- 1Federal University of Rio De Janeiro, School of Chemistry, Department of Biochemical Engineering, Rio de Janeiro, Brazil
- 2Centre of Biological Engineering, Universidade Do Minho, Braga, Portugal
- 3LABBELS—Associate Laboratory in Biotechnology, Bioengineering and Microelectromechanical Systems, Braga, Portugal
- 4Goiano Federal Institute of Education, Science and Technology, Rio Verde, Goiás, Brazil
Brewer’s spent yeast (BSY) is a by-product generated during beer production. After heat inactivation, large quantities of BSY are discarded or sold as a low-cost animal feed supplement. Fortunately, BSY can be a good source of valuable compounds such as β-glucan, which has several biological and techno-functional properties for application as a food ingredient. Practical application of β-glucan from BSY requires disruption cell wall and purification steps that significantly influences the yield, cost, biological, physic-chemical, and technological characteristics of this compound. This mini-review presents the use of BSY as a source of β-glucan, the available methods to extract it, and its biological and techno-functional properties.
Introduction
Beer is a beverage obtained by the alcoholic fermentation of brewer’s wort from barley malt and water, with the addition of hops (Farber and Barth, 2019). In the brewing process, yeasts utilize fermentable sugars to produce ethanol and carbon dioxide (Mohammadi et al., 2011). Some by-products are generated during beer production, which includes malt bagasse, brewer’s spent yeast (BSY), and trub (particles denser than the wort that settle at the bottom, e.g., coagulated proteins, hop residue, tannins, among others) (Onofre et al., 2017; Liu et al., 2021).
Yeasts are reused in new fermentations within a limit that considers inoculum contamination and cell vitality/viability. After reaching the maximum potential for use in fermentation, the BSY must be processed due to its complex composition and high biochemical oxygen demand. BSY is often destined for animal feed, but in other cases, it is still improperly discarded (Jaeger et al., 2020; Marson et al., 2020).
Fortunately, BSY presents a rich composition in terms of proteins (about 40–60%) and carbohydrates (about 29–54%) (Onofre et al., 2017; Liu et al., 2021), which encourages its reuse as a potential source of macro compounds for food application (Bacha et al., 2017). Approximately 1.5 kg–3 kg of BSY is produced for every 100 L of beer, reaching an annual production of up to 400 million kilograms of biomass available to obtain valuable compounds (Marson et al., 2020; Olajire, 2020). Among the macro components in yeasts are β-glucan, a polysaccharide formed by branched glucose molecules interconnected by glycosidic bonds and with several biological and technological activities (Nakashima et al., 2018; Bastos et al., 2022).
Although yeast contains an appreciable amount of β-glucan (30–60% of the dry weight) (Yang and Huang, 2021), the recovery and isolation of this compound could be difficult due to the compact and rigid cell wall (Bacha et al., 2017). Several disruption cell wall methods have been developed to extract β-glucan (Tian et al., 2019; Zheng et al., 2019; Takalloo et al., 2020), considering its yield, purity, and biological and technological properties (Figure 1). In this mini-review, the potential of BSY β-glucan as a food ingredient will be highlighted by discussing the different extraction methods to obtain it and presenting its techno-functional and biological properties.
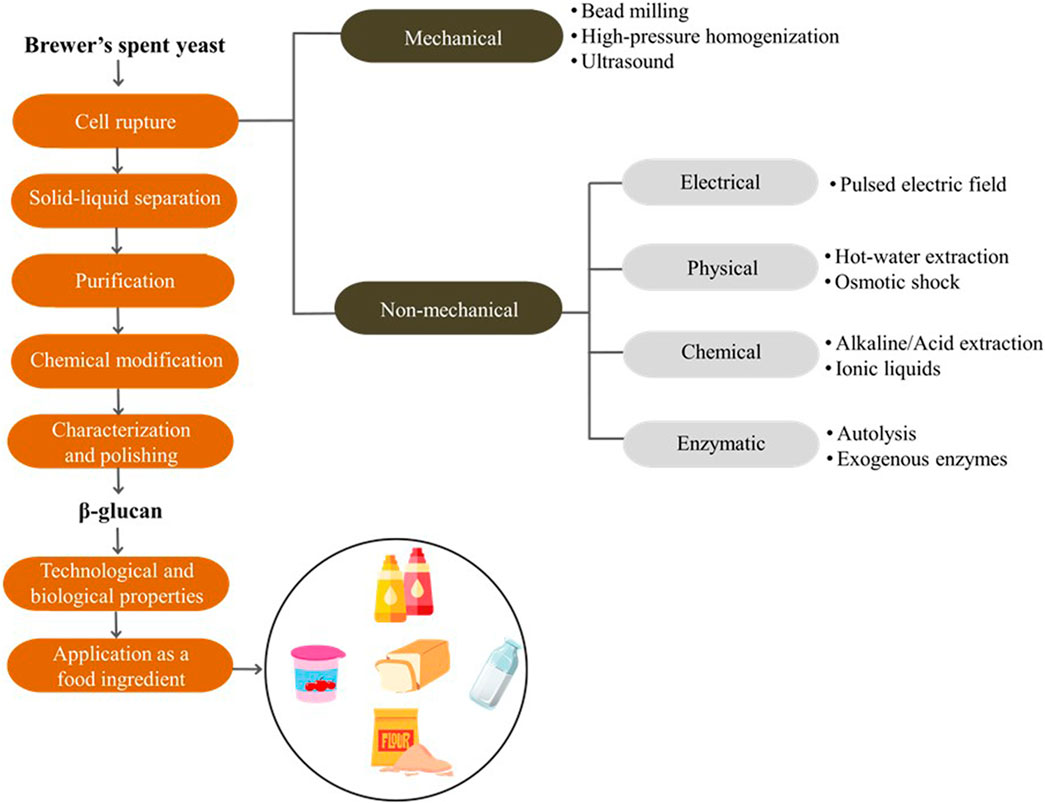
FIGURE 1. β-glucan extraction methods, characterization in terms of biological and technological properties, and application as a food ingredient.
Yeast β-glucan
Yeast β-glucan is a polysaccharide formed by branched glucose molecules interconnected by other glucose chains, especially in the β-(1,3) and β-(1,6) bonds. About 50–55% of β-(1,3) bonds are found in the β-glucan structure, which are responsible for cell elasticity, and 10–15% of β-(1,6), which serves as an anchor for cell structure and integrity (Teparić et al., 2020).
The β-glucan is abundant in the yeast cell wall (i.e., accounting for 30–60% of the dry weight) (Yang and Huang, 2021) and associated with other compounds as chitin and mannoproteins. The amount of β-glucan and the type of binding can be highly variable according to yeast type, usage cycle, cell vitality and viability, and exposure to cellular depletion factors, which include as follows: temperature shock, osmotic and oxidative stress, oxygen availability, hydrostatic pressure, ethanol concentration, internal acidification, and nutrient limitation (Bastos et al., 2022).
The biological and technological activities of β-glucan vary in relation to the molecular weight, types of bonds, degree of branching, and structural arrangement, which can impact β-glucan solubility (Du et al., 2019; Bastos et al., 2022). Generally, β-glucan is classified with respect to its solubility in aqueous solution, including water-soluble, alkali-soluble, and alkali-insoluble β-glucan (Bastos et al., 2022). In addition, its properties can be influenced by the disruption/extraction methods applied.
Cell disruption methods for β-glucan extraction
Cell rupture methods for β-glucan include mechanical and non-mechanical strategies usually applied as sole (Takalloo et al., 2020), combined (Tian et al., 2019), or in sequence (Zheng et al., 2019). The choice of the rupture method depends partly on the disruption effectiveness and efficiency (Jacob et al., 2019), and it considerably affects the yield, purity, physicochemical, and functional properties of the β-glucan obtained (Fu et al., 2022).
Generally, the methods used to β-glucan extraction from BSY are the same as those applied to disrupt and lysis yeast’s cells. Mechanical methods favor scalability and present low operational cost but are not selective and can result in fragments of β-glucan with low purity. Some examples include agitation with glass beads (also known as milling) (Avramia and Amariei, 2022), homogenization at high pressure (Tian et al., 2019; Dimopoulos et al., 2020), and ultrasound (Bzducha-Wróbel et al., 2014; Zheng et al., 2019; Dimopoulos et al., 2020). Non-mechanical methods, in turn, are mostly selective but limited regarding the potential for scale-up (Liu et al., 2016). These include pulsed electric field (Ganeva et al., 2020), alkaline/acid extraction (Bacha et al., 2017), hot water extraction (Bzducha-Wróbel et al., 2020), autolysis (Vieira et al., 2017), and hydrolysis with commercial enzymes (Marson et al., 2019). Emerging technologies involving ionic liquids are also reported to release β-glucan (Khanh et al., 2020).
Alkaline and alkaline-acid extractions are extensively applied to recover β-glucan—the first consists of using an alkaline solution combined with heating and time. For example, a typical alkaline extraction utilizes sodium hydroxide as a solvent at 90°C for 2 h (Bacha et al., 2017) or potassium hydroxide with different concentrations at room temperature for 2 h (Pinto et al., 2015). Parameters such as alkali concentration, extraction time, and temperature can be evaluated during β-glucan extractions (Varelas et al., 2016; Vaithanomsat et al., 2022). The extraction results in the precipitate (insoluble β-glucan) and supernatant fractions after a centrifugation step, where the last one is commonly mixed with ethanol to obtain soluble β-glucan. Sometimes the alkali method is followed by acid extraction (Pengkumsri et al., 2016; Dimopoulos et al., 2020; Mahmoud Amer et al., 2021), where the insoluble fraction is mixed with hydrochloric acid, acetic acid, and phosphoric acid (Krpan et al., 2010; Bzducha-Wróbel et al., 2020; Mahmoud Amer et al., 2021), among others.
Hot water extraction is another common method for β-glucan extraction that utilizes pressurized steam to release protein and some carbohydrates to the soluble phase while maintaining β-glucan in the solid phase. Some reports mention that hot water extraction occurs with a biomass suspension in water/buffer in a steam autoclave at 121°C and 1.1 atm for 1–5 h (Borchani et al., 2014; Bzducha-Wróbel et al., 2020). At the end of the process, phases are separated, and the sediment containing insoluble β-glucan is recovered; the liquid phase one can be added with ethanol to precipitate soluble β-glucan.
Among enzymatic approaches for cell wall disintegration, autolysis is an extensively applied treatment that utilizes endogenous enzymes in the yeast biomass and usually occurs between 50 and 60°C for 24–48 h (Pengkumsri et al., 2016; Bertolo et al., 2019; Jacob et al., 2019; Dimopoulos et al., 2020; Takalloo et al., 2020). It can be performed by adding some lytic promoters, such as inorganic salts (e.g., sodium chloride) (Bertolo et al., 2019; Jacob et al., 2019) and organic solvents (e.g., ethanol) (Takalloo et al., 2020), and optimized regarding operational parameters (e.g., temperature and autolysis time) (Vieira et al., 2017). Enzymatic hydrolysis, in turn, applies endo or exoproteases (Alcalase®, Flavourzyme®, Protamex®, Savinase®, Neutrase®) (Borchani et al., 2014; Lee et al., 2015; Marson et al., 2019; Vaithanomsat et al., 2022) to hydrolyze β-glucan-associated proteins, resulting in both β-glucan fraction and a protein-rich hydrolysate. Sometimes carbohydrases are applied to hydrolyze polysaccharides in the yeast cell wall, which could result in soluble β-glucan fragments (Zheng et al., 2019). In both cases, parameters, including enzyme amount, temperature, pH, time, and yeast biomass concentration, can be evaluated during β-glucan extraction (Marson et al., 2019; Zheng et al., 2019). Enzymatic procedures are completed by heating (above 80°C) the final medium to inactivate enzymes (Bertolo et al., 2019; Vaithanomsat et al., 2022), followed by a solid-liquid separation step (e.g., centrifugation).
Steps such as purification, chemical modification (for insoluble β-glucan), and polishing commonly occur after extraction (Pinto et al., 2015). The characterization of β-glucan in terms of structure and biological and technological properties is also recommended (Pinto et al., 2015; Bacha et al., 2017; Mahmoud Amer et al., 2021) to better knowledge for future applications.
Technological properties and applications of BSY β-glucan as an ingredient
The food industry is continuously looking for novel healthy ingredients to enhance the products’ nutritional and functional value and reduce production costs. Yeast β-glucans are already considered safe food ingredients by the European Food Safety Authority (EFSA. Scientific, 2011) and received the generally recognized as safe (GRAS) status from the United States Food and Drug Administration (FDA Services DoHH, 2008). In fact, several food products have been proposed with the addition of yeast β-glucans (Krpan et al., 2009) and this carbohydrate is commercially available for food supplementation (e.g., Wellmune®, Goldcell® or Yestimun®). In particular, the β-glucans extracted from the BSY have been used in feed applications as alternative diets or supplements for shrimps (Suphantharika et al., 2003) or pigs (Bo et al., 2020).
β-glucan can be used as an ingredient and potentiator for other ingredients promoting the characteristics of foods and beverages. β-glucan can also act as a substitute for components, e.g., fat, with an effect on the nutritional, techno-functional, and sensory properties; and provide thickening and assist in the emulsification, stabilization, and gelation of different foods, which include bakery, meat, and dairy products (Mykhalevych et al., 2022; Sengul and Ufuk, 2022). Additionally, β-glucans act as a water retainer and are a suitable fat replacer, having a good mouthfeel similar to fat (Thammakiti et al., 2004) and enabling the reduction or exclusion of fat levels in foods. This compound can change the sensory properties, viscosity, and rheology of added products, even in small concentrations (Sengul and Ufuk, 2022).
The technological potential of β-glucan in dairy products includes the increasing of overrun ice cream, binding of free moisture, milk fat mimetic, structure formation, and increasing the yield of cheeses, even if low concentrations of β-glucan in the formulations (in the range of 0.5–3.0%) (Mykhalevych et al., 2022). Furthermore, BSY β-glucan as a fat replacer in skim milk yogurt has already resulted in better rheological properties and physical stability (Mejri et al., 2014).
In bakery products, the inclusion of β-glucan can modify the expansion rate, reduce the volume, increase the firmness of the bread, promote an adverse effect on the gluten matrix, increase the water consumption of the dough due to the increase in the fibrous fraction, and increase the number of pores in bread, among other effects. Also, the incorporation of BSY β-glucan in bread preparations improved the nutritional/health-promoting properties of the product (Martins et al., 2018) and enhanced the quality and shelf life during the chilled storage (Suwannarong et al., 2020). Thus, it is essential to establish the consequences of adding β-glucan and evaluate its suitable concentration that results in less impact on the product and, whenever possible, to correlate with its biological properties (Andrzej et al., 2019).
In meat products, β-glucan can reduce fat content, acting as a substitute ingredient. The β-glucan also acts as an agent to minimize cooking losses of emulsions and increase viscosity and retention of moisture and fat due to its ability to create a three-dimensional network. Additionally, a reduction in textural parameters is verified, indicating the use of β-glucan in meat products with a softer texture, for example (Álvarez and Barbut, 2013).
BSY β-glucans can be incorporated in mayonnaises as a fat replacer, contributing to higher storage stability and a lower caloric value (Worrasinchai et al., 2006; Marinescu et al., 2011). It also exhibit a protective effect of lactobacilli during freeze-drying, refrigerated storage and exposure to simulated gastrointestinal conditions, which make them interesting additives for functional foods containing probiotics (Guedes et al., 2019).
Due to its characteristics, yeast β-glucan can be used as a substitute for traditional ingredients applied at an industrial scale, such as alginates, gum arabic, pectin, and carboxymethylcellulose, with similar or improved properties (Sengul and Ufuk, 2022). However, as yeast β-glucan is a new ingredient, recently applied at industrial scale in food products, it is essential to evaluate the impact of its incorporation in food matrices. The same applies to BSY, which industrial use is still limited but has shown a technological potential to be inserted into different food categories with distinct purposes, as discussed above.
Biological properties of BSY β-glucan
Several studies have reported the multi-bioactivity of yeast β-glucans, considering them as interesting compounds for food, pharmaceutical and biomedical applications (Geller et al., 2019; Caruso et al., 2022). Nevertheless, only a few numbers of works described the biological effect of β-glucans extracted from BSY as by-product of the brewing industry (Table 1).
BSY β-glucans positively affected the immunogenic activity in different animals. This bioactivity was demonstrated both in vitro and in vivo by increasing of some important immune index indicators, such as the phenoloxidase activity in black tiger shrimps (Penaeus monodon) fed with BSY β-glucan (Thanardkit et al., 2002; Suphantharika et al., 2003).
Furthermore, an increase in the number of haemocytes and in the antibacterial activity against the pathogen Vibrio harveyi was also achieved for the shrimps (Thanardkit et al., 2002). The immunogenic activity was also demonstrated in murine peritoneal macrophages after a carboxymethylation reaction to obtain soluble BSY β-glucan (Liepins et al., 2015). The carboxymethylated β-glucan was able to mediate the induction of tumor necrosis factor-alpha (TNF-α). The hypocholesterolemic activity of dried BSY, as well as the soluble and insoluble β-glucans isolated from it, was reported by Waszkiewicz-Robak and Bartnikowska (Waszkiewicz-Robak and Bartnikowska, 2009). In this study, 6 weeks of a supplemented diet containing dried BSY or BSY β-glucans positively affected the lipid metabolism in blood and liver of mice by reducing the cholesterol and triacylglycerols. The bioactivity of BSY β-glucan, namely the antioxidant potential, was also demonstrated in humans (Araújo et al., 2015). The oral administration of carboxymethylated BSY β-glucan promoted a significant reduction of malondialdehyde levels in healthy men, thus suggesting the positive action of this carbohydrate in preventing oxidative damage.
Overall, it is expected that the promising bioactivity results already obtained for BSY β-glucan could lead to additional studies where the specific structure of the extracted carbohydrates would be directly associated with their biological effect. These studies would greatly contribute to defining and establishing suitable methodologies for β-glucan extraction from BSY.
Conclusion and perspectives
BSY is an important source of β-glucan with already demonstrated biological and technological properties. For application in foods and beverages, β-glucan needs to be extracted, purified, and fully characterized to a better knowledge of its potential applications. The available scientific information is not enough to establish an exact interconnection between the extraction strategy, molecular structure, biological and techno-functional properties. Therefore, additional studies are needed to define suitable strategies for BSY valorization and β-glucan application.
Governmental disposal policies are needed to implement reuse practices in the beer industry, promoting the efficient conversion of by-products into value-added compounds (circular economy).
Author contributions
All authors listed have made a substantial, direct, and intellectual contribution to the work and approved it for publication.
Acknowledgments
The authors acknowledge the CNPq, CAPES, FAPEG, IF Goiano and UFRJ by support. SS acknowledges the Portuguese Foundation for Science and Technology (FCT) under the scope of the strategic funding of UIDB/04469/2020 unit, and by LABBELS—Associate Laboratory in Biotechnology, Bioengineering and Microelectromechanical Systems, LA/P/0029/2020.
Conflict of interest
The authors declare that the research was conducted in the absence of any commercial or financial relationships that could be construed as a potential conflict of interest.
Publisher’s note
All claims expressed in this article are solely those of the authors and do not necessarily represent those of their affiliated organizations, or those of the publisher, the editors and the reviewers. Any product that may be evaluated in this article, or claim that may be made by its manufacturer, is not guaranteed or endorsed by the publisher.
References
Álvarez, D., and Barbut, S. (2013). Effect of inulin, β-Glucan and their mixtures on emulsion stability, color and textural parameters of cooked meat batters. Meat Sci. 94 (3), 320–327. doi:10.1016/j.meatsci.2013.02.011
Andrzej, K. M., Małgorzata, M., Sabina, K., Horbańczuk, O. K., and Rodak, E. (2019). Application of rich in β-glucan flours and preparations in bread baked from frozen dough. Food Sci. Technol. Int. 26 (1), 53–64. doi:10.1177/1082013219865379
Araújo, V. BdS., De Melo, A. N. F., De Souza, N. T., Da Silva, V. M. B., Castro-Gomez, R. H., Silva, A. S., et al. (2015). Oral intake of carboxymethyl-glucan (CM-G) from yeast (Saccharomyces uvarum) reduces malondialdehyde levels in healthy men. Mol. (Basel, Switz. 20 (8), 14950–14958. doi:10.3390/molecules200814950
Avramia, I., and Amariei, S. A. (2022). A simple and efficient mechanical cell disruption method using glass beads to extract β-glucans from spent brewer’s yeast. Appl. Sci. 12 (2), 648. doi:10.3390/app12020648
Bacha, U., Nasir, M., Iqbal, S., and Anjum, A. A. (2017). Nutraceutical, anti-inflammatory, and immune modulatory effects of β-glucan isolated from yeast. Biomed. Res. Int. 2017, 8972678. doi:10.1155/2017/8972678
Bastos, R., Oliveira, P. G., Gaspar, V. M., Mano, J. F., Coimbra, M. A., and Coelho, E. (2022). Brewer's yeast polysaccharides — a review of their exquisite structural features and biomedical applications. Carbohydr. Polym. 277, 118826. doi:10.1016/j.carbpol.2021.118826
Bertolo, A. P., Biz, A. P., Kempka, A. P., Rigo, E., and Cavalheiro, D. (2019). Yeast (Saccharomyces cerevisiae): Evaluation of cellular disruption processes, chemical composition, functional properties and digestibility. J. Food Sci. Technol. 56 (8), 3697–3706. doi:10.1007/s13197-019-03833-3
Bo, H. X., Anh, H. T., Hao, P. X., Tuoi, P. T., and Luc, D. D. (2020). Effects of replacement of fish meal and soybean meal by brewers’ yeast extract on growth and feed conversion of Landrace x Yorkshire pigs. Livest. Res. Rural Dev. 32 (6).
Borchani, C., Fonteyn, F., Jamin, G., Paquot, M., Blecker, C., and Thonart, P. (2014). Enzymatic process for the fractionation of baker’s yeast cell wall (Saccharomyces cerevisiae). Food Chem. 163, 108–113. doi:10.1016/j.foodchem.2014.04.086
Bzducha-Wróbel, A., Błażejak, S., Kawarska, A., Stasiak-Różańska, L., Gientka, I., and Majewska, E. (2014). Evaluation of the efficiency of different disruption methods on yeast cell wall preparation for β-glucan isolation. Mol. (Basel, Switz. 19 (12), 20941–20961. doi:10.3390/molecules191220941
Bzducha-Wróbel, A., Koczoń, P., Błażejak, S., Kozera, J., and Kieliszek, M. (2020). Valorization of deproteinated potato juice water into β-glucan preparation of C. Utilis origin: Comparative study of preparations obtained by two isolation methods. Waste Biomass Valorization 11 (7), 3257–3271. doi:10.1007/s12649-019-00641-w
Caruso, M. A., Piermaria, J. A., Abraham, A. G., and Medrano, M. (2022). β-glucans obtained from beer spent yeasts as functional food grade additive: Focus on biological activity. Food Hydrocoll. 133, 107963. doi:10.1016/j.foodhyd.2022.107963
Dimopoulos, G., Tsantes, M., and Taoukis, P. (2020). Effect of high pressure homogenization on the production of yeast extract via autolysis and beta-glucan recovery. Innovative Food Sci. Emerg. Technol. 62, 102340. doi:10.1016/j.ifset.2020.102340
Du, B., Meenu, M., Liu, H., and Xu, B. (2019). A concise review on the molecular structure and function relationship of β-glucan. Int. J. Mol. Sci. 20 (16), 4032. doi:10.3390/ijms20164032
EFSA. Scientific (2011). Opinion on the safety of ‘yeast beta-glucans’ as a Novel Food ingredient. EFSA J. 9 (5), 1–22.
Farber, M., and Barth, R. (2019). Mastering brewing science: Quality and production. UK: Wiley, 592.
FDA (2008). in GRN No. 239 - bakers yeast beta-glucan. Editor Services DoHH (Silver Spring, MD (EUA): U.S. Food and Drug Administration). Available at: https://www.cfsanappsexternal.fda.gov/scripts/fdcc/?set=GRASNotices&id=239&sort=GRN_No&order=DESC&startrow=1&type=basic&search=beta%2Dglucan.
Fu, W., Zhao, G., and Liu, J. (2022). Effect of preparation methods on physiochemical and functional properties of yeast β-glucan. LWT 160, 113284. doi:10.1016/j.lwt.2022.113284
Ganeva, V., Angelova, B., Galutzov, B., Goltsev, V., and Zhiponova, M. (2020). Extraction of proteins and other intracellular bioactive compounds from baker’s yeasts by pulsed electric field treatment. Front. Bioeng. Biotechnol. 8, 552335. doi:10.3389/fbioe.2020.552335
Geller, A., Shrestha, R., and Yan, J. (2019). Yeast-derived β-glucan in cancer: Novel uses of a traditional therapeutic. Int. J. Mol. Sci. 20 (15), 3618. doi:10.3390/ijms20153618
Guedes, J. D. S., Pimentel, T. C., Diniz-Silva, H. T., Almeida, E. T. D. C., Tavares, J. F., Souza, E. L. D., et al. (2019). Protective effects of β-glucan extracted from spent brewer yeast during freeze-drying, storage and exposure to simulated gastrointestinal conditions of probiotic lactobacilli. LWT 116, 108496. doi:10.1016/j.lwt.2019.108496
Jacob, F. F., Striegel, L., Rychlik, M., Hutzler, M., and Methner, F-J. (2019). Yeast extract production using spent yeast from beer manufacture: Influence of industrially applicable disruption methods on selected substance groups with biotechnological relevance. Eur. Food Res. Technol. 245 (6), 1169–1182. doi:10.1007/s00217-019-03237-9
Jaeger, A., Arendt, E. K., Zannini, E., and Sahin, A. W. (2020). Brewer’s spent yeast (BSY), an underutilized brewing by-product. Fermentation 6 (4), 123. doi:10.3390/fermentation6040123
P. N. Khanh, N. D. Nhut, and N. M. Cuong (2020). New method for preparing purity β -D-glucans (beta-Glucan) from baker’s yeast (Saccharomyces cerevisiae). SSci. Tech. Dev. J. 23 (3), 673–678.
Krpan, V., Petravic-Tominac, V., Gospodaric, I., Sajli, L., Djaković, S., and Filipovic-Grcic, J. (2010). Characterization of beta-glucans isolated from brewer's yeast and dried by different methods. Food Technol. Biotechnol. 48, 189–197.
Krpan, V., Vlatka, P-T., Krbavčić, I., Slobodan, G., and Katarina, B. (2009). Potential application of yeast β-glucans in food industry. Agric. Conspec. Sci. (ACS) 74 (4), 277–282.
Lee, H. J., Son, H. S., Park, C., and Suh, H. J. (2015). Preparation of yeast hydrolysate enriched in cyclo-his-pro (CHP) by enzymatic hydrolysis and evaluation of its functionality. Prev. Nutr. Food Sci. 20 (4), 284–291. doi:10.3746/pnf.2015.20.4.284
Liepins, J., Kovačova, E., Shvirksts, K., Grube, M., Rapoport, A., and Kogan, G. (2015). Drying enhances immunoactivity of spent brewer's yeast cell wall β-d-glucans. J. Biotechnol. 206, 12–16. doi:10.1016/j.jbiotec.2015.03.024
Liu, D., Ding, L., Sun, J., Boussetta, N., and Vorobiev, E. (2016). Yeast cell disruption strategies for recovery of intracellular bio-active compounds — a review. Innovative Food Sci. Emerg. Technol. 36, 181–192. doi:10.1016/j.ifset.2016.06.017
Liu, Y., Wu, Q., Wu, X., Algharib, S. A., Gong, F., Hu, J., et al. (2021). Structure, preparation, modification, and bioactivities of β-glucan and mannan from yeast cell wall: A review. Int. J. Biol. Macromol. 173, 445–456. doi:10.1016/j.ijbiomac.2021.01.125
Mahmoud Amer, E., Saber, S. H., Abo Markeb, A., Elkhawaga, A. A., Mekhemer, I. M. A., Zohri, A. A., et al. (2021). Enhancement of β-glucan biological activity using a modified acid-base extraction method from Saccharomyces cerevisiae. Mol. (Basel, Switz. 26 (8), 2113. doi:10.3390/molecules26082113
Marinescu, G., Stoicescu, A., and Patrascu, L. (2011). The preparation of mayonnaise containing spent brewer’s yeast β- glucan as a fat replacer. Romanian Biotechnol. Lett. 16, 6017–6025.
Marson, G. V., de Castro, R. J. S., Belleville, M-P., and Hubinger, M. D. (2020). Spent brewer’s yeast as a source of high added value molecules: A systematic review on its characteristics, processing and potential applications. World J. Microbiol. Biotechnol. 36 (7), 95. doi:10.1007/s11274-020-02866-7
Marson, G. V., Machado, M. TdC., Castro, R. J. S., and Hubinger, M. D. (2019). Sequential hydrolysis of spent brewer's yeast improved its physico-chemical characteristics and antioxidant properties: A strategy to transform waste into added-value biomolecules. Process Biochem. 84, 91–102. doi:10.1016/j.procbio.2019.06.018
Martins, Z. E., Pinho, O., and Ferreira, I. (2018). Impact of new ingredients obtained from brewer's spent yeast on bread characteristics. J. Food Sci. Technol. 55 (5), 1966–1971. doi:10.1007/s13197-018-3107-0
Mejri, W., Bornaz, S., and Sahli, A. A. A. (2014). Formulation of non-fat yogurt with β-glucan from spent brewer's yeast. J. Hyg. Eng. Des. 8, 163–173.
Mohammadi, A., Razavi, S. H., Mousavi, S. M., and Rezaei, K. (2011). A comparison between sugar consumption and ethanol production in wort by immobilized Saccharomyces Cerevisiae, Saccharomyces Ludwigii and Saccharomyces Rouxii on Brewer's Spent Grain. Braz. J. Microbiol. 42, 605–615. doi:10.1590/S1517-838220110002000025
Mykhalevych, A., Polishchuk, G., Nassar, K., Osmak, T., and Buniowska-Olejnik, M. (2022). Beta-glucan as a techno-functional ingredient in dairy and milk-based products: A review. Mol. (Basel, Switz. 27 (19), 6313. doi:10.3390/molecules27196313
Nakashima, A., Yamada, K., Iwata, O., Sugimoto, R., Atsuji, K., Ogawa, T., et al. (2018). β-Glucan in foods and its physiological functions. J. Nutr. Sci. Vitaminol. 64 (1), 8–17. doi:10.3177/jnsv.64.8
Olajire, A. A. (2020). The brewing industry and environmental challenges. J. Clean. Prod. 256, 102817. doi:10.1016/j.jclepro.2012.03.003
Onofre, S., Bertoldo, I., Abatti, D., and Refosco, D. (2017). Chemical composition of the biomass of Saccharomyces cerevisiae - (meyen ex E. C. Hansen, 1883) yeast obtained from the beer manufacturing process. Int. J. Environ. Agric. Biotechnol. 2, 558–562. doi:10.22161/ijeab/2.2.2
Pengkumsri, N., Sivamaruthi, B., Sirilun, S., Peerajan, S., Kesika, P., Chaiyasut, K., et al. (2016). Extraction of β-glucan from Saccharomyces cerevisiae: Comparison of different extraction methods and in vivo assessment of immunomodulatory effect in mice. Food Sci. Technol. 37, 124–130. doi:10.1590/1678-457x.10716
Pinto, M., Coelho, E., Nunes, A., Brandão, T., and Coimbra, M. A. (2015). Valuation of brewers spent yeast polysaccharides: A structural characterization approach. Carbohydr. Polym. 116, 215–222. doi:10.1016/j.carbpol.2014.03.010
Sengul, M., and Ufuk, S. (2022). Therapeutic and functional properties of beta-glucan, and its effects on health. Eurasian J. Food Sci. Technol. 6 (1), 29–41.
Suphantharika, M., Khunrae, P., Thanardkit, P., and Verduyn, C. (2003). Preparation of spent brewer’s yeast β-glucans with a potential application as an immunostimulant for black tiger shrimp, Penaeus monodon. Bioresour. Technol. 88 (1), 55–60. doi:10.1016/s0960-8524(02)00257-2
Suwannarong, S., Wongsagonsup, R., and Suphantharika, M. (2020). Effect of spent brewer's yeast β-D-glucan on properties of wheat flour dough and bread during chilled storage. Int. J. Biol. Macromol. 156, 381–393. doi:10.1016/j.ijbiomac.2020.04.001
Takalloo, Z., Nikkhah, M., Nemati, R., Jalilian, N., and Sajedi, R. H. (2020). Autolysis, plasmolysis and enzymatic hydrolysis of baker's yeast (Saccharomyces cerevisiae): A comparative study. World J. Microbiol. Biotechnol. 36 (5), 68. doi:10.1007/s11274-020-02840-3
Teparić, R., Lozančić, M., and Mrša, V. (2020). Evolutionary overview of molecular interactions and enzymatic activities in the yeast cell walls. Int. J. Mol. Sci. 21 (23), 8996–9016. doi:10.3390/ijms21238996
Thammakiti, S., Suphantharika, M., Phaesuwan, T., and Verduyn, C. (2004). Preparation of spent brewer's yeast β-glucans for potential applications in the food industry. Int. J. Food Sci. Technol. 39 (1), 21–29. doi:10.1111/j.1365-2621.2004.00742.x
Thanardkit, P., Khunrae, P., Suphantharika, M., and Verduyn, C. (2002). Glucan from spent brewer's yeast: Preparation, analysis and use as a potential immunostimulant in shrimp feed. World J. Microbiol. Biotechnol. 18 (6), 527–539. doi:10.1023/a:1016322227535
Tian, X., Yang, P., and Jiang, W. (2019). Effect of alkali treatment combined with high pressure on extraction efficiency of β-d-Glucan from spent brewer’s yeast. Waste Biomass Valorization 10 (5), 1131–1140. doi:10.1007/s12649-017-0130-8
Vaithanomsat, P., Boonlum, N., Trakunjae, C., Apiwatanapiwat, W., Janchai, P., Boondaeng, A., et al. (2022). Functionality of yeast β-glucan recovered from kluyveromyces marxianus by alkaline and enzymatic processes. Polymers 14 (8), 1582. doi:10.3390/polym14081582
Varelas, V., Tataridis, P., Liouni, M., and Nerantzis, E. T. (2016). Valorization of winery spent yeast waste biomass as a new source for the production of β-glucan. Waste Biomass Valorization 7 (4), 807–817. doi:10.1007/s12649-016-9530-4
Vieira, E. F., Melo, A., and Ferreira, I. M. P. L. V. O. (2017). Autolysis of intracellular content of Brewer's spent yeast to maximize ACE-inhibitory and antioxidant activities. LWT - Food Sci. Technol. 82, 255–259. doi:10.1016/j.lwt.2017.04.046
Waszkiewicz-Robak, B., and Bartnikowska, E. (2009). Effects of spent brewer’s yeast and biological β-glucans on selected parameters of lipid metabolism in blood and liver in rats. J. Anim. Feed Sci. 18 (4), 699–708. doi:10.22358/jafs/66443/2009
Worrasinchai, S., Suphantharika, M., Pinjai, S., and Jamnong, P. (2006). β-Glucan prepared from spent brewer's yeast as a fat replacer in mayonnaise. Food Hydrocoll. 20 (1), 68–78. doi:10.1016/j.foodhyd.2005.03.005
Yang, W., and Huang, G. (2021). Extraction methods and activities of natural glucans. Trends Food Sci. Technol. 112, 50–57. doi:10.1016/j.tifs.2021.03.025
Keywords: polysaccharide, yeast cell wall, disruption methods, biological properties, technological properties
Citation: Gautério GV, Silvério SIDC, Egea MB and Lemes AC (2022) β-glucan from brewer’s spent yeast as a techno-functional food ingredient. Front. Food. Sci. Technol. 2:1074505. doi: 10.3389/frfst.2022.1074505
Received: 19 October 2022; Accepted: 22 November 2022;
Published: 01 December 2022.
Edited by:
Omer Said Toker, Yıldız Technical University, TurkeyReviewed by:
Bin Du, Hebei Normal University of Science and Technology, ChinaCopyright © 2022 Gautério, Silvério, Egea and Lemes. This is an open-access article distributed under the terms of the Creative Commons Attribution License (CC BY). The use, distribution or reproduction in other forums is permitted, provided the original author(s) and the copyright owner(s) are credited and that the original publication in this journal is cited, in accordance with accepted academic practice. No use, distribution or reproduction is permitted which does not comply with these terms.
*Correspondence: Ailton Cesar Lemes, ailtonlemes@eq.ufrj.br
†These authors have contributed equally to this work