- 1Instituto Politécnico Nacional, CEPROBI, Yautepec, Mexico
- 2Tecnológico Nacional de México, Instituto Tecnológico de Acapulco, Acapulco, Mexico
- 3Tecnológico Nacional de México/Instituto Tecnológico de Tuxtepec, San Juan Bautista Tuxtepec, Oaxaca, Mexico
- 4Conacyt-Instituto Politécnico Nacional, CEPROBI, Yautepec, Mexico
- 5Departamento de Ingenieria de Procesos e Hidraulica, Universidad Autónoma Metropolitana-Iztapalapa, Iztapalapa, Mexico
Semolina pasta with improved nutritional properties is increasingly demanded by consumers. High protein content and low starch digestibility are features desired for a functional pasta. In this work, amaranth flour was used for 50% supplementation of semolina in pasta preparation. Raw amaranth flour increased protein from 11.7 g/100 g to about 14.0 g/100, dietary fiber from 6.8 g/100 g to about 8.0–10.0 g/100 g. However, raw amaranth flour deteriorated the texture of cooked pasta by increasing hardness from 15.7 to 52.8 N and reducing cohesiveness from 0.71 to 0.55. Pregelatinizations at 50 and 100% were explored to reduce the adverse effects of raw amaranth flour. The gelatinized amaranth flour mimicked the texture of semolina pasta, although cooking loss increased from 3.3 g/100 g to about 9.2 g/100 g, and the water absorption showed a marked reduction from 116.4% to about 80.0–84.0%. The in vitro starch digestibility was similar for pasta made with semolina and pasta containing pregelatinized starch, although digestibility decreased from 95 to 85% for raw amaranth flour. Overall, the results showed that amaranth flour offered advantages and drawbacks for the formulation of pasta with improved nutritional features.
Introduction
The formulation and characterization of functional foods have been a growing field in academic research and industrial production. An increasing number of consumers are demanding food products with health benefits. Functional ingredients in food products include dietary fiber, prebiotics and probiotics, antioxidant compounds and vitamins. The challenge to producing functional foods is to achieve sensory, texture, and cooking quality that are similar to traditional food (Alongi and Anese, 2021).
Pasta is consumed in practically all world regions, is easy to produce and prepare and relatively inexpensive. Pasta formulation offers flexibility for supplementation to increase protein content (Jayawardena et al., 2018), dietary fiber (Tudoricǎ et al., 2002), or antioxidant capacity (Laus et al., 2017). Dehulled pulses (e.g., lentils and peas) are important sources of protein supplementation for pasta (Bresciani et al., 2021). Oat, wheat and rice brans are commonly used to improve soluble fiber content (Krishnan et al., 2012). Polyphenols from different sources are increasingly incorporated in commercial pasta formulations (Turco et al., 2019). However, the use of non-wheat ingredients commonly yields pasta with undesired characteristics, including disrupted texture and sensorial attributes. On the other hand, the surge of consumers with healthy (e.g., natural ingredients) and socio-ecological (non-animal ingredients) demands triggered the research on new pasta formulations. In particular, pulses offer certain economical and dietary advantages, since these flours have been explored for the management of diabetes (Ramdath et al., 2016). Reports have shown that pasta produced from amaranth flour exhibits decreased texture firmness and cooking time, while pasta from quinoa mainly shows an increased cooking loss (Schoenlechner et al., 2010). Amaranth has high protein with essential amino acids and waxy type starch with small granule size, which can confer specific characteristics to foods (pasta, bakery products, snacks, etc.) in the form of pregelatinized ingredients (Garcia-Valle et al., 2021). Green banana flour is a by-product that has been proposed for improving pasta quality and nutritional properties (Garcia-Valle et al., 2021). The high protein content of the chickpea flour (21.73 g/100 g) is advantageous to improve the nutrition characteristic of semolina pasta. The addition of chickpea flour in the formulation led to reduced in vitro starch digestibility as a consequence of the high protein and dietary fiber content. Interactions of dietary fiber with starch (Zheng et al., 2016) and inhibition of α-amylase by cellulose (Dhital et al., 2015) are potential mechanisms involved in decreased starch digestibility. Pregelatinization by extrusion was used for conditioning mango flour to improve texture and starch digestibility in pasta formulations (Garcia-Valle et al., 2021). Yellow lentil flour was subjected to extrusion-cooking to obtain pasta with improved texture (Bresciani et al., 2021).
Non-conventional flours offer nutritional advantages over traditional cereal flour. For instance, pulses are relatively inexpensive and commonly contain large amounts of proteins. However, the preparation of pasta incorporating non-conventional wheat flour commonly results in texture problems. Hydrocolloids (e.g., gums) have been used in combination with non-conventional flours to fix the texture requirements for pasta (Chauhan et al., 2017). However, hydrocolloids might increase pasta fabrication costs and add undesired sensorial attributes. Partial or total gelatinized flours are ad hoc alternatives for hydrocolloids (Bento et al., 2021). Pasta prepared with gelatinized flour exhibited optimal cooking time as well as high protein and dietary fiber contents. Motivated by the aforementioned reports, the present study aimed to evaluate the gelatinization degree (50 and 100%) of amaranth flour in the structuration of the pasta and its effect on the texture and in vitro starch digestion. Semolina pasta and pasta with raw amaranth flour were used as control.
Materials and Methods
Materials
Wheat flour (Triticum durum) was obtained from San Blas Milling Co. (Puebla, Mexico). Amaranth grains were collected in an experimental crop field in Central Mexico (Texcoco, Estado de México, México). Amaranth grains were milled and sieved at 40 mesh. The different chemical reagents were purchased at JT Baker (Avantor Performance Materials, Center Valley, PA, Unite States), Sigma Aldrich (Sigma Chemical Co., St Louis MO, USA), and Fermont (Productos Químicos Monterrey, Monterrey, México).
Flour Extrusion
Amaranth flour was extruded with a single screw-extruded (Beutelspacher, CDMX, Mexico) at a constant rotation rate of 75 rpm. Before extrusion, flour was conditioned for 18 h with a water content of 35% (base of dry flour) in a plastic bag. Two constant temperatures were used in the three zones of the extruder (first zone of the barrel, blend zone, and end zone): 50 and 80 °C. Two batches were prepared for each sample. After extrusion, the pellets were milled and sieved (mesh 40, mean particle size 0.042 cm). Two degrees of flour gelatinization of 50% (E50) and 100% (E100) were used for pasta preparation.
Pasta Formulation and Processing
Pasta formulations consisted of blends of durum wheat semolina and amaranth flours: S100 denotes 100% semolina, S50-A50 denotes 50% semolina and 50% amaranth flour, S50-E50 denotes 50% semolina and 50% amaranth flour with 50% gelatinization, and S50-E100 denotes 50% semolina and 50% amaranth flour with 100% of gelatinization. Sheeting was carried out with a manual Pasta & Co. machine (Metaltex Molsheim, France). Afterward, the pasta formulations were dried at 45°C for 4 h in an oven under a relative humidity of about 40% (Biotecnica del Bajio S.A. de C.V., Celaya, Mexico). The pasta formulation was stored at 25 ± 2°C in sealed plastic containers until further analysis.
Chemical Composition
Moisture, ash, fat and protein (N × 6.25) contents were determined according to AACC Method 44–15, 08–01, 30–25, and 46–13, respectively (AACC, 2010). Total starch was measured according to AACC method 76.13 (AACC, 2010) using a total starch analysis kit from Megazyme (Wicklow, Ireland). Total dietary fiber (TDF) was assessed for cooked samples at the optimum cooking time, using two different methods: 1) Method AACC 2000 32–05, which quantifies the indigestible material after heat treatment of the food sample (i.e., non-starch polysaccharides), and 2) enzyme-based method proposed by McCleary (McCleary, 2007), which allows total resistant starch fractions to remain associated to the TDF (non-starch polysaccharides) residues, under conditions that resemble the physiological behavior. All analyses were performed in triplicate.
Thermal Properties
The gelatinization degree (DG) of raw pasta was performed with differential scanning calorimetry (DSC). Approx. 2.0 mg sample and 7 µL of deionized water were equilibrated for 12 h at room temperature in a DSC pan, and subjected to the heating ramp for the range 20–120°C at a heating rate of 10°C/min in a DSC model 2010 (TA Instrument, New Castle, NJ). The temperature of the phase transition and enthalpy (area under the phase transition curve) were calculated with the software of the equipment. The degree of gelatinization (DG) was calculated as:
Cooking Quality
The cooking quality (optimum cooking time and cooking loss) of the pasta formulations was assessed according to AACC 2000 66–50 method (AACC, 2010). The water absorption was determined for 10 g of pasta sample (cut into 5 cm long pieces), and cooked in 300 ml at optimum cooking time. The pasta was then drained and rinsed with 20 ml of distilled water at room temperature for 2 min. The sample was weighed after reaching temperature. The water absorption was determined as follows:
Texture Analysis
Two batches of each pasta formulation were cooked at boiling temperature for optimum cooking time. Five subsamples were used for texture profile analysis (TPA) using the texture analyzer (Brookfield CT3, Middleboro, MA 02346–1031) within 5 min after cooking. The measurements were carried out with a cylindrical probe (TA 4/1000, DD 38.1 mm, L 20 mm) and 10 kg load cell. The samples were compressed to 75% of their original height. The determining variables were pasta hardness, adhesiveness, cohesiveness, elasticity, and chewiness. TexturePro CT software was used to record the data and estimate the texture parameters.
In vitro Starch Digestibility
The in vitro starch digestibility was determined according to the procedure described by Bello-Pérez et al. (Bello-Perez et al., 2019), where starch digestion was carried out from the mouth to the small intestine. Results were presented as a percentage of digested on a dry basis.
Statistical Analysis
Results were reported as the mean ± standard error. Data were analyzed by one-way analysis variance (ANOVA) (p < 0.05) followed by LSD multiple comparison procedure to determine significant differences among samples (SPSS v. 20 IBM, NY).
Results and Discussion
Chemical Composition
Pasta made with semolina-amaranth flour blends contained reduced moisture contents (4.0–4.7 g/100 g) relative to the pasta made with semolina (6.6 g/100 g). The differences can be attributed to the reduced gluten content in pasta containing amaranth flour. Gluten exhibits a marked capacity for water retention, providing pasta with enhanced elasticity (Almutawah et al., 2007). Protein, ash and fat contents increased for pasta supplemented with amaranth flour, and the increase was not dependent on whether the flour was gelatinized or not. The increase of such fractions is linked to the high contents in amaranth flour, posing this flour as a suitable ingredient to increase the nutritional characteristics of pasta. On the other hand, the total starch content showed an important decrease from about 65.0 g/100 g for semolina pasta to about 55.0 g/100 g for pasta with amaranth flour. Decreased starch content is an important issue given the metabolic syndrome problems related to high ingesta of digestible carbohydrates (Regalado-Rentería et al., 2020). Dietary fiber showed an important increase caused by the non-starch components of the amaranth flour (Tamsen et al., 2018). It should be recalled that the method AACC 200 32–05 determines total dietary fiber (non-starch polysaccharides), but resistant starch (fraction of the dietary fiber) is not quantified. The second method determines both non-starch polysaccharides and resistant starch. Hence, the results in Table 1 indicated that the pasta contains resistant starch that acted as dietary fiber.
Cooking Quality
The optimum cooking time of semolina pasta was about 16.6 min and decreased with the addition of the amaranth flour (Table 2). Gelatinization reduced the optimum cooking time to about 10–12 min, which was an expected result given the preconditioned structure of pasta with gelatinized starch. The optimum cooking time agrees well with the values reported by Yalcin and Basman (Yalcin and Basman, 2008) for gluten-free corn noodles prepared with different proportions of gelatinized starch. The cooking time reduction was accompanied by a negative effect as reflected by the increased levels of cooking loss, which increased from about 3.3 g/100 g for semolina pasta to about 9.2 g/100 g for pasta made with gelatinized flour.
The cooking loss parameter reflects the solids that were leached in the cooking process. It is an index of the resistance to disintegration during pasta cooking (Table 2). The cooking loss of semolina pasta was about 3.3 g/100 g, and increased to 9.2 g/100 g for pasta containing gelatinized amaranth flour. This pattern is related to short chains present in amaranth starch (Paredes-López et al., 1994) that were disorganized during the extrusion (partial or complete gelatinization) and during cooking. The absence of a gluten network to entrap the pasta components (e.g., proteins and starch) and the availability of amylose chains by the gelatinization process led to pasta with high losses during cooking (Marti and Pagani, 2013). These results agree with Larrosa et al. (Larrosa et al., 2016), who found that gluten-free pasta exhibited high cooking loss values as a consequence of the lack of a robust network to stabilize the pasta structure in the cooking process. The water absorption capacity was negatively correlated (Pearson correlation = −0.96, p < 0.03) with the cooking loss. Gluten is hydrated to form a stabilizing network with a high capacity for water retention (Roccia et al., 2009). The reduced or even lack of gluten fraction in a pasta formulation weakened the structure, leading to a poor capacity of water retention during cooking.
The addition of amaranth flour produced pasta with reduced width and thickness dimensions. This effect is probably due to the reduced water retention capacity of pasta with lowered gluten content. However, the effect was not visible for the width of cooked pasta where the dimension differences were not statistically significant (p < 0.05). In contrast, the thickness was higher for pasta containing gelatinized flour. The amylose released in the gelatinization process produced a pasta structure with the capacity of retaining water during the cooking process.
Texture
Cooked semolina pasta (S100) and cooked pasta containing gelatinized flour (S50-E50 and S50-E100) exhibited similar hardness values (13.0–15.0 N), although the latter pasta with slightly smaller values (Table 3). In contrast, the hardness of cooked pasta containing non-gelatinized amaranth flour (S50-A50) was markedly high (52.8 N). Probably, the native starch granules of the amaranth flour (small size and high amylopectin content) induced the increased hardness value of the cooked pasta. This effect was reflected in adhesiveness, which was the smallest value for semolina pasta (0.07 N-s), increased for pasta containing gelatinized flour (0.43–0.62 N.s) and achieved the highest value (2.04 N.s) for pasta with non-gelatinized flour. Chewiness showed a similar trend. In contrast, cohesiveness and elasticity were only slightly affected by the addition of amaranth flour. In particular, the amaranth flour addition reduced the pasta elasticity, an effect that can be ascribed to the reduced gluten content. Schoenlechner et al. (Schoenlechner et al., 2010) reported that the reduced firmness of amaranth pasta may be attributed to the lack of gluten, which led to a weak structural matrix. They used casein and egg protein to supplement gluten and improve pasta texture. The results in Table 3 showed that pregelatinized flour improved some texture features (e.g., hardness and elasticity), while maintaining the reduced gluten content.
Thermal Properties
Figure 1. a illustrates the thermal flow patterns for the different pasta formulations. Three endothermic peaks were obtained, which can be attributed as follows (Table 4). The first peak at the lowest peak temperatures of 63–65°C reflects the denaturation of the gluten (Falcão-Rodrigues et al., 2005) and the proteins contained in the amaranth flour (Martínez and Añón, 1996). The second peak located at about 74.5–77.0°C can be linked to starch gelatinization, whereas the peak at the highest temperature of 85.0–99.0°C to amylose-lipid complexes (Biliaderis et al., 1985). Figure 1. b shows the thermal flow pattern of the different pasta formulations. Except for the semolina pasta S100, the pasta formulations containing amaranth flour exhibited the three endothermic peaks illustrated in Figure 1. a. The onset and peak denaturation temperatures showed a slight increase with the addition of the amaranth flour. However, the denaturation enthalpy showed an important decrease from 7.9 J/g for S100 to 0.9 J/g for S50-E100. This suggests that gluten from semolina interacted with proteins to form a weak matrix with lowered ability to retain water (Table 2). As expected, the addition of gelatinized amaranth flour led to a decrease in the gelatinization enthalpy. Also, the gelatinized amaranth flour produced pasta with a reduced content of amylose-lipid inclusion complexes, as revealed by the reduced enthalpy of the thirst endothermic peak.
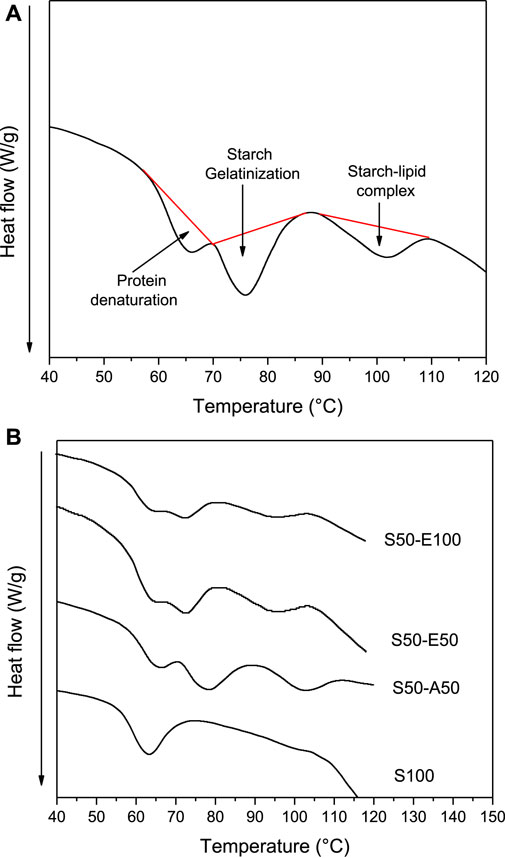
FIGURE 1. Differential scanning calorimetry (DSC) thermograms. (A) Phase transitions. (B) DSC-traces of raw pastas.
In vitro Digestibility
Figure 2 shows the digestograms of the cooked pasta. All cases presented an initial fast starch hydrolysis rate, achieving starch conversions of the order of 25–30%. For longer times, the hydrolysis curves evolved in different directions with an apparent slower hydrolysis rate. A log-of-slope (LOS) analysis of the digestograms was carried out to verify the presence of two phases in the starch hydrolysis kinetics. Figure 2 also shows the LOS results where two-phase hydrolysis was exhibited. The slope of the LOS plots corresponds to the starch hydrolysis rate according to first-order kinetics. The enzymatic hydrolysis transited from a fast rate for small times, up to 100 min, to a slow rate for longer times. This means that the enzymatic hydrolysis of starch in the pasta formulations did not follow a uniform pattern, but rather it transited to a slower regimen at a certain time (Butterworth et al., 2012). The transition might be linked to changes in the pasta structure as a consequence of the enzymatic action. For relatively short times, starch is highly available for amylolytic enzyme binding. The advance in the starch hydrolysis disrupted the pasta structure, dispersing the starch chains in the aqueous dispersion and hardening the interaction between the enzyme and the substrate. The results in Figure 2 suggested that the digestograms can be fitted with a double exponential function of the form
where
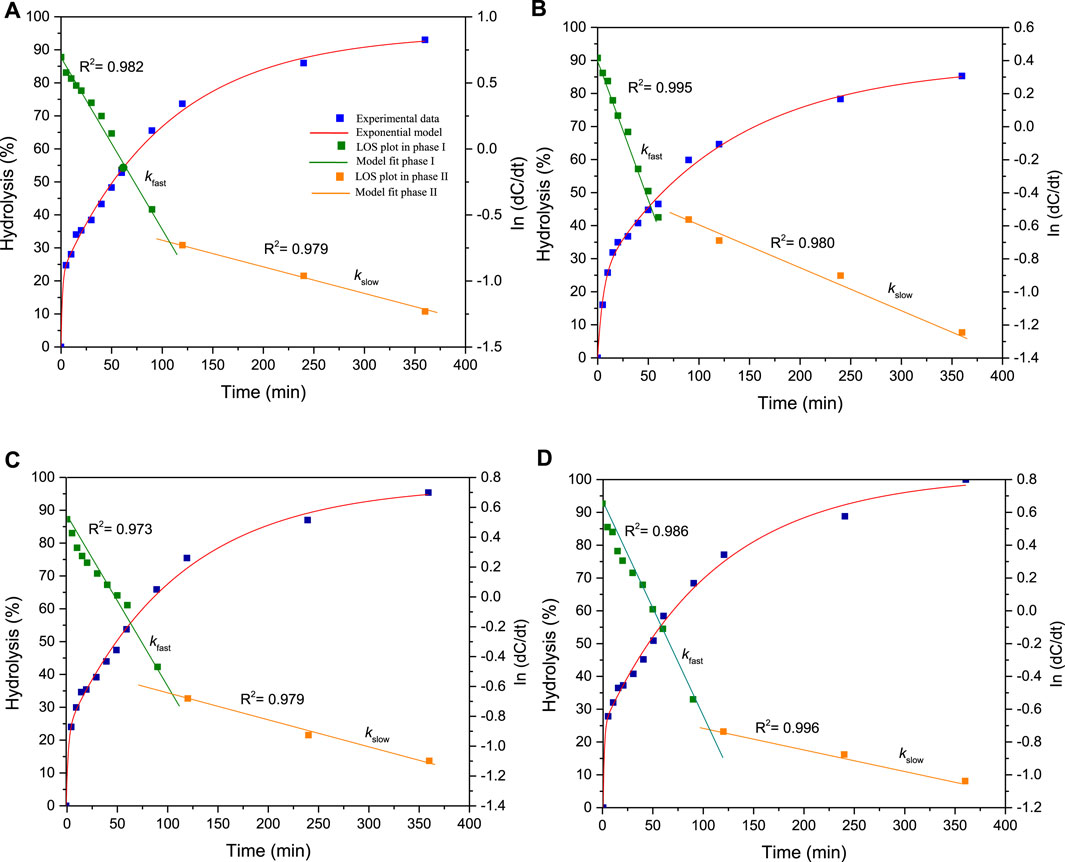
FIGURE 2. LOS computations showing two hydrolysis time scales. (A) Cooked S100, (B) Cooked S50 A50. (C) S50-E50. (D) S50-E100.
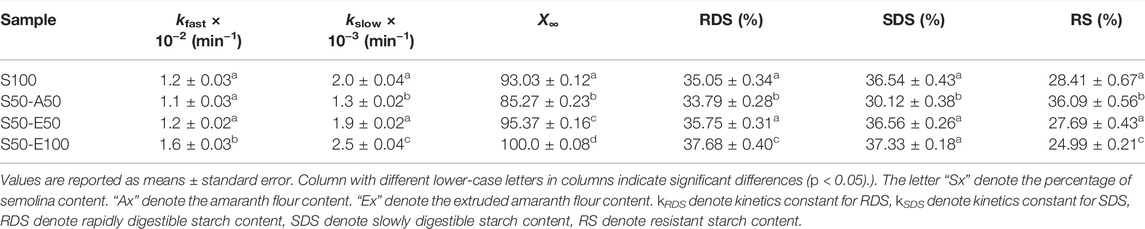
TABLE 5. Kinetics constants and digestibility fractions of cooked pasta made with wheat semolina and amaranth flours.
Discussion
The use of non-traditional flours as innovative ingredients for functional starchy food formulations has been a research and commercial trend in the recent decade (Naqash et al., 2017). The results of this work showed that amaranth flour is a visible complement to improving the nutritional quality of semolina pasta. Amaranth flour adds proteins and dietary fiber while reducing the total starch content of pasta, which are improvements over semolina pasta. However, the incorporation of amaranth flour degraded the pasta texture, and possibly the acceptability of consumers. Many studies have proposed to use of different polysaccharides to compensate for the degradation of pasta texture by non-conventional flours. For instance, Cai et al. (Cai et al., 2016) used xanthan gum for improving the cohesiveness of rice noodles. Feng et al. (Feng et al., 2020) studies chitosan, xanthan gum and sodium alginate as texture supplements for pasta based on wet sweet potato. The results showed that such polysaccharides improved the texture and cooking properties of the pasta. However, there is an increasing group of consumers preferring food products without additives. The notion that natural and traditional recipes are linked to a healthy food practice has motivated the exploration of other alternatives to improve pasta quality. The results of this work showed that gelatinized flour can play the role of a texture improver to mimic the characteristics of semolina pasta. Gelatinization had the drawback that the starch digestibility might be increased. Besides texture improvement, pasta additives should maintain or even reduce starch digestibility, a must that should be considered in the face of the so-called metabolic syndrome. Overall, the results reported in the present study are motivating, although more research is required to reduce the adverse effects of gelatinized starch on pasta digestibility. The combination of gelatinized flour with a minimal amount of polysaccharides might be a way to obtain pasta with desired physicochemical, textural and digestibility characteristics.
Conclusion
The addition of amaranth flour in the formulation of traditional pasta based on semolina increased the nutritional characteristics. Protein and dietary fiber increased by the supplementation of semolina with amaranth flour. However, native amaranth flour degraded the cooked pasta texture by increasing hardness and reducing elasticity. Gelatinized amaranth flour was used instead to alleviate the drawbacks of granular amaranth flour in texture, leading to pasta that mimics the textural characteristics of semolina pasta. However, gelatinized amaranth flour induced undesired effects, such as increased cooking loss and in vitro starch digestibility. Overall, gelatinized amaranth flour is a viable ingredient to improve the nutritional characteristics of semolina pasta, although its incorporation should be tuned to reduce the adverse effects on cooking quality and starch digestibility. The results of this work showed that amaranth flour with a partial gelatinization degree might be used to obtain some nutritional advantages while reducing adverse effects in texture and digestibility.
Data Availability Statement
The raw data supporting the conclusions of this article will be made available by the authors, without undue reservation.
Author Contributions
LAB‐P conceived the study, analyzed, and interpreted the data. JAC‐V performed the experiments. R‐C‐G, OP‐R, and JA‐R carried out the analysis and interpretation of the data. LAB‐P and JA‐R drafted the manuscript. All authors read and approved the final version of the paper.
Funding
We appreciate the economic support from SIP-IPN, EDI-IPN CONACYT-México and COFAA-IPN. The technical assistance of Daniel E. Garcia-Valle is acknowledged.
Conflict of Interest
The authors declare that the research was conducted in the absence of any commercial or financial relationships that could be construed as a potential conflict of interest.
Publisher’s Note
All claims expressed in this article are solely those of the authors and do not necessarily represent those of their affiliated organizations, or those of the publisher, the editors and the reviewers. Any product that may be evaluated in this article, or claim that may be made by its manufacturer, is not guaranteed or endorsed by the publisher.
References
AACC (2010). Approved Methods of the AACC. 10th ed. St. Paul, MN, USA: American Association of Cereal Chemist.
Almutawah, A., Barker, S. A., and Belton, P. S. (2007). Hydration of Gluten: a Dielectric, Calorimetric, and Fourier Transform Infrared Study. Biomacromolecules 8, 1601–1606. doi:10.1021/bm061206g
Alongi, M., and Anese, M. (2021). Re-thinking Functional Food Development through a Holistic Approach. J. Funct. Foods 81, 104466. doi:10.1016/j.jff.2021.104466
Bello-Perez, L. A., Agama-Acevedo, E., Garcia-Valle, D. E., and Alvarez-Ramirez, J. (2019). A Multiscale Kinetics Model for the Analysis of Starch Amylolysis. Int. J. Biol. Macromol. 122, 405–409. doi:10.1016/j.ijbiomac.2018.10.16
Bento, J. A. C., Bassinello, P. Z., Morais, D. K., Souza Neto, M. A. d., Bataus, L. A. M., Carvalho, R. N., et al. (2021). Pre-gelatinized Flours of Black and Carioca Bean By-Products: Development of Gluten-free Instant Pasta and Baked Snacks. Int. J. Gastron. Food Sci. 25, 100383. doi:10.1016/j.ijgfs.2021.100383
Biliaderis, C. G., Page, C. M., Slade, L., and Sirett, R. R. (1985). Thermal Behavior of Amylose-Lipid Complexes. Carbohydr. Polym. 5, 367–389. doi:10.1016/0144-8617(85)90044-x
Bresciani, A., Giuberti, G., Cervini, M., and Marti, A. (2021). Pasta from Yellow Lentils: How Process Affects Starch Features and Pasta Quality. Food Chem. 364, 130387. doi:10.1016/j.foodchem.2021.130387
Butterworth, P. J., Warren, F. J., Grassby, T., Patel, H., and Ellis, P. R. (2012). Analysis of Starch Amylolysis Using Plots for First-Order Kinetics. Carbohydr. Polym. 87, 2189–2197. doi:10.1016/j.carbpol.2011.10.048
Cai, J., Chiang, J. H., Tan, M. Y. P., Saw, L. K., Xu, Y., and Ngan-Loong, M. N. (2016). Physicochemical Properties of Hydrothermally Treated Glutinous Rice Flour and Xanthan Gum Mixture and its Application in Gluten-free Noodles. J. Food Eng. 186, 1–9. doi:10.1016/j.jfoodeng.2016.03.033
Chauhan, A., Saxena, D. C., and Singh, S. (2017). Effect of Hydrocolloids on Microstructure, Texture and Quality Characteristics of Gluten-free Pasta. Food Meas. 11, 1188–1195. doi:10.1007/s11694-017-9495-4
Dhital, S., Gidley, M. J., and Warren, F. J. (2015). Inhibition of α-amylase Activity by Cellulose: Kinetic Analysis and Nutritional Implications. Carbohydr. Polym. 123, 305–312. doi:10.1016/j.carbpol.2015.01.039
Englyst, H. N., Kingman, S. M., and Cummings, J. H. (1992). Classification and Measurement of Nutritionally Important Starch Fractions. Eur. J. Clin. Nutr. 46 (Suppl. 2), S33–S50. doi:10.1080/096374803/61976
Falcão-Rodrigues, M. M., Moldão-Martins, M., and Beirao-da-Costa, M. L. (2005). Thermal Properties of Gluten Proteins of Two Soft Wheat Varieties. Food Chem. 93, 459–465. doi:10.1016/j.foodchem.2004.10.023
Feng, Y.-Y., Mu, T.-H., Zhang, M., and Ma, M.-M. (2020). Effects of Different Polysaccharides and Proteins on Dough Rheological Properties, Texture, Structure and In Vitro Starch Digestibility of Wet Sweet Potato Vermicelli. Int. J. Biol. Macromol. 148, 1–10. doi:10.1016/j.ijbiomac.2019.12.225
Garcia-Valle, D. E., Agama-Acevedo, E., Nuñez-Santiago, M. d. C., Alvarez-Ramirez, J., and Bello-Pérez, L. A. (2021). Extrusion Pregelatinization Improves Texture, Viscoelasticity and In Vitro Starch Digestibility of Mango and Amaranth Flours. J. Funct. Foods 80, 104441. doi:10.1016/j.jff.2021.104441
Jayawardena, S. R., Morton, J. D., Brennan, C. S., and Bekhit, A. E. D. A. (2018). Utilisation of Beef Lung Protein Powder as a Functional Ingredient to Enhance Protein and Iron Content of Fresh Pasta. Int. J. Food Sci. Technol. 54 (4). doi:10.1111/ijfs.13927
Krishnan, J. G., Menon, R., Padmaja, G., Sajeev, M. S., and Moorthy, S. N. (2012). Evaluation of Nutritional and Physico-Mechanical Characteristics of Dietary Fiber-Enriched Sweet Potato Pasta. Eur. Food Res. Technol. 234, 467–476. doi:10.1007/s00217-011-1657-8
Larrosa, V., Lorenzo, G., Zaritzky, N., and Califano, A. (2016). Improvement of the Texture and Quality of Cooked Gluten-free Pasta. LWT 70, 96–103. doi:10.1016/j.lwt.2016.02.039
Laus, M. N., Soccio, M., Alfarano, M., Pasqualone, A., Lenucci, M. S., Di Miceli, G., et al. (2017). Different Effectiveness of Two Pastas Supplemented with Either Lipophilic or Hydrophilic/phenolic Antioxidants in Affecting Serum as Evaluated by the Novel Antioxidant/Oxidant Balance Approach. Food Chem. 221, 278–288. doi:10.1016/j.foodchem.2016.10.050
Marti, A., and Pagani, M. A. (2013). What Can Play the Role of Gluten in Gluten Free Pasta? Trends Food Sci. Technol. 31, 63–71. doi:10.1016/j.tifs.2013.03.001
Martínez, E. N., and Añón, M. C. (1996). Composition and Structural Characterization of Amaranth Protein Isolates. An Electrophoretic and Calorimetric Study. J. Agric. Food Chem. 44, 2523–2530. doi:10.1021/jf960169p
McCleary, B. V. (2007). An Integrated Procedure for the Measurement of Total Dietary Fibre (Including Resistant Starch), Non-digestible Oligosaccharides and Available Carbohydrates. Anal. Bioanal. Chem. 389, 291–308. doi:10.1007/s00216-007-1389-6
Naqash, F., Gani, A., Gani, A., and Masoodi, F. A. (2017). Gluten-free Baking: Combating the Challenges - A Review. Trends Food Sci. Technol. 66, 98–107. doi:10.1016/j.tifs.2017.06.004
Paredes-López, O., Bello-Pérez, L. A., and López, M. G. (1994). Amylopectin: Structural, Gelatinisation and Retrogradation Studies. Food Chem. 50, 411–417. doi:10.1016/0308-8146(94)90215-1
Ramdath, D., Renwick, S., and Duncan, A. M. (2016). The Role of Pulses in the Dietary Management of Diabetes. Can. J. Diabetes 40, 355–363. doi:10.1016/j.jcjd.2016.05.015
Regalado-Rentería, E., Aguirre-Rivera, J. R., Godínez-Hernández, C. I., García-López, J. C., Oros-Ovalle, A. C., Martínez-Gutiérrez, F., et al. (2020). Effects of Agave Fructans, Inulin, and Starch on Metabolic Syndrome Aspects in Healthy Wistar Rats. ACS omega 5, 10740–10749. doi:10.1021/acsomega.0c00272
Roccia, P., Ribotta, P. D., Pérez, G. T., and León, A. E. (2009). Influence of Soy Protein on Rheological Properties and Water Retention Capacity of Wheat Gluten. LWT - Food Sci. Technol. 42, 358–362. doi:10.1016/j.lwt.2008.03.002
Schoenlechner, R., Drausinger, J., Ottenschlaeger, V., Jurackova, K., and Berghofer, E. (2010). Functional Properties of Gluten-free Pasta Produced from Amaranth, Quinoa and Buckwheat. Plant Foods Hum. Nutr. 65, 339–349. doi:10.1007/s11130-010-0194-0
Tamsen, M., Shekarchizadeh, H., and Soltanizadeh, N. (2018). Evaluation of Wheat Flour Substitution with Amaranth Flour on Chicken Nugget Properties. LWT 91, 580–587. doi:10.1016/j.lwt.2018.02.001
Tudoricǎ, C. M., Kuri, V., and Brennan, C. S. (2002). Nutritional and Physicochemical Characteristics of Dietary Fiber Enriched Pasta. J. Agric. Food Chem. 50, 347–356. doi:10.1021/jf0106953
Turco, I., Bacchetti, T., Morresi, C., Padalino, L., and Ferretti, G. (2019). Polyphenols and the Glycaemic Index of Legume Pasta. Food Funct. 10, 5931–5938. doi:10.1039/c9fo00696f
Yalcin, S., and Basman, A. (2008). Quality Characteristics of Corn Noodles Containing Gelatinized Starch, Transglutaminase and Gum. J. Food Qual. 31, 465–479. doi:10.1111/j.1745-4557.2008.00212.x
Keywords: pasta, semolina, amaranth flour, starch gelatinization, pasta texture
Citation: Bello-Pérez LA, Cabello-Vazquez JA, Carmona-Garcia R, Patiño-Rodriguez O and Alvarez-Ramirez J (2022) Preparation of Functional Pasta Supplemented with Amaranth Pregelatinized Extruded Flour. Front. Food. Sci. Technol. 2:881714. doi: 10.3389/frfst.2022.881714
Received: 23 February 2022; Accepted: 13 June 2022;
Published: 30 June 2022.
Edited by:
Viridiana Tejada-Ortigoza, Monterrey Institute of Technology and Higher Education (ITESM), MexicoReviewed by:
Amit Baran Das, Tezpur University, IndiaLilian Elizabeth Abugoch, University of Chile, Chile
Copyright © 2022 Bello-Pérez, Cabello-Vazquez, Carmona-Garcia, Patiño-Rodriguez and Alvarez-Ramirez. This is an open-access article distributed under the terms of the Creative Commons Attribution License (CC BY). The use, distribution or reproduction in other forums is permitted, provided the original author(s) and the copyright owner(s) are credited and that the original publication in this journal is cited, in accordance with accepted academic practice. No use, distribution or reproduction is permitted which does not comply with these terms.
*Correspondence: Luis A. Bello-Pérez, labellop@ipn.mx