- 1Department of Physiology, Escola Paulista de Medicina (EPM), Universidade Federal de São Paulo, São Paulo, Brazil
- 2Department of Nutrition, Universidade Metropolitana de Santos (UNIMES), Santos, Brazil
- 3LEAF—Linking Landscape, Environment, Agriculture and Food Research Center, Associated Laboratory TERRA, School of Agriculture, University of Lisbon, Lisbon, Portugal
- 4Nutrition Laboratory, Faculty of Medicine, University of Lisbon, Lisbon, Portugal
- 5Nutrition and Food Service Research Center, Universidade Federal de São Paulo (UNIFESP), Santos, Brazil
- 6Department of Biosciences, Universidade Federal de São Paulo (UNIFESP), Santos, Brazil
- 7Department of Chemical Engineering, Universidade Federal de São Paulo (UNIFESP), São Paulo, Brazil
Introduction: Following consumers’ demand for healthier alternatives, Spirulina biomass has been included as a functional ingredient in different types of food as a sustainable alternative to improve physicochemical characteristics and nutritional aspects in the diet. This study aimed to explore the use of Spirulina biomass (SB) and residual biomass (RB) obtained after C-phycocyanin (C-PC) extraction in the production of wheat bread (WB).
Methods: The dough rheology, texture, color, nutritional aspects, and bioaccessibility of the different manufactured bread samples were accessed to achieve this goal.
Results and Discussion: This is the first research to exploit RB. It was possible to replace 3% of wheat flour for SB or RB in bread-making; this substitution did not impact the rheological characteristics of the dough, even though the pH increased with the addition of SB or RB (5.63 and 5.70, respectively). SB and RB addition improved bread volume and enhanced the nutritional profile, increasing the antioxidant capacity (DPPH and FRAP) compared to WB. Heavy metals (Ni, Cd, and Pb) were not found in any of the samples (<0.2 mg/100 g). The in vitro protein digestibility in bread was better than in SB and RB raw materials, which indicates that bread manufacturing may contribute to improving protein digestibility. In addition, SB needs greater appreciation for sustainable food practices worldwide and in Brazilian exploration, requiring management strategies with industry and society working together. Further studies are necessary, focusing on acceptability to understand the viability of these ingredients to target consumers’ preferences.
1 Introduction
Since 6000 B.C., bread-making has been stated as a symbolic tradition of different cultures around the world (Belderok, 2000; Nawar, 2020). Bread has been recognized as a secular daily food consumed over the years, contributing not only to the macro and micronutrient requirement but also because of the physical and, consequently, sensorial aspects (Dewettinck et al., 2008).
Although bread has a high nutritional value, mainly comprising carbohydrate and protein content and micronutrients such as lipids, vitamins, minerals, and bioactive compounds (Shewry and Hey, 2015), nowadays, the quality of wheat flour for bread manufacture has undergone several changes, such as genetic statistics, cytogenetic research, hybrid and mutation breeding, phenotyping advances, and genomic selection (Venske et al., 2019).
Thus, to achieve food requirements and positive repercussions on health (Amoah et al., 2022), the addition of plant-based ingredients in wheat bread production—besides the use of flour, water, salt, sugar, and yeast ingredients—such as fibers, proteins, enzymes, bioactive compounds, and algae, has been demonstrated as a reality (Onwezen et al., 2021; Zain et al., 2022; Ferreira et al., 2023).
Moreover, healthy products and habits have been increasing in consumer demand (Asioli et al., 2017; Feil et al., 2020; Burt et al., 2021), not only individually but alongside the environmental preservation of fauna and flora, constituting the One Health concept (Banwo et al., 2021). The whole use of biomass produced globally, such as Spirulina (Arthrospira platensis), stimulates the circular economy in a more sustainable manufacturing path, mainly using renewable sources such as cyanobacterium and algae (Amarante and Braga, 2021). To change this scenario, it is necessary to adopt changes in the food and bioprocessing industries (Godfray et al., 2010; Braga et al., 2020).
In this way, Spirulina biomass has been included as a functional ingredient in crostini (toasted bread) (Niccolai et al., 2019) and other bread (Saharan and Jood, 2021), breadsticks (Uribe-Wandurraga et al., 2019), cookies (Batista et al., 2017), wheat crackers (Batista et al., 2019), pasta (Raczyk et al., 2022), and dairy products (Hernández et al., 2022) to increase the food industry’s repertoire, generally providing an intense color (generally green) and flavor, and also to improve nutritional and technological aspects (Lafarga et al., 2020).
It is essential to highlight that this is one of the few studies demonstrating the potential of Spirulina’s residual biomass (RB) for food production. Two previous works from our research group (Fratelli et al., 2022; Braga et al., 2023) mentioned its potential, and the first report on use of residual biomass as an ingredient was recently published.
Spirulina has already been regarded as a sustainable protein source since it contains up to 70% protein in its dry mass, grows faster than terrestrial plants, and has higher protein productivity per area than other crops such as soybean, legumes, or wheat. Given the developments in the circular economy based on Spirulina biomass (SB) and RB after extracting the C-Phycocyanin (C-PC) for better diets and food industry innovation, the current work is an important step in this approach (Braga et al., 2023).
Furthermore, there has been a greater emphasis on optimizing Spirulina production in order to promote economic feasibility and environmental sustainability. Even when C-PC is extracted from Spirulina biomass, the residual biomass (RB) retains significant levels of antioxidants, vitamins, and minerals, making it a viable raw material in high-value goods (Fratelli et al., 2021; Fratelli et al., 2022).
The use of Spirulina in the formulation of wheat bread with the addition of 10% of SB was demonstrated in the literature by Burcu et al. (2016). However, the sensory analysis tests showed that the higher the percentage of SB, the lower the acceptability, as confirmed by Morsy et al. (2014), indicating that the highest overall acceptability of Spirulina snack food was found with a Spirulina biomass addition rate of 2.5% and corn flour addition rate of 97.5%.
In a more recent paper by Saharan and Jood (2021), it was shown that wheat flour bread could be formulated with a ‘liked moderately’ overall acceptability, fortified with Spirulina platensis powder in 2, 4, and 6% levels up to 2 days of storage. The values of acceptability of the control bread (without Spirulina powder supplementation) and bread with the addition of 2% of Spirulina powder were very similar, which may indicate that consumers prefer lower values of Spirulina fortification. The similarities and differences between bread groups could be assured with statistical analysis, which the authors did not present. In addition, several authors have reported that even low-percentage additions of SB and C-PC present a significant improvement of the manufactured products in terms of bioactivity, particularly considering antioxidant activity (Capelli and Cysewski, 2010; El Baky et al., 2015; Palanisamy et al., 2019; Amarante et al., 2020; Niccolai et al., 2021; Braga et al., 2023). Since consumer acceptance is limited regarding the flavors related with microalgae as ingredients (Sanjari et al., 2018), for now, the addition of low percentages is a strategy to include Spirulina and other alternative sources of nutrients in the daily diet of people.
Therefore, this study aimed to apply two innovative ingredients, SB and RB, obtained after C-PC extraction, in the production of wheat bread (WB) to study and compare the dough rheology, texture, color, nutritional aspects, and bioaccessibility between the different manufactured bread samples.
2 Material and methods
2.1 Raw materials
The ingredients used in the bread-making process were obtained from the local Portuguese market, which included commercial wheat flour of type 65 without yeast (Pérola®, Porto, Portugal), distilled water, white cane sugar in sticks (Modelo Continente Hipermercados, S.A., Senhora da Hora, Portugal), refined salt (Modelo Continente Hipermercados, S.A., Senhora da Hora, Portugal), and dehydrated yeast in powder (Fermipan®, Lallemand Iberia, Portugal). The organic and biodynamic Spirulina biomass was supplied by Fazenda Tamanduá® (Paraíba, Brazil).
2.2 Obtention of residual biomass (RB) from Spirulina
According to Fratelli et al. (2022), after the solid–liquid C-PC extraction and centrifugation (for 120 min, with breaks every 30 min, at 10,000 × g (NT 816, Nova Analítica®, Brazil), the RB was obtained for application in bread for the full utilization of SB. Thereby, the supernatant, C-PC, was separated from the remaining biomass, named residual biomass in this work, and stored in an ultra-freezer at −38°C. After RB collection, the RB samples were freeze-dried for 72 h in a freeze dryer (Liobras® K105, São Paulo, Brazil).
2.3 Wheat dough samples: pH and rheological characteristics
The pH measurements were performed in triplicate in the unleavened dough of WB samples using a pH-Meter Basic 20 potentiometer (Crison Instruments®, Spain) until stabilization.
The wheat flour moisture quantification (moisture analyzer PMB 202—Adam Equipment Co. Ltd., United Kingdom) with or without 3% of SB or RB was determined to evaluate the rheological analysis of the unleavened dough. Mixing and pasting characteristics were analyzed in the Micro-doughLAB 2800 equipment (Perten Corp., Sweden) on a small scale (4 g ± 0.01, standardized at 14% of moisture). The viscoelastic behavior was assessed in a HAAKE MARS 60 rheometer (Thermo Scientific®, Karlsruhe, Germany), coupled with a UTC—Peltier system (for temperature control at 18°C), using a serrated parallel-plate sensor system with 20 mm diameter (PP20). All tests were performed at least in triplicate.
The mixing curves were based on the AACC International Method 54–70.01 (AACC, 2014) with laboratory adaptations (at a speed of 120 rpm, 30°C, for 10 min). The water absorption (WA) was adjusted to obtain the maximum torque value of 130 mN.m ± 4% for each dough (target peak). The following parameters were evaluated: I) water absorption (WA); II) degree of softening (DS); III) dough development time (DDT); IV) stability time (ST).
The pasting characteristics were determined according to the AACC International Method 54–60.01 (AACC, 2010), with adaptations by Dang and Bason (2015) and Martins et al. (2020). Thus, the method is based on a constant mixing rate at 63 rpm for 43 min to understand each dough’s behavior after adding water, previously predetermined by water absorption (%) in the Micro-doughLAB 2800 equipment (Perten Corp., Sweden), through a series of temperature cycles: I) 30°C for 6 min; II) temperature increment to 90°C for 15 min; III) constant temperature of 90°C for 7 min; IV) decrease to 50°C for 10 min; and V) constant temperature of 50°C for another 5 min, until the end of the test.
The dough viscoelastic behavior parameters were evaluated by small-amplitude oscillatory shear measurements (SAOS). First, a stress sweep test was performed at 6.28 rad/s (1 Hz) to determine the linear viscoelastic region (LVR). In all the samples, a constant shear stress of 10 Pa was applied to proceed to the frequency sweep test conducted inside the LVR from 0.063 to 396 rad/s. Dough samples were coated with paraffin to prevent dryness during the measurements.
2.4 Production and image analysis of wheat bread samples
The WB formulation was performed in six steps. I) The thermoprocessor (position 3 for 30 s) was used to activate the dehydrated yeast with sugar and warm water at 37°C. II) The addition of wheat flour and refined salt with or without 3% of SB or RB was carried out in WB, WB-SB, and WB-RB doughs. The mixture was processed in the thermoprocessor (position 6 at room temperature). III) The WB doughs were kneaded in the thermoprocessor (cob position for 2 min). IV) WB samples were molded (220 g each) and placed into greased trays (9.1 × 18 cm of width and length dimensions). V) WB samples were fermented for 60 min at 37°C in a fermentation chamber. VI) WB samples were baked for 60 min at 160°C in an electric convection oven. The samples were cooled down for 1 hour and stored in plastic food bags.
The equipment used in the bread-making process were Bimby® TM31 thermoprocessor (Wuppertal, Germany), Arianna XLT133 Unox® fermentation chamber (Cadoneghe, Italy), and Arianna XFT133 Unox® electric convection oven (Cadoneghe, Italy). The ingredients’ proportion can be seen in Table 1, according to preliminary tests based on the study of Graça et al. (2018).
The WB image samples were captured using a mobile camera (SAMSUNG® S 22 Plus). The binary images were set in the central point of each image with a resolution of 72 dpi, both horizontal and vertical. The images were analyzed using ImageJ (Available at: http://rsb.info.nih.gov/ij/) as described by Rahimi et al. (2020).
2.5 Wheat bread physical property evaluation during storage time
The specific volume was determined as a ratio between the volume and weight according to the AACC Method 10–05.01 (AACC, 2010). The crumb moisture content was measured in an air oven at 100°C, and the baking loss was calculated after 48 h, according to the AACC Method 44–15.02 (AACC, 2009). All the physical analyses for estimating bake loss and crumb moisture were performed in triplicate and at six replicates for specific volumes. The aw at 20°C of bread samples were measured in triplicate using a HygroPalm HP23 portable water activity analyzer (Rotronic®, São Paulo).
The texture of WB was determined by texture profile analysis (TPA) using a TA-XTplus texturometer (Stable MicroSystems®, Surrey, United Kingdom), with a load cell of 5 kg. TPA consists of double compression of a piece of food that mimics the action of the jaw, as described by Graça et al. (2018). Each WB sample was cut into a 20-mm-thick slice, and the analysis was performed with an acrylic cylindrical probe of 10 mm diameter piercing 5 mm of the sample at 1 mm/s of crosshead speed, with a resting time of 5 s between cycles. The texture profile of WB, WB-SB, and WB-RB samples was compared regarding hardness (N) and cohesiveness (dimensionless), considered the most representative parameters in these samples, calculated by the TA.XTplus software. The measurements were repeated six times for each sample at 0 h, 24 h, and 48 h after bread production.
The WB crumb and crust samples were evaluated in a portable Chroma-Meter CR400 colorimeter (Konica Minolta®, Japan) according to the CIE L*a*b* system (International Commission on Illumination) using the color parameters L*, a*, and b*, where L* indicates a brightness of 0 (blackness) or 100 (whiteness), a* indicates the degree of redness (+60) or greenness (−60), and b* indicates the degree of yellowness (+60) or blueness (−60). The measurements were replicated 9 times. Data were demonstrated by the total color difference (ΔE) that was measured as in Eq. 1. Lo*, ao*, and bo* were the values obtained from WB:
The color differences between the samples were classified as ΔE ≤ 1—imperceptible by human eyes; 1 < ΔE ≤ 2—cognizable by close observation; 2 < ΔE ≤ 10—cognizable at a glance; 10 < ΔE ≤ 50—a clear difference noticeable in colors; ΔE ≥ 50—exactly two different colors (Li et al., 2017).
2.6 Proximate composition, phenolic compounds, and antioxidant activity of wheat bread samples
The proximate composition of WB samples was determined through the American Association of Cereal Chemists with adaptations, formerly the AACC International Method (AACC, 2023). The ash, fat, protein, and mineral contents were analyzed in dried WB samples in triplicate. The ash content was determined by muffle furnace combustion at 550°C overnight. Soxhlet extraction with petroleum ether as the solvent at a medium temperature for 6 h determined the total fat content. The protein content was determined using the Dumas method (Thermo Quest® NA 2100 Nitrogen and Protein Analyzer, Interscience, Breda, Netherlands), considering the protein-to-nitrogen conversion factor of 6.25. The mineral and heavy metal profiles were evaluated by inductively coupled plasma optical emission spectrometry—ICP-AES (Thermo Fisher® Scientific iCAP PRO-7000 Series, Massachusetts, United States) (Khemiri et al., 2021). The total content of carbohydrates was calculated by difference as in Eq. 2:
The phenolic compound and antioxidant activity analysis was performed in triplicate. Methanolic (80%) extracts of WB, WB-SB, and WB-RB dried samples were resuspended in 20% DMSO. The final extracts were concentrated at 20 mg/mL. The total phenolic compound content was determined by the Folin–Ciocalteu methodology, as described by Mohankumar et al. (2018). The antioxidant activity was performed against two free radicals: DPPH by Brand-Williams et al. (1995) and FRAP by Benzie and Strain (1996).
2.7 Bioaccessibility of wheat bread: in vitro digestion by the INFOGEST protocol
The in vitro digestibility of WB, WB-SB, and WB-RB samples was evaluated following the INFOGEST protocol described by Minekus et al. (2014) and Brodkorb et al. (2019). The INFOGEST—in vitro tool was used to calculate the solution amount in each stage (available at: http://www.proteomics.ch/IVD/). Thus, 2.5 g of each sample and blanks were measured in triplicate, and the phases were run to mimic digestion: a) oral phase (2 min at pH 7)—with simulated salivary fluid (SSF) containing amylase. The samples required adding more water to allow their homogenization to form a paste-like consistency. The water quantities were noted; b) gastric phase (2 h at pH 3)—the bolus was diluted with simulated gastric fluid (SGF) containing the gastric enzyme pepsin; c) intestinal phase (2 h at pH 7)—the simulated intestinal fluid (SIF) containing pancreatin and bile salts was added into the mixture. The entire protocol was performed at 37°C in a continuous and gentle mixing process in a rotary agitator.
Then, the intestinal phase was stopped using protease inhibitor 4-(2 aminoethyl) benzensulfonylfluoride (Pefabloc®, 500 mmol/L, Roche, Basel, Switzerland). Then, the samples were finally centrifuged (8.000 rpm at 10°C for 10 min), and the undigested pellets were collected and dried in Falcon tubes at 80°C for 3 h and then at 45°C for 1 h until constant weight. The digested part (supernatant) was filtered using a Buchner funnel with Whatman® qualitative filter paper (125 mm). The filter paper was weighed before and after filtering the contents of each Falcon tube. The crude protein in vitro digestibility (IVD %) of wheat bread (WB, WB-SB, and WB-RB) and raw material (SB and RB) was calculated from the difference between the initial biomass and the undigested biomass, divided by the initial biomass and multiplied by 100, as described by Khemiri et al. (2021).
2.8 Statistical analysis
Analyses of variance between samples were performed by one-way ANOVA with Tukey’s post-hoc test at a significance level of 95% (p < 0.05) using the STATISTICA 8.0 software (StatSoft, Inc., Tulsa, United States). The percentage variation (PV) was calculated for firmness using Eq. 3:
The Windows version of ImageJ was used for measurement of WB binary images of alveolar cell structure (n = 150), performed independently, and expressed as the mean and ± standard deviation.
3 Results and discussion
3.1 Impact of Spirulina fractions (SB and RB) in wheat flour dough samples
The rheological characteristics of the doughs with or without Spirulina counterparts, described in Table 2, showed that the water absorption (%) required in WB-RB was higher than in WB and WB-SB, which showed the same significant value to reach the predetermined standard torque value of 130 mN.m, which is the ideal torque for wheat bread dough. Amoriello et al. (2021) showed that functional bread with Spirulina requires the same values for water absorption as described in our study, between 50.9% and 53.9%.
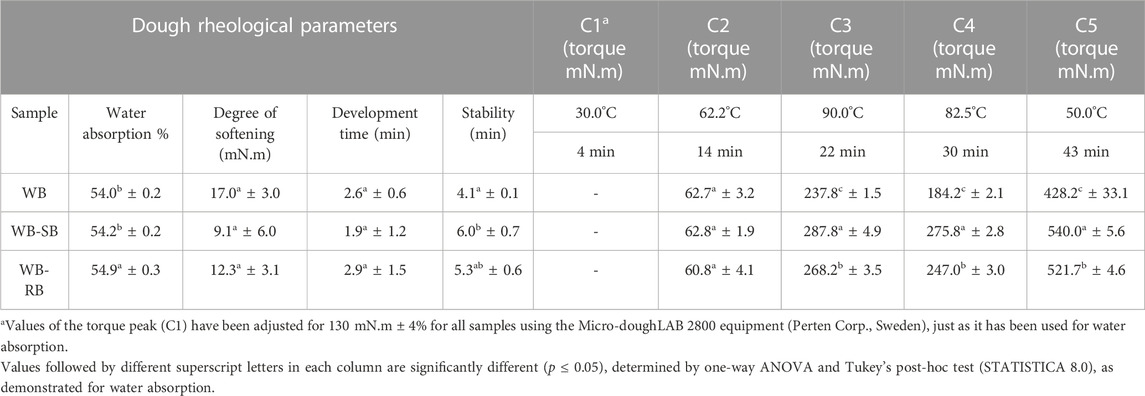
TABLE 2. Rheological parameters and pasting curves (C1–C5 with standardized time and temperature) of developed dough in Micro-doughLAB values are shown in mean ± standard deviation.
The differences between the water absorption capacity of the samples (WB, WB-SB, and WB-RB) could be explained by the addition of other protein sources, such as SB and RB in wheat flour, which compete for water with other components in the dough system, resulting in doughs with increased farinograph water absorption, as described by Graça et al. (2018) with the addition of Chlorella vulgaris in different concentrations (1.0, 2.0, 3.0, 4.0, and 5.0 g/100 g of wheat flour). With values higher than 3 g, the amount of water required was high. In addition, water quantity is an important factor to be adjusted for the distribution of the dough ingredients, their hydration, and the gluten protein network development, as well as to increase the production of yield (Graça et al., 2018).
The results for degrees of softening (the difference in torque between the maximum torque and the medium line of the curve 8 min after the development time) and the dough development time (time to reach the torque value of 130 mN.m) were similar for all samples; thus, the addition of SB or RB did not impact these dough characteristics. The dough stability time was higher in WB–SB than in WB, which suggests that SB affected the tolerance of the flour to withstand torque, which resulted in a positive effect on the physical properties of bread, such as higher specific volume and lower bake loss for WB-SB and WB-RB, which is in line with the results of Amoriello et al. (2021), due to the presence of hydrocolloids, mainly alginate, agar, and carrageenan. However, it is different from what was shown by Graça et al. (2018) with Chlorella vulgaris in higher concentrations (≥3%), whereby the protein content of wheat flour was somehow disrupted, which resulted in lower dough strength and loaf volume, which had a negative effect on the quality characteristics of bread.
It is important to know that the course of wheat dough behavior was described by five basic torque points C1–C5, expressed in mN.m (AACC, 2010). The results showed that until C2, the dough behavior was equal among the samples. However, in C3, C4, and C5, the SB and RB impacted with higher values, and WB showed the lowest values. These points (C3 to C5) are related to bread characteristics (Table 2).
It is known that Spirulina interferes in the starch gelatinization process because the components in this food matrix are mainly polysaccharides, considering its origin and quantity (Amoriello et al., 2021). Other factors depend on the particle properties, such as size and distribution, morphology, and hardness (Martínez-Sanza et al., 2020). The particle size of the added raw materials (SB ≤ 106 µm and RB > 106 µm) was not standardized, which could impact pasting curve parameters as the nutritional profile of the dough with SB and RB addition.
The mechanical spectra presented in (Figure 1A) suggest that adding 3% of SB or RB did not interfere with the rheological parameters of unleavened dough of WB, WB-SB, and WB-RB. All samples showed a higher elastic behavior (G′) than a viscous behavior (G″), which can be evidenced by Figure 1B. This demonstrates that the tan <1 corresponds to a predominant elastic behavior for all samples. It is essential to highlight that this viscoelastic behavior depends on the frequency, usually associated with poorly structured systems, as seen in pasta with Spirulina (Fradinho et al., 2019). The values of G′ at 6.283 rad/s (1 Hz) and 62.83 rad/s (10 Hz) were similar (p > 0.05 ANOVA and Tukey’s post-hoc test) for all samples, suggesting that the Spirulina counterpart’s addition did not impact the elastic behavior of doughs.
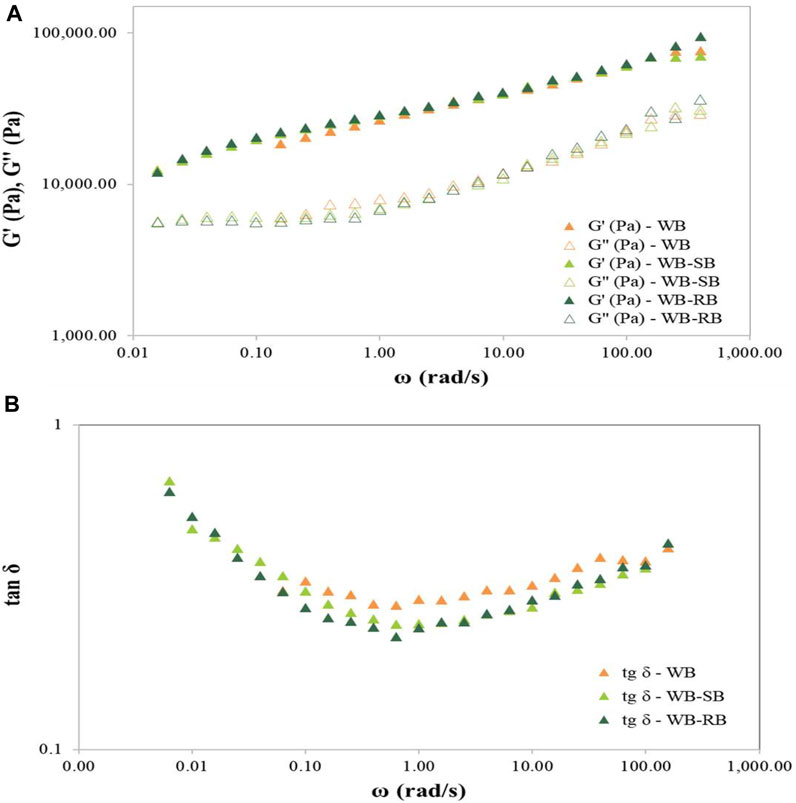
FIGURE 1. Mechanical spectra (A) and tan δ vs. angular frequency (B) of wheat bread dough with 3% of Spirulina biomass (WB-SB) or residual biomass (WB-RB) compared to the control wheat bread (WB). G′ corresponds to the elastic modulus, and G″ corresponds to the viscous modulus. The test was performed in triplicate, and the most representative curve for each sample is presented.
Nunes et al. (2020a) demonstrated that the incorporation of Chlorella vulgaris at 1% in wheat bread led to higher G′ values, suggesting that the microalgae caused a strengthening of the dough structure, and the dough with the incorporation of Tetraselmis chuii showed lower G′ values than the control (Nunes et al., 2020b), suggesting a destabilization of the structure by the addition of microalgae, contrary to what was observed in this study that WB with SB or RB, or without Spirulina, showed the same performance. Thus, this behavior is in accordance with Spirulina suspensions (more elastic) in comparison to other algae Microchloropsis gaditana and Scenedesmus almeriensis, with more viscous (liquid) rheological characteristics (Martínez-Sanza et al., 2020).
Dough pH demonstrated an increase with the addition of 3% of Spirulina fractions SB (5.63a ± 0.05) or RB (5.70a ± 0.05) when compared to WB (5.42b ± 0.05). Differently from the study conducted by Garzon et al. (2021), it was observed that the wheat flour doughs, conventionally prepared using compressed baker’s yeast, demonstrated superior pH when compared to the dough samples with the addition of 1, 2, or 3% of C. vulgaris powder. However, it is essential to note that the algae type influences the pH behavior and the type of yeast, which are different between the studies. Morsy et al. (2014) highlighted that Spirulina presents a pH of 6.93, which can enhance food pH levels. As we could see in this study, this can be stated because Spirulina is naturally found in tropical regions with a high concentration of NaCl and bicarbonates, mainly found in inhabiting alkaline lakes (pH 11) (Volkmann et al., 2008).
3.2 Physicochemical properties of wheat bread samples with or without Spirulina fractions (SB and RB)
The inclusion of SB or RB in WB development did not affect the aw and crumb moisture of the produced bread, indicating that the addition of Spirulina and its counterpart does not imply these two physicochemical properties of bread. The same results were found by García-Segovia et al. (2017) with the addition of different types of algae in wheat bread formulation (Isochrysis galbana, Tetraselmis suecica, Scenedesmus almeriensis, and Microchloropsis gaditana). In rice flour gluten-free bread with 1% or 4% of Spirulina and additives in different concentrations (1.5 or 2.2% of methylcellulose and 0.2 or 0.6% of transglutaminase), the average crumb moisture was 45%, as shown by Belkina et al. (2022a) with the addition of 0.5% of Spirulina in wheat bread formulations, a little bit higher than what was found in this paper.
The specific volume, considered a bread expansion measurement, was positively affected by SB and RB addition, as described by Amoriello et al. (2021), adding 1, 2.5, or 4% of Spirulina in the soft wheat bread development. These differences in outputs with SB and RB addition could be due to the extensibility enhancement and gluten network improvement related in the literature. In addition, the presence of algae could positively affect specific volumes, except for alginate, mainly because of polysaccharides and fiber content (Rosell et al., 2001; Belkina et al., 2022b).
One reason for the cessation of dough expansion during baking is the resistance of the dough to extension, which depends on rheological properties like elasticity and viscosity. Starch gelatinization in the cell membranes occurs above 65°C, increases dough viscosity, and impairs the extensibility of the dough, which results in increased pressure in closed gas cells, leading to rupture of the cell membranes. As a result, the gas molecules will exchange between adjacent cells and ultimately be transported to the outside of the dough, resulting in a loss of gas and presumably limited capacity for expansion (Mondal and Datta, 2008), as seen in WB. The bake loss of WB was higher than that in bread with the SB and RB addition, as shown in Table 3.
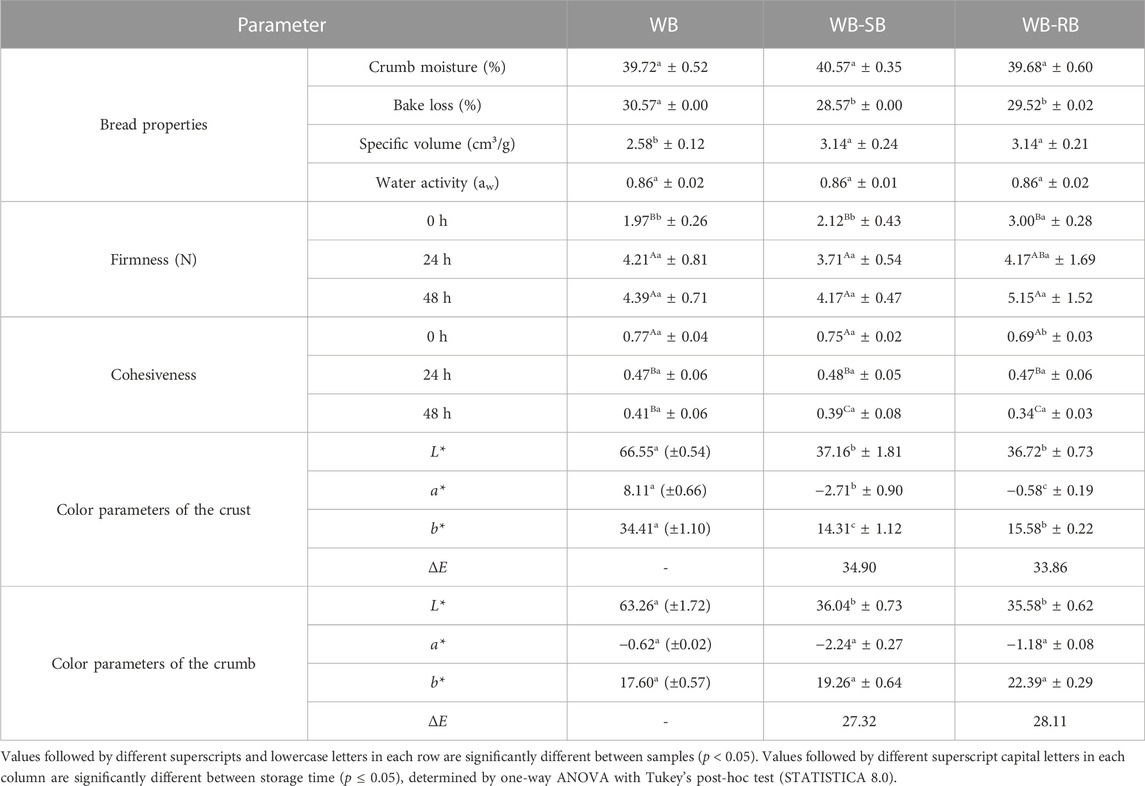
TABLE 3. Means ± standard deviation of the physical properties of wheat bread (WB) formulations with 3% of Spirulina counterparts (WB-SB and WB-RB) or without (WB).
These results disagree with the results of Montevecchi et al. (2022), which indicated that the % weight loss during baking was similar in wheat bread with or without Spirulina addition, demonstrating a baking loss reduction of around 13.76%, whereas in our study, the baking loss (%) was higher (around 30%).
As Kotoki and Deka (2010) highlighted, when a high percentage of moisture is lost, bread will present a dry crust with less weight, which we can see in the WB, WB-SB, and WB-RB samples. The inclusion of SB and RB helped in the reduction of baking loss, which is still high, which confirms that the addition of baking agents and dough improvers may overcome these problems, as well as can help with the freshness maintenance of bread and other baked goods, which age earlier and become stale because of a high moisture loss (Kohajdová and Karovičová, 2009; Moayedallaie et al., 2010).
Hardness (bread firmness) represents the maximum resistance to probe penetration, and cohesiveness is recognized as the force that can deform a material before it breaks; in other words, it is the strength of the internal bonds that make up the body of the product (Rosenthal and Thompson, 2021), which is obtained using the area of the first bite divided by the area of the second bite.
The results of WB and WB-SB firmness (N), demonstrated in Table 3, at 0 h of storage were lower when compared to that of WB-RB, indicating that adding 3% of RB negatively affected bread firmness, making the bread crumb harder. Graça et al. (2018) found similar results at 0 h time with C. vulgaris addition, instead of Spirulina, showing that the added algae concentration did not influence bread firmness. However, for RB, the conclusions have been controversial, and it is a matrix that is poorly elucidated in the literature.
Bread firmness at 24 h and 48 h showed similarity after 24 h storage time in all samples. However, it is crucial to highlight that the percentage variation of WB showed the highest increase in bread firmness during 48 h (55.12%), the lowest for WB-RB (41.71%), and moderate for WB-SB (49.17%), which can also justify the use of Spirulina and its counterparts to help in bread firmness during 48 h of the storage period, which was differently observed by Graça et al. (2018) during 72 h, that showed a competition for water absorption by the C. vulgaris in the wheat bread that should cause acceleration in the aging kinetics of bread, expressed in terms of firmness increase within short time.
Bread cohesiveness showed higher and similar results for WB and WB-SB, the same as described by Sanjari et al. (2018) with Spirulina powder in baguette bread; comparable results of bread cohesiveness were also found in sourdough wheat bread made by García-Segovia et al. (2017), and lower results of bread cohesiveness were found for WB-RB. After 48 h of storage, all values become similar for all samples, with a percentage of variation (PV) between 47% for WB and 50% for WB-RB, which indicates that after 48 h, bread with Spirulina and its counterparts needed less force to deform before it breaks when compared to WB. These changes may be due to several factors related to aging in bread hardness and cohesiveness, such as starch retrogradation, protein denaturation, decrease in moisture content, and formation of the bond between starch and protein (Sanjari et al., 2018).
In addition to the use of Spirulina as an ingredient in the development of functional foods, these cyanobacteria are also used as a marketing strategy or as a coloring agent due to the presence of colored compounds such as chlorophylls, carotenoids, and phycobiliproteins, which is among the top trends in the food industry these days (Lafarga et al., 2020).
As shown in Table 3, WB bread has a whiter crust and crumb (L*); WB-SB and WB-RB have greener crust and crumb, although WB also showed negative values for a* WB, with SB and RB being higher; and the crust of WB is yellower than the other samples (b*), but for the crumb, all samples showed yellowness with positive values for b*. ΔE of WB-SB and WB-RB, when compared to WB, demonstrated that 10 < ΔE ≤ 50; this can guarantee that the color difference is clearly perceived by human eyes, which can be seen in Figure 2. Other studies show these color differences in algae products (Batista et al., 2019; Khemiri et al., 2020; Lafarga et al., 2020).
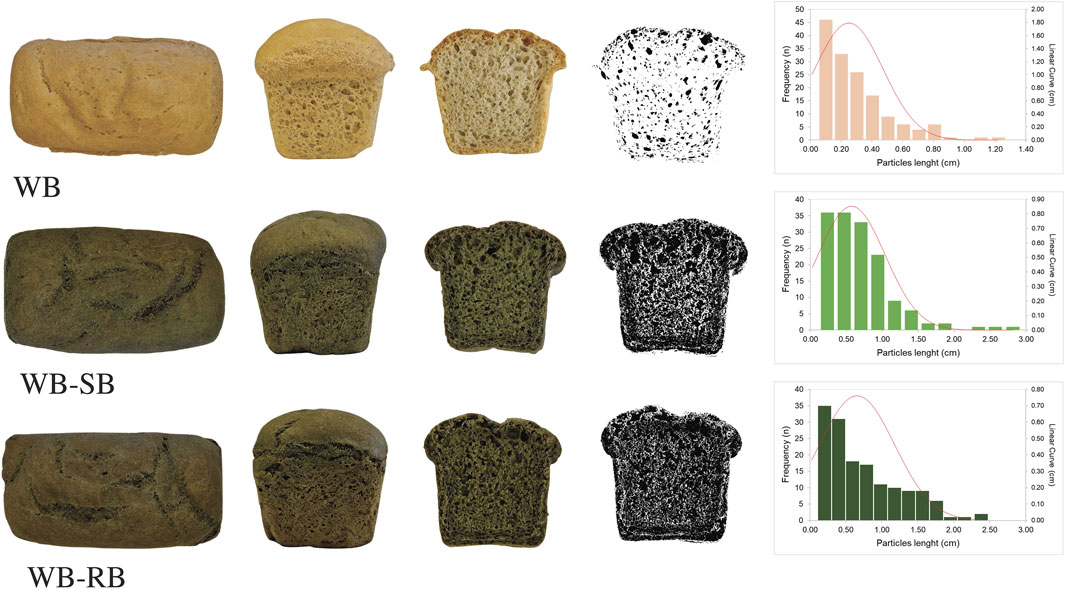
FIGURE 2. Representative images. Binary images in black and white of the region of interest (ROI) to represent the whole surface of slices of each bread loaf (72 × 72 DPI) and frequency (n) of particle length (cm) of formulated WB, WB-SB, and WB-RB samples.
3.3 Nutrition profile, phenolic compounds, and antioxidant activity of wheat bread
From the results shown in Table 4, it could be observed that the carbohydrate and ash content in WB, without the Spirulina counterparts, showed higher values. This may indicate that substituting 3% of wheat flour—in flour basis—for SB or RB influenced the amount of these nutrients. Ash is one of the most critical indicators of wheat flour’s quality and use and consists of mineral compounds such as phosphorus (P), potassium (K), calcium (Ca), magnesium (Mg), iron (Fe), zinc (Zn), and copper (Cu) (Czaja et al., 2020). Thus, the ash values are high because its main constituents, Fe and K, were around 1% and 45% in ash analysis, respectively, which were also higher in WB. Boron (B) was higher in WB than in WB-SB and WB-RB.
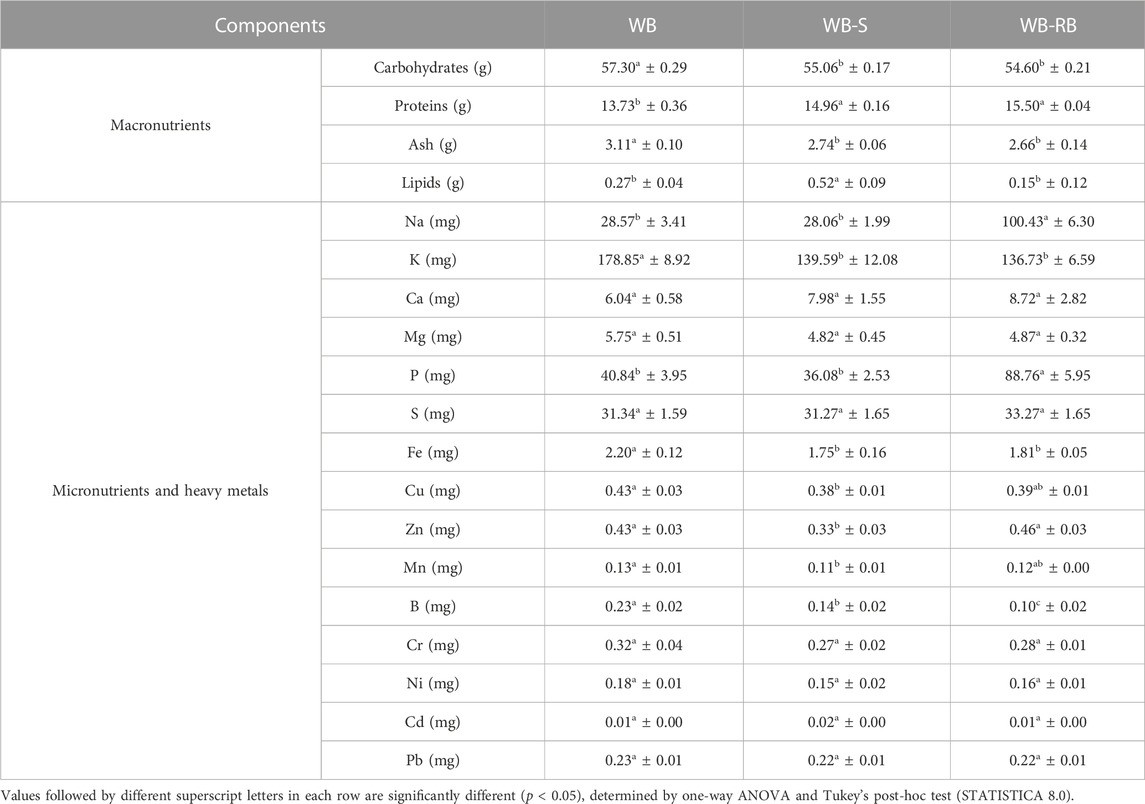
TABLE 4. Macronutrients (g/100 g of dried sample), micronutrients, and heavy metals (mg/100 g of dried sample) of control wheat bread (WB) formulated bread with 3% of Spirulina biomass (WB-SB) and 3% of residual biomass (WB-RB). The results are demonstrated as average ± standard deviation.
The total proteins of SB and RB raw materials, in dry weight, used in this study are 57.54 (±0.24) and 66.58 (±0.39) g/100 of dried samples, respectively. The result for SB agrees with that found in the literature (ranging from 17% to 73%, more commonly up to 60%, but with high nutritional variability, requiring further optimization of cultivation and post-processing conditions) (Muys et al., 2019). The proximate composition of RB was not found in the literature, but the increase of protein in this raw material could be explained by the dry matter concentration, even without C-PC phycobiliproteins after extraction. For this reason, WB-SB and WB-RB showed higher values of proteins than WB. The lipid content of SB ranges between 9% and 17% (Muys et al., 2019), and in wheat flour, it ranges between 0.1% and 2.1% (Prabhasankar and Rao, 1999); hence, WB-SB showed higher lipid values than WB and WB-RB. The C-PC extraction possibly influenced the lipid content in RB, which could explain the lower lipid values in bread.
Na and P contents are higher in the WB-RB sample, which could not be explained by Na and P values of raw materials that showed 1333.38 mg and 1120.39 mg of Na in SB and RB, respectively, and 963.18 mg and 664.51 mg of P in SB and RB respectively. Cu, Zn, and Mn were higher in WB and WB-RB samples. One of the hypotheses for WB mineral higher levels is the concentration of minerals, due to greater water loss in WB. None of the bread samples showed relevant heavy metals such as Ni, Cd, and Pb values.
FRAP analysis (Table 5) showed higher results in WB-SB and WB-RB. DPPH showed higher values in WB-SB. However, the analysis of total phenolic compounds showed the highest values in WB-RB. The lowest values for antioxidant analysis through FRAP and DPPH radicals and phenolic compounds were found in WB; therefore, 3% of SB and RB in substituting wheat flour for wheat bread development can improve the antioxidant capacity and phenolic compounds. This can be explained because the antioxidant properties of Spirulina are attributed to molecules such as phycocyanin, β-carotene, tocopherol, γ-linolenic acid, and phenolic compounds (Kumar et al., 2022), that showed inhibitory activity against lipid peroxidation radical scavenging and metal chelating potential (Bermejo et al., 2008).
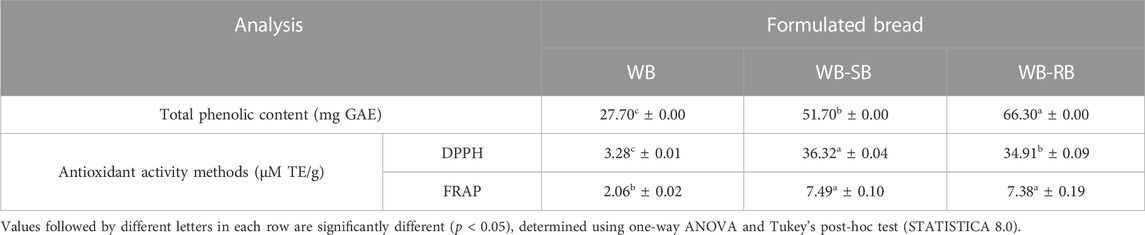
TABLE 5. Total phenolic content and antioxidant activity of three formulated wheat breads with (WB-SB and WB-RB) or without (WB) Spirulina counterparts. The results are shown as average (± standard deviation) made with 1 g of dry material bread samples.
Spirulina is a photosynthetic organism and, like all photosynthetic organisms, has developed defense mechanisms to protect the chloroplast from reactive oxygen species. Despite Spirulina having low concentrations of phenolic compounds, there are still some in its cellular matrix; few studies have recently investigated the role of phenolic compounds in algae and microalgae (Kepekçi and Saygideger, 2012). Even in low concentrations, the phenolic compounds were higher in WB-SB and WB-RB than in WB, which could also enhance antioxidant capacity.
3.4 Image analysis of wheat bread with or without Spirulina counterparts
It was possible to develop WB by adding 3% of SB or its counterpart, RB, as seen in the WB images in Figure 2, where we can also see the binary images of the WB samples with measurements of the alveolar cell structure (n = 150) of each loaf. It was possible to establish that the particle length of WB-RB, in general, and the average size was larger (0.65 cm ± 0.52), showing a maximum and minimum length of 2.38 cm and 0.05 cm, respectively. WB-SB showed similar measurements to WB-RS, with an average size of 0.57 cm ± 0.47, a maximum length of 2.82 cm, and a minimum length of 0.02 cm. WB had the smallest measurements, with an average of 0.57 cm ± 0.47, a maximum size of 1.22 cm, and a minimum size of 0.01.
In addition, as described by Rahimi et al. (2020), dough rheological characteristics and gas cell stability are the two factors that are responsible for impacting the baking process and bread quality. Gluten protein in wheat flour helps generate a strong dough network that retains the incorporated air, prevents the product from collapsing, and maintains a nice porous structure. The pore size homogeneity delivers a product with an appealing texture, appearance, and other quality characteristics. The main ingredients incorporated in developing bakery products are flour, water, and chemical or biological leavening ingredients, and the gas cells are occluded as small nuclei in the dough (Rahimi et al., 2020). SB and RB helped maintain the bread structure.
3.5 In vitro digestibility of bread and raw material for bread production
In vitro digestibility, also known as bioaccessibility, as described by Demarco et al. (2022), is related to the digestive transformations in food through the use of enzymes to understand the maximum fraction from food matrix release into the digestive tract to measure the intestinal absorption and pre-systematic metabolism of food. The method used in this paper to analyze the protein bioaccessibility in WB samples, INFOGEST static in vitro simulation, is the most reliable and standardized protocol for bioaccessibility analysis in food samples (Minekus et al., 2014). Therefore, more studies are needed to comprehend microalgae digestibility in food products (Demarco et al., 2022).
In Figure 3, it can be seen that protein in vitro digestibility (IVD) in dry matter was similar in all WB samples (an average of 94%), but it was lower in raw materials SB and RB (an average of 57%). After digestion, the values of IVD (%) were similar in all WB samples (WB, WB-SB, and WB-RB), with an average of 81% lower than in dry matter. This also happened in raw materials (SB and RB), representing an average IVD (%) of 56% with no differences between SB and RB. Some studies have demonstrated the digestibility of proteins in Spirulina food products, as cited in the review by Demarco et al. (2022), such as chocolate biscuits (86%—with 1% of Spirulina biomass), snacks (89%—with 2.6% of Spirulina biomass), and bread wheat pasta (showed an increase in protein digestibility; the authors did not show the percentage).
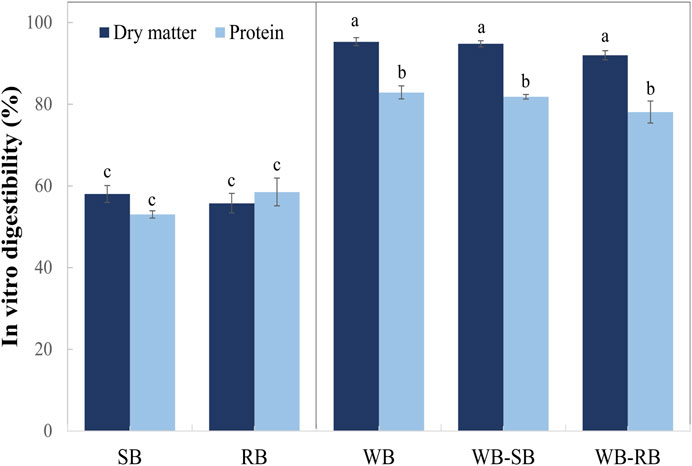
FIGURE 3. In vitro digestibility (%) of Spirulina microalgae fractions (Spirulina biomass—SB and residual biomass—RB) and wheat bread with (WB-SB and WB-RB) or without (WB) Spirulina counterparts. The colored bars are the average, and the error bars are the standard deviation. Different letters in each sample indicate significant differences between samples by ANOVA and Tukey’s post-hoc test (p < 0.05).
Spirulina biomass showed a protein digestibility of 82% in other studies, higher than that observed in our study (Demarco et al., 2022). Moreover, none of the studies showed similar results to wheat bread with Spirulina counterparts, but as shown in this study with WB samples, no differences were found between microalgae cookies and the control (IVD 87%–95%) (Batista et al., 2017). The nature of the formulated product can explain these results. The pre-treatment in Spirulina biomass may facilitate cell disruption, and the cooking techniques could help the protein digestibility of microalgae (Demarco et al., 2022).
It should be emphasized that introducing Spirulina and its counterparts in recipes requiring algae addition could be easier in human diet acceptance. The replacement of wheat flour by Spirulina fractions (SB and RB) does not decrease the protein digestibility, which is an interesting finding in this study, so even the RB (residual biomass of Spirulina) is digestible, but not yet appreciated.
4 Conclusion
SB and RB can be applied as innovative ingredients to replace 3% of wheat flour in wheat bread development. It can be noted that both raw materials from microalgae affected the technological aspects of WB in a positive way, mainly in the rheological parameters of the dough and quality properties of WB. SB and RB enhanced the nutritional profile, with the highest values of proteins, some minerals, and phenolic compounds in WB-BS and WB-RS compared to the control WB, improving the antioxidant capacity. Heavy metals (Ni, Cd, and Pb) were not found in any of the samples (values <0.2 mg/100 g of dried samples).
Moreover, the in vitro protein digestibility was better in WB than in the raw materials (SB and RB), which indicates that the bread-making process may help with protein digestibility. However, no difference was found in the digestibility of bread with or without the addition of 3% of SB or RB, which indicates that RB is digestible, but has not yet been valued. Therefore, the authors expected this work to draw researchers’ attention to RB, an unexplored ingredient. In addition, SB needs greater appreciation for sustainable food practices worldwide and in Brazilian exploration, requiring management strategies involving the industry and society working together. It is essential to highlight that further studies focusing on acceptability are needed to understand the viability of these ingredients in targeting consumers’ preferences.
Data availability statement
The raw data supporting the conclusion of this article will be made available by the authors, without undue reservation.
Author contributions
CF: Data curation, Methodology, Writing–original draft, Investigation, and Writing–review and editing. MN: Data curation, Writing–original draft, Writing–review and editing, and Investigation. VD: Conceptualization, Methodology, Visualization, and Writing–review and editing. AR: Data curation, Supervision, Validation, Writing–original draft, and Writing–review and editing. AB: Conceptualization, Investigation, Methodology, Supervision, Visualization, and Writing–review and editing.
Funding
The author(s) declare financial support was received for the research, authorship, and/or publication of this article. This work was financially supported by “Coordenação de Aperfeiçoamento de Pessoal de Nível Superior—Brasil (CAPES)” through the scholarship process no. 88887.364166/2019-00. This study was also supported by “Fundação de Amparo à Pesquisa do Estado de São Paulo—FAPESP” through grant process nos. 2023/00857-0, 2022/06293-9, and 2020/06732-7.
Acknowledgments
The authors thank “Fazenda Tamanduá®” for providing organic powdered Spirulina and the Laboratory of Food Bioactive Compounds of the Universidade Federal de São Paulo (UNIFESP). The authors also thank FCT—Fundação para a Ciência e a Tecnologia, I.P., under the project UIDB/04129/2020 of LEAF—Linking Landscape, Environment, Agriculture, and Food Research Center.
Conflict of interest
The authors CN, AR and ARCB declared that they were editorial board members of Frontiers at the time of submission. This had no impact on the peer review process and the final decision.
The remaining authors declare that the research was conducted in the absence of any commercial or financial relationships that could be construed as a potential conflict of interest.
Publisher’s note
All claims expressed in this article are solely those of the authors and do not necessarily represent those of their affiliated organizations, or those of the publisher, the editors, and the reviewers. Any product that may be evaluated in this article, or claim that may be made by its manufacturer, is not guaranteed or endorsed by the publisher.
References
AACC (2023). Approved methods of analysis. 11. Available at: https://www.cerealsgrains.org/resources/Methods/Pages/default.aspx (Accessed September 17, 2023).
AACC International (2010). “Guidelines for measurement of volume by rapeseed displacement,” in AACC international approved methods 10-05.01 (St. Paul, MN, USA: AACC International).
AACC International Method (2010). Determination of rheological behavior as a function of mixing and temperature increase in wheat flour and whole wheat meal by Mixolab. AACC Int. Method 54-60, 1–6. doi:10.1094/AACCIntMethod-54-60.01
AACC International Method (2014). High-Speed mixing rheology of wheat flour using the doughLAB. AACC Int. Method 54-70, 1–5. doi:10.1094/AACCIntMethod-54-70.01
AACC International (2009). “Moisture- air-oven methods,” in AACC international approved methods international 44-15.02 (St. Paul, MN, USA: AACC International), 1–4. doi:10.1094/aaccintmethod-44-15.02
Amarante, M. C. A., and Braga, A. R. C. (2021). Biofuels and oils from amazon crops: challenges and opportunities for the sustainable use of biodiversity resources. Ind. Biotechnol. 17, 204–213. doi:10.1089/ind.2021.0005
Amarante, M. C. A., Braga, A. R. C., Sala, L., and Kalil, S. J. (2020). Colour stability and antioxidant activity of C-phycocyanin-added ice creams after in vitro digestion. Food Res. Int. 137, 109602. doi:10.1016/j.foodres.2020.109602
Amoah, I., Cairncross, C., Osei, E. O., Yeboah, J. A., Cobbinah, J. C., and Rush, E. (2022). Bioactive properties of bread formulated with plant-based functional ingredients before consumption and possible links with health outcomes after consumption- A review. Plant Foods Hum. Nutr. 77, 329–339. doi:10.1007/s11130-022-00993-0
Amoriello, T., Mellara, F., Amoriello, M., Ceccarelli, D., and Ciccoritti, R. (2021). Powdered seaweeds as a valuable ingredient for functional breads. Eur. Food Res. Technol. 247, 2431–2443. doi:10.1007/s00217-021-03804-z
Asioli, D., Aschemann-Witzel, J., Caputo, V., Vecchio, R., Annunziata, A., Næs, T., et al. (2017). Making sense of the “clean label” trends: A review of consumer food choice behavior and discussion of industry implications. Food Res. Int. 99, 58–71. doi:10.1016/j.foodres.2017.07.022
Banwo, K., Olojede, A. O., Adesulu-Dahunsi, A. T., Verma, D. K., Thakur, M., Tripathy, S., et al. (2021). Functional importance of bioactive compounds of foods with potential health benefits: A review on recent trends. Food Biosci. 43, 101320. doi:10.1016/j.fbio.2021.101320
Batista, A. P., Niccolai, A., Bursic, I., Sousa, I., Raymundo, A., Rodolfi, L., et al. (2019). Microalgae as functional ingredients in savory food products: application to wheat crackers. Foods 8, 611. doi:10.3390/foods8120611
Batista, A. P., Niccolai, A., Fradinho, P., Fragoso, S., Bursic, I., Rodolfi, L., et al. (2017). Microalgae biomass as an alternative ingredient in cookies: sensory, physical and chemical properties, antioxidant activity and in vitro digestibility. Algal Res. 26, 161–171. doi:10.1016/j.algal.2017.07.017
Belkina, R. I., Letyago, Y. A., Gryaznova, O. A., and Gryaznov, A. A. (2022a). “Development of bread recipes based on natural fortifiers,” in IOP conference series: earth and environmental science (Bristol, United Kingdom: IOP Publishing Ltd). doi:10.1088/1755-1315/954/1/012045
Belkina, R. I., Letyago, Y. A., Gryaznova, O. A., and Gryaznov, A. A. (2022b). “Development of bread recipes based on natural fortifiers,” in IOP conference series: earth and environmental science (Bristol, United Kingdom: IOP Publishing Ltd). doi:10.1088/1755-1315/954/1/012045
Benzie, I. F. F., and Strain, J. J. (1996). The ferric reducing ability of Plasma (FRAP) as a measure of “‘Antioxidant power’”: the FRAP assay.
Bermejo, P., Piñero, E., and Villar, Á. M. (2008). Iron-chelating ability and antioxidant properties of phycocyanin isolated from a protean extract of Spirulina platensis. Food Chem. 110, 436–445. doi:10.1016/j.foodchem.2008.02.021
Braga, A. R. C., de Rosso, V. V., Harayashiki, C. A. Y., Jimenez, P. C., and Castro, Í. B. (2020). Global health risks from pesticide use in Brazil. Nat. Food 1, 312–314. doi:10.1038/s43016-020-0100-3
Braga, A. R. C., Nunes, M. C., and Raymundo, A. (2023). The experimental development of emulsions enriched and stabilized by recovering matter from spirulina biomass: valorization of residue into a sustainable protein source. Molecules 28, 6179. doi:10.3390/molecules28176179
Brand-Williams, W., Cuvelier, M. E., and Berset, C. (1995). Use of a free radical method to evaluate antioxidant activity.
Brodkorb, A., Egger, L., Alminger, M., Alvito, P., Assunção, R., Ballance, S., et al. (2019). INFOGEST static in vitro simulation of gastrointestinal food digestion. Nat. Protoc. 14, 991–1014. doi:10.1038/s41596-018-0119-1
Burcu, A., Avşaroğlu, E., Işık, O., Özyurt, G., Kafkas, E., Etyemez, M., et al. (2016). Nutritional and physicochemical characteristics of bread enriched with microalgae Spirulina platensis. Available at: www.ijera.com.
Burt, K. G., Fera, J., and Lewin-Zwerdling, A. (2021). Differences in US adults’ value of and preferences for sustainable food by race/ethnicity, income, and education. J. Hunger Environ. Nutr. 16, 321–335. doi:10.1080/19320248.2020.1823927
Capelli, B., and Cysewski, G. R. (2010). Potential health benefits of Spirulina microalgae*. Nutrafoods 9, 19–26. doi:10.1007/BF03223332
Czaja, T., Sobota, A., and Szostak, R. (2020). Quantification of ash and moisture in wheat flour by Raman spectroscopy. Foods 9, 280. doi:10.3390/foods9030280
Dang, J. M. C., and Bason, M. L. (2015). “Comparison of old and new dough mixing methods and their utility in predicting bread quality,” in Proceedings of the 65th Australian Cereal Chemistry Conference, Coogee, NSW, Australia, 16-18 September 2015, 16–18.
Demarco, M., Oliveira de Moraes, J., Matos, Â. P., Derner, R. B., de Farias Neves, F., and Tribuzi, G. (2022). Digestibility, bioaccessibility and bioactivity of compounds from algae. Trends Food Sci. Technol. 121, 114–128. doi:10.1016/j.tifs.2022.02.004
Dewettinck, K., Van Bockstaele, F., Kühne, B., Van de Walle, D., Courtens, T. M., and Gellynck, X. (2008). Nutritional value of bread: influence of processing, food interaction and consumer perception. J. Cereal Sci. 48, 243–257. doi:10.1016/j.jcs.2008.01.003
El Baky, H. H. A., El Baroty, G. S., and Ibrahem, E. A. (2015). Functional characters evaluation of biscuits sublimated with pure phycocyanin isolated from Spirulina and Spirulina biomass. Nutr. Hosp. 32, 231–241. doi:10.3305/NH.2015.32.1.8804
Feil, A. A., Cyrne, C. C., da, S., Sindelar, F. C. W., Barden, J. E., and Dalmoro, M. (2020). Profiles of sustainable food consumption: consumer behavior toward organic food in southern region of brazil. J. Clean. Prod. 258, 120690. doi:10.1016/j.jclepro.2020.120690
Ferreira, I., Oliveira, J., and Montanari, F. (2023). Alternative proteins in the EU: the case of algae. Effl. Rep. Altern. Proteins Regul., 146–147. doi:10.2826/605949
Fradinho, P., Niccolai, A., Soares, R., Rodolfi, L., Biondi, N., Tredici, M. R., et al. (2019). Effect of Arthrospira platensis (Spirulina) incorporation on the rheological and bioactive properties of gluten-free fresh pasta. doi:10.1016/j.algal.2019.101743
Fratelli, C., Burck, M., Amarante, M. C. A., and Braga, A. R. C. (2021). Antioxidant potential of nature’s “something blue”: something new in the marriage of biological activity and extraction methods applied to c-phycocyanin. Trends Food Sci. Technol. 107, 309–323. doi:10.1016/j.tifs.2020.10.043
Fratelli, C., Bürck, M., Silva-Neto, A. F., Oyama, L. M., De Rosso, V. V., and Braga, A. R. C. (2022). Green extraction process of food grade C-phycocyanin: biological effects and metabolic study in mice. Processes 10, 1793. doi:10.3390/pr10091793
García-Segovia, P., Pagán-Moreno, M. J., Lara, I. F., and Martínez-Monzó, J. (2017). Effect of microalgae incorporation on physicochemical and textural properties in wheat bread formulation. Food Sci. Technol. Int. 23, 437–447. doi:10.1177/1082013217700259
Garzon, R., Skendi, A., Lazo-Velez, M. A., Papageorgiou, M., and Rosell, C. M. (2021). Interaction of dough acidity and microalga level on bread quality and antioxidant properties. Food Chem. 344, 1–9. doi:10.1016/j.foodchem.2020.128710
Godfray, H. C. J., Beddington, J. R., Crute, I. R., Haddad, L., Lawrence, D., Muir, J. F., et al. (2010). Food security: the challenge of feeding 9 billion people. Science 327, 812–818. doi:10.1126/science.1185383
Graça, C., Fradinho, P., Sousa, I., and Raymundo, A. (2018). Impact of Chlorella vulgaris on the rheology of wheat flour dough and bread texture. LWT 89, 466–474. doi:10.1016/j.lwt.2017.11.024
Hernández, H., Nunes, M. C., Prista, C., and Raymundo, A. (2022). Innovative and healthier dairy products through the addition of microalgae: A review. Foods 11, 755. doi:10.3390/foods11050755
Kepekçi, R. A., and Saygideger, S. D. (2012). Enhancement of phenolic compound production in Spirulina platensis by two-step batch mode cultivation. J. Appl. Phycol. 24, 897–905. doi:10.1007/s10811-011-9710-3
Khemiri, S., Khelifi, N., Nunes, M. C., Ferreira, A., Gouveia, L., Smaali, I., et al. (2020). Microalgae biomass as an additional ingredient of gluten-free bread: dough rheology, texture quality and nutritional properties. Algal Res. 50, 101998. doi:10.1016/j.algal.2020.101998
Khemiri, S., Nunes, M. C., Bessa, R. J. B., Alves, S. P., Smaali, I., and Raymundo, A. (2021). Technological feasibility of couscous-algae-supplemented formulae: process description, nutritional properties and in vitro digestibility. Foods 10, 3159. doi:10.3390/foods10123159
Kohajdová, Z., and Karovičová, J. (2009). Application of hydrocolloids as baking improvers. Chem. Pap. 63, 26–38. doi:10.2478/s11696-008-0085-0
Kotoki, D., and Deka, S. C. (2010). Baking loss of bread with special emphasis on increasing water holding capacity. J. Food Sci. Technol. 47, 128–131. doi:10.1007/s13197-010-0008-2
Kumar, A., Ramamoorthy, D., Verma, D. K., Kumar, A., Kumar, N., Kanak, K. R., et al. (2022). Antioxidant and phytonutrient activities of Spirulina platensis. Energy Nexus 6, 100070. doi:10.1016/j.nexus.2022.100070
Lafarga, T., Fernández-Sevilla, J. M., González-López, C., and Acién-Fernández, F. G. (2020). Spirulina for the food and functional food industries. Food Res. Int. 137, 109356. doi:10.1016/j.foodres.2020.109356
Li, D., Fan, Z., and Tang, W. K. S. (2017). Domain learning naming game for color categorization. PLoS One 12, e0188164. doi:10.1371/journal.pone.0188164
Martínez-Sanza, M., Garrido-Fernández, A., Mijlkovic, A., Krona, A., Martínez-Abad, A., JoséColl-Marqués, M., et al. (2020). Composition and rheological properties of microalgae suspensions: impact of ultrasound processing. Algal Res. 49, 1–12. doi:10.1016/j.algal.2020.101960
Martins, R. B., Nunes, M. C., Ferreira, L. M. M., Peres, J. A., Barros, A. I. R. N. A., and Raymundo, A. (2020). Impact of acorn flour on gluten-free dough rheology properties. Foods 9, 560. doi:10.3390/foods9050560
Minekus, M., Alminger, M., Alvito, P., Ballance, S., Bohn, T., Bourlieu, C., et al. (2014). A standardised static in vitro digestion method suitable for food-an international consensus. Food Funct. 5, 1113–1124. doi:10.1039/c3fo60702j
Moayedallaie, S., Mirzaei, M., and Paterson, J. (2010). Bread improvers: comparison of a range of lipases with a traditional emulsifier. Food Chem. 122, 495–499. doi:10.1016/j.foodchem.2009.10.033
Mohankumar, J. B., Uthira, L., and Maheswari, S. U. (2018). Total phenolic content of organic and conventional green leafy vegetables. J. Nutr. Hum. Health 2, 1–6. doi:10.35841/nutrition-human-health.2.1.1-6
Mohd Jusoh, Y. M., Chin, N. L., Yusof, Y. A., and Abdul Rahman, R. (2009). Bread crust thickness measurement using digital imaging and L a b colour system. J. Food Eng. 94, 366–371. doi:10.1016/j.jfoodeng.2009.04.002
Mondal, A., and Datta, A. K. (2008). Bread baking - a review. J. Food Eng. 86, 465–474. doi:10.1016/j.jfoodeng.2007.11.014
Morsy, O. M., Sharoba, A. M., El-Desouky, A. I., Bahlol, H. E. M., and Abd El Mawla, E. M. (2014). Production and evaluation of some extruded food products using spirulina algae. Ann. Agric. Sci., Moshtohor 52, 495–510. doi:10.13140/RG.2.1.2263.6963
Muys, M., Sui, Y., Schwaiger, B., Lesueur, C., Vandenheuvel, D., Vermeir, P., et al. (2019). High variability in nutritional value and safety of commercially available Chlorella and Spirulina biomass indicates the need for smart production strategies. Bioresour. Technol. 275, 247–257. doi:10.1016/j.biortech.2018.12.059
Nawar, H. (2020). Collective bread diaries: cultural identities in an artificial intelligence framework. AI Soc. 35, 409–416. doi:10.1007/s00146-019-00882-2
Niccolai, A., Venturi, M., Galli, V., Pini, N., Rodolfi, L., Biondi, N., et al. (2019). Development of new microalgae-based sourdough “crostini”: functional effects of arthrospira platensis (spirulina) addition. Sci. Rep. 9, 19433. doi:10.1038/s41598-019-55840-1
Niccolai, A., Venturi, M., Galli, V., Pini, N., Rodolfi, L., Biondi, N., et al. (2021). Vegetable oils protect phycocyanin from thermal degradation during cooking of spirulina-based “crostini”. LWT 138, 110776. doi:10.1016/j.lwt.2020.110776
Nunes, M. C., Fernandes, I., Vasco, I., Sousa, I., and Raymundo, A. (2020a). Tetraselmis chuii as a sustainable and healthy ingredient to produce gluten-free bread: impact on structure, colour and bioactivity. Foods 9, 579. doi:10.3390/foods9050579
Nunes, M. C., Graça, C., Vlaisavljević, S., Tenreiro, A., Sousa, I., and Raymundo, A. (2020b). Microalgal cell disruption: effect on the bioactivity and rheology of wheat bread. Algal Res. 45, 101749. doi:10.1016/j.algal.2019.101749
Onwezen, M. C., Bouwman, E. P., Reinders, M. J., and Dagevos, H. (2021). A systematic review on consumer acceptance of alternative proteins: pulses, algae, insects, plant-based meat alternatives, and cultured meat. Appetite 159, 105058. doi:10.1016/j.appet.2020.105058
Palanisamy, M., Töpfl, S., Berger, R. G., and Hertel, C. (2019). Physico-chemical and nutritional properties of meat analogues based on Spirulina/lupin protein mixtures. Eur. Food Res. Technol. 245, 1889–1898. doi:10.1007/s00217-019-03298-w
Prabhasankar, P., and Haridas Rao, P. (1999). Lipids in wheat flour streams. J. Cereal Sci. 30, 315–322. doi:10.1006/jcrs.1999.0289
Raczyk, M., Polanowska, K., Kruszewski, B., Grygier, A., and Michałowska, D. (2022). Effect of spirulina (Arthrospira platensis) supplementation on physical and chemical properties of semolina (Triticum durum) based fresh pasta. Molecules 27, 355. doi:10.3390/molecules27020355
Rahimi, J., Baur, J., and Singh, A. (2020). Digital imaging as a tool to study the structure of porous baked foods. J. Cereal Sci. 95, 103084. doi:10.1016/j.jcs.2020.103084
Rosell, C. M., Rojas, J. A., and Benedito De Barber, C. (2001). Influence of hydrocolloids on dough rheology and bread quality. Available at: www.elsevier.com/locate/foodhyd.
Rosenthal, A. J., and Thompson, P. (2021). What is cohesiveness? A linguistic exploration of the food texture testing literature. J. Texture Stud. 52, 294–302. doi:10.1111/jtxs.12586
Saharan, V., and Jood, S. (2021). Effect of storage on Spirulina platensis powder supplemented breads. J. Food Sci. Technol. 58, 978–984. doi:10.1007/s13197-020-04612-1
Sanjari, S., Sarhadi, H., and Shahdadi, F. (2018). Investigating the effect of spirulina platensis microalgae on textural and sensory properties of baguette bread. J. Nutr. Food Secur. 3, 218–225. doi:10.18502/JNFS.V3I4.166
Shewry, P. R., and Hey, S. J. (2015). The contribution of wheat to human diet and health. Food Energy Secur 4, 178–202. doi:10.1002/FES3.64
Uribe-Wandurraga, Z. N., Igual, M., García-Segovia, P., and Martínez-Monzó, J. (2019). Effect of microalgae addition on mineral content, colour and mechanical properties of breadsticks. Food Funct. 10, 4685–4692. doi:10.1039/c9fo00286c
Venske, E., Dos Santos, R. S., Busanello, C., Gustafson, P., and Costa de Oliveira, A. (2019). Bread wheat: A role model for plant domestication and breeding. Hereditas 156, 16. doi:10.1186/s41065-019-0093-9
Volkmann, H., Imianovsky, U., Oliveira, J. L. B., and Sant’anna, E. S. (2008). Cultivation of Arthrospira (spirulina) platensis in desalinator wastewater and salinated synthetic medium: protein content and amino-acid profile. Braz. J. Microbiol. 39, 98–101. doi:10.1590/S1517-838220080001000022
Keywords: wheat bread development, Arthrospira platensis, functional ingredients, rheological properties, in vitro digestibility, sustainability
Citation: Fratelli C, Nunes MC, De Rosso VV, Raymundo A and Braga ARC (2023) Spirulina and its residual biomass as alternative sustainable ingredients: impact on the rheological and nutritional features of wheat bread manufacture. Front. Food. Sci. Technol. 3:1258219. doi: 10.3389/frfst.2023.1258219
Received: 13 July 2023; Accepted: 26 September 2023;
Published: 16 October 2023.
Edited by:
Noemi Elisabet Zaritzky, National University of La Plata, ArgentinaReviewed by:
Luís Miguel Cunha, University of Porto, PortugalCristiane Canan, Universidade Tecnológica Federal do Paraná, Brazil
Copyright © 2023 Fratelli, Nunes, De Rosso, Raymundo and Braga. This is an open-access article distributed under the terms of the Creative Commons Attribution License (CC BY). The use, distribution or reproduction in other forums is permitted, provided the original author(s) and the copyright owner(s) are credited and that the original publication in this journal is cited, in accordance with accepted academic practice. No use, distribution or reproduction is permitted which does not comply with these terms.
*Correspondence: Anna Rafaela Cavalcante Braga, YW5uYS5icmFnYUB1bmlmZXNwLmJy