- 1Laboratory of Physical Chemistry of Colloids and Surfaces, Department of Chemistry, Faculty of Philosophy, Sciences and Letters at Ribeirão Preto, Universidade de São Paulo, São Paulo, Brazil
- 2Laboratory of Food Engineering, Department of Chemical Engineering, Escola Politécnica, Universidade de São Paulo, São Paulo, Brazil
- 3Food Research Center (FoRC/NAPAN), Universidade de São Paulo, São Paulo, Brazil
Polypropylene/babassu thermoplastic starch (PP/TPS, 70:30 w/w) blend sheets were prepared as an alternative material to use in disposable packaging, reducing the negative environmental impact of synthetic polymer. Three different carboxyl acids: palmitic (PP/TPS/P), citric (PP/TPS/C), and stearic (PP/TPS/S), were used as natural compatibilizing agents (NCA) and compared to the conventional synthetic maleic anhydride (PP/TPS/M), concerning mechanical, physical, crystallinity, and morphological properties of the blend sheets. The blend sheets without or with compatibilizing agents resulted in dark coloration, higher opacity, higher thickness, lower resistance to break, flexibility and rigidity, higher moisture, solubility in water, hydrophilicity, lower crystallinity, and more heterogeneous morphology in comparison to PP sheets. The presence of compatibilizing agents was observed to improve the mechanical and physical properties of the blend sheets, resulting in materials stronger and less hydrophilic. The citric acid was able to act similarly to the synthetic maleic anhydride, resulting in blend sheets with similar mechanical and physical properties. Finally, this work shows that the natural compatibilizing agent citric acid was analogous to the commercial one, synthetic maleic anhydride, bringing a more sustainable alternative. In addition, this work allowed assessing a new source of starch and offering one more alternative for applying this source to the plastic packaging sector.
1 Introduction
The growing use of plastic packaging based on synthetic polymers has created serious environmental concerns due to its nonbiodegradability and recycling difficulties. This scenario results in the accumulation of plastic risk in landfills as well as the marine environment, adversely affecting both ecosystems (Palai et al., 2019). Thus, there is a growing number of investigations on the development and use of new alternatives for minimizing the environmental impact caused by the use of synthetic packaging materials (Din et al., 2020). These problems can be greatly avoided by effectively managing and recycling plastic waste, as well as by seeking alternative sources of raw materials, particularly natural and renewable sources, such as starch to produce biodegradable plastics (Martins et al., 2021). Starch is a renewable, inexpensive and naturally biodegradable polymer; however, native starch itself does not show thermoplastic behavior. The addition and exposition of plasticizers to thermomechanical energy lead to disruption of the semicrystalline structure, resulting in thermoplastic starch (TPS), which is an amorphous material. The properties of TPS allow the use of traditional processing conditions (blowing, extrusion, and injection molding) (do Val Siqueira et al., 2021).
Several studies on TPS have been widely and globally conducted for various starch sources, such as cassava, potato, and corn (Diyana et al., 2021). However, the use of these starch sources for producing plastics would imply competition with other crucial sectors, such as food. Thus, the search for other starchy sources represents an interesting alternative, mainly in terms of cost and availability.
Babassu is a new source of starch that has been investigated and identified as having a high potential for application in the packaging sector (Maniglia et al., 2017; Alves Lopes et al., 2020; Saraiva Rodrigues et al., 2020). Babassu palm (Orbignya sp.) is native to Brazil, Guyana, Suriname, and Bolivia. A valuable natural resource in the North and Northeast regions of Brazil, it produces fruit, which consists of four parts: epicarp (11%), mesocarp (23%), endocarp (59%), and almond (7%) (do Nascimento et al., 2019). Babassu mesocarp is a portion rich in starch (50%–70%) and is commonly consumed as flour for humans and also added to animal feed preparations (Maniglia and Tapia-Blácido, 2016; Alves Lopes et al., 2020).
In general, TPS shows a very hydrophilic character with limited performance. This characteristic leads to low processability, dimensional stability, and mechanical properties of the final products. Thus, the production of blends from the combination of TPS with petroleum-based polymers is an economic and ecological alternative that can result in plastic with competitive properties, facilitate production and reduce operating costs when compared to the development of new polymerization processes, and even bring partial biodegradability (do Val Siqueira et al., 2021; Jacob et al., 2020).
Among synthetic polymers, polypropylene (PP) is a petroleum-derived polymer most used due to its great versatility, because it is easy to manufacture and can be used in rigid or flexible packaging, has a good barrier against gases, chemical resistance, and low density (Zaferani, 2018). However, this polymer has the disadvantage that it is not biodegradable, which makes the formation of blends with biodegradable materials interesting. In this way, the new material can show partial biodegradability while maintaining the good mechanical and functional properties of the synthetic polymers. However, an obstacle in the formulation of blends with these materials is that thermoplastic starches (TPS) have a hydrophilic nature, while synthetic polymers generally present hydrophobic characteristics, which represent considerable immiscibility (Raee et al., 2020). To improve the compatibility of these materials, providing a consequent improvement in physical and mechanical properties, the use of compatibilizing agents has been used (Martins and Santana, 2016; Shirai et al., 2018; Martins et al., 2021).
Compatibilizing agents are capable of reducing the interfacial energy between the polar starch and the nonpolar synthetic polymer. Currently, functionalized polyolefins have been the most used as a compatibilizing agent, giving prominence to maleic anhydride (Taguet et al., 2014; Aksit et al., 2020; Lin et al., 2020; Chauhan et al., 2021). However, synthetic agents, such as maleic anhydride, are expensive and come from petroleum, which is a non-renewable source and is difficult to manufacture (Cerclé et al., 2013).
In search of new alternatives, compatibilizing agents (CA) from renewable sources have been increasingly studied. In the literature, carboxylic acids, such as citric, stearic, and palmitic, have been shown to be a good option to replace synthetic agents due to the compatibility of their chemical structure, high availability, and biodegradability. These acids (structural and empirical formulas in Figure 1) have a polar part (carboxyl) and a nonpolar one (carbon chain), being able to interact with all the polymers present (Shirai et al., 2018; Martins et al., 2021).
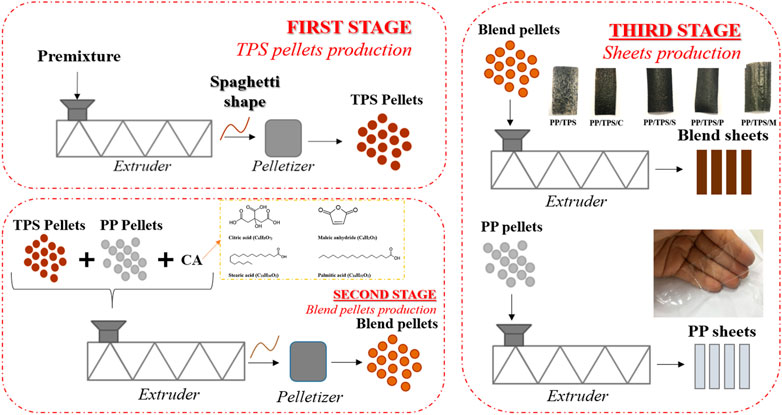
FIGURE 1. Flow chart of TPS pellets (first stage), blend pellets based on TPS, PP, and compatibilizing agent—CA (second stage), and blend or PP sheets (third stage). Pictures of the PP and blend sheets elaborated without (PP/TPS) and with compatibilizing agents (PP/TPS/C, PP/TPS/S, PP/TPS/P, and PP/TPS/M). PP: polypropylene sheets, PP/TPS: blend sheets based on polypropylene and babassu thermoplastic starch without compatibilizing agent, PP/TPS/C: blend sheets based on polypropylene and babassu thermoplastic starch with citric acid as compatibilizing agent, PP/TPS/S: blend sheets based on polypropylene and babassu thermoplastic starch with stearic acid as compatibilizing agent, PP/TPS/P: blend sheets based on polypropylene and babassu thermoplastic starch with palmitic acid as compatibilizing agent, and PP/TPS/M: blend sheets based on polypropylene and babassu thermoplastic starch with maleic anhydride as compatibilizing agent.
This work aimed to evaluate: 1) the potential of babassu starch in the production of TPS for elaborating blend sheets with polypropylene; 2) the effect on crystallinity, mechanical, physical, and morphological properties of the blend sheets in the presence of different carboxylic acids as natural compatibilizing agents, and 3) as a sustainable alternative to the synthetic maleic anhydride, currently used commercially. Thus, this work allows the appreciation of an alternative source of starch as one additional alternative to apply this source to the plastic packaging sector.
2 Material and methods
2.1 Material
The residue of babassu oil extraction, babassu mesocarp, was supplied by Florestas Brasileiras (Itapecuru-Mirim-Maranhão, Brazil). Palmitic acid and maleic anhydride were purchased from Dynamics—Química Contemporânea (São Paulo, Brazil). Citric acid, stearic acid, and glycerol were purchased from Synth (Diadema, Brazil). Polypropylene B0246 pellets were supplied by PoliBrasil (Piracicaba, Brazil).
2.2 Babassu starch isolation
The babassu starch was isolated from the babassu mesocarp following the alkaline method described by Maniglia et al. (2017). Briefly, the babassu oil extraction residue was soaked in NaOH solution at 0.25 g/100 ml, in a 1:2 ratio (pH 10.0). The resulting mixture was left to stand at 5°C for 18 h. The extracted mixture was then milled for 2 min in a food processor (Wallita) operating at maximum power. The milled material was filtered through 80-mesh stainless steel sieves. The material retained in the sieves was reprocessed and sieved again four additional times. The resulting liquid was centrifuged at 1,500 × g at ambient temperature (25 ± 2°C) for 10 min. The supernatant was discarded, and the precipitate was resuspended in water, neutralized with 1 M HCl until pH 7.0, and centrifuged again, twice. The fraction resulting from the centrifugation procedure was dried in an air-circulating oven at 40°C for 6 h. The dry starch was milled and sieved through 80-mesh sieves. The babassu starch was stored in sealed dark flasks at 5°C. The babassu oil extraction residue and the isolated babassu starch had the centesimal composition characterized by AOAC methods (AOAC, 2005). The apparent amylose content was analyzed using the simplified iodine colorimetric method, according to the methodology proposed by Juliano (Juliano, 1971) and adapted by Martínez and Cuevas (Martínez and Cuevas, 1989).
2.3 Extrusion process
Three processing stages were conducted in the extruder: production of the TPS pellets, production of the blend pellets, and production of the sheets (PP and blend) (Figure 1).
2.3.1 First stage: Production of thermoplastic starch pellets
Pellets of babassu thermoplastic starch (TPS) were prepared using premixtures with the following proportion of babassu starch: glycerol: Milli-Q water (60:25:15 g/100 g) in a mixer (Philips Walita Daily RI7000/41, Brazil) at speed 2 (80 rpm), according to previous work developed by our research group (Vedove et al., 2021). After that, they were stored at 10°C, in thermoset plastic bags.
The premixes were submitted to extrusion, with a feed flow of 0.3 kg/h, through a twin-screw feeder (Volumetric Minitwin Process 11, Brabender, Germany). The extruder used was a co-rotating twin-screw (Process 11, ThermoElectron, Germany), with the following characteristics: 11-mm bolt diameter and L/D 40, eight heating zones set at a temperature profile (80, 90, 90, 100, 110, 110, 105, and 100) °C, an output matrix (circular die) of 3 mm and speed of the threads of 80 rpm. From the extruded material (spaghetti shape), 2 mm long pellets were produced using pelletizer equipment (Varicut Pelletizer, 16 mm, ThermoScientific, Germany).
2.3.2 Second stage: Production of blend pellets
Blend pellets were produced in the proportion of 70:30 w/w (PP:TPS) without or with compatibilizing agents following Martins and Santana (2016) (14). Different types of natural compatibilizing agents (NCA) were evaluated, citric acid, stearic acid, and palmitic acid, in comparison to maleic anhydride, which is synthetic. Compatibilizing agents were used in a fixed amount of 3 g/100 g(PP+TPS) in all cases throughout the study. As a control, the blend pellets were prepared without the presence of the compatibilizing agent. The pellets were fed to the same extruder from a conical feeder (Conic Brabender, Germany), with a feed flow of 0.4 kg/h. The extrusion parameters were the same, except for the temperature profile from feed (Palai et al., 2019) to the eighth heating zone, which was (160, 165, 165, 170, 170, 170, 180, 180) °C. From the extruded material (spaghetti shape), 2 mm long pellets were produced using pelletizer equipment (Varicut Pelletizer, 16 mm, ThermoScientific, Germany).
2.3.3 Third stage: Polypropylene and blends sheets production
The blend sheets were produced from the extrusion of the pellets produced in the second stage. The PP sheets were produced from the extrusion of the PP pellets. The pellets were submitted to extrusion at the same parameters described for the production of the blend pellets with the sheet-forming mold die at 185°C. PP sheets produced using PP pellets were produced in the same conditions as in the blend sheets production to be used as a control sample. Table 1 shows the symbols adopted for the respective compositions of the PP and blend sheets produced in this work.
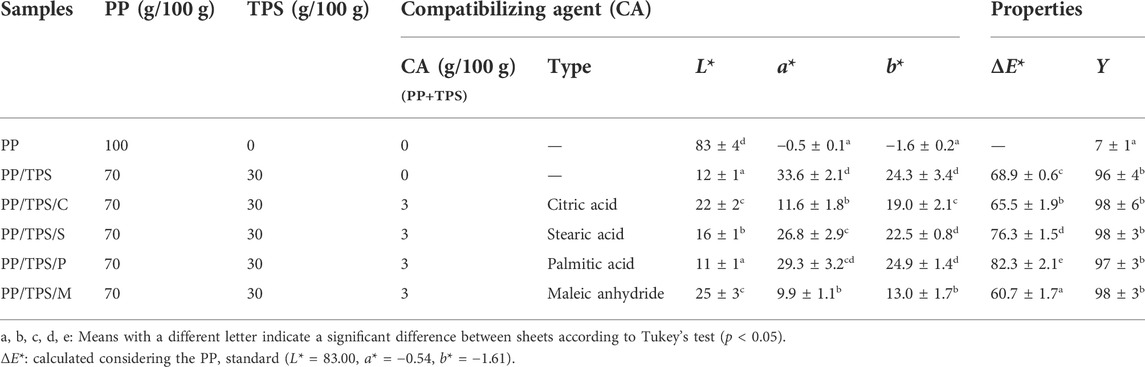
TABLE 1. Symbols and composition, luminosity (L*), color parameters (a* and b*), the total color difference (ΔE*), and opacity (Y) of the PP and blend sheets elaborated without (PP/TPS) and with compatibilizing agents (PP/TPS/C, PP/TPS/S, PP/TPS/P, and PP/TPS/M).
2.4 Sheets characterization
All PP and blend sheets were conditioned (75% RH and 25°C) for at least 48 h to ensure standardization of the final relative humidity of the sheets before characterization analyses. The high relative humidity used during conditioning was chosen to evaluate the performance of the sheets in a typical tropical climate condition (Souza et al., 2012).
2.4.1 Thickness
The thickness was measured in 10 random positions of each specimen, using a Zaas-Precision digital micrometer (1 μm resolution).
2.4.2 Mechanical properties
Mechanical properties were obtained using a texture analyzer (TAXT2i Stable Micro Systems, United Kingdom) with a load cell of 294 N, with the A/TGT self-tightening roller grips fixture, following the ASTM Standard method D882-12 (2018) (ASTM Standard D882-12, 2018). Sample sheets were cut into 1.0-cm-wide strips with a length of at least 10 cm. The initial grip separation and the crosshead speed were 50 and 1.0 mm s−1, respectively. Using Texture Expert software v.1.22 (SMS), the tensile strength (TS) [MPa], the elongation at break (E) [%], and Young’s modulus (YM) were determined. Ten strips (100 mm × 10 mm) were evaluated for each formulation, and only those sheets that were broken far from the grips were considered for the results.
2.4.3 Physical properties
The solubility in water, moisture content, and wettability of PP and blend sheets were analyzed in triplicate. The solubility in water was determined as the percentage of dry matter of the solubilized samples (discs 20 mm in diameter) after immersion in water (50 ml) at 25 ± 2°C for 24 h, under mechanical stirring (Gontard et al., 1992). The moisture was determined according to the ASTM Standard method D644– (ASTM Standard D644-07, 2007). The wettability of the sheets was analyzed according to ASTM Standard Methods D7334–08 (2013) (ASTM Standard D 7334-08, 2013) using an OCA15 -Dataphysiscs (OCA 15, Dataphysiscs, Germany). Waterdrop (surface tension = 72.7 mN/m) images were taken every 3 s, at ambient temperature. The contact angle was determined using the SCA-20U image processing software and six angle measurements were obtained for each formulation.
2.4.4 Morphology
The morphological characteristics of PP and the blend sheets were obtained by scanning electron microscopy (SEM) (FEI, QUANTA FEG 650—United States) under an accelerating voltage (5 kV), and magnification of ×200. After being frozen using liquid nitrogen, the blend sheets were mounted on aluminum stubs and coated with platin in a sputtering system (Bal-Tec MED 020, Coating System, Netherlands).
2.4.5 Crystallinity of the blend sheets by X-ray diffraction
An X-ray diffractometer PANalytical (model X'Pert PRO, United Kingdom, with detector X’Celerator) was used to measure the crystallinity of the blend sheets, under the following operating conditions: Cu radiation, 5 mA, 40 kV, scanning speed of 0.02°/s, time/step of 200 s and angle 2θ ranging from (2–40) ° (2θ). The relative crystallinity (RC) (%) of the blend sheets was quantitatively estimated using Origin 8.1 software (OriginLab Corporation, United States), according to Nara and Komiya (Nara and Komiya, 1983).
2.4.6 Opacity and color
The color parameters of the blend sheets were determined with a spectrophotometer (Color Quest XE, HunterLab, United States), a standard illuminant, and observer D65 and 10°, respectively (ASTM Standard D7251, 2011). The blend sheets were placed on the surface of the standard white plate and the parameters L* (lightness index), a* (color shades from red to green), and b* (color shades from yellow to blue) were determined. The results are expressed as the average of five measurements at different parts of the blend sheets surface. The total color difference (ΔE*) was calculated based on Eq. 1:
wherein ∆L*, ∆a*, and ∆b* are the differences between the parameters L*, a*, and b* of the blend sheets and the standard PP sheet (L* = 83.00, a* = −0.54, and b* = −1.61, respectively).
The opacity was measured according to the Hunterlab method. The opacity (Y) was calculated according to
2.5 Statistical analyses
The differences between the materials analyzed were evaluated using the analysis of variance (ANOVA) and Tukey’s test (5% significance level) performed using the Statgraphics Centurion XVI software.
3 Results and discussion
3.1 Babassu starch isolation
The centesimal composition of the residue of babassu oil extraction was 10.37 ± 0.05 g of moisture (w.b.), 1.25 ± 0.02 g of lipids, 2.36 ± 0.11 g of protein, 2.93 ± 0.03 g of ash, 29.97 ± 1.65 g of fibers, and 63.49 ± 1.32 g of starch (d.b.). While, the babassu starch was 9.36 ± 0.19 g of moisture (w.b.), 0.33 ± 0.02 g of lipids, 1.07 ± 0.11 g of protein, 1.22 ± 0.03 g of ash, 5.53 ± 0.09 g of fibers, and 91.85 ± 0.15 g of starch (d.b.). We can observe that the method of babassu starch isolation was effective, since the contents of lipids, proteins, and fibers were reduced by the presence of an alkaline medium that, if present, can cause hydrolysis in these materials. The apparent amylose content of the babassu starch was 21.90 ± 0.34 g of/100 g starch (d.b.), which is slightly lower (24.9 g/100 g) than the apparent amylose content of babassu starch isolated by the alkaline method reported by Maniglia et al. (Maniglia and Tapia-Blácido, 2016).
3.2 Visual aspect, color and opacity
Figure 1 also shows pictures of the PP, blend sheets elaborated without (PP/TPS) and with the compatibilizing agents (PP/TPS/C, PP/TPS/S, PP/TPS/P, and PP/TPS/M). The PP sheets showed greater malleability, visible homogeneity, and high transparency. The blend sheets made with PP/TPS without compatibilizing agents showed a heterogeneous matrix with the presence of distinct phases (TPS agglomerates and very transparent zones, characteristic of the PP sheet), indicating a lack of compatibility between the materials involved. The presence of compatibilizing agents allowed for good dispersion of TPS in the matrix. In general, all blend sheets had a much more rigid structure and a very dark coloration compared to PP sheets. According to Maniglia et al. (Maniglia and Tapia-Blácido, 2016), babassu starch shows the residual presence of phenolic compounds (8.2 mg/100 g starch); therefore, the high temperature of the extrusion process may have promoted the oxidation of these compounds, resulting in darker material. In addition, the color of the sheets can be associated with the Maillard reaction, which involves the formation of protein-carbohydrate conjugates between the carbonyl group of a reducing carbohydrate and an available amino group in the protein, leading to a Schiff base with water release (Arora et al., 2020). In addition, carbohydrates can suffer the caramelization process, leading to brown pigments (Martins et al., 2021).
Table 1 also shows the luminosity (L*), color parameters (a* and b*), the total color difference (ΔE*), and opacity of the PP and blend sheets elaborated without (PP/TPS) and with compatibilizing agents (PP/TPS/C, PP/TPS/S, PP/TPS/P, and PP/TPS/M). As can be seen in Table 1, the lightness (L* from 0 (black) to 100 (white)) was higher for the PP/TPS/C and PP/TPS/M sheets indicating that the compatibilizing agents prevented the staining and firing of the starch, giving rise to lighter materials with less evidence of thermomechanical degradation. Concerning the color parameters a* and b*, PP/TPS/P showed the highest value, and PP/TPS/M had the lowest values among the sheets with compatibilizing agents. These findings indicate that the starch granules are protected by to polymer matrix due the better compatibilization, avoiding their degradation, and consequently giving rise to less reddish and yellowish blends (a* and b*, respectively).
As already mentioned, for determining the ΔE*, the color parameters of the PP sheet were considered standard. Therefore, the higher the ΔE* value of the blend sheets, the more pronounced the color difference of the material to PP. PP/TPS showed the lowest ΔE*, mainly due to its high heterogeneity with some parts composed only of PP and others with agglomerated TPS. The blend sheets PP/TPS/S and PP/TPS/P showed the highest ΔE* values. Concerning the high ΔE* values, as already explained, it can be associated with the oxidation of the phenolic groups, Maillard reaction, or caramelization processes of the TPS babassu due to, mainly, the heating involved in the extrusion process. In addition, it was noticed that the presence of the compatibilizing agents affected the color of the blend sheets. PP/TPS/C and PP/TPS/M were the lesser colored blend sheets, which may be associated with the fact that these compatibilizing agents, citric acid and maleic anhydride, can act as better thermal protectors, reducing the degradation of the compounds. Again, the thermal protection mechanism of the compatibilizing agents can be associated with the protection of the starch granules by the polymeric matrix, avoiding their degradation (Martins and Santana, 2016).
The blend sheets without or with compatibilizing agents showed much higher opacity (∼98%) than PP sheets, which indicates that they would not be suitable for storing products that need to be seen inside the packaging for consumer choice. However, these blend sheets are interesting for helping preserve food from light oxidation.
3.3 Mechanical properties
Figure 2 shows the thickness and the mechanical properties of the PP and blend sheets without (PP/TPS) and with compatibilizing agents (PP/TPS/C, PP/TPS/S, PP/TPS/P, and PP/TPS/M).
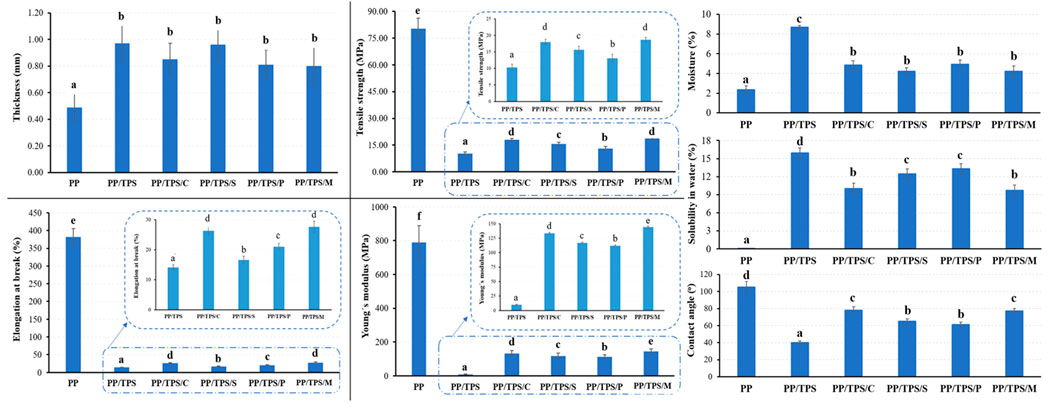
FIGURE 2. Thickness, mechanical and physical properties of the PP and blend sheets without (PP/TPS) and with compatibilizing agents (PP/TPS/C, PP/TPS/S, PP/TPS/P, and PP/TPS/M). Means with a different letter indicate a significant difference between sheets according to Tukey’s test (p < 0.05).
The blend sheets had higher thicknesses than those of the PP, and there was no significant difference (p < 0.05) in the thickness of the blend sheets produced with or without compatibilizing agents. We named the materials produced in this work as sheets, as their thickness is greater than 0.25 mm, following the ASTM D882-12 standard (2018) (26). This fact can be explained by the flowability of polymers when they are extruded. As pure PP has greater fluidity than blends, pure PP flows more during the extrusion process, forming thinner films. The greater thickness of the blends reflects the molecular interaction of TPS with PP during processing (Gómez-Contreras et al., 2021).
Regarding mechanical properties, the addition of TPS to the PP matrix including or not compatibilizing agents, significantly modified these properties. The blend sheets were less resistant to break, rigid, and flexible than the PP sheets. Other authors also found that the addition of starch to the synthetic polymer matrix decreased its rigidity and flexibility (Martins and Santana, 2016; Palai et al., 2019).
Among the sheet blends, the blend sheet without compatibilizing agent (PP/TPS) showed the lowest resistance at break, rigidity, and flexibility. As discussed by Martins and Santana (Martins and Santana, 2016), there was one incompatibility between hydrophilic TPS and hydrophobic PP; therefore, the mechanical properties of the PP/TPS blends were quite weak. Also, TPS acts as an unreinforced agent and as a stress concentrator, reducing the resulting tensile strength, increasing brittleness, and inducing cracks during the tensile test (Moghaddam et al., 2018). TPS materials based on cassava starch showed lower resistance at break and rigidity and higher flexibility than the TPS/PP blends shown in this work (tensile strength of 1.8 MPa, 50% of elongation at break, and 10.5 MPa of Young´s modulus) (Vedove et al., 2021).
Based on Figure 2, it is noteworthy that the presence of the compatibilizing agents improved the mechanical properties of the blend sheets. The citric acid (PP/TPS/C) and maleic anhydride (PP/TPS/M) provide greater elongation and resistance at break than the blend sheets with stearic acid (PP/TPS/S) and palmitic acid (PP/TPS/P). Maleic anhydride contains an anhydride group, as the name implies, and citric acid contains three carboxyl groups and a hydroxyl group. Carboxylic acids have a polar group (COOH) that can react with starch hydroxyl groups through secondary bonding forces, decreasing starch hydrophilicity and increasing the compatibility of TPS with the hydrophobic polymeric chain PP (González Seligra et al., 2016; Martins et al., 2018). Regarding its influence on the rigidity (Young´s modulus) of the blend sheets, the presence of maleic anhydride (PP/TPS/M) presented a slightly higher value than with other compatibilizing agents. The stearic acid resulted in blend sheets slightly more resistant at break and rigid, and less flexible than that added of palmitic acid. As can be seen in Figure 1, the structures of stearic acid and palmitic acid are similar, with the presence of a single carboxyl group and a long unsaturated chain without branches, while maleic anhydride and citric acid are smaller molecules. In the case of stearic acid (PP/TPS/S) and palmitic acid (PP/TPS/P), the higher chain carbonic of the stearic acid (C18H36O2) can have shown new potential reactive points, promoting greater interaction between phases, resulting in more resistant samples than the blend sheets with palmitic acid (C16H32O2). In general, in this work, we observed that citric acid was the best compatibilizing agent among carboxylic acids, and it can be associated with the presence of more polar groups in a molecule with lower molecular size, allowing more interactions with TPS and PP.
Martins et al. (2018) observed different behavior for low-density polyethylene and cornstarch blends processed in the twin-screw extruder. The authors described that the compatibilizing agent citric acid showed a negative effect on the blend’s mechanical properties, while palmitic acid allowed the formulations to overcome the deficiencies associated with the incompatibility. This different behavior can be associated with the amylose apparent content, the starch source used by these authors was a conventional corn that shows a lower amylose content (28 g/100 g) than babassu starch (21.90 g/100 g). According to Zhou et al. (Zhou et al., 2007), double bonds in unsaturated fatty acids and the presence of more functional groups (e.g., hydroxyl, carbonyl, and carboxyl) restrained the formation of the complex while saturated fatty acids such as palmitic and stearic acid were more favorable to complex formation with the starch with higher amylose content. Therefore, the interactions involved in the blend sheet structures are particular to each type of starch source and synthetic polymer.
3.4 Physical properties
Figure 2 also shows the physical properties (moisture, solubility in water, contact angle—wettability) of the PP and blend sheets without (PP/TPS) and with compatibilizing agents (PP/TPS/C, PP/TPS/S, PP/TPS/P, and PP/TPS/M).
Figure 2 allows us to observe that all the blend sheets showed a higher moisture content and solubility in water than the PP sheets, which are practically insoluble in water. The blend sheet without a compatibilizing agent (PP/TPS) showed the highest moisture and solubility value in water (∼9% and 16%, respectively). Blends of thermoplastic sugar palm starch (TPS)/poly(lactic acid) (PLA) (30:70) showed a lower value of moisture (3.46%) and higher value of solubility in water (23.16%) (Nazrin et al., 2020). However, the blend sheets with the compatibilizing agents showed lower moisture (∼5%) and solubility in water (∼10%–13%), indicating the presence of these agents contributed to reducing the free sites of the blend sheets matrix which interact with water.
The contact angle values reflect the wettability of the materials (Pan et al., 2021). PP sheets showed hydrophobic character since the contact angle is >90 o as observed in the literature (Sun et al., 2020). Concerning the blend sheets, all showed hydrophilic character (contact angle <90o).
PP/TPS/C and PP/TPS/M showed significant differences (p < 0.05) concerning hydrophilicity and water solubility when compared to PP/TPS/S and PP/TPS/P, and these sheets were less hydrophilic (∼80°) and soluble in water (∼10%) (PP/TPS/S and PP/TPS/P: contact angle ∼60° and solubility in water ∼13%). A possible explanation is that in the presence of compatibilizing agents, there is a reduction in the number of hydroxyl groups available on the surface of the material, contributing to a decrease in hydrophilicity (Fourati et al., 2021). Furthermore, these agents probably also contribute to the retention and immobilization of the glycerol plasticizer within the TPS sheet through hydrogen bonding between the compatibilizing agents and the glycerol, reducing its tendency to migrate to the surface of the blend sheets (Carmona et al., 2015). As already observed in the behavior of mechanical properties, the compatibilizing agents, citric acid and maleic anhydride, showed the best interaction with the matrix. Therefore, it can be concluded that the structure of blend sheets formed by these agents has fewer sites free from interaction with water and thus a less hydrophilic character than those in the presence of the other compatibilizing agents evaluated.
Finally, the values of water solubility for the blend sheets show that the alternative proposed herein can contribute to the environment since these materials partially solubilize resulting in less accumulation of plastic waste than if they were entirely composed of the synthetic polymer PP.
3.5 Crystallinity by X-ray diffraction
The XRD results for the PP and the blend sheets are shown in Figure 3. PP sheets showed peaks at 14.2° (110), 17.3° (040), 18.9° (130), and 21.6° (111), which are characteristics of the monoclinic α-crystal of PP (Wang and Gardner, 2017). The blend sheets displayed peaks at 13.1°, 17.3°, 19.9°, 21.6°, 23.1°, 24.2°, and 27.3° (2θ). According to Maniglia et al. (Maniglia et al., 2017), the babassu starch sheets displayed peaks at 17°, 19°, and 22° which are characteristics of B-type polymorphs. Therefore, we can observe that some characteristic peaks of the PP sheets are still present in the sheet blends, but other peaks are also shown (13.1°, 19.9°, 23.1°, and 27.3°), indicating that a new matrix organization was formed in these materials. Although the PP content is superior to the TPS content (PP/TPS, 70:30) in the blend sheets, the diffraction pattern of the TPS overlapped with that of the PP crystals. Lopes, Oliveira, Talabi, and Lucas (Lopes et al., 2021) also mentioned that the persistent presence of the characteristic peaks of type B starch may indicate the residual granular structure of starches in the blend sheets.
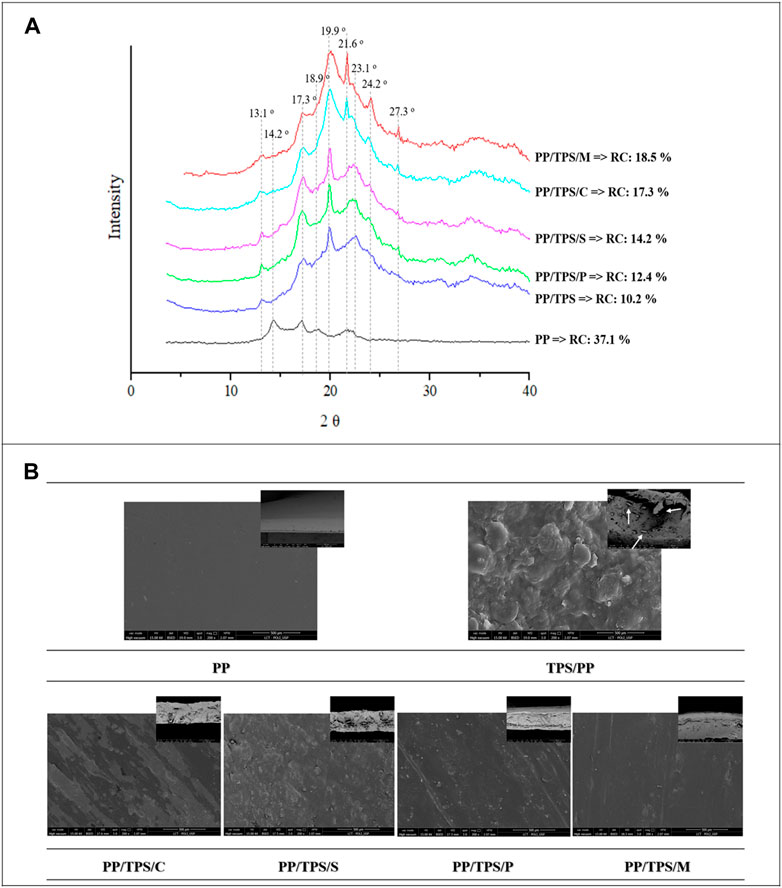
FIGURE 3. (A) XRD patterns and (B) SEM of the surface and cross section (Magnification ×400) of the PP and blend sheets without (PP/TPS) and with compatibilizing agents (PP/TPS/C, PP/TPS/S, PP/TPS/P, and PP/TPS/M). RC: relative crystallinity.
The diffraction peak close to 20° shows the highest intensity in all blend sheets and is attributed to the recrystallization induction process in a simple helical amylose molecule during cooling after processing and corresponds to the Vh-type crystalline arrangement (Lai et al., 2016). Additionally, peaks close to 13°, 20°, and 22° are attributed to the Vh-type amylose crystals complexed with other substances such as glycerol and the compatibilizing agents formed during cooling after the process (Azevedo et al., 2017).
Regarding relative crystallinity (RC), the blend sheets showed lower values (∼10%–19%) when compared to PP (37.1%), indicating that the inclusion of TPS in the PP matrix, even in the presence of compatibilizing agents, resulted in a reduction of the organization of the matrix. It explained the lower resistance at break and rigidity values of the blend sheets when compared to PP sheets. Furthermore, the blend sheets PP/TPS/C (17.3%) and PP/TPS/M (18.5%) showed the highest values of relative crystallinity, which explains the superior mechanical properties shown by these materials when compared to the other blend sheets. As discussed above, citric acid and maleic anhydride led to greater interaction with the polymer chains, improving the interaction and alignment, resulting in a more ordered matrix.
3.6 Morphology by scanning electron microscopy
The SEM microscopy (magnification of ×200) of the PP and blend sheets is shown in Figure 3, in which it can be observed that the PP sheets are very homogeneous in comparison to the blend sheets.
The PP/TPS shows a structure much more heterogeneous than the blend sheets with the compatibilizing agents, and in the transversal microscopy, it is possible to see the presence of intact starch granules, which indicates that the compatibilizing agents may also have cooperated in the gelatinization of the starch granules. Surface segregation is caused by different surface energies between the immiscible mixture, driving the low surface energy component to the surface (Panrong et al., 2019). The weak adhesion between phases could explain the low values of the mechanical properties presented by the PP/TPS blend sheets.
In turn, the blend sheets with compatibilizing agents showed a more homogeneous matrix structure, with emphasis on the PP/TPS/C and PP/TPS/M, with a lower presence of nongelatinized starch granules compared to the PP/TPS/S and PP/TPS/P blend sheets. This allows the conclusion that the good mechanical properties and lower hydrophilicity of these PP/TPS/C and PP/TPS/M blends can be associated with a more organized and homogeneous matrix, which can be observed in the SEM and XRD results.
4 Conclusion
In this work, an innovative starchy source, babassu starch, was incorporated into a polypropylene matrix to investigate the effect of different carboxylic acids as natural compatibilizing agents (NCA) on the crystallinity, mechanical, physical, and morphological properties of the blend sheets (PP/TPS). In general, compared to the PP films, the addition of TPS in the PP matrix, including or not compatibilizing agents, contributed to dark color, higher opacity, higher thickness, lower resistance at break, flexibility, rigidity, and crystallinity, higher moisture, solubility in water, and hydrophilicity. However, in comparison of the blend sheets, it was observed that the presence of the compatibilizing agents in the PP/TPS blend sheets resulted in better mechanical properties, lower hydrophilicity, and lower solubility in water, highlighting citric acid, which showed the best capacity among the NCAs and similar behavior to that of synthetic maleic anhydride. The results suggest that the improved compatibility was attributed to a chemical reaction between hydroxyl groups of starch and carboxyl groups of acids, and the interaction between the hydrocarbon chain of the acid and the PP chain. The results from XRD and SEM confirmed that the compatibilizing agents produced more homogeneous matrices, highlighting citric acid that, among the NCAs, showed the best performance, resulting in a matrix with a higher level of organization and crystallinity.
Finally, this work allowed assessing a new source of starch and offering one more alternative for applying this source to the plastic packaging sector. Furthermore, this work showed that the natural compatibilizing agent citric acid was analogous to the commercial one, making synthetic maleic anhydride a more sustainable alternative.
Data availability statement
The raw data supporting the conclusion of this article will be made available by the authors, without undue reservation.
Author contributions
BM: Conceptualization, Methodology, Validation, Formal analysis, Investigation, Data curation, Writing—original draft, Visualization. TA: Formal analysis, Investigation, Visualization, Data curation. CT: Conceptualization, Methodology, Validation, Resources, Writing—review and editing, Supervision, Project administration, Funding acquisition.
Acknowledgments
The authors acknowledge the State of São Paulo Research Foundation (FAPESP) for grants, 2016/12385-2, 2020/08727-0, and 2021/05947-2, and the National Council for Scientific and Technological Development (CNPq, Brazil) for grants 405694/2017-2 and 309548/2021-7. We would like to thank the Food Research Center—FoRC for financial support under the FAPESP grant 2013/07914-8.
Conflict of interest
The authors declare that the research was conducted in the absence of any commercial or financial relationships that could be construed as a potential conflict of interest.
Publisher’s note
All claims expressed in this article are solely those of the authors and do not necessarily represent those of their affiliated organizations, or those of the publisher, the editors and the reviewers. Any product that may be evaluated in this article, or claim that may be made by its manufacturer, is not guaranteed or endorsed by the publisher.
References
Aksit, A., Menzel, T., Aksit, M., and Altstädt, V. (2020). Properties of styrene–maleic anhydride copolymer compatibilized polyamide 66/poly (phenylene ether) blends: Effect of maleic anhydride concentration and copolymer content. Mater. (Basel) 13 (5), 1237. doi:10.3390/ma13051237
Alves Lopes, I., Coelho Paixão, L., Souza da Silva, L. J., Almeida Rocha, A, D., Barros Filho, A. K., and Amorim Santana, A. (2020). Elaboration and characterization of biopolymer films with alginate and babassu coconut mesocarp. Carbohydr. Polym. 234, 115747. doi:10.1016/j.carbpol.2019.115747
AOAC (2005). Method 926.86. Official methods of analysis of AOAC International. 18th ed. Gaithersburg, Maryl: Assoc Off Anal Chem.
Arora, B., Yoon, A., Sriram, M., Singha, P., and Rizvi, S. S. H. (2020). Reactive extrusion: A review of the physicochemical changes in food systems. Innov. Food Sci. Emerg. Technol. 64, 102429. doi:10.1016/j.ifset.2020.102429
ASTM Standard D 7334-08 (2013). “Standard practice for surface wettability of coatings, substrates and pigments by advancing contact angle measurement,” in Annual book of ASTM standards (West Conshohocken, PA: ASTM International).
ASTM Standard D644-07 (2007). “Standard test methods for moisture content of paper and paperboard by oven drying,” in Annu B ASTM stand (West Conshohocken, PA: ASTM International).
ASTM Standard D7251 (2011). “Standard specification for color and appearance retention of variegated color plastic siding products,” in Annual book of ASTM standards (Philadelphia: American Society for Testing Materials).
ASTM Standard D882-12 (2018). “Standard test method for tensile properties of thin plastic sheeting,” in Annual book of ASTM standards (West Conshohocken, PA: ASTM International).
Azevedo, V. M., Borges, S. V., Marconcini, J. M., Yoshida, M. I., Neto, A. R. S., Pereira, T. C., et al. (2017). Effect of replacement of corn starch by whey protein isolate in biodegradable film blends obtained by extrusion. Carbohydr. Polym. 157, 971–980. doi:10.1016/j.carbpol.2016.10.046
Carmona, V. B., Corrêa, A. C., Marconcini, J. M., and Mattoso, L. H. C. (2015). Properties of a biodegradable ternary blend of thermoplastic starch (TPS), poly(ε-caprolactone) (PCL) and poly(lactic acid) (PLA). J. Polym. Environ. 23 (1), 83–89. doi:10.1007/s10924-014-0666-7
Cerclé, C., Sarazin, P., and Favis, B. D. (2013). High performance polyethylene/thermoplastic starch blends through controlled emulsification phenomena. Carbohydr. Polym. 92 (1), 138–148. doi:10.1016/j.carbpol.2012.08.107
Chauhan, S., Raghu, N., and Raj, A. (2021). Effect of maleic anhydride grafted polylactic acid concentration on mechanical and thermal properties of thermoplasticized starch filled polylactic acid blends. Polym. Polym. Compos 29 (9S), S400–S410. doi:10.1177/09673911211004194
Din, M. I., Ghaffar, T., Najeeb, J., Hussain, Z., Khalid, R., and Zahid, H. (2020). Potential perspectives of biodegradable plastics for food packaging application-review of properties and recent developments. Food Addit. Contam. Part A Chem. Anal. Control Expo. Risk Assess. 37 (4), 665–680. doi:10.1080/19440049.2020.1718219
Diyana, Z. N., Jumaidin, R., Selamat, M. Z., Ghazali, I., Julmohammad, N., Huda, N., et al. (2021). Physical properties of thermoplastic starch derived from natural resources and its blends: A review. Polym. (Basel) 13 (9), 1396. doi:10.3390/polym13091396
do Nascimento, J. M., de Oliveira, J. D., and Leite, S. G. F. (2019). Chemical characterization of biomass flour of the babassu coconut mesocarp (Orbignya speciosa) during biosorption process of copper ions. Environ. Technol. Innov. 16, 100440. doi:10.1016/j.eti.2019.100440
do Val Siqueira, L., Arias, C. I. L. F., Maniglia, B. C., and Tadini, C. C. (2021). Starch-based biodegradable plastics: methods of production, challenges and future perspectives. Curr. Opin. Food Sci. 38, 122–130. doi:10.1016/j.cofs.2020.10.020
Fourati, Y., Tarrés, Q., Delgado-Aguilar, M., Mutjé, P., and Boufi, S. (2021). Cellulose nanofibrils reinforced PBAT/TPS blends: Mechanical and rheological properties. Int. J. Biol. Macromol. 183, 267–275. doi:10.1016/j.ijbiomac.2021.04.102
Gómez-Contreras, P., Contreras-Camacho, M., Avalos-Belmontes, F., Collazo-Bigliardi, S., and Ortega-Toro, R. (2021). Physicochemical properties of composite materials based on thermoplastic yam starch and polylactic acid improved with the addition of epoxidized sesame oil. J. Polym. Environ. 29 (10), 3324–3334. doi:10.1007/s10924-021-02119-0
Gontard, N., Guilbert, S., and Cuq, J. L. (1992). Edible wheat gluten films: influence of the main process variables on film properties using response surface methodology. J. Food Sci. 57 (1), 190–195. doi:10.1111/j.1365-2621.1992.tb05453.x
González Seligra, P., Eloy Moura, L., Famá, L., Druzian, J. I., and Goyanes, S. (2016). Influence of incorporation of starch nanoparticles in PBAT/TPS composite films. Polym. Int. 65 (8), 938–945. doi:10.1002/pi.5127
Jacob, J., Thomas, S., Loganathan, S., and Valapa, R. B. (2020). “Antioxidant incorporated biopolymer composites for active packaging,” in Processing and development of polysaccharide-based biopolymers for packaging applications (Netherlands: Elsevier), 239–260.
Lai, J. C., Rahman, W., Averous, L., and Lim, T. H. (2016). Study and characterisation of the post processing ageing of sago pith waste biocomposites. Sains Malays. 45 (4), 633–641.
Lin, T. A., Lin, M-C., Lin, J-Y., Lin, J-H., Chuang, Y-C., and Lou, C-W. (2020). Modified polypropylene/thermoplastic polyurethane blends with maleic-anhydride grafted polypropylene: blending morphology and mechanical behaviors. J. Polym. Res. 27 (2), 34. doi:10.1007/s10965-019-1974-3
Lopes, H. S. M., Oliveira, G. H. M., Talabi, S. I., and Lucas, A. A. (2021). Production of thermoplastic starch and poly (butylene adipate-co-terephthalate) films assisted by solid-state shear pulverization. Carbohydr. Polym. 258, 117732. doi:10.1016/j.carbpol.2021.117732
Maniglia, B. C., and Tapia-Blácido, D. R. (2016). Isolation and characterization of starch from babassu mesocarp. Food Hydrocoll. 55, 47–55. doi:10.1016/j.foodhyd.2015.11.001
Maniglia, B. C., Tessaro, L., Lucas, A. A., and Tapia-Blácido, D. R. (2017). Bioactive films based on babassu mesocarp flour and starch. Food Hydrocoll. 70, 383–391. doi:10.1016/j.foodhyd.2017.04.022
Martínez, C., and Cuevas, F. (1989). Evaluación de la calidad culinaria y molinera del arroz. Guia de studio. Cali: CIAT, 75.
Martins, A. B., and Santana, R. M. C. (2016). Effect of carboxylic acids as compatibilizer agent on mechanical properties of thermoplastic starch and polypropylene blends. Carbohydr. Polym. 135, 79–85. doi:10.1016/j.carbpol.2015.08.074
Martins, A. B., Cattelan, A. K., and Santana, R. M. C. (2018). How the compatibility between polyethylene and thermoplastic starch can be improved by adding organic acids? Polym. Bull. Berl. 75 (5), 2197–2212. doi:10.1007/s00289-017-2147-3
Martins, A. B., Silveira, A. M., Morisso, F. D. P., and Santana, R. M. C. (2021). Gelatinized and nongelatinized starch/pp blends: effect of starch source and carboxylic and incorporation. J. Polym. Res. 28 (1), 9. doi:10.1007/s10965-020-02372-2
Moghaddam, M. R. A., Razavi, S. M. A., and Jahani, Y. (2018). Effects of compatibilizer and thermoplastic starch (TPS) concentration on morphological, rheological, tensile, thermal and moisture sorption properties of plasticized polylactic acid/TPS blends. J. Polym. Environ. 26 (8), 3202–3215. doi:10.1007/s10924-018-1206-7
Nara, S., and Komiya, T. (1983). Studies on the relationship between water-saturated state and crystallinity by the diffraction method for moistened potato starch. Starch/Starke. 35 (12), 407–410. doi:10.1002/star.19830351202
Nazrin, A., Sapuan, S. M., and Zuhri, M. Y. M. (2020). Mechanical, physical and thermal properties of sugar palm nanocellulose reinforced thermoplastic starch (TPS)/Poly (lactic acid) (PLA) blend bionanocomposites. Polym. (Basel) 12 (2216), E2216. doi:10.3390/polym12102216
Palai, B., Biswal, M., Mohanty, S., and Nayak, S. K. (2019). In situ reactive compatibilization of polylactic acid (PLA) and thermoplastic starch (TPS) blends; synthesis and evaluation of extrusion blown films thereof. Ind. Crops Prod. 141, 111748. doi:10.1016/j.indcrop.2019.111748
Pan, B., Clarkson, C. R., Debuhr, C., Younis, A., Song, C., Ghanizadeh, A., et al. (2021). Low-permeability reservoir sample wettability characterization at multiple scales: Pore-micro- and macro-contact angles. J. Nat. Gas. Sci. Eng. 95, 104229. doi:10.1016/j.jngse.2021.104229
Panrong, T., Karbowiak, T., and Harnkarnsujarit, N. (2019). Thermoplastic starch and green tea blends with LLDPE films for active packaging of meat and oil-based products. Food packag. Shelf Life 21, 100331. doi:10.1016/j.fpsl.2019.100331
Raee, E., Avid, A., and Kaffashi, B. (2020). Effect of compatibilizer concentration on dynamic rheological behavior and morphology of thermoplastic starch/polypropylene blends. J. Appl. Polym. Sci. 137 (22), 48742. doi:10.1002/app.48742
Saraiva Rodrigues, S. C., Silva, A. S., Carvalho, L. H. de, Alves, T. S., and Barbosa, R. (2020). Morphological, structural, thermal properties of a native starch obtained from babassu mesocarp for food packaging application. J. Mat. Res. Technol. 9 (6), 15670–15678. doi:10.1016/j.jmrt.2020.11.030
Shirai, M. A., Zanela, J., Kunita, M. H., Pereira, G. M., Rubira, A. F., Müller, C. M. O., et al. (2018). Influence of carboxylic acids on poly(lactic acid)/thermoplastic starch biodegradable sheets produced by calendering-extrusion. Adv. Polym. Technol. 37 (2), 332–338. doi:10.1002/adv.21671
Souza, A. C., Benze, R., Ferrão, E. S., Ditchfield, C., Coelho, A. C. V., and Tadini, C. C. (2012). Cassava starch biodegradable films: Influence of glycerol and clay nanoparticles content on tensile and barrier properties and glass transition temperature. LWT - Food Sci. Technol. 46 (1), 110–117. doi:10.1016/j.lwt.2011.10.018
Sun, F., Li, T-T., Zhang, X., Shiu, B-C., Zhang, Y., Ren, H-T., et al. (2020). Facile fabrication of hydrophilic-underwater superoleophobic poly(N-isopropylacrylamide) coated PP/LPET nonwoven fabrics for highly efficient oil/water separation. Prog. Org. Coat. 148, 105780. doi:10.1016/j.porgcoat.2020.105780
Taguet, A., Bureau, M. N., Huneault, M. A., and Favis, B. D. (2014). Toughening mechanisms in interfacially modified HDPE/thermoplastic starch blends. Carbohydr. Polym. 114, 222–229. doi:10.1016/j.carbpol.2014.07.073
Vedove, T. M. A. R. D., Maniglia, B. C., and Tadini, C. C. (2021). Production of sustainable smart packaging based on cassava starch and anthocyanin by an extrusion process. J. Food Eng. 289, 110274. doi:10.1016/j.jfoodeng.2020.110274
Wang, L., and Gardner, D. J. (2017). Effect of fused layer modeling (FLM) processing parameters on impact strength of cellular polypropylene. Polymer 113, 74–80. doi:10.1016/j.polymer.2017.02.055
Zaferani, S. H. (2018). “Introduction of polymer-based nanocomposites,” in Polymer-based nanocomposites for energy and environmental applications (Netherlands: Elsevier), 1–25.
Keywords: alternative starchy source, extrusion process, biodegradable packaging, mechanical properties, new materials
Citation: Maniglia BC, Vedove TMARD and Tadini CC (2022) Evaluation of carboxylic acids as sustainable compatibilizing agents on blend sheets properties based on thermoplastic babassu starch and polypropylene. Front. Food. Sci. Technol. 2:1011119. doi: 10.3389/frfst.2022.1011119
Received: 03 August 2022; Accepted: 09 September 2022;
Published: 23 September 2022.
Edited by:
Fabiola Cornejo, ESPOL Polytechnic University, EcuadorReviewed by:
Saraswati Masti, Karnatak University, IndiaBarbara Teixeira-Costa, Federal University of Amazonas, Brazil
Copyright © 2022 Maniglia, Vedove and Tadini. This is an open-access article distributed under the terms of the Creative Commons Attribution License (CC BY). The use, distribution or reproduction in other forums is permitted, provided the original author(s) and the copyright owner(s) are credited and that the original publication in this journal is cited, in accordance with accepted academic practice. No use, distribution or reproduction is permitted which does not comply with these terms.
*Correspondence: Carmen Cecília Tadini, catadini@usp.br