- 1School of Food and Nutritional Sciences, University College Cork, Cork, Ireland
- 2Dairy Processing Technology Centre, University College Cork, Cork, Ireland
- 3Centre of Excellence in Agri-food Technologies, National Centre for Food Manufacturing, College of Sciences, University of Lincoln, Holbeach, United Kingdom
Milk protein concentrates (MPCs) are highly functional ingredients, with high-protein variants increasingly used in numerous applications. The objective of this study was to determine the impact of homogenisation, as part of the rehydration process, on solubility and heat stability of MPC. An 80% protein MPC powder was reconstituted (3% protein, w/v) and homogenised at 50°C using a pilot-scale, two-stage, valve homogeniser at different total pressures of 0, 5, 10, 15 and 20 MPa. Rehydrated samples were analysed for solubility, particle size, protein profile and heat stability (change in particle size distribution on heating in an oil bath at 140°C for 5 min). The results showed a considerable increase in solubility after applying homogenisation at 5 MPa. Homogenisation at pressures of 5–10 MPa reduced particle size of MPC dispersions further, with further increases in pressure having no additional effect. Increased heat stability was observed on increasing homogenisation pressures up to 10 MPa. This work demonstrates the positive impact of homogenisation on particle dispersion in MPCs and identifies a possible link between improved dispersion and heat stability. This would be applied as a strategy in dairy plants to reduce fouling in heat surfaces which suggests a significant economic impact in dairy processing.
1 Introduction
Milk protein concentrate (MPC) powders are obtained from skim milk by membrane processing (ultrafiltration, UF, with optional diafiltration, DF), evaporation and drying, containing all the caseins and whey proteins in the same ratio as the original skim milk (Martin et al., 2010). MPCs provide a protein-rich nutritional source of milk solids, and have found application in the production of many food products such as cheese, oral nutritional supplements and yoghurt (Patel and Patel, 2014). MPC powders are generally reconstituted and subjected to various heat treatments during processing, while a defect associated with high-protein MPCs is their poor rehydration properties (Mimouni et al., 2010b). The poor solubility of MPC powders has been attributed to the slow release of casein micelles from powder particles (Crowley et al., 2015).
MPC powder solubility can be aided by reconstituting the powder at high temperature of 50°C–60°C (Fang et al., 2011). This approach is widely used, but for cost reasons, lower reconstitution temperatures might be preferable.
Several strategies have been proposed and evaluated to produce high protein MPCs with improved solubility by applying physical treatments before drying, such as subjecting UF concentrate to high hydrostatic pressure (HHP) (Udabage et al., 2012), high shear mixing (Augustin et al., 2012), ultrasonication (McCarthy et al., 2014; Yanjun et al., 2014) and microfluidisation and high pressure homogenisation (Augustin et al., 2012). Although these approaches have shown some promise, the poor solubility of high-protein MPC powders remains a challenge. Moreover, most of these strategies have been applied to the MPC concentrates before spray drying; however, the study of approaches to improve solubility of finished powder during rehydration is more limited.
During manufacture of food products in which MPC ingredients are used, various heating treatments are applied and thus, MPC ingredients have to resist severe heat treatments. However, poor solubility, due to particle aggregates may result in poor heat stability during heating. Fouling on stainless steel surfaces during thermal processing is one of the consequences of poor heat stability of milk proteins. This can negatively impact production, affect product quality and increase production costs, as additional heating and pumping are needed to maintain the required temperature of the product and recover the drop in pressure during the process. Moreover, cleaning the deposit generated needs energy and substantial amount of water and chemicals (Goode, Asteriadou, Robbins, and Fryer, 2013). Gandhi et al. (2017) reported that poor solubility of MPC80, due to high storage temperature of the powder, resulted in high amounts of fouling. The fouling experiments in that study was conducted at 90°C on a plate heat exchanger however, there are no relevant studies using sterilisation temperatures.
Although several technologies have been applied to improve rehydration of MPC ingredients, including high-pressure homogenisation (Chandrapala et al., 2014), sonication (McCarthy et al., 2014) and hydrodynamic cavitation (HC) (Pathania et al., 2018), valve homogenisation as an established technology in the dairy industry have not been sufficiently studied. In valve homogenisation, a fluid under pressure is forced through a small valve, resulting in a large pressure gradient between the inlet and outlet of the valve (Grácia-Juliá et al., 2008) and intense shear forces are produced which have been suggested to be the primary force for decreasing globule size (Sandra & Dalgleish, 2005). Sikand et al. (2012) reported that reconstituting MPC powder in milk permeate at 37°C, followed by homogenisation at 13.8 MPa resulted in higher solubility (sample was deemed completely soluble using a centrifugation-based solubility assay). To the best of our knowledge, a study that focuses on linking between powder protein structure changes during valve-homogenisation, solubility on rehydration and heat stability (especially at sterilisation temperatures, i.e., UHT) has not been conducted so far.
In the present study, MPC80 powder dispersions were subjected to two-stage conventional homogenisation at different total homogenisation pressures (5, 10, 15 and 20 MPa), in a single pass, to investigate the effect of homogenisation pressure on protein structure, solubility and heat stability of the resultant dispersions. A range of physicochemical analyses including solubility, particle size distribution, protein profiling using gel electrophoresis and heat stability testing using an oil bath at 140°C were used to develop a more fundamental understanding of the effects of homogenisation on solubility and heat stability.
2 Materials and methods
2.1 Materials
Milk protein concentrate (MPC) was obtained from an Irish dairy ingredient manufacturer. The MPC contained 95.45 g dry solids per 100 g powder, and on a dry basis (w/w), 83.4% protein, 6.72% ash, 1.07% fat and 1.6% lactose, as given by the manufacturer. Hydrochloric acid (HCl) and sodium hydroxide (NaOH) were of analytical grade and were supplied by Sigma Chemical Co. (St Louis, MO, United States).
2.2 Rehydration of milk protein concentrate powders
MPC powder was reconstituted to 3% protein, w/v, in ultrapure water at 50°C for 20 min using a Silverson L5RT laboratory shear mixer operating at 5,000 rpm (Figure 1). The dispersions were stirred using a laboratory overhead stirrer at low speed with no vortex for 2 h, after which the pH was adjusted to pH 6.8 using 1 M NaOH or 1 M HCl and the dispersions were magnetically mixed under conditions described above for a further 2 h, after which pH was readjusted to pH 6.8, if required. The dispersions were then stored at 4°C overnight, with low speed magnetic stirring, to facilitate complete rehydration and equilibration of minerals. After overnight rehydration, the samples were equilibrated to 22°C for 2 h, after which the pH was checked and re-adjusted to pH 6.8, as required. MPC dispersions were then heated to 50°C in a water bath and subsequently homogenised using a two-stage, pilot-scale, valve homogeniser (APV GEA Niro-Soavi S. p.A., Parma, Italy). Samples were passed through the homogeniser with no pressure applied (0 MPa) or treated at various first and second stage pressure combinations (4:1, 8:2, 12:3 and 16:4 MPa) to give a total pressure of 5, 10, 15 and 20 MPa. The homogenisation conditions were applied to cover the normal practise in the dairy industry (20 MPa) and lower pressures to allow the choice of the lowest and most effective pressure. Samples were collected immediately after homogenisation and analysed for solubility and heat stability.
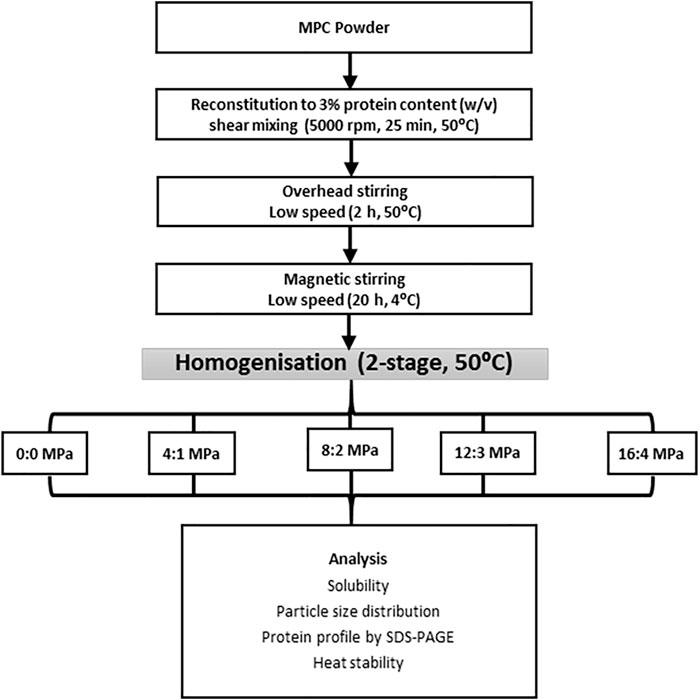
FIGURE 1. Flow diagram of reconstitution and homogenisation conditions as part of the rehydration process and subsequent analysis of milk protein concentrate dispersions.
2.3 Determination of solubility
The solubility of the MPC dispersions was determined using the method described by Sikand et al. (2012), with some modifications. After MPC dispersion preparation, each sample (3% w/v protein) was placed in a pre-weighed moisture dish. The weights of the moisture dishes containing the bulk dispersion samples were recorded, after which the dishes were placed in an oven for drying at 105°C over 3 h. After drying, dishes were placed in a desiccator for approximately 30 min, then weighed, and the total solids content was calculated. A subset of the remaining dispersion was centrifuged at 700 ×g for 10 min, after which the supernatant was removed for total solids content determination as described above. The solubility was calculated as follows:
2.4 Particle size distribution
Particle size distribution (PSD) of the MPC dispersions (before and after heating at 140°C × 5 min, as detailed in Section 2.6) was measured using a laser light diffraction unit (Mastersizer 3000 S, Malvern Instruments Ltd., Worcestershire, United Kingdom) equipped with a 300 RF (reverse Fourier) lens and He–Ne laser (λ of 633 nm) according to the method by Crowley et al. (2015). The samples were introduced to the dispersing unit using ultrapure water as dispersant to reach an obscuration of 14% (±1%). Analysis of PSD was performed using the generalised polydisperse model, with particle refractive index of 1.46, absorption of 0.1 and dispersant refractive index of 1.33. Duplicate measurements were carried out for each sample.
2.5 Electrophoretic protein profile analysis
Sodium dodecyl sulphate-polyacrylamide gel electrophoresis (SDS-PAGE) was performed under non-reducing and reducing conditions according to Laemmli (1970), with the following modifications: MPC dispersions (2 mg/ml) were incubated in a reducing sample buffer containing 0.3 M Tris-HCl (pH 6.8), 5% SDS, 50% glycerol and 100 mM dithiothreitol (Pierce, Rockford, IL, United States), before being heated at 95°C for 5 min and loading of protein into the wells of a pre-cast 4%–20% acrylamide, 10 × 10 cm, Tris-glycine protein gel system in an AcquaTank mini gel unit (Acquascience, Uckfield, United Kingdom). Following separation by electrophoresis at a constant voltage (160 V for 35–45 min), gels were removed from the electrophoresis unit, fixed, stained for protein (18 h at 22°C) with Coomassie brilliant blue R-250 and destained (O'Regan and Mulvihill, 2009).
2.6 Heat stability
The heat stability of the MPC dispersions was determined at 140°C (pH 6.8), using the heat coagulation time (HCT) assay first described by Davies and White (1966), with some modifications. Samples (2.5 ml) were filled into glass tubes (length, 130 mm; external diameter, 10 mm; wall thickness, 2 mm) and placed in an oil bath at 140°C for 5 min, with gentle rocking at a rate of ∼8 min−1. Samples were retrieved and visually assessed for stability; samples were categorized as being unstable if they displayed any visible flecks, flocs or particles of aggregated protein. Samples were also analysed for particle size distribution, as detailed in Section 2.4. Any increases in particle size after heating, compared with before heating, were also used as a measure of heat stability.
2.7 Statistical data analysis
All experiments were performed using the same batch of milk protein concentrate powder. Replicate measurements were carried out using freshly prepared MPC dispersions, with results presented as the means and standard deviations. One-way analysis of variance (one-way ANOVA) was carried out, followed by Tukey’s mean comparison test, to find the significance in the differences between the means using the R Commander program V.2.15.0 statistical analysis package (The R Foundation for Statistical Computing, Vienna, Austria). The level of significance was determined at p < 0.05.
3 Results
Investigating the solubility and heat stability of milk protein powders is of great interest as they are commonly used in research or industrial applications as substitute for fresh milk concentrates. The MPC sample prepared by means of shear mixing alone had relatively low solubility (87%; Figure 2) after overnight rehydration. The application of homogenisation increased MPC solubility (Figure 2). Solubility increased slightly from 87% in MPC sample reconstituted using high shear mixing to 88% after passing the sample through the homogeniser with no pressure applied (0 MPa), although this increase in solubility was not statistically significant. The use of homogenisation at 5 MPa resulted in a significant increase in solubility from 87% to 91%, with no further improvement in solubility on increasing homogenisation pressure.
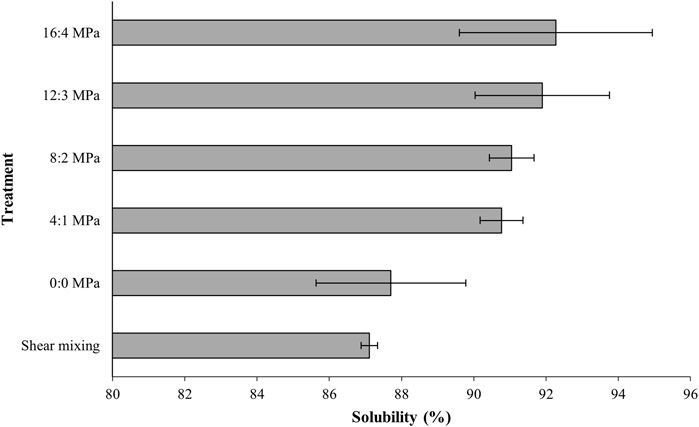
FIGURE 2. Solubility index (%) of milk protein concentrate dispersions with high shear mixing at 5,000 rpm and two-stage homogenisation at 0, 5, 10, 15 and 20 MPa total pressure, as part of the rehydration process.
Homogenization was conducted between 5 and 20 MPa to figure out the lowest necessary pressure to achieve a monomodal particle size distribution around casein micelle size. Changes in particle size distribution (PSD) in samples treated by high shear mixing and homogenisation are summarised in Table 1 and Figure 3. Analysis of the PSD of the MPC sample treated with high shear mixing showed a bimodal distribution with the first peak representing particles <1 µm and the second peak representing larger particles with mean particle size ∼50 µm (Figure 3A). Passing the MPC dispersions through the homogeniser with no pressure applied (0 MPa) significantly decreased the particle size, as shown in Table 1, but did not lead to complete elimination of the second peak (Figure 3B). In contrast, MPC dispersions subjected to homogenisation at 5 MPa had significantly (p < 0.05) smaller particle size and displayed a significant decrease in the second peak, indicating that originally undissolved powder particles were fragmented, dissociated and dissolved. Although the second peak in the PSD was almost completely eliminated when pressure greater than 10 MPa was applied (Figures 3C–F), no further significant decrease in particle size was observed (Table 1).
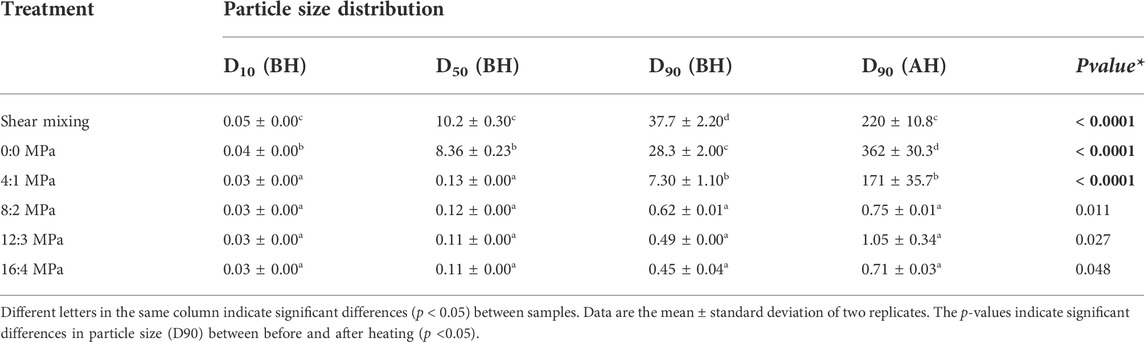
TABLE 1. Particle size distribution parameters for milk protein concentrate dispersions before (BH) and after (AH) heating, prepared with high shear mixing at 5,000 rpm followed by two-stage homogenisation at 0, 5, 10, 15 and 20 MPa, as part of the rehydration process.
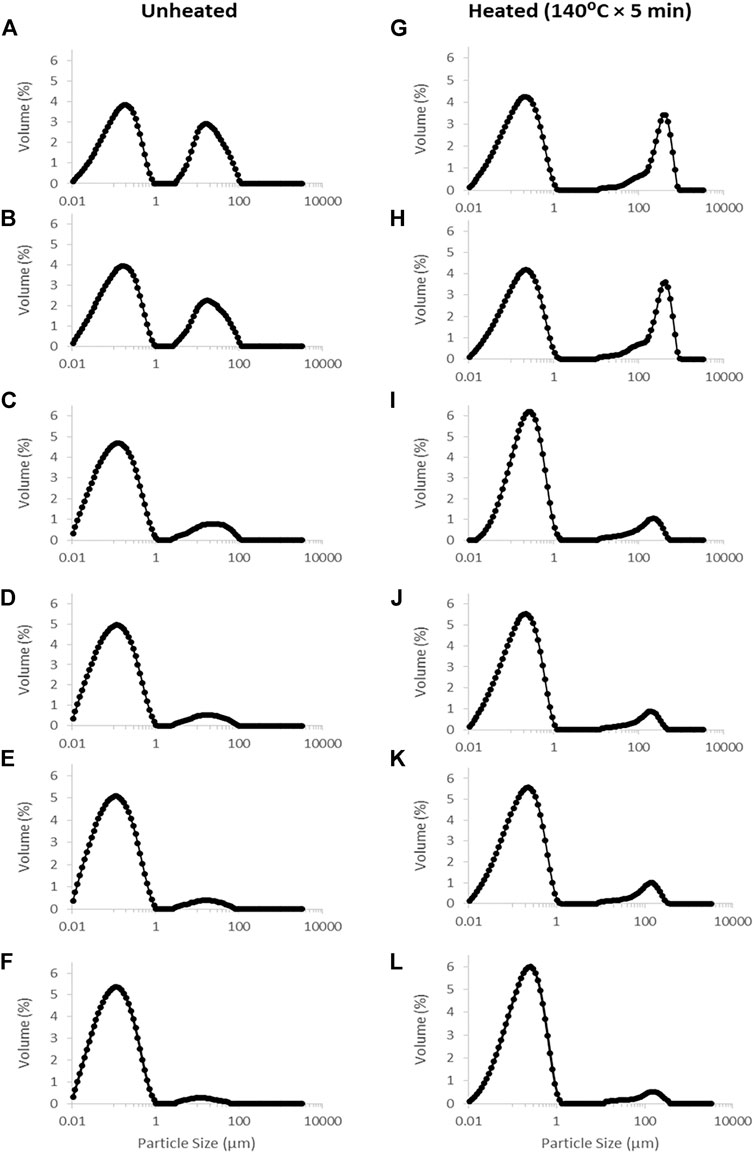
FIGURE 3. Particle size distribution before (A–F) and after (G–L) heating (140°C for 5 min) of milk protein concentrate dispersions with high shear mixing at 5,000 rpm (A,G) and 2-stage homogenisation at 0 (B,H), 5 (C,I), 10 (D,J), 15 (E,K) and 20 MPa (F,L), as part of the rehydration process.
The protein profile of the MPC dispersions prepared by high shear mixing and homogenisation treatment at different pressures (0–20 MPa) was performed using SDS-PAGE under non-reducing and reducing conditions to determine if homogenisation treatment influenced the types of protein-protein interactions (Figure 4). In non-reducing SDS-PAGE, the intensity of the major casein bands (αS- and β-CN) in the homogenised samples was slightly higher than those of the control, especially in samples homogenised at 15 and 20 MPa, while the band corresponding to κ-CN seemed to be absent under non-reducing conditions. Bands corresponding to the whey proteins (α-lactalbumin and β-lactoglobulin) showed the same intensity with no differences between control and treated samples.
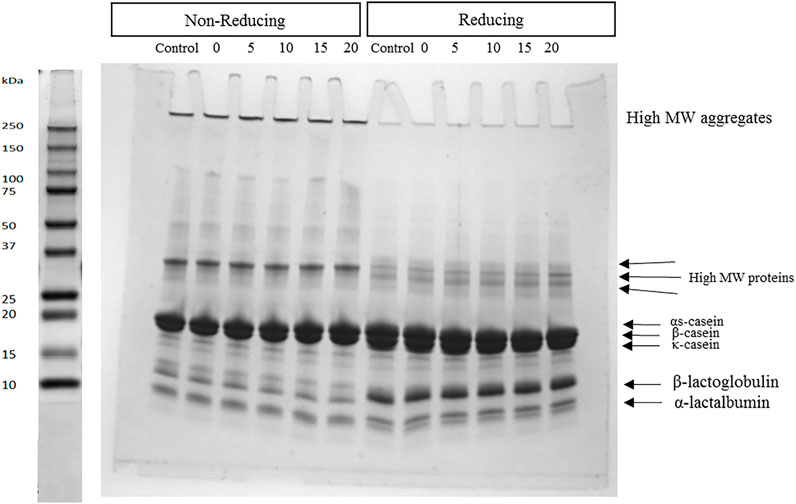
FIGURE 4. Sodium dodecyl sulfate-polyacrylamide gel electrophoresis under non-reducing and reducing conditions of milk protein concentrate samples prepared with high shear mixing at 5,000 rpm (control) and two-stage homogenisation at 0, 5, 10, 15 and 20 MPa total pressure, as part of the rehydration process and subsequent treatments.
Under non-reducing conditions, all samples showed the presence of high molecular weight aggregates (>200 kDa) with similar band intensities. As β-mercaptoethanol was applied as a reducing agent, the high MW aggregates were eliminated fully with no difference between control and homogenised samples. In addition, the intensity of whey protein bands increased in all samples, compared with non-reducing conditions. Moreover, the κ-CN band was evident under reducing conditions, and its intensity was highest in the control sample.
The heat stability of MPC dispersions before and after homogenisation was assessed by heating in an oil bath at 140°C for 5 min at pH 6.8. As a measure of heat stability, the PSD was measured in each sample after heating and compared with its unheated counterpart (Table 1 and Figure 3). After heating, the sample subjected to high shear mixing showed a bimodal distribution (i.e., similar to PSD before heating) but the second peak was shifted towards considerably larger particle size (200–300 µm), compared to before heating (50–60 μm; Figures 3A,G). Passing the MPC dispersion through the homogeniser with no pressure applied (0 MPa) resulted in a similar PSD to the control non-homogenised sample, but larger D90 value (droplet size in the 90% quantile of the distribution) (Table 1 and Figures 3B,H). Although applying homogenisation at 5 MPa resulted in a lower D90 value after heating, compared with the high shear mixing and 0 Mpa samples, the data demonstrate that using homogenisation at 5 MPa pressure was not sufficient to increase heat stability of MPC dispersions; and effect of homogenisation on heat stability only became apparent when the pressure was increased to 10 MPa. However, no further decrease in D90 value of heated samples was observed when pressures greater than 10 MPa were applied.
4 Discussion
The main problem with highly concentrated protein powders like MPC is their low solubility and insufficient dissolving ability (Carr and Golding, 2016). The results of the current study show poor solubility of MPC powder when rehydrated at room temperature using shear mixing, similar to what has been reported previously for MPC80 (Eshpari et al., 2014). This was reported by other researchers (Anema et al., 2006) to be due to poor dissolution of casein during rehydration, which many factors can affect including composition of the aqueous bulk solution, the processing conditions during powder manufacture (Felix da Silva et al., 2018), powder storage conditions (Barbosa-Cánovas et al., 2005), the particle structure (Gaiani et al., 2006) and the rehydration conditions (Gaiani et al., 2009). Mimouni et al. (2010a) and McKenna (2000) reported an interaction between casein micelles, caused by cross-linking of micelles by non-micellar proteins, which increases during powder storage resulting in a compact and closely packed micellar structure, which enhance the formation of poorly dispersible aggregates.
Applying shear mixing as only treatment to the dispersions was not effective in dissolving the powder particles and improving solubility. However, applying valve-homogenisation significantly improved solubility. It was reported that obtaining a particle size reduction and a monomodal distribution is difficult when shear mixers are used to rehydrate MPC powders (Chandrapala et al., 2014). The authors reported that high shear rotor stator systems (Ultraturrax) compared to low shear does not significantly downsize the particle size distribution (PSD) of MPC90 and micellar casein (MC). They also stated that, although high-pressure homogenisation (12 and 20 MPa) shifted the PSD towards casein micelle size, it was insufficient to achieve monomodally distributed particle sizes around ∼0.2 μm for MPC90 and MC, respectively. This also supports the results of PSD in the current study which have shown a decrease in the second peak, but not a complete elimination.
The increases in solubility of MPC dispersions after applying the homogenisation treatment may be a result of several different mechanisms, including, (1) induced phenomena such as shear, turbulence, cavitation and temperature increase (Bouaouina et al., 2006), (2) casein micelle disintegration, which increases solubility of calcium and phosphorus salts (Kielczewska et al., 2006) and/or (3) hydrogen and hydrophobic bond breakage by cavitation (Jambrak et al., 2009). SDS-PAGE (Figure 4) has shown that homogenisation acted on the disruption of the physical linkages between protein powder particles, without affecting the individual protein components or their interactions with each other. Similar results were also reported by other studies using high-pressure homogenisation (Chandrapala et al., 2014) and ultrasonication (Jambrak et al., 2009; Tang et al., 2009).
The results of the present study are in line with previous studies using homogenisation. In the study of Sikand et al. (2012), MPC80 dispersions reconstituted in milk permeate at 37°C and subsequently homogenised at 14 MPa showed the highest solubility (100%), which was attributed by the authors to decreases in particle size. High-pressure homogenisation (HPH) has also been used to improve solubility and other functional properties of proteins such as glycinin (Luo et al., 2010) and whey proteins (Dissanayake and Vasiljevic, 2009).
The rate, and indeed extent, of dairy powder solubilisation can be monitored by measuring reductions in particle size of protein dispersions (Bouvier et al., 2013). Other researchers (Crowley et al., 2015; McCarthy et al., 2014; Pathania et al., 2018) reported similar PSD profiles for MPC80 to what was found in the current study. Crowley et al. (2015) defined the two peaks as (1) casein micelles at <1 µm and (2) primary powder particles at ˃10 µm. This second peak could be associated with larger powder particles formed by noncovalent interactions during both concentration and drying processes (casein-casein and/or casein-whey protein interactions) forming aggregates (Zisu et al., 2010). Similar PSD profiles were reported by Chandrapala et al. (2014) for MPC90 using single stage homogenisation. The authors reported that using homogenisation resulted in extreme shear forces that caused nearly a complete destruction of the original particles (100 µm) prepared by low-speed overhead stirring to smaller particles of 1–10 µm diameter. The authors reported no additional decrease in particle size when the pressure applied was increased from 8 to 20 MPa, which is in line with the results of the present study.
The results indicated poor heat stability of MPC80 on heating which was reported to be due to their inherently high calcium ion activity, high protein content and poor solubility. Homogenization treatment resulted in an improvement in heat stability which might be attributed to the ability of homogenisation treatment to disrupt the physical linkages between powder particles, as seen in the SDS-PAGE (Figure 4). Similar results have been reported by Koh et al. (2014) on application of high-shear mixing, ultrasound and homogenisation to whey protein concentrate (WPC80) dispersions, which improved heat stability of the protein dispersions.
The present study showed an interaction between the solubility, PSD and heat stability of MPC80, as reported by Gandhi et al. (2017). The authors reported a high degree of fouling of MPC80 (which is directly associated with heat stability) as a consequence of a significant decrease in solubility on storage of the powders at high temperatures. In a study aimed at understanding the effects of pH adjustment prior to UF of skim milk on membrane performance and physical functionality of MPC, Luo et al. (2015) reported that solubility and heat stability followed the same trend as the pH of the feed changed.
In the present study, it is possible that the relationship between solubility and heat stability is due to the undissolved powder particles in the MPC acting as seeds or nuclei for further aggregation/coagulation. Adding aggregate seeds of a particular protein to a solution of monomers of the same protein enables efficient aggregation by exogenous seeding, thereby accelerating aggregation (Jarrett & Lansbury, 1993). Homogenisation may have increased heat stability of MPC dispersions, preventing further aggregation on heating in the oil bath through (1) increasing the negative surface charge (zeta potential) on proteins in MPC, increasing electrostatic repulsion between protein particles, or (2) formation of soluble protein aggregates by disrupting the hydrogen bonds and hydrophobic interactions by cavitation, inhibiting formation of further aggregates (Jiang et al., 2014) and improving heat stability (Ryan et al., 2013).
5 Conclusion
Homogenisation greatly increased the solubility and heat stability of milk protein concentrate dispersions, with heat stability increasing with increasing solubility. Applying pressures up to 10 MPa was sufficient to increase both solubility and heat stability; however, no further improvement were observed when higher pressures were applied. This work provides new insights into the use of homogenisation to modify the rehydration and heat stability of MPC ingredients. Future work needs to consider other parameters to gain more understanding of structural changes because of homogenisation such as surface charge using zeta potential and changes in rheological behaviour. This would also consider the fouling behaviour of the reconstituted powder at different heat regimes.
Data availability statement
The raw data supporting the conclusions of this article will be made available by the authors, without undue reservation.
Author contributions
EH: Data curation; formal analysis; investigation; methodology; writing—original draft, supervision. ML: Data curation; formal analysis; investigation. SC: investigation; methodology, supervision. JO: Conceptualization; funding acquisition; project administration; resources; supervision; validation; visualization.
Funding
This work was supported by the Irish State through funding from the Technology Centres programme (Grant Number TC/2014/0016).
Acknowledgments
The authors would like to acknowledge the Dairy Processing Technology Centre (DPTC), an Enterprise Ireland initiative, for financial support of, and permission to publish this work.
Conflict of interest
The authors declare that the research was conducted in the absence of any commercial or financial relationships that could be construed as a potential conflict of interest.
Publisher’s note
All claims expressed in this article are solely those of the authors and do not necessarily represent those of their affiliated organizations, or those of the publisher, the editors and the reviewers. Any product that may be evaluated in this article, or claim that may be made by its manufacturer, is not guaranteed or endorsed by the publisher.
References
Anema, S. G., Pinder, D. N., Hunter, R. J., and Hemar, Y. (2006). Effects of storage temperature on the solubility of milk protein concentrate (MPC85). Food Hydrocoll. 20 (2-3), 386–393. doi:10.1016/j.foodhyd.2005.03.015
Augustin, M. A., Sanguansri, P., Williams, R., and Andrews, H. (2012). High shear treatment of concentrates and drying conditions influence the solubility of milk protein concentrate powders. J. Dairy Res. 79, 459–468. doi:10.1017/S0022029912000489
Barbosa-Cánovas, G. V., Ortega-Rivas, E., Juliano, P., and Yan, H. (2005). Food powders: Physical properties, processing, and functionality, 86. New York: Kluwer Academic/Plenum Publishers, 71–75.
Bouaouina, H., Desraumax, A., Loisel, C., and Legrand, J. (2006). Functional properties of whey proteins as affected by dynamic high-pressure treatment. Int. Dairy J. 16, 275–284. doi:10.1016/j.idairyj.2005.05.004
Bouvier, J. M., Collado, M., Gardiner, D., Scott, M., and Schuck, P. (2013). Physical and rehydration properties of milk protein concentrates: Comparison of spray-dried and extrusion-porosified powders. Dairy Sci. Technol. 93, 387–399. doi:10.1007/s13594-012-0100-7
Carr, A., and Golding, M. (2016). “Functional milk proteins production and utilization: Casein-based ingredients,” in Advanced dairy chemistry. Editors P. McSweeney, and J. O'Mahony (New York, NY: Springer), 35–66. doi:10.1007/978-1-4939-2800-2_2
Chandrapala, J., Martin, G. J., Kentish, S. E., and Ashokkumar, M. (2014). Dissolution and reconstitution of casein micelle containing dairy powders by high shear using ultrasonic and physical methods. Ultrason. Sonochem. 21, 1658–1665. doi:10.1016/j.ultsonch.2014.04.006
Crowley, S. V., Desautel, B., Gazi, I., Kelly, A. L., Huppertz, T., and O’Mahony, J. A. (2015). Rehydration characteristics of milk protein concentrate powders. J. Food Eng. 149, 105–113. doi:10.1016/j.jfoodeng.2014.09.033
Davies, D. T., and White, J. C. D. (1966). The stability of milk protein to heat: I. Subjective measurement of heat stability of milk. J. Dairy Res. 33, 67–81. doi:10.1017/s0022029900011730
Dissanayake, M., and Vasiljevic, T. (2009). Functional properties of whey proteins affected by heat treatment and hydrodynamic high-pressure shearing. J. Dairy Sci. 92, 1387–1397. doi:10.3168/jds.2008-1791
Eshpari, H., Tong, P. S., and Corredig, M. (2014). Changes in the physical properties, solubility, and heat stability of milk protein concentrates prepared from partially acidified milk. J. Dairy Sci. 97, 7394–7401. doi:10.3168/jds.2014-8609
Fang, Y., Selomulya, C., Ainsworth, S., Palmer, M., and Chen, X. D. (2011). On quantifying the dissolution behaviour of milk protein concentrate. Food Hydrocoll. 25, 503–510. doi:10.1016/j.foodhyd.2010.07.030
Felix da Silva, D., Ahrné, L., Ipsen, R., and Hougaard, A. B. (2018). Casein‐based powders: Characteristics and rehydration properties. Compr. Rev. Food Sci. Food Saf. 17 (1), 240–254. doi:10.1111/1541-4337.12319
Gaiani, C., Ehrhardt, J. J., Scher, J., Hardy, J., Desobry, S., and Banon, S. (2006). Surface composition of dairy powders observed by X-ray photoelectron spectroscopy and effects on their rehydration properties. Colloids Surf. B Biointerfaces 49 (1), 71–78. doi:10.1016/j.colsurfb.2006.02.015
Gaiani, C., Scher, J., Schuck, P., Desobry, S., and Banon, S. (2009). Use of a turbidity sensor to determine dairy powder rehydration properties. Powder Technol. 190 (1-2), 2–5. doi:10.1016/j.powtec.2008.04.042
Gandhi, G., Amamcharla, J. K., and Boyle, D. (2017). Effect of milk protein concentrate (MPC80) quality on susceptibility to fouling during thermal processing. LWT - Food Sci. Technol. 81, 170–179. doi:10.1016/j.lwt.2017.03.063
Grácia-Juliá, A., René, M., Cortés-Muñoz, M., Picart, M., López-Pedemonte, T., Chevalier, D., et al. (2008). Effect of dynamic high pressure on whey protein aggregation: A comparison with the effect of continuous short-time thermal treatments. Food Hydrocoll. 22, 1014–1032. doi:10.1016/j.foodhyd.2007.05.017
Goode, K.R., Asteriadou, K., Robbins, P.T., and Fryer, P.J. (2013). Fouling and Cleaning Studies in the Food and Beverage Industry Classified by Cleaning Type. Comprehensive Reviews in Food Science and Food Safety 12, 121–143. doi:10.1111/1541-4337.12000
Jambrak, A. R., Lelas, V., Mason, T. J., Krešić, G., and Badanjak, M. (2009). Physical properties of ultrasound treated soy proteins. J. Food Eng. 93, 386–393. doi:10.1016/j.jfoodeng.2009.02.001
Jarrett, J. T., and Lansbury, P. T. (1993). Seeding "one-dimensional crystallization" of amyloid: A pathogenic mechanism in alzheimer's disease and scrapie? Cell 73, 1055–1058. doi:10.1016/0092-8674(93)90635-4
Jiang, L., Wang, J., Li, Y., Wang, Z., Liang, J., Wang, R., et al. (2014). Effects of ultrasound on the structure and physical properties of black bean protein isolates. Food Res. Int. 62, 595–601. doi:10.1016/j.foodres.2014.04.022
Koh, L. L. A., Chandrapala, J., Zisu, B., Martin, G. J. O., Kentish, S. E., and Ashokkumar, M. (2014). A comparison of the effectiveness of sonication, high shear mixing and homogenisation on improving the heat stability of whey protein solutions. Food bioproc. Tech. 7, 556–566. doi:10.1007/s11947-013-1072-1
Laemmli, U. K. (1970). Cleavage of structural proteins during the assembly of the head of bacteriophage T4. Nature 227, 680–685. doi:10.1038/227680a0
Luo, D., Zhao, Q., Zhao, M., Yang, B., Long, X., Ren, J., et al. (2010). Effects of limited proteolysis and high-pressure homogenisation on structural and functional characteristics of glycinin. Food Chem. 122, 25–30. doi:10.1016/j.foodchem.2010.02.011
Luo, X., Ramchandran, L., and Vasiljevic, T. (2015). Lower ultrafiltration temperature improves membrane performance and emulsifying properties of milk protein concentrates. Dairy Sci. Technol. 95, 15–31. doi:10.1007/s13594-014-0192-3
Martin, G. J. O., Williams, R. P. W., and Dunstan, D. E. (2010). Effect of manufacture and reconstitution of milk protein concentrate powder on the size and rennet gelation behaviour of casein micelles. Int. Dairy J. 20, 128–131. doi:10.1016/j.idairyj.2009.08.007
McCarthy, N. A., Kelly, P. M., Maher, P. G., and Fenelon, M. A. (2014). Dissolution of milk protein concentrate (MPC) powders by ultrasonication. J. Food Eng. 126, 142–148. doi:10.1016/j.jfoodeng.2013.11.002
McKenna, A. B. (2000). “Effect of processing and storage on the reconstitution properties of whole milk and ultrafiltered skim milk powders: Thesis presented in partial fulfilment of the requirement for the degree of doctor of philosophy in food technology,” (Palmerston North, New Zealand: Massey University). Doctoral dissertation.
Mimouni, A., Deeth, H. C., Whittaker, A. K., Gidley, M. J., and Bhandari, B. R. (2010a). Investigation of the microstructure of milk protein concentrate powders during rehydration: Alterations during storage. J. Dairy Sci. 93, 463–472. doi:10.3168/jds.2009-2369
Mimouni, A., Deeth, H. C., Whittaker, A. K., Gidley, M. J., and Bhandari, B. R. (2010b). Rehydration of high-protein-containing dairy powder: Slow-and fast-dissolving components and storage effects. Dairy Sci. Technol. 90 (2), 335–344. doi:10.1051/dst/2010002
O'Regan, J., and Mulvihill, D. M. (2009). Heat stability and freeze–thaw stability of oil-in-water emulsions stabilised by sodium caseinate–maltodextrin conjugates. Food Chem. 119, 182–190. doi:10.1016/j.foodchem.2009.06.019
Patel, H., and Patel, S. (2014) Milk protein concentrates: Manufacturing and applications US Dairy Export Council, 3–4.
Pathania, S., Ho, Q. T., Hogan, S. A., McCarthy, N., and Tobin, J. T. (2018). Applications of hydrodynamic cavitation for instant rehydration of high protein milk powders. J. Food Eng. 225, 18–25. doi:10.1016/j.jfoodeng.2018.01.005
Ryan, K. N., Zhong, Q., and Foegeding, E. A. (2013). Use of whey protein soluble aggregates for thermal stability - a hypothesis paper. J. Food Sci. 78, 1105–1115. doi:10.1111/1750-3841.12207
Sandra, S., and Dalgleish, D. G. (2005). Effects of ultra-high-pressure homogenization and heating on structural properties of casein micelles in reconstituted skim milk powder. Int. Dairy J. 15, 1095–1104. doi:10.1016/j.idairyj.2004.11.015
Sikand, V., Tong, P., Vink, S., and Walker, J. (2012). Effect of powder source and processing conditions on the solubility of milk protein concentrates 80. Milchwissenschaft 67, 300–303.
Tang, C. H., Wang, X. Y., Yang, X. Q., and Li, L. (2009). Formation of soluble aggregates from insoluble commercial soy protein isolate by means of ultrasonic treatment and their gelling properties. J. Food Eng. 92, 432–437. doi:10.1016/j.jfoodeng.2008.12.017
Udabage, P., Puvanenthiran, A., Yoo, J. A., Versteeg, C., and Augustin, M. A. (2012). Modified water solubility of milk protein concentrate powders through the application of static high pressure treatment fied water solubility of milk protein concentrate powders through the application of static high pressure treatment. J. Dairy Res. 79, 76–83. doi:10.1017/S0022029911000793
Yanjun, S., Jianhang, C., Shuwen, Z., Hongjuan, L., Jing, L., Lu, L., et al. (2014). Effect of power ultrasound pre-treatment on the physical and functional properties of reconstituted milk protein concentrate. J. Food Eng. 124, 11–18. doi:10.1016/j.jfoodeng.2013.09.013
Keywords: milk protein concentrate, solubility, heat stability, valve homogenisation, homogenisation pressure
Citation: Hebishy E, Le Berre M, Crowley SV and O’Mahony JA (2022) Two-stage valve homogenisation enhances particle dispersion in milk protein concentrates during reconstitution and reduces heat-induced particle aggregation in resultant dispersions. Front. Food. Sci. Technol. 2:1032373. doi: 10.3389/frfst.2022.1032373
Received: 30 August 2022; Accepted: 21 September 2022;
Published: 10 October 2022.
Edited by:
Filiz Icier, Ege University, TurkeyReviewed by:
Kaavya Rathnakumar, University of Wisconsin-Madison, United StatesJayani Chandrapala, RMIT University, Australia
Copyright © 2022 Hebishy, Le Berre, Crowley and O’Mahony. This is an open-access article distributed under the terms of the Creative Commons Attribution License (CC BY). The use, distribution or reproduction in other forums is permitted, provided the original author(s) and the copyright owner(s) are credited and that the original publication in this journal is cited, in accordance with accepted academic practice. No use, distribution or reproduction is permitted which does not comply with these terms.
*Correspondence: James A. O’Mahony, c2Eub21haG9ueUB1Y2MuaWU=