- 1FFoQSI GmbH—Austrian Competence Centre for Feed and Food Quality, Safety and Innovation, Tulln, Austria
- 2Institute of Food Technology, University of Natural Resources and Life Sciences (BOKU), Vienna, Austria
Peeling is a standard food processing operation that removes the outer layer of fruits and vegetables. It can improve the appearance and texture of many fruits and vegetables and is often necessary for further food preparation. Developing new and innovative peeling methods to minimise losses and enhance product quality is an area of active research in the food industry. The objective of this study was to evaluate how PEF affects the peeling ability of tomatoes and kiwi fruits, as well as the chemical and physical characteristics of the resulting peeled products. In detail, monopolar exponential decay pulses were applied, with an electric field strength of 1.0 kV/cm and a resulting total energy input in the range of 0.6 and 5.0 kJ/kg for tomatoes and 1.2 and 12.6 kJ/kg for kiwi fruits. Two methods were used to compare the effectiveness of PEF treatments with traditional peeling methods: hot-water blanching (98°C for 60 s) and lye peeling (98°C for 45 s, in 2% NaOH solution). The peeling efficiency was evaluated through manual and mechanical methods as well as measuring weight loss. The quality of the final peeled product was assessed by mechanical properties of the pericarp, colour (L*, a*, b* scale), ascorbic acid content, chlorophyll a and b, carotenoids, total polyphenols content and antioxidant activity. The PEF treatment applied to whole red tomatoes (1.0 kV/cm, 5.0 kJ/kg) and whole kiwi fruits (1.0 kV/cm, 12.6 kJ/kg) resulted in a significant decrease (p ≤ 0.05) of up to 43% and 83% in the force required for mechanical peeling, respectively. The PEF treatment showed comparable or superior peeling ability and significantly reduced product losses compared to hot-water blanching and lye peeling methods. However, the softening and the weight losses tend to increase by increasing the pulses for both investigated matrices. Moreover, by applying PEF instead of blanching or lye peeling, the chemical quality and the colour were better preserved (ΔE < 2). This study proved that PEF could be a promising non-thermal technology to better peel tomatoes and kiwi fruits without affecting their final quality. Consequently, applying PEF as a treatment to facilitate peeling has remarkable potential as an industrial application to reduce energy consumption and issues related to wastewater management typically occurring during the peeling with lye agents.
1 Introduction
Peeling is a fundamental unit operation in the fruit and vegetable industry, and it is usually performed to prepare plant products for subsequent processes such as cooking, drying, freezing, canning and packaging. It involves eliminating undesired or inedible outer material from fruits and vegetables. However, removing the outer layer can cause damage to fruits and vegetables due to the unwanted cellular damage to the pericarp. Generally, manual peeling is the method that gives the highest quality end product in terms of weight loss of the flesh; however, it is not feasible for large-scale production, and it is high labour and time demanding (Kohli et al., 2021). Industrially, peeling is performed by abrasion, blanching, pressurised steam and lye peeling (Garcia and Barrett, 2006b; Rock et al., 2012; Gómez-López et al., 2014). These thermal, chemical and mechanical peeling methods require large amounts of energy, water or chemicals. Evidence suggests that the peeling process can be further improved to reduce peeling losses, improve the final product quality and minimise the environmental impact and energy consumption (Garofalo et al., 2017). In particular, the chemical and energetic problems associated with lye and steam peeling of tomatoes necessitate alternative and sustainable peeling methods that can maintain peeling performances while minimising peeling losses and the impact on product quality (Li et al., 2014a). In recent years, there has been a growing body of literature that recognises the importance of finding alternative novel peeling methods such as infrared radiation, ultrasound, ohmic heating and pulsed electric fields assisted peeling (Li et al., 2014b; Li et al., 2014c; Gao et al., 2018; Andreou et al., 2020; Gavahian and Sastry, 2020). Among these technologies, PEF has been proven to be an excellent alternative technology in tomato peeling, and its implementation in existing industrial plants has proven feasible and environmentally favourable (Arnal et al., 2018; Pataro et al., 2018). Moreover, in the last decade, PEF has been highlighted as the potential critical process toward a more sustainable and efficient food supply chain (Buchmann and Mathys, 2019). Specifically, PEF is an innovative technology primarily investigated to improve conventional processes in the food industry (Barba et al., 2015). Depending on the set parameters of the PEF (i.e., electric field strength, energy input, pulse duration, treatment time) and the specific plant cell characteristics (i.e., size, shape, composition, orientation in the electric field), the cell can be electroporated reversibly or irreversibly (Knorr et al., 2011), to enhance the rates of heat and mass transfer phenomena and achieve efficient microbial inactivation (Raso et al., 2016). However, the PEF-induced mechanism responsible for improving tomatoes’ peeling ability is still unclear. Additionally, based on the author’s knowledge, using PEF to improve the peeling ability of other fruits and vegetables successfully has not been reported previously. This study aimed to examine the effect of PEF on the peeling performance of tomatoes and kiwi fruits and the chemical and physical quality of the peeled products. Another aim was to provide new insights into the potential replacement or improvement of the currently energy-intensive and environmentally damaging peeling methods using PEF.
2 Materials and methods
2.1 Materials
Tomatoes (Lycopersicon esculentum cv. Daniela) and green kiwi fruits (Actinidia deliciosa cv. Hayward) were purchased from a local market (Vienna, Austria). The tomatoes were selected based on the ripening stage and were characterised by more than 90% red colour on the surface, as defined by the US standard for grades of fresh tomatoes (USDA, 1991). Only non-damaged fruits with similar sizes and similar external appearance were selected. In particular, tomatoes of 57–67 mm diameter calibre and hue angle = 43.3 ± 2.7 and kiwi fruits 85–95 g/piece calibre were selected to use fruits with homogeneous size and ripening stage. Additional analyses such as total soluble solids (Tomatoes = 6.21 ± 0.1 °Brix, kiwi = 12.5 ± 0.4 °Brix), pH (Tomatoes = 4.32 ± 0.03, kiwi = 3.54 ± 0.24), titratable acidity (Tomatoes = 0.46 ± 0.07 g citric acid/100 g fresh weight, kiwi = 1.41 ± 0.05 g citric acid/100 g fresh weight) and moisture content (Tomatoes = 91.5 ± 0.9%, kiwi = 83.2 ± 1.1%) were assessed for fresh fruits randomly selected from each batch. HPLC-grade acetone, ascorbic acid and 2,4,6 tripyridyl-s-triazine (TPTZ) were purchased from Sigma-Aldrich (Steinheim, Germany). Sodium hydroxide in pellets and ammonium acetate were purchased from Merck (Darmstadt, Germany). ABTS⋅+ (2,2′-azino-bis- (3-ethylbenzothiazolin-6-sulfonic) was purchased from Fluka (Buchs, Switzerland). Hydrochloric acid (HCl) was purchased from Carl Roth (Karlsruhe, Germany).
2.2 Methods
2.2.1 Pulsed electric field treatments
PEF treatments were carried out using a laboratory scale batch system (Cellcrack 1, DIL, Germany) consisting of a high-voltage power supply, a capacitor with a capacity of 0.5 μF and a spark gap used as a switch. The system can generate monopolar exponential decay pulses. The generator was connected to a parallel plate treatment chamber with an electrode distance of 13.5 cm and a total volume of 4.0 L. For each treatment, 500 ± 20 g of tomatoes and kiwi fruits were placed in the treatment chamber and filled up with tap water (T = 20°C ± 1°C, conductivity 290–310 μS/cm) to a final weight of 1.8 kg. This specific sample/water ratio was chosen to guarantee that the sample was fully immersed in the treatment medium. The applied electric field was fixed to 1 kV/cm, the number of pulses was between 10 and 500, and the applied frequency was 2 Hz. This PEF treatment delivered an energy of 45.6 J/pulse, resulting in total specific energy for tomatoes and kiwi fruits between 0.25–5.0 and 0.63–12.6 kJ/kg, respectively. The energy per pulse and the total specific energy were calculated as reported by Ostermeier et al. (2018). The actual voltage was measured using a high-voltage probe (P6015A, Tektronix, United Kingdom) and an oscilloscope (TBS 1102B-EDU, Tektronix, United Kingdom). The pulse width was determined at 37% of the peak voltage detected and was equivalent to 100 μs. Thus, the resulting treatment time varied between 1 and 50 ms. The listed PEF parameters were selected based on preliminary trials. To establish a control reference (untreated), samples of tomatoes and kiwi fruits were prepared by immersing them in tap water for the same duration and at the same sample/water ratio used in the PEF treatments. The medium’s electrical conductivity and temperature were measured before and after each PEF treatment using a digital thermometer (Testo 922, Testo GmbH, Austria) and a conductivity meter (FiveGo F3, Mettler Toledo, Switzerland). However, a temperature difference lower than 3°C and a medium conductivity difference lower than 50 μS/cm were recorded after the treatments, independently of the applied energy input.
2.2.2 Blanching and lye peeling
To compare PEF treatment with conventional peeling methods, the whole fruits were subjected to hot water blanching and lye peeling. The blanching treatment was performed in a stainless-steel tank with a diameter of 15 cm, and a total volume of 4.0 L placed on a magnetic stirrer with a ceramic heating plate (C-MAG HS 7, IKA, Germany). For each treatment, 500 ± 20 g of tomatoes, and kiwi fruits were immersed in 1.3 L of hot water (98°C) for 60 s (Rock et al., 2012). The sample/water ratio was chosen to guarantee the complete immersion of the samples in the treatment medium. Lye peeling, also known as caustic peeling, was conducted in 1.3 kg of NaOH solution (2%), as Rock et al. (2012) suggested. The solution was placed in a glass tank and heated to 98°C. For each treatment, 500 ± 20 g of tomatoes and kiwi fruits were immersed in the hot solution for 45 s. The temperature of the peeling solution was constantly monitored using a digital thermometer (Testo 922, Testo GmbH, Austria). After both peeling treatments, the fruits were rapidly cooled at 18°C, submerging them in ice-cold tap water to prevent any additional cooking effect. At least ten repetitions per treatment were conducted for both raw materials.
2.2.3 Cell disintegration index
The degree of cell permeabilisation after PEF, blanching and lye peeling was determined by calculating the cell disintegration index (CDI). The electrical conductivity was measured with an impedance measurement device (Impedance Analyser, Sigma Check, Germany). The frequency was in the range of 1.4 kHz—11.2 MHz. The samples were obtained by cutting a cylindrical shape (diameter 1.0 cm, length 1.0 cm) from the fruit pericarp. A polyethylene tube held together two stainless steel electrodes between which the samples were positioned. Impedance curves were obtained for both the intact and the treated samples. CDI was calculated using the equation described by Knorr and Angersbach (1998), considering the impedance values at a frequency of 5.5 kHz (low-frequency) and 1.4 MHz (high-frequency), as reported by Fauster et al. (2018). This equation measures the percentage of permeabilised cells in the plant material and is commonly used to evaluate the effectiveness of the PEF parameters. Specifically, the CDI value varies between 0 (representing intact cells) and 1 (representing total disintegrated cells). For the preparation of totally disintegrated tissue, the freezing-thawing cycles were applied, as reported by Wang et al. (2020). Specifically, untreated samples were subjected to three cycles, each comprising a freezing stage (−10°C, 2 h) followed by a thawing stage at room temperature (≈22°C, 2 h). At least ten repetitions were conducted for both raw and treated materials.
2.2.4 Visual peeling assessment and peeling losses
The subjective grading system reported by Li et al. (2009) was used to assess the peeling ability of tomatoes and kiwi fruits treated under different conditions. The system is based on five levels of peeling ability, and they are described in Table 1. Before peeling, a pre-cut (1.5 cm × 5.0 cm) was made in the fruit peel with a custom-made cutting device consisting of two sharp stainless-steel blades set at a fixed distance (1.5 cm). During peeling, a sharp blade was utilised to detach the peel from the pericarp. Each fruit was manually peeled for a maximum of 60 s and exceeded the maximum time the sample was automatically assigned to a grade of 1 (Grade 1—Removal of the peel is too difficult). A score higher than 4 was considered an acceptable level for peeling ability. Ten peeling repetitions per treatment condition were conducted for raw and treated materials. Peeling losses were calculated using an electronic balance based on the weight change of the investigated fruits before and after peeling and were expressed as a percentage (%), as reported by Garcia and Barrett (2006a).
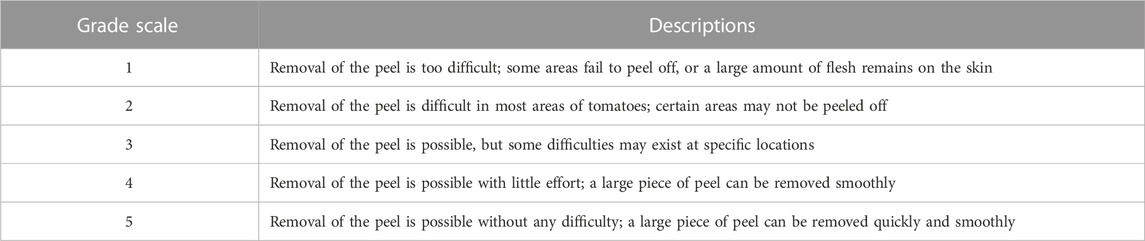
TABLE 1. Definitions of the five levels to assess the peeling ability based on Li et al. (2009).
2.2.5 Mechanical peeling
The use of texture analysers for mechanical peeling has mainly been considered a valid approach to compare emerging peeling methods with established industrial methods (Li et al., 2014a; Vidyarthi et al., 2019b; Andreou et al., 2020). In this study, mechanical peeling was performed using a texture analyser (MODEL TA-XT2i; StableMicro Systems, Godalming, Surrey, United Kingdom) for untreated and treated whole fruits, slightly modifying the method reported by Andreou et al. (2020). A rectangular pre-cut (1.5 cm × 5.0 cm) was made on the tomato surface along the direction from the stem to the blossom end with a custom-made cutting device. The first 1.5 cm2 of the fruit peel area was placed into a suitable clamp probe. The peel was removed with a velocity of 2 mm/s for a total distance of 30 mm, keeping the peel perpendicular to the direction of the probe. The area under the resulting force curve (N*mm) was recorded and considered as the work required to detach the peel from the fruit pericarp. At least ten mechanical peeling repetitions per treatment condition were conducted for raw and treated materials.
2.2.6 Mechanical properties of pericarp
The firmness of the pericarp after peeling was deemed a crucial factor in determining product acceptability and quality. It is generally stated that the higher firmness corresponds to a better quality of the pericarp (Vidyarthi et al., 2019a). The fruit was placed horizontally on a suitable stand to perform the method, and the measurements were conducted on its equatorial area. A penetration test was performed through a texture analyser (MODEL TA-XT2i; StableMicro Systems, Godalming, Surrey, United Kingdom) equipped with a 5 kg load cell and a 6 mm diameter flat cylindrical probe. The test velocity was set at 1 mm/s for a total distance of 10 mm. The maximum force (N) was considered the firmness of the pericarp. The described method was performed 10 times per treatment condition for raw and treated materials.
2.2.7 Colour of fruit pericarp after peeling
The colour of the peeled tomatoes and kiwi fruits was used to assess the quality of the final product. The colour of the flesh of fruit untreated and treated under different conditions was determined using an image analysis system (IRIS, Alpha MOS, France). The analysis was performed at room temperature and approximately 10 min after the peeling procedures. The colour parameters L*, a*, and b* were measured from a 1 cm2 squared area on the tomato and kiwi flesh. To ensure the homogeneous evaluation of the samples, each fruit was measured in five different areas around the equatorial fruit axes. The parameter L* indicates the lightness and ranges from 0 (black) to 100 (white), the a* value ranges from green (<0) to red (>0), and the b* value ranges from blue (<0) to yellow (>0). Additionally, the colour was evaluated by measuring hue angle (h°), chroma (C*) and colour difference (ΔE) between the sample colour (L2*, a2*, b2*) and the reference colour (untreated) (L1*, a1*, b1*), according to the following equations:
Small ΔE values indicate minor colour changes after the peeling process. Differences in perceivable colour can be analytically classified as very distinct (ΔE > 3), distinct (1.5 < ΔE < 3) and minor differences (ΔE < 1.5) (Adekunte et al., 2010). Five fruits for both raw material and each treatment condition were used for the colour measurements.
2.2.8 Ascorbic acid content
Ascorbic acid (AA) content was determined using the method described by Munyaka et al. (2010), with few modifications. About 5 g of peeled tomatoes and kiwi fruits were mixed with 25 ml extraction solution (20 mM NaH2PO4, pH 2.1, 1 mM EDTA) and homogenised using an ultra Turrax mixer (T25, Ika, Germany) at 9,500 rpm for 30 s. After homogenisation, 2 ml of the sample was centrifuged at 20,000 × g at 8°C for 5 min. The supernatant was filtered through a 0.45 µm cellulose acetate syringe filter and immediately analysed through high-pressure liquid chromatography (HPLC) analysis. Samples from each raw material and each treatment condition were extracted in triplicate, and three technical repetitions were performed. The HPLC apparatus (Azura, Knauer, Germany) comprised an autosampler set at 5°C, a quaternary pump, a degasser and a UV detector. The mobile phase consisted of isocratic elution of ammonium acetate (10 mM, 1 mM EDTA, pH 3.0) for 5 min. The flow rate was set at 0.5 ml/min. The reverse phase C18 column (180 mm × 3 mm, 3 μm) was constantly maintained at 30°C, and the injection volume was 20 μl. The ascorbic acid content in the samples was identified by comparison with the standard ascorbic acid solutions (5–100 μg/ml) through the standard external method.
2.2.9 Chlorophyll a and b and total carotenoid content
The chlorophyll a and b and the total carotenoid content in tomato and kiwi flesh were determined according to the method described by Wellburn (1994). Fresh tomato and kiwi flesh (25 ± 1 g) was homogenised in a blender (Thermomix, TM 9, Vorwerk, Germany) for 30 s, and pure acetone was added for the extraction. The absorbance of the extracts was measured at 470, 645, and 662 nm through a UV/Visible scanning spectrophotometer (Shimadzu UV-1800, Shimadzu, Japan), and pure acetone was used as a blank. The chlorophyll a, the chlorophyll b and the total carotenoids content were calculated from the following equations:
where Ca is the content of chlorophyll a, Cb is the content of chlorophyll b, and Cx is the total content of carotenoids. The extractions were performed in triplicate, and three technical measurements were performed for each treatment condition.
2.2.10 Total phenolic content
Acidic methanolic extracts were evaluated to estimate tomatoes and kiwi pericarp’s total phenolic content (TPC). The extracts were prepared according to Martínez-Valverde et al. (2002), with slight modifications. An aliquot of 5.0 ± 0.1 g of the pulp was mixed with 50 ml of acidic methanol (MeOH:HCl, 85:15), shaken for 20 min in the dark and centrifuged for 20 min at 3,100 rcf. The TPC was estimated using the Folin Ciocalteu’s reagent, according to Singleton and Rossi (1965). Gallic acid (GA) was used as the reference standard (0.1–0.8 mg/ml), and the results were expressed as μg GA Equivalents/g fresh weight (FW).
2.2.11 Antioxidant activity (AOA)
The antioxidant activity of the extracts, which were obtained as outlined in Section 2.2.10, was assessed using two different in vitro assays. The first was conducted according to the method described by Re et al. (1999), with the modifications reported by Neri et al. (2020). Briefly, the ABTS + (2,2′-azino-bis- (3-ethylbenzothiazolin-6-sulfonic) was dissolved in deionised water to a final concentration of 7 mM; subsequently, the radical was formed by reaction with 2.45 mM potassium persulphate. The ABTS radical solution was diluted with deionised water to reach an absorbance of 0.70 ± 0.02 at 734 nm through a UV/Visible scanning spectrophotometer (Shimadzu UV-1800, Shimadzu, Japan). An aliquot of 10 μl of the extract was added to 990 μl of ABTS + solution, and the absorbance was recorded after 7 min. Finally, the percentage of inhibition (I%) was plotted as a function of concentration and the TEAC (Trolox Equivalent Antioxidant Capacity). Results are expressed as µmol of Trolox equivalents per gram of fresh weight (FW). The second assay was performed using the ferric-reducing ability of plasma (FRAP) as described by Benzie and Strain (1999). Specifically, the reduction of the ferric tripyridyl triazine (FeIII-TPTZ) complex to the ferrous form was evaluated by measuring the change in absorption at 593 nm through a UV/Visible scanning spectrophotometer (Shimadzu UV-1800, Shimadzu, Japan). The reagent was prepared by mixing acetate buffer 0.3 M (3.6 pH), TPTZ solution (10 mM) and FeCl3 solution (20 mM) in a ratio of 10:1:1. Finally, an aliquot of 0.2 ml of the extract was mixed with 1.3 ml of FRAP reagent and vortexed for 10 s. The reaction mixture was incubated for 30 min at 37°C, and the final absorbance was measured. FeSO4 solution (25–1,000 μmol) was the reference standard. The FRAP was expressed as µmol of Fe2+ Equivalent per gram of fresh weight (FW). The experiments were performed on three biological replicates with three technical replicates for raw and treated materials.
2.2.12 Statistics
The statistical analysis was performed with Statgraphics centurion 19 software (Statpoint Technologies, Virginia, United States). The significance level for the one-way ANOVA was set to p ≤ 0.05, and the Tukey test was applied to assess significant differences between the investigated parameters. The plotted results are reported as mean value ± standard deviation.
3 Results
3.1 Cell disintegration index (CDI)
Figure 1 illustrates the effect of PEF and thermal pre-treatments on tomatoes and kiwi fruits. The results indicate that the degree of cell disintegration in PEF-treated samples was significantly affected by the amount of energy input applied. Specifically, a higher energy input resulted in more significant cell disintegration, as evidenced by increased CDI values. The increasing energy input from 0.2 to 5.0 kJ/kg for tomatoes and from 0.6 to 12.6 kJ/kg for kiwi fruits leads to increased cell disruption up to 0.85 and 0.84, respectively. Previous studies have reported a similar relationship between PEF intensities and cell disintegration for the same investigated materials (Tylewicz et al., 2019; Andreou et al., 2020). The most intensive PEF treatments (5.0 and 12.6 kJ/kg) resulted in a CDI of 0.85 ± 0.02 for tomatoes and 0.84 ± 0.05 for kiwi fruits. The blanched and lye peeled tomatoes showed a CDI of 0.52 ± 0.02 and 0.49 ± 0.02, respectively. While the blanched and lye peeled kiwi fruits resulted in a CDI of 0.61 ± 0.03 and 0.62 ± 0.03, respectively. Therefore, with these specific experimental conditions, it can be assumed that the heating causes the effect on the cell disintegration index without an additional effect of the lye agent. The same cell disintegration obtained with blanching and lye peeling was achieved with 1.2 kJ/kg for the tomatoes and 3.2 kJ/kg for the kiwi fruits. As already reported by Angersbach et al. (2000); Fauster et al. (2020), this investigation demonstrates that depending on the selected raw material, applied energy inputs reached significant differences regarding CDI. The differences can be explained by the different characteristics of the investigated fruits in terms of cell sizes, structure, and electrical properties (Toepfl et al., 2014).
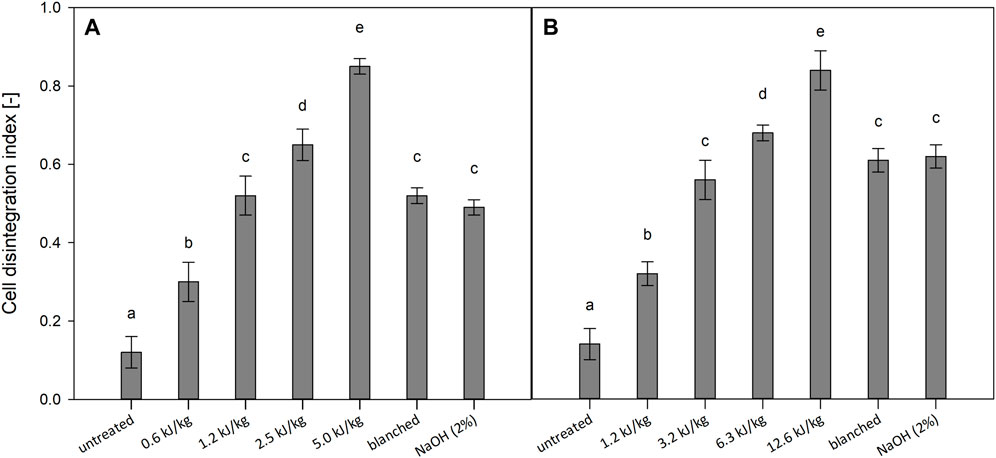
FIGURE 1. Cell disintegration index of PEF treated, blanched and lye peeled tomatoes (A) and kiwi fruits (B). Significant differences are indicated by different letters (p < 0.05).
3.2 Peeling ability assessment
The peeling ability based on the two selected methods is reported in Figure 2 for both investigated fruits. The peeling assessment of untreated tomatoes and kiwi fruits indicated that removing the peel manually was challenging for both materials, and this is consistent with prior findings by Koch et al. (2022) on tomatoes. The PEF treated tomatoes resulted in a visual peeling score from 4.0 to 4.9; therefore, the manual peeling ability was significantly improved for all the applied energy inputs. The blanching and the lye peeling led to a visual score of 4.4 and 4.9, respectively. These results are consistent with the ones reported by Li et al. (2014b) and Pan et al. (2009), who performed the lye peeling (10% NaOH) for 60 s and obtained a visual score of 4.7. It is noticeable that the PEF treated samples with an energy input between 1.2 and 5.0 kJ/kg showed the same peeling performance as the traditional processes (blanching and lye peeling). For the PEF treated kiwi fruits, a visual peeling score from 3.1 to 4.9 was assessed, and these results are similar to those reported for the tomatoes. Based on these experimental results, PEF specific energy input appeared to have a significant influence only between 1.2 and 3.2 kJ/kg, and an additional increase of the specific energy did not increase the peeling score in a statistically significant way. As reported in previous studies (Guldas and Bayindirli, 2004; Gómez-López et al., 2014), blanching and lye peeling of kiwi fruits resulted in a significant improvement of the peeling ability and a visual score of 4.5 and 4.8, respectively. The kiwi fruits PEF treated with energy input between 3.2 and 12.6 kJ/kg performed statistically similar to the blanched and lye peeled samples.
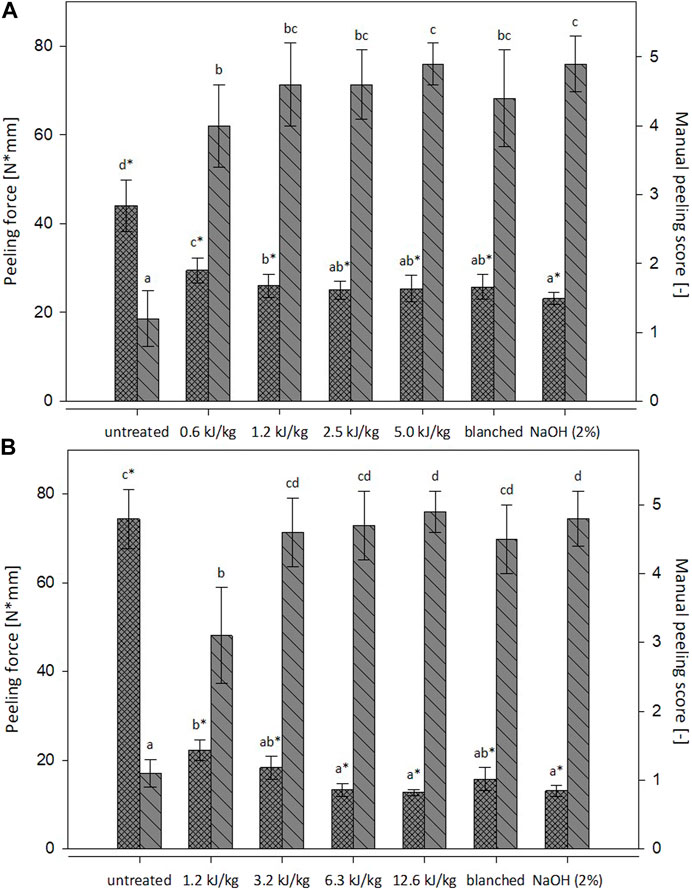
FIGURE 2. Mechanical peeling force (N*mm, ) and manual peeling score (-,
) for untreated, PEF-treated, blanched and lye-peeled tomatoes (A) and kiwi fruits (B). Significant differences within the same peeling method (mechanical peeling = a*, manual peeling = a) are indicated by different letters (p < 0.05).
Concerning the mechanical peeling, the force necessary for the detachment of the peel was statistically reduced for all the treated samples in both raw materials. Compared to the reference untreated samples, the PEF treatments reduced the mechanical force from 33% to 43% for the tomatoes and 70%–83% for the kiwi fruits. Andreou et al. (2020) reported similar results for PEF peeled tomatoes. Compared to the blanched and lye peeled samples, the PEF treated samples showed no significant difference, except for the lowest energy inputs applied for both fruits (0.6 and 1.2 kJ/kg). Both methods for the peeling ability assessment confirmed that PEF performance was comparable with blanching and lye peeling for both investigated materials. However, as Koch et al. (2022) reported, there is no proportional relationship between the energy input applied and the peeling ability. These results confirmed that the PEF treatment could improve the peeling ability of tomatoes and kiwi fruits even at low energy input (1.2 and 3.2 kJ/kg, respectively) and that the peeling performance is comparable to the blanching and lye peeling.
The resulting peeling losses are reported in Figure 3. Overall, peeling losses for PEF peeled tomatoes varied from 7.1% to 8.3%, whereas it was 9.8% and 10.3% for blanched and lye peeled, respectively. Other authors already reported peeling loss for lye peeled tomatoes between 10% and 20% (Pan et al., 2009; Vidyarthi et al., 2019a). Compared to untreated tomatoes, PEF reduced peeling loss from 33% to 43%, while the peeling loss was reduced by only 22% and 18% with blanching and lye peeling, respectively. It can be noted that increasing the energy input shows a statistical increase in the peeling losses. However, even applying the most intense PEF treatment (5.0 kJ/kg), the peeling losses are statistically lower than the ones obtained with blanching and lye peeling. The peeling losses for PEF peeled kiwi fruits varied from 7.6% to 10.9%, whereas 10.7% and 13.1% for blanched and lye peeled samples, respectively. The values are consistent with the ones previously reported by Gómez-López et al. (2014) for kiwi fruits immersed in hot water for 30 s and in NaOH (15%) solution for 4 min and slightly higher than the ones reported by Mohammadi et al. (2019) for lye peeling (12% NaOH, up to 4.5 min). As reported for the tomatoes, compared to the untreated kiwi fruits, the PEF treated samples had higher peeling losses reduction: up to 66% reduction for the PEF treated samples and 53% and 42% for blanched and lye peeled samples, respectively.
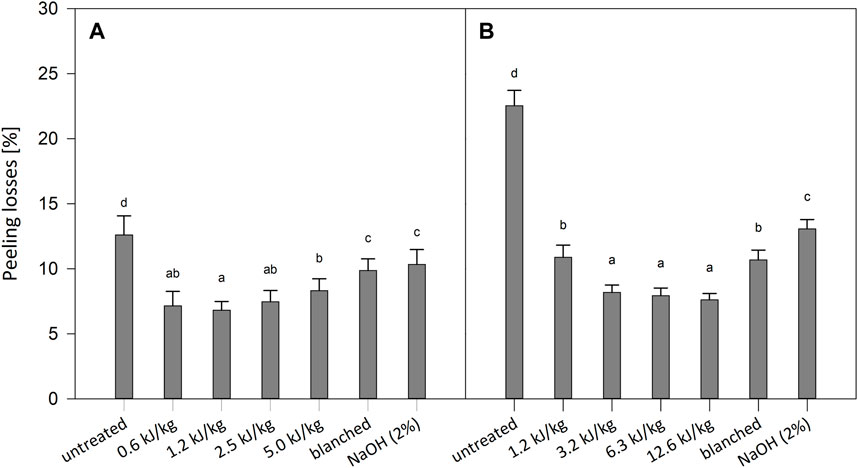
FIGURE 3. Peeling loss for untreated, PEF treated, blanched and lye peeled tomatoes (A) and kiwi fruits (B). Significant differences are indicated by different letters (p < 0.05).
3.3 Peeled products physical quality
Textural integrity and colour of the peeled vegetables are considered good indicators for evaluating product quality. As shown in Figure 4, the mechanical properties of tomatoes and kiwi fruits were significantly affected, showing a general reduction in the hardness. All the applied pre-treatments had softened effects compared to the untreated samples for both investigated materials. In particular, for the PEF treatments, increasing the energy input resulted in a statistical decrease in the pericarp hardness. Compared to the untreated tomatoes, the PEF treated tomatoes showed a hardness reduction between 28% and 67%. Previously, Andreou et al. (2020) reported a similar softening effect on PEF peeled tomatoes (1.5 kV/cm, 500 pulses). The PEF-induced softening shown by the mechanical property changes is likely caused by the loss of turgor of the treated fruit pericarps (Lebovka et al., 2007; Grimi et al., 2009). The peeling by blanching and lye agents caused a hardness reduction of 53% and 71%, respectively. It might be explained by the high temperature inducing the thermal degradation of cell wall components and the loss of turgor in the cells (Wang et al., 2018). In addition, the degradation reaction caused within the cell wall matrix by the lye agent might have further reduced the pericarp hardness (Garcia and Barrett, 2006b). In the case of the kiwi fruits, the hardness of the PEF samples was statistically lower than that of the untreated samples and tent to increase with higher energy inputs. Specifically, the hardness was reduced from 30% to 80%, compared to the untreated sample. The peeling by blanching and lye agents caused a statistical hardness reduction of 60% and 62%, respectively. The firmness of kiwi fruits’ pericarp tends to decrease with tissue temperature increase, and it is generally affected even after only 1 min of heating (Llano et al., 2003). In a previous study, Mohammadi et al. (2019) reported the same softening effect of the lye peeling on kiwi fruits.
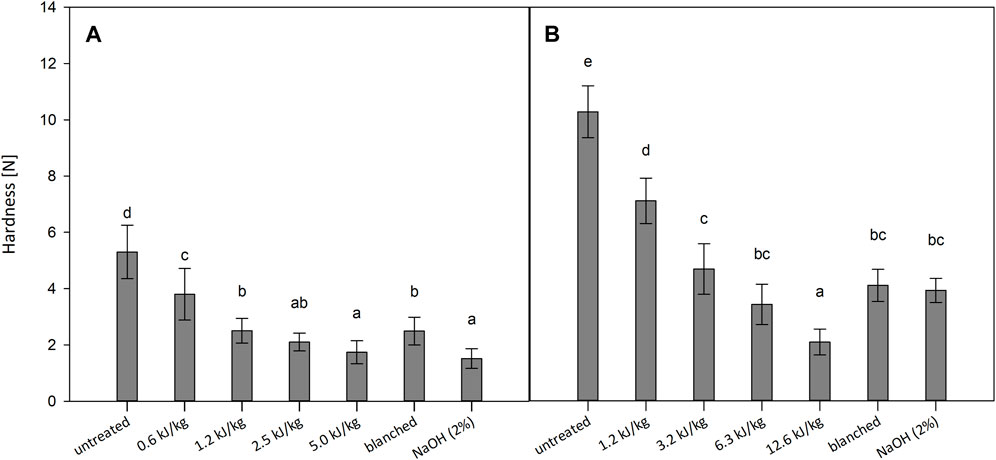
FIGURE 4. Hardness values for untreated, PEF treated, blanched and lye peeled tomatoes (A) and kiwi fruits (B). Significant differences are indicated by different letters (p < 0.05).
The optical properties of untreated, PEF treated, blanched, and lye peeled tomatoes and kiwi fruits are reported in Table 2. In particular, in terms of colour, the L*, a*, and b* values of the tomatoes peeled by blanching and lye peeling were all significantly different than those of the untreated and PEF treated samples. Compared with untreated and PEF treated peeled samples, lye peeled samples had a higher yellowness (b*) value and lower redness (a*) value, suggesting that PEF peeling was better at preserving the natural red colour of the pericarp. Moreover, the hue° values of blanching and lye peeled tomatoes were 25% higher than the hue° values of untreated, and PEF treated tomatoes. The treatment of tomatoes with heat and lye agents commonly resulted in more severe damage to the red layer and consequent higher hue values (Garcia and Barrett, 2006b; Gao et al., 2018). The hue value of the blanched and lye peeled tomatoes is consistent with the ones reported by Urbonaviciene et al. (2012) and Gao et al. (2018). These additional adverse effects from heating are further supported by the ΔE values that are statistically higher for blanched and lye peeled samples, confirming that the colour difference could be easily perceived just by visual evaluation (Adekunte et al., 2010). Applying PEF did not affect any of the investigated optical parameters concerning the peeled kiwi fruits. On the contrary, in the processes involving the heat application, an increase in the a* values and a drop in the b* values were recorded. This variation represents a slight change from green to yellow, only observed in the blanched and lye peeled kiwi fruits. This colour evolution was previously reported by Guldas (2003) and Mohammadi et al. (2019) for kiwi fruits lye peeled at 95°C for 4 min. As for the tomatoes, the colour change (ΔE) showed high values for blanching and lye peeling treatments while lower than 1 for PEF treated kiwi fruits. Therefore, PEF resulted in no visually perceivable colour changes.
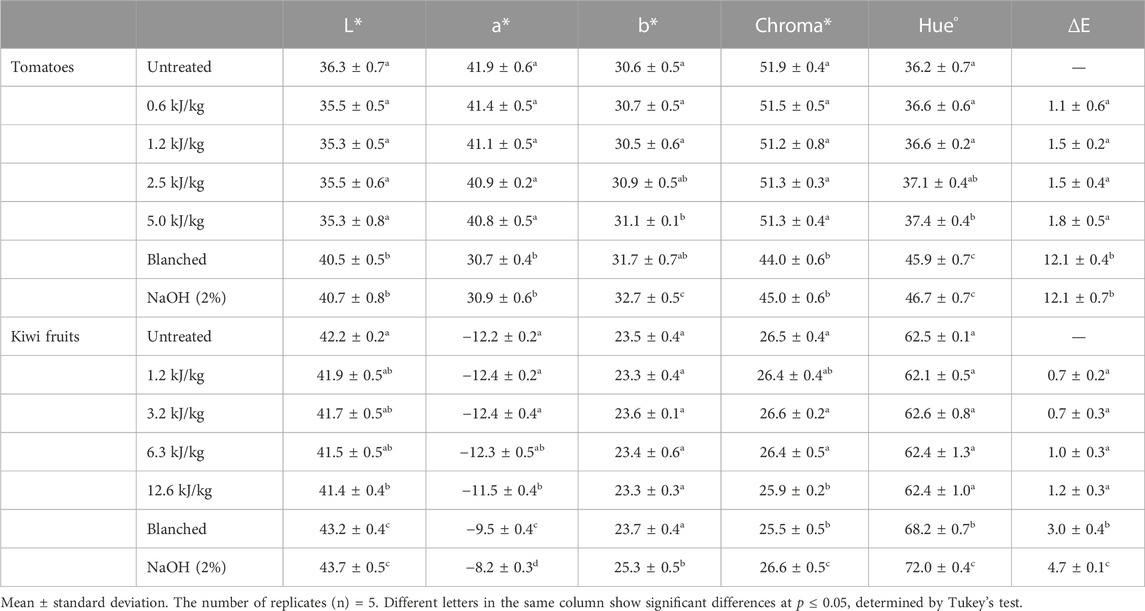
TABLE 2. L*, a*, b*, Chroma*, Hue° and colour difference (ΔE) values for untreated, PEF treated (at different energy inputs, kJ/kg), blanched and lye peeled tomatoes and kiwi fruits. Different letters in the same column of each raw material indicate significant differences (p < 0.05).
3.4 Chemical quality of peeled products
The investigated parameters to determine the chemical quality of the peeled product are reported in Table 3. Untreated tomatoes exhibited an initial total phenolic content (TPC) of 203 ± 32 μg/g FW. This value is within the range Valdivia-Nájar et al. (2018) observed in the same tomato cultivar (231.26 μg/g). All the PEF treated tomatoes did not exhibit significantly lower phenolic contents than untreated samples. A significant decrease in TPC was observed in both tomato samples subjected to blanching and lye peeling (30% and 33%, respectively). The initial TPC of kiwi fruits was 861 ± 67 μg/g FW, as already reported by Latocha et al. (2013). Regarding the different peeling methods, as for the tomatoes, the TPC of kiwi fruits was significantly lower only in the blanched and lye peeled samples (7% and 8%, respectively). The same trends were found in the case of the AOA in both the performed assays and in both investigated materials. The blanched and lye peeled samples were the only samples with significantly reduced AOA. In particular, compared to the untreated samples, the blanched tomatoes had a 33% (ABTS) and 10% (FRAP) reduction of the AOA, and the lye peeled tomatoes a reduction of 55% (ABTS) and 20% (FRAP) of the AOA. While the blanched kiwi fruits showed a reduction of 33% (ABTS) and 33% (FRAP) of the AOA, and the lye peeled kiwi fruit showed a reduction of 47% (ABTS) and 29% (FRAP) of the AOA. The results obtained in this study are in agreement with those published by some authors who reported a decrease in the phenolic contents and antioxidant activity after the application of heat treatments on fruit and vegetables due to oxidation and leaching phenomena (Larrauri et al., 1997; Nicoli et al., 1999; Patras et al., 2009).
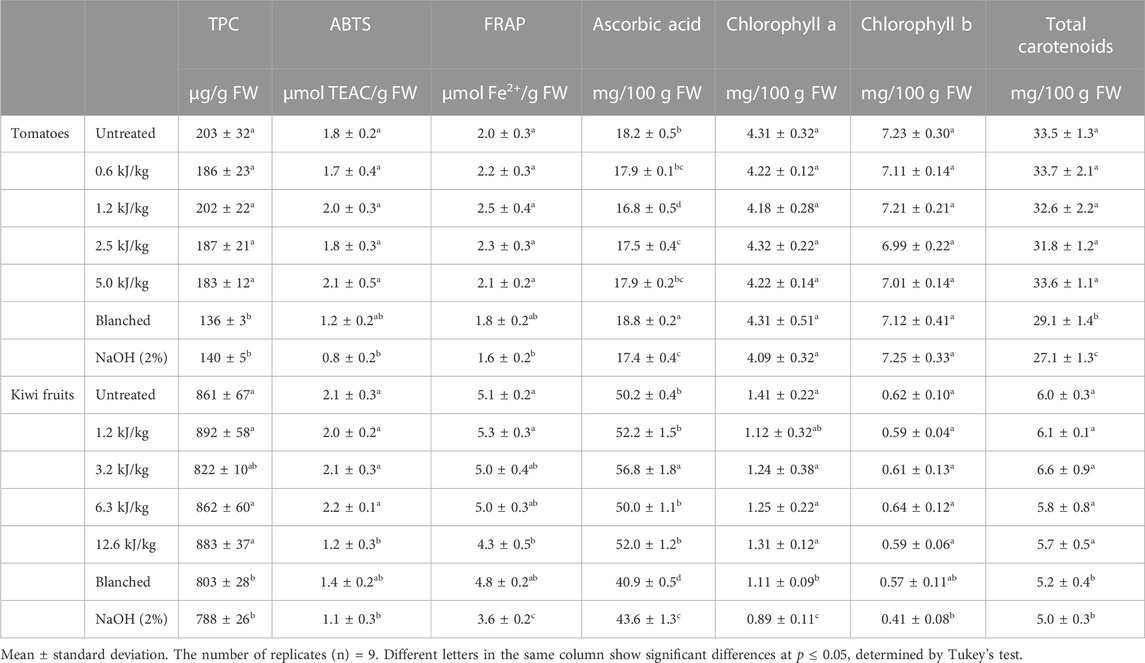
TABLE 3. Total phenolic content (TPC), antioxidant activity as ABTS and FRAP, ascorbic acid content, chlorophylls a and b and total carotenoids content for untreated, PEF treated (at different energy inputs, kJ/kg), blanched and lye peeled tomatoes and kiwi fruits. Different letters in the same column of each raw material indicate significant differences (p < 0.05).
The initial ascorbic acid content in untreated tomatoes was 18.2 mg/100 g FW, slightly higher than the values reported by Valdivia-Nájar et al. (2018) for the same variety but consistent with the values previously reported for other tomato varieties (Ilahy et al., 2019). The ascorbic acid content in all the treated tomato samples tends to decrease slightly, except for the sample subject to blanching. A similar effect has been reported for the same or different raw materials subjected to PEF and thermal treatments (Elez-Martinez and Martin-Belloso, 2007; Oms-Oliu et al., 2009; Jafari et al., 2017). The initial ascorbic acid content in untreated kiwi fruits was 50.2 mg/100 g FW, which is consistent with values previously reported in the literature for the same kiwi variety (Guldas, 2003; Tavarini et al., 2008). In contrast to the tomato samples, the ascorbic acid content in kiwi fruits was significantly reduced only in the samples subjected to blanching and lye peeling.
The chlorophyll a, b and total carotenoid content for the investigated tomatoes were 4.31 ± 0.32, 7.23 ± 0.30 and 33.5 ± 1.3 mg/100 g FW, respectively. These values are consistent with those Costache et al. (2012) reported for acetone extracts from different tomato varieties. Compared to the untreated sample, the PEF treated tomatoes showed no significant difference for chlorophylls and carotenoids. In contrast, blanching and lye peeling caused a significant reduction of the total carotenoid content (13% and 19%, respectively) without affecting the chlorophyll content. The lower carotenoid content recorded in the blanched and lye peeled tomatoes confirmed the values reported for the colour, and it can be attributable to the loss of the red layer of the tomatoes during peeling (Gao et al., 2018). The chlorophyll a, b and total carotenoid content for the investigated untreated kiwi fruits were 1.41 ± 0.22, 0.62 ± 0.10 and 6.0 ± 0.3 mg/100 g FW, respectively. These values are consistent with the values reported by Nishiyama et al. (2005) for the same kiwi fruit variety. Compared to the untreated kiwi fruits, the PEF treated samples showed no significant difference for chlorophylls and carotenoids. On the contrary, the blanched and lye peeled kiwi showed a significant reduction in chlorophyll a (21% and 37%), chlorophyll b (8% and 33%) and total carotenoids (13% and 17%), as already reported by Guldas and Bayindirli (2004).
4 Discussion
This research has demonstrated that using pulsed electric fields (PEF) can result in comparable or even superior performance to traditional methods such as hot water blanching and lye peeling. Applying PEF can minimise adverse effects such as product loss and excessive softening of peeled fruits without using thermal or chemical agents. Moreover, reducing the softening effects could streamline the canning process and prevent the peeled products from breaking. Additionally, PEF can help preserve the natural colour and bioactive compounds found in the investigated fruits by reducing the adverse effects of thermal treatments. These results confirmed what has already been reported on the improvement of the peeling ability in tomatoes (Pataro et al., 2018; Andreou et al., 2020; Koch et al., 2022) and, in addition, showed that there is no adverse effect on the chemical quality of PEF peeled fruits. The study findings suggest that improving peeling ability is not proportionally linked to cellular disintegration. Specifically, as the degree of cellular disintegration increased (>0.5), there was no significant change in peeling ability. The mechanism behind the peeling ability improvement is still under discussion. Several authors suggested a correlation between the electroporation phenomenon, the release of water below the fruit peel and the consequent pressure difference across the skin (Pataro et al., 2018; Andreou et al., 2020). However, this cannot fully explain the phenomenon since it is not valid for many other fruits and vegetables, as already demonstrated by Koch et al. (2022). Previous studies have explored the relationships between peeling and pericarp softening phenomena. For instance, in understanding the peeling ability of kiwi fruits, Harker et al. (2011) reported that the peel-pericarp adhesion is not essential as long as the pericarp has softened enough to form a discontinuity at the peel-pericarp interface. It can also explain why a more advanced degree of ripening facilitates peeling. In fact, during the storage, a decrease in branching and the concomitant higher solubility is described only for pectin from some specific fruits like tomatoes and kiwi, with consequent softening (Ranganathan et al., 2016). Therefore, it is plausible to hypothesise that PEF may influence the rigidity of the cell wall and induce a disruption at the peel-pericarp interface exclusively in certain plant materials, resulting in improved peeling efficiency. Although the mechanism is still unclear, this study confirmed that PEF could improve the detachment of the external layer of tomato and kiwi peel, potentially facilitating its splitting and removal by subsequent industrial peel eliminators (i.e., core scrubber and pinch rollers). This information is crucial for a better understanding the PEF-assisted peeling process and provides guidance for further scale-up towards industrial application.
5 Conclusion
Novel strategies need to be implemented in the food industry to reduce product losses, improve the final product quality and minimise the environmental impact and the energy consumption connected with conventional peeling methods. The findings in this study are a significant step towards an industrial application of PEF assisted peeling. Fundamental information on the effects of the applied PEF conditions on the peeling performance and product quality has been reported. In particular, PEF treatments with low energy inputs (1.2 kJ/kg for tomatoes and 3.2 kJ/kg for kiwi fruits) showed peeling performances comparable with the conventional peeling methods of blanching and lye peeling. Moreover, the PEF treatment reduced the weight losses and the negative impact of thermal peeling on colour and bioactive compounds. Nowadays, the mechanism of peeling improvement is still not fully understood. However, ongoing analysis and investigation on a cellular level are focusing on explaining the mechanism behind the peeling ability improvement determined by the PEF treatment application on tomatoes and kiwi fruits. Comparative studies on the industrial scale should be performed to confirm the results of the present work and to evaluate the advantages of PEF peeling against the conventional peeling processes from an energetic and environmental point of view.
Data availability statement
The original contributions presented in the study are included in the article/supplementary material, further inquiries can be directed to the corresponding author.
Author contributions
MG: Conceptualization, Methodology, Investigation, Data curation, Formal analysis, and Writing—original draft. HJ: Writing—review and editing, Supervision. All authors contributed to the article and approved the submitted version.
Funding
This work was created within a research project of the Austrian Competence Centre for Feed and Food Quality, Safety and Innovation (FFoQSI). The COMET-K1 competence centre FFoQSI is funded by the Austrian federal ministries BMK, BMDW and the Austrian provinces Lower Austria, Upper Austria and Vienna within the scope of COMET—Competence Centers for Excellent Technologies. The programme COMET is handled by the Austrian Research Promotion Agency FFG. The funder was not involved in the study design, collection, analysis, interpretation of data, the writing of this article, or the decision to submit it for publication.
Acknowledgments
Part of the equipment used in this study was provided by the EQ-BOKU VIBT GmbH and the BOKU Core Facility Food and Bio Processing.
Conflict of interest
Author MG was employed by FFoQSI GmbH—Austrian Competence Centre for Feed and Food Quality, Safety and Innovation.
The remaining author declares that the research was conducted in the absence of any commercial or financial relationships that could be construed as a potential conflict of interest.
Publisher’s note
All claims expressed in this article are solely those of the authors and do not necessarily represent those of their affiliated organizations, or those of the publisher, the editors and the reviewers. Any product that may be evaluated in this article, or claim that may be made by its manufacturer, is not guaranteed or endorsed by the publisher.
References
Adekunte, A., Tiwari, B., Cullen, P., Scannell, A., and O'donnell, C. (2010). Effect of sonication on colour, ascorbic acid and yeast inactivation in tomato juice. Food Chem. 122 (3), 500–507. doi:10.1016/j.foodchem.2010.01.026
Andreou, V., Dimopoulos, G., Dermesonlouoglou, E., and Taoukis, P. (2020). Application of pulsed electric fields to improve product yield and waste valorization in industrial tomato processing. J. Food Eng. 270, 109778. doi:10.1016/j.jfoodeng.2019.109778
Angersbach, A., Heinz, V., and Knorr, D. (2000). Effects of pulsed electric fields on cell membranes in real food systems. Innovative Food Sci. Emerg. Technol. 1 (2), 135–149. doi:10.1016/S1466-8564(00)00010-2
Arnal, Á. J., Royo, P., Pataro, G., Ferrari, G., Ferreira, V. J., López-Sabirón, A. M., et al. (2018). Implementation of PEF treatment at real-scale tomatoes processing considering LCA methodology as an innovation strategy in the agri-food sector. Sustainability 10 (4), 979. doi:10.3390/su10040979
Barba, F. J., Parniakov, O., Pereira, S. A., Wiktor, A., Grimi, N., Boussetta, N., et al. (2015). Current applications and new opportunities for the use of pulsed electric fields in food science and industry. Food Res. Int. 77, 773–798. doi:10.1016/j.foodres.2015.09.015
Benzie, I. F., and Strain, J. (1999). Ferric reducing/antioxidant power assay: Direct measure of total antioxidant activity of biological fluids and modified version for simultaneous measurement of total antioxidant power and ascorbic acid concentration. Methods Enzym. 299, 15–27. doi:10.1016/S0076-6879(99)99005-5
Buchmann, L., and Mathys, A. (2019). Perspective on pulsed electric field treatment in the bio-based industry. Front. Bioeng. Biotechnol. 7, 265. doi:10.3389/fbioe.2019.00265
Costache, M. A., Campeanu, G., and Neata, G. (2012). Studies concerning the extraction of chlorophyll and total carotenoids from vegetables. Romanian Biotechnol. Lett. 17 (5), 7702–7708.
Elez-Martinez, P., and Martin-Belloso, O. (2007). Effects of high intensity pulsed electric field processing conditions on vitamin C and antioxidant capacity of orange juice and gazpacho, a cold vegetable soup. Food Chem. 102 (1), 201–209. doi:10.1016/j.foodchem.2006.04.048
Fauster, T., Schlossnikl, D., Rath, F., Ostermeier, R., Teufel, F., Toepfl, S., et al. (2018). Impact of pulsed electric field (PEF) pre-treatment on process performance of industrial French fries production. J. Food Eng. 235, 16–22. doi:10.1016/j.jfoodeng.2018.04.023
Fauster, T., Giancaterino, M., Pittia, P., and Jaeger, H. (2020). Effect of pulsed electric field pre-treatment on shrinkage, rehydration capacity and texture of freeze-dried plant materials. LWT 121, 108937. doi:10.1016/j.lwt.2019.108937
Gao, R., Ye, F., Lu, Z., Wang, J., Shen, X. L., and Zhao, G. (2018). A novel two-step ultrasound post-assisted lye peeling regime for tomatoes: Reducing pollution while improving product yield and quality. Ultrason. Sonochem. 45, 267–278. doi:10.1016/j.ultsonch.2018.03.021
Garcia, E., and Barrett, D. M. (2006a). Evaluation of processing tomatoes from two consecutive growing seasons: Quality attributes, peelability and yield. J. food Process. Preserv. 30 (1), 20–36. doi:10.1111/j.1745-4549.2005.00044.x
Garcia, E., and Barrett, D. M. (2006b). Peelability and yield of processing tomatoes by steam or lye. J. food Process. Preserv. 30 (1), 3–14. doi:10.1111/j.1745-4549.2005.00042.x
Garofalo, P., D'Andrea, L., Tomaiuolo, M., Venezia, A., and Castrignanò, A. (2017). Environmental sustainability of agri-food supply chains in Italy: The case of the whole-peeled tomato production under life cycle assessment methodology. J. Food Eng. 200, 1–12. doi:10.1016/j.jfoodeng.2016.12.007
Gavahian, M., and Sastry, S. K. (2020). Ohmic-assisted peeling of fruits: Understanding the mechanisms involved, effective parameters, and prospective applications in the food industry. Trends Food Sci. Technol. 106, 345–354. doi:10.1016/j.tifs.2020.10.027
Gómez-López, M., García-Quiroga, M., Arbones-Maciñeira, E., Vázquez-Odériz, M. L., and Romero-Rodríguez, M. A. (2014). Comparison of different peeling systems for kiwifruit (A ctinidia deliciosa, cv H ayward). Int. J. food Sci. Technol. 49 (1), 107–113. doi:10.1111/ijfs.12281
Grimi, N., Lebovka, N., Vorobiev, E., and Vaxelaire, J. (2009). Compressing behavior and texture evaluation for potatoes pretreated by pulsed electric field. J. texture Stud. 40 (2), 208–224. doi:10.1111/j.1745-4603.2009.00177.x
Guldas, M., and Bayindirli, L. (2004). Mathematical analysis of caustic peeling of kiwifruits. Biotechnol. Biotechnol. Equip. 18 (2), 112–117. doi:10.1080/13102818.2004.10817096
Guldas, M. (2003). Peeling and the physical and chemical properties of kiwi fruit. J. food Process. Preserv. 27 (4), 271–284. doi:10.1111/j.1745-4549.2003.tb00517.x
Harker, F. R., Hallett, I. C., White, A., and Seal, A. G. (2011). Measurement of fruit peelability in the genus Actinidia. J. texture Stud. 42 (4), 237–246. doi:10.1111/j.1745-4603.2010.00270.x
Ilahy, R., Tlili, I., Siddiqui, M. W., Hdider, C., and Lenucci, M. S. (2019). Inside and beyond color: Comparative overview of functional quality of tomato and watermelon fruits. Front. plant Sci. 10, 769. doi:10.3389/fpls.2019.00769
Jafari, S., Jabari, S., Dehnad, D., and Shahidi, S. (2017). Effects of thermal processing by nanofluids on vitamin C, total phenolics and total soluble solids of tomato juice. J. food Sci. Technol. 54 (3), 679–686. doi:10.1007/s13197-017-2505-z
Knorr, D., and Angersbach, A. (1998). Impact of high-intensity electric field pulses on plant membrane permeabilization. Trends Food Sci. Technol. 9 (5), 185–191. doi:10.1016/S0924-2244(98)00040-5
Knorr, D., Froehling, A., Jaeger, H., Reineke, K., Schlueter, O., and Schoessler, K. (2011). Emerging technologies in food processing. Annu. Rev. food Sci. Technol. 2, 203–235. doi:10.1146/annurev.food.102308.124129
Koch, Y., Witt, J., Lammerskitten, A., Siemer, C., and Toepfl, S. (2022). The influence of Pulsed Electric Fields (PEF) on the peeling ability of different fruits and vegetables. J. Food Eng. 322, 110938. doi:10.1016/j.jfoodeng.2021.110938
Kohli, D., Champawat, P. S., Mudgal, V. D., Jain, S. K., and Tiwari, B. K. (2021). Advances in peeling techniques for fresh produce. J. Food Process Eng. 44 (10), e13826. doi:10.1111/jfpe.13826
Larrauri, J. A., Rupérez, P., and Saura-Calixto, F. (1997). Effect of drying temperature on the stability of polyphenols and antioxidant activity of red grape pomace peels. J. Agric. food Chem. 45 (4), 1390–1393. doi:10.1021/jf960282f
Latocha, P., Wołosiak, R., Worobiej, E., and Krupa, T. (2013). Clonal differences in antioxidant activity and bioactive constituents of hardy kiwifruit (Actinidia arguta) and its year-to-year variability. J. Sci. Food Agric. 93 (6), 1412–1419. doi:10.1002/jsfa.5909
Lebovka, N., Shynkaryk, M., and Vorobiev, E. (2007). Moderate electric field treatment of sugarbeet tissues. Biosyst. Eng. 96 (1), 47–56. doi:10.1016/j.biosystemseng.2006.09.005
Li, X., Pan, Z., Bingol, G., McHugh, T. H., and Atungulu, G. G. (2009). “Feasibility study of using infrared radiation heating as a sustainable tomato peeling method,” in 2009 reno, Nevada, june 21-june 24, 2009 (American Society of Agricultural and Biological Engineers). doi:10.13031/2013.27171
Li, X., Pan, Z., Atungulu, G. G., Wood, D., and McHugh, T. (2014a). Peeling mechanism of tomato under infrared heating: Peel loosening and cracking. J. Food Eng. 128, 79–87. doi:10.1016/j.jfoodeng.2013.12.020
Li, X., Pan, Z., Atungulu, G. G., Zheng, X., Wood, D., Delwiche, M., et al. (2014b). Peeling of tomatoes using novel infrared radiation heating technology. Innovative Food Sci. Emerg. Technol. 21, 123–130. doi:10.1016/j.ifset.2013.10.011
Li, X., Zhang, A., Atungulu, G. G., Delwiche, M., Milczarek, R., Wood, D., et al. (2014c). Effects of infrared radiation heating on peeling performance and quality attributes of clingstone peaches. LWT-Food Sci. Technol. 55 (1), 34–42. doi:10.1016/j.lwt.2013.08.020
Llano, K., Haedo, A., Gerschenson, L., and Rojas, A. (2003). Mechanical and biochemical response of kiwifruit tissue to steam blanching. Food Res. Int. 36 (8), 767–775. doi:10.1016/S0963-9969(03)00071-1
Martínez-Valverde, I., Periago, M. J., Provan, G., and Chesson, A. (2002). Phenolic compounds, lycopene and antioxidant activity in commercial varieties of tomato (Lycopersicum esculentum). J. Sci. Food Agric. 82 (3), 323–330. doi:10.1002/jsfa.1035
Mohammadi, Z., Kashaninejad, M., Ziaiifar, A. M., and Ghorbani, M. (2019). Peeling of kiwifruit using infrared heating technology: A feasibility and optimization study. Lwt 99, 128–137. doi:10.1016/j.lwt.2018.09.037
Munyaka, A. W., Oey, I., Van Loey, A., and Hendrickx, M. (2010). Application of thermal inactivation of enzymes during vitamin C analysis to study the influence of acidification, crushing and blanching on vitamin C stability in Broccoli (Brassica oleracea L var. italica). Food Chem. 120 (2), 591–598. doi:10.1016/j.foodchem.2009.10.029
Neri, L., Giancaterino, M., Rocchi, R., Tylewicz, U., Valbonetti, L., Faieta, M., et al. (2020). Pulsed electric fields (PEF) as hot air drying pre-treatment: Effect on quality and functional properties of saffron (Crocus sativus L.). Innovative Food Sci. Emerg. Technol. 67, 102592. doi:10.1016/j.ifset.2020.102592
Nicoli, M., Anese, M., and Parpinel, M. (1999). Influence of processing on the antioxidant properties of fruit and vegetables. Trends Food Sci. Technol. 10 (3), 94–100. doi:10.1016/S0924-2244(99)00023-0
Nishiyama, I., Fukuda, T., and Oota, T. (2005). Genotypic differences in chlorophyll, lutein, and β-carotene contents in the fruits of Actinidia species. J. Agric. Food Chem. 53 (16), 6403–6407. doi:10.1021/jf050785y
Oms-Oliu, G., Odriozola-Serrano, I., Soliva-Fortuny, R., and Martín-Belloso, O. (2009). Effects of high-intensity pulsed electric field processing conditions on lycopene, vitamin C and antioxidant capacity of watermelon juice. Food Chem. 115 (4), 1312–1319. doi:10.1016/j.foodchem.2009.01.049
Ostermeier, R., Giersemehl, P., Siemer, C., Töpfl, S., and Jäger, H. (2018). Influence of pulsed electric field (PEF) pre-treatment on the convective drying kinetics of onions. J. Food Eng. 237, 110–117. doi:10.1016/j.jfoodeng.2018.05.010
Pan, Z., Li, X., Bingol, G., McHugh, T., and Atungulu, G. (2009). Technical note: Development of infrared radiation heating method for sustainable tomato peeling. Appl. Eng. Agric. 25 (6), 935–941. doi:10.13031/2013.29227
Pataro, G., Carullo, D., Siddique, M. A. B., Falcone, M., Donsì, F., and Ferrari, G. (2018). Improved extractability of carotenoids from tomato peels as side benefits of PEF treatment of tomato fruit for more energy-efficient steam-assisted peeling. J. Food Eng. 233, 65–73. doi:10.1016/j.jfoodeng.2018.03.029
Patras, A., Brunton, N., Da Pieve, S., Butler, F., and Downey, G. (2009). Effect of thermal and high pressure processing on antioxidant activity and instrumental colour of tomato and carrot purées. Innovative food Sci. Emerg. Technol. 10 (1), 16–22. doi:10.1016/j.ifset.2008.09.008
Ranganathan, K., Subramanian, V., and Shanmugam, N. (2016). Effect of thermal and nonthermal processing on textural quality of plant tissues. Crit. Rev. food Sci. Nutr. 56 (16), 2665–2694. doi:10.1080/10408398.2014.908348
Raso, J., Frey, W., Ferrari, G., Pataro, G., Knorr, D., Teissie, J., et al. (2016). Recommendations guidelines on the key information to be reported in studies of application of PEF technology in food and biotechnological processes. Innovative Food Sci. Emerg. Technol. 37, 312–321. doi:10.1016/j.ifset.2016.08.003
Re, R., Pellegrini, N., Proteggente, A., Pannala, A., Yang, M., and Rice-Evans, C. (1999). Antioxidant activity applying an improved ABTS radical cation decolorization assay. Free Radic. Biol. Med. 26 (9-10), 1231–1237. doi:10.1016/S0891-5849(98)00315-3
Redgwell, R. J., Curti, D., and Gehin-Delval, C. (2008). Physicochemical properties of cell wall materials from apple, kiwifruit and tomato. Eur. Food Res. Technol. 227 (2), 607–618. doi:10.1007/s00217-007-0762-1
Rock, C., Yang, W., Goodrich-Schneider, R., and Feng, H. (2012). Conventional and alternative methods for tomato peeling. Food Eng. Rev. 4 (1), 1–15. doi:10.1007/s12393-011-9047-3
Seymour, G. B., Colquhoun, I. J., Dupont, M. S., Parsley, K. R., and Selvendran, R. R. (1990). Composition and structural features of cell wall polysaccharides from tomato fruits. Phytochemistry 29 (3), 725–731. doi:10.1016/0031-9422(90)80008-5
Singleton, V. L., and Rossi, J. A. (1965). Colorimetry of total phenolics with phosphomolybdic-phosphotungstic acid reagents. Am. J. Enology Vitic. 16 (3), 144–158.
Tavarini, S., Degl'Innocenti, E., Remorini, D., Massai, R., and Guidi, L. (2008). Antioxidant capacity, ascorbic acid, total phenols and carotenoids changes during harvest and after storage of Hayward kiwifruit. Food Chem. 107 (1), 282–288. doi:10.1016/j.foodchem.2007.08.015
Toepfl, S., Siemer, C., Saldaña-Navarro, G., and Heinz, V. (2014). “Overview of pulsed electric fields processing for food, Emerging technologies for food processing,” in Emerging technologies for food processing, 93–114. doi:10.1016/B978-0-12-411479-1.00006-1
Tylewicz, U., Tappi, S., Genovese, J., Mozzon, M., and Rocculi, P. (2019). Metabolic response of organic strawberries and kiwifruit subjected to PEF assisted-osmotic dehydration. Innovative Food Sci. Emerg. Technol. 56, 102190. doi:10.1016/j.ifset.2019.102190
Urbonaviciene, D., Viskelis, P., Viskelis, J., Jankauskiene, J., and Bobinas, C. (2012). Lycopene and β-carotene in non-blanched and blanched tomatoes. J. Food Agric. Environ. 10, 142–146. doi:10.13140/2.1.4302.0800
Valdivia-Nájar, C. G., Martín-Belloso, O., and Soliva-Fortuny, R. (2018). Kinetics of the changes in the antioxidant potential of fresh-cut tomatoes as affected by pulsed light treatments and storage time. J. Food Eng. 237, 146–153. doi:10.1016/j.jfoodeng.2018.05.029
Vidyarthi, S. K., El-Mashad, H. M., Khir, R., Zhang, R., McHugh, T. H., and Pan, Z. (2019a). Tomato peeling performance under pilot scale catalytic infrared heating. J. Food Eng. 246, 224–231. doi:10.1016/j.jfoodeng.2018.11.002
Vidyarthi, S. K., El Mashad, H. M., Khir, R., Zhang, R., Tiwari, R., and Pan, Z. (2019b). Quasi-static mechanical properties of tomato peels produced from catalytic infrared and lye peeling. J. Food Eng. 254, 10–16. doi:10.1016/j.jfoodeng.2019.03.001
Wang, D., Yeats, T. H., Uluisik, S., Rose, J. K., and Seymour, G. B. (2018). Fruit softening: Revisiting the role of pectin. Trends plant Sci. 23 (4), 302–310. doi:10.1016/j.tplants.2018.01.006
Wang, L., Boussetta, N., Lebovka, N., and Vorobiev, E. (2020). Cell disintegration of apple peels induced by pulsed electric field and efficiency of bio-compound extraction. Food Bioprod. Process. 122, 13–21. doi:10.1016/j.fbp.2020.03.004
Keywords: pulsed electric fields, peeling improvement, tomato peeling, kiwi fruits peeling, alternative peeling method
Citation: Giancaterino M and Jaeger H (2023) Impact of pulsed electric fields (PEF) treatment on the peeling ability of tomatoes and kiwi fruits. Front. Food. Sci. Technol. 3:1152111. doi: 10.3389/frfst.2023.1152111
Received: 27 January 2023; Accepted: 30 March 2023;
Published: 12 April 2023.
Edited by:
Samo Mahnič-Kalamiza, University of Ljubljana, SloveniaReviewed by:
Naveen Kumar Mahanti, Dr. Y. S. R. Horticultural University, IndiaGulsun Akdemir Evrendilek, Abant Izzet Baysal University, Türkiye
Gianpiero Pataro, University of Salerno, Italy
Copyright © 2023 Giancaterino and Jaeger. This is an open-access article distributed under the terms of the Creative Commons Attribution License (CC BY). The use, distribution or reproduction in other forums is permitted, provided the original author(s) and the copyright owner(s) are credited and that the original publication in this journal is cited, in accordance with accepted academic practice. No use, distribution or reproduction is permitted which does not comply with these terms.
*Correspondence: Marianna Giancaterino, bWFyaWFubmEuZ2lhbmNhdGVyaW5vQGJva3UuYWMuYXQ=