- 1Department of Food Science and Microbiology, Faculty of Health and Environment Sciences, Auckland University of Technology, Auckland, New Zealand
- 2Faculty of Health and Environmental Sciences, Auckland University of Technology, Auckland, New Zealand
Avocado by-products present a waste issue for cold-pressed avocado oil processors in New Zealand. The avocado seed contains many extractable compounds that are beneficial to health. This work aims to evaluate the effects of roasting fermented avocado seed to produce a food ingredient with beneficial antioxidant and anticancer properties. Avocado seeds were subjected to natural fermentation and inoculated fermentation with either Lactobacillus plantarum or kefir. The fermented samples were evaluated in terms of total plate count values of lactic acid bacteria acetic acid bacteria and yeasts. The number of microorganisms increased significantly (p < 0.001) over the 7 days of fermentation for all samples. Fermentation with L. plantarum resulted in significantly (p < 0.05) higher total phenolic content (TPC) and antioxidant activities compared to kefir and naturally fermented samples. The fermented avocado seeds were further subjected to roasting to yield a shelf-stable dried powder. Roasted samples fermented by L. plantarum had significantly (p < 0.001) higher total polyphenolic content and antioxidant capacity (CUPRAC and Ferric Reducing Antioxidant Power assays) compared to kefir and naturally fermented samples. The avocado seed powder showed the best inhibition effect on Hep G2 followed by the MDA-MB-231 and MCF-7 cancer cell lines. The roasting conditions for optimal antioxidant and anticancer activities were determined to be at 127°C for 24.7 min. This study demonstrated that fermentation of avocado seeds in combination with roasting yielded a powder with good antioxidant and anticancer activities, which can potentially be incorporated into food for added health benefits.
1 Highlights
• Fermentation with Lactobacillus plantarum resulted in high total phenolic content (TPC) and antioxidant activities.
• Roasted and fermented avocado seed powder exhibited anticancer effects on Hep G2 MDA-MB-231 and MCF-7 cell lines.
• An increase in roasting time increased the antioxidant activity of fermented avocado seed.
• Increased roasting temperature and time-reduced anticancer activities.
2 Introduction
Avocado is a highly nutritious fruit with beneficial health effects. In New Zealand, the production of cold-pressed avocado oil yields 78 kg of oil, 448 kg of wastewater, 274 kg of skin and seeds, 150 kg of pomace, and 50 kg residual water for every 1000 kg of avocado fruit processed (Permal, Leong Chang et al., 2020). The avocado seed makes up between 13 and 18% of the whole fruit weight (Padilla-Camberos et al., 2013; Ejiofor et al., 2018; O'Brien 2018). Zhang (2017) reported that New Zealand ‘Hass’ avocado seeds comprised 49.09% moisture, 2.42% fat, 3.47% protein and 45.02% carbohydrate. The seeds were also reported to have high antioxidant activities (Rodríguez-Carpena et al., 2011; Permal, Leong Chang et al., 2020). This demonstrated the potential of using avocado seed as a food ingredient with a natural source of antioxidants.
Fermentation with microorganisms like lactic acid bacteria (LAB), acetic acid bacteria, and fungi can produce bioactive compounds in foods (Kachouri et al., 2015; Palani et al., 2016). Lactic acid fermentation has been widely used in food processing to improve the shelf life, bioactivity, and functional properties of food. Fermentation of seeds can modify phenolic compounds that can further enhance bioactivity in seeds due to the liberation of enzymes during cell wall break down that subsequently release bound phenolic compounds (Wang et al., 2019). Kuligowski, Pawłowska et al. (2017) reported the highest concentration of polyphenols in soybean fermented for 4 days using the Rhizopus oligosporus strain. Đorđević, Šiler-Marinković et al. (2010) found that fermentation of buckwheat, wheat germ, barley, and rye with Lactobacillus rhamnosus resulted in higher antioxidant activities than samples fermented with Saccharomyces cerevisiae. The higher antioxidant activity of LAB-fermented samples than natural- and yeast-fermented samples was attributed to the release of bound antioxidants due to plant cell wall breakdown (Gan et al., 2017). In addition, plant-based LAB are reported to have antioxidant activities. Hubert, Berger et al. (2008) found that soy germ extracts fermented by LAB exhibited a higher inhibition effect against the superoxide anion radical, and lesser but significant ferric-reducing and DPPH radical scavenging effects compared with raw soy germ. Fernandez-Orozco, Frias et al. (2007) demonstrated that soybean fermentation by Lactobacillus plantarum resulted in higher antioxidant capacity than natural fermentation. Similarly, Torino, Limón et al. (2013) showed that the liquid state fermentation of lentils using L. plantarum resulted in higher antioxidant activity than natural fermentation. The metabolism of phenolic compounds during plant fermentation has a physiological significance for LAB, and their metabolic end-products may benefit human health (Filannino et al., 2018).
Roasting can influence the physicochemical properties of seeds and beans. Spada, Zerbeto et al. (2017) reported that roasting time and temperature were important factors that influenced the total phenolic content (TPC) and antioxidant activities of jackfruit seeds. Açar, Gökmen et al. (2009) found that the antioxidant activities of hazelnut, peanut and sunflower seeds decreased when roasting time was less than 30 min, and significantly increased thereafter reaching a maximum value at 60 min. The initial decrease was attributed to the reduction of antioxidant compounds, while the increase with longer roasting time was due to the generation of antioxidant products during the Maillard reaction, especially in starch-rich materials. Roasted fermented coffee beans contained significantly higher levels of chlorogenic acid and total acids after fermentation (Bressani et al., 2018). Jan, Ahmad et al. (2019) further stated that the TPC and reducing power of kalonji seeds significantly improved with pan and microwave roasting. Similarly, the TPC and antioxidant activities of almond seeds significantly increased after roasting (Lin et al., 2016). Interestingly, Zieliński, et al. (2019) further showed that roasting significantly increased the antioxidant capacity of buckwheat fermented with L. plantarum W42 using the FRAP assay.
The avocado seed is a valuable source of potentially health-promoting bioactive compounds that possess anticancer activities. Lee et al. (2008) reported treatment of MDA-MB-231 human breast cancer cells with a methanolic extract of avocado seed led to the induction of apoptosis. Lara-Marquez, Baez-Magana et al. (2020) further found that the lipid extract from Mexican avocado seed was cytotoxic towards Caco-2 cells. The extract had an IC50 of 28 µg/ml and induced apoptosis through the activation of caspases 8 and 9. In another study, a chloroform extract of dried avocado seed powder demonstrated strong cytotoxic activity against the MCF-7 cell line, with an IC50 value of 94.87 (Widiyastuti et al., 2018). Kristanty, Suriawati et al. (2014) further found that the aqueous and ethanolic extract of avocado seeds inhibited the T47D breast cancer cell line with IC50 values of 560.2 μg/ml and 107.2 μg/ml, respectively. Further partitioning of the chloroform extract with methanol yielded soluble and non-soluble methanol fractions that further increased cytotoxic activity against MCF-7 cell lines, with IC50 values of 34.52 and 66.03 μg/ml, respectively. The compounds that contributed to anticancer activities of avocado seeds are somewhat limited. Triterpenoids of avocado seed inhibited cell proliferation of MCF-7 and HepG2 cell lines, with IC50 values of 62 μg/ml and 12 μg/ml respectively but were safe to normal cells (Abubakar et al., 2017). Lee A. V et al. (2015) identified avocatin B, a mixture of avocadene and avocadyne from avocado pulps and seeds that showed cytotoxic activity in acute myeloid leukaemia (AML). At concentrations as high as 20 μmol/L, avocatin B reduced the viability of primary AML patient cells with an EC50 of 3.9 ± 2.5 μmol/L, and did not affect the viability of normal Peripheral blood stem cells (PBSCs). In addition, avocatin B (3 μmol/L) when added into the culture medium reduced the clonogenic growth of AML patient cells and did not affect normal cells.
Antioxidant activities of plant by-products have gained more attention since they benefit human health and can potentially be converted to health-promoting food ingredients. Avocado seeds contain bioactive compounds that possess anticancer activities. Studies have shown that fermentation and roasting can increase the antioxidant activities of seeds and nuts. However, the combined effects of fermentation and roasting on the physicochemical properties and bioactivities of avocado seeds are currently not well defined. Hence this study aimed to determine how roasting of avocado seeds fermented using Lactobacillus plantarum influenced antioxidant and anticancer activities.
3 Materials and methods
3.1 Sample treatments
3.1.1 Fermentation and microbial analysis
Avocado (Persea americana, Hass variety) seeds were sourced from Olivado Ltd. in Kerikeri, New Zealand. Prior to fermentation, the ground avocado seed particles were divided into three batches for natural fermentation and fermentation with either L. plantarum or kefir. Each fermentation batch contained approximately 900g of the ground samples. Three batches of ground avocado seed particles were subjected to fermentation in triplicates by i) 1% L. plantarum ii) 1% kefir and iii) natural fermentation. The avocado seeds were fermented by L. plantarum under anaerobic conditions at 37°C for 5 days. 1ml stock culture of L. plantarum (Fonterra Research Center, Palmerston North, New Zealand), with an approximate bacterial suspension of 9.0x108 cfu/ml (3.0 McFarland standard) was stored in 20% glycerol at −80°C. Kefir (1g) was obtained from Body Ecology TM (CA, United States). It was inoculated into 100 ml De Man, Rogosa and Sharpe (MRS) broth (Fort Richard company, New Zealand), and incubated at 37°C for 24 h. The inoculated broths were placed into BD BBL™ GasPak™ anaerobic jars, containing BD GasPak™ EZ Anaerobe Container System Sachets (Thermo Fisher, New Zealand), and incubated under anaerobic conditions at 35°C for 24 h. For L. plantarum, the purity of the stock culture was checked by the gram staining method. Then 100 ml MRS broth was inoculated with 1 ml of the pure stock culture and incubated under anaerobic conditions at 35°C for 24 h. After incubation, 1% of this broth was used to inoculate the avocado seeds particles. After inoculation, the fermented samples were incubated in a carbon dioxide incubator (Thermo Fisher Scientific, United States) (37 ± 2°C) for fermentation over 7 days. This created a relatively anaerobic condition for the growth of L. plantarum. kefir and naturally fermented samples.
In terms of microbial analysis, the fermented ground avocado seed particles were collected at 37°C on 0, 2, 5 and 7 days of fermentation with L. plantarum and kefir, as well as natural fermentation. The number of microflorae on the culture medium was evaluated by plate counting. Briefly, the fermented ground avocado seed particles (1g) were diluted aseptically with 9 ml of peptone water to prepare a series of seven dilutions (10–1, 10–2, 10–3, 10–4, 10–5, 10–6, and 10–7). After that, the diluted samples (0.1 ml) were spread on specific growth media, and plate counts were determined daily over 7 days of fermentation. In total, triplicate experiments were carried out for the three fermentation conditions. For each treatment carried out, duplicate determinations of plate counts were carried out.
For L. plantarum fermented samples, the Lactobacillus MRS agar (Fort Richard company, New Zealand) was used to determine microbiological growth. With the kefir and naturally fermented samples, Lactobacillus MRS agar, Acetobacter agar, and Malt Extract agar (Fort Richard Laboratories, New Zealand) were employed to determine microbiological growth. The microflora on Lactobacillus MRS agar was enumerated after anaerobic incubation under CO2 at 37°C for 7 days. The microflora on Acetobacter agar and Malt Exact agar were enumerated after aerobic incubation at 37°C for 7 days. The results of plate counts were expressed as log cfu/g.
3.1.2 Roasting using a central composite design
Fermented avocado seed particles were dried in a desiccator (Harvest Maid Deluxe FD1000) without cover at 60°C for 48 h to remove the moisture before roasting until they reached a consistent weight. After the drying process in the desiccator, the moisture content was 47.5 ± 0.4%. Drying was carried out to reduce microbial deterioration of samples before roasting. Drying prior to roasting has been carried out on benniseed (Yusuf et al., 2008), jackfruit seed (Spada et al., 2017), and rambutan seed (Chai et al., 2018). Dried avocado seed samples were kept in a desiccator until the roasting process began. The dried avocado samples were wrapped in aluminium foil and subjected to roasting using a conventional oven (Magellano Digital, PIRON, Italy) with different roasting times and temperatures. After roasting, the total moisture content was found to be 57.7 ± 0.3%.
A central composite design (CCD) was used to investigate the effects of roasting fermented seed particles using a two-factor five-point pattern (Spada et al., 2017). The two factors, roasting time (15–45 min) and roasting temperature (110–160°C), were tested at five points with four repetitions at the central point giving a total of 12 treatments (Supplementary Table S1). Each treatment was carried out in triplicates. Response surface methodology (RSM) was used to evaluate the effect of roasting conditions on fermented avocado seed particles, in terms of TPC, antioxidant and anticancer activities.
A RSM equation Y = f (X1, X2) for each variable, in terms of two independent factors X1 (roasting temperature) and X2 (roasting time), was assumed. The quadratic function was used to approximate the function, f:
where B0, B1, B2, B11, B22, and B12 are the corresponding regression coefficients and X1 and X2 are the two coded factors for roasting time (min) and temperature (°C), respectively.
After roasting, the avocado seed particles were cooled to 25°C and further ground into powder using a pestle and mortar. The powder was stored in food-grade plastic bags in a freezer (−17°C) until further chemical analysis.
3.1.3 Cell lines
All cell lines used in this study were purchased from the American Type Culture Collection (ATCC, United States). MCF-7, an acronym for Michigan Cancer Foundation-7, was a breast cancer cell line isolated in 1970 from a 69-year-old Caucasian woman (Lee E. A. et al., 2015). MDA-MB-231 was a human breast cancer cell line obtained from a pleural effusion of a 51-year-old Caucasian female with metastatic mammary adenocarcinoma (Relda et al., 1978). Hep G2 was a human liver cancer cell line derived from a liver hepatocellular carcinoma of a 15-year-old Caucasian male (Aden et al., 1979).
3.2 Antioxidant and anticancer analysis
3.2.1 Sample extraction
Firstly, 0.1 g of either the fermented or roasted ground avocado seed was weighed in a centrifuge tube and extracted with 4 ml of 50% methanol (Sigma-Aldrich, New Zealand). The sample was homogenized using a disperser (IKA, T 25 digital ULTRA-TURRAX®) at 12,000 rpm for 90s and left for 1 h. Then, the sample was centrifuged (Eppendorf, Centrifuge 5810R, South Pacific) at 1,500 rpm for 15 min at 4°C. Supernatant liquid from the top was transferred into a 10 ml volumetric flask. Secondly, the remaining residue was re-extracted using 4 ml of 70% acetone (Sigma-Aldrich, New Zealand) for 1 h, followed by centrifugation. The supernatant from the two separate methanol and acetone extractions was pooled and made up to a total volume of 10 ml using deionised distilled water. The mixture (1 ml) was then added to a 10 ml volumetric flask and diluted to the 10 ml mark using deionised water. The extract was then stored at −20°C until further analysis.
3.2.2 Determination of total phenolic content
The determination of TPC of fermented avocado seed or roasted fermented avocado seed powder was determined by the Folin-Ciocalteu assay (Romero-Cortes et al., 2013). A 1000 mg/L stock solution of gallic acid (Sigma-Aldrich, New Zealand) was prepared by dissolving gallic acid (0.1g) in deionised water and made up to volume in a 100 ml volumetric flask. Different concentrations of standard solutions were prepared by diluting the stock solution with deionised water (2.5, 5, 10, 20, 40, and 80 mg/L).
The standard solution or extract (1 ml) obtained in Section 3.2.1 was mixed with 0.5 ml Folin-Ciocalteu reagent (Sigma-Aldrich, New Zealand) in a glass vial and kept for 5 min at room temperature. Then 1.5 ml of 20% of Na2CO3 (Sigma-Aldrich, New Zealand) was added and the solution was incubated for 2 h in the dark. Absorbance was measured at 765 nm using an Ultrospec 2100 Pro spectrophotometer (Amersham Pharmacia Biotech, United Kingdom). The TPC of avocado seed extracts was expressed as mg gallic acid equivalents (GAE)/g of sample extract weight. All the determinations were performed in triplicates.
3.2.3 Cupric reducing antioxidant capacity assay
The CUPRAC assay was employed to determine the antioxidant activity of sample extracts as described by Ioannou et al. (2015). In this assay, 0.1 g ascorbic acid (Sigma-Aldrich, New Zealand) was dissolved in deionised water and diluted in a 100 ml volumetric flask to make a 1000 mg/L stock solution. All the different concentrations of standard solutions were prepared by diluting this stock solution with deionised water to obtain concentrations of 2.5, 5, 10, 20, 40, and 80 mg/L. The sample extract (1 ml) obtained in Section 3.2.1 or distilled water (for blanks) was mixed with 1 ml CuCl2 (0.01M) (Sigma-Aldrich, New Zealand), 1 ml NH4AC (1 M, pH7) (Sigma-Aldrich, New Zealand), 1 ml Neocuproine (0.075 M) (Sigma-Aldrich, New Zealand), and 0.1 ml deionised water to yield a total volume of 4.1 ml. The extracts were incubated for 5 min at room temperature, and the absorbance was measured at 450 nm using a Ultrospec 2100 Pro (Amersham Pharmacia Biotech, United Kingdom) spectrophotometer. The CUPRAC values of the avocado seed extracts were expressed as mg ascorbic acid equivalent/g of sample. All the determinations were performed in triplicates.
3.2.4 Ferric reducing antioxidant power assay
The FRAP assay used to determine the antioxidant capacity of sample extracts was carried out as described by Ioannou, Chaaban et al. (2015). Ascorbic acid standard solutions for the standard curve were prepared using the same method described for the CUPRAC assay. The FRAP reagent was prepared by mixing 10 ml 300 mM acetate buffer (Sigma-Aldrich, New Zealand), 1 ml 10 mM 2,4,6-Tris (2-pyridyl)-s-triazine (TPTZ) (Sigma-Aldrich, New Zealand), and 1 ml 20 mM FeCl3 (Sigma-Aldrich, New Zealand), and the mixture was heated in a 36°C water bath. The colour of this reagent should be orange-yellow in colour. After that, 2 ml of FRAP reagent, 0.1 ml of sample extract, and 0.9 ml deionised water were mixed in a cuvette (Interlab, New Zealand) and left for 4 min to react. Absorbance value of the solution was measured at 593 nm using a Ultrospec 2100 Pro (Amersham Pharmacia Biotech, United Kingdom) spectrophotometer. The FRAP value of avocado seed extracts was expressed as mg ascorbic acid equivalent/g of sample. All the determinations were performed in triplicates.
3.2.5 Cell viability and MTT assay
For this assay, the [3-(4,5-dimethylthiazol-2-yl) -2,5-diphenyltetrazolium bromide] MTT reagent (Sigma-Aldrich, New Zealand) was prepared before the experiment by dissolving the dye in phosphate-buffered saline (Sigma-Aldrich, New Zealand) at a concentration of 5 mg/ml. Cells were grown in a 96-well plate containing a growth medium that was made up of 90% Roswell Park Memorial Institute (RPMI 1640, no glutamine) media with 10% (v/v) FBS, 1.1% (v/v) penicillin-streptomycin and 1.1% l-glutamine mixed solution (Life Technologies, New Zealand). Each well contained 5 × 103 cells that were allowed to attach to the well for 18 h before treatment. Upon attachment, cells were treated with varying concentrations of avocado seed extract. The sample prepared in Section 3.2.1 and RPMI 1640 medium were used for serial double dilution to yield concentrations of 6.24, 3.12, 1.56, 0.78, 0.39, 0.195, 0.0975, 0.04875 and 0.024375 mg/ml, and further incubated at 37°C for 72 h. Cell viability following this treatment was measured using the MTT assay. Before adding the MTT solution, all the old medium containing the seed extracts was removed completely as the avocado seed extracts were coloured and will influence the plate readings. Then, 100 μl of a new complete medium was added to each well of the plate. The MTT reagent (10 μl) was added to each well, and the plate was incubated for 4 h at 37°C. Then 75 μl of growth media was subsequently removed and replaced with 100 μl of dimethyl sulfoxide (DMSO). The sample absorbance was measured at 540 nm using a microplate reader (Tecan Infinite M1000 Pro). Cell viability was calculated based on the following formula:
Percentage of inhibition (%) = [1-(Atc–Ab)/(Ac-Ab)] × 100%
Where Ac is the absorbance of the control, Ab is the absorbance with blank (no cells) and Atc is the absorbance of treated cells.
A plot of cell viability (%) versus concentration was constructed and the concentration of the seed extract that reduced cell viability by 50% (IC50) was determined. Preliminary analyses of cell viability were performed on all cells using a high concentration (μg/ml) of the seed extracts to screen them for antiproliferative activities.
3.3 Statistical analysis
For microbial analysis, the mean value of the total plate counts of LAB, yeast and AAB under the different fermentation treatments (L. plantarum, kefir and natural fermentation) over 7 days was determined. Two-way ANOVA was carried out using Minitab v. 17 Statistical Software (Minitab Inc., Coventry, United Kingdom). The two variables were fermentation days (0, 2, 5, and 7 days) and culture (L. plantarum, kefir and natural fermentation).
In terms of chemical analysis, two-way ANOVA was carried out on the TPC, CUPRAC and FRAP results of both unfermented (day 0) and fermented ground avocado seed samples using the Minitab v. 18 Statistical Software (Minitab Inc., Coventry, United Kingdom). The two independent variables examined were fermentation (with or without fermentation) and culture (L. plantarum, kefir and natural fermentation). Post-hoc comparisons were performed using the Tukey’s HSD test to evaluate whether the differences between means were significant (p < 0.05).
All analytical determinations of roasted avocado seed powders were carried out in triplicates. Response surface methodology (RSM) analysis was carried out on results for TPC, CUPRAC and FRAP results using the Minitab v. 18 Statistical Software (Minitab Inc., Coventry, United Kingdom). The generated two-way ANOVA table included regression coefficients of all linear, quadratic, and interaction terms for two variables (temperature and time) and one covariate (culture). The significance (p < 0.05) of all parameters (temperature, time, and culture) were determined. The main effect and interactions of two variables (temperature and time), and one covariate (culture) were examined. Main effect plots, interaction plots and contour plots were then generated. The response surface plots were also generated and these plots displayed the effects of roasted avocado seed powders on TPC, antioxidant and anticancer activities.
For cancer cytotoxicity results, the GraphPad Prism 8 (GraphPad Software, San Diego, United States) software was used to run non-linear regressions to transform the sample concentration into log10 dose. Then, the IC50 values were calculated.
3.3.1 Optimisation of roasting conditions
The optimisation of roasting conditions of fermented avocado seeds was carried out based on antioxidant and anticancer results obtained using the “Response Optimizer” function for response surface model analysis. The response optimizer function was used to identify the combination of roasting conditions that optimized the antioxidants and anticancer responses.
4 Results and discussion
4.1 Microbial analysis
Fermentation of avocado seed particles was carried out by either natural fermentation (without inoculation) or by inoculation with either L. plantarum or kefir. The fermented samples were evaluated in terms of visual plate count values of lactic acid bacteria (LAB), acetic acid bacteria (AAB), and yeasts. The number of LAB, AAB, and yeasts in avocado seeds significantly (p < 0.05) increased over 7 days of fermentation under all three fermentation conditions (L. plantarum, kefir and natural fermentation) in comparison to unfermented seed (day 0) samples (Table 1). Fleet and Zhao (2018) also reported that the numbers of LAB, AAB, and yeasts in spontaneously and inoculated fermented cocoa beans significantly increased after fermentation for 5 days compared to the unfermented (day 0) sample.
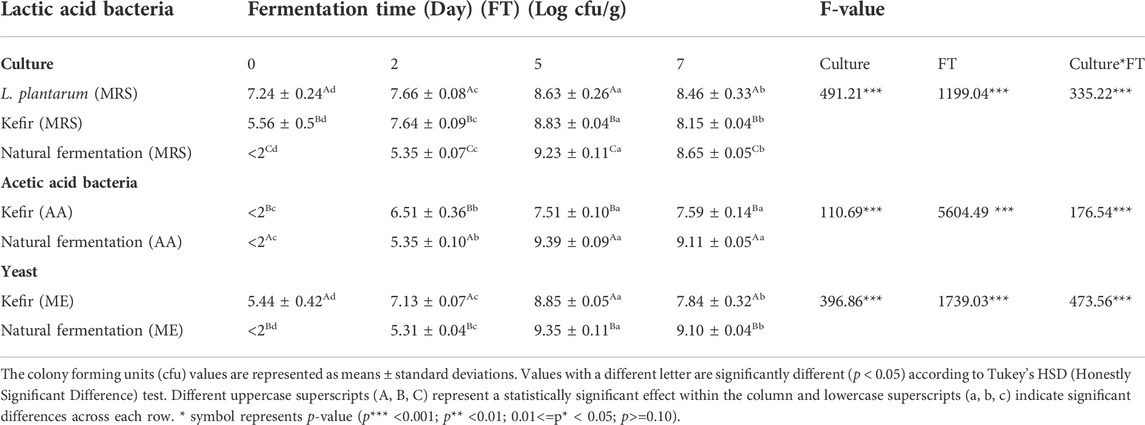
TABLE 1. The number of lactic acid bacteria cells in fermented avocado seeds over 7 days of fermentation.
The plate counts of LAB, AAB, and yeasts (Table 1) significantly (p < 0.001) increased in the first 5 days compared to the control. The differences were no more than 1 log cfu between the days 5 and 7 counts of LAB and yeasts, indicating that the growth had reached its stationary phase. Naturally fermented avocado seeds had significantly higher LAB, AAB and yeasts counts (Table 1) after 5 days of fermentation compared to inoculated fermentation with kefir and L. plantarum samples. This indicated that some microorganisms were naturally present in the avocado seeds and that the fermentation conditions supported their growth. Some mutual interactions occurring between these microorganisms may also account for their favourable growth (Papalexandratou et al., 2013).
4.2 Phenolic content and antioxidant capacity of fermented avocado seeds
Table 2 illustrated the changes in TPC and antioxidant capacities of fermented avocado seeds inoculated with L. plantarum and kefir, as well as samples that were naturally fermented. Results indicated that the TPC and antioxidant capacities of both inoculated (L. plantarum and kefir) and naturally fermented avocado seeds significantly (p < 0.001) decreased compared to the unfermented day 0 samples. Similarly, Gan et al. (2017) reported the reduction of TPC in some naturally fermented and LAB-fermented beans, compared to unfermented samples. This suggested that the metabolism of polyphenols occurred both in spontaneous and inoculated fermentations. Othman, Roblain et al. (2009) also found that spontaneously and inoculated fermented Chétoui olives resulted in a 32–58% reduction in TPC and a 50–72% reduction in antioxidant activity, compared to the unfermented sample. After fermentation, hydroxytyrosol and caffeic acid concentrations increased, while protocatechuic acid, ferulic acid and oleuropein concentrations decreased. After spontaneous and controlled fermentation, the hydroxytyrosol concentration in black olives increased from 165 mg/100 g dry weight to 312 and 380 mg/100 g dry weight, respectively. On the other hand, the oleuropein concentration in green olives decreased after spontaneous and controlled fermentation from 266 mg/100 g dry weight to 30.7 and 16.1 mg/100 g dry weight, respectively. During olive fermentation, the phenolic loss was attributed to the diffusion of these compounds into the brine. The main phenolic compound identified and quantified in the different brines was hydroxytyrosol.
4.2.1 Total phenolic content
Only the significant main factors and interactions are further discussed using the main effect and interaction plots based on the two-way ANOVA model that was used in this study (Supplementary Table S2). In terms of TPC, the linear models for roasting time and culture parameters were significant (p < 0.05). The main effect plots of TPC are shown in Supplementary Figure S1. TPC of roasted fermented avocado seeds increased with increasing roasting time (Supplementary Figure S1A). This was following results reported by the study of Lin et al. (2016) who reported that the TPC of almond kernels increased significantly with increasing roasting time from 5 to 20 min at 150°C, 180°C, and 200°C. The increase in TPC in roasted seeds may be due to thermal processing that can enhance the release of bound polyphenols into free forms that can lead to formation of higher antioxidant activity products (Lin et al., 2016; Jan et al., 2019). Similarly, TPC increased with increasing roasting temperature and time for roasted kalonji seeds (Jan et al., 2019). In this study, roasted avocado samples inoculated with L. plantarum had the highest TPC (Supplementary Figure S1B) compared to kefir and naturally fermented samples. This high TPC may be related to LAB-induced liberation of bound polyphenols by enzymes (e.g. tannase, glucosidase) or hydrolysis (Hur et al., 2014), which can increase TPC.
4.2.2 Antioxidant analysis
The linear culture and temperature–time interaction models were significant (p < 0.05) in the CUPRAC assay results (Table 3). In addition, the linear models for roasting temperature, roasting time, and culture, as well as the quadratic roasting temperature model were significant (p < 0.05) for the FRAP assay results. Supplementary Figures S2 and S3 showed the main effect plots for culture effects on CUPRAC and FRAP antioxidant activities respectively of roasted fermented avocado seeds. The antioxidant capacity of roasted avocado seeds fermented by L. plantarum was significantly the highest in comparison to kefir and naturally fermented samples. This may be due to the liberation of bound polyphenols into free radicals by enzymatic hydrolysis during fermentation, as well as the antioxidant potential of L. plantarum itself, which has antioxidative activity against superoxide dismutase (Kullisaar et al., 2002).
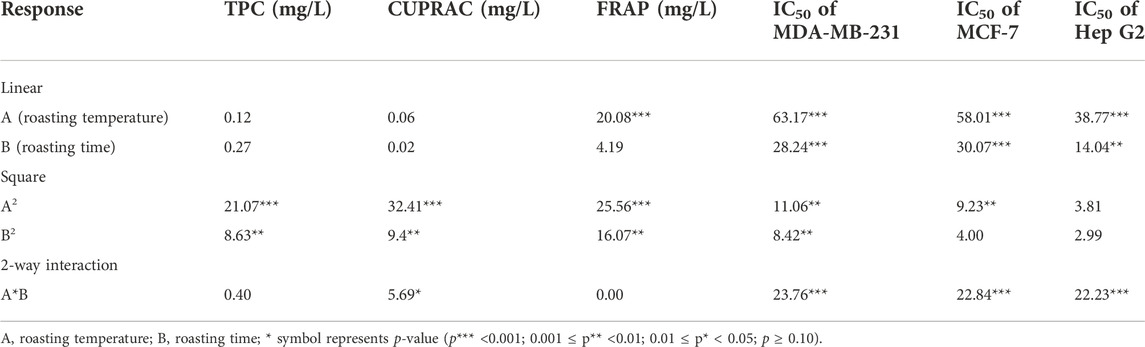
TABLE 3. The analysis of variance results of the response surface regression models in the roasting of avocado seeds fermented by Lactobacillus plantarum.
Differences existed in the results obtained for CUPRAC and FRAP assays of roasted fermented avocado seeds. This may be due to the different sensitivities of the assays for specific antioxidants (Bean et al., 2009). It was reported that the FRAP assay had higher sensitivity to ascorbic acid in rabbit tissues than the CUPRAC assay (Bean et al., 2009). Çelik et al. (2010) also found that the Trolox equivalent antioxidant capacity (TEAC) of different antioxidant compounds (quercetin, ferulic acid, and catechin) in green tea were significantly different using the CUPRAC assay, in comparison to the FRAP assay. The authors further explained that this may be due to the redox reaction that occurred at different pH values as the CUPRAC, FRAP and Folin-Ciocalteu assays were carried out at pH 7, pH 3.6, and pH 10 respectively. Bean, Radu et al. (2009) reported that a pH value less than 7 would contribute to the inhibition of reducing capacity. Furthermore, lipophilic antioxidants that are not detected in the FRAP assay can be detected by the CUPRAC assay, which can also account for the differences between these two assays (Çelik et al., 2010).
4.2.2.1 CUPRAC
The response surface contour plot for CUPRAC results is shown in Figure 1A. The highest CUPRAC assay values were obtained when fermented avocado seeds were roasted between 117 and 153°C for 17–43 min. This increase may be associated with the release of bound antioxidant components or the formation of higher antioxidant activity compounds (Lin et al., 2016). The increase in antioxidant capacity with longer roasting time was likely related to the formation of antioxidant Maillard reaction products, especially in starch-rich materials (Açar et al., 2009). Santos et al. (2016) reported that avocado seeds are rich in starch (42.2%).
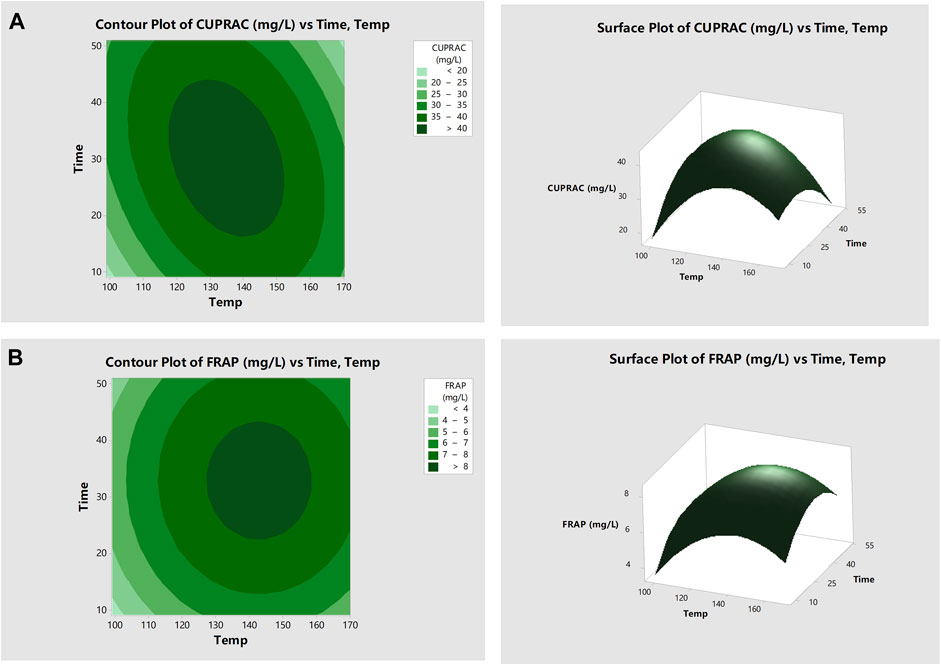
FIGURE 1. Response surface plots showing how roasting of fermented avocado seeds at varying time and temperature influenced antioxidant activities. Surface plots depict antioxidant activities determined using (A) Cupric-reducing antioxidant capacity (CUPRAC); and (B) Ferric-reducing antioxidant power (FRAP) assays.
4.2.2.2 FRAP
In Supplementary Figure S3A, FRAP assay results decreased with increasing roasting temperature up to 120°C and then increased with increasing roasting temperature, reaching a maximum at 170°C. Moreover, with increased roasting time, the FRAP assay values increased as well as seen in Supplementary Figure S3B. As shown in the response surface plots in Figure 1B, the highest FRAP assay values were obtained when roasting from 130 to 157°C for between 23 and 43 min approximately. Similarly, FRAP assay values decreased and then increased during the roasting of fermented cocoa beans (Ioannone et al., 2015). The initial decrease may be due to the reduction of antioxidant polyphenols, and the later increase may be related to the liberation of bound antioxidant polyphenols with higher roasting temperatures. Açar, Gökmen et al. (2009) further demonstrated that increased roasting time at 150°C increased total antioxidant capacity (TEAC) values significantly for most pulses (borlotti bean, black bean, giant lentils, chickpea, yellow soybean), cashew nut and pine nut that reached a maximum level at the end of 60 min of roasting. The authors suggested that the increase in TEAC with longer roasting time was likely due to the release of bound antioxidant components and generation of new antioxidants through Maillard reactions, especially in the starch-rich materials.
4.3 Anticancer properties
The effects of roasting time and temperature on IC50 values of three different cancer cell lines were determined. For the MDA-MB-231 cell line IC50 values, the linear effects of roasting temperature and time (Figure 2B), quadratic effects of roasting temperature and time, and the roasting temperature and time interaction were significant (p < 0.05) as seen in Table 3. In addition, for the IC50 values of the MCF-7 cell line, the linear effects of roasting temperature and time (Figure 2A), quadratic effects of roasting temperature, and the roasting temperature and time interaction were significant (p < 0.05) (Table 3). Meanwhile, for the IC50 value for the Hep G2 cell line, the effects of linear roasting temperature and time (Figure 2C), and the roasting temperature and time interaction were significant (p < 0.05) (Table 3).
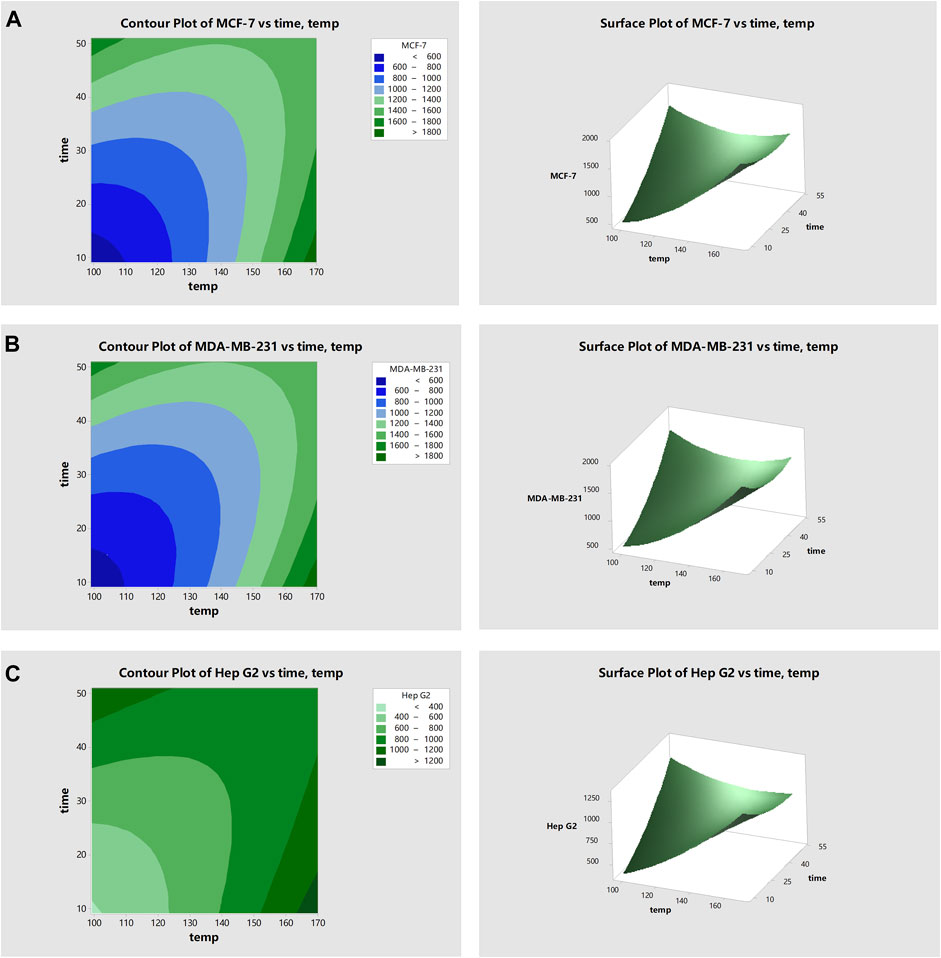
FIGURE 2. Response surface plots showing how roasting of fermented avocado seeds at varying time and temperature influenced the IC50 values of three different cancer cell lines. Surface plots depict (A) IC50 value of breast cancer cell line, MCF-7; (B) IC50 value of breast cancer cell line, MDA-MB-231; and (C) IC50 value of liver cancer cell line, Hep G2.
Figure 2 shows the response surface plots of IC50 values for the three cancer cell lines (MDA-MB-231, MCF-7 and Hep G2). With increasing roasting temperature and roasting time, the IC50 values of all three cell lines increased, indicating a decrease in anticancer activity. For surface plots of IC50 of MCF-7, the lowest IC50 values (highest anticancer activities) were obtained when roasting from 99 to 125°C for between 9 and 23 min. For MDA-MB-231, the lowest IC50 values were obtained when roasting from 99 to 124°C for between 9 and 26 min.
For Hep G2, the lowest IC50 values were obtained when roasting the fermented seeds from 99 to 122°C for between 9 and 26 min. This increase in anticancer activity may be attributed to the Maillard reaction. Yamabe, Kim et al. (2013) reported that Ginsenoside Re, one of the major triol-type ginsenosides of Panax ginseng was transformed into less-polar ginsenosides by antioxidant Maillard reaction products. The generation of less-polar ginsenosides increased the anticancer effect of the ginsenoside Re–lysine mixture upon heat processing.
4.4 Response optimisation
The optimisation of roasting time and temperature was based on maximising antioxidant activities and anticancer activities (by minimising IC50 values) as shown in Figure 3. Using the response optimizer function in Minitab, the IC50 values of MCF-7, MDA-MB-231 and Hep G2 were minimised because the lower IC50 value corresponded to higher anticancer activity. Results indicated that roasting of L. plantarum fermented avocado seed at 127°C for 24.7 min resulted in a powder with high anticancer and antioxidant activities. The corresponding predicted values of IC50 on MDA-MB-231, MCF-7 and Hep G2 of avocado seeds powder under the optimised roasting conditions were 851.60, 925.63 and 680.70 μg/ml respectively. Among the three cancer cell lines, the lowest IC50 value was for Hep G2, which is a liver cancer cell. The corresponding predicted values for CUPRAC and FRAP antioxidant activities were 41.29 and 7.76 mg ascorbic acid equivalent/g respectively under the optimum conditions.
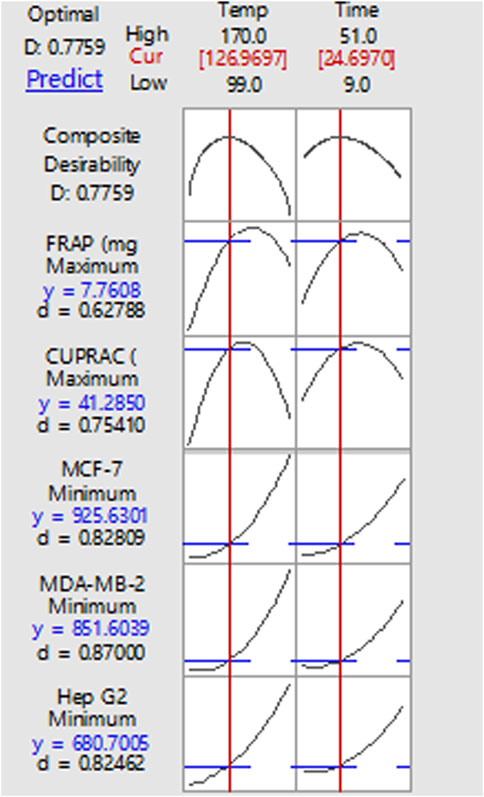
FIGURE 3. Response optimizer plot to determine the optimum roasting temperature and time conditions based on IC50 values and antioxidant activities. d value is the composite desirability and y value is the predicted response.
5 Conclusion
This study set out to determine how the fermentation of avocado seeds in combination with roasting influenced antioxidant and anticancer activities, as well as TPC. The results showed that avocado seeds fermented with L. plantarum yielded the highest antioxidant activities compared to naturally and kefir fermented samples. The roasting of fermented avocado seeds further increased antioxidant activities. However, increasing roasting temperature and time reduced anticancer activities. Roasting of fermented avocado seeds showed the best inhibition effect on the Hep G2 cancer cell line. Optimisation of roasting conditions of fermented avocado seeds for maximum antioxidant and anticancer activities was found to be at 127°C and 25 min. Processing of avocado seeds with fermentation and roasting successfully produced a powder with improved bioactivities that can potentially be used as a functional food ingredient. Future research should focus on further chemical characterisation of the avocado seed powder in terms of bioactive components and investigate its use as a food ingredient or natural antioxidant.
Data availability statement
The original contributions presented in the study are included in the article/Supplementary Material, further inquiries can be directed to the corresponding author.
Author contributions
ZZ—Conceptualization, Methodology, Validation, Formal analysis, Investigation, Writing—Original Draft, Writing—Review and Editing, Visualization NH—Conceptualization, Methodology, Software, Resources, Writing—Original Draft, Writing—Review and Editing, Supervision, Project administration, Funding acquisition LQ—Methodology, Formal analysis, Investigation, Visualization NG-M—Conceptualization, Methodology, Resources, Writing—Original Draft, Supervision, Funding acquisition KK—Methodology, Software, Formal analysis, Data Curation, Writing—Original Draft, Visualization, Project administration KW—Methodology, Writing—Review & Editing JL—Methodology, Resources, Supervision, TL—Writing—Review and Editing, Supervision.
Acknowledgments
The authors acknowledge the support of Olivado Ltd who kindly provided the avocado seeds.
Conflict of interest
The authors declare that the research was conducted in the absence of any commercial or financial relationships that could be construed as a potential conflict of interest.
Publisher’s note
All claims expressed in this article are solely those of the authors and do not necessarily represent those of their affiliated organizations, or those of the publisher, the editors and the reviewers. Any product that may be evaluated in this article, or claim that may be made by its manufacturer, is not guaranteed or endorsed by the publisher.
Supplementary material
The Supplementary Material for this article can be found online at: https://www.frontiersin.org/articles/10.3389/frfst.2022.986868/full#supplementary-material
References
Abubakar, A. N. F., Achmadi, S. S., and Suparto, I. H. (2017). Triterpenoid of avocado (Persea americana) seed and its cytotoxic activity toward breast MCF-7 and liver HepG2 cancer cells. Asian Pac. J. Trop. Biomed. 7 (5), 397–400. doi:10.1016/j.apjtb.2017.01.010
Açar, Ö. Ç., Gökmen, V., Pellegrini, N., and Fogliano, V. (2009). Direct evaluation of the total antioxidant capacity of raw and roasted pulses, nuts and seeds. Eur. Food Res. Technol. 229 (6), 961–969. doi:10.1007/s00217-009-1131-z
Aden, D. P., Fogel, A., Plotkin, S., Damjanov, I., and Knowles, B. B. (1979). Controlled synthesis of HBsAg in a differentiated human liver carcinoma-derived cell line. Nature 282 (5739), 615–616. doi:10.1038/282615a0
Bean, H., Radu, F., De, E., Schuler, C., Leggett, R. E., and Levin, R. M. (2009). Comparative evaluation of antioxidant reactivity within obstructed and control rabbit urinary bladder tissue using FRAP and CUPRAC assays. Mol. Cell. Biochem. 323 (1-2), 139–142. doi:10.1007/s11010-008-9972-5
Bressani, A. P. P., Martinez, S. J., Evangelista, S. R., Dias, D. R., and Schwan, R. F. (2018). Characteristics of fermented coffee inoculated with yeast starter cultures using different inoculation methods. LWT 92, 212–219. doi:10.1016/j.lwt.2018.02.029
Çelik, S. E., Özyürek, M., Güçlü, K., and Apak, R. (2010). Solvent effects on the antioxidant capacity of lipophilic and hydrophilic antioxidants measured by CUPRAC, ABTS/persulphate and FRAP methods. Talanta 81 (4-5), 1300–1309. doi:10.1016/j.talanta.2010.02.025
Chai, K. F., Chang, L. S., Adzahan, N. M., Karim, R., Rukayadi, Y., and Ghazali, H. M. (2018). Physicochemical properties and toxicity of cocoa powder-like product from roasted seeds of fermented rambutan (Nephelium lappaceum L.) fruit. Food Chem. 271, 298–308. doi:10.1016/j.foodchem.2018.07.155
Đorđević, T. M., Šiler-Marinković, S. S., and Dimitrijević-Branković, S. I. (2010). Effect of fermentation on antioxidant properties of some cereals and pseudo cereals. Food Chem. 119 (3), 957–963. doi:10.1016/j.foodchem.2009.07.049
Ejiofor, N., Ezeagu, I., Ayoola, M., and Umera, E. (2018). Determination of the chemical composition of avocado (Persea americana) seed. Adv. Food Technol. Nutr. Sci. Open J., S51–S55. doi:10.17140/aftnsoj-se-2-107
Fernandez-Orozco, R., Frias, J., Muñoz, R., Zielinski, H., Piskula, M. K., Kozlowska, H., et al. (2007). Fermentation as a bio-process to obtain functional soybean flours. J. Agric. Food Chem. 55 (22), 8972–8979. doi:10.1021/jf071823b
Filannino, P., Di Cagno, R., and Gobbetti, M. (2018). Metabolic and functional paths of lactic acid bacteria in plant foods: Get out of the labyrinth. Curr. Opin. Biotechnol. 49, 64–72. doi:10.1016/j.copbio.2017.07.016
Fleet, G. H., and Zhao, J. (2018). Unravelling the contribution of lactic acid bacteria and acetic acid bacteria to cocoa fermentation using inoculated organisms. Int. J. Food Microbiol. 279, 43–56. doi:10.1016/j.ijfoodmicro.2018.04.040
Gan, R. Y., Li, H. B., Gunaratne, A., Sui, Z. Q., and Corke, H. (2017). Effects of fermented edible seeds and their products on human health: Bioactive components and bioactivities. Compr. Rev. Food Sci. Food Saf. 16 (3), 489–531. doi:10.1111/1541-4337.12257
Hubert, J., Berger, M., Nepveu, F., Paul, F., and Daydé, J. (2008). Effects of fermentation on the phytochemical composition and antioxidant properties of soy germ. Food Chem. 109 (4), 709–721. doi:10.1016/j.foodchem.2007.12.081
Hur, S. J., Lee, S. Y., Kim, Y.-C., Choi, I., and Kim, G.-B. (2014). Effect of fermentation on the antioxidant activity in plant-based foods. Food Chem. 160, 346–356. doi:10.1016/j.foodchem.2014.03.112
Ioannone, F., Di Mattia, C. D., De Gregorio, M., Sergi, M., Serafini, M., and Sacchetti, G. (2015). Flavanols, proanthocyanidins and antioxidant activity changes during cocoa (Theobroma cacao L.) roasting as affected by temperature and time of processing. Food Chem. 174, 256–262. doi:10.1016/j.foodchem.2014.11.019
Ioannou, I., Chaaban, H., Slimane, M., and Ghoul, M. (2015). Origin of the variability of the antioxidant activity determination of food material. Biotechnology, 77–92.
Jan, K., Ahmad, M., Rehman, S., Gani, A., and Khaqan, K. (2019). Effect of roasting on physicochemical and antioxidant properties of kalonji (Nigella sativa) seed flour. Food Meas. 13 (2), 1364–1372. doi:10.1007/s11694-019-00052-4
Kachouri, F., Ksontini, H., Kraiem, M., Setti, K., Mechmeche, M., and Hamdi, M. (2015). Involvement of antioxidant activity of Lactobacillus plantarum on functional properties of olive phenolic compounds. J. Food Sci. Technol. 52 (12), 7924–7933. doi:10.1007/s13197-015-1912-2
Kristanty, R. E., Suriawati, J., and Sulistiyo, J. (2014). Cytotoxic activity of avocado seeds extracts (Persea americana mill.) on t47d cell lines. Int. Res. J. Pharm. 5 (7), 557–559. doi:10.7897/2230-8407.0507113
Kuligowski, M., Pawłowska, K., Jasińska-Kuligowska, I., and Nowak, J. (2017). Isoflavone composition, polyphenols content and antioxidative activity of soybean seeds during tempeh fermentation. CyTA-Journal Food 15 (1), 27–33.
Kullisaar, T., Zilmer, M., Mikelsaar, M., Vihalemm, T., Annuk, H., Kairane, C., et al. (2002). Two antioxidative lactobacilli strains as promising probiotics. Int. J. Food Microbiol. 72 (3), 215–224. doi:10.1016/s0168-1605(01)00674-2
Lara-Marquez, M., Baez-Magana, M., Raymundo-Ramos, C., Spagnuolo, P. A., Macias-Rodriguez, L., Salgado-Garciglia, R., et al. (2020). Lipid-rich extract from Mexican avocado (Persea americana var. drymifolia) induces apoptosis and modulates the inflammatory response in Caco-2 human colon cancer cells. J. Funct. Foods 64, 103658. doi:10.1016/j.jff.2019.103658
Lee, A. V., Oesterreich, S., and Davidson, N. E. (2015). MCF-7 cells-changing the course of breast cancer research and care for 45 years, 107.
Lee, E. A., Angka, L., Rota, S.-G., Hanlon, T., Mitchell, A., Hurren, R., et al. (2015). Targeting mitochondria with avocatin B induces selective leukemia cell death. Cancer Res. 75 (12), 2478–2488. doi:10.1158/0008-5472.CAN-14-2676
Lee, S.-G., Yu, M.-H., Lee, S.-P., and Lee, I.-S. (2008). Antioxidant activities and induction of apoptosis by methanol extracts from avocado. J. Korean Soc. Food Sci. Nutr. 37 (3), 269–275. doi:10.3746/jkfn.2008.37.3.269
Lin, J.-T., Liu, S.-C., Hu, C.-C., Shyu, Y.-S., Hsu, C.-Y., and Yang, D.-J. (2016). Effects of roasting temperature and duration on fatty acid composition, phenolic composition, Maillard reaction degree and antioxidant attribute of almond (Prunus dulcis) kernel. Food Chem. 190, 520–528. doi:10.1016/j.foodchem.2015.06.004
Othman, N. B., Roblain, D., Chammen, N., Thonart, P., and Hamdi, M. (2009). Antioxidant phenolic compounds loss during the fermentation of Chétoui olives. Food Chem. 116 (3), 662–669. doi:10.1016/j.foodchem.2009.02.084
Padilla-Camberos, E. (2013). Acute toxicity and genotoxic activity of avocado seed extract (persea americana mill., c.v. Hass). Sci. World J. 2013, 4.
Palani, K., Harbaum-Piayda, B., Meske, D., Keppler, J. K., Bockelmann, W., Heller, K. J., et al. (2016). Influence of fermentation on glucosinolates and glucobrassicin degradation products in sauerkraut. Food Chem. 190, 755–762. doi:10.1016/j.foodchem.2015.06.012
Papalexandratou, Z., Lefeber, T., Bahrim, B., Lee, O. S., Daniel, H.-M., and De Vuyst, L. (2013). Hanseniaspora opuntiae, Saccharomyces cerevisiae, Lactobacillus fermentum, and Acetobacter pasteurianus predominate during well-performed Malaysian cocoa bean box fermentations, underlining the importance of these microbial species for a successful cocoa bean fermentation process. Food Microbiol. 35 (2), 73–85. doi:10.1016/j.fm.2013.02.015
Permal, R., Leong Chang, W., Seale, B., Hamid, N., and Kam, R. (2020). Converting industrial organic waste from the cold-pressed avocado oil production line into a potential food preservative. Food Chem. 306, 125635. doi:10.1016/j.foodchem.2019.125635
Relda, C., Matilde, O., and Quita, V. J. C. (1978). Long-term human breast carcinoma cell lines of metastatic origin: Preliminary characterization. Vitro 14 (11), 911–915. doi:10.1007/BF02616120
Rodríguez-Carpena, J.-G., Morcuende, D., Andrade, M.-J., Kylli, P., and Estévez, M. (2011). Avocado (Persea americana Mill.) phenolics, in vitro antioxidant and antimicrobial activities, and inhibition of lipid and protein oxidation in porcine patties. J. Agric. Food Chem. 59 (10), 5625–5635. doi:10.1021/jf1048832
Romero-Cortes, T., Salgado-Cervantes, M. A., García-Alamilla, P., García-Alvarado, M. A., del C Rodríguez-Jimenes, G., Hidalgo-Morales, M., et al. (2013). Relationship between fermentation index and other biochemical changes evaluated during the fermentation of Mexican cocoa (Theobroma cacao) beans. J. Sci. Food Agric. 93 (10), 2596–2604. doi:10.1002/jsfa.6088
Santos, D. M., Ascheri, D. P. R., Bukzem, A., Morais, C., Piler Carvalho, C., and Ascheri, J. L. (2016). Physicochemical properties of starch from avocado seed. Persea Americana mill, 34.
Spada, F. P., Zerbeto, L. M., Ragazi, G. B. C., Gutierrez, E. r. M. R., Souza, M. C., Parker, J. K., et al. (2017). Optimization of postharvest conditions to produce chocolate aroma from jackfruit seeds. J. Agric. Food Chem. 65 (6), 1196–1208. doi:10.1021/acs.jafc.6b04836
Torino, M. I., Limón, R. I., Martínez-Villaluenga, C., Mäkinen, S., Pihlanto, A., Vidal-Valverde, C., et al. (2013). Antioxidant and antihypertensive properties of liquid and solid state fermented lentils. Food Chem. 136 (2), 1030–1037. doi:10.1016/j.foodchem.2012.09.015
Wang, Y., Compaoré-Sérémé, D., Sawadogo-Lingani, H., Coda, R., Katina, K., and Maina, N. H. (2019). Influence of dextran synthesized in situ on the rheological, technological and nutritional properties of whole grain pearl millet bread. Food Chem. 285, 221–230. doi:10.1016/j.foodchem.2019.01.126
Widiyastuti, Y., Pratiwi, R., Riyanto, S., and Wahyuono, S. (2018). Cytotoxic activity and apoptosis induction of avocado Persea americana Mill. seed extract on MCF-7 cancer cell line. Indones. J. Biotechnol. 23 (2), 61–67. doi:10.22146/ijbiotech.32141
Yamabe, N., Kim, Y.-J., Lee, S., Cho, E.-J., Park, S.-H., Ham, J., et al. (2013). Increase in antioxidant and anticancer effects of ginsenoside Re–lysine mixture by Maillard reaction. Food Chem. 138 (2), 876–883. doi:10.1016/j.foodchem.2012.12.004
Yusuf, A., Ayedun, H., and Sanni, L. (2008). Chemical composition and functional properties of raw and roasted Nigerian benniseed (Sesamum indicum) and Bambara groundnut (Vigna subterranean). Food Chem. 111 (2), 277–282. doi:10.1016/j.foodchem.2007.12.014
Zhang, M. (2017). Proximate and chemical composition of New Zealand avocado by-products (Persea americana Mill. cv Hass). Auckland University of Technology.
Keywords: fermentation, roasting, Hass avocado, antioxidant, anticancer
Citation: Zhao Z, Hamid N, Qian L, Gutierrez-Maddox N, Kam R, Kantono K, Wang K, Lu J and Le TT (2022) The effects of roasting on antioxidant and anticancer activities of fermented Hass avocado seeds. Front. Food. Sci. Technol. 2:986868. doi: 10.3389/frfst.2022.986868
Received: 05 July 2022; Accepted: 15 November 2022;
Published: 25 November 2022.
Edited by:
Vicente M. Gómez López, Catholic University San Antonio of Murcia, SpainReviewed by:
Oluwafemi Adeleke Ojo, Bowen University, NigeriaCarmen Hernandez-Brenes, Tecnologico de Monterrey, Mexico
Copyright © 2022 Zhao, Hamid, Qian, Gutierrez-Maddox, Kam, Kantono, Wang, Lu and Le. This is an open-access article distributed under the terms of the Creative Commons Attribution License (CC BY). The use, distribution or reproduction in other forums is permitted, provided the original author(s) and the copyright owner(s) are credited and that the original publication in this journal is cited, in accordance with accepted academic practice. No use, distribution or reproduction is permitted which does not comply with these terms.
*Correspondence: Nazimah Hamid, nazimah.hamid@aut.ac.nz