- 1LEAF (Linking Landscape Environment Agriculture and Food) Research Center, Associate Laboratory TERRA, Instituto Superior de Agronomia, Universidade de Lisboa, Lisbon, Portugal
- 2Department of Chemical Engineering, Science Faculty, Universidade de Vigo (Campus Ourense), Ourense, Spain
The aim of the present work was to study the potential health benefits of 3D snacks enriched with increasing levels of incorporation of C. vulgaris (2%–18% w/w). Health impact was evaluated based on the nutritional profile of snacks, as well as protein digestibility, mineral bioaccessibility, glycemic index and antioxidant activity. Higher additions of Chlorella vulgaris to snack’s formulation led to obtain a new food product with elevated nutritional properties, that is a source of protein and minerals, that presents a lower glycemic index, a boosted antioxidant activity and an enhanced mineral bioaccessibility. Despite substantial evidence on the health benefits of microalgae-derived food products, functional food value of algae products remains largely qualitative. The present work contributed to provide insights on bioaccessibility of nutritional compounds of a microalgae food—3D snack–aiming to provide a more sustainable healthy food choice.
1 Introduction
Microalgae are photosynthetic unicellular microorganisms that transform into organic bioactives large quantities of CO2, supply about half of the atmospheric oxygen to the planet and can adapt to different cultivation conditions. Furthermore, they produce twenty times more protein per ha than other common crops such as corn and even soybeans (Loke, 2022). In addition to offering solutions for a high in demand sustainability-driven new food production systems, microalgae have increasingly received attention since they present a valuable nutritional composition with potential health benefits, including long-term chronic diseases like cancer (Batista et al., 2019; Cotas et al., 2021). These include high-value proteins with a balanced amino acid composition, long-chain polyunsaturated fatty acids, pigments, vitamins, minerals and phenolic compounds (Wells et al., 2017; Pina-Pérez et al., 2019).
The global microalgae products market size is expected to reach USD 1485.1 million by 2028, according to an Allied Market Research report (Kumar and Deshmukh, 2021). Key companies in the microalgae market framework have adopted a competitive investment in microalgae-based food products research and development, leading to a boom in new microalgae food products launch in the market. Many recent studies have shown that microalgae can be used to enrich different food applications (Torres and González, 2019; Bhattacharya et al., 2021). Regarding cereal-based food products, microalgae biomass have been incorporated into products such as bread (Ak et al., 2016; García-Segovia et al., 2017; Nunes et al., 2020), cookies (Babuskin et al., 2014; Batista et al., 2017; Bolanho et al., 2014; Hossain et al., 2017; Şahin, 2020; Z. N; Uribe-Wandurraga et al., 2020a; Vieira et al., 2020), biscuits (Gouveia et al., 2008), snacks (Lucas et al., 2018; Z. N; Uribe-Wandurraga et al., 2020c), crackers (Batista et al., 2019), breadsticks (Z. N. Uribe-Wandurraga et al., 2019), crostini (Niccolai et al., 2019) and pasta (Bandarra et al., 2010; Fradique et al., 2013; Babuskin et al., 2014; Fradinho et al., 2020). In particular, snacks are considered a convenient on-the-go type of food product, that can address and adapt to different lifestyles, while still being a potential promotor for heathy-based diets as the snacking market has been evolving to meet consumers’ demands for more healthy food options (Z. N. Uribe-Wandurraga et al., 2020c). As consumers become more health-conscious, the need for nutritional, yet delicious, products has been booming. Inclusion of microalgae in food products, namely, bakery products, requires an optimization of technological properties of the products as microalgae immensely affect sensory parameters, notably texture and taste (Batista et al., 2017; C; Graça et al., 2018; Niccolai, et al., 2019b; Şahin, 2020; Vieira et al., 2020). Previous studies on incorporation of microalgae in bakery products suggest that low incorporations are generally well accepted by consumers (Batista et al., 2017; Şahin, 2020). On the other hand, authors reported that sensory properties are adversely affected when microalgae additions exceed 5% (w/w) (Bolanho et al., 2014; Singh et al., 2015). In this sense, 3D food printing (3DFP), a promising technology to offer differentiated and innovative food products, can excite customers, help overcoming possible aversion to algae and contribute to boost desirability of algae-based foods.
Many studies report the potential nutritional or bioactive content of different algae food; however, few quantify the bioavailability of its nutrients. This study aimed to assess the health impact of increasing incorporations of Chlorella vulgaris in 3D snacks. For this purpose, chemical and amino acid composition, antioxidant activity, in vitro starch and protein digestibility and bioaccessibility of minerals and antibacterial activity were determined.
2 Material and methods
2.1 Raw materials and 3D printing of snacks
Chlorella vulgaris was produced and provided by A2F (https://www.nibio.no/en/projects/algae-to-future-a2f) partners in NORCE/UiB (Bergen, Norway). Table 1 presents the formulations of snacks with increasing incorporations of C. vulgaris (2%—18% w/w), by replacing whole meal oat flour and corn starch with additions of C. vulgaris. Ingredients included in the snack’s formulation (except C. vulgaris biomass) were purchased from a local supermarket.
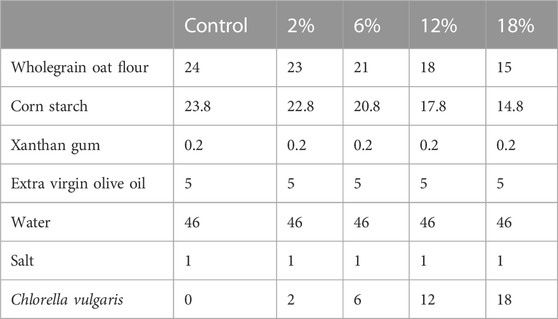
TABLE 1. Snacks’ formulations (% w/w). Replacement of wholegrain oat flour and corn starch by increasing amounts of Chlorella vulgaris.
Preparation of snacks was performed according to procedures described by (Oliveira et al., 2022). Briefly, ingredients were mixed until homogeneous doughs were obtained. After mixing, doughs were let to rest for 15 min, at room temperature, and then transferred to printing capsules.
Printing was performed at conditions mentioned by Oliveira et al. (2022). After printing, snacks were baked (15 min, at 170°C) and cooled down at room temperature to be finally stored under vacuum, at - 18°C, until further analysis.
2.2 Proximate chemical composition
Moisture content of snacks was determined gravimetrically, by oven drying at 105°C until constant weight (AACC International, 1999). Total ash content was determined gravimetrically by incineration, for approximately 24 h, at 550°C, in a muffle furnace (AACC International, 2009a). Crude protein was determined by the DUMAS method (NDA 702 Dual Carrier Gas Dumas Nitrogen Analyzer, Velp Scientifica). For the DUMAS method 100 mg of dry samples were wrapped and tightly pelleted in tin foil cups. Samples were individually transferred into the combustion chamber (1,030°C) and burnt in the presence of catalysts, in a controlled oxygen atmosphere (1.6 mL of O2 per mg of sample and correspondent rate of 400 mL/min). The combustion gas NOx passed through the reduction furnace, where was reduced to N2, being finally quantified with a thermal conductivity detector. EDTA was used as the nitrogen calibration standard. The nitrogen content was multiplied by a conversion factor of 6.25 (baked and digested snacks) and 4.78 (microalga) to obtain the protein content (Tibbetts et al., 2015). Crude fat content was determined by the Soxhlet method (AACC International, 2009b). Total and resistant starch were determined according to methods described by Goni et al. (1997). Carbohydrates were calculated by difference.
Mineral profile was determined following a method previously described, by Inductively Coupled Plasma - Optical Emission Spectrometry (Thermo Scientific™ iCAP 7,000 Series) (Martins et al., 2020).
Amino acids profile of snacks was analyzed according to the methods described by Cabrol et al. (2022). Cysteine and methionine were previously oxidized to cysteic acid and methionine sulphone, respectively, before hydrolysis. All the other amino acids, except tryptophane, were analyzed as hydrolysates of unoxidized samples. Reverse-phase HPLC (Agilent 1,100 Series, Palo Alto, CA, United States) analysis was performed by fluorimetric detection (Waters Assoc., Milford, MA, United States) after automatic precolumn derivatization by treatment with o-phthalaldehyde for primary amino acids and 9-fluorenylmethyl chloroformate for secondary amino acids. A mixture of norvaline and sarcosine (20 mM) was used as an internal standard. Separation was performed at 40°C using a gradient between two solvents: 40 mM sodium phosphate at pH 7.8 (solvent A) and a solution of acetonitrile:methanol:water (45:45:10 v/v) (solvent B). Amino acids determination was conducted at the flow rate of 2 mL/min and the eluted derivatives were detected by monitoring their fluorescence signal at 450 and 340 nm for emission and excitation, respectively (Cabrol et al., 2022).
Forms of vitamin E (tocotrienols and tocopherols) were extracted following a method involving direct saponification, a single n-hexane extraction, and HPLC analysis using fluorescence (excitation wavelength of 295 nm and emission wavelength of 325 nm) (Prates et al., 2006). Total energy content was calculated according to EU Council Directive 1,169/2011 (The European Parliment and the Council of the European Union, 2011).
All measurements were conducted in triplicate for each sample. Results are presented as average ±standard deviation.
For the determination of the amino acids profile and forms of vitamin E, only snacks with 2% and 6% C. vulgaris incorporation were analyzed due to technical limitation of the laboratory at the time.
2.3 In vitro digestion and bioaccessibility
The in vitro digestion protocol employed in this article was based on the Infogest model (Brodkorb et al., 2019). Enzymes’ activities were previously determined for alpha-amylase (EC 3.2.1.1, Sigma), pepsin (EC 3.4.23.1), pancreatin (EC 3.4.21.4) and bile salts (total bile acids kit, DiaSys). Briefly, for the oral phase, 1 g of each snack was moistened with 1 mL of water. Samples were incubated with simulated salivary fluid (SSF) (1:1, (wt/wt)) and 100 U/mg alpha-amylase (A6814, Sigma-Aldrich) during 2 min, at 37°C, with continuous agitation (100 rpm). For gastric phase, oral bolus was diluted 1:1 (v/v) with simulated gastric fluid (SGF) and 3000 U/mg pepsin (P6887, Sigma-Aldrich). For the small intestine phase, the chyme was diluted 1:1 (v/v) with pre-warmed simulated intestinal fluid (SIF). Pancreatin (P7545, Sigma-Aldrich) with trypsin activity of 6 U/mg and 0.667 mmol/g bile salts (B3883, Sigma-Aldrich) were added to the chyme and samples were further incubated for 120 min, at 37°C, pH 7. For pH corrections, HCl (1 M) and NaOH (1 M) were used. The whole digestion protocol was performed at 37°C under constant mixing using an overhead shaker (Hei-MIX Reax 2, Heidolph, Germany). Digestion was stopped after 120 min of the intestinal phase using the enzyme inhibitor Pefabloc SC (76,307, Sigma-Aldrich). Immediately after stopping digestion, all the samples were frozen for 24 h. After unfreezing, centrifuged samples were separated (6,000 rpm, at 4°C, for 10 min) into soluble and insoluble fractions by collecting the supernatant and the pellet (residue), respectively. Bioaccessibility of digested samples was performed for mineral and protein content, following the methods previously described (Section 2.2) for biochemical composition of samples. Analyses were run in duplicates.
2.3.1 Calculations
For the in vitro protein digestibility (IVPD), the amount of total dissolved protein of each sample was calculated by multiplying the protein concentration (obtained from the Dumas method) with the total liquid volume of each sample (sample moisture content, total sum of simulated fluids and total liquid volume of HCl/NaOH spent on pH adjustments) before subtracting the protein contribution of the blank. The amount of insoluble protein for each sample was calculated from the weight and protein content of the respective pellets.
Protein digestibility and mineral bioaccessibility were calculated based on equations used by Cabrol et al. (2022):
Where W0 is the protein content (mg) of snacks before digestion and W1 is protein content (mg) of snacks in the residue, after removing blank, after digestion.
Where BC is the bioaccessible (i.e., supernatant collected after digestion) mineral fraction of snacks, after removing blank, and TC is the total mineral content of snacks, before digestion.
Soluble protein fraction, insoluble protein fraction and protein recovery content were calculated as explained by Cabrol et al. (2022).
2.4 Total starch, in vitro starch digestibility and predicted glycemic index
2.4.1 Total starch
Total starch was determined according by methods described by Goni et al. (1997). Briefly, 100 mg of snack were treated with 1 mL of aqueous ethanol (80% v/v) to remove the free sugars, followed by the addition of 2 mL of 2 M KOH to dissolve the resistant starch, at 4°C, for 30 min, with continuous shaking at 100 rpm (Hei-MIX Reax 2, Heidolph, Germany). Subsequently, 3 mL of 0.1 M Tris-maleate buffer (pH 6.9) and 1 mL of 5 U/mg pancreatic alpha-amylase (A3176, Sigma-Aldrich) were added to start first incubation (37°C, 45 min). Alfa-amylase enzymatic activity was previously determined according to the method described in Sigma (EC 3.2.1.1). To hydrolyze digested starch into glucose, 3 mL of 0.1 M sodium acetate buffer (pH 4.75) and 60 μL of 3300 U/mL amyloglucosidase (E-AMGDF, Megazyme) were added and samples were incubated (60°C, 45 min). Amyloglucosidase enzymatic activity was previously determined according to the method described by Sigma (EC 3.2.1.3). After incubation sample aliquots of 1 mL were taken and centrifuged at 10,000 rpm for 15 min. Total starch was spectrophotometric (540 nm) determined through the reducing sugars DNS (3,5-dinitrosalicyclic) assay, using maltose as standard.
2.4.2 Resistant starch
Resistant starch was determined according to the methodology described by Goni et al. (1997). Samples (100 mg) were incubated (37°C, 60 min) with 10 mL of HCl–KCl buffer (pH 1.5) and 2000 U/mL pepsin solution (P6887, Sigma-Aldrich). Subsequently, 200 U/mg pancreatic α-amylase (A6814, Sigma-Aldrich) was added and incubated (37°C, 16 h) to perform starch hydrolysis. After hydrolysis, the pellet was isolated by centrifugation and further subjected to digestion with 4 M KOH (Goñi et al., 1997). This solution was incubated (60°C, 45 min) with 80 µL of 3300 U/mL amiloglucosidase to hydrolyze the remaining resistant starch to glucose. Resistant starch was determined according to the DNS method as previously described for total starch.
2.4.3 In vitro starch digestibility and predicted glycemic index
In vitro starch digestibility was determined by the method described by Germaine et al. (2008). Briefly, the pH of samples of each snack containing 1 g of total starch was adjusted to 6.9.110 U pancreatic alpha-amylase (A3176, Sigma-Aldrich) was added, and the volume was made up to 100 mL with 0.05 M sodium potassium phosphate buffer (pH 6.9). Samples were incubated at 37°C with constant shaking at 100 rpm. Sample aliquots (1 mL) were taken at different times (0, 5, 10, 20, 30, 60, 90, 120 and 180 min) to Eppendorf tubes. To inactivate the enzyme tubes were placed in a boiling water bath for 5 min. Aliquots were centrifuged (10,000 rpm for 15 min) and supernatant was mixed with the DNS reagent to determine the reducing sugars.
2.4.4 Calculations
Starch hydrolysis rate was expressed as the percentage of total starch hydrolyzed at different times (0, 5, 10, 20, 30, 60, 90, 120, and 180 min). Digestible starch was classified as Rapidly digestible starch (RDS), defined as the percentage of starch digested at 30 min, and Slowly Digestible Starch (SDS), defined as the percentage of starch digested at 120 min, were determined according to methodology employed by Englyst et al. (1992).
The area under each of the hydrolysis curves (AUC) was calculated for each sample. The hydrolysis index (HI), that represents the rate of starch digestion, was obtained by dividing the AUC of each sample by the AUC of a reference sample (wheat bread). The average AUC of control wheat bread (CWB) was given an HI = 100. Finally, based on the linear relation between hydrolysis index (HI) and glycemic index (GI), the predicted glycemic index (pGI) was estimated according to an Equation 3 proposed by Goñi et al. (1992):
2.5 Total phenolics, antioxidant activity and pigments
2.5.1 Extracts preparation
To prepare the extracts snacks were processed into powder and sieved (Fritsch pulverisette 14, 0.5 mm diameter). Powdered samples were lyophilized (48 h, −58°C, 0.002 mbar) and stored under vacuum until further analysis.
The powdered samples (1 g of snacks and 1 g of lyophilized C. vulgaris biomass) were mixed with 10 mL of ethanol (96%). Before extraction, samples were homogenized (Ika® ultra-turrax® T-25 basic), at 8,000 rpm, for 2 min and placed in an ultrasonic water bath (Elma Transsonic 700), at 35 kHz, for 10 min.
Samples were extracted at room temperature, at 150 rpm, for 2 h, in a circulating water bath (Thermo Scientific Precision 2,864), based on the procedure described by Barreira et al. (2014). The extracts were centrifuged, and supernatants were stored (4°C). The residue was then re-extracted three additional times with equal portions (10 mL) of ethanol. The combined supernatants were filtered through syringe disk filters (0.20 μm pore size) and evaporated, at 35°C (rotavapor, R-200; Büchi, Flawil, Switzerland), to remove the solvent (Barreira et al., 2014). The dry extracts were then re-dissolved in DMSO to obtain stock solutions, prepared according to the yield of the dry extracts. Stock solutions’ concentrations were set at 15 mg/mL for snacks’ extracts and 1 mg/mL for C. vulgaris’ extract, to determine bioactive compounds, antioxidant activity and antibacterial activiy.
2.5.2 Total phenolic compounds
Total phenolic compounds (TPC) of extracts were determined by the Folin–Ciocalteu colorimetric method, based on the procedure described by Mohankumar et al. (2018). In brief, 150 µL of sample’s extract was mixed with 2.4 mL of distilled water, 150 µL of 12.5% (v/v) Folin-Ciocalteu reagent and, after 3 min, 300 µL of 10% (w/v) Na2CO3 was added. Water was used as blank. Absorbances were recorded after 2 h of incubation, in the dark, at room temperature, at 725 nm (Agilent Cary 100 UV-Visible spectrophotometer) (Mohankumar et al., 2018). TPC values were calculated from the calibration curve for gallic acid (0–250 μg/mL) and expressed as mg of gallic acid equivalents per Gram of dry extract (mg GAE/g DE).
2.5.3 Antioxidant activity
The antioxidant activity was determined for snack’s extracts (2.5.1), based on free-radical scavenging effect against 1,1-diphenyl-2-picrylhydrazyl (DPPH) and 2,2′-azino-bis-(3-ethylbenzothiazoline-6-sulfonic acid (ABTS) and on Fe3+ reducing power (FRAP). All antioxidant activity measurements were carried out in triplicate and presented as average ±standard deviation.
2.5.3.1 Radical-scavenging activity
For the DPPH assay, based on procedure described by Brand-Williams et al. (1995), 100 µL of sample’s extract were mixed with 3.9 mL of 0.06 mM DPPH solution. Methanol was used as a blank, while methanol with DPPH solution was used as control. Absorbances were recorded after 1 h of incubation, in the dark, at room temperature, at 515 nm (Agilent Cary 100 UV-Visible spectrophotometer) (Brand-Williams et al., 1995). DPPH values were calculated from the calibration curve for ascorbic acid (0–125 μg/mL) and expressed as mg of ascorbic acid equivalents per Gram of dry extract (mg AAE/g DE).
For the ABTS assay, based on procedure described by Re et al. (1999), 30 µL of sample’s extract was mixed with 3 mL of 7 mM ABTS solution, previously prepared: 5 mL of 7 mM ABTS stock solution was mixed with 88 µL of 140 mM potassium persulfate, stored in the dark, at room temperature, for 16 h. Final ABTS solution was then diluted with ethanol until a final absorbance of 0.7 was obtained at 734 nm. Ethanol was used as a blank, while ethanol with ABTS solution was used as control. Absorbances were recorded after 6 min of incubation, in the dark, at room temperature, at 734 nm (Agilent Cary 100 UV-Visible spectrophotometer) (Re et al., 1999). ABTS values were calculated from the calibration curve for ascorbic acid (0–250 μg/mL) and expressed as mg of ascorbic acid equivalents per Gram of dry extract (mg AAE/g DE).
2.5.3.2 Ferric-reducing ability of plasma
FRAP assay was determined according to procedure described by Benzie and Strain (1996) with some modifications. FRAP reagent was prepared freshly by mixing sodium acetate buffer (300 mM, pH 3.6), 10 mM TPTZ in 40 mM HCl and 20 mM FeCl3•6H2O solution, in a proportion of 10:1:1 (v/v/v), respectively. Ninety μL of sample’s extracts were added to 270 μL of distilled water and 2.7 mL of working FRAP reagent. Water was used as a blank. Absorbances were recorded after 30 min of incubation, at 37°C, in the dark, at 595 nm (Agilent Cary 100 UV-Visible spectrophotometer) (Benzie and Strain, 1996). FRAP values were calculated from the calibration curve for ascorbic acid (0–75 μg/mL) and expressed as mg of ascorbic acid equivalents per Gram of dry extract (mg AAE/g DE).
2.5.4 Pigments
Content of chlorophylls and carotenoids were estimated spectrophotometrically, based on the maximum absorbances of chlorophyll a (Chla), chlorophyll b (Chlb) and total carotenoids (TC), found at the wavelengths of 664, 648 and 470 nm, respectively (Lichtenthaler and Buschmann, 2001). Content of pigments was calculated according to Eqs (4)–(6), described by Lichtenthaler, as follows:
Content of chlorophyll a (Chla) and b (Chlb) is expressed as total chlorophyll. Results were expressed as mg per Gram of dry extract (mg/g DE).
2.6 Antibacterial activity
Antibacterial activity of baked snacks and C. vulgaris raw biomass was performed according to the disc diffusion method with the selected bacteria (Salmonella typhimurium, Escherichia coli, Staphylococcus aureus, Bacillus cereus and Lactiplantibacillus plantarum). Extracts of samples were prepared according to procedure described in section 2.5.1. (Extracts preparation), except for the resuspension of dry extracts, which was performed with ethanol, in place of DMSO, to avoid inhibiting bacterial growth. The stock cultures (106 CFU) were inoculated by spreading over the Mueller-Hinton Agar. Sterile filter paper discs (0.6 cm in diameter) were loaded 3 times with 10 µL of the extracts to achieve the concentration of 3 mg per disc and then placed on the inoculated plates using sterile forceps. Chloramphenicol (10 μg per disc) was used as a positive control, while ethanol was used as a negative control. The plates were incubated for 24 h, at 25°C, and the inhibition zone was measured in centimeters (Jafari et al., 2018).
3 Results and discussion
3.1 Proximate chemical composition
Table 2 presents the proximate chemical composition of the snacks and C. vulgaris biomass. Regarding the protein content, increasing incorporation of C. vulgaris, by replacing whole meal oat flour and corn starch in snack’s formulations, led to a significant (p < 0.05) increased content of protein. Significantly higher protein content is in agreement with previous studies in the literature for microalgae-enriched bakery food products (Batista et al., 2017; 2019). Moreover, snacks with 2% and higher C. vulgaris incorporations meet the requirement for being a “source of protein”, as stated on the European Commission Regulation N° 1,169/2011 (The European Parliment and the Council of the European Union, 2011).
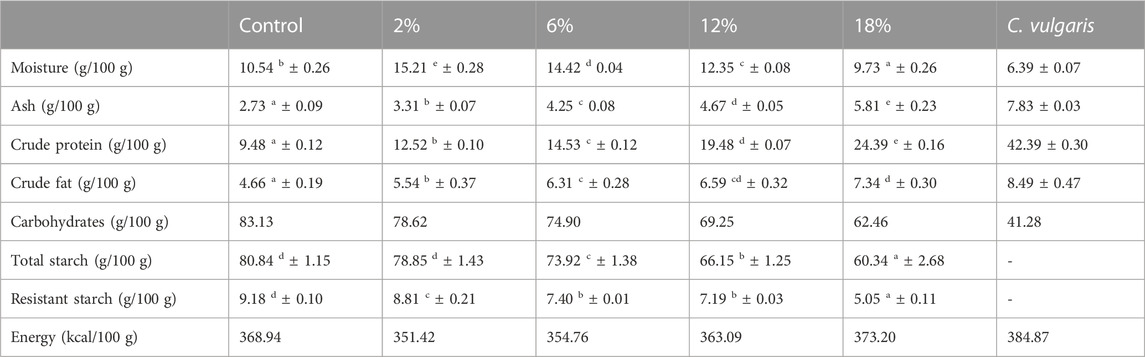
TABLE 2. Proximate chemical composition (moisture, ash, crude protein, crude fat, carbohydrates, total and resistant starch, and energy) of snacks and Chlorella vulgaris biomass. Values (g/100 g DW) are given as mean ± standard deviation (n = 3) (except for carbohydrates, calculated by difference, and energy). Means with different letters, within the same line, differ significantly (p < 0.05).
In terms of fat content, increasing incorporations for C. vulgaris also led to significantly higher fat contents between control and C. vulgaris-enriched snacks. Previous studies found that the C. vulgaris is rich in polyunsaturated fatty acids (PUFAs), in particular, ω3-PUFA such as α-linolenic acid, which are essential fatty acids that must be supplied in the diet, as they cannot be synthesized by the human body (Batista et al., 2013; Canelli et al., 2020). In this sense, increase of fat content in snacks is likely to be linked to a supplementation of PUFAs in snacks. As for total starch content, it was observed that it significantly decreased with additions of C. vulgaris. Results obtained for total starch content closely coincide with snack’s carbohydrates content, indicating a high contribution of starch for the total content of carbohydrates, representing a main source of energy in snacks. Furthermore, the decrease of both total starch and the resistant starch fraction is in agreement with the results obtained from the starch hydrolysis (Figure 2) that show a decrease of snacks’ glycemic index (Table 7) with increasing C. vulgaris additions.
Regarding mineral content, significant differences were also found in terms of total ash content, between control and C. vulgaris-enriched snacks and among snacks with incorporated C. vulgaris. Mineral profile of snacks, presented in Table 3, shows a general significant increase of mineral content, for all analyzed minerals, with C. vulgaris incorporation. Based on the values obtained for mineral content, snacks enriched with C. vulgaris could be considered as an interesting mineral-enriched bakery product, that meets the requirements for nutritional claims, stated on the European Commission Regulation N° 1,169/2011, which declares that a food product is high in minerals if the product contains at least twice the value stipulated for its daily reference intake (The European Parliment and the Council of the European Union, 2011).
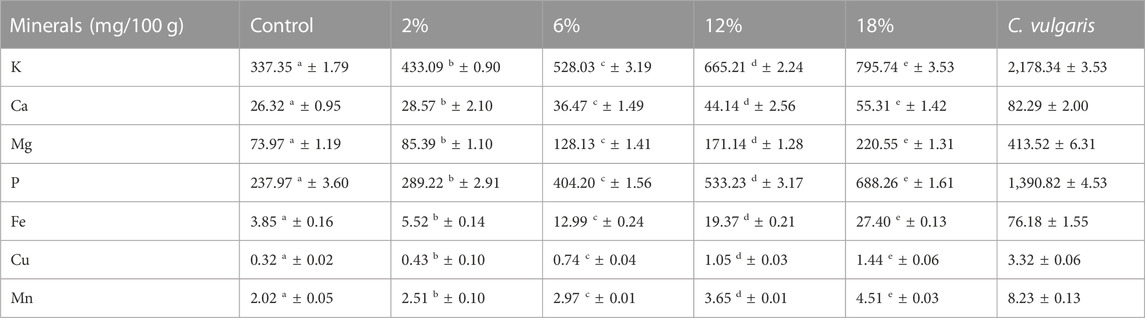
TABLE 3. Mineral profile of snacks and Chlorella vulgaris. Values (mg/100 g DW) are given as mean ± standard (n = 3). Means with different letters, within the same line, differ significantly (p < 0.05).
Table 4 presents the amino acids profile of snacks (control, 2% and 6% C. vulgaris incorporation). Results show there is a tendency for increasing amounts of amino acids in snacks with increasing incorporations of C. vulgaris, with the presence of all 9 essential amino acids. However, it can be observed that this increase is generally not significant (p < 0.05). Previous studies suggest that C. vulgaris is particularly rich in glutamic acid (9.7 g/100 g DW), aspartic acid (7.8 g/100 g DW) and alanine (7.2 g/100 g DW) (Tibbetts et al., 2015). In accordance, these are the amino acids that present a significant (p < 0.05) difference between control snack and 6% C. vulgaris snack. Despite the overall tendency for new microalgae-based products to be launched, there are very few reports on the amino acid content and profile of foods enriched with C. vulgaris. To the best of our knowledge no information on the amino acids profile of cereal-based foods is available. A study conducted by Cabrol et al. (2022), regarding breast muscle of broiler chickens fed with 10% microalga-enriched feed, indicates that lysine (14.14 g/100 g), glutamic acid (13.20 g/100 g) and arginine (12.13 g/100 g) presented the highest amounts of amino acids. Although these amino acids are naturally abundant in broiler breast meat, these results are in agreement with the present study (Cabrol et al., 2022).
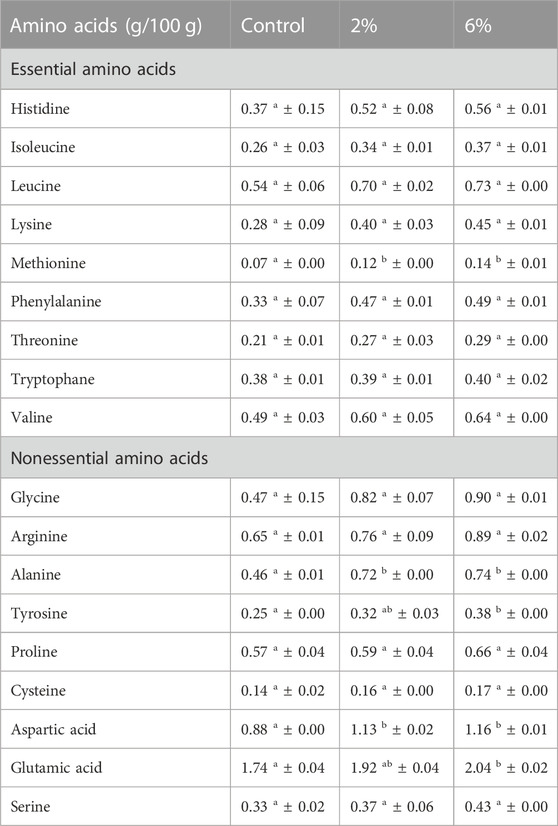
TABLE 4. Amino acids profile of snacks (control, 2% Chlorella vulgaris and 6% Chlorella vulgaris). Values (g/100 g DW) are given as mean ± standard (n = 2). Means with different letters, within the same line, differ significantly (p < 0.05).
3.2 In vitro digestion and bioaccessibility
The in vitro protein digestibility (IVPD) of snacks is presented in Table 5. The bioaccessibility of the protein content represents the fraction–soluble fraction–of the broken down oligopeptides released from the snack matrix in the gastrointestinal tract that become available for absorption (Caporgno and Mathys, 2018). The assessment of the bioaccessibility of protein, together with its content in essential amino acids (Table 4) can contribute to better analyze the quality of proteins presented in snacks (Demarco et al., 2022). Providing information pertaining to the bioaccessibility of nutrients of the C. vulgaris snacks contributes to a better understanding of how nutrients can play a real role on the health impact on the human body. This information is particularly relevant as few studies provide any information regarding the bioaccessibility of algae foods.

TABLE 5. Protein digestibility and recovered fractions (soluble and insoluble) of snacks (control, 2%, 6%, 12% and 18% Chlorella vulgaris). Values (%) are given as mean ± standard (n = 2). Means with different letters, within the same line, differ significantly (p < 0.05).
Results show that increasing incorporations of C. vulgaris led to a poorer digestibility of snacks. According to the literature, IVPD of C. vulgaris raw biomass ranges between 61.2% and 87.2% (Tibbetts et al., 2015; Niccolai et al., 2019b; Qazi et al., 2021). Comparison of results obtained from different studies is difficult as no definitive model for bioaccessibility currently exists. Such difference of reported values can be attributed to considerable different methods applied in simulating digestion and quantifying protein content, as well as due to intrinsic microalga biochemical attributes such as nutritional composition and presence of a rigid cell wall. Previous studies have shown that thick-walled microalgae, like C. vulgaris, exhibit poor digestibility compared to species with thin cell walls or even that lack cell wall (Kose et al., 2017). C. vulgaris presents a rigid cell wall composed mainly of cellulose and hemicellulose and also presents algaenan, a resistant aliphatic biopolymer composed of ether-linked long alkyl chains of esterified monomers (Galafat et al., 2022). Presence of a rigid cell wall can be considered as one of the main factors for the increase of the insoluble protein fraction and a decrease in the soluble fraction, with increasing incorporations of C. vulgaris in snacks.
Although previous studies suggest that cereal-based products (bread) present high protein recovery rates, our results show a comparable recovery rate for control snack (92%), which is in accordance with the value (99%) reported by Rieder et al. (2021), using the same INFOGEST protocol (Rieder et al., 2021). In addition, our results have shown a similar digestibility-like behavior reported by other authors who have also accounted for a decrease of protein digestibility for microalgae-enriched cereal food products like bread, pasta and couscous (Khemiri et al., 2021; Marco et al., 2014; W. M; Qazi et al., 2021).
Figure 1 presents the results for the mineral’s bioaccessibility of snacks. Increasing incorporations of C. vulgaris in snacks led to greater bioaccessibility of minerals for absorption in the human body. Manganese (Mn) was the mineral with the highest bioaccessibility, ranging between 87 (control snack) and 91% (18% C. vulgaris snack). However, there were no significant differences for Mn bioaccessibility between snacks. This is likely linked to the poor content of this mineral in the raw microalga biomass (Table 3), thus explaining why increasing incorporations of C. vulgaris did not contribute to enhance Mn content. Second highest mineral bioaccessibility was magnesium (Mg), ranging from 68 (control snack) to 83% (18% C. vulgaris snack). Uribe-Wandurraga et al. (2020b) studied the mineral bioaccessibility of C. vulgaris-enriched cookies (0%–2% microalga incorporation) and reported lower Mg bioaccessibility for control cookie (6.3%) and cookies with 2% microalga (12.3%) (Z. Uribe-Wandurraga et al., 2020a). The authors suggest that minerals’ decreased bioaccessibility can be explained by the presence of absorption inhibitors, like phytic acid and its salt (phytates), which are present in C. vulgaris. When phytic acid is consumed, it binds to other minerals to create phytates which can inhibit the intestinal absorption of certain minerals, by chelating positively charged cations like Ca, Fe, and Mg to form an insoluble complex (Bito et al., 2020; Demarco et al., 2022). Although a low Mg bioaccessibility is not the case in the present study, lower bioaccessibility for other minerals like Ca and Cu could possibly be due to such phenomena. Our results obtained for Ca, K and P are comparable to results presented by Uribe-Wandurraga et al., who report similar, yet lower, values.
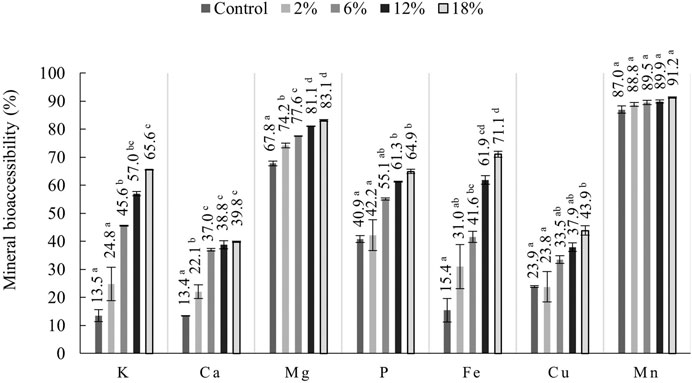
FIGURE 1. Mineral bioaccessibility (%) of snacks. Values are graphically presented as mean and error bar (n = 2). Means with different letters, for the same mineral, differ significantly (p < 0.05).
In the present study, iron (Fe) bioaccessibility varied from 15.4 (control snack) to 71.1% (18% C. vulgaris snack). Iron deficiency is one of the most common nutritional deficiencies in the world and is often added to foods such as breakfast cereals (Bito et al., 2020). As mentioned previously, C. vulgaris-enriched snacks are rich in Fe, as according to the nutritional claim (Table 3), and its high bioaccessibility suggests that snacks could contribute to tackle current worldwide iron deficiency. In the study conducted by Uribe-Wandurraga et al. (2020b) cookies presented lower bioaccessibility for Fe, namely, 0.2% for control cookie and 0.6% for 2% C. vulgaris cookie. Such difference between results could be linked to the chelation ability of certain peptides present in snacks and in cookies (Hayes, 2018).
3.3 Total starch, in vitro starch digestibility and predicted glycemic index
Figure 2 presents the hydrolysis index obtained from the in vitro starch digestibility over time (0—180 min). Increasing additions of C. vulgaris to snacks produced a significant (p < 0.05) reduction in starch hydrolysis, comparing to control wheat bread (CWB) and control snack, also rendered by the hydrolysis indices (HI), presented on Table 6, which vary from 100% (CBW) to 48% (18% C. vulgaris snack).
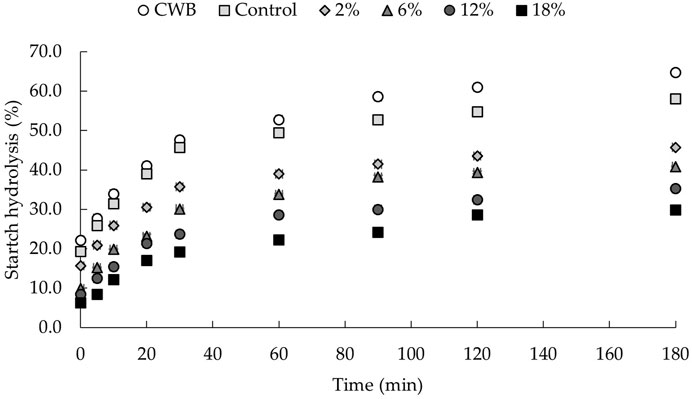
FIGURE 2. Starch hydrolysis (%) of snacks. Values are graphically presented as mean and error bar (n = 3).
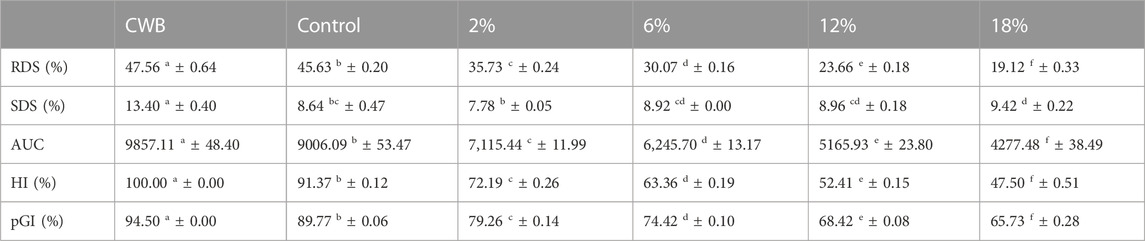
TABLE 6. In vitro starch digestion parameters (RDS, SDS, AUC, HI) and predicted glycemic index of snacks. Values are given as mean ± standard deviation (n = 3). Means with different letters, within the same line, differ significantly (p < 0.05).
Predicted glycemic indices of snacks, obtained from Eq. 3 and presented in Table 6, show a significant (p < 0.05) reduction with increasing C. vulgaris incorporations. High-GI foods are defined as those that present a GI value ≥70, medium-GI foods are those with a GI from 56 to 69, and low-GI foods are those with a GI value ≤55 (Atkinson et al., 2021). According to this classification, control snack and C. vulgaris-enriched snacks with 2% and 6% incorporation are classified as high-GI food products. Snacks enriched with 12% and 18% C. vulgaris incorporation present a glycemic index lower than 69 and are thus classified as medium-GI food products, presenting a reduction of GI by 24% and 27%, respectively, compared to the GI of control snack. The replacement of whole meal oat flour and corn starch by C. vulgaris biomass led to a significant decrease of the total starch content and a significant increase in protein content (Table 2). In turn, competition for water is enhanced as the water content was kept constant (46% w/w) with increasing additions of C. vulgaris (Oliveira et al., 2022). Increased water absorption of the dough, enhanced by the increase of the protein content, can cause a decrease of the amount of fully hydrated starch granules, which in turn may reduce starch gelatinization due to limited water availability. As starch is less available to swell and rupture, enzymes activity on starch granules is reduced and starch hydrolysis is limited (Graça et al., 2020). The significant decrease of the RDS fraction (Table 6) is likely to be linked to the limiting degree of starch gelatinization (Graça et al., 2020). Our results corroborate previous studies that also reported a reduction of different starch fractions with increasing microalgae incorporation (Marco et al., 2014).
3.4 Total phenolics, antioxidant activity, pigments and vitamins
Table 7 presents the determination of the total phenolic compounds (TPC), antioxidant activity and pigments of snacks and C. vulgaris raw biomass.
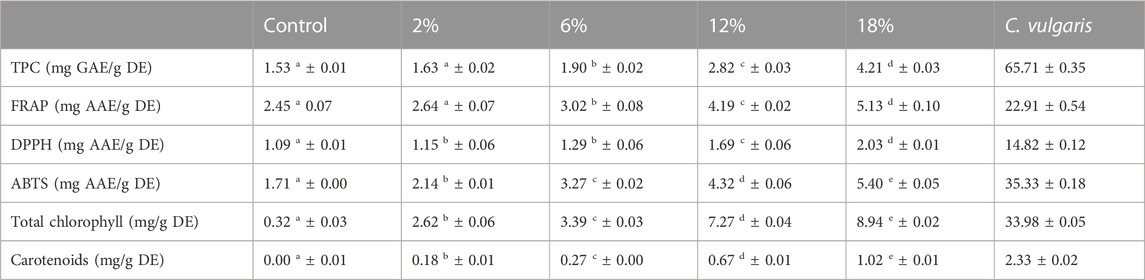
TABLE 7. Total phenolic compounds, antioxidant activity (FRAP, DPPH and ABTS assays) and pigments (total chlorophyll and carotenoids) of snacks’ extracts (15 mg/mL) and Chlorella vulgaris’ extract (1 mg/mL). Values are given as mean ± standard deviation (n = 3). Means with different letters, within the same line, differ significantly (p < 0.05).
The addition of microalgae to snacks resulted in an increase in total phenolic compounds. This increase in TPC shows to be significant above 12% C. vulgaris incorporation. Previous studies presented lower TPC values in baked products with C. vulgaris incorporation. Batista et al. (2017) reported that cookies with 6% C. vulgaris incorporation presented TPC below 0.3 mg GAE/g (Batista et al., 2017). In a study with wheat crackers, authors found that crackers with 6% C. vulgaris incorporation presented 1.7 mg GAE/g (in present study: 1.9 mg GAE/g DE) (Batista et al., 2019). Differences in TPC can be attributed to different factors such as sample’s processing conditions and extract’s concentration. In both studies, cookies and crackers were baked in an oven with different temperature-time conditions: 110°C for 40 min and 180°C for 10 min, respectively; in present study 170°C for 15 min were applied.
C. vulgaris presented a high content of TPC, possibly due to an overestimation of TPC. Certain non-phenolic reducing agents present in food products contribute to the reduction of the Folin-Ciocalteau reagent. In the case of C. vulgaris, presence of certain amino acids might be responsible for the obtained content of TPC (Shahidi and Zhong, 2015).
Antioxidant activity was measured by the DPPH, ABTS and FRAP assays. Results obtained from these three methods show that antioxidant activity increased with increasing C. vulgaris additions, being significantly different between snacks with C. vulgaris incorporation above 6%. A strong positive correlation was obtained between TPC and the three methods used to measure the antioxidant activity: DPPH (r = 0.97), FRAP (r = 0.98) and ABTS (r = 0.96).
Microalgae have been studied for years as a source of pigments, which are known to enhance immunity through protection of the cell from oxidative stress (Hayes, 2018). Increasing incorporations of C. vulgaris biomass enhanced pigments’ content of snacks. Qazi et al. (2022) reported a similar concentration of total chlorophyll (chlorophyll a and b) for bread with 4% C. vulgaris incorporation (∼2.4 mg/g) (Qazi et al., 2022). Pigments are known to be powerful antioxidants and a strong positive correlation was found between total chlorophylls and antioxidant activity, using the methods previously described: DPPH (r = 0.97), FRAP (r = 0.97) and ABTS (r = 0.93) (Pina-Pérez et al., 2019).
Table 8 presents the content of tocotrienols and tocopherols of snacks (control, 2% and 6% C. vulgaris incorporation). Tocotrienols and tocopherols, two different forms of vitamin E, are synthesized by photosynthetic organisms and require continuous assimilation through diet (Del Mondo et al., 2020). These bioactive compounds are known to present great antioxidant activity, including retardation of PUFAs oxidation (López-Hernández et al., 2020). Results presented in Table 8 show a slight increase for all forms of vitamin E; however, only significant for tocopherols (alpha and gamma) between control snack and 6% C. vulgaris snack. Previous studies reported a significant content of α-tocopherol in Chlorella raw biomass (2000 μg/g DW). (Del Mondo et al., 2020). In addition to Chlorella being a source of α-tocopherol, this vitamin E form is also present in vegetable oils, such as olive oil which presents α-tocopherol as the most abundant form of vitamin E (Cunha et al., 2006). In this sense, increasing content of α-tocopherol in snacks is likely to have contributed to enhance snacks’ antioxidant activity, as this form of vitamin E is present in two main ingredients of snacks’ formulation: extra virgin olive oil (5% w/w) and C. vulgaris biomass (2%—18% w/w).
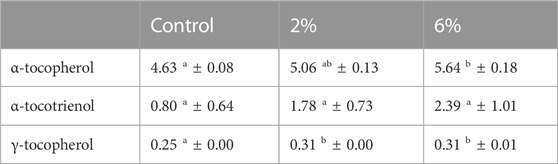
TABLE 8. Content of tocotrienols and tocopherols of snacks (control, 2% Chlorella vulgaris and 6% Chlorella vulgaris). Values (µg/g DW) are given as mean ± standard (n = 2). Means with different letters, within the same line, differ significantly (p < 0.05).
3.5 Antibacterial activity
Table 9 presents the antibacterial activity of snacks and C. vulgaris raw biomass. Results suggest that increasing C. vulgaris incorporations have a positive effect on limiting bacterial activity on snacks. Snacks with C. vulgaris incorporations above 6% and 12% presented a significant (p < 0.05) reduction of bacterial activity for strains L. plantarum and B. cereus, respectively, when compared to control snack. Only a few studies provide evidence of C. vulgaris’ antibacterial activity and to the best of our knowledge no information on antibacterial activity of microalgae-enriched food is available. C. vulgaris raw biomass presented greater antibacterial activity against B. cereus (1.5 cm of inhibition zone). Previous studies have confirmed that C. vulgaris presents antibacterial activity against Bacillus: 1.3 cm of inhibition zone (Syed et al., 2015). Regarding E. coli, previous studies reported greater inhibition zones for this bacterium. Pradhan (2010) reported antibacterial activity against two serotypes of E. coli: 1.5 cm (O111) and 1.4 cm (O115) and Hussein (2018) reported an inhibition zone of 1.5 cm (Pradhan, 2010; Hussein et al., 2018).
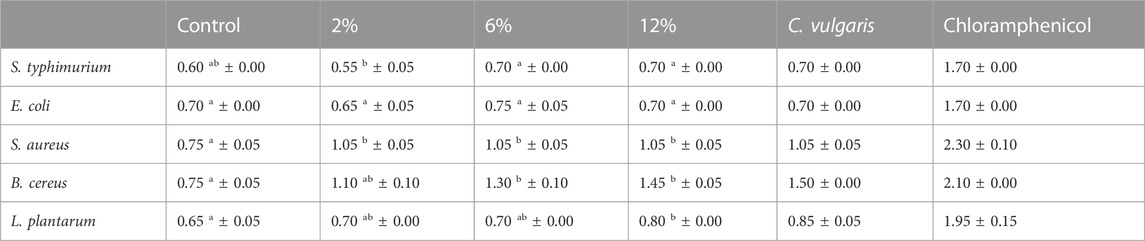
TABLE 9. Antibacterial activity of snacks’ and Chlorella vulgaris’ extracts (3 mg/disc) and chloramphenicol (10 µg/disc). Values are given as mean ± standard deviation (n = 2). Means with different letters, within the same line, differ significantly (p < 0.05).
4 Conclusion
Chlorella vulgaris is a valuable food source, rich in bioactive compounds with potential health benefits. Incorporation of C. vulgaris in food products can help promoting more sustainable healthy food options. In this study, snacks were enriched with increasing incorporations of C. vulgaris (2%—18% w/w). Results show an improvement of snacks’ nutritional composition, notably in minerals and proteins, permitting to claim that snacks are a “source of protein” and “high in” a wide selection of minerals. In vitro digestion results showed that protein digestibility decreased with additional amounts of C. vulgaris. In contrast, mineral bioaccessibility was enhanced by the microalga’s incorporation. Results from starch digestibility showed that incorporation of C. vulgaris helped decreasing snacks’ glycemic index. Antioxidant activity was promoted by the presence of bioactive compounds (phenolics, pigments and vitamins) derived from the incorporation of C. vulgaris biomass in snacks. Findings from antibacterial activity revealed the snacks enriched with C. vulgaris present a positive effect on limiting bacterial activity for L. plantarum and Bacillus cereus.
Data availability statement
The original contributions presented in the study are included in the article/supplementary material, further inquiries can be directed to the corresponding author.
Author contributions
SO: Formal Analysis, Methodology, Writing–original draft. MT: Supervision, Writing–review and editing. IS: Methodology, Supervision, Writing–review and editing. AR: Methodology, Supervision, Writing–review and editing.
Funding
The author(s) declare financial support was received for the research, authorship, and/or publication of this article. This work was funded by national funds through FCT–Fundação para a Ciência e a Tecnologia, I.P., under the project UIDB/04129/2020 of LEAF-Linking Landscape, Environment, Agriculture and Food, Research Unit, and PhD Grant 2020.07207. BD. MT acknowledges the Ministry of Science, Innovation and Universities of Spain for her postdoctoral grants (RYC 2018-024454-I) and the Consellería de Cultura, Educación e Universidade da Xunta de Galicia (ED431F 2020/01).
Acknowledgments
The authors wish to thank A2F–Algae to Future project (267872/E50 Research Council of Norway's BIONÆR Programme) for the supply of Chlorella vulgaris biomass.
Conflict of interest
The authors (IS) and (AR) declared that they were editorial board members of Frontiers at the time of submission. This had no impact on the peer review process and the final decision.
The remaining authors declare that the research was conducted in the absence of any commercial or financial relationships that could be construed as a potential conflict of interest.
Publisher’s note
All claims expressed in this article are solely those of the authors and do not necessarily represent those of their affiliated organizations, or those of the publisher, the editors and the reviewers. Any product that may be evaluated in this article, or claim that may be made by its manufacturer, is not guaranteed or endorsed by the publisher.
References
AACC International (1999). “Approved methods of analysis,” in Method 44-15.02. Moisture—air-Oven methods final (Eagan, Minnesota: Cereals and Grains Association).
AACC International (2009a). “Ash--Basic method,” in AACC international approved methods, 8–9. doi:10.1094/aaccintmethod-08-01.01
AACC International (2009b). “Crude fat in wheat, corn, and soy flour, feeds, and mixed feeds,” in AACC international approved methods (Eagan, Minnesota: Cereals and Grains Association), 2–3. doi:10.1094/aaccintmethod-30-25.01
Ak, B., Avşaroğlu, E., Işık, O., Özyurt, G., Kafkas, E., Etyemez, M., et al. (2016). Nutritional and physicochemical characteristics of bread enriched with microalgae spirulina platensis. Int. J. Eng. Res. Appl. 6 (12), 30–38.
Atkinson, F. S., Brand-miller, J. C., Foster-powell, K., Buyken, A. E., and Goletzke, J. (2021). International tables of glycemic index and glycemic load values 2021: a systematic review. Am. J. Clin. Nutr. 114, 1625–1632. doi:10.1093/ajcn/nqab233
Babuskin, S., Krishnan, K. R., Babu, P. A. S., Sivarajan, M., and Sukumar, M. (2014). Functional foods enriched with marine microalga Nannochloropsis oculata as a source of ω-3 fatty acids. Food Technol. Biotechnol. 52 (3), 292–299.
Bandarra, N. M., Batista, A. P., Fradique, M., Raymundo, A., Gouveia, L., and Nunes, M. C. (2010). Incorporation of Chlorella vulgaris and Spirulina maxima biomass in pasta products. Part 1: preparation and evaluation. J. Sci. Food Agric. 90 (10), 1656–1664. doi:10.1002/jsfa.3999
Barreira, J. C. M., Dias, M. I., Živković, J., Stojkovic, D., Soković, M., Santos-Buelga, C., et al. (2014). Phenolic profiling of Veronica spp. grown in mountain, urban and sandy soil environments. Food Chem. 163, 275–283. doi:10.1016/j.foodchem.2014.04.117
Batista, A. P., Gouveia, L., Bandarra, N. M., Franco, J. M., and Raymundo, A. (2013). Comparison of microalgal biomass profiles as novel functional ingredient for food products. Algal Res. 2 (2), 164–173. doi:10.1016/j.algal.2013.01.004
Batista, A. P., Niccolai, A., Bursic, I., Sousa, I., Raymundo, A., Rodolfi, L., et al. (2019). Microalgae as functional ingredients in savory food products: application to wheat crackers. Foods 8 (12), 611. doi:10.3390/foods8120611
Batista, A. P., Niccolai, A., Fradinho, P., Fragoso, S., Bursic, I., Rodolfi, L., et al. (2017). Microalgae biomass as an alternative ingredient in cookies: sensory, physical and chemical properties, antioxidant activity and in vitro digestibility. Algal Res. 26, 161–171. doi:10.1016/j.algal.2017.07.017
Benzie, I. F., and Strain, J. J. (1996). The ferric reducing ability of plasma (FRAP) as a measure of “antioxidant power”: the FRAP assay. Anal. Biochem. 239 (1), 70–76. doi:10.1006/abio.1996.0292
Bhattacharya, T., Rather, G., Akter, R., Tanvir, K., Rauf, A., and Rahman, H. (2021). Nutraceuticals and bio-inspired materials from microalgae and their future perspectives. Curr. Top. Med. Chem. 21 (12), 1037–1051. doi:10.2174/1568026621666210524095925
Bito, T., Okumura, E., Fujishima, M., and Watanabe, F. (2020). Potential of chlorella as a dietary supplement to promote human health. Nutrients 12 (9), 2524–2621. doi:10.3390/nu12092524
Bolanho, B. C., Egea, M. B., Jácome, A. L. M., Campos, I., de Carvalho, J. C. M., and Danesi, E. D. G. (2014). Antioxidant and nutritional potential of cookies enriched with Spirulina platensis and sources of fibre. J. Food Nutr. Res. 53 (2), 171–179.
Brand-Williams, W., Cuvelier, M. E., and Berset, C. (1995). Use of a free radical method to evaluate antioxidant activity. LWT - Food Sci. Technol. 28 (1), 25–30. doi:10.1016/S0023-6438(95)80008-5
Brodkorb, A., Egger, L., Alminger, M., Alvito, P., Assunção, R., Ballance, S., et al. (2019). INFOGEST static in vitro simulation of gastrointestinal food digestion. Nat. Protoc. 14 (4), 991–1014. doi:10.1038/s41596-018-0119-1
Cabrol, M. B., Martins, J. C., Malhão, L. P., Alfaia, C. M., Prates, J. A. M., Almeida, A. M., et al. (2022). Digestibility of meat mineral and proteins from broilers fed with graded levels of chlorella vulgaris. Foods 11 (9), 1–17. doi:10.3390/foods11091345
Canelli, G., Tarnutzer, C., Carpine, R., Neutsch, L., Bolten, C. J., Dionisi, F., et al. (2020). Biochemical and nutritional evaluation of chlorella and auxenochlorella biomasses relevant for food application. Front. Nutr. 7, 565996–565999. doi:10.3389/fnut.2020.565996
Caporgno, M. P., and Mathys, A. (2018). Trends in microalgae incorporation into innovative food products with potential health benefits. Front. Nutr. 5, 58–10. doi:10.3389/fnut.2018.00058
Cotas, J., Pacheco, D., Gonçalves, A. M. M., Silva, P., Carvalho, L. G., and Pereira, L. (2021). Seaweeds’ nutraceutical and biomedical potential in cancer therapy: a concise review. J. Cancer Metastasis Treat. 7. doi:10.20517/2394-4722.2020.134
Cunha, S. C., Amaral, J. S., Fernandes, J. O., and Oliveira, M. B. P. P. (2006). Quantification of tocopherols and tocotrienols in Portuguese olive oils using HPLC with three different detection systems. J. Agric. Food Chem. 54 (9), 3351–3356. doi:10.1021/jf053102n
Del Mondo, A., Smerilli, A., Sané, E., Sansone, C., and Brunet, C. (2020). Challenging microalgal vitamins for human health. Microb. Cell Factories 19 (1), 201–223. doi:10.1186/s12934-020-01459-1
Demarco, M., Oliveira de Moraes, J., Matos, Â. P., Derner, R. B., de Farias Neves, F., and Tribuzi, G. (2022). Digestibility, bioaccessibility and bioactivity of compounds from algae. Trends Food Sci. Technol. 121, 114–128. doi:10.1016/j.tifs.2022.02.004
Fradinho, P., Niccolai, A., Soares, R., Rodolfi, L., Biondi, N., Tredici, M. R., et al. (2020). Effect of Arthrospira platensis (spirulina) incorporation on the rheological and bioactive properties of gluten-free fresh pasta. Algal Res. 45, 101743. doi:10.1016/j.algal.2019.101743
Fradique, M., Batista, A. P., Nunes, M. C., Gouveia, L., Bandarra, N. M., and Raymundo, A. (2013). Isochrysis galbana and Diacronema vlkianum biomass incorporation in pasta products as PUFA’s source. LWT - Food Sci. Technol. 50 (1), 312–319. doi:10.1016/j.lwt.2012.05.006
Galafat, A., Jesús, A., María, V., Sáez, I., Luis, J., Pinchetti, G., et al. (2022). Evaluation of the in vitro protein bioaccessibility of several microalgae and cyanobacteria as potential dietary ingredients in gilthead seabream (Sparus aurata) juveniles. J. Appl. Phycol. 34 (4), 1–14. doi:10.1007/s10811-022-02764-5
García-Segovia, P., Pagán-Moreno, M. J., Lara, I. F., and Martínez-Monzó, J. (2017). Effect of microalgae incorporation on physicochemical and textural properties in wheat bread formulation. Food Sci. Technol. Int. 23 (5), 437–447. doi:10.1177/1082013217700259
Germaine, K. A., Samman, S., Fryirs, C. G, Griffiths, P. J., Johnson, S. K, Quail, K. J., et al. (2008). Comparison of in Vitro Starch Digestibility Methods for Predicting the Glycaemic Index of Grain Foods. J. Sci. Food Agric. 88, (4), 652–658. doi:10.1002/jsfa.3130
Goñi, I., Garcia-Alonso, A., and Saura-Calixto, F. (1997). A starch hydrolysis procedure to estimate glycemic index. Nutr. Res. 17 (3), 427–437. doi:10.1016/S0271-5317(97)00010-9
Gouveia, L., Coutinho, C., Mendonça, E., Batista, A. P., Sousa, I., Bandarra, N. M., et al. (2008). Functional biscuits with PUFA-ω3 from Isochrysis galbana. J. Sci. Food Agric. 88, 891–896. doi:10.1002/jsfa.3166
Graça, C., Fradinho, P., Sousa, I., and Raymundo, A. (2018). Impact of Chlorella vulgaris on the rheology of wheat flour dough and bread texture. LWT - Food Sci. Technol. 89, 466–474. doi:10.1016/j.lwt.2017.11.024
Graça, C., Mota, J., Lima, A., Ferreira, R. B., Raymundo, A., and Sousa, I. (2020). Glycemic response and bioactive properties of gluten-free bread with yoghurt or curd-cheese addition. Foods 9 (10), 1410. doi:10.3390/foods9101410
Hayes, M. (2018). Novel proteins for food, pharmaceuticals, and agriculture: sources, applications, and advances. Hoboken,NJ, USA: Wiley-Blackwell.
Hossain, A. K. M. M., Brennan, M. A., Mason, S. L., Guo, X., Zeng, X. A., and Brennan, C. S. (2017). The effect of astaxanthin-rich microalgae “haematococcus pluvialis” and wholemeal flours incorporation in improving the physical and functional properties of cookies. Foods 6 (8), 57. doi:10.3390/foods6080057
Hussein, H. J., Naji, S. S., and Al-Khafaji, N. M. S. (2018). Antibacterial properties of the Chlorella vulgaris isolated from polluted water in Iraq. J. Pharm. Sci. Res. 10 (10), 2457–2460.
Jafari, S., Mobasher, M. A., Najafipour, S., Ghasemi, Y., Mohkam, M., Ebrahimi, M. A., et al. (2018). Antibacterial potential of Chlorella vulgaris and Dunaliella salina extracts against Streptococcus mutans. Jundishapur J. Nat. Pharm. Prod. 13 (2). doi:10.5812/jjnpp.13226
Khemiri, S., Nunes, M. C., Bessa, R. J. B., Alves, S. P., Smaali, I., and Raymundo, A. (2021). Technological feasibility of couscous-algae-supplemented formulae: process description, nutritional properties and in vitro digestibility. Foods 10 (12), 3159. doi:10.3390/foods10123159
Kose, A., Ozen, M. O., Elibol, M., and Oncel, S. S. (2017). Investigation of in vitro digestibility of dietary microalga Chlorella vulgaris and cyanobacterium Spirulina platensis as a nutritional supplement. Biotech 7 (3), 170–177. doi:10.1007/s13205-017-0832-4
Kumar, S., and Deshmukh, R. (2021). Global microalgae market opportunity analysis and industry forecast. Available at: https://www.alliedmarketresearch.com/microalgae-market-A13419.
Lichtenthaler, H. K., and Buschmann, C. (2001). Chlorophylls and carotenoids: measurement and characterization by UV-VIS spectroscopy. Curr. Protoc. Food Anal. Chem. 1 (1). doi:10.1002/0471142913.faf0403s01
Loke, P. (2022). Global market and economic analysis of microalgae technology: status and perspectives. Bioresour. Technol. 357, 127329. doi:10.1016/j.biortech.2022.127329
López-Hernández, J. F., García-Alamilla, P., Palma-Ramírez, D., Álvarez-González, C. A., Paredes-Rojas, J. C., and Márquez-Rocha, F. J. (2020). Continuous microalgal cultivation for antioxidants production. Molecules 25 (18), 4171. doi:10.3390/molecules25184171
Lucas, B. F., Morais, M. G., de Santos, T. D., and Costa, J. A. V. (2018). Spirulina for snack enrichment: nutritional, physical and sensory evaluations. LWT - Food Sci. Technol. 90, 270–276. doi:10.1016/j.lwt.2017.12.032
Marco, E. R.De, Steffolani, M. E., Martínez, C. S., and León, A. E. (2014). Effects of spirulina biomass on the technological and nutritional quality of bread wheat pasta. LWT - Food Sci. Technol. 58 (1), 102–108. doi:10.1016/j.lwt.2014.02.054
Martins, R. B., Gouvinhas, I., Nunes, M. C., Peres, J. A., Raymundo, A., and Barros, A. I. R. N. A. (2020). Acorn flour as a source of bioactive compounds in gluten-free bread. Molecules 25 (16), 1–19. doi:10.3390/molecules25163568
Mohankumar, J. B., Uthira, L., and Su, M. (2018). Total phenolic content of organic and conventional green leafy vegetables. J. Nutr. Hum. Health 02 (01). doi:10.35841/nutrition-human-health.2.1.1-6
Niccolai, A., Chini Zittelli, G., Rodolfi, L., Biondi, N., and Tredici, M. R. (2019a). Microalgae of interest as food source: biochemical composition and digestibility. Algal Res. 42, 101617. doi:10.1016/j.algal.2019.101617
Niccolai, A., Venturi, M., Galli, V., Pini, N., Rodolfi, L., Biondi, N., et al. (2019b). Development of new microalgae-based sourdough “crostini”: functional effects of Arthrospira platensis (spirulina) addition. Sci. Rep. 9 (1), 19433–19512. doi:10.1038/s41598-019-55840-1
Nunes, C., Graça, C., Vlaisavljević, S., Tenreiro, A., Sousa, I., and Raymundo, A. (2020). Microalgal cell disruption: effect on the bioactivity and rheology of wheat bread. Algal Res. 45, 101749. doi:10.1016/j.algal.2019.101749
Oliveira, S., Sousa, I., and Raymundo, A. (2022). Printability evaluation of Chlorella vulgaris snacks. Algal Res. 68, 102879. doi:10.1016/j.algal.2022.102879
Pina-Pérez, M. C., Brück, W. M., Brück, T., and Beyrer, M. (2019). “Microalgae as healthy ingredients for functional foods,” in The role of alternative and innovative food ingredients and products in consumer wellness (Amsterdam, Netherlands: Elsevier). doi:10.1016/b978-0-12-816453-2.00004-8
Pradhan, J. (2010). Antibacterial properties of selected freshwater microalgae against pathogenic bacteria. Indian J. Fish. 57 (2), 61–66.
Prates, J. A. M., Quaresma, M. A. G., Bessa, R. J. B., Fontes, C. M. G. ., and Alfaia, C. M. P. M. (2006). Simultaneous HPLC quantification of total cholesterol, tocopherols and β-carotene in Barrosã-PDO veal. Food Chem. 94, 469–477. doi:10.1016/j.foodchem.2005.01.021
Qazi, M. W., Nunes, M. C., Sousa, I., and Raymundo, A. (2022). Improving the nutritional, structural, and sensory properties of gluten-free bread with different species of microalgae. Foods 11, 397. doi:10.3390/foods11030397
Qazi, W. M., Ballance, S., Kousoulaki, K., Uhlen, A. K., Kleinegris, D. M. M., Skjånes, K., et al. (2021). Protein enrichment of wheat bread with microalgae: microchloropsis gaditana, tetraselmis chui and chlorella vulgaris. Foods 10 (12), 3078. doi:10.3390/foods10123078
Re, R., Pellegrini, N., Proteggente, A., Pannala, A., Yang, M., and Rice-Evans, C. (1999). Antioxidant activity applying an improved ABTS radical cation decolorization assay. Free Radic. Biol. Med. 26 (9–10), 1231–1237. doi:10.1016/S0891-5849(98)00315-3
Rieder, A., Afseth, N. K., Böcker, U., Knutsen, S. H., Kirkhus, B., Mæhre, H. K., et al. (2021). Improved estimation of in vitro protein digestibility of different foods using size exclusion chromatography. Food Chem. 358, 129830. doi:10.1016/j.foodchem.2021.129830
Şahin, O. I. (2020). Functional and sensorial properties of cookies enriched with SPIRULINA and DUNALIELLA biomass. J. Food Sci. Technol. 57 (10), 3639–3646. doi:10.1007/s13197-020-04396-4
Shahidi, F., and Zhong, Y. (2015). Measurement of antioxidant activity. J. Funct. Foods 18, 757–781. doi:10.1016/j.jff.2015.01.047
Singh, P., Singh, R., Jha, A., Rasane, P., and Gautam, A. K. (2015). Optimization of a process for high fibre and high protein biscuit. J. Food Sci. Technol. 52, 1394–1403. doi:10.1007/s13197-013-1139-z
Syed, S., Arasu, A., and Ponnuswamy, I. (2015). The uses of Chlorella Vulgaris as antimicrobial agent and as a diet: the presence of bio-active compounds which caters the vitamins, minerals in general. Int. J. Bio-Science Bio-Technology 7 (1), 185–190. doi:10.14257/ijbsbt.2015.7.1.19
The European Parliment and the Council of the European Union (2011). Regulation (EU) 1169/2011. Official J. Eur. Union 17 (1169), 18–63.
Tibbetts, S. M., Milley, J. E., and Lall, S. P. (2015). Chemical composition and nutritional properties of freshwater and marine microalgal biomass cultured in photobioreactors. J. Appl. Phycol. 27 (3), 1109–1119. doi:10.1007/s10811-014-0428-x
Torres, M. D., and González, H. (2019). Valorising seaweed by products. Hauppauge: Nova Science Publishers, Inc.
Uribe-Wandurraga, Z., Igual, M., García-segovia, P., and Martínez-monzó, J. (2020a). In vitro bioaccessibility of minerals from microalgae-enriched cookies. Food & Funct. 11, 2186–2194. doi:10.1039/c9fo02603g
Uribe-Wandurraga, Z. N., Igual, M., García-Segovia, P., and Martínez-Monzó, J. (2019). Effect of microalgae addition on mineral content, colour and mechanical properties of breadsticks. Food Funct. 10 (8), 4685–4692. doi:10.1039/c9fo00286c
Uribe-Wandurraga, Z. N., Igual, M., Reino-Moyón, J., García-Segovia, P., and Martínez-Monzó, J. (2020b). Effect of microalgae (arthrospira platensis and chlorella vulgaris) addition on 3D printed cookies. Food Biophys. 16, 27–39. doi:10.1007/s11483-020-09642-y
Uribe-Wandurraga, Z. N., Zhang, L., Noort, M. W. J., Schutyser, M. A. I., García-Segovia, P., and Martínez-Monzó, J. (2020c). Printability and physicochemical properties of microalgae-enriched 3D-printed snacks. Food Bioprocess Technol. 13 (11), 2029–2042. doi:10.1007/s11947-020-02544-4
Vieira, M. V., Oliveira, S. M., Amado, I. R., Fasolin, L. H., Vicente, A. A., Pastrana, L. M., et al. (2020). 3D printed functional cookies fortified with Arthrospira platensis: evaluation of its antioxidant potential and physical-chemical characterization. Food Hydrocoll. 107, 105893. doi:10.1016/j.foodhyd.2020.105893
Keywords: Chlorella vulgaris, 3D food printing, antioxidant activity, digestibility, bioaccessibility, glycemic index
Citation: Oliveira S, Torres Pérez MD, Sousa I and Raymundo A (2023) 3D-printed Chlorella vulgaris snacks: a contribution to a healthy diet. Front. Food. Sci. Technol. 3:1265828. doi: 10.3389/frfst.2023.1265828
Received: 24 July 2023; Accepted: 28 September 2023;
Published: 14 November 2023.
Edited by:
Christos Soukoulis, Luxembourg Institute of Science and Technology (LIST), LuxembourgReviewed by:
Hugo Oliveira, International Iberian Nanotechnology Laboratory (INL), PortugalMine Gültekin-Özgüven, Istanbul Technical University, Türkiye
Copyright © 2023 Oliveira, Torres Pérez, Sousa and Raymundo. This is an open-access article distributed under the terms of the Creative Commons Attribution License (CC BY). The use, distribution or reproduction in other forums is permitted, provided the original author(s) and the copyright owner(s) are credited and that the original publication in this journal is cited, in accordance with accepted academic practice. No use, distribution or reproduction is permitted which does not comply with these terms.
*Correspondence: Sónia Oliveira, c29saXZlaXJhQGlzYS51bGlzYm9hLnB0