- 1Department of Biochemistry, Kwara State University Malete, Ilorin, Nigeria
- 2Department Pharmaceutical and Medicinal Chemistry, University of Ilorin, Ilorin, Nigeria
- 3Department of Biochemistry, University of Abuja, Abuja, Nigeria
The use of gluten-free ingredients in the baking of cookies is increasing due to gluten-associated disorder and other nutritional-related non-communicable diseases, and consumer's pursuit for health-promoting foods. In this study, gluten-free cookies (GFC) were made from biofortified yellow maize supplemented with sodium carboxyl methylcellulose (SCMC), a reference hydrocolloid, and Brachystegia eurycoma flour (BEF) at four different proportions (2.5, 5, 7.5, and 10%), respectively. The GFC were then assessed for their bioactive components (flavonoids, tannins, and saponins), carotenoids, starch-hydrolyzing enzymes (alpha-amylase and alphaglucosidase), and glycemic index. Cookies made from commercial wheat flour (100% CWF) served as control. The total flavonoid contents of the 100% BYF cookies (144.48 ± 0.18 mg/g) and saponins (1.04 ± 0.00 mg/g) were significantly (p < 0.05) higher than the 100% CWF cookies (93.84 ± 0.36 mg/g; 1.02 ± 0.00 mg/g). The total tannin contents of the cookies ranged from 3.12 ± 0.00 mg/g (100% CWF) to 4.87 ± 0.00 mg/g (2.5%BEF-97.5% BYF). The carotenoid profiles indicated the presence of lutein (range from 0.62 to 1.63 μg/g), β-carotene (range from 3.81 to 6.06 μg/g), β-cryptoxanthin (range from 0.19 to 1.75 μg/g) and zeaxanthin (range from 0.83 to 2.48 μg/g) in the cookies. Further, the GF cookies significantly (p < 0.05) inhibited the alpha-amylase (IC50 value ranged from 1.42 ± 0.01 μg/mL (100% BYF) to 2.63 ± 0.01 μg/mL (100% CWF)) and alpha-glucosidase with IC50 value range from 1.12 ± 0.01 μg/mL (2.5%SCMC-97.5% BYF) to 6.72 ± 0.01 (7.5%BEF-92.5% BYF). The GF cookies showed a low glycemic index (GI) value (GI < 50). The biofortified yellow maize-based GFC have significant amounts of bioactive compounds and anti-hyperglycemic properties, and low GI. Hence, they can serve as functional baked food with potential health benefits.
1 Introduction
Cookies are among the bakery products consumed as a snack by human beings (Susman et al., 2021). It is characterized by low water content which contributes to its lengthy shelf life due to the absence of microbiological deterioration (Dhankhar, 2013). Cookies are usually made from refined wheat flour, which contains gluten that gives its dough a remarkable viscoelastic properties (Elif and Duygu, 2020; Irondi et al., 2023; Imam et al., 2024). However, there has been an increase in the baking of cookies using gluten-free (GF) ingredients owing to gluten-associated disorders like the increasing prevalence of celiac disease, consumers’ pursuit for health-promoting foods as well as other nutrition-related non-communicable diseases (Irondi et al., 2023; Imam et al., 2024). Pseudocereals, tubers, seeds, legumes, nuts, fruits, and cereals constitute a few of these elements (Jnawali et al., 2016; Bashir et al., 2020; Xu et al., 2020; Irondi et al., 2023; Imam et al., 2024). Previous investigation has suggested that GF products can provide medical advantages including anti-diabetic, anti-cancer, anti-hypertensive, and hypo-cholesterolemia characteristics (Manzoor, et al., 2020; Irondi et al., 2023; Imam et al., 2024). These have been related to their chemical composition, particularly the presence of bioactive substances (Fouad and Rehab, 2013; Sethiya et al., 2014). Typical GF cookie ingredients including flour, rice starch, and corn starch have been linked to possible worries among those with metabolic issues (such as diabetes and obesity). Risks include a high glycemic index after ingesting owing to their starch content (Elif and Duygu, 2020). When making GF cookies, ingredients that might mitigate these risks and have superior health benefits might be a good substitute (Di Cairano et al., 2018).
Maize (Zea mays L.), is an arable crop cultivated predominantly for its grains, which are crucial for both human and animal nutrition. It is the third-popular grain cultivated worldwide following rice and wheat (Yousaf et al., 2020) and serves as an excellent source of minerals, iron, vitamin B, carbohydrates, and protein (Siwela et al., 2020). Biofortified yellow maize (provitamin A biofortified maize) is a major dietary source of bioactive substances like carotenoids, vitamin C, polyphenolic compounds, and anthocyanins (Alamu et al., 2018; Beta and Hwang, 2018; Elemosho et al., 2020). These bioactive chemicals may provide anti-obesity, antidiabetic, and antioxidant effects on health (Žilić et al., 2012; Irondi et al., 2019a). Moreover, Brachystegia eurycoma (BE) is a naturally occurring hydrocolloid source that is underutilized and can imitate the viscoelastic properties of gluten (Irondi et al., 2021a; Irondi et al., 2022). Additionally, it provides nutrients including protein, calories, carbs, minerals, and vitamins (Nwosu, 2012) as well as antioxidants and important phytochemicals such as flavonoids, saponins, phenolic compounds, tannins that render health benefits (Irondi et al., 2015; Irondi et al., 2021a). The health advantages of gluten-free cookies can be increased by baking them with biofortified yellow maize and Brachystegia eurycoma. Therefore, the aim of this study is to evaluate the bioactive content and anti-hyperglycemic qualities of biofortified gluten-free cookies made from biofortified yellow maize and Brachystegia eurycoma.
2 Materials and methods
2.1 Materials
The materials used in this study were biofortified yellow maize (BYM) grains and B. eurycoma (BE) seed (Figure 1), commercial wheat flour (CWF) (Triticum aestivum), and sodium carboxyl methylcellulose (SCMC) (reference hydrocolloids). These materials were purchased from a neighborhood market in Ilorin, Kwara State. The chemicals and reagents used in this study were of analytical grade.
2.2 Methods
2.2.1 Preparation of flour samples used for cookie production
BE seeds and BYM grains were ground into distinct flours (Figure 2). The biofortified yellow maize flour (BYF) blends used to make BYF-based cookies were formulated by adding SCMC and BE flour (BEF) to BYF at different proportions (2.5, 5, 7.5, and 10%), respectively. CWF was used to produce the control cookies.
2.2.2 Baking of cookies
The cookies were baked by employing Okaka and Isieh’s (1990) method. Using a Kenwood Chef, the 200 g flour sample was mixed with 100 g margarine until a light and fluffy consistency was obtained. When the speed mark reached the 6 marks on the indicator, 60 g of whole egg, 0.1 g of baking powder, 20 g of powdered milk, and 1 g of salt were combined with the flour sample to create a batter or stiff paste. Next, the batter was rolled to thickness (0.2–0.3 cm) using a floured board and rolling pin. After shaping the rolled batter into forms, it was placed on a tray coated with oil and cooked for 20 min at 150°C. The cookies (as seen in Figure 3) were removed and placed in cellophane bags for further analysis.
2.2.3 Preparation of methanolic extract
The methanolic extract of the cookies flour was prepared using the procedure of Chan et al., 2007. Methanol (10 mL) was added to a capped 50 mL centrifuge tube containing 1 g of the cookie samples. After a few minutes of vigorous shaking at ambient temperature, the mixture was left overnight. It was then filtered to obtain the supernatant (methanolic extract) and was stored at −4°C until analysis.
2.2.4 Quantitative determination of bioactive compounds of the cookies
The following standard techniques were followed to quantitatively quantify the bioactive chemicals present in the samples.
2.2.4.1 Determination of total flavonoids content
The aluminum chloride method as reported by Kale et al. (2010) was used to quantify the total flavonoid contents. Methanolic extract (0.5 mL) was dispensed into a test tube, followed by 1.5 mL of methanol, 0.1 mL of aluminum chloride (10%), 0.1 mL of 1 M potassium acetate, and 2.8 mL of distilled water. The reaction mixture was shaken, incubated at room temperature for 30 min and absorbance was then read at 514 nm. The total flavonoid content was expressed as quercetin equivalent (QE) in mg/g material.
2.2.4.2 Determination of total tannin contents
The tannin content of the sample was measured using the technique described by Amorim et al. (2008). To sum up, 1 mL of 35% sodium carbonate solution, 7.5 mL of distilled water, 0.5 mL of Folin-Denis reagent, and 0.1 mL of supernatant were combined and then further diluted to 10 mL using distilled water. A UV/Visible spectrophotometer was then used to measure the mixture’s absorbance (760 nm) after shaking it and letting it sit at room temperature for 30 min. The tannic acid equivalent was used to express the amount of tannin in mg/g of material.
2.2.4.3 Determination of total saponins
The total saponins content of the extract was determined by the method described by Makkar et al. (2007). An aliquot (0.25 mL) of the supernatant was pipette into a test tube after which 0.25 mL vanillin reagent (8% vanillin in ethanol) and 72% aqueous H2SO4 (2.5 mL) were added. The reaction mixture in the tubes was heated in a water bath (60 °C) for 10 min. The tubes were cooled in ice for 4 min and allowed to acclimatize to room temperature. Thereafter, the absorbance was read in a UV/Visible spectrophotometer at 544 nm. Diosgenin was used as a standard, and the result obtained was expressed as mg diosgenin equivalent per gram.
2.2.5 Quantification of carotenoid contents of the cookies
The carotenoid content of the cookie sample was quantified using the procedure outlined by Howe and Tanumihardjo (2006). In a nutshell, 0.6 g of the sample and 6 mL of ethanol (with 0.1% butylated hydroxyl toluene) were combined to extract carotenoids from the flours. The mixture spent 5 minutes in an 85°C water bath. The interfering oil in the mixture was then saponified for 5 minutes at 85°C in a water bath using potassium hydroxide (80% w/v). After combining the suspension with a vortex, it was put back into the water bath for an additional 5 minutes. It was put into an ice-cold bath right away, and 3 mL of deionized water were added. Three times in a row, the mixture’s carotenoid contents were extracted using 3 mL of n-hexane and centrifuged at 1,000 rpm for 10 s. A 50 mL concentrator tube was filled with the upper layer of the mixture. The mixed hexane fraction was centrifuged for 10 s at 1,000 rpm, vortexed three times, and then cleaned with deionized water. Using a Turbo Vap (LIV) Concentrator with nitrogen gas for 25 min, the n-hexane fraction was dried down. Then, an aliquot (100 µL) was injected into the HPLC system to quantify the carotenoids after the dried extract was reconstituted with 1 mL of methanol/dichloromethane, 50:50 v/v.
The HPLC system (Water Corporation, Milford, MA, USA) included an auto-sampler (Waters 717), a photodiode array detector (Waters 2996), a binary HPLC pump (Waters 626), a guard column, and a C30 YMC carotenoid column (4.6 × 250 mm, 3 µM). Empower one software (Waters Corporation) was used to run the system. The mobile phase was made up of solvent B, which contained 100% methyl tertiary-butyl ether, and solvent A, which included methanol-water (92:8 v/v) with 10 mmol/L ammonium acetate. Gradient elution was carried out in the following circumstances, with a flow rate of 1 mL/min: There were four linear gradients in total—29 min from 83% to 59% A, 6 min from 59% to 30% A, 1 min at 30% A, 4 min from 30% to 83% A, and 4 min at 83%. Chromatograms were generated at 450 nm, and their carotenoids were identified and quantified using the external standards approach. This involved comparing the absorption spectrum and co-elution with standard carotenoids, as well as utilizing the calibration curve from pure standards.
2.2.6 In vitro starch -digesting enzymes (alpha-amylase and alpha-glucosidase) inhibitory assays of the cookies
2.2.6.1 In vitro αlpha-glucosidase inhibition assay
Alpha-glucosidase inhibitory activity was conducted following the method reported by Kim et al. (2005) using α-glucosidase (EC 3.2.1.20) and para-nitrophenyl glucopyranoside (PNPG) as the substrate. An aliquot of α-glucosidase (Five units) was incubated with cookies methanol extracts (20 μg/mL) for 15 min. Next, 3 mM PNPG was dissolved in 20 mM phosphate buffer (pH 6.9) as a substrate to initiate the hydrolytic reaction. The hydrolytic reaction was allowed to proceed for 20 min at 37°C, after which it was terminated by adding 0.1 M Na2CO3 (2 mL). The absorbance of the yellow p-nitrophenol released from PNPG hydrolysis was read at 400 nm. The percentage (%) inhibition of α-glucosidase was calculated as follows:
2.2.6.2 In Vitro alpha-amylase inhibition assay
The method described by Kwon et al. (2008) was used to conduct alpha-amylase inhibition assay. Porcine pancreas α-amylase (EC 3.2.1.1) and soluble starch were used in this assay as enzyme and substrate respectively. Appropriate dilutions of methanol extract of cookies samples (500 μL) and 0.02 M sodium phosphate buffer (500 μL) (pH 6.9 with 0.006 M NaCl) containing 0.5 mg/mL α-amylase solution were incubated (37°C) for 10 min. Afterward, 1% starch solution (500 μL) in 0.02 M sodium phosphate buffer was added. Next, the reaction mixture was incubated at 37°C for 15 min, after which 1.0 mL of DNSA color reagent (1% 3, 5-dinitro salicylic acid, and 12% sodium potassium tartrate in 0.4 M NaOH) was added to terminate the reaction. Next, the reaction mixture was incubated for 5 min in a boiling water bath, cooled to room temperature, and diluted with 10 mL of distilled water. The absorbance was read at 540 nm. The percentage (%) inhibition of α-amylase will be calculated as follows:
2.3 Evaluation of the glycemic index of cookies
The study on the glycemic index (GI) was approved by the Kwara State Ministry of Health, Ilorin with approval number MOH/KS/EU/777/493. Sixty (6) healthy, non-diabetic human subjects (30 males and 30 females) between 28 and 50 years were recruited for the clinical trials upon obtaining their information consent. Measurement and calculation of GI were based on the method described by FAO/WHO (1998). Subjects used had no known diseases and were not on any medications which could influence the results of the study. They were strictly informed to abstain from cigarette smoking or alcohol and caffeine-containing drinks within the period of the study. All subjects fasted overnight (7.30 p.m.–7.30 a.m.) before the morning testing and were instructed not to engage in strenuous physical activities before testing days. Data on height, weight, and age were obtained for each subject before the test.
2.4 Data analysis
Results of triplicate experiments were expressed as mean ± standard deviation (SD). Analysis of variance (ANOVA) was carried out on the result data and Duncan’s Multiple Range Test was performed to compare the means of the data at a 95% confidence level using SPSS software (21st version). Graph pad prism eight was used in plotting the graphs.
3 Result and discussion
3.1 Bioactive compounds of the cookies
The result of the bioactive compounds determination is presented in Table 1. From the result, 100% BYF had the highest value of total flavonoid content (144.48 ± 0.18 mg/g) which is significantly higher (p < 0.05) than that of the 100% CWF cookies (93.84 ± 0.36 mg/g). The lowest flavonoid content was found in 7.5%SCMC-92.5%BYF cookies (58.71 ± 0.36 mg/g) and is significantly comparable (p > 0.05) with 10%BEF-90%BYF (59.35 ± 0.54 mg/g) and 2.5%SCMC-97.5% BYF (59.48 ± 0.36 mg/g) cookies. Further, the total tannin contents of the cookies ranged from 3.12 ± 0.00 mg/g (100% CWF) to 4.87 ± 0.00 mg/g (2.5%BEF-97.5%BYF). The tannins contents of 100%BYF (3.70 ± 0.02 mg/g) were significantly (p < 0.05) higher than the 100% CWF (3.12 ± 0.00 mg/g). A similar trend has previously been reported for yellow maize and wheat flour by Irondi et al. (2021b). The total saponin level of 100% CWF cookies (1.02 ± 0.00 mg/g) was significantly (p < 0.05) lower than 100% BYF (1.04 ± 0.00 mg/g) cookies and the BEF-BYF cookies. The bioactive compounds (flavonoids, and tannins) found in the study are renowned for their positive impact on health. According to reports, the presence of these substances in gluten-free products may offer anti-obesity, anti-hypertensive, anti-diabetic, and antioxidant advantages (Bai et al., 2017; Irondi et al., 2018; Irondi et al., 2021a; Avila-Roman et al., 2021; Irondi et al., 2021b; Irondi et al., 2022) have reported that besides their health advantages, they may additionally prevent oxidative storage, prolong food shelf-life, and conserve food’s nutritional value.
3.2 Carotenoid contents of the cookies
The carotenoid profile of the cookies is shown in Table 2. Based on HPLC fingerprinting, the results indicated that all cookie samples had significant (p < 0.05) amounts of carotenoid. Specifically, lutein (range from 0.62 to 1.63 μg/g), β-carotene (range from 3.81 to 6.06 μg/g), β-cryptoxanthin (range from 0.19 to 1.75 μg/g), and zeaxanthin (range from 0.83 to 2.48 μg/g) were among the principal carotenoid. The cookies with 2.5%BEF-97.5% BYF had the lowest retinol activity (0.76 μg/g), whereas the cookies with 5%SCMC-95% BYF had the highest value (1.14 μg/g). The presence of carotenoids in the cookies suggests that the BYF-based cookies may have health benefits. Carotenoids have anti-oxidant and pro-vitamin A properties (Seifried et al., 2007; Elemosho et al., 2020); they guard against cancer, including prostate cancer (Agarwal and Rao, 2000; Elemosho et al., 2020); they prevent chronic diseases (Gammone et al., 2017) and type 2 diabetes (Sugiura et al., 2015), insulin resistance (Ni et al., 2015), and hyperglycemia associated with diabetic conditions (Elemosho et al., 2020).
3.3 Starch-digesting enzymes (alpha-amylase and alpha-glucosidase) inhibitory activity of the cookies
The alpha-amylase and alpha-glucosidase inhibitory activities of the cookies were presented in Table 3 as the concentrations of extracts of the cookies that caused 50% inhibition (IC50). The cookies of 100% BYF with IC50 values of 1.42 ± 0.01 μg/mL (alpha-amylase and alpha-glucosidase respectively) showed better inhibition against alpha-amylase and alpha-glucosidase than 100% CWF cookies (alpha-amylase, 2.63 ± 0.01 and alpha-glucosidase, 2.29 ± 0.02 μg/mL). A lower IC50 value shows stronger inhibitory activity against an enzyme (Irondi et al., 2021b; Irondi et al., 2022). Further, the IC50 value ranged from 1.42 ± 0.01 μg/mL (100% BYF) to 2.63 ± 0.01 μg/mL (100% CWF) for alpha-amylase and 1.12 ± 0.01 μg/mL (2.5%SCMC-97.5% BYF) to 6.72 ± 0.01 (7.5%BEF-92.5% BYF) for alpha-glucosidase. Among the composite cookies, 7.5%SCMC-92.5%BYF cookies (1.77 ± 0.00 μg/mL) have better inhibition against alpha-amylase while 2.5%SCMC-97.5% BYF (1.12 ± 0.01 μg/mL) has better inhibition against alpha-glucosidase.
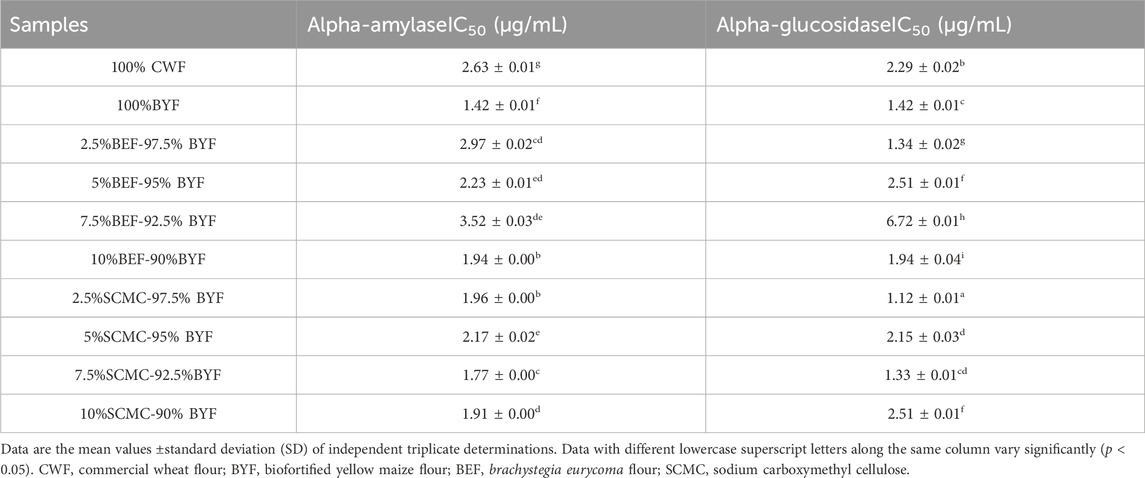
Table 3. Starch -digesting enzymes (alpha-amylase and alpha-glucosidase) inhibitory activity of the cookies.
The digestion of dietary carbohydrates, especially starch, is facilitated by both alpha-amylase and alpha-glucosidase (Kareem et al., 2022). Alpha-amylase catalyzes the first stage of the starch hydrolysis process, resulting in the production of maltose, dextrin, and maltotriose. After alpha-glucosidase hydrolyzes these products, absorbable monosaccharides (such as fructose and glucose) are produced (Irondi et al., 2019b; Kareem et al., 2022). As per Butterworth et al. (2022), the inhibition of α-amylase and α-glucosidase enzymes is strongly linked to a reduction in blood glucose response. This implies that inhibiting these enzymes can be a therapeutic method for managing Type-2 Diabetes by reducing postprandial hyperglycemia (Irondi et al., 2019b). Many anti-diabetes therapies (natural items, medications, and functional foods) work by inhibiting α-amylase and α-glucosidase and a meal that has this ability also has anti-hyperglycemic properties (Irondi et al., 2021b). The result therefore showed that the cookie samples had significant (p < 0.05) anti-hyperglycemic qualities and could be used as functional foods to treat obesity and type 2 diabetes.
3.4 Glycemic index of the cookies
Figure 4 shows the glycemic index (GI) of all the cookies at 0, 30, 60, and 90 min intervals. There was a significant (p < 0.05) increase in the blood glucose concentration within 30 min of cookies consumption. A peak level of blood glucose concentration was observed at 60 min. The 7.5%SCMC-92.5%BYF cookie (FO31) has the lowest GI mean value at 30 min time whereas, 7.5%BEF-92.5%BYF cookie (AO23) has the highest. The GI mean of 7.5%BEF-92.5%BYF cookies (AO23) significantly decreased (p < 0.05) after 30 min of consumption. According to Zhu (2019), GI values are determined by how quickly carbohydrates are metabolized. those with a slow pace of digestion are classified as low-GI (GI < 55), while those with a high rate of digestion (GI > 70) are classified as high-GI. All of the cookies fall into the low GI category because their average GI varied between10 to 45. High GI diets, such as those high in carbohydrates, are connected to several health challenges, including metabolic disorders and non-communicable diseases like hyperlipidemia, heart problems, obesity, cardiovascular diseases (CVDs), and diabetes (Zhu and Li, 2019; Gorenand et al., 2023). Since low-GI diets were not associated with these illnesses, it is possible that they can be used in place of high-GI foods to help manage these health issues (Butterworth et al., 2022; Haini et al., 2022). Therefore, the cookie samples with low GI values can be regarded as high-quality baked goods.
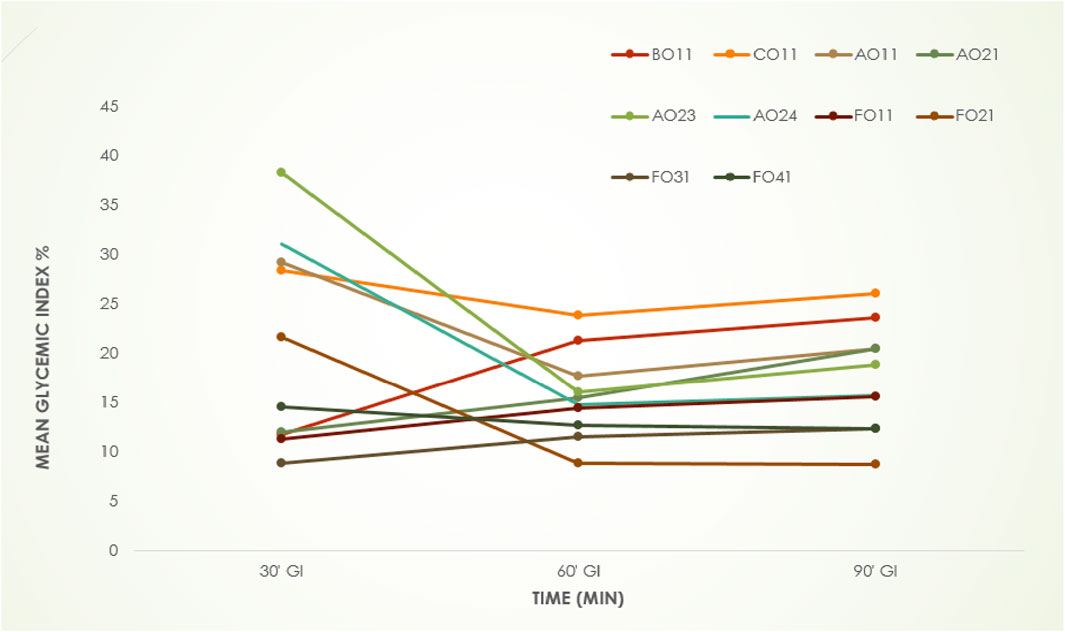
Figure 4. Glycemic index (GI) of the Cookies Keys: BO11 = 100%BYF, C011 = 100%WF, AO11 = 2.5%BEF-97.5%BYF, AO21 = 5%BEF-95%BYF, AO23 = 7.5%BEF-92.5%BYF, AO24 = 10%BEF-90%BYF, FO11 = 2.5%SCMC-97.5%BYF, FO21 = 5%SCMC-95%BYF, FO31 = 7.5%SCMC-92.5%BYF, FO41 = 10%SCMC-90% BYF CWF, commercial wheat flour, BYM, biofortified yellow maize flour; BEF, Brachystegia eurycoma flour, SCMC, sodium carboxymethyl cellulose.
4 Conclusion
A Biofortified yellow maize-based gluten-free cookie was produced from biofortified yellow maize flour (BYF). Additionally, the biofortified yellow maize flour (BYF) was combined with Brachystegia eurycoma flour (BEF) and sodium carboxyl methylcellulose (SCMC), a reference hydrocolloid, in four different amounts (2.5, 5, 7.5, and 10%), respectively. The resulting blends were then used to manufacture gluten-free cookies. Compared to conventional wheat flour cookies (100% CWF), the BYF (100%) and BYF-BEF cookies have a lower glycemic index, greater bioactive components and carotenoid concentrations, and superior starch-hydrolyzing enzyme inhibition. This implied that eating 100% BYF and BYF-BEF cookies may be beneficial to one’s health and that they may be used as a baked good to manage diseases like diabetes, heart disease, hyperlipidemia, hyperglycemia, obesity, and celiac disease: disorders linked to nutrition.
Data availability statement
The raw data supporting the conclusion of this article will be made available by the authors, without undue reservation.
Ethics statement
The studies involving humans were approved by the Kwara State Ministry of Health, Ilorin with approval number MOH/KS/EU/777/493. The studies were conducted in accordance with the local legislation and institutional requirements. The participants provided their written informed consent to participate in this study.
Author contributions
KA: Writing–original draft, Formal Analysis, Investigation. YI: Writing–original draft, Writing–review and editing. EI: Conceptualization, Supervision, Writing–review and editing. EA: Supervision, Writing–review and editing.
Funding
The author(s) declare that no financial support was received for the research, authorship, and/or publication of this article.
Conflict of interest
The authors declare that the research was conducted in the absence of any commercial or financial relationships that could be construed as a potential conflict of interest.
Publisher’s note
All claims expressed in this article are solely those of the authors and do not necessarily represent those of their affiliated organizations, or those of the publisher, the editors and the reviewers. Any product that may be evaluated in this article, or claim that may be made by its manufacturer, is not guaranteed or endorsed by the publisher.
References
Agarwal, L. S., and Rao, A. V. (2000). Carotenoids and chronic diseases. Drug Metabolism Drug Interact. 17, 189–210. doi:10.1515/DMDI.2000.17.1-4.189
Alamu, E. O., Maziya-Dixon, B., Menkir, A., and Olaofe, O. (2018). Bioactive compounds of freshly harvested open pollinated varieties (OPV) of orange maize (Zea mays): varietal, maturity, and boiling methods effects. Cogent Chem. 4, 1507489. doi:10.1080/23312009.2018.1507489
Amorim, E. L. C., Nascimento, J. E., Monteiro, J. M., Sobrinho, P., Araujo, T. A. S., and Albuquerque, U. A. P. (2008). A simple and accurate procedure for the determination of tannins and flavonoid levels and some applications in ethnobotany and ethnopharmacology. Funct. Ecosyst. Communities 2 (1), 88–94.
Avila-Roman, J., Soliz-Rueda, J. R., Bravo, F. I., Aragones, G., Suarez, M., Arola-Arnal, A., et al. (2021). Phenolic compounds and biological rhythms: who takes the lead? Trends Food Sci. Technol. 113, 77–85. doi:10.1016/j.tifs.2021.04.050
Bai, Y., Xu, Y., Wang, B., Li, S., Guo, F., Hua, H., et al. (2017). Comparison of phenolic compounds, antioxidant and antidiabetic activities between selected edible beans and their different growth periods leaves. J. Funct. Foods 35, 694–702. doi:10.1016/j.jff.2017.06.036
Bashir, S., Yaseen, M., Sharma, V., Purohit, S. R., Barak, S., and Mudgil, D. (2020). Rheological and textural properties of gluten-free cookies based on pearl millet and flaxseed. Biointerface Res. Appl. Chem. J. 10 (5), 6565–6576. doi:10.33263/BRIAC105.65656576
Beta, T., and Hwang, T. (2018). Influence of heat and moisture treatment on carotenoids, phenolic content, and antioxidant capacity of orange maize flour. Food Chem. 246, 58–64. doi:10.1016/j.foodchem.2017.10.150
Butterwort, P. J., Bajka, B. H., Edwards, C. H., Warren, F. J., and Ellis, P. R. (2022). Enzyme kinetic approach for mechanistic insight and predictions of in vivo starch digestibility and the glycaemic index of foods. Trends food Sci. Technol. 120, 254–264. doi:10.1016/j.tifs.2021.11.015
Chan, E. W. C., Lim, Y. Y., and Chew, Y. L. (2007). Antioxidant activity of Camellia sinensis leaves and tea from a lowland plantation in Malaysia. Food Chem. 102, 1214–1222. doi:10.1016/j.foodchem.2006.07.009
Dhankhar, P. (2013). A study on development of coconut based gluten free cookies. Int. J. Eng. Sci. Invent. 2, 10–19.
Di Cairano, M., Galgano, F., Tolve, R., Caruso, M. C., and Condelli, N. (2018). Focus on gluten free biscuits: ingredients and issues. Trends Food Sci. Technol. 81, 203–212. doi:10.1016/j.tifs.2018.09.006
Elemosho, A. O., Irondi, E. A., Alamu, E. O., Ajani, E. O., Maziya-Dixon, B., and Menkir, A. (2020). Characterization of Striga-resistant yellow-orange maize hybrids for bioactive, carbohydrate, and pasting properties. Front. Sustain. Food Syst. 4, 585865. doi:10.3389/fsufs.2020.585865
Elif, Y., and Duygu, G. (2020). Use of almond flour and stevia in rice-based gluten-free cookie production. J. Food Sci. Technol. 58, 940–951. doi:10.1007/s13197-020-04608-x
FAO/WHO (1998). “Carbohydrates in human nutrition,” in Report of a joint FAO/WHO expert consultation, Rome, 14-18 april 1997. FAO food and nutrition paper no.66 (Rome: FAO).
Fouad, A. A., and Rehab, F. M. (2013). Bioactive compounds and antioxidant activity of fresh and processed white cauliflower. Bio Med. Res. Int. 9. doi:10.1155/2013/367819
Gammone, M. A., Pluchinotta, F. R., Bergante, S., Tettamanti, G., and D’Orazio, N. (2017). Prevention of cardiovascular diseases with carotenoids. Front. Biosci. 9, 165–171. doi:10.2741/s480
Gorenand, P. Y., Chandrakant, G. D., and Hari, N. M. (2023). Preparation of low glycemic rice and comparison of its physicochemical properties, cooking characteristics, starch digestibility and microstructure with raw rice (swarna cv). Food Sci. Eng. 4 (1), 30–43. doi:10.37256/fse.4120231882
Haini, N., Jau-Shya, L., RamlahRosli, G. M., and Mama, H. (2022). Effects of high-amylose maize starch on the glycemic index of Chinese steamed buns (CSB). Heliyon 8 (2022), e09375. doi:10.1016/j.heliyon.2022.e09375
Howe, J. A., and Tanumihardjo, S. A. (2006). Evaluation of analytical methods for carotenoid extraction from biofortified maize (Zea mayssp). J. Agric. Food Chem. 54, 7992–7997. doi:10.1021/jf062256f
Imam, Y. T., Irondi, E. A., Awoyale, W., Ajani, E. O., and Alamu, E. O. (2024). Application of legumes in the formulation of gluten-free foods: functional, nutritional and nutraceutical importance. Front. Sustain. Food Syst. 8, 1251760. doi:10.3389/fsufs.2024.1251760
Irondi, A. E., Oboh, G., and Akindahunsi, A. A. (2015). Methanol extracts of Brachystegia eurycoma and Detarium microcarpum seeds flours inhibit some key enzymes linked to the pathology and complications of type 2 diabetes in vitro. Food Sci. Hum. Wellness 4, 162–168. doi:10.1016/j.fshw.2015.08.002
Irondi, E. A., Adegoke, B. M., Effion, E. S., Oyewo, S. O., Alamu, E. O., and Boligon, A. A. (2019a). Enzymes inhibitory property, antioxidant activity and phenolics profile of raw and roasted red sorghum grains in vitro. Food Sci. Hum. Wellness 8, 142–148. doi:10.1016/j.fshw.2019.03.012
Irondi, E. A., Agboola, S. O., and Boligon, A. A. (2018). Inhibitory effects of tropical almond leaf extract on xanthine oxidase, pancreatic lipase, and angiotensin I- converting enzyme, in vitro. J. Food Biochem. 42, e12481. doi:10.1111/jfbc.12481
Irondi, E. A., Ajani, E. O., Aliyu, O. M., OlatoyeAbdulameed, K. K. H. T., and Ogbebor, O. F. (2021b). Bioactive components, enzymes inhibitory and antioxidant activities of biofortified yellow maize (zea mays L.) and cowpea (vigna unguiculata L. Walp) composite biscuits. Ann. Univ. Dunarea de Jos Galati Fascicle VI – Food Technol. 45 (1), 86–101. doi:10.35219/foodtechnology.2021.1.06
Irondi, E. A., Imam, Y. T., and Ajani, E. O. (2021a). Effect of Brachystegia eurycoma flour addition on the physicochemical properties of whole millet flour and the sensory attributes of its gluten-free bread. Acta Univ. Cibiniensis Ser. E Food Technol. 43 (1), 43–52. doi:10.2478/aucft-2021-0004
Irondi, E. A., Imam, Y. T., and Ajani, E. O. (2022). Physicochemical, antioxidant and starch-digesting enzymes inhibitory properties of pearl millet and sweet detar gluten-free flour blends, and sensory qualities of their breads. Front. Food Sci. Technol. 2, 974588. doi:10.3389/frfst.2022.974588
Irondi, E. A., Imam, Y. T., Ajani, E. O., and Alamu, E. O. (2023). Natural and modified food hydrocolloids as gluten replacement in baked foods: functional benefits. Grain Oil Sci. Technol. 6, 163–171. doi:10.1016/j.gaost.2023.10.001
Irondi, E. A., Ogunsanmi, A. O., Ahmad, R. S., Ajani, E. O., Adegoke, B. M., and Boligon, A. A. (2019b). Effect of roasting on phenolics composition, enzymes inhibitory and antioxidant properties of cowpea pulses. J. Food Meas. Charact. 13, 1489–1496. doi:10.1007/s11694-019-00064-0
Jnawali, P., Kumar, V., and Tanwar, B. (2016). Celiac disease: overview and considerations for development of gluten-free foods. Food Sci. Hum. Wellness 5, 169–176. doi:10.1016/j.fshw.2016.09.003
Kale, A., Gaikwad, S., Mundhe, K., Deshpande, N., and Salvekar, J. (2010). Quantification of phenolics and flavonoids by spectrophotometer from. Juglansregia. Int. J. Pharm. Biol. Sci. 1, 1–4.
Kareem, B., Irondi, E. A., Alamu, E. O., Ajani, E. O., Abass, A., Adesokan, M., et al. (2022). Influence of traditional processing and genotypes on the antioxidant and anti-hyperglycaemic activities of yellow-fleshed cassava. Front. Nutr. 9, 894843. doi:10.3389/fnut.2022.894843
Kim, Y. M., Jeong, Y. K., Wang, M. H., Lee, Y. H., and Rhee, H. I. (2005). Inhibitory effect of pine extract on alpha-glucosidase activity and postprandial hyperglycemia. Nutrition 21, 756–761. doi:10.1016/j.nut.2004.10.014
Kwon, Y. I., Apostolidis, E., and Shetty, K. (2008). Inhibitory Potential Of Wine And Tea Against Α-Amylase And Α-Glucosidase For Management Of Hyperglycemia Linked To Type 2 Diabetes. J. Food Biochem. 32, 15–31. doi:10.1111/j.1745-4514.2007.00165.x
Makkar, H. P. S., Siddhuraju, P., and Becker, K. (2007) Plant secondary metabolites. Totowa, NJ, USA: Humana Press Inc. doi:10.1007/978-1-59745-425-4
Manzoor, M., Ahmad, M., Bandral, J. D., Gani, A., Singh, J., and Shams, R. (2020). Food hydrocolloids: functional, nutraceutical and novel applications for delivery of bioactive compounds. Int. J. Biol. Macromol. 165 (Part A), 554–567. doi:10.1016/j.ijbiomac.2020.09.182
Ni, Y., Nagashimada, M., Zhan, L., Nagata, N., Kobori, M., Sugiura, M., et al. (2015). Prevention and reversal of lipotoxicity-induced hepatic insulin resistance and steatohepatitis in mice by an antioxidant carotenoid, β-cryptoxanthin. Endocrinol 156, 987–999. doi:10.1210/en.2014-1776
Nwosu, J. N. (2012). The rheological and proximate properties of some food thickeners (“Ukpo”, “achi” and “ofo”) as affected by processing. Int. J. Basic Appl. Sci. 1 (4), 304–312. ISSN: 2277-1921.
Okaka, J. C., and Isieh, M. I. (1990). Development and quality evaluation of cowpea-wheat biscuit. Niger. food J. 8, 56–62.
Seifried, H. E., Anderson, D. E., Fisher, E. I., and Milner, J. A. (2007). A review of the interaction among dietary antioxidants and reactive oxygen species. J. Nutr. Biochem. 18, 567–579. doi:10.1016/j.jnutbio.2006.10.007
Sethiya, N. K., Trivedi, A., and Mishra, S. (2014). The total antioxidant content and radical scavenging investigation on 17 phytochemical from dietary plant sources used globally as functional food. Biomed. Prev. Nutr. 4, 439–444. doi:10.1016/j.bionut.2014.03.007
Siwela, M., Pillay, K., Govender, L., Lottering, S., Mudau, F. N., Modi, A. T., et al. (2020). Biofortified crops for combating hidden hunger in South Africa: availability, acceptability, micronutrient retention and bioavailability. Foods 9 (6), 815. doi:10.3390/foods9060815
Sugiura, M., Nakamura, M., Ogawa, K., Ikoma, Y., and Yano, M. (2015). High serum carotenoids associated with lower risk for developing type 2 diabetes among Japanese subjects: mikkabi cohort study. BMJ Open Diabetes Res. Care 3, e000147. doi:10.1136/bmjdrc-2015-000147
Susman, I., Schimbator, M., Culețu, A., and Popa, M. E. (2021). Formulation of gluten-free cookies with enhanced quality and nutritional value. Bull. Univ. Agric. Sci. Veterinary Med. Cluj-Napoca Food Sci. Technol. 78 (1), 113–121. doi:10.15835/buasvmcn-fst:2020.0046
Xu, J., Zhang, Y., Wang, W., and Li, Y. (2020). Advanced properties of gluten-free cookies, cakes, and crackers: a review. Trends Food Sci. Technol. 103, 200–213. doi:10.1016/j.tifs.2020.07.017
Yousaf, M. I., Hussain, K., Hussain, S., Ghani, A., Shehzad, A., Mumtaz, A., et al. (2020). Seasonal influence, heat unit accumulation and heat use efficiency in relation to maize grain yield in Pakistan. Maydica 64 (3), 9.
Zhu, F. (2019). Glycemic control in Chinese steamed bread: strategies and opportunities. Trends Food Sci. Technol. 86, 252–259. doi:10.1016/j.tifs.2019.02.038
Zhu, F., and Li, J. (2019). Physicochemical properties of steamed bread fortified with ground linseed (Linumusitatissimum). Int. J. Food Sci. Technol. 54, 1670–1676. doi:10.1111/ijfs.14043
Keywords: biofortified yellow maize, carotenoid, bioactive compound, gluten-free cookies, Brachystegia eurycoma flour
Citation: Abdulrazaaq KO, Imam YT, Irondi EA and Ajani EO (2024) Bioactive composition and anti-hyperglycemic properties of biofortified yellow maize-based gluten-free cookies. Front. Food. Sci. Technol. 4:1365021. doi: 10.3389/frfst.2024.1365021
Received: 03 January 2024; Accepted: 26 April 2024;
Published: 22 May 2024.
Edited by:
Rosa Perestrelo, Universidade da Madeira, PortugalReviewed by:
Monika Sujka, University of Life Sciences of Lublin, PolandShonisani Eugenia Ramashia, University of Venda, South Africa
Copyright © 2024 Abdulrazaaq, Imam, Irondi and Ajani. This is an open-access article distributed under the terms of the Creative Commons Attribution License (CC BY). The use, distribution or reproduction in other forums is permitted, provided the original author(s) and the copyright owner(s) are credited and that the original publication in this journal is cited, in accordance with accepted academic practice. No use, distribution or reproduction is permitted which does not comply with these terms.
*Correspondence: Yunus Temitayo Imam, eXVzaW1hdDEyMTJAZ21haWwuY29t; Emmanuel Anyachukwu Irondi, ZW1tYW51ZWwuaXJvbmRpQGt3YXN1LmVkdS5uZw==