- Department of Plant Breeding, The Swedish University of Agricultural Sciences, Lomma, Sweden
Global climate change is causing an increasing number of drought events, which might impact the stability of wheat breadmaking quality. In this study, 73 spring wheat lines with diverse genetic backgrounds (modern, old, and wheat–rye introgression) were drought treated, and the grains were analyzed by high-performance liquid chromatography for protein composition traits related to breadmaking quality. The amount of total sodium dodecyl sulfate-extractable and -unextractable proteins (TOTE, which correlates to grain protein content) increased significantly under late drought, while no effect of early drought was found on the analyzed protein composition traits. Under control treatment, genotypes with 3R showed significantly higher TOTE than genotypes with 1R, 1RS, and 2R, indicating the potential role of 3R in increasing grain protein concentration. The lower percentage of sodium dodecyl sulfate-unextractable polymeric protein in the total polymeric protein (%UPP) found in 1R and 1RS genotypes as compared to modern and old genotypes suggested a gluten strength reduction induced by 1R and 1RS. Despite the negative yield–protein correlation found in this study, lines 252 (3R), 253 (3R), and 258 (2R) displayed the presence of germplasm with both high yield and protein concentration. The %UPP was found to be positively correlated to spike-size-related traits (grains per spike, grain weight per spike, and spike length) across all three treatments. Additionally, high and stable TOTE was mainly obtained in genotypes with 3R, while old genotypes showed dominant performance in %UPP. Thus, genes responsible for high and stable protein concentration and gluten strength should be explicitly searched among introgression lines with chromosome 3R and old Swedish cultivars, respectively.
1 Introduction
With climate change, the global average temperature has increased over the decades. It is predicted to increase even faster in the future (Allen et al., 2019), thereby resulting in increased frequency and length of droughts. Drought has been reported as the major limiting factor to crop production as it significantly restricts plant growth and impacts the physiological, biochemical, morphological, and molecular characteristics of plants (Gregorova et al., 2015; Chaudhry and Sidhu, 2022). Thus, significant drought-induced yield losses have been reported from different regions of the world (Fischer and Maurer, 1978; Giunta et al., 1993; Senapati et al., 2019). Although grain yield is generally considered the most important trait, the end-use quality of certain crops is directly linked to processing efficiency, and drought-related effects on such parameters are also of relevance (Li et al., 2013).
Wheat (Triticum aestivum) is one of the three major crops worldwide and, thus, necessary in the human diet as a source of calories and proteins (Shiferaw et al., 2013). The breadmaking quality of wheat is an essential end-use character, which has been found to be highly correlated to protein composition (Branlard and Dardevet, 1985; Payne et al., 1987; Park et al., 2006). Proteins present in the wheat grain are divided into albumins, globulins, gliadins, and glutenins based on their solubility (Osborne, 1907). The gliadins and glutenins, also determined as gluten proteins, comprise 85% of the wheat grain proteins. The gluten proteins form polymers and monomers in the wheat grain (Markgren et al., 2020), which are reformed and rearranged during processing (Johansson et al., 2013). The specific distribution of polymeric and monomeric proteins determines the flour properties, including breadmaking quality (Gupta et al., 1993; Gupta et al., 1996; Zhang et al., 2008; Johansson et al., 2013). In addition, the polymerization behavior of the proteins is known to affect the breadmaking quality; e.g., the unextractable polymeric protein in total polymeric protein (%UPP) correlates positively with gluten strength (Gupta et al., 1993; Jia et al., 1996), while the total amount of SDS-extractable proteins (TOTE) correlates positively with the grain protein content (Johansson et al., 2013). Therefore, %UPP and TOTE are the two main protein parameters determining the end-use quality of the wheat grain.
Wheat protein composition is a grain quality property that varies among genotypes with different genetic backgrounds (Shewry et al., 1994; Veraverbeke and Delcour, 2002; Johansson et al., 2013). Compared to modern wheat, old wheat has a higher ratio of gliadins to glutenins, which contributes to weaker gluten, being less suitable for modern bread-baking processes (Desheva et al., 2014; Geisslitz et al., 2019). The introgression of the rye chromosome 1RS to the wheat genome has, in several previous studies, been reported to reduce the breadmaking quality by producing dough with unusually high stickiness and low strength (Dhaliwal et al., 1987; Graybosch et al., 1993; Fenn et al., 1994), even though the introgression of 1RS brought improvements in yield and disease resistance (Kim et al., 2004; Purnhauser et al., 2011; Ren et al., 2018).
In addition, environmental factors such as temperature, nitrogen, and soil moisture have been reported to contribute to differences in protein concentration and composition (Graybosch et al., 1995; Johansson et al., 2013; Rozbicki et al., 2015). Both grain protein concentration and bread loaf volume have been reported to increase under drought treatment (Kimball et al., 2001; Guzmán et al., 2016), while increases in %UPP and TOTE were noted under drought at the heading stage (Leiva et al., 2021). Furthermore, prolonged heat and drought in 2018 resulted in an increase in %UPP, though with a decrease in protein content, compared to the data from 2017 in Sweden (Lama, 2023). Differences in gluten strength were found as a result of variations in precipitation and temperature during the crop season (Johansson et al., 2020; Mkhabela et al., 2022). Although several observations have been made evaluating the effects of drought on wheat gluten, more studies are urgently needed to deepen our understanding of the relationship between yield performance, protein composition and drought stress in the context of fast global climate change.
The present study aimed to evaluate the effects of early and late drought on protein content and composition in a wide variety of spring wheat genotypes. Changes in different protein parameters were related to the diverse genetic background of the wheat evaluated, i.e., the modern, old, and wheat–rye introgression lines. Furthermore, previously obtained yield data were included in this study to establish the connection between drought effects on grain yield and quality. This entire study was designed based on the hypothesis that genes from different genetic sources impact baking quality performance under drought stress.
2 Materials and methods
2.1 Plant materials
A total of 73 spring wheat (T. aestivum L.) genotypes with a wide genetic background (Lan et al., 2022) consisting of 9 modern wheat cultivars and breeding lines received from the breeding company Lantmännen, 14 Swedish old cultivars released between 1928 and 1990 (Johansson et al., 2021), and 50 wheat-alien introgression lines (Merker, 1984) with rye chromosomes 1R, 2R, 3R, 4R, 5R, 6R, and Leymus racemosus (wild rye) chromosome N in the form of translocation and substitution (Rahmatov, 2016) were used in this study.
2.2 Growing conditions and drought treatments
All grain samples were collected from an experiment carried out in 2020 under strictly controlled conditions in the Biotron facility at the Swedish University of Agricultural Sciences, Alnarp, Sweden, as has previously been described (Lan et al., 2022). Thus, the hourly regulated temperature and humidity were set according to the mean climate data of Malmö, Sweden, from 2010–2019, obtained from the Swedish Meteorological and Hydrological Institute (SMHI). Three growing conditions were implemented for all the genotypes, i.e., standard growing conditions used as a control (C) where plants were watered every second day throughout the experiment, early drought stress (EDS) growing conditions where a 28-day water withholding was imposed from 30 days after planting, and late drought stress (LDS) growing conditions where a 14-day water withholding was imposed from 60 days after planting. Details of the temperature, humidity, and treatments for the present study are as previously described by Lan et al. (2022).
2.3 Grain protein content
The protein content of flour samples (73 × 3 biological replicates) harvested from the control condition was determined using the elemental particle analyzer method (EPA, Flash 2000 Elemental Analyzer, Thermo Scientific). A conversion factor of 5.7 was used to convert the nitrogen content into protein content (Mosse, 1990).
2.4 Size-exclusion HPLC
For each genotype (total of 73), spikes of three biological replicates for each growing condition were sampled, resulting in a total of 657 samples. After threshing, the husked grains of each genotype were milled to flour for 30 s by using a laboratory mill (Mixer Mill 400 MM, RETSCH) to obtain the whole-grain flour samples.
Extraction of proteins was carried out on two technical replicates of each of the 657 whole-grain flour samples, following a previously developed two-step protocol (Gupta et al., 1993) with minor modifications. In the first step, 16.5 mg of each flour sample was used to extract proteins with 1.4 ml of 0.5% SDS-phosphate buffer (pH 6.9). The samples were subjected to 10 s of vortexing (VOTEX-GENIE 2, Scientific Industries) followed by 5 min of stirring (VIBRAX, VXR basic, IKA) at 2,000 rpm and centrifugation (LEGEND MICRO 17, Thermo Scientific) for 30 min at 10,000 rpm. According to the work of Larroque et al. (2000), the supernatant was subjected to a 2 min water bath at 80°C (SUB Aqua Pro, Grant) to avoid degradation of polymeric proteins by deactivating the enzyme protease followed by a 2 min ice-water bath to cool the supernatant down to a suitable status for SE-HPLC. In the second step, 1.4 ml of 0.5% SDS-phosphate buffer (pH 6.9) was added to each of the residual pellet from the first extraction, which were then subjected to sonication for 45 s in an ultrasonic disintegrator (Soniprep 150, Tamro, Mölndal, Sweden) with a 3 mm exponential microtip at an amplitude of 5 µm. After sonication, all the samples were centrifuged for 30 min at 10,000 rpm, the supernatant of each sample was collected, and following the work of Larroque et al. (2000), it was treated for 2 min at 80°C and then in an ice-water bath.
All samples (total 2628) from the two-step extraction procedure were loaded onto a Waters (Milford, MA, United States) HPLC system with a BioSep SEC-4000 Phenomenex column. Each sample was separated according to molecular size distribution under 30 min, and the proteins were detected by using a diode array detector at a UV absorbance of 210 nm.
The results of SE-HPLC were output in the form of two chromatograms (Figures 1A, B), one from the first extraction and one from the second extraction. The wheat proteins consist of gliadins, which are monomeric in their native form, and glutenins, which are polymeric in their native form (Markgren et al., 2020). However, during any kind of processing, cross-links are broken and reformed among the proteins, which results in gliadins being trapped (Johansson et al., 2013) or cross-linked through disulfide, peptide, or lanthionine bonds or through hydrophobic clustering into the polymer (Markgren et al., 2022). Extraction of the proteins using SDS-phosphate buffer for the first step and sonication for the second step is also known to not break all of the disulfide bonds of the polymers (Johansson et al., 2013). Therefore, both of the chromatograms visualized the presence of both polymeric and monomeric proteins of various sizes, which has previously been described in various studies (Gupta et al., 1993; Johansson et al., 2013). Therefore, both chromatograms of each sample were divided into four sections according to molecular size, namely, large polymeric proteins (LPP), smaller polymeric proteins (SPP), large monomeric proteins (LMP), and smaller monomeric proteins (SMP). The relative amounts of these four types of proteins were calculated based on areas covered by the absorbance curve (Malik, 2012). Six protein parameters (Malik, 2012), including total SDS-extractable protein, total SDS-unextractable protein (TOTU), the percentage of SDS-unextractable polymeric protein in the total polymeric protein (%UPP), the percentage of large SDS-unextractable polymeric protein in the total large polymeric protein (%LargeUPP), the percentage of large SDS-unextractable monomeric protein in the total large monomeric protein (%LUMP), and the ratio of total monomeric protein to the total polymeric protein (Mon/Pol), were calculated as follows:
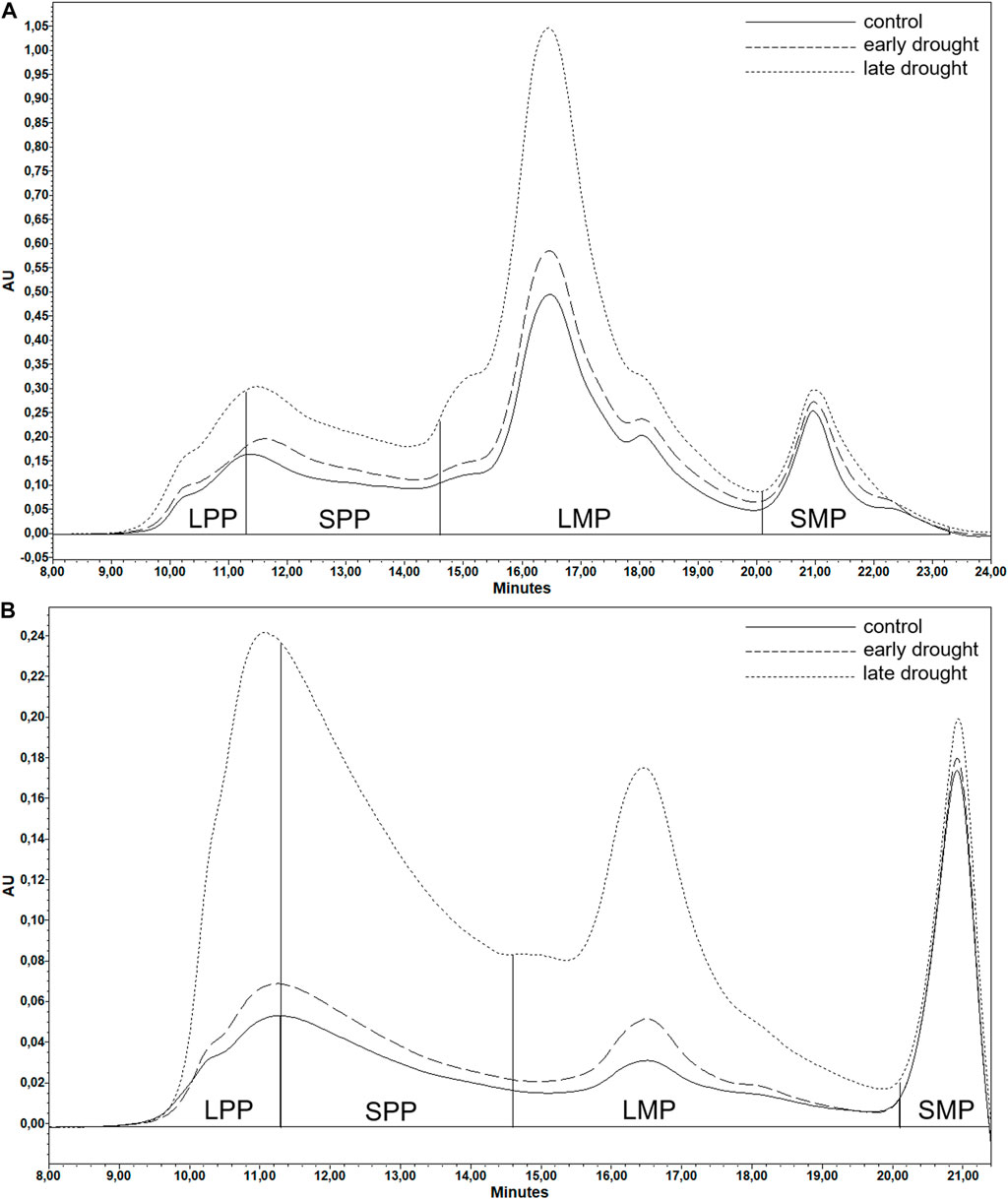
FIGURE 1. Example (introgression genotype 256, chromosome: 3R) of SE-HPLC chromatograms of (A) SDS-extractable and (B) SDS-unextractable gluten proteins under different treatments. Chromatograms were divided into four sections representing large polymeric proteins, smaller polymeric proteins, large monomeric proteins, and smaller monomeric proteins. The area of each section under the chromatograms represents the amount of the corresponding protein fraction. AU, absorbance unit of the UV detector.
Here, ‘e’ and ‘u’ represent SDS-extractable and SDS-unextractable proteins, respectively. Based on previous results, TOTE was used in the present study as an indicator of grain protein content, and similarly, %UPP was used as an indicator of gluten strength (Malik, 2012). To verify the strong correlation between TOTE and grain protein content, a correlation analysis between the two parameters was carried out as shown in the appendix (Supplementary Figure S1).
2.5 Plant physiological yield traits
Yield-related traits obtained from the physiological development of the plant, such as spike length (SPL), spike number per plant (SNPP), productive spikes per plant (PSPP), 1000-grain weight (TGW), grains per spike (GPS), grain weight per spike (GWPS), grain weight per plant (GWPP), and grains per plant (GPP), were reported in a previous study (Lan et al., 2022). Here, we evaluated the relationship between these yield traits and the grain protein content and composition obtained from the aforementioned HPLC analyses.
2.6 Data analysis
All the statistical analyses were carried out using the software RStudio (Team, 2015). A two-way analysis of variance (ANOVA) was conducted for each protein parameter to detect significant variations between treatments and among genotypes. A mean comparison was performed to verify the significant differences between treatments pairwise and compare different genotype groups (modern, old, 1R, 1RS, 2R, and 3R) using the LSD post hoc test with the R package “agricolae.” Pearson’s correlation coefficients between the six protein composition traits and eight previously obtained yield traits, i.e., SPL, SPP, PSPP, TGW, GPS, GWPS, GWPP, and GPP (Lan et al., 2022), were calculated and visualized using R packages “Hmisc” and “corrplot,” respectively. Principal component analysis (PCA) was computed and visualized using the R package “ggfortify” to further study the relationship among different traits and treatments. The harmonic mean of genotypic values (HMGV), relative performance of genotypic values (RPGV), and harmonic mean of relative performance of genotypic values (HMRPGV) were computed based on the best linear unbiased prediction (BLUP) using the R package ‘metan’ to rank genotypes with the highest TOTE and %UPP stability. The additive main effects and multiplicative interaction (AMMI) was performed using the R package ‘metan’ to study the TOTE and %UPP stability of genotypes across treatments.
3 Results
3.1 Effect of drought stresses on protein fractions
The grain protein content of the C samples varied between 8% and 15% (Supplementary Table S2) and was found to significantly and strongly correlate with TOTE (Supplementary Figure S1). Therefore, the TOTE values were used to evaluate the effects of drought on grain protein content. The ANOVA showed a significant effect of drought stress on three of the studied protein parameters, i.e., TOTE, TOTU, and Mon/Pol (Supplementary Table S2). Significantly higher TOTE and TOTU levels were obtained for LDS than C plants (C: 1.32 × 108, LDS: 2.33 × 108 and C: 3.2 × 107, LDS: 5.5 × 107, respectively; Supplementary Table S3). Drought stress at different stages affected the mean of the six protein composition traits evaluated (TOTE, TOTU, %UPP, %LargeUPP, %LUMP, and Mon/Pol) differently among the genotype groups (modern, old, 1R, 1RS, 2R, and 3R; Figure 2; Supplementary Figure S2). Thus, both TOTE and TOTU were significantly higher in all the six genotype groups under LDS as compared to C (Figure 2A, B), and only two genotype groups (modern and old) showed a significantly lower Mon/Pol under LDS than C (Figure 2D). For %UPP, %LargeUPP, and %LUMP, no significant effects of drought treatments were noted among treatments, although significant differences were found among genotype groups (Figure 2C; Supplementary Figure S2).
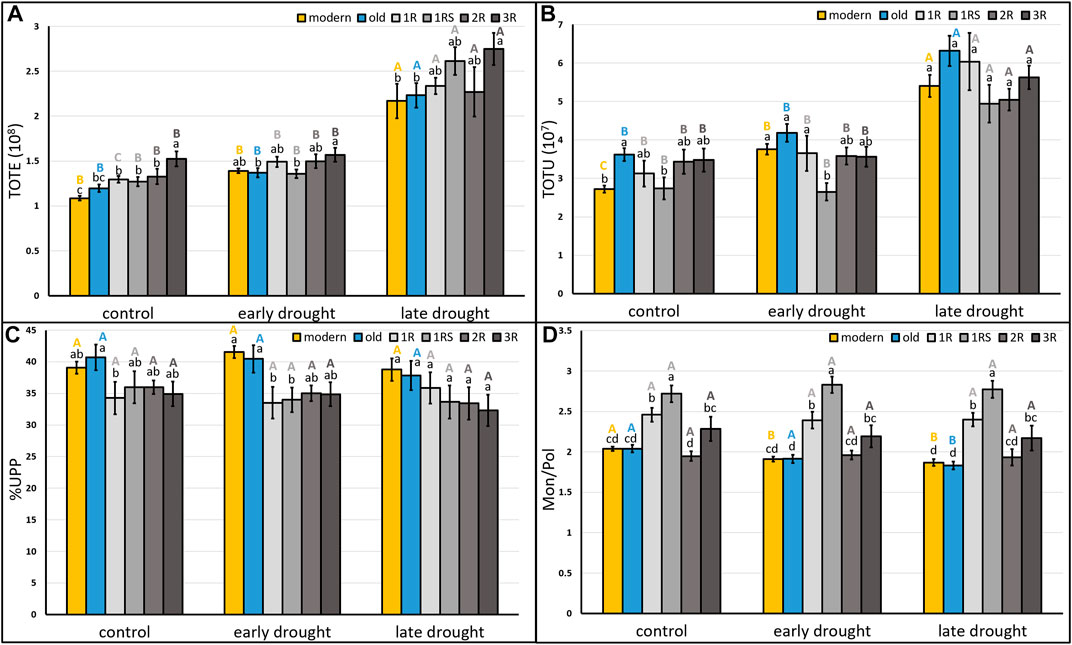
FIGURE 2. Mean (A) total SDS-extractable proteins, (B) total SDS-unextractable proteins, and (C) the percentage of SDS-unextractable polymeric proteins in the total polymeric proteins; (D) the ratio of total monomeric proteins to the total polymeric proteins of each genotype group under control, early drought, and late drought treatments. Modern = approved cultivars and breeding lines received from company Lantmännen. Old = cultivars released from 1928 to 1990. 1R = introgressions of chromosome 1R. 1RS = introgressions of chromosome 1RS. 2R = introgressions of chromosome 2R. 3R = introgressions of chromosome 3R. Means of the same genotype group between treatments marked by the same capital letters do not differ significantly. Means between different genotype groups within each treatment marked by the same lower letters do not differ significantly (LSD post hoc test at p < 0.05).
A significantly higher TOTU was found for old genotypes than for modern genotypes under C (modern: 2.72 × 107; old: 3.61× 107), while a higher %LargeUPP was found for modern than old genotypes under EDS (modern: 48.03; old: 42.53) (Figure 2B; Supplementary Figure S2A). For the introgression genotypes, the 3R genotypes showed a significantly higher TOTE compared to 1R, 1RS, and 2R under C (Figure 2A). Furthermore, 1RS genotypes showed a lower TOTU than 1R genotypes under EDS (Figure 2B). The 1R showed a higher %LUMP than 1RS under LDS (Supplementary Figure S2B). Significantly, the highest Mon/Pol was found for 1RS under all three treatments, and 1R genotypes showed a higher value than 2R genotypes (Figure 2D).
3.2 Relationships among treatments, protein composition, and yield traits
The PCA combining six protein composition traits (TOTE, TOTU, %UPP, %LargeUPP, %LUMP, and Mon/Pol) and eight previously obtained yield traits (SPL, SNPP, PSPP, TGW, GPS, GWPS, GWPP, and GPP) divided the samples based on drought treatment along the first principal component (PC1) axis, explaining 40.2% of the variation (Figure 3). Thus, C samples were located with negative values on the PC1, where the yield-related traits were found, indicating generally high values of these traits on the C samples. The LDS samples were found with basically positive values on the PC1, which also was the case for TOTE, indicating high grain protein concentration in the LDS samples. The second principal component (PC2), explaining 24.6% of the variation, generally differentiated the samples in relation to gluten-strength-related parameters (%UPP, %LargeUPP, %LUMP, and Mon/Pol), with a clear negative relationship between Mon/Pol versus %UPP, %LargeUPP, and %LUMP (Figure 3). Samples of all three treatments were differentiated along the PC2, thus indicating a variation in gluten strength in the plant material used independent of drought treatment.
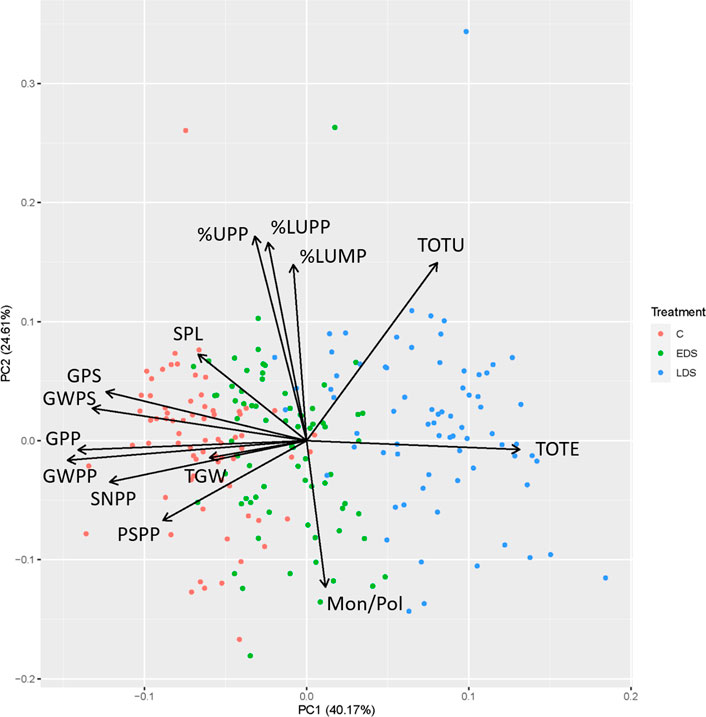
FIGURE 3. Biplot of principal component analysis for protein composition traits, total SDS-extractable proteins, total SDS-unextractable proteins, the percentage of SDS-unextractable polymeric proteins in the total polymeric proteins, the percentage of large SDS-unextractable polymeric proteins in the total large polymeric proteins, the percentage of large SDS-unextractable monomeric proteins in the total large monomeric proteins, the ratio of total monomeric proteins to the total polymeric proteins and yield traits, spike length, spike number per plant, productive spikes per plant, 1000-grain weight, grains per spike, grain weight per spike, grain weight per plant, and grains per plant of genotypes studied under control, early drought stress, and late drought stress.
3.3 Relationships between protein and yield traits
This study showed several significant correlations among the six protein composition traits (TOTE, TOTU, %UPP, %LargeUPP, %LUMP, and Mon/Pol) and eight yield traits, including three spike parameters (SPL, SNPP, and PSPP) and five grain-yield components (TGW, GPS, GWPS, GWPP, and GPP) under different treatments. A significantly negative correlation between TOTE and %UPP was noted and retained under C (−0.42***), EDS (−0.37**), and LDS (−0.49***; Figure 4).
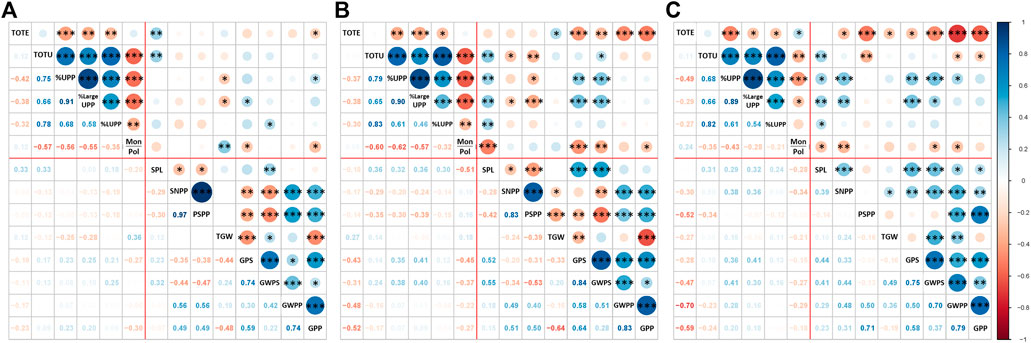
FIGURE 4. Correlation among protein composition traits and previously studied yield traits, total SDS-extractable proteins, total SDS-unextractable proteins, the percentage of SDS-unextractable polymeric protein in the total polymeric protein, the percentage of large SDS-unextractable polymeric protein in the total large polymeric protein, the percentage of large SDS-unextractable monomeric protein in the total large monomeric protein, the ratio of total monomeric proteins to the total polymeric proteins, spike length, spike number per plant, productive spikes per plant, 1000-grain weight, grains per spike, grain weight per spike, grain weight per plant, and grains per plant under (A) control (C), (B) early drought, and (C) late drought stress. The significance level for Pearson’s correlation coefficient was indicated by ***: p < 0.001, **: p < 0.01, and *: p < 0.05.
In the control group (C), two protein traits (TOTE and TOTU) correlated significantly and positively with the spike length (SPL); meanwhile, correlations between protein traits and grain yield traits were mainly lacking (Figure 4A). For the EDS and LDS plants, a higher number (than for C plants) of significant relationships were found among protein parameters and yield traits. Thus, several of the protein parameters (TOTU, %UPP, %LargeUPP, and %LUMP) correlated significantly and positively with SPL, but TOTE and Mon/Pol correlated significantly and negatively with grain yield components (Figures 4B, C).
3.4 Genotypes with the highest and lowest 10% of TOTE
The 10% genotypes with the highest and lowest TOTE showed values of 1.69–2.06 × 108 and 0.90–1.03 × 108 under C, 1.73–1.99 × 108 and 1.13–1.19 × 108 under EDS, and 3.27–3.94 × 108 and 1.33–1.63 × 108 under LDS, respectively (Table 1). The 10% genotypes with the highest TOTE were represented by wheat–rye introgression genotypes across three treatments, except for one modern and one old genotype appearing in LDS. In contrast, primarily modern and old genotypes were found among the 10% of the genotypes with the lowest TOTE under C and EDS, respectively.
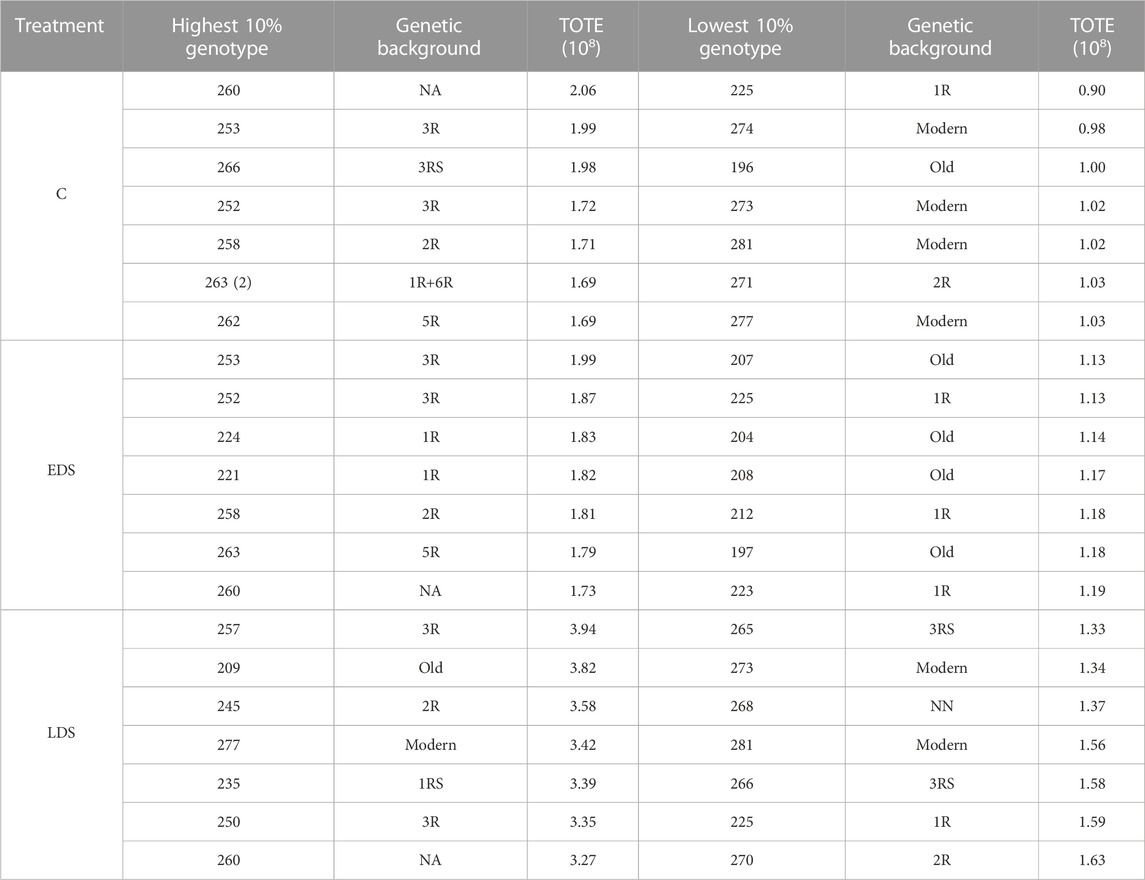
TABLE 1. 10% genotypes with the highest and lowest grain TOTE under control, early drought stress, and late drought stress treatments.
A PCA comparing the effect of eight previously obtained yield components (SPL, SNPP, PSPP, TGW, GPS, GWPS, GWPP, and GPP) on the 10% genotypes with the highest and lowest TOTE clearly showed the impact of the yield components on the grain protein concentration (TOTE) for all treatments (Figure 5). High-yield components were related to low TOTE for both the C treatment (Figure 5A) and the EDS (Figure 5B) and LDS (Figure 5C). However, a few genotypes, 252 (3R), 253 (3R), and specifically, 258 (2R), were found with both high values on the yield components and high TOTE under the C treatment (Figure 5B).
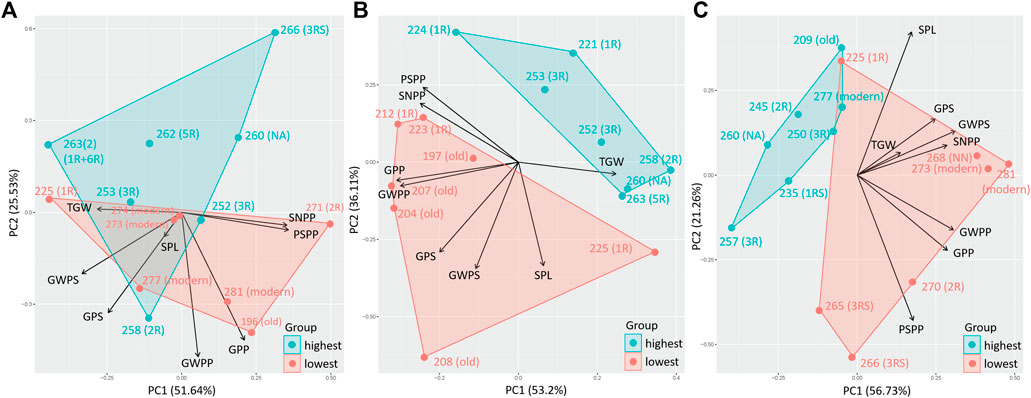
FIGURE 5. Principal component analysis for yield components, spike length, spike number per plant, productive spikes per plant, 1000-grain weight, grains per spike, grain weight per spike, grain weight per plant, and grains per plant of genotypes with the highest 10% (highest) and lowest 10% (lowest) TOTE under (A) control (C), (B) early drought, and (C) late drought stress.
3.5 Genotypes with the highest and lowest %UPP
The 10% genotypes with the highest and lowest %UPP showed values of 45.29%–57.79% and 12.24%–23.29% under C, 44.65%–55.29% and 15.05%–23.64% under EDS, and 45.01%–59.41% and 15.39%–22.53% under LDS, respectively (Table 2). The old genotypes were found to dominate the 10% genotypes with the highest %UPP while 1R and 1RS genotypes dominated the 10% genotypes with the lowest %UPP, in both cases, across all three treatments.
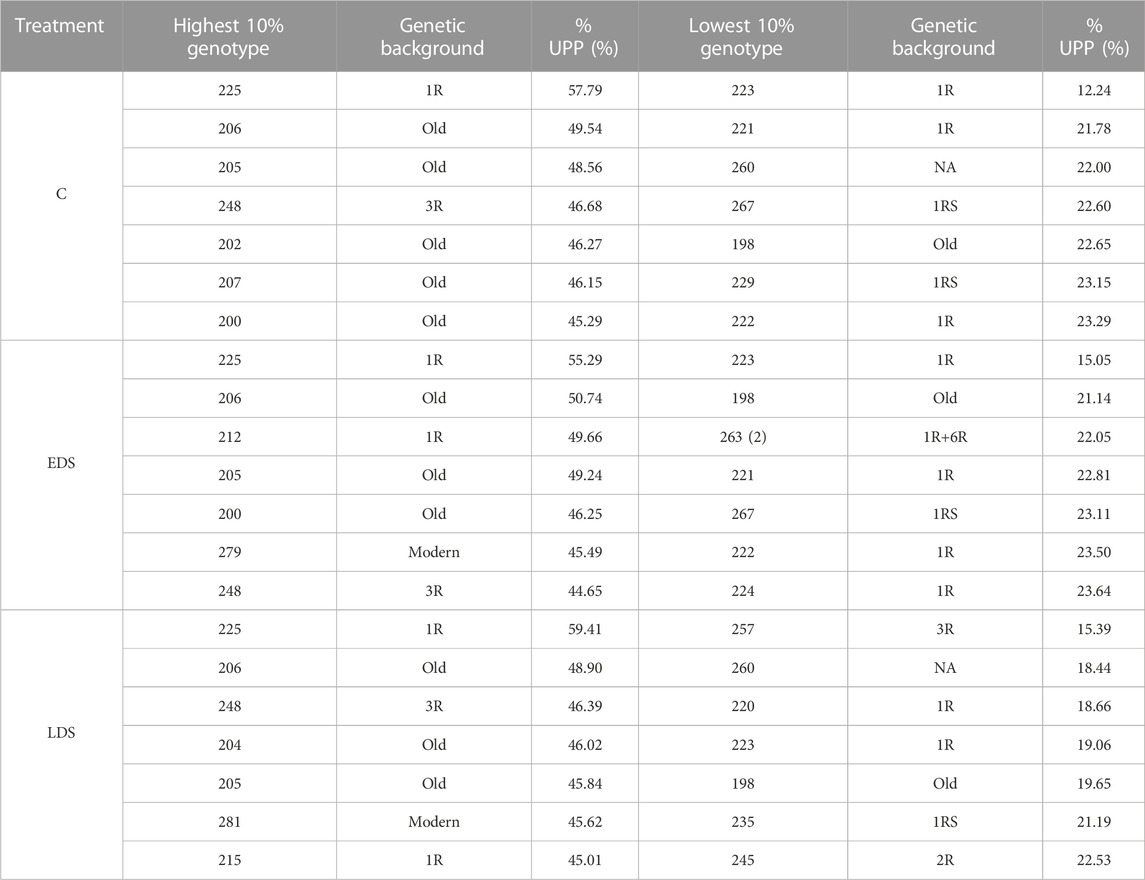
TABLE 2. 10% genotypes with the highest and lowest %UPP under control, early drought stress, and late drought stress treatments.
A PCA comparing the effect of eight yield components (SPL, SNPP, PSPP, TGW, GPS, GWPS, GWPP, and GPP) on the 10% genotypes with the highest and lowest %UPP showed a clear impact of spike characters (GPS—grains per spike, GWPS—grain weight per spike, and SPL—spike length) on %UPP, independent of treatment (Figures 6A–C). Thus, in principle, a higher protein polymerization is obtained with increased spike size.
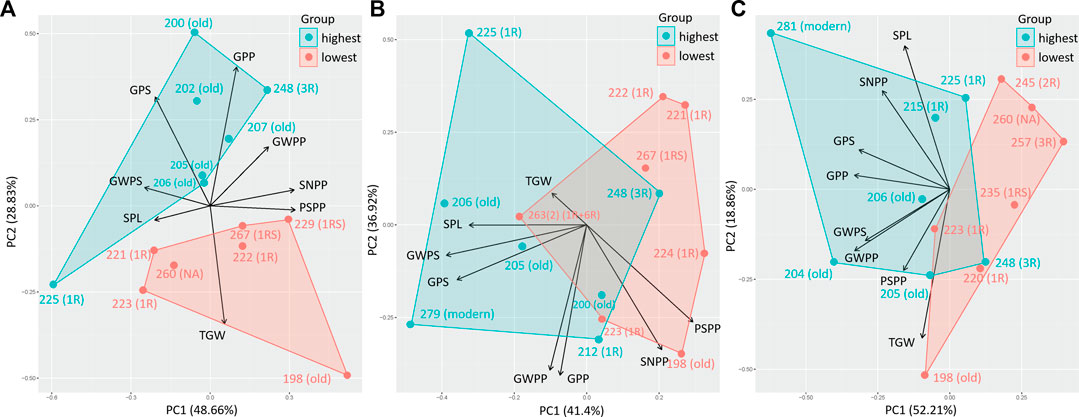
FIGURE 6. Principal component analysis for yield components, spike length, spike number per plant, productive spikes per plant, 1000-grain weight, grains per spike, grain weight per spike, grain weight per plant, and grains per plant of genotypes with the highest 10% (highest) and lowest 10% (lowest) %UPP under (A) control (C), (B) early drought, and (C) late drought stress.
Under LDS, additional yield components were found related to the 10% genotypes with the highest %UPP (Figure 6C). However, this relationship might be partly a result of the strong negative correlation between grain yield components (GWPS, GWPP, and GPP) and grain protein concentration (TOTE) and between grain protein concentration (TOTE) and gluten strength (%UPP) under LDS.
3.6 Genotypes with the highest stability and adaptability of TOTE and %UPP
The 10% most stable and adaptable genotypes in TOTE showed the harmonic mean of genotypic value of 1.91–2.12 × 108, the relative performance of genotypic value of 1.18–1.32, and the harmonic mean of relative performance of genotypic value of 1.16–1.31. Meanwhile, the 10% most stable and adaptable %UPP ranged from 43.44%–56.86% in HMGV, 1.21–1.58 in RPGV, and 1.21–1.58 in HMRPGV (Table 3).
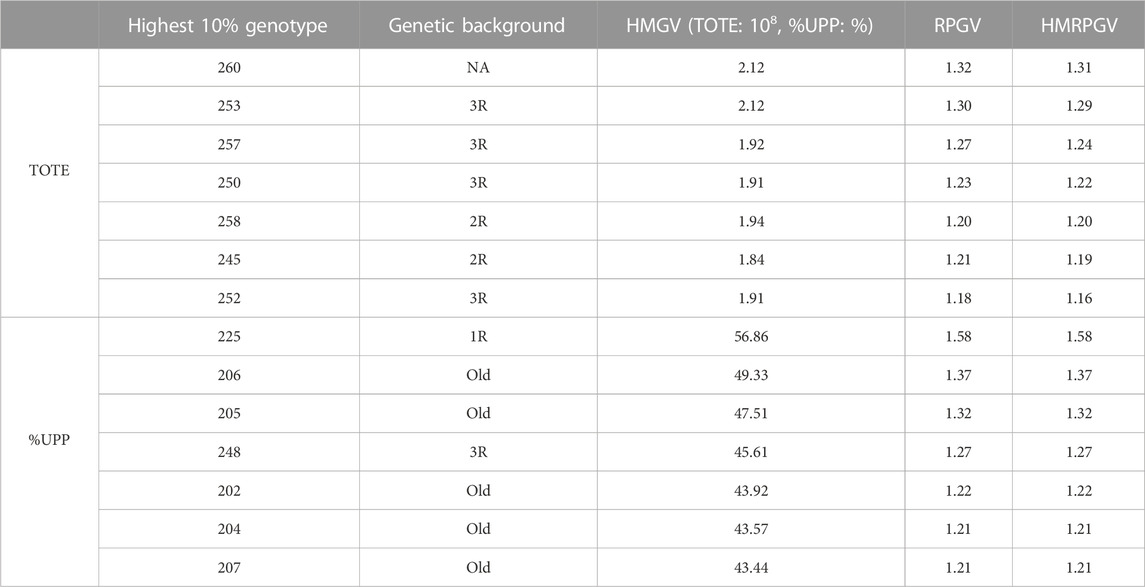
TABLE 3. 10% genotypes with the highest, most stable, and most adaptable TOTE and %UPP based on BLUP indexes including the harmonic mean of genotypic values indicating stability, the relative performance of genotypic values indicating adaptability to drought stress, and the harmonic mean of the relative performance of genotypic values indicating stability and adaptability simultaneously.
Genotype 260, also identified as the highest in Table 1 under C, showed the most stable grain protein concentration (TOTE) performance simultaneously with the highest HMGV (2.12 × 108), RPGV (1.32), and HMRPGV (1.31). Genotype 225, also identified as the highest in Table 2 under C, EDS, and LDS, showed the most stable gluten strength (%UPP) simultaneously with the highest HMGV (56.86%), RPGV (1.58), and HMRPGV (1.58; Table 3). Genotypes with chromosome 3R (253, 257, 250, and 252) and old genotypes (206, 205, 202, 204, and 207) outnumbered other genotype groups among the 10% most stable TOTE and %UPP genotypes, respectively (Table 3).
The additive main effects and multiplicative interaction identified the strongest interaction force under LDS due to its longest vector, and C and EDS showed similar interaction forces with genotypes for both TOTE and %UPP (Figure 7). The highest and most stable TOTE was found in genotype 260 (NA), followed by 253 (3R), 258 (2R), 203 (old), and 224 (1R), with relatively high and stable values of TOTE (Figure 7A). Meanwhile, the highest and most stable %UPP was found in genotype 225 (1R), followed mainly by old genotypes 206 (old), 205 (old), 248 (3R), and 202 (old), with relatively high and stable values of %UPP (Figure 7B).
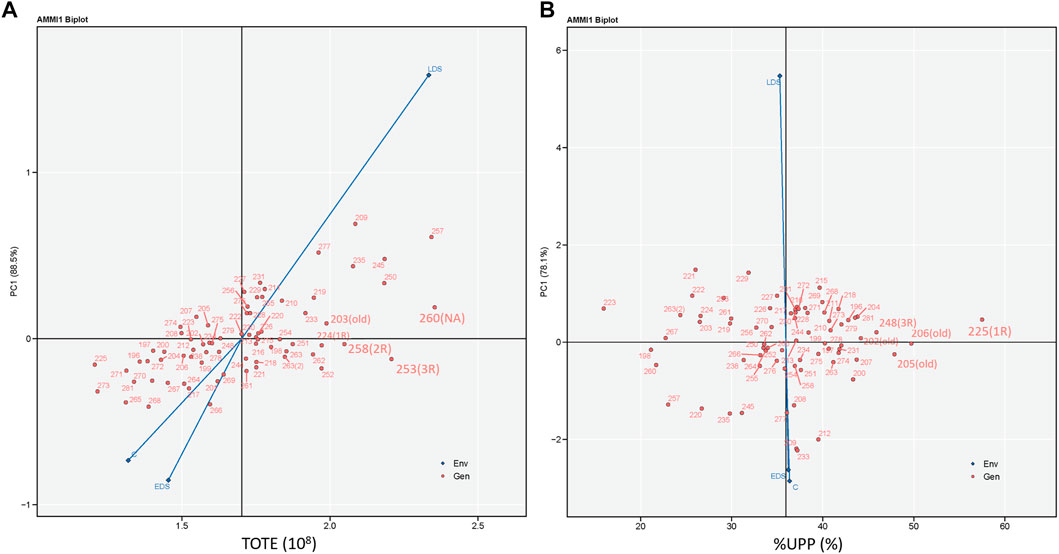
FIGURE 7. Additive main effects and multiplicative interaction biplots showing (A) TOTE (108) and (B) %UPP (%) versus the first principal component score of 73 genotypes and three growing conditions including control (abbreviated as C), early drought stress (EDS), and late drought stress (LDS). Genotypes located closer to the horizontal axis (score 0 on PC1) had relatively higher stability in TOTE and %UPP across three growing conditions.
4 Discussion
The present study clearly showed the importance of the sources of wheat germplasm for the performance in terms of grain protein composition under drought stress. Genotypes containing 3R were well represented with high TOTE both during control conditions and at EDS and LDS. Thus, these genotypes were also found stable for TOTE over optimal and drought conditions at various stages of wheat development. A high %UPP was found in some of the old genotypes at control conditions and also at EDS and LDS, which resulted in the highest stability for some of the old genotypes across control and drought conditions. However, part of the variations in grain protein concentration (TOTE) was related to yield differences among the genotypes, and also, a correlation prevailed between gluten strength (%UPP) and some of the plant physiological parameters. Thus, when searching for genotypes with high stability across environments in quality traits, the relationship resulting in decreases in yield has to be taken into consideration. However, the 3R genotypes of the present study have previously been shown with a high yield at EDS, indicating that a reduction in yield is not the major explanation for the high grain protein concentration in these genotypes.
The significantly higher grain protein content, measured as TOTE, in 3R genotypes than in 1R, 1RS, 2R, modern, and old genotypes under C, indicated the presence of genes in 3R that contribute to high grain protein concentration. The low TOTE in modern wheat may be attributed to a high yield performance of these genotypes, with a high transfer of starch to the grains, thereby diluting the protein content. Unlike modern wheat, known to have high and stable yield performance as a result of yield-oriented breeding programs, the introgressed wheat lines have been developed attempting to introduce resistance genes from wild or distant relatives to wheat. Several successful transfers of rye genes have been reported, which contribute to disease resistance in adapted wheat cultivars (Friebe et al., 1996; Rahmatov, 2016; Crespo-Herrera et al., 2017). The chromosome 3R has been reported to increase stem rust resistance (Miller, 1984; Rahmatov, 2016), tolerance to early drought (Lan et al., 2022), and aluminum (Salvador-Moreno et al., 2018) in wheat. However, there is no report about the effect of 3R on breadmaking quality. In addition, studies on the contribution of 3R in breeding are fewer than those on other rye chromosome transfers, such as from 1R. The lack of studies on 3R may be attributed to its poor transmission rate (25.0%) compared to 1R (51.6%) and 2R (51.6%) while performing backcrossing (Li et al., 2018). In addition to contributing to high TOTE at control conditions, 3R was also found to contribute to stability in TOTE across drought environments, as demonstrated by the BLUP-based values of HMGV, RPGV, and HMRPGV. Thus, three wheat–rye introgression genotypes (252, 253, and 258) were found with both high TOTE and yield, as well as with high stability of TOTE across drought environments. Furthermore, the results of AMMI confirmed the high and stable TOTE of 253 and 258, and it also identified the strongest genotype × environment interaction in LDS. As our results demonstrated a significantly higher grain protein concentration with 3R than other rye chromosomes (1R, 1RS, and 2R) and genetic backgrounds (modern and old), and also stability across environments, without a reduction in yield, studies should be performed to identify the genes behind these characteristics and to further explore the effect of 3R on wheat grain quality.
The negative relationship between TOTE and yield traits identified in a correlation analysis was further confirmed by a PCA evaluating the impact of yield components on the highest and lowest TOTE. Across all three treatments, a high yield was mainly associated with the 10% genotypes with the lowest TOTE. A negative yield–protein relationship in wheat has been reported by a range of studies (Kibite and Evans, 1984; Simmonds, 1995; Feil, 1997; Cooper et al., 2001) and has long been viewed as the main difficulty in developing cultivars with both high protein content and yield (Blackman and Payne, 1987). Both grain yield and protein concentration of wheat have been reported as highly prone to genotype × environment interactions (Oury et al., 2003; Asseng and Turner, 2007). However, grain protein concentration and grain yield have been assigned as separate characters governed by different genes. Instead, this negative yield–protein correlation is attributed to the fact that grain yield is closely related to the starch content in the grain (Bhullar and Jenner, 1985; Hakim et al., 2012). Thus, a decrease in grain starch content results in a reduction in yield, and if not, protein accumulation is impacted in a higher grain protein concentration. In this study, three of the top 10% TOTE genotypes were found with high yield components, i.e., 252 (3R) with a relatively high number of spikes (SNPP and PSPP), 253 (3R) with large grain size (TGW), and 258 (2R) with both high grain number (GPS) and grain weight (GWPP). The fact that the yield components responsible for the high yield despite high TOTE varied among the three genotypes identifies different genetic-based potential breeding solutions for a combination of high yield and high protein concentration in the same genotype. Thus, the genetic backgrounds for these phenotypic characteristics require further investigation.
All genotypic and environmental characters impacting plant development, e.g., days to heading and days to anthesis, have, in previous studies, been shown to affect %UPP and, thereby, gluten strength and breadmaking quality (Malik, 2012; Johansson et al., 2013; Johansson et al., 2020). Thus, factors such as nitrogen regimes and temperature have been shown largely impacting protein polymerization and gluten strength (Johansson et al., 2013; Gagliardi et al., 2020). However, the drought conditions used in the present study have previously been shown not to significantly alter the days to heading or days to anthesis (Lan et al., 2022). These findings correspond well with the fact that no significant differences in %UPP were found among C and the drought treatments EDS and LDS used here. However, despite no general effect of drought on %UPP being noted, significant variations were found for genotype groups. Thus, 1R genotypes showed a significantly lower %UPP than old genotypes under C, and moreover, the %UPP of both 1R and 1RS was significantly lower than that of both modern and old genotypes under EDS, verifying 1R and 1RS, contributing to a decreased gluten strength, as has been described in previous studies (Graybosch et al., 1993; Fenn et al., 1994). The 1B chromosome, substituted to 1R, is known to contain genes for gluten proteins important for gluten strength, and the secalins that the 1R adds cannot contribute similar properties (Josephides et al., 1987; Graybosch et al., 1993). The significantly higher Mon/Pol found in 1RS than in 1R, 2R, and 3R, as well as modern and old genotypes in the present study, also indicates the issue with less polymerization of the proteins related to the substitution of gluten genes in 1B with secalin genes in 1R. This increased Mon/Pol from 1RS obtained across all three treatments further suggested that genes on the short arm of 1R played a major role in forming weak and sticky dough as these quality defects were independent of environments. Despite the negative effects on breadmaking quality brought by 1RS, it is still one of the most widely used sources of alien genes for wheat breeding due to several traits being improved with the successful introgression of 1RS, especially disease resistance, such as yellow rust (Yang et al., 2016), stem rust (Mago et al., 2002), leaf rust (Hsam et al., 2000), and powdery mildew (Mohler et al., 2001). Furthermore, 1RS was also reported to increase root length (Lan et al., 2022), drought tolerance (Ludlow and Muchow, 1990), and final yield (Ren et al., 2018).
Regarding genotypes with the highest and lowest 10% %UPP, old genotypes and 1R (including 1RS) dominated the highest and lowest %UPP ranking, respectively, which implied high gluten strength in old Swedish cultivars and further confirmed the poor breadmaking quality brought by 1R and 1RS, as discussed above. Among different yield components, GPS, GWPS, and SPL were found to relate to high %UPP across the three treatments, indicating the association between increased spike size and high gluten strength. Previous studies have reported a positive correlation between grain weight and gluten strength measured by mixograph peak height (Tahir et al., 2006) and a negative correlation between spike density and gluten strength indicated by sodium dodecyl sulfate (SDS) sedimentation volume (Hailu and Merker, 2008). However, the eventual relationship between spike size and gluten strength, as reported here, needs to be further elaborated on, and genes for its determination need to be further determined. The fact that protein composition in the grains differs along the wheat spike (Johansson et al., 2013) may contribute to the results obtained. In addition to showing a high %UPP, old Swedish cultivars also showed a stable %UPP over control and drought stress conditions of different times during plant development. All the five old genotypes (202, 204, 205, 206, and 207) identified as among the 10% of genotypes with the highest %UPP and also showed high stability by HMGV, RPGV, and HMRPGV, and the high-and-stable %UPP attribute of 202, 205, and 206 was confirmed by AMMI. In general, high gluten strength has not been attributed to old wheat genotypes in previous studies (Fois et al., 2011; De Santis et al., 2017), which correspond to the results of the present study, where old genotypes all over do not show significantly higher %UPP values than modern wheat, and the %LargeUPP is even significantly lower than in modern wheat for some treatments. Thus, from the present study, it is shown that some specific old genotypes were identified with high and stable gluten strength over control and drought environments. The genetic background for this performance needs to be further elaborated on.
Grain yield and quality are the two factors determining the sufficiency and usefulness of wheat, respectively. Unfortunately, both are under threat of drought stress with the increasing global temperature (Allen et al., 2019). The extensive daily consumption of different types of wheat products such as bread, pastries, noodles, biscuits, and porridge amplifies the challenges of climate change on the end-use quality (Johansson et al., 2020; Johansson et al., 2023), which urged the need to improve wheat processing quality, especially from genetic aspects. Using molecular markers, several studies have reported the identification of responsible QTLs for grain protein concentration in common bread wheat (Prasad et al., 2003; Leonova et al., 2022), durum wheat (Blanco et al., 2006), and synthetic hexaploid wheat with emmer background (Kunert et al., 2007). By manipulating the expression of storage protein genes, satisfactory protein content was found to be retained while increasing the yield by altering field nitrogen supply and plant density (Zheng et al., 2022). However, due to the genetic complexity of wheat grain quality traits, the utilization of specific genes (especially transferred from alien species or wild relatives) for improving protein traits is difficult (Kulwal et al., 2005; Balyan et al., 2013). Therefore, valuable genetic resources need to be tested more widely in future studies to achieve successful quality breeding.
5 Conclusion
Global climate change is threatening food security in two aspects, i.e., quantity and quality. Improvements in the stability of the breadmaking quality of wheat are urgently needed against increasing drought events. The baking quality is determined by its protein composition parameters, especially the protein concentration and gluten strength, which are known to be affected by genotype × environment interactions. Therefore, in search of genotypic resources and candidate genes contributing to high and stable quality, this study investigated the impact of drought on the protein composition of wheat materials with a wide genetic background. Due to its highest total extractable proteins among genotype groups, chromosome 3R demonstrated a promising effect in increasing protein concentration that has been reduced in modern breeding lines. Furthermore, the simultaneous high performance in protein content and yield found in 252(3R), 253(3R), and 258 (2R) proposed possibilities of using wheat-alien introgression lines as genetic resources to achieve high protein quality without losing yield. Our stability analysis results also supported the contribution of 3R to high and stable protein content across varying environments. Differently, some of the old Swedish cultivars were found to be a potential genetic resource for high and stable gluten strength. Thus, this study identifies 3R and some specific old Swedish cultivars as the two major sources for genes related to protein concentration and gluten strength. Breeding targets need to be adjusted accordingly to achieve wheat lines with stable and high baking quality under increasing drought conditions.
Data availability statement
The raw data supporting the conclusion of this article will be made available by the authors, without undue reservation.
Author contributions
EJ, RK, AC, and YL contributed to conception and design of the study. YL collected the raw data. YL performed the statistical analysis. YL wrote the first draft of the manuscript. EJ, RK, and AC provided supervision on this work. EJ contributed to project administration and funding acquisition. All authors contributed to the article and approved the submitted version.
Funding
This research was funded by Trees and Crops for the Future (TC4F) and SLU Grogrund.
Acknowledgments
Special thanks go to Maria Luisa Prieto-Linde for providing significant technical and laboratory assistance throughout this study.
Conflict of interest
The authors declare that the research was conducted in the absence of any commercial or financial relationships that could be construed as a potential conflict of interest.
Publisher’s note
All claims expressed in this article are solely those of the authors and do not necessarily represent those of their affiliated organizations, or those of the publisher, the editors, and the reviewers. Any product that may be evaluated in this article, or claim that may be made by its manufacturer, is not guaranteed or endorsed by the publisher.
Supplementary material
The Supplementary Material for this article can be found online at: https://www.frontiersin.org/articles/10.3389/frfst.2023.1163412/full#supplementary-material
Abbreviations
C, control treatment; EDS, early drought treatment; LDS, late drought treatment; LPP, large polymeric proteins; SPP, smaller polymeric protein; LMP, large monomeric protein; SMP, smaller monomeric protein; TOTE, total SDS-extractable protein; TOTU, total SDS-unextractable protein; %UPP, the percentage of SDS-unextractable polymeric protein in the total polymeric protein; %LargeUPP, the percentage of large SDS-unextractable polymeric protein in the total large polymeric protein; %LUMP, the percentage of large SDS-unextractable monomeric protein in the total large monomeric protein; Mon/Pol, the ratio of total monomeric protein to the total polymeric protein; SNPP, spike number per plant; SPL, spike length; PSPP, productive spikes per plant; TGW, 1000-grain weight; GPS, grains per spike; GWPS, grain weight per spike; GWPP, grain weight per plant; and GPP, grains per plant.
References
Allen, M., Antwi-Agyei, P., Aragon-Durand, F., Babiker, M., Bertoldi, P., Bind, M., et al. (2019). Technical Summary: Global warming of 1.5° C. An IPCC Special Report on the impacts of global warming of 1.5° C above pre-industrial levels and related global greenhouse gas emission pathways. context Strength. Glob. response threat Clim. change, Sustain. Dev. efforts eradicate poverty.
Asseng, S., and Turner, N. (2007). Modelling genotype× environment× management interactions to improve yield, water use efficiency and grain protein in wheat. Frontis, 91–102.
Balyan, H. S., Gupta, P. K., Kumar, S., Dhariwal, R., Jaiswal, V., Tyagi, S., et al. (2013). Genetic improvement of grain protein content and other health-related constituents of wheat grain. Plant Breed. 132 (5), 446–457. doi:10.1111/pbr.12047
Bhullar, S., and Jenner, C. (1985). Differential responses to high temperatures of starch and nitrogen accumulation in the grain of four cultivars of wheat. Funct. Plant Biol. 12 (4), 363–375. doi:10.1071/PP9850363
Blanco, A., Simeone, R., and Gadaleta, A. (2006). Detection of QTLs for grain protein content in durum wheat. Theor. Appl. Genet. 112 (7), 1195–1204. doi:10.1007/s00122-006-0221-6
Branlard, G., and Dardevet, M. (1985). Diversity of grain proteins and bread wheat quality: I. Correlation between gliadin bands and flour quality characteristics. J. Cereal Sci. 3 (4), 329–343. doi:10.1016/S0733-5210(85)80006-0
Chaudhry, S., and Sidhu, G. P. S. (2022). Climate change regulated abiotic stress mechanisms in plants: A comprehensive review. Plant Cell Rep. 41 (1), 1–31. doi:10.1007/s00299-021-02759-5
Cooper, M., Woodruff, D. R., Phillips, I. G., Basford, K. E., and Gilmour, A. R. (2001). Genotype-by-management interactions for grain yield and grain protein concentration of wheat. Field Crops Res. 69 (1), 47–67. doi:10.1016/S0378-4290(00)00131-3
Crespo-Herrera, L. A., Garkava-Gustavsson, L., and Åhman, I. (2017). A systematic review of rye (Secale cereale L) as a source of resistance to pathogens and pests in wheat (Triticum aestivum L). Hereditas 154 (1), 14. doi:10.1186/s41065-017-0033-5
De Santis, M. A., Giuliani, M. M., Giuzio, L., De Vita, P., Lovegrove, A., Shewry, P. R., et al. (2017). Differences in gluten protein composition between old and modern durum wheat genotypes in relation to 20th century breeding in Italy. Eur. J. Agron. 87, 19–29. doi:10.1016/j.eja.2017.04.003
Desheva, G., Valchinova, E., Kyosev, B., and Stoyanova, S. (2014). Grain physical characteristics and bread-making quality of alternative cereals<br>towards common and durum wheat. Emir. J. Food Agric. 26, 418–424. doi:10.9755/ejfa.v26i5.17960
Dhaliwal, A., Mares, D., and Marshall, D. (1987). Effect of 1B/1R chromosome translocation on melling and quality characteristics of bread wheats. Cereal Chem. 64 (2), 72–76.
Feil, B. (1997). The inverse yield-protein relationship in cereals: Possibilities and limitations for genetically improving the grain protein yield. Trends Agron. 1 (103), e119.
Fenn, D., Lukow, O., Bushuk, W., and Depauw, R. (1994). Milling and baking quality of 1BL/1RS translocation wheats. I: Effects of genotype and environment. Cereal Chem. 71 (2), 189–195.
Fischer, R., and Maurer, R. (1978). Drought resistance in spring wheat cultivars. I. Grain yield responses. Aust. J. Agric. Res. 29 (5), 897–912. doi:10.1071/AR9780897
Fois, S., Schlichting, L., Marchylo, B., Dexter, J., Motzo, R., and Giunta, F. (2011). Environmental conditions affect semolina quality in durum wheat (Triticum turgidum ssp. durum L) cultivars with different gluten strength and gluten protein composition. J. Sci. Food Agric. 91 (14), 2664–2673. doi:10.1002/jsfa.4509
Friebe, B., Jiang, J., Raupp, W., McIntosh, R., and Gill, B. (1996). Characterization of wheat-alien translocations conferring resistance to diseases and pests: Current status. Euphytica 91 (1), 59–87. doi:10.1007/BF00035277
Gagliardi, A., Carucci, F., Masci, S., Flagella, Z., Gatta, G., and Giuliani, M. M. (2020). Effects of genotype, growing season and nitrogen level on gluten protein assembly of durum wheat grown under mediterranean conditions. Agronomy 10 (5), 755. doi:10.3390/agronomy10050755
Geisslitz, S., Longin, C. F. H., Scherf, K. A., and Koehler, P. (2019). Comparative study on gluten protein composition of ancient (einkorn, emmer and spelt) and modern wheat species (durum and common wheat). Foods 8 (9), 409. doi:10.3390/foods8090409
Giunta, F., Motzo, R., and Deidda, M. (1993). Effect of drought on yield and yield components of durum wheat and triticale in a Mediterranean environment. Field Crops Res. 33 (4), 399–409. doi:10.1016/0378-4290(93)90161-F
Graybosch, R. A., Peterson, C., Baenziger, P. S., and Shelton, D. (1995). Environmental modification of hard red winter wheat flour protein composition. J. Cereal Sci. 22 (1), 45–51. doi:10.1016/S0733-5210(05)80006-2
Graybosch, R. A., Peterson, C., Hansen, L., Worrall, D., Shelton, D., and Lukaszewski, A. (1993). Comparative flour quality and protein characteristics of 1BL/1RS and 1AL/1RS wheat-rye translocation lines. J. Cereal Sci. 17 (2), 95–106. doi:10.1006/jcrs.1993.1010
Gregorova, Z., Kovacik, J., Klejdus, B., Maglovski, M., Kuna, R., Hauptvogel, P., et al. (2015). Drought-induced responses of physiology, metabolites, and PR proteins in Triticum aestivum. J. Agric. food Chem. 63 (37), 8125–8133. doi:10.1021/acs.jafc.5b02951
Gupta, R. B., Khan, K., and Macritchie, F. (1993). Biochemical basis of flour properties in bread wheats. I. Effects of variation in the quantity and size distribution of polymeric protein. J. Cereal Sci. 18 (1), 23–41. doi:10.1006/jcrs.1993.1031
Gupta, R. B., Masci, S., Lafiandra, D., Bariana, H. S., and MacRitchie, F. (1996). Accumulation of protein subunits and their polymers in developing grains of hexaploid wheats. J. Exp. Bot. 47 (9), 1377–1385. doi:10.1093/jxb/47.9.1377
Guzmán, C., Autrique, J. E., Mondal, S., Singh, R. P., Govindan, V., Morales-Dorantes, A., et al. (2016). Response to drought and heat stress on wheat quality, with special emphasis on bread-making quality, in durum wheat. Field Crops Res. 186, 157–165. doi:10.1016/j.fcr.2015.12.002
Hailu, F., and Merker, A. (2008). Variation in gluten strength and yellow pigment in Ethiopian tetraploid wheat germplasm. Genet. Resour. Crop Evol. 55 (2), 277–285. doi:10.1007/s10722-007-9233-6
Hakim, M., Hossain, A., da Silva, J. A. T., Zvolinsky, V., and Khan, M. (2012). Protein and starch content of 20 wheat (Triticum aestivum) genotypes exposed to high temperature under late sowing conditions. J. Sci. Res. 4 (2), 477. doi:10.3329/jsr.v4i2.8679
Hsam, S., Mohler, V., Hartl, L., Wenzel, G., and Zeller, F. (2000). Mapping of powdery mildew and leaf rust resistance genes on the wheat-rye translocated chromosome T1BL· 1RS using molecular and biochemical markers. Plant Breed. 119 (1), 87–89. doi:10.1046/j.1439-0523.2000.00444.x
Jia, Y., Fabre, J., and Aussenac, T. (1996). Effects of growing location on response of protein polymerization to increased nitrogen fertilization for the common wheat cultivar soissons: Relationship with some aspects of the breadmaking quality. Cereal Chem. 73 (5), 526–532.
Johansson, E., Branlard, G., Cuniberti, M., Flagella, Z., Hüsken, A., Nurit, E., et al. (2020). “Genotypic and environmental effects on wheat technological and nutritional quality,” in Wheat quality for improving processing and human health (Cham: Springer), 171–204.
Johansson, E., Kuktaite, R., Labuschagne, M., Lama, S., Lan, Y., Nakimbugwe, D., et al. (2023). “Adaptation to abiotic stress factors and their effects on cereal and pseudocereal grain quality,” in Developing sustainable and health promoting cereals and pseudocereals (Amsterdam, Netherlands: Elsevier), 339–358.
Johansson, E., Malik, A. H., Hussain, A., Rasheed, F., Newson, W. R., Plivelic, T., et al. (2013). Wheat gluten polymer structures: The impact of genotype, environment, and processing on their functionality in various applications. Cereal Chem. 90 (4), 367–376. doi:10.1094/CCHEM-08-12-0105-FI
Johansson, E., Prieto-Linde, M. L., and Larsson, H. (2021). Locally adapted and organically grown landrace and ancient spring cereals—a unique source of minerals in the human diet. Foods 10 (2), 393. doi:10.3390/foods10020393
Josephides, C., Joppa, L., and Youngs, V. (1987). Effect of chromosome 1B on gluten strength and other characteristics of durum wheat 1. Crop Sci. 27 (2), 212–216. doi:10.2135/cropsci1987.0011183X002700020016x
Kibite, S., and Evans, L. (1984). Causes of negative correlations between grain yield and grain protein concentration in common wheat. Euphytica 33 (3), 801–810. doi:10.1007/BF00021906
Kim, W., Johnson, J. W., Baenziger, P. S., Lukaszewski, A. J., and Gaines, C. S. (2004). Agronomic effect of wheat-rye translocation carrying rye chromatin (1R) from different sources. Crop Sci. 44 (4), 1254–1258. doi:10.2135/cropsci2004.1254
Kimball, B. A., Morris, C. F., Pinter, P. J., Wall, G. W., Hunsaker, D. J., Adamsen, F. J., et al. (2001). Elevated CO2, drought and soil nitrogen effects on wheat grain quality. New Phytol. 150 (2), 295–303. doi:10.1046/j.1469-8137.2001.00107.x
Kulwal, P., Kumar, N., Kumar, A., Gupta, R. K., Balyan, H. S., and Gupta, P. K. (2005). Gene networks in hexaploid wheat: Interacting quantitative trait loci for grain protein content. Funct. Integr. genomics 5 (4), 254–259. doi:10.1007/s10142-005-0136-3
Kunert, A., Naz, A. A., Dedeck, O., Pillen, K., and Léon, J. (2007). AB-QTL analysis in winter wheat: I. Synthetic hexaploid wheat (T. Turgidum ssp. dicoccoides x T. Tauschii) as a source of favourable alleles for milling and baking quality traits. Theor. Appl. Genet. 115 (5), 683–695. doi:10.1007/s00122-007-0600-7
Lama, S. (2023). Wheat quality under a climate spell: A focus on protein, physico-chemical and growth characteristics evaluated by innovatively combined approaches. Alnarp: LTV Faculty. doi:10.54612/a.7kcg0aghdm
Lan, Y., Chawade, A., Kuktaite, R., and Johansson, E. (2022). Climate change impact on wheat performance—effects on vigour, plant traits and yield from early and late drought stress in diverse lines. Int. J. Mol. Sci. 23 (6), 3333. doi:10.3390/ijms23063333
Larroque, O. R., Gianibelli, M. C., Sanchez, M. G., and MacRitchie, F. (2000). Procedure for obtaining stable protein extracts of cereal flour and whole meal for size-exclusion HPLC analysis. Cereal Chem. 77 (4), 448–450. doi:10.1094/CCHEM.2000.77.4.448
Leiva, F., Vallenback, P., Ekblad, T., Johansson, E., and Chawade, A. (2021). Phenocave: An automated, standalone, and affordable phenotyping system for controlled growth conditions. Plants 10 (9), 1817. doi:10.3390/plants10091817
Leonova, I. N., Kiseleva, A. A., Berezhnaya, A. A., Stasyuk, A. I., Likhenko, I. E., and Salina, E. A. (2022). Identification of QTLs for grain protein content in Russian spring wheat varieties. Plants 11 (3), 437. doi:10.3390/plants11030437
Li, F., Li, Y., Cao, L., Liu, P., Geng, M., Zhang, Q., et al. (2018). Simultaneous transfer of leaf rust and powdery mildew resistance genes from hexaploid triticale cultivar Sorento into bread wheat. Front. Plant Sci. 9, 85. doi:10.3389/fpls.2018.00085
Li, Y., Wu, Y., Hernandez-Espinosa, N., and Peña, R. J. (2013). The influence of drought and heat stress on the expression of end-use quality parameters of common wheat. J. Cereal Sci. 57 (1), 73–78. doi:10.1016/j.jcs.2012.09.014
Ludlow, M. M., and Muchow, R. C. (1990). A critical evaluation of traits for improving crop yields in water-limited environments. Adv. Agron. 43, 107–153. doi:10.1016/S0065-2113(08)60477-0
Mago, R., Spielmeyer, W., Lawrence, G., Lagudah, E., Ellis, J., and Pryor, A. (2002). Identification and mapping of molecular markers linked to rust resistance genes located on chromosome 1RS of rye using wheat-rye translocation lines. Theor. Appl. Genet. 104 (8), 1317–1324. doi:10.1007/s00122-002-0879-3
Malik, A. H. (2012). “Governing grain protein concentration and composition in wheat and barley,”. Doctoral thesis (Missouri: SLU publication database).
Markgren, J., Hedenqvist, M., Rasheed, F., Skepö, M., and Johansson, E. (2020). Glutenin and gliadin, a piece in the puzzle of their structural properties in the cell described through Monte Carlo simulations. Biomolecules 10 (8), 1095. doi:10.3390/biom10081095
Markgren, J., Rasheed, F., Hedenqvist, M. S., Skepö, M., and Johansson, E. (2022). Clustering and cross-linking of the wheat storage protein α-gliadin: A combined experimental and theoretical approach. Int. J. Biol. Macromol. 211, 592–615. doi:10.1016/j.ijbiomac.2022.05.032
Merker, A. (1984). The rye genome in wheat breeding. Hereditas 100 (2), 183–191. doi:10.1111/j.1601-5223.1984.tb00118.x
Miller, T. (1984). The homoeologous relationship between the chromosomes of rye and wheat. Current status. Can. J. Genet. Cytol. 26 (5), 578–589. doi:10.1139/g84-091
Mkhabela, M., Bullock, P., Sapirstein, H., Courcelles, J., Abbasi, S., and Koksel, F. (2022). Exploring the influence of weather on gluten strength of hard red spring wheat (Triticum aestivum L) on the Canadian Prairies. J. Cereal Sci. 104, 103410. doi:10.1016/j.jcs.2021.103410
Mohler, V., Hsam, S., Zeller, F., and Wenzel, G. (2001). An STS marker distinguishing the rye-derived powdery mildew resistance alleles at the Pm8/Pm17 locus of common wheat. Plant Breed. 120 (5), 448–450. doi:10.1046/j.1439-0523.2001.00622.x
Mosse, J. (1990). Nitrogen-to-protein conversion factor for ten cereals and six legumes or oilseeds. A reappraisal of its definition and determination. Variation according to species and to seed protein content. J. Agric. food Chem. 38 (1), 18–24. doi:10.1021/jf00091a004
Osborne, T. (1907). The proteins of the wheat kernel. Washington, DC: Carnegie Institution of Washington, Publication no. 84, Judd & Detweiler. Inc. doi:10.1126/science.26.677.865
Oury, F.-X., Berard, P., Brancourt-Hulmel, M., Heumez, E., Pluchard, P., Rousset, M., et al. (2003). Yield and grain protein concentration in bread wheat: A review and a study of multi-annual data from a French breeding program [Triticum aestivum L]. J. Genet. Breed. (Italy).
Park, S. H., Bean, S., Chung, O., and Seib, P. (2006). Levels of protein and protein composition in hard winter wheat flours and the relationship to breadmaking. Cereal Chem. 83 (4), 418–423. doi:10.1094/CC-83-0418
Payne, P. I., Nightingale, M. A., Krattiger, A. F., and Holt, L. M. (1987). The relationship between HMW glutenin subunit composition and the bread-making quality of British-grown wheat varieties. J. Sci. Food Agric. 40 (1), 51–65. doi:10.1002/jsfa.2740400108
Prasad, M., Kumar, N., Kulwal, P., Röder, M., Balyan, H., Dhaliwal, H., et al. (2003). QTL analysis for grain protein content using SSR markers and validation studies using NILs in bread wheat. Theor. Appl. Genet. 106 (4), 659–667. doi:10.1007/s00122-002-1114-y
Purnhauser, L., Bóna, L., and Láng, L. (2011). Occurrence of 1BL 1RS wheat-rye chromosome translocation and of Sr36/Pm6 resistance gene cluster in wheat cultivars registered in Hungary. Euphytica 179 (2), 287–295. doi:10.1007/s10681-010-0312-y
Rahmatov, M. (2016). “Genetic characterisation of novel resistance alleles to stem rust and stripe rust in wheat-alien introgression lines. Doctoral thesis,” in SLU epsilon open archive (Missouri: SLU publication database).
Ren, T., Ren, Z., Yang, M., Yan, B., Tan, F., Fu, S., et al. (2018). A novel PPARα/γ agonist, propane-2-sulfonic acid octadec-9-enyl-amide, ameliorates insulin resistance and gluconeogenesis in vivo and vitro. Mol. Breed. 38 (8), 1–8. doi:10.1016/j.ejphar.2018.02.029
Rozbicki, J., Ceglińska, A., Gozdowski, D., Jakubczak, M., Cacak-Pietrzak, G., Mądry, W., et al. (2015). Influence of the cultivar, environment and management on the grain yield and bread-making quality in winter wheat. J. Cereal Sci. 61, 126–132. doi:10.1016/j.jcs.2014.11.001
Salvador-Moreno, N., Ryan, P., Holguín, I., Delhaize, E., Benito, C., and Gallego, F. (2018). Transcriptional profiling of wheat and wheat-rye addition lines to identify candidate genes for aluminum tolerance. Biol. Plant. 62 (4), 741–749. doi:10.1007/s10535-018-0804-5
Senapati, N., Stratonovitch, P., Paul, M. J., and Semenov, M. A. (2019). Drought tolerance during reproductive development is important for increasing wheat yield potential under climate change in Europe. J. Exp. Bot. 70 (9), 2549–2560. doi:10.1093/jxb/ery226
Shewry, P. R., Tatham, A. S., Halford, N. G., Barker, J. H. A., Hannappel, U., Gallois, P., et al. (1994). Opportunities for manipulating the seed protein composition of wheat and barley in order to improve quality. Transgenic Res. 3 (1), 3–12. doi:10.1007/BF01976021
Shiferaw, B., Smale, M., Braun, H.-J., Duveiller, E., Reynolds, M., and Muricho, G. (2013). Crops that feed the world 10. Past successes and future challenges to the role played by wheat in global food security. Food Secur. 5 (3), 291–317. doi:10.1007/s12571-013-0263-y
Simmonds, N. W. (1995). The relation between yield and protein in cereal grain. J. Sci. Food Agric. 67 (3), 309–315. doi:10.1002/jsfa.2740670306
Tahir, I. S. A., Nakata, N., Ali, A. M., Mustafa, H. M., Saad, A. S. I., Takata, K., et al. (2006). Genotypic and temperature effects on wheat grain yield and quality in a hot irrigated environment. Plant Breed. 125 (4), 323–330. doi:10.1111/j.1439-0523.2006.01236.x
Veraverbeke, W. S., and Delcour, J. A. (2002). Wheat protein composition and properties of wheat glutenin in relation to breadmaking functionality. Crit. Rev. Food Sci. Nutr. 42 (3), 179–208. doi:10.1080/10408690290825510
Yang, E., Li, G., Li, L., Zhang, Z., Yang, W., Peng, Y., et al. (2016). Characterization of stripe rust resistance genes in the wheat cultivar Chuanmai45. Int. J. Mol. Sci. 17 (4), 601. doi:10.3390/ijms17040601
Zhang, P., He, Z., Zhang, Y., Xia, X., Chen, D., and Zhang, Y. (2008). Association between % SDS-unextractable polymeric protein (%UPP) and end-use quality in Chinese bread wheat cultivars. Cereal Chem. 85 (5), 696–700. doi:10.1094/CCHEM-85-5-0696
Zheng, B., Jiang, J., Wang, L., Huang, M., Zhou, Q., Cai, J., et al. (2022). Reducing nitrogen rate and increasing plant density accomplished high yields with satisfied grain quality of soft wheat via modifying the free amino acid supply and storage protein gene expression. J. Agric. food Chem. 70 (7), 2146–2159. doi:10.1021/acs.jafc.1c07033
Keywords: protein composition, protein concentration, gluten strength, drought, yield, wheat
Citation: Lan Y, Kuktaite R, Chawade A and Johansson E (2023) Diverse wheat lines to mitigate the effect of drought on end-use quality. Front. Food. Sci. Technol. 3:1163412. doi: 10.3389/frfst.2023.1163412
Received: 10 February 2023; Accepted: 27 June 2023;
Published: 11 July 2023.
Edited by:
Nikolai I. Lebovka, National Academy of Sciences of Ukraine, UkraineReviewed by:
Nicole Frantová, Mendel University in Brno, CzechiaBin Xiao Fu, Canadian Grains Commission (CGC), Canada
Copyright © 2023 Lan, Kuktaite, Chawade and Johansson. This is an open-access article distributed under the terms of the Creative Commons Attribution License (CC BY). The use, distribution or reproduction in other forums is permitted, provided the original author(s) and the copyright owner(s) are credited and that the original publication in this journal is cited, in accordance with accepted academic practice. No use, distribution or reproduction is permitted which does not comply with these terms.
*Correspondence: Eva Johansson, eva.johansson@slu.se