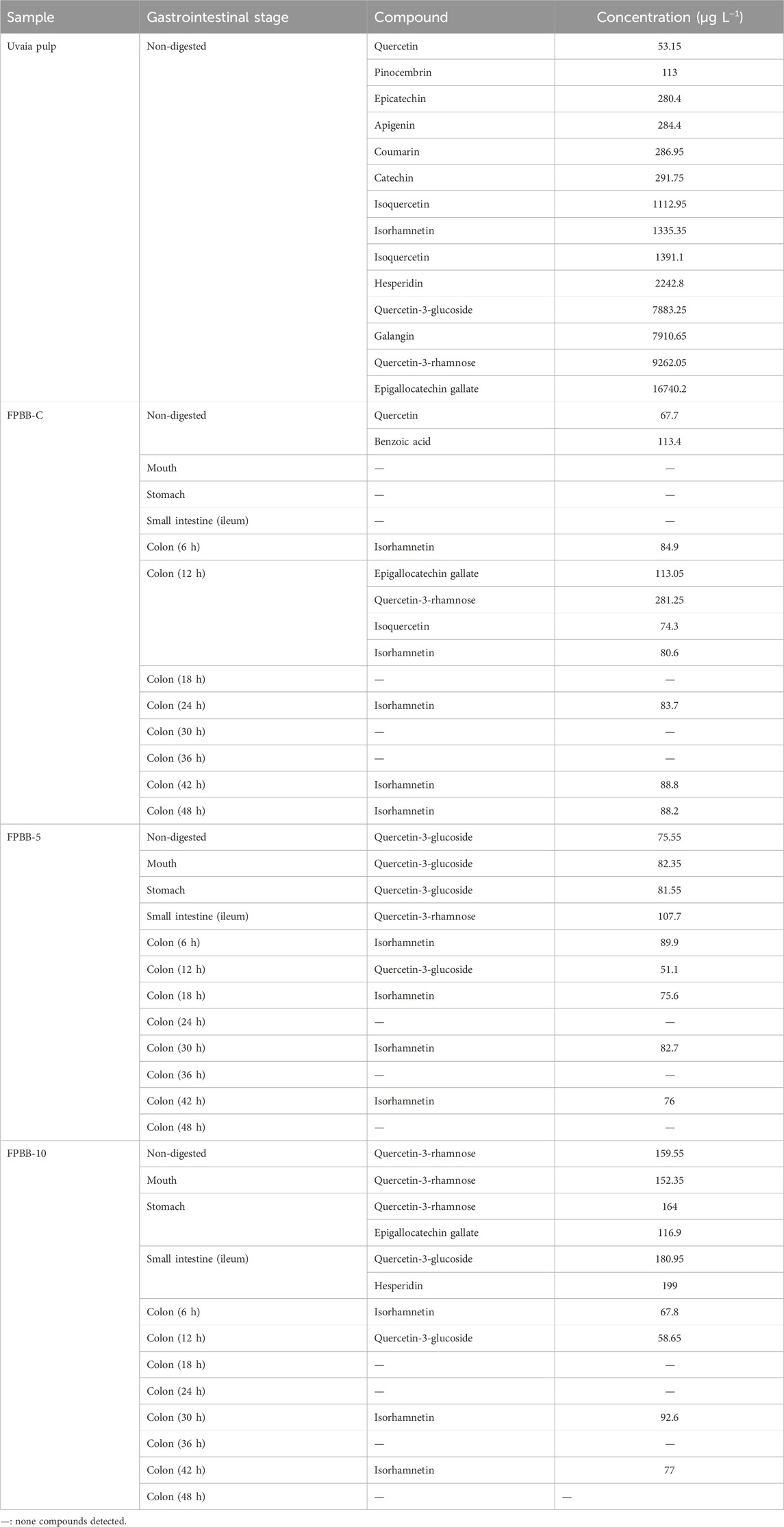
Table 2. Phenolic profile of in natura uvaia pulp and the effect of simulated in vitro digestion on phenolic compounds of FPBB formulations.
In addition, those complexes can control the release of probiotics in the gastrointestinal tract, ensuring their survival and delivery to the target site. Furthermore, these complexes can enhance oxidative stability, control the release of probiotics in the gastrointestinal tract, and contribute to the sensory properties of the beverage. The key interactions between proteins and polysaccharides, such as hydrogen bonding, hydrophobic interactions, and ionic bonds, are crucial in forming these complexes and their protective properties (Mohamadzadeh et al., 2024). In terms of stability during storage, these complexes can positively impact the shelf-life of the beverages by preventing the degradation of both the probiotics and the bioactive components (Gentile, 2020). The gel-like structure helps maintain the integrity of the formulations, reducing the likelihood of separation or sedimentation (Kupikowska-Stobba et al., 2024). In terms of sensory properties, the formation of the complex can contribute to the desired texture of the beverage, enhancing its sensory appeal. Also, the complex can help mask undesirable flavors, improving the overall taste of the FPBB (Gentile, 2020; Kupikowska-Stobba et al., 2024).
In the small intestine simulation, a more pronounced decrease (p < 0.05) was noted (FPBB-C: 8.19, FPBB-5: 8.49, and FPBB-10: 8.42 UFC g−1) in comparison to the previous stages. The survival rates observed in this stage were 88.06, 89.94, and 89.10% for FPBB-C, FPBB-5, and FPBB-10, respectively. This behavior can be attributed to the influence of bile salts within the simulated intestinal fluid (SIF). Bile salts, recognized for their detergent-like characteristics, have been reported to interact with microbial membranes, cause DNA damage, and trigger bacterial death (Chai et al., 2023), reducing the recovery rate in this gastrointestinal stage.
Finally, in the large intestine simulation, the favorable conditions, characterized by low acidity and the presence of previously unabsorbed substrates (Verruck et al., 2020), facilitated the proliferation of L. rhamnosus GG (until 18 h), as can be confirmed by the high L. rhamnosus GG count (FPBB-C: 10.73, FPBB-5: 10.59, and FPBB-10: 10.50). However, after 18 h of incubation, FPBB-10 exhibited a significantly lower recovery rate (p < 0.05) than FPBB-C and FPBB-5, which may be associated with microbial adaptation to less readily available substrates, such as high molecular weight proteins and oligosaccharides (McCoubrey et al., 2023). Also, it is widely recognized that microorganisms forming the gut microbiota and phenolic compounds present a mutual effect. Dietary polyphenols can modulate the composition of intestinal microbiota (as a prebiotic); in turn, gut microbiota catabolizes polyphenols to release more active and better-absorbed bioactive metabolites (Wang et al., 2022). Nevertheless, some studies have suggested that excessive amounts of phenolic compounds reaching the colon could inhibit the growth of beneficial intestinal microorganisms. Thus, the high concentration of uvaia pulp in FPBB-10 probably contributed to the release of considerable amounts of phenolic compounds during this stage, inhibiting the growth of L. rhamnosus GG after 18 h of the gastrointestinal simulation. Liu and Firrman (2016) reported similar results, showing that quercetin (phenolic compound also quantified in this study in the colon samples, quercetin-3-rhamnose, Section 3.4) and naringenin exhibited a dose-dependent inhibition of L. rhamnosus GG growth at 8 h post-inoculation. The same phytochemicals also demonstrated dose-dependent inhibitory effects on the growth of other probiotic cells, including Lactobacillus sp. and Bifidobacterium catenulatum (Duda-Chodak, 2012).
In conclusion, after in vitro digestibility, the bacterial counts consistently remained elevated (>9 log CFU g−1), indicating the promising viability of the FPBB matrix as a potential alternative carrier for L. rhamnosus GG. However, it is noteworthy that in this study, FPBB-10 formulation cannot be considered the optimal option for carrying L. rhamnosus GG, due to the reduced survival rate in the large intestine (colon), compared with FPBB-C and FPBB-5 formulations.
3.2 Effect of in vitro simulated gastrointestinal digestion on total phenolic content
It is well-known that gastrointestinal digestion may alter the phenolic composition of food products. In this line, the effect of in vitro simulated gastrointestinal digestion on phenolic compounds was determined by measuring TPC values before and after each gastrointestinal stage. The TPC results and the released phenolic fractions (%) are shown in Supplementary Table S3 (Supplementary Material) and Figure 3, respectively.
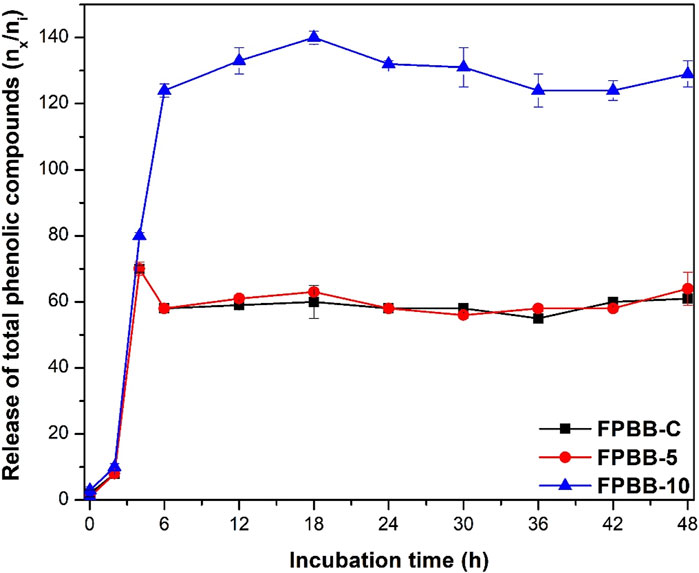
Figure 3. Release of total phenolic compounds after exposure to simulated gastrointestinal conditions. (FPBB-C: Fermented beverage without uvaia pulp; FPBB-5: Fermented beverage enriched with 5% uvaia pulp; FPBB-10: Fermented beverage enriched with 10% uvaia pulp). Incubation time refers to the stages of the gastrointestinal system. Lines are used for better visualization.
All samples exhibited a significant increase at p < 0.05 (FPBB-C: 61%, FPBB-5: 64%, and FPBB-10: 129%) after 48 h of digestion, compared with the non-digested samples. As depicted in Figure 3, both FPBB-C and FPBB-5 formulations exhibited a similar pattern of behavior despite the statistical difference in the final TPC values. First, at the beginning of the gastrointestinal assay (after mouth), no substantial changes were found in the TPC results. A notable increase in TPC values was observed after the ileum stage, 0.70 mg GAE mL-1 (Supplementary Table S3), which reflected an increase of 70% (for both formulations) compared to the amount determined in the non-digested samples. This behavior was observed for fermented beverages with Aloe vera Juice (Cuvas-Limón et al., 2022) and Dragon fruit-kiwi (Tang et al., 2023) and may be justified by the presence of an acidic environment in the stomach and intestinal stages, which could affect the FPBB structural matrix through hydrolysis of polyphenolic-proteins (linked through hydrogen or amide bonds) or polyphenolic-carbohydrate complexes (linked through hydrophobic bonds) (Tang et al., 2023). Similarly, the increase in TPC values can also be explained by the action of digestive enzymes (α-amylase, pepsin, and pancreatin) on the linkage between phenolic and the uvaia pulp cell wall, particularly through ester bonds, thereby releasing phenolic acids (Wu C. et al., 2023). The release of intact or hydrolyzed phenolic compounds makes them more prone to react with the Follin-Ciocalteu reagent, increasing the TPC values.
Following the in vitro digestion, a slight decrease was observed until the colon at 36 h (55% and 58% for FPBB-C and FPBB-5, respectively) and increasing again until reaching the maximum TPC in the colon at 48 h. For the FPBB-10 formulation, the highest TPC value was detected in the colon at 18 h (1.40 mg GAE mL−1), with a slight reduction until the colon at 48 h (1.29 mg GAE mL−1), maintaining the total phenolic content significantly higher (at all gastrointestinal stages) when compared to FPBB-C and FPBB-5. As the colon exhibits a notable increase in L. rhamnosus GG multiplication, as indicated by the viable cell count after 24 h (Supplementary Table S3), the colonic microflora probably facilitates the structural transformation and metabolism of phenolic compounds (Zhang et al., 2023; Cattivelli et al., 2023), explaining the progressive increase throughout in vitro digestibility, Figure 3, which in fact, is a positive effect, since the phenolic metabolites generated by the action of gut microbiota are generally considered to be better absorbed and responsible for the beneficial effects of FPBB, as previously reported (Section 3.1).
Unlike this work results, Farias et al. (2021) detected a significant decrease (41%) in TPC values and an increase in the total flavonoid content (109%) after the intestinal phase of uvaia pulp. The same authors suggested that phenolic acids (identified and quantified in uvaia pulp by HPLC) are more susceptible to digestive degradation, probably due to less structural complexity than flavonoids (mainly found in glycosylated form - linked with carbohydrate molecules). It is important to highlight that FPBB is a complex food matrix. The interaction among the chemical compounds presents in the beverage components (rice and pea proteins, sucrose, soy oil, and soy lecithin) and the phenolics from uvaia pulp may protect the phenolic compounds (like an encapsulation agents), contributing to a slower release and consequently contributing to the high TPC results after digestion compared with the studies by Farias et al. (2021), which evaluated the in nature fruit.
In summary, our findings reinforce that FPBB is an excellent food matrix for delivering polyphenols from uvaia pulp at a concentration of 5% since the phenolic compounds increased during in vitro gastrointestinal digestion without compromising the viability of the L. rhamnosus GG cells, configuring FPBB as a probiotic and prebiotic product.
3.3 Antioxidant activity (AA) changes during the gastrointestinal digestion simulation
Natural antioxidants play a crucial role in human health. Those compounds can inhibit or delay undesired oxidation reactions and thus prevent oxidative stress related to a wide range of human body disorders, like high blood pressure, neurodegenerative disorders, or cancer (Rumpf et al., 2023). Assessing their activity during in vitro gastrointestinal digestion is an important approach to screening their bioavailability and effectiveness in supplying antioxidant benefits to the body. This assessment is also crucial in refining new food formulations to maximize their effectiveness after ingestion. Examining more than one AA method enhances the qualification and quantification of a product’s antioxidant activity (AA). This approach is valuable because the antioxidant capacity is influenced by the polarity of the numerous compounds in the food matrix and their diverse interactions and synergistic effects with the specific antioxidant radical (Rumpf et al., 2023).
The effect of simulated gastrointestinal digestion on the antioxidant capacity of FPBB formulations, assessed through DPPH, ABTS, and FRAP methods, is outlined in Figure 4.
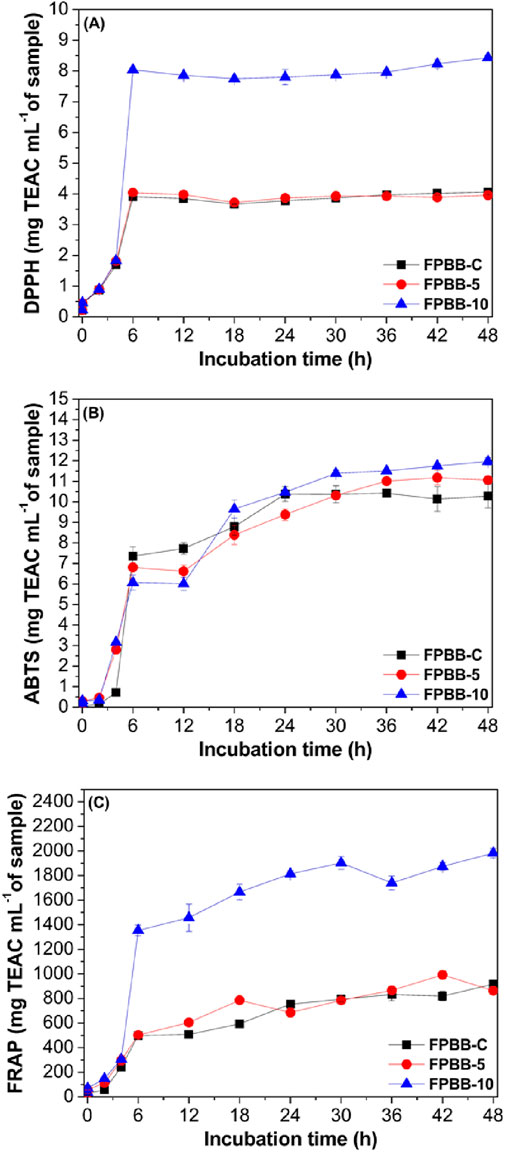
Figure 4. Antioxidant activities (mg TEAC mL−1 of sample) by DPPH (A), ABTS (B), and FRAP (C) of FPBB formulations after simulated gastrointestinal simulation. Incubation time refers to the stages of the gastrointestinal system. Lines are used for better visualization. FPBB-C, Fermented beverage without uvaia pulp; FPBB-5:Fermented beverage enriched with 5% uvaia pulp, FPBB-10: Fermented beverage enriched with 10% uvaia pulp. DPPH (2,2-diphenyl-1-picrylhydrazyl); ABTS (2,2-AZINO-BIS(3-ethylbenzene-thiazoline-6-sulfonic acid) diammonium salt); FRAP (Ferric ion Reducing Antioxidant Power).
According to the DPPH method (Figure 4A), all samples exhibited a considerable increase in AA (p < 0.05) after the in vitro digestibility. In addition, the observed antioxidant activity (AA) behavior remained consistent among all formulations. Initially, there was a gradual increase in AA until the ileum phase, succeeded by a significant increase at 6 h in the colon. Subsequently, there was a decrease after 30 h (FPBB-C), 42 h (FPBB-5), and 36 h (FPBB-10). After that, AA increased again, reaching its maximum values in the colon at 48 h reaching the maximum values (FPBB-C: 4.06, FPBB-5: 3.96, and FPBB-10: 8.44 mg TEAC mL−1 of sample). Still, for the DPPH method, no significant differences (p < 0.05) were observed between FPBB-C and FPBB-5. However, FPBB-10 formulation showed higher AA (p < 0.05) than FPBB-C and FPBB-5, corroborating with TPC results (Section 3.2).
The same behavior was observed for the ABTS method (Figure 4B). All samples showed an increase in AA after in vitro digestibility (p < 0.05), with the maximum value being obtained in the colon at 48 h (FPBB-C: 10.28, FPBB-5: 11.06, and FPBB-10: 11.97 mg TEAC mL−1 of sample). When analyzing the maximum AA between the formulations (FPBB-C, FPBB-5, and FPBB-10), FPBB-5 and FPBB-10 showed higher values than FPBB-C. Consequently, the results demonstrated that uvaia pulp has antioxidant potential when added in amounts equal to or greater than 10% in the fermented vegetable drink by the ABTS radical scavenging activity.
For the FRAP method (Figure 4C), all samples also showed a gradual increase from the beginning of in vitro digestibility until reaching their maximum values of antioxidant activity in the colon in 48 h, with AA 58-, 31-, and 54-fold higher than the undigested FPBB-C, FPBB-5, and FPBB-10 samples, respectively.
The increase in AA (for all methods) observed when the samples reach the descending colon can be attributed to interactions of phenolic compounds with the intestinal microbiota. As previously reported in Sections 3.1, 3.2, these interactions possibly involve structural transformations and the release of more reactive phenolic compounds with higher AA. Flavonols released from FPBB (Table 2), mainly (isoquercetin and quercetin-3-glucosidase), present in higher concentrations at the colon stage and probably contribute to its antioxidant activity. A study by Rodrigues et al. (2021) also identified quercetin in uvaia residue (peel and seed) extracts, attributing the high antioxidant activity (ABTS: 14.22, DPPH: 9.60, and FRAP: 20.79 mmol TE g−1 extract) due to the presence of quercetin and other phenolic compounds.
Besides the presence of phenolic compounds in uvaia pulp, the pea, and rice proteins hydrolysis by the action of pepsin in the stomach phase can lead to the formation and liberation of shorter bioactive peptides with improved antioxidant properties during the ileum and colon digestion of the fermented beverage (Manus et al., 2021), contributing to the increase in AA of the samples. A study by Olagunju et al. (2018) showed that pancreatin-hydrolyzed pea protein with low molecular weight (1–5 kDa) showed better antioxidant activity by the DPPH method (EC50 1.02–1.26 mg mL−1) than the higher molecular weight fractions (EC50 value of 2.44 mg mL−1). Another study by Chen et al. (2023) also showed that rice peptides with low molecular weight (<1 kDa, IC50 26.7 mg mL−1) possess higher antioxidant capacity than high molecular weight peptides (>10 kDa, IC50 39.4 mg mL−1) by the DPPH method.
Others bioactive compounds can also contribute to the AA of the FPBB. Uvaia fruits are abundant in vitamin C, a crucial antioxidant molecule, with concentrations ranging between 9.45 and 122.51 mg per 100 g (Silva et al., 2022), and carotenoids, with concentrations ranging between 1.3 and 441.26 mg per 100 g (Sganzerla et al., 2021), the interaction of those compounds with the radicals can also increase the AA of the FPBB.
In summary, FPBB-10 formulation presented enhanced antioxidant activity for the digested and non-digested samples compared to FPBB-C and FPBB-5. The increased AA in the formulation added 10% uvaia pulp can be related to different factors. As reported in Section 3.2, FPBB-10 contains a significantly higher amount of phenolic compounds than FPBB-C and FPBB-5. This higher concentration directly boosts the antioxidant activity. Additionally, the synergistic effects between different phenolic compounds further enhance this activity. Non-phenolic compounds such as vitamins C and A (previously identified in uvaia pulp (Sganzerla et al., 2021; Spricigo et al., 2023; Silva et al., 2022)), also present in uvaia pulp, contribute to the overall antioxidant effect. Furthermore, polysaccharides and peptides in the formulation support antioxidant activity by scavenging free radicals, chelating metal ions, and stabilizing other antioxidant compounds (Zhang et al., 2024; Fernandes and Coimbra, 2023). Therefore, the superior antioxidant activity of FPBB-10 results from the combined effects, such as a higher concentration of phenolic compounds, synergistic interactions, and the contributions of non-phenolic compounds, polysaccharides, and peptides.
Given the observed reduction in probiotic survival in FPBB-10 during the large intestine phase, it is crucial to determine an optimal uvaia pulp concentration that balances probiotic viability with antioxidant benefits. A concentration between 5% and 10% uvaia pulp appears promising. The 5% concentration (FPBB-5) maintains higher probiotic survival while offering substantial antioxidant benefits. In contrast, the 10% concentration (FPBB-10) significantly enhances antioxidant properties but at the cost of reduced probiotic viability. Thus, a concentration closer to 5% might offer a better balance between maintaining probiotic viability and providing antioxidant benefits.
Upon analyzing the changes in the AA of FPBB formulations, a significant difference was observed in the increase in antioxidant activity, indicating that adding uvaia pulp to fermented vegetable beverages can be a valuable strategy for enhancing the functionality of such beverages.
3.4 Phenolic profiles of uvaia pulp and digested and non-digested FPBB formulations
As previously reported (Section 3.2), including uvaia pulp in the FPBB formulation resulted in an increase in TPC values. Consequently, HPLC analysis was undertaken to identify the phenolic compounds potentially introduced into the preparation through the incorporation of uvaia pulp and to assess any biotransformation of these compounds during the in vitro gastrointestinal assay. The chemical composition (phenolic compounds) of the in natura uvaia pulp (non-digested) and FPBBs formulations were evaluated qualitatively and quantitatively by HPLC-DAD-ESI-MS. The compounds were identified based on their retention times, UV–Vis absorption and mass spectra. HPLC results are shown in Table 2.
Fourteen compounds were identified and quantified in uvaia pulp (undigested sample), reaching a total phenolic content of 49188.00 μg L−1. Most compounds were flavonoids, notably featuring the presence of epigallocatechin gallate (16740.2 μg L−1), quercetin-3-rhamnose (9262.05 μg L−1), and quercetin-3-glucoside (7883.25 μg L−1). Besides that, a significant amount of galangin (7910.65 μg L−1), hesperidin (2242.8 μg L−1), and isoquercetin (1112.95 μg L−1) were detected in the in natura uvaia pulp. For instance, Wanderley et al. (Wanderley et al., 2022) evaluated the phenolic profile of uvaia pulp from Urupema, Santa Catarina, Brazil, identifying (+)-catechin (6.54 mg 100 g−1) as the major phenolic compound. In addition, epicatechin (4.43 mg 100 g-1) and quercetin (0.38 mg 100 g−1) were also detected by the authors, corroborating the HPLC results from this work. Another study by Rodrigues et al. (2021) also identified isoquercitrin, quercetin, and epicatechin in uvaia residue extracts from Paraibuna, SP, Brazil. Nevertheless, these authors did not detect pinocembrin, apigenin, galangin, and coumarin; to our knowledge, this is the first report that has detected those compounds in uvaia pulp. It is known that those compounds are widely reported in fruits and vegetables and that their consumption promotes several benefits to human health, such as anti-inflammatory, antibacterial, anti-cancer properties, and anti-Alzheimer, among others, through its antioxidant activity against free radicals (Elbatreek et al., 2023; Hoff et al., 2022). However, their concentration can vary widely due to plant species, genetics, growing environment, developmental stage, and part of the plant (Spricigo et al., 2023; Rodrigues et al., 2021). This fact also justifies the different phenolic profiles and concentrations of uvaia products among those works.
Only two phenolic compounds were quantified in the non-digested control beverage (FPBB-C): quercetin (67.7 μg L−1) and benzoic acid (113.4 μg L−1). Nevertheless, with the progress of digestion (particularly at the colon), other compounds were liberated from the FPBB-C matrix. For instance, isorhamnetin (84.9 μg L−1) was the only compound detected at colon (6 h). After 12 h of colon fermentation, other compounds were released, including epigallocatechin gallate (113.05 μg L−1), quercetin-3-rhamnose (281.25 μg L−1), isoquercetin (74.3 μg L−1), and also isorhamnetin (80.6 μg L-1). After this gastrointestinal stage (colon 12 h), isorhamnetin was the only compound detected to reach the final GI stage (colon 48 h), being detected again in the colon (24 h) (83.7 μg L−1), colon (42 h) (88.8 μg L−1), and colon (48 h) (88.2 μg L−1). Isorhamnetin-3-O-glucoside, a flavonol compound linked with a carbohydrate, is one of the most abundant flavonoids widely distributed in rice varieties (Wang et al., 2023). Also, isorhamnetin 3-rutinoside has been found in pea seeds, pods, sprouts, and leaves (Wu D. T. et al., 2023). As previously reported, the digestive enzymes and simulated fluids acted on pea and rice proteins (not hydrolyzed by L. rhamnosus GG cells during fermentation) from FPBB-C, converting them into peptides and amino acids, which released and metabolized isorhamnetin. Consistent with the findings from the HPLC analysis, it is likely that isorhamnetin-3-O-glucoside contributed to the high antioxidant activity observed by the DPPH, ABTS, and FRAP methods at 42 and 48 h in the colon.
Regarding the FPBB-5 sample, Table 2 showed a significant release of quercetin-3-glucoside during the oral and gastric, with 82.35 and 81.55 μg L−1, respectively, and quercetin-3-rhamnose in the small intestine (107.7 μg L−1). In our study, isorhamnetin was mainly detected in the later stages of the large intestine, with notable concentrations of 89.9 μg L−1 in the colon at 6 h, 82.7 μg L−1 after 30 h, and 76 μg L−1 at 42 h (Figure 5). This is an interesting finding. According to Olthof et al. (2000), approximately 50% of quercetin glucosides are absorbed in the small intestine. Also, according to the same authors, the non-absorbed quercetin-3-glucoside in the small intestine can be metabolized by the colonic microflora (in this study represented by L. rhamnosus GG) into quercetin aglycone and phenolic acids (for example, into isorhamnetin), which might be absorbed from the colon, the action L. rhamnosus GG in quercetin-3-glucoside (observed at the beginning of the digestion, mouth, and stomach) during the colon digestion may justify the existence of this compound in the colon at 30 and 42 h.
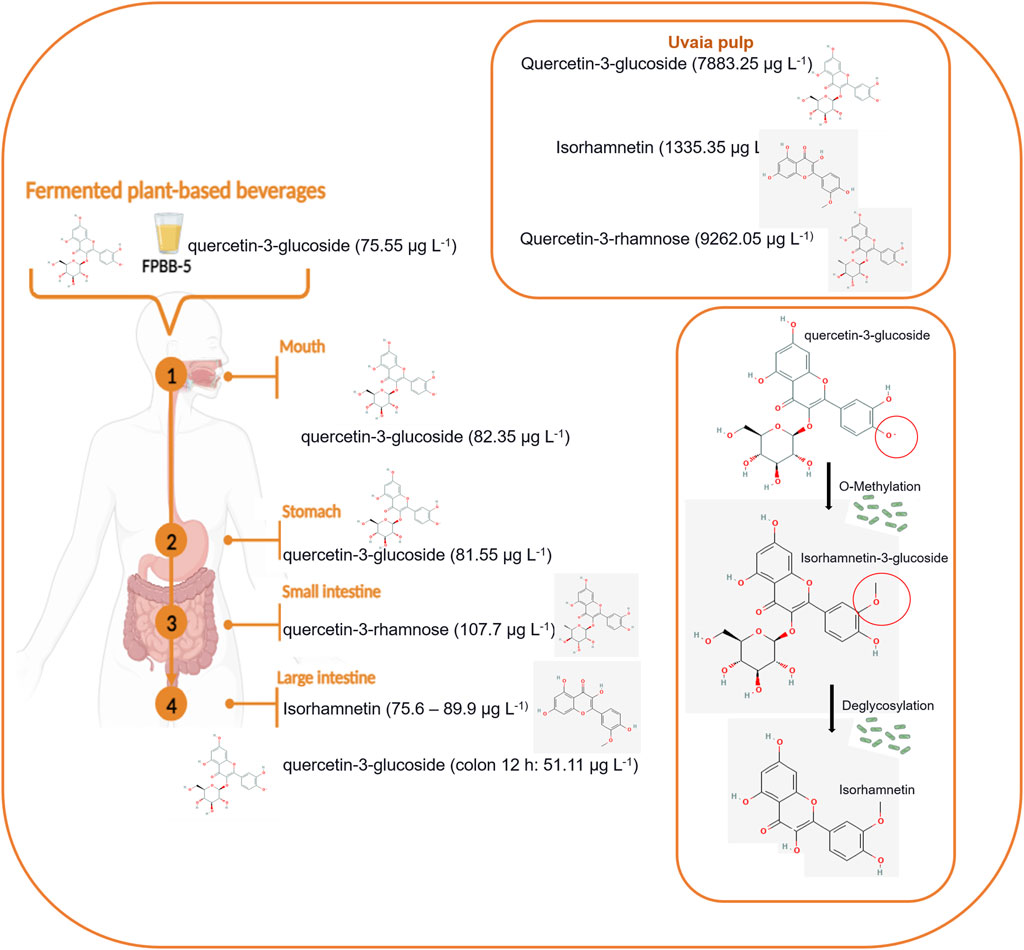
Figure 5. Transformation of quercetin-3-glucoside into isorhamnetin during digestion using FPBB-5 sample as model.
The data from Table 2 about FPBB-10 formulation reveals the continuous presence of quercetin-derived compounds throughout the digestive process, such as quercetin-3-rhamnose, in the mouth (152.35 μg L−1), stomach (164 μg L−1) and quercetin-3-glucosidase, in the small intestine (180.95 μg L−1) and colon 12 h (58.65 μg L−1). Consistent with FPBB-5 formulation, isorhamnetin was also observed at colon 30 h and 42 h with 92.6 and 77 μg L−1 values, respectively. A recent study by Ahn et al. (2020) proved that Bifidobacterium animalis subsp. lactis AD011, isolated from infant feces, catalyzed more than 85% of quercetin-3-glucoside and isorhamnetin-3-glucoside into quercetin and isorhamnetin, respectively, in 2 h. The bio-transformed molecules, quercetin, and isorhamnetin showed improved anti-inflammatory activity compared to the non-bio-transformed molecules against lipopolysaccharide-induced RAW 264.7 macrophages.
The precise enzymatic mechanism underlying the conversion of quercetin-3-glucoside to isorhamnetin by gut microbiota, particularly L. rhamnosus GG, remains largely unknown. According to Williamson and Clifford (2024), specific enzymes and conditions in the gastrointestinal tract facilitate this transformation during digestion. First, quercetin-3-glucoside is hydrolyzed to release quercetin. While there is no evidence of biotransformation by L. rhamnosus GG, other studies, such as those by Ahn et al. (2020), show that produced by Bifidobacterium animalis subsp. lactis can catalyze this biotransformation through the hydrolysis of the β-1,4-glycosidic linkage, promoted by enzymes like β-glucosidase (Terao, 2023). Subsequently, quercetin can undergo a series of metabolic reactions, including methylation, where a methyl group is added to the molecule, transforming it into isorhamnetin. These reactions can be catalyzed by UDP-glucuronosyl transferases, sulfotransferases, and catechol O-methyltransferases (Boonpawa et al., 2014). This transformation is crucial for these compounds’ bioavailability and biological activity, influencing their effects on the body during digestion and absorption. Also, these results corroborate the high TPC results (Supplementary Table S3) and antioxidant capacity of ABTS and FRAP methods (Figure 4), indicating a high correlation between the antioxidant activity and those compounds.
Finally, the presence of quercetin-3-glucoside in uvaia pulp (7883.25 μg L−1), FPBB-5 (non-digested: 75.55 μg L−1), and FPBB-10 (small intestine: 180.95 and colon 12 h: 58.65 μg L−1) and hesperidin (small intestine: 199 μg L−1) in FPBB-10, but its absence in the control formulation emphasizes uvaia pulp’s significance as a source of these compounds in the functional beverage.
Our findings indicate that the bioconversion of phenolic compounds frequently occurs during the fermentation of FPBB ingredients by L. rhamnosus GG, and variations in phenolic profiles can be attributed to the activity of specific enzymes. Furthermore, the composition of phenolic compounds is a dual contribution from the ingredients used - pea and rice proteins- together with uvaia pulp.
4 Conclusions
Fermented plant-based beverages were successfully produced with pea and rice proteins and 5% and 10% uvaia pulp through lactic fermentation with L. rhamnosus GG. During in vitro gastrointestinal simulation assays, the viability of L. rhamnosus GG cells remained high, with concentrations of viable cells according to the classification of FPBB as probiotics (FPBB-C: 9.94 log CFU g−1, FPBB-5: 10.37 log CFU g−1, and FPBB-10: 9.66 log CFU g−1). These results demonstrate that the rice and pea protein matrix effectively carry the L. rhamnosus GG strain. Adding uvaia increased the total phenolic content at the end of the gastrointestinal digestion, indicating an increase in the prebiotic capacity of the beverages. All formulations showed an improvement of antioxidant activity by DPPH, ABTS, and FRAP methods at the end of in vitro digestibility, demonstrating that adding uvaia is relevant and interesting for preparing vegetable beverages, improving their nutritional qualities. Fourteen compounds were identified and quantified in uvaia pulp by HPLC-DAD-ESI-MS, most of them classified as flavonols, being epigallocatechin gallate (16740.2 μg L−1), quercetin-3-rhamnose (9262.05 μg L−1), and quercetin-3-glucoside (7883.25 μg L−1) main phenolics quantified in the pulp. In addition, isorhamnetin was the main phenolic compound detected in the colon for all formulations, probably due to the transformation of quercetin-3-glucoside by the probiotic cells. Finally, this study demonstrates that the food industry can explore new beverage alternatives containing the pea and rice matrix enriched with uvaia pulp, providing an option to consume beverages of plant-based. The preliminary findings of this study are encouraging; however, future research should include clinical trials to confirm the efficacy and health benefits of FPBB formulations in vivo, as in vitro assays may not fully replicate human digestive conditions. Furthermore, it is important to highlight that there is still a gap to be filled in the sensory analysis of the product. The addition of probiotics and uvaia pulp, although promising in functional terms, may affect the organoleptic perception of the product. Therefore, detailed sensory studies are needed to assess consumer response to taste, texture, and overall acceptability of plant-based beverages. Lastly, assessing the scalability and economic feasibility of incorporating uvaia pulp into large-scale production is essential for practical application in the food industry.
Data availability statement
The raw data supporting the conclusions of this article will be made available by the authors, without undue reservation.
Author contributions
TS: Formal Analysis, Investigation, Writing–original draft, Writing–review and editing. RS: Formal Analysis, Investigation, Writing–original draft, Writing–review and editing. GA: Data curation, Formal Analysis, Investigation, Validation, Writing–original draft, Writing–review and editing. GS: Data curation, Writing–original draft, Writing–review and editing. CC-S: Data curation, Formal Analysis, Methodology, Validation, Writing–original draft, Writing–review and editing. RH: Data curation, Formal Analysis, Investigation, Methodology, Supervision, Validation, Writing–original draft, Writing–review and editing. SV: Conceptualization, Data curation, Formal Analysis, Funding acquisition, Methodology, Project administration, Resources, Supervision, Validation, Writing–original draft, Writing–review and editing.
Funding
The author(s) declare that financial support was received for the research, authorship, and/or publication of this article. The authors are thankful to the National Council for Scientific and Technological Development (CNPq—Brazil), grant number [402338/2023-5], and Coordination for the Improvement of Higher Level Personnel (CAPES—Brazil) for the financial support and scholarships (Code 001).
Acknowledgments
They are also thankful to V. C. Zanetti, E. M. Maran, and G. Mendes for helping prepare material for some analyses and to M. Cargnin for providing the uvaia pulp.
Conflict of interest
The authors declare that the research was conducted in the absence of any commercial or financial relationships that could be construed as a potential conflict of interest.
Publisher’s note
All claims expressed in this article are solely those of the authors and do not necessarily represent those of their affiliated organizations, or those of the publisher, the editors and the reviewers. Any product that may be evaluated in this article, or claim that may be made by its manufacturer, is not guaranteed or endorsed by the publisher.
Supplementary material
The Supplementary Material for this article can be found online at: https://www.frontiersin.org/articles/10.3389/frfst.2024.1460402/full#supplementary-material
References
Ahn, H. J., You, H. J., Park, M. S., Li, Z., Choe, D., Johnston, T. V., et al. (2020). Microbial biocatalysis of quercetin-3-glucoside and isorhamnetin-3-glucoside inSalicornia herbaceaand their contribution to improved anti-inflammatory activity. RSC Adv. 10, 5339–5350. doi:10.1039/c9ra08059g
Alcorta, A., Porta, A., Tárrega, A., Alvarez, M. D., and Pilar Vaquero, M. (2021). Foods for plant-based diets: challenges and innovations. Foods 10, 293. doi:10.3390/foods10020293
Arend, G. D., Almeida, É. S., Byruchko, R. T., Pinto, M. E. G., da Cruz, A. B., Verruck, S., et al. (2022). Gravitational and microwave-assisted multi-stages block freeze concentration process to obtain enriched concentrated beet (Beta vulgaris L.) by-products extract: bioactive compounds and simulated gastrointestinal profile. Food Bioprod. Process. 133, 77–86. doi:10.1016/j.fbp.2022.03.004
Bianchini, C. B., Vieira, M. P. T., Arriola, N. D. A., Dias, C. O., Seraglio, S. K. T., Costa, A. C. O., et al. (2020). Incorporation of uvaia (Eugenia pyriformisCambess) pulp in yogurt: a promising application in the lactose-free dairy product market. J. Food Process Preserv 44, 1–8. doi:10.1111/jfpp.14829
Boonpawa, R., Spenkelink, A., Rietjens, I. M. C. M., and Punt, A. (2014). A physiologically based kinetic (PBK) model describing plasma concentrations of quercetin and its metabolites in rats. Biochem. Pharmacol. 89, 287–299. doi:10.1016/j.bcp.2014.02.007
Brodkorb, A., Egger, L., Alminger, M., Alvito, P., Assunção, R., Ballance, S., et al. (2019). INFOGEST static in vitro simulation of gastrointestinal food digestion. Nat. Protoc. 14, 991–1014. doi:10.1038/s41596-018-0119-1
Camelo-Silva, C., Mota e Souza, B., Vicente, R., Arend, G. D., Sanches, M. A. R., Barreto, P. L. M., et al. (2024). Polyfunctional sugar-free white chocolate fortified with Lacticaseibacillus rhamnosus GG co-encapsulated with beet residue extract (Beta vulgaris L.). Food Res. Int. 179, 114016. doi:10.1016/j.foodres.2024.114016
Castro-López, C., Romero-Luna, H. E., García, H. S., Vallejo-Cordoba, B., González-Córdova, A. F., and Hernández-Mendoza, A. (2023). Key stress response mechanisms of probiotics during their journey through the digestive system: a review. Probiotics Antimicrob. Proteins 15, 1250–1270. doi:10.1007/s12602-022-09981-x
Cattivelli, A., Nissen, L., Casciano, F., Tagliazucchi, D., and Gianotti, A. (2023). Impact of cooking methods of red-skinned onion on metabolic transformation of phenolic compounds and gut microbiota changes. Food Funct. 14, 3509–3525. doi:10.1039/d3fo00085k
Chai, L. N., Wu, H., Wang, X. J., He, L. J., and Guo, C. F. (2023). The mechanism of antimicrobial activity of conjugated bile acids against lactic acid bacilli. Microorganisms 11, 1823. doi:10.3390/microorganisms11071823
Chen, H. J., Dai, F. J., Chen, C. Y., Fan, S. L., Zheng, J. H., Chau, C. F., et al. (2023). Effects of molecular weight fraction on antioxidation capacity of rice protein hydrolysates. Sci. Rep. 13, 3464. doi:10.1038/s41598-022-14314-7
Cuvas-Limón, R. B., Ferreira-Santos, P., Cruz, M., Teixeira, J. A., Belmares, R., and Nobre, C. (2022). Novel bio-functional Aloe vera beverages fermented by probiotic Enterococcus faecium and Lactobacillus lactis. Molecules 27, 2473. doi:10.3390/molecules27082473
Deziderio, M. A., de Souza, H. F., Kamimura, E. S., and Petrus, R. R. (2023). Plant-based fermented beverages: development and characterization. Foods 12, 4128. doi:10.3390/foods12224128
Duda-Chodak, A. (2012). The inhibitory effect of polyphenols on human gut microbiota. J. Physiology Pharmacol. 63, 497–503.
Elbatreek, M. H., Mahdi, I., Ouchari, W., Mahmoud, M. F., and Sobeh, M. (2023). Current advances on the therapeutic potential of pinocembrin: an updated review. Biomed. Pharmacother. 157, 114032. doi:10.1016/j.biopha.2022.114032
Farias, D. de P., Araújo, F. F., Neri-Numa, I. A., Dias-Audibert, F. L., Delafiori, J., Catharino, R. R., et al. (2021). Effect of in vitro digestion on the bioaccessibility and bioactivity of phenolic compounds in fractions of Eugenia pyriformis fruit. Food Res. Int. 150, 110767. doi:10.1016/j.foodres.2021.110767
Fernandes, P. A. R., and Coimbra, M. A. (2023). The antioxidant activity of polysaccharides: a structure-function relationship overview. Carbohydr. Polym. 314, 120965. doi:10.1016/j.carbpol.2023.120965
Galanakis, C. M. (2021). Functionality of Food Components and Emerging Technologies. Foods 10, 128. doi:10.3390/foods10010128
Gentile, L. (2020). Protein–polysaccharide interactions and aggregates in food formulations. Curr. Opin. Colloid Interface Sci. 48, 18–27. doi:10.1016/j.cocis.2020.03.002
Grand View Research (2024). Dairy alternatives market size and trends. Rep. ID GVR-1-68038-070-5. Available at: https://www.grandviewresearch.com/industry-analysis/dairy-alternatives-market (Accessed August, 2024).
Hoff, R., Daguer, H., Deolindo, C. T. P., de Melo, A. P. Z., and Durigon, J. (2022). Phenolic compounds profile and main nutrients parameters of two underestimated non-conventional edible plants: pereskia aculeata Mill. (ora-pro-nóbis) and Vitex megapotamica (Spreng.) Moldenke (tarumã) fruits. Food Res. Int. 162, 112042. doi:10.1016/j.foodres.2022.112042
Kupikowska-Stobba, B., Domagała, J., and Kasprzak, M. M. (2024). Critical review of techniques for food emulsion characterization. Appl. Sci. 14, 1069. doi:10.3390/app14031069
Liu, L., and Firrman, J. (2016). Genetic expression profile analysis of the temporal inhibition of quercetin and naringenin on Lactobacillus rhamnosus GG. J. Probiotics Health 04, 1000139. doi:10.4172/2329-8901.1000139
Lopes, J. M. M., Lage, N. N., Guerra, J. F. C., Silva, M., Bonomo, L. F., Paulino, A. H. S., et al. (2018). A preliminary exploration of the potential of Eugenia uvalha Cambess juice intake to counter oxidative stress. Food Res. Int. 105, 563–569. doi:10.1016/j.foodres.2017.11.067
Lu, Z. X., He, J. F., Zhang, Y. C., and Bing, D. J. (2020). Composition, physicochemical properties of pea protein and its application in functional foods. Crit. Rev. Food Sci. Nutr. 60, 2593–2605. doi:10.1080/10408398.2019.1651248
Ma, J., Miao, Y., Li, J., Ma, Y., Wu, M., Wang, W., et al. (2022). Incorporation of blue honeysuckle juice into fermented goat milk: physicochemical, sensory and antioxidant characteristics and in vitro gastrointestinal digestion. Foods 11, 3065. doi:10.3390/foods11193065
Manus, J., Millette, M., Uscanga, B. R. A., Salmieri, S., Maherani, B., and Lacroix, M. (2021). In vitro protein digestibility and physico-chemical properties of lactic acid bacteria fermented beverages enriched with plant proteins. J. Food Sci. 86, 4172–4182. doi:10.1111/1750-3841.15859
Masiá, C., Geppel, A., Jensen, P. E., Buldo, P., Smith, C. J., and Lluna, A. G. (2021). Effect of Lactobacillus rhamnosus on physicochemical properties of fermented plant-based raw materials. Foods 10, 573. doi:10.3390/foods10030573
McCoubrey, L. E., Favaron, A., Awad, A., Orlu, M., Gaisford, S., and Basit, A. W. (2023). Colonic drug delivery: formulating the next generation of colon-targeted therapeutics. J. Control. Release 353, 1107–1126. doi:10.1016/j.jconrel.2022.12.029
Mohamadzadeh, M., Fazeli, A., and Shojaosadati, S. A. (2024). Polysaccharides and proteins-based bionanocomposites for microencapsulation of probiotics to improve stability and viability in the gastrointestinal tract: a review. Int. J. Biol. Macromol. 259, 129287. doi:10.1016/j.ijbiomac.2024.129287
Muncey, L., and Hekmat, S. (2021). Development of probiotic almond beverage using Lacticaseibacillus rhamnosus GR-1 fortified with short-chain and long-chain inulin fibre. Fermentation 7, 90. doi:10.3390/fermentation7020090
Olagunju, A. I., Omoba, O. S., Enujiugha, V. N., Alashi, A. M., and Aluko, R. E. (2018). Pigeon pea enzymatic protein hydrolysates and ultrafiltration peptide fractions as potential sources of antioxidant peptides: an in vitro study. LWT 97, 269–278. doi:10.1016/j.lwt.2018.07.003
Olthof, M. R., Hollman, P. C. H., Vree, T. B., and Katan, M. B. (2000). Bioavailabilities of quercetin-3-glucoside and quercetin-4'-glucoside do not differ in humans. J. Nutr. 130 (5), 1200–1203. doi:10.1093/jn/130.5.1200
Popova, A., Mihaylova, D., and Lante, A. (2023). Insights and Perspectives on Plant-Based Beverages. Plants, 12, 3345. doi:10.3390/plants12193345
Prestes, A. A., Verruck, S., Vargas, M. O., Canella, M. H. M., Silva, C. C., da Silva Barros, E. L., et al. (2021). Influence of guabiroba pulp (campomanesia xanthocarpa o. berg) added to fermented milk on probiotic survival under in vitro simulated gastrointestinal conditions. Food Res. Int. 141, 110135. doi:10.1016/j.foodres.2021.110135
Ramirez, M. R., Schnorr, C. E., Feistauer, L. B., Apel, M., Henriques, A. T., Moreira, J. C. F., et al. (2012). Evaluating the polyphenolic content, anti-inflammatory, and antioxidant activities of total extract from Eugenia pyriformes (uvaia) fruit. J. Food Biochem. 36, 405–412. doi:10.1111/j.1745-4514.2011.00558.x
Reese, I., Schäfer, C., Ballmer-Weber, B., Beyer, K., Dölle-Bierke, S., van Dullemen, S., et al. (2023). Vegan diets from an allergy point of view - position paper of the DGAKI working group on food allergy. Allergol. Sel. 7, 57–83. doi:10.5414/ALX02400E
Rodrigues, L. M., Romanini, E. B., Silva, E., Pilau, E. J., Da Costa, S. C., and Madrona, G. S. (2021). Uvaia (Eugenia pyriformis Cambess) residue as a source of antioxidants: an approach to ecofriendly extraction. LWT 138, 110785. doi:10.1016/j.lwt.2020.110785
Rufino, S. M., Alves, R. E., De Brito, E. S., Selene, M. D. M., Sampaio, C. D. G., Pérez-Jiménez, J., et al. (2007). Scientific methodology: determination of total antioxidant activity in fruits by DPPH free radical capture. Brasília, DF (Brazil): EMBRAPA, 127.
Rumpf, J., Burger, R., and Schulze, M. (2023). Statistical evaluation of DPPH, ABTS, FRAP, and Folin-Ciocalteu assays to assess the antioxidant capacity of lignins. Int. J. Biol. Macromol. 233, 123470. doi:10.1016/j.ijbiomac.2023.123470
Sganzerla, W. G., Komatsu, R. A., Mangrich dos Passos, J. F., Costa, M. D., Dobler Stroschein, M. R., Schons, P. F., et al. (2021). Variability in the molecular, phenological, and physicochemical characteristics of uvaia (Eugenia pyriformis Cambess - Myrtaceae) accessions from the Brazilian Atlantic rainforest. Biocatal. Agric. Biotechnol. 35, 102082. doi:10.1016/j.bcab.2021.102082
Sheen, F., Lim, A. J. Y., and Forde, C. G. (2023). Diversity among flexitarian consumers; stratifying meat reducers by their underlying motivations to move to a plant-based diet. Food qual prefer 112, 105022.
Sigüenza-Andrés, T., Gómez, M., Rodríguez-Nogales, J. M., and Caro, I. (2023). Development of a fermented plant-based beverage from discarded bread flour. LWT 182, 114795. doi:10.1016/j.lwt.2023.114795
Silva, A. P. G., Sganzerla, W. G., Jacomino, A. P., da Silva, E. P., Xiao, J., and Simal-Gandara, J. (2022). Chemical composition, bioactive compounds, and perspectives for the industrial formulation of health products from uvaia (Eugenia pyriformis Cambess – Myrtaceae): a comprehensive review. J. Food Compos. Analysis 109, 104500. doi:10.1016/j.jfca.2022.104500
Singleton, V. L., and Rossi, J. A. (1965). Colorimetry of total phenolics with phosphomolybdic-phosphotungstic acid reagents. Am. J. Enol. Vitic. 16, 144–158. doi:10.5344/ajev.1965.16.3.144
Spricigo, P. C., Almeida, L. S., Ribeiro, G. H., Correia, B. S. B., Taver, I. B., Jacomino, A. P., et al. (2023). Quality attributes and metabolic profiles of uvaia (eugenia pyriformis), a native Brazilian atlantic forest fruit. Foods 12, 1881. doi:10.3390/foods12091881
Tang, Z., Zhao, Z., Chen, S., Lin, W., Wang, Q., Shen, N., et al. (2023). Dragon fruit-kiwi fermented beverage: in vitro digestion, untargeted metabolome analysis and anti-aging activity in Caenorhabditis elegans. Front. Nutr. 9, 1–15. doi:10.3389/fnut.2022.1052818
Terao, J. (2023). Potential role of quercetin glycosides as anti-atherosclerotic food-derived factors for human health. Antioxidants 12, 258. doi:10.3390/antiox12020258
Verruck, S., Barretta, C., Miotto, M., Canella, M. H. M., de Liz, G. R., Maran, B. M., et al. (2020). Evaluation of the interaction between microencapsulated Bifidobacterium BB-12 added in goat's milk Frozen Yogurt and Escherichia coli in the large intestine. Food Res. Int. 127, 108690. doi:10.1016/j.foodres.2019.108690
Verruck, S., Prudêncio, E. S., Vieira, C. R. W., Amante, E. R., and de Mello Castanho Amboni, R. D. (2015). The buffalo Minas Frescal cheese as a protective matrix of Bifidobacterium BB-12 under in vitro simulated gastrointestinal conditions. LWT 63, 1179–1183. doi:10.1016/j.lwt.2015.04.014
Wanderley, B. R. da S. M., Haas, I. C. da S., Biluca, F. C., Brugnerotto, P., Gomes, T. M., Aquino, A. C. M. de S., et al. (2022). Phenolic profiling, organic acids and sugars composition of feijoa (Acca sellowiana (O. Berg) Burret) and uvaia (Eugenia pyriformis Cambess) from the southern Brazilian highlands. Cienc. Rural. 52, 1–6. doi:10.1590/0103-8478cr20210458
Wang, H., Chen, L., Yang, B., Du, J., Chen, L., Li, Y., et al. (2023). Structures, sources, identification/quantification methods, health benefits, bioaccessibility, and products of isorhamnetin glycosides as Phytonutrients. Nutrients 15, 1947. doi:10.3390/nu15081947
Wang, X., Qi, Y., and Zheng, H. (2022). Dietary polyphenol, gut microbiota, and health benefits. Antioxidants 11, 1212. doi:10.3390/antiox11061212
Williamson, G., and Clifford, M. N. (2024). A critical examination of human data for the biological activity of quercetin and its phase-2 conjugates. Crit. Rev. Food Sci. Nutr., 1–37. doi:10.1080/10408398.2023.2299329
Wu, C., Wang, J., Liu, N., Chen, X., Xu, H., and Lei, H. (2023a). Phytochemical properties and antioxidant capacities of apple juice fermented by probiotics during refrigerated storage and simulated gastrointestinal digestion. Appl. Biochem. Biotechnol. 195, 6032–6049. doi:10.1007/s12010-022-04255-2
Wu, D. T., Li, W. X., Wan, J. J., Hu, Y. C., Gan, R. Y., Zou, L., et al. (2023b). Comparison of soluble dietary fibers extracted from ten traditional legumes: physicochemical properties and biological functions. Foods 12, 2352. doi:10.3390/foods12122352
Zhang, L., Wu, T., Zhang, Y., Chen, Y., Ge, X., Sui, W., et al. (2023). Release of bound polyphenols from wheat bran soluble dietary fiber during simulated gastrointestinal digestion and colonic fermentation in vitro. Food Chem. 402, 134111. doi:10.1016/j.foodchem.2022.134111
Keywords: rice protein, pea protein, Brazilian native fruit, Lacticaseibacillus rhamnosus GG, plant-based beverage
Citation: Santana de Oliveira T, Sant’Anna R, Arend GD, Sorita GD, Camelo-Silva C, Hoff RB and Verruck S (2024) Fermented plant-based beverage supplemented with uvaia (Eugenia pyriformis) pulp: an innovative and pioneering approach to diversify plant-based diet product market. Front. Food. Sci. Technol. 4:1460402. doi: 10.3389/frfst.2024.1460402
Received: 06 July 2024; Accepted: 09 September 2024;
Published: 23 September 2024.
Edited by:
Renata Bonomo, Universidade Estadual do Sudoeste da Bahia, BrazilReviewed by:
Adriana Cristina Urcan, University of Agricultural Sciences and Veterinary Medicine of Cluj-Napoca, RomaniaMaria Simona Chis, University of Agricultural Sciences and Veterinary Medicine of Cluj-Napoca, Romania
Copyright © 2024 Santana de Oliveira, Sant’Anna, Arend, Sorita, Camelo-Silva, Hoff and Verruck. This is an open-access article distributed under the terms of the Creative Commons Attribution License (CC BY). The use, distribution or reproduction in other forums is permitted, provided the original author(s) and the copyright owner(s) are credited and that the original publication in this journal is cited, in accordance with accepted academic practice. No use, distribution or reproduction is permitted which does not comply with these terms.
*Correspondence: Silvani Verruck, c2lsdmFuaS52ZXJydWNrQHVmc2MuYnI=