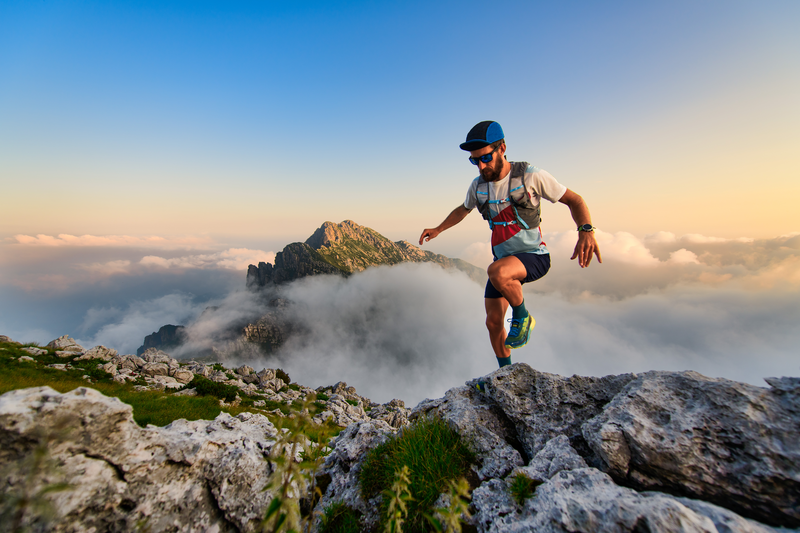
94% of researchers rate our articles as excellent or good
Learn more about the work of our research integrity team to safeguard the quality of each article we publish.
Find out more
ORIGINAL RESEARCH article
Front. Environ. Sci. , 06 January 2025
Sec. Toxicology, Pollution and the Environment
Volume 12 - 2024 | https://doi.org/10.3389/fenvs.2024.1514062
This article is part of the Research Topic Emerging Contaminants and Aquatic Ecosystem Health View all 4 articles
Emerging contaminants such as microplastics and pharmaceuticals in freshwater ecosystems are a growing concern, seriously affecting aquatic organisms. Ibuprofen, a widely used anti-inflammatory drug, is commonly found in rivers, streams, and water systems where it is discharged. As a result, organisms that inhabit these environments, such as snails, are affected in their natural habitats. This study examines the effects of microplastics (10 μg/L), ibuprofen (500 μg/L), and a combination of ibuprofen (500 μg/L) and microplastics (10 μg/L) on growth, movement, and reproduction in Physella acuta, a freshwater snail species. While no significant effects were observed on movement or number of egg clusters, there was a significant decrease in growth when snails were exposed to microplastics or the combination of microplastics and ibuprofen (p = 0.021). Additionally, snail egg production decreased when exposed to ibuprofen (p = 0.001) but increased when exposed to microplastics (p = 0.002). Microplastic exposure produced more eggs than ibuprofen (p < 0.001) and ibuprofen and microplastics combined (p < 0.001). Our results suggest that ibuprofen affects growth and the total number of eggs, likely due to oxidative stress, neurotoxicity, or disrupted hormonal pathways. In contrast, microplastics may have caused digestive system blockage, thus affecting energy allocation for growth and reproduction. Changes in snail fitness can directly and indirectly affect food webs and nutrient cycles, highlighting the need for research on these pollutants to understand their chronic and long-term effects on ecosystems.
The global human population is projected to reach 9.7 billion by 2050, significantly increasing the demand for products and services, including medicines, food, and clothing (Sadigov, 2022; Albahri et al., 2023). A negative consequence of this growing demand is the rise in waste production, including pharmaceuticals and plastics, which contribute to environmental pollution. From 1950 to 2018, plastic production exceeded 6.3 billion tons, with 370 million tons produced in 2019 alone, representing 16% of the previous 68 years (Alabi et al., 2019; Ritchie et al., 2023). Further, it is estimated that 23 million tons of plastic entered aquatic ecosystems in 2016, and it is projected to reach 53 million tons by 2030 (Borrelle et al., 2020). Improper disposal of plastic, with 21% of this plastic being recycled or incinerated, leads to pollution in aquatic and terrestrial ecosystems (Kumar et al., 2021; Shetty et al., 2023). This widespread plastic pollution highlights the critical need to study its long-term ecological impacts.
Microplastics, defined as plastic particles smaller than 5 mm, are an emerging concern due to their potential effects on marine organisms and human health (Prata et al., 2020). Microplastics have multiple applications. For example, polylactic acid (PLA), polyethylene terephthalate (PET) and polyethylene (PE) are found in bottles, polyhydroxyalkanoates (PHA) are used in disposable items, polycaprolactone (PCL) is common in medical devices and food packaging, polypropylene in clothing, polystyrene in food containers, and polyurethane is found in tires, furniture, and insulation (Iwata, 2015; Lambert and Wagner, 2018; González-Pleiter et al., 2019; Krueger et al., 2015; Majewsky et al., 2016; Zhang et al., 2020; Shah et al., 2008).
These particles can persist in the environment for thousands of years without degrading (Andrady and Koongolla, 2022). Microplastics have been detected in aquatic and terrestrial ecosystems, with polyethylene microplastics accounting for more than 50% of total microplastics floating in the ocean (Issac and Kandasubramanian, 2021), thus representing a risk for multiple species and human health (Li et al., 2023). They have been found in daphnia, salmon, tuna, and shellfish (Guzzetti et al., 2018; Prata et al., 2020; Li et al., 2023) Despite their frequent detection in the aquatic and terrestrial habitats, microplastics effects on ecosystem health remain poorly understood, specifically when combined with other pollutants.
The production of pharmaceuticals has also seen a sharp increase. Similar to plastic, pharmaceuticals contribute significantly to environmental pollution. Ibuprofen (e.g., Advil, Motrin, Midol, Nurofen, Dalsi, etc.) is one of the most used non-steroidal anti-inflammatory drugs (NSAIDs) globally and is considered an essential medicine by the World Health Organization (Miranda et al., 2021; Michalaki and Grintzalis, 2023). The global ibuprofen market is valued at USD 294.4 million in 2020 and is estimated to grow to USD 447.6 million by 2026 (Makuch et al., 2021). In the US, ibuprofen was prescribed 21.3 million times in 2016 (Thammineni et al., 2019). Additionally, 45% of global sales of pharmaceuticals are attributed to NSAIDs and ibuprofen being a major contributor (Schmidt and Redshaw, 2015).
Ibuprofen is frequently detected in water systems due to its widespread use and improper disposal (Austin et al., 2022; Petrie and Camacho-Muñoz, 2021).
Ibuprofen mechanism of action involves the reversible inhibition of cyclooxygenase enzymes (i.e., COX-1 and COX-2). These enzymes are part of the synthesis of prostaglandins, which mediate inflammation and pain (Negres, 2019). Ibuprofen inhibiting these enzymes reduces the levels of prostaglandins and decreases inflammation and pain perception (Negres, 2019).
In aquatic organisms, ibuprofen has been linked to oxidative stress, cytotoxicity, genotoxicity, and neurotoxic effects, including changes in behavior (Sibiya et al., 2023). Changes in enzyme activity, including glutathione S-transferase (GST), catalase, lipid peroxidation, and protein carbonyls, are biomarkers indicating oxidative damage in multiple aquatic organisms such as Chironomus riparius (Muñiz-González, 2021), Danio rerio (Falfushynska et al., 2022), and Dreissena polymorpha (Gonzalez-Rey and Bebianno, 2012). Ibuprofen’s effects on acetylcholinesterase (AChE) activity can lead to neurotoxic effects, such as altered behavior, growth, and reproduction. For example, ibuprofen exposure inhibited AChE in Carassius auratus, disrupting normal neurotransmission and causing physiological stress (Yang et al., 2019). Similarly, when exposed to ibuprofen, Daphnia magna showed altered spontaneous movement, free-swimming distance, duration, and speed under dark conditions (Michalaki and Grintzalis, 2023). In addition, Kovacevic et al. (2016) demonstrated neurotoxic impacts in D. magna using metabolomic analysis, revealing disruptions in physiological functions and reductions in organismal fitness.
Both pharmaceuticals and microplastics in freshwater environments present a significant risk to aquatic organisms and water quality. Thus, the co-occurrence of these pollutants in aquatic ecosystems highlights the importance of understanding their combined effects on freshwater species.
Research has shown that microplastics affect marine organisms’ health, reproduction, and survival (Pantos, 2022), though the full ecological consequences remain unclear (Santillo et al., 2017; Mishra et al., 2021). For example, European Perch (Perca fluviatilis) exposed to polystyrene microplastics displayed inhibited hatching, reduced growth rates, altered feeding preferences, and increased predation risk (Lönnstedt and Eklöv, 2016). In European seabass (Dicentrarchus labrax), microplastics inhibited he activity of acetylcholinesterase enzyme, increased lipid oxidation in the brain (Li et al., 2023). Therefore, these studies emphasize the need to further explore the broader ecological impacts of microplastic exposure.
Overusing pharmaceuticals like ibuprofen contributes to high waterway concentrations, negatively impacting aquatic organisms. Ibuprofen exposure has been linked to cytotoxic and genotoxic effects, oxidative stress, and adverse impacts on growth, reproduction, and behavior (Sibiya et al., 2023). Pharmaceuticals and microplastics pose significant risks to water quality and aquatic life due to their bioactivity and toxic metabolites (Chopra and Kumar, 2020).
Ibuprofen is commonly detected in surface waters at concentrations ranging from ng/L to μg/L. However, significantly higher concentrations have been reported in streams influenced by effluents from hospitals and wastewater treatment plants. Specifically, maximum concentrations of 280 μg/L (Taiwan), 414 μg/L (Korea), and 603 μg/L (United Kingdom) have been detected (Almeida et al., 2013; Luo et al., 2014; Jan-Roblero and Cruz-Maya, 2023). These concentrations in effluent-impacted streams highlight the localized contribution of wastewater discharges to pharmaceutical contamination in streams. Understanding the interactions between ibuprofen and microplastics and their combined effects on freshwater ecosystems is crucial for predicting long-term environmental consequences.
Freshwater organisms, including Physella acuta, are particularly susceptible to pollutants. Physella acuta plays a key role in the ecosystems as a food source for larger species and by contributing to nutrient cycling (McClain et al., 2012; Naldi et al., 2020; Konschak et al., 2021). Due to its rapid population growth and adaptability to various environments, Physella acuta has become an important model organism for studying the impact of environmental pollution (Camargo and Alonso, 2017; Prieto-Amador et al., 2021; Nandy et al., 2024). Assessing the effects of ibuprofen and microplastics on P. acuta provides valuable information into potential disruptions in nutrient cycling and aquatic food webs.
Our study explores the individual and combined effects of ibuprofen and microplastic exposure on the growth, movement, and reproduction of Physella acuta, including the number of egg clusters and total eggs produced. Previous research has focused on the independent effects of microplastics (e.g., Meaza et al., 2021; Mason et al., 2022; Pantos, 2022) and ibuprofen (e.g., De Lange et al., 2006; Muñiz-González, 2021; Jan-Roblero and Cruz-Maya, 2023) on aquatic organisms. However, limited research has addressed their combined effect, particularly on freshwater snails.
We hypothesize that 1) ibuprofen will decrease snail growth, movement, and reproduction by affecting energy allocation due to oxidative stress and neurotoxicity, 2) microplastic exposure will impair metabolic processes (i.e., digestion through physical blockage), reducing growth, movement, and reproduction, and 3) combined exposure will have a greater negative effect on these endpoints than ibuprofen or microplastics alone.
Snails (Physella acuta) were purchased from Carolina Biological Supply. Snails were kept in a 40 L glass aquarium with aerated synthetic spring water under a 16:8 photoperiod, room temperature (∼20°C), and fed fish flakes (Tetramin®). Physella acuta snails were estimated to be juveniles and adults based on their shell lengths (Núñez, 2010) ranging from 3.94 mm to 8.47 mm, with a mean of 6.68 mm (SD 1.045 mm). Three snails were placed in 200 mL glass jars. Each treatment included five replications (n = 20 experimental units; 60 snails). Water changes, treatment renewals, and feedings were performed biweekly. Similar to Bartolini et al. (2017) and Rivi et al., 2021, snails were marked using a permanent marker to differentiate between each other. No detrimental effects were observed as in Garlick-Ott and Wright (2022).
Clear polyethylene microspheres (density: 0.96 g/cm³, size range: 10–90 µm) were purchased from Cospheric LLC (United States) to study the effects of microplastic exposure. Ibuprofen stock solutions (≥98% purity, Sigma Aldrich, United States) were prepared, and aliquots were added to each glass jar to achieve the target treatment concentration of 500 μg/L.
Snails were exposed for 14 days to four treatments: control, ibuprofen (IBU, 500 μg/L), microplastics (MP, 10 μg/L), and ibuprofen and microplastics mixture (IBU × MP, 500 μg/L and 10 μg/L). Environmentally relevant concentrations of ibuprofen (500 μg/L) and microplastics (10 μg/L) were chosen to represent concentrations commonly detected in contaminated aquatic ecosystems (ibuprofen: Pharms UBA Umwelt Bundesamt, 2024; Jan-Roblero and Cruz-Maya, 2023, and microplastics: Goldstein et al., 2012; Oliveira et al., 2013; Rochman et al., 2014).
Snail shell length was measured using ImageJ (version 1.53) software to determine snail growth. Individual snails were placed in a 40 L aquarium filled with synthetic spring water (5 cm height) for quantifying movement. A one cm2 grid paper was placed underneath the aquarium. Distance traveled was not recorded for the first 10 s to allow snails to acclimatize but was recorded for the next one minute. Snail reproduction was measured using a stereo microscope by counting the number of egg clusters and the total number of eggs.
Four treatment groups (IBU alone, MP alone, IBU and MP, and control) were tested for their effects on growth, movement, number of egg clusters, and total number of eggs. Growth and movement are continuous variables modeled using ANOVA for normally distributed responses or the Kruskal-Wallis test for non-normally distributed responses. If statistically significant differences were found among treatments, a Tukey’s Honest Significant difference test was used for multiple comparisons across groups for normally distributed response variables. Dunn’s test was used for multiple comparisons across groups for non-normally distributed response variables. Normality was checked using the Shapiro-Wilkes test. The number of egg clusters and the total number of eggs are count data, and the effects of the treatment groups were assessed using a generalized linear model with a Poisson error distribution. Multiple comparisons across groups were made using the expected marginal means estimated with the emmeans () function and the contrast () function from the emmeans package version 1.10.4 (Lenth, 2024). Alpha was set at 0.05 for all analyses. All analysis was conducted using R version 4.4.1 (R Core Team, 2024). Figures were constructed in R using the ggplot2 () package version 3.5.1 (Wickham, 2016).
Snail growth ranged from 0.16 mm to 0.32 mm after 14 days of treatment exposure (Figure 1; Table 1). Snails exposed to microplastics alone and ibuprofen and microplastics combined showed a 50% significant decrease in mean growth compared to the control group (p = 0.021). Snails exposed to ibuprofen alone exhibited a 15.6% decrease in mean growth.
Figure 1. Physella acuta growth (mm) as a result of exposure to ibuprofen (500 μg/L; IBU), microplastics (10 μg/L; MP), and combined ibuprofen (500 μg/L) and microplastics (10 μg/L). Different letters represent significant differences between treatments.
Table 1. Changes in Physella acuta growth, movement, number of egg clusters, and total number of eggs after 14 days of exposure to control, ibuprofen (IBU; 500 μg/L), microplastics (MP; 10 μg/L), and ibuprofen and microplastics (IBU × MP; 500 μg/L × 10 μg/L). Numbers in parentheses represent the standard deviation.
Snail movement ranged from 0.02 cm/s to 0.07 cm/s after 14 days of treatment exposure (Figure 2; Table 1). Mean snail movement decreased by 50% in the combined ibuprofen and microplastics treatment (0.02 cm/min) compared to the control (0.04 cm/min). However, snails exposed to microplastics showed a 75% increase in the mean movement (0.07 cm/min), and those exposed to ibuprofen alone showed a 50% increase (0.06 cm/min) compared to the control. There was no significant difference between treatments.
Figure 2. Physella acuta movement (cm/s) as a result of exposure to ibuprofen (500 μg/L; IBU), microplastics (10 μg/L; MP), and combined ibuprofen (500 μg/L) and microplastics (10 μg/L). There were no significant differences between treatments.
The number of egg clusters ranged from 0.6 to 2.8 after 14 days of treatment exposure (Figure 3; Table 1). Compared to the control, mean snail production of egg clusters increased 1.33× (33%) when exposed to ibuprofen, 4.67× (367%) when exposed to microplastics, and 3× (200%) when exposed to ibuprofen and microplastics combined. There was no significant difference between treatments.
Figure 3. Physella acuta total number of eggs as a result of exposure to ibuprofen (500 μg/L; IBU), microplastics (10 μg/L; MP), and combined ibuprofen (500 μg/L) and microplastics (10 μg/L). Different letters represent significant differences between treatments.
Total egg count ranged from 8 to 16.4 after 14 days of exposure to treatments.(Figure 4; Table 1) Mean snail total egg count decreased by 51% when exposed to ibuprofen (p = 0.001), by 22% when exposed to combined ibuprofen and microplastics, and increased by 78% when exposed to microplastics (p = 0.002). Additionally, snails exposed to microplastics produced 265% × (3.65) more eggs on average than snails exposed to ibuprofen (p < 0.001) and 56% × (2.3) more eggs on average than snails exposed to ibuprofen and microplastics combined (p < 0.001).
Figure 4. Physella acuta number of egg clusters as a result of exposure to ibuprofen (500 μg/L; IBU), microplastics (10 μg/L; MP), and combined ibuprofen (500 μg/L) and microplastics (10 μg/L). There were no significant differences between treatments.
This study assessed the individual and combined effects of ibuprofen and microplastic exposure on Physella acuta growth, movement, and reproduction. Snail growth significantly decreased when exposed to microplastics alone and in combination with ibuprofen (p = 0.021; Figure 1). These findings support our hypotheses, implying that the reduction in growth likely results from a trade-off between growth and detoxification. Specifically, nutrient absorption impairment caused by microplastic physical blockage of the digestive system likely contributed to these effects (Imhof et al., 2013; Jeyavani and Vaseeharan, 2023). Previous studies on Physa fontinalis, amphipods, and rotifers also indicated reduced growth when exposed to microplastics (Au et al., 2015; Jeong et al., 2016; Michler-Kozma et al., 2022). This highlights the susceptibility of freshwater biota to short-term pollutant exposure, which could lead to effects on ecosystem functions.
Although we did not directly assess chemical interactions (i.e., synergistic, additive, antagonistic), the reduced growth observed with ibuprofen and microplastics combined may suggest that in addition to an impaired digestive system, oxidative stress from ibuprofen likely contributed to the effects of microplastics alone (Srain et al., 2021; Jan-Roblero and Cruz-Maya, 2023). In response to ibuprofen, aquatic organisms exhibit detoxification responses (Batucan et al., 2022). For example, glutathione S-transferase (GST) activity increased to prevent oxidative damage in Chironomus riparius after ibuprofen exposure (Muñiz-González, 2021). In Danio rerio (zebrafish), ibuprofen increased oxidative stress markers, including catalase, glutathione S-transferase, and protein carbonyls (Falfushynska et al., 2022). For Dreissena polymorpha (zebra mussel), ibuprofen elicited lipid peroxidation and disrupted enzymatic responses (Gonzalez-Rey and Bebianno, 2012). These findings suggest that oxidative stress and impaired detoxification processes are key mechanisms reducing snail fitness when exposed to ibuprofen and microplastics.
Reduced growth in P. acuta can disrupt ecosystem dynamics. For example, decreased feeding is associated with smaller snails (e.g., Silva et al., 2020). Thus, lower grazing may promote algal growth, affecting food available to primary consumers (Lowe and Hunter, 1988; Konschak et al., 2021). A reduction in snail populations and a corresponding decrease in egestion may also affect nutrient cycling (Mulholland et al., 1991; Perrotta et al., 2020), altering nitrogen and phosphorus availability (Elias and Bernot, 2017). Additionally, smaller snail populations can affect predator-prey dynamics by reducing food sources for predators (Justice and Bernot, 2014; Krupsi et al., 2018). These changes can affect biodiversity and community structure (Swamikannu and Hoagland, 1989; Konschak et al., 2021; Kumari et al., 2023).
Reproductive output was measured by counting the number of egg clusters and the total number of eggs (Table 1). Treatments did not affect number of egg clusters (Figure 3) but significantly decreased (ibuprofen) or increased (microplastics) the total number of eggs (Figure 4). Contrary to previous research (e.g., Michler-Kozma et al., 2022; Kumari et al., 2023; Merbt et al., 2024) that reported reduced reproductive outputs under microplastic exposure, we found a significant increase in egg production with microplastic exposure (p < 0.001). Several factors could explain this difference: 1) microplastic type and size influence their bioavailability and toxicity (Alak et al., 2022). The size and characteristics of polyethylene (PE) microspheres used in this study (10–90 µm) likely influenced their bioavailability and interaction with Physella acuta. Particles in this size range are small enough to be ingested but large enough to cause physical blockages in the digestive tract, impairing nutrient absorption. Additionally, these microplastics may adhere to mucus membranes, causing stress responses (Alak et al., 2022; Imhof et al., 2013). In addition to physical effects, PE microplastics often contain chemical additives, such as plasticizers, stabilizers, and flame retardants, which can act as endocrine-disrupting chemicals (Chen et al., 2019; Lin et al., 2023; Bucci et al., 2024). Furthermore, the hydrophobic nature of PE microplastics allows them to adsorb other contaminants which can worsen their toxicity. 2) exposure period and concentration can influence reproductive outcomes. Pedersen et al. (2020) found no changes in quagga mussel reproduction within 24 h, even at elevated microplastic concentrations of 0.8 g/L, however reduced sac production and egg hatching was observed on Physella acuta after 93 days exposure of 150 mg/L of polystyrene (Kumari et al., 2023); 3) reproductive stage and experimental conditions contribute to egg production. Saha et al. (2019) found that P. acuta produces more eggs with age. Feeding schedules, water quality, and organism density per experimental unit may also influence energy allocation toward reproduction, contributing to variability across studies. These findings highlight the need for standardized protocols to assess the complexity of ibuprofen and microplastics effects on reproduction accurately (de Ruijter et al., 2020).
Ibuprofen exposure decreased the total egg count. Although research on ibuprofen’s impact on P. acuta is limited, similar effects on reproduction have been reported in other aquatic organisms and pollutants. For example, P. acuta egg production decreased when exposed to fluoxetine (Sánchez-Argüello et al., 2009; Henry et al., 2022) and reclaimed water (Aquilino et al., 2018). When exposed to ibuprofen, impaired reproduction was reported for Planorbis carinatus, Moina macrocopa, and Oryzias latipes (Das et al., 2019). When exposed to ibuprofen and microplastics combined, there was no significant effect on the total number of eggs, thus complicating predictions of multiple-pollutant exposure (Fent et al., 2006; Di Poi et al., 2018; Zhang et al., 2022). Reduced reproduction in P. acuta could alter community structure and function. Specifically, declining snail populations and their corresponding roles as grazers and detritivores (Wikström and Hillebrand, 2012; Tchakonte et al., 2023) and as a food source for predators (Gilioli et al., 2017) may reduce biodiversity. Similarly, smaller populations can impact nutrient cycling in freshwater ecosystems (Auld, 2018).
Movement increased when exposed to combined ibuprofen and microplastics (Figure 2). Kumari et al. (2023) reported decreased movement when exposed to microplastics alone. Even though this increase was not statistically significant, it may have biological relevance as it can reflect behavioral changes or stress responses (Calow and Forbes, 1998; Almeida and Nunes, 2019), including feeding behaviors and predator avoidance (Justice and Bernot, 2014; Elias and Bernot, 2017), and changes in food web dynamics and nutrient cycling (Newman et al., 1996; Hall et al., 2003; Vannatta, 2021).
In addition to changes in ecosystem structure and function, long term effects of reduced Physella snails’ populations can be significant. Smaller populations are more vulnerable to genetic drift, inbreeding, and disrupted adaptive responses which eventually can affect survival and reproduction success. Genetic diversity is critical for an effective population size and adaptive responses (Grueber et al., 2019). For example, for colonizing populations, especially since Physella acuta is considered an invasive species, smaller populations could lead to reduced fecundity, earlier maturation (Fruh et al., 2017; Chapuis et al., 2024), reduced population density, and persistence of this conditions over generations (Szucs et al., 2017). Further, smaller populations exposed to chronic pollution are more susceptible to environmental pressures affecting birth and death rates. Thus, leading to impaired recovery from perturbations (Lopez et al., 2009).
Our study partially supports our hypothesis: microplastic and ibuprofen exposure negatively affect P. acuta. Specifically, microplastics alone and combined with ibuprofen significantly reduced snail growth, likely because of nutrient absorption impairment and energy trade-offs with detoxification processes. Ibuprofen decreased the total egg count because of impaired reproductive function. In contrast, microplastic exposure increased egg production, indicating variability in reproductive responses potentially influenced microplastic type, exposure duration, and reproductive stage. Ibuprofen and microplastics combined did not significantly affect egg count. Although increased movement with combined exposure was not statistically significant, it may still indicate biological stress responses, which could impact feeding behaviors and food web dynamics. Overall, based on our findings, we suggest that oxidative stress, neurotoxicity, and impaired digestive system affect snail fitness. The effects of these pollutants on P. acuta identified in this study: reduced growth and reduced reproductive incomes can lead to ecological impacts, including disrupted nutrient cycling, altered prey-predator dynamics, and decreased biodiversity in freshwater ecosystems.
Further, these findings contribute to the larger literature, by exploring the combined effects of ibuprofen and microplastics on Physella acuta, by addressing the knowledge gap of these pollutants and understanding multiple stressor exposure which is more relevant of real environmental conditions. The 14-day exposure period can serve as a starting point for other research to address long term impacts of ibuprofen and microplastics alone and combined. Specifically, exploring multi generation effects of these pollutants.
Future research should explore interactions between pollutants (i.e., synergistic, additive, antagonistic), wider range of pollutant concentrations, exposure times, and additional endpoints. For example, multi-generational studies would be able to address chronic impacts on P. acuta populations and their adaptive responses. Additionally, studies need to confirm the different mechanisms we suggested as key factors affecting snail fitness, therefore integrating biochemical assays in addition to ecotoxicological dose-response experiments. Further, to better understand the effects of these pollutants, other ecological relevant endpoints should be studied including egg sizes, ingestion volume, and snail speed. Furthermore, standardization of protocols to reduce variability, including microplastic types, sizes, and concentrations, is critical for reliable risk assessment and management strategies. We also propose field studies that explore the larger ecological impacts of reduced snail fitness on community structure and function. Therefore, continuing research like this contributes to and strengthens conservation strategies and mitigation efforts to protect freshwater environments from pharmaceuticals and microplastics.
The original contributions presented in the study are included in the article/Supplementary Material, further inquiries can be directed to the corresponding author.
DE: Conceptualization, Data curation, Formal Analysis, Funding acquisition, Investigation, Methodology, Project administration, Resources, Software, Supervision, Validation, Visualization, Writing–original draft, Writing–review and editing. JR: Writing–original draft. MF: Writing–original draft. JL: Writing–original draft. AH: Writing–original draft. JD: Formal Analysis, Methodology, Validation, Visualization, Writing–review and editing.
The author(s) declare that financial support was received for the research, authorship, and/or publication of this article. This research was supported by internal grants for professional development and the Evan and Marcy Duff Professional Development Grant, both awarded by North Carolina Wesleyan University.
The authors declare that the research was conducted in the absence of any commercial or financial relationships that could be construed as a potential conflict of interest.
The author(s) declare that Generative AI was used in the creation of this manuscript. Grammar and typos check.
All claims expressed in this article are solely those of the authors and do not necessarily represent those of their affiliated organizations, or those of the publisher, the editors and the reviewers. Any product that may be evaluated in this article, or claim that may be made by its manufacturer, is not guaranteed or endorsed by the publisher.
The Supplementary Material for this article can be found online at: https://www.frontiersin.org/articles/10.3389/fenvs.2024.1514062/full#supplementary-material
Alabi, O. A., Ologbonjaye, K. I., Awosolu, O., and Alalade, O. E. (2019). Public and environmental health effects of plastic wastes disposal: a review. J. Toxicol. Risk Assess. 5 (021), 1–13. doi:10.23937/2572-4061.1510021
Alak, G., Uçar, A., Parlak, V., and Atamanalp, M. (2022). Identification, characterisation of microplastic and their effects on aquatic organisms. Chem. Ecol. 38 (10), 967–987. doi:10.1080/02757540.2022.2126461
Albahri, G., Alyamani, A. A., Badran, A., Hijazi, A., Nasser, M., Maresca, M., et al. (2023). Enhancing essential grains yield for sustainable food security and bio-safe agriculture through latest innovative approaches. Agronomy 13 (7), 1709. doi:10.3390/agronomy13071709
Almeida, B., Kjeldal, H., Lolas, I., Knudsen, A. D., Carvalho, G., Nielsen, K. L., et al. (2013). Quantitative proteomic analysis of ibuprofen-degrading Patulibacter sp. strain I11. Biodegradation 24, 615–630. doi:10.1007/s10532-012-9610-5
Almeida, F., and Nunes, B. (2019). Effects of acetaminophen in oxidative stress and neurotoxicity biomarkers of the gastropod Phorcus lineatus. Environ. Sci. Pollut. Res. 26, 9823–9831. doi:10.1007/s11356-019-04349-1
Andrady, A. L., and Koongolla, B. (2022). “Degradation and fragmentation of microplastics,” in Plastics and the ocean: origin, characterization, fate, and impacts, 227–268.
Aquilino, M., Martínez-Guitarte, J. L., García, P., Beltrán, E. M., Fernández, C., and Sánchez-Argüello, P. (2018). Combining the assessment of apical endpoints and gene expression in the freshwater snail Physa acuta after exposure to reclaimed water. Sci. total Environ. 642, 180–189. doi:10.1016/j.scitotenv.2018.06.054
Au, S. Y., Bruce, T. F., Bridges, W. C., and Klaine, S. J. (2015). Responses of Hyalella azteca to acute and chronic microplastic exposures. Environ. Toxicol. Chem. 34 (11), 2564–2572. doi:10.1002/etc.3093
Auld, J. R. (2018). The effects of diet and mating system on reproductive (and post-reproductive) life span in a freshwater snail. Ecol. Evol. 8 (23), 12260–12270. doi:10.1002/ece3.4689
Austin, T., Bregoli, F., Höhne, D., Hendriks, A. J., and Ragas, A. M. (2022). Ibuprofen exposure in Europe; ePiE as an alternative to costly environmental monitoring. Environ. Res. 209, 112777. doi:10.1016/j.envres.2022.112777
Bartolini, F., Aquiloni, L., Nisi, B., Nuccio, C., Vaselli, O., and Cianfanelli, S. (2017). Divergent demographic patterns and perspectives for conservation of endemic species in extreme environments: a case study of the springsnail Melanopsis etrusca (Gastropoda: melanopsidae). Invertebr. Biol. 136 (4), 441–455. doi:10.1111/ivb.12197
Batucan, N. S. P., Tremblay, L. A., Northcott, G. L., and Matthaei, C. D. (2022). Medicating the environment? A critical review on the risks of carbamazepine, diclofenac and ibuprofen to aquatic organisms. Environ. Adv. 7, 100164. doi:10.1016/j.envadv.2021.100164
Borrelle, S. B., Ringma, J., Law, K. L., Monnahan, C. C., Lebreton, L., McGivern, A., et al. (2020). Predicted growth in plastic waste exceeds efforts to mitigate plastic pollution. Science 369 (6510), 1515–1518. doi:10.1126/science.aba3656
Bucci, K., Bayoumi, M., Stevack, K., Watson-Leung, T., and Rochman, C. M. (2024). Microplastics may induce food dilution and endocrine disrupting effects in fathead minnows (Pimephales promelas), and decrease offspring quality. Environ. Pollut. 345, 123551. doi:10.1016/j.envpol.2024.123551
Calow, P., and Forbes, V. E. (1998). How do physiological responses to stress translate into ecological and evolutionary processes? Comp. Biochem. Physiology Part A Mol. and Integr. Physiology 120 (1), 11–16. doi:10.1016/s1095-6433(98)10003-x
Camargo, J. A., and Alonso, Á. (2017). Ecotoxicological assessment of the impact of fluoride (F−) and turbidity on the freshwater snail Physella acuta in a polluted river receiving an industrial effluent. Environ. Sci. Pollut. Res. 24, 15667–15677. doi:10.1007/s11356-017-9208-x
Chapuis, E., Jarne, P., and David, P. (2024). Rapid life-history evolution reinforces competitive asymmetry between invasive and resident species. Peer Community J. 4, e25. doi:10.24072/pcjournal.394
Chen, Q., Allgeier, A., Yin, D., and Hollert, H. (2019). Leaching of endocrine disrupting chemicals from marine microplastics and mesoplastics under common life stress conditions. Environ. Int. 130, 104938. doi:10.1016/j.envint.2019.104938
Chopra, S., and Kumar, D. (2020). Ibuprofen as an emerging organic contaminant in environment, distribution and remediation. Heliyon 6 (6), e04087. doi:10.1016/j.heliyon.2020.e04087
Das, S. A., Karmakar, S., Chhaba, B., and Rout, S. K. (2019). Ibuprofen: its toxic effect on aquatic organisms. J. Exp. Zoology India 22 (2).
De Lange, H. J., Noordoven, W., Murk, A. J., Lürling, M. F., and Peeters, E. T. (2006). Behavioural responses of Gammarus pulex (Crustacea, Amphipoda) to low concentrations of pharmaceuticals. Aquat. Toxicol. 78 (3), 209–216. doi:10.1016/j.aquatox.2006.03.002
de Ruijter, V. N., Redondo-Hasselerharm, P. E., Gouin, T., and Koelmans, A. A. (2020). Quality criteria for microplastic effect studies in the context of risk assessment: a critical review. Environ. Sci. and Technol. 54 (19), 11692–11705. doi:10.1021/acs.est.0c03057
Di Poi, C., Costil, K., Bouchart, V., and Halm-Lemeille, M. P. (2018). Toxicity assessment of five emerging pollutants, alone and in binary or ternary mixtures, towards three aquatic organisms. Environ. Sci. Pollut. Res. 25, 6122–6134. doi:10.1007/s11356-017-9306-9
Elias, D., and Bernot, M. J. (2017). Effects of individual and combined pesticide commercial formulations exposure to egestion and movement of common freshwater snails, Physa acuta and Helisoma anceps. Am. Midl. Nat. 178 (1), 97–111. doi:10.1674/0003-0031-178.1.97
Falfushynska, H., Poznanskyi, D., Kasianchuk, N., Horyn, O., and Bodnar, O. (2022). Multimarker responses of Zebrafish to the effect of ibuprofen and gemfibrozil in environmentally relevant concentrations. Bull. Environ. Contam. Toxicol. 109 (6), 1010–1017. doi:10.1007/s00128-022-03607-2
Fent, K., Weston, A. A., and Caminada, D. (2006). Ecotoxicology of human pharmaceuticals. Aquat. Toxicol. 76 (2), 122–159. doi:10.1016/j.aquatox.2005.09.009
Früh, D., Haase, P., and Stoll, S. (2017). Temperature drives asymmetric competition between alien and indigenous freshwater snail species, Physa acuta and Physa fontinalis. Aquat. Sci. 79 (1), 187–195. doi:10.1007/s00027-016-0489-9
Garlick-Ott, K., and Wright, J. C. (2022). Factors shaping gender role in the freshwater hermaphrodite snail Physella acuta. Am. Malacol. Bull. 39 (1), 1–7. doi:10.4003/006.039.0101
Gilioli, G., Schrader, G., Carlsson, N., van Donk, E., van Leeuwen, C. H., Martín, P. R., et al. (2017). Environmental risk assessment for invasive alien species: a case study of apple snails affecting ecosystem services in Europe. Environ. Impact Assess. Rev. 65, 1–11. doi:10.1016/j.eiar.2017.03.008
Goldstein, M. C., Rosenberg, M., and Cheng, L. (2012). Increased oceanic microplastic debris enhances oviposition in an endemic pelagic insect. Biol. Lett. 8 (5), 817–820. doi:10.1098/rsbl.2012.0298
González-Pleiter, M., Tamayo-Belda, M., Pulido-Reyes, G., Amariei, G., Leganés, F., Rosal, R., et al. (2019). Secondary nanoplastics released from a biodegradable microplastic severely impact freshwater environments. Environ. Sci. Nano 6 (5), 1382–1392. doi:10.1039/c8en01427b
Gonzalez-Rey, M., and Bebianno, M. J. (2012). Does non-steroidal anti-inflammatory (NSAID) ibuprofen induce antioxidant stress and endocrine disruption in mussel Mytilus galloprovincialis? Environ. Toxicol. Pharmacol. 33 (2), 361–371. doi:10.1016/j.etap.2011.12.017
Grueber, C. E., Fox, S., McLennan, E. A., Gooley, R. M., Pemberton, D., Hogg, C. J., et al. (2019). Complex problems need detailed solutions: harnessing multiple data types to inform genetic management in the wild. Evol. Appl. 12 (2), 280–291. doi:10.1111/eva.12715
Guzzetti, E., Sureda, A., Tejada, S., and Faggio, C. (2018). Microplastic in marine organism: environmental and toxicological effects. Environ. Toxicol. Pharmacol. 64, 164–171. doi:10.1016/j.etap.2018.10.009
Hall, R. O., Tank, J. L., and Dybdahl, M. F. (2003). Exotic snails dominate nitrogen and carbon cycling in a highly productive stream. Front. Ecol. Environ. 1 (8), 407–411. doi:10.2307/3868137
Henry, J., Brand, J. A., Bai, Y., Martin, J. M., Wong, B. B., and Wlodkowic, D. (2022). Multi-generational impacts of exposure to antidepressant fluoxetine on behaviour, reproduction, and morphology of freshwater snail Physa acuta. Sci. Total Environ. 814, 152731. doi:10.1016/j.scitotenv.2021.152731
Imhof, H. K., Ivleva, N. P., Schmid, J., Niessner, R., and Laforsch, C. (2013). Contamination of beach sediments of a subalpine lake with microplastic particles. Curr. Biol. 23 (19), R867–R868. doi:10.1016/j.cub.2013.09.001
Issac, M. N., and Kandasubramanian, B. (2021). Effect of microplastics in water and aquatic systems. Environ. Sci. Pollut. Res. 28, 19544–19562. doi:10.1007/s11356-021-13184-2
Iwata, T. (2015). Biodegradable and bio-based polymers: future prospects of eco-friendly plastics. Angewandte Chemie Int. ed. Engl. 54 (11), 3210–3215. doi:10.1002/anie.201410770
Jan-Roblero, J., and Cruz-Maya, J. A. (2023). Ibuprofen: toxicology and biodegradation of an emerging contaminant. Molecules 28 (5), 2097. doi:10.3390/molecules28052097
Jeong, C. B., Won, E. J., Kang, H. M., Lee, M. C., Hwang, D. S., Hwang, U. K., et al. (2016). Microplastic size-dependent toxicity, oxidative stress induction, and p-JNK and p-p38 activation in the monogonont rotifer (Brachionus koreanus). Environ. Sci. and Technol. 50 (16), 8849–8857. doi:10.1021/acs.est.6b01441
Jeyavani, J., and Vaseeharan, B. (2023). Combined toxic effects of environmental predominant microplastics and ZnO nanoparticles in freshwater snail Pomaceae paludosa. Environ. Pollut. 325, 121427. doi:10.1016/j.envpol.2023.121427
Justice, J. R., and Bernot, R. J. (2014). Nanosilver inhibits freshwater gastropod (Physa acuta) ability to assess predation risk. Am. Midl. Nat. 171 (2), 340–349. doi:10.1674/0003-0031-171.2.340
Konschak, M., Zubrod, J. P., Duque Acosta, T. S., Bouchez, A., Kroll, A., Feckler, A., et al. (2021). Herbicide-induced shifts in the periphyton community composition indirectly affect feeding activity and physiology of the gastropod grazer Physella acuta. Environ. Sci. and Technol. 55 (21), 14699–14709. doi:10.1021/acs.est.1c01819
Kovacevic, V., Simpson, A. J., and Simpson, M. J. (2016). 1H NMR-based metabolomics of Daphnia magna responses after sub-lethal exposure to triclosan, carbamazepine and ibuprofen. Comp. Biochem. Physiology Part D Genomics Proteomics 19, 199–210. doi:10.1016/j.cbd.2016.01.004
Krueger, M. C., Harms, H., and Schlosser, D. (2015). Prospects for microbiological solutions to environmental pollution with plastics. Appl. Microbiol. Biotechnol. 99, 8857–8874. doi:10.1007/s00253-015-6879-4
Krupski, A., Karasek, T., and Koperski, P. (2018). Differences between two physid species (Gastropoda: Physidae) in antipredator behaviour induced by leeches. J. Molluscan Stud. 84 (1), 96–102. doi:10.1093/mollus/eyx049
Kumar, M., Chen, H., Sarsaiya, S., Qin, S., Liu, H., Awasthi, M. K., et al. (2021). Current research trends on micro-and nano-plastics as an emerging threat to global environment: a review. J. Hazard. Mater. 409, 124967. doi:10.1016/j.jhazmat.2020.124967
Kumari, N., Samantaray, B. P., Patel, A., and Kumar, R. (2023). Microplastics affect rates of locomotion and reproduction via dietary uptake in globally invasive snail Physa acuta. Water 15 (5), 928. doi:10.3390/w15050928
Lambert, S., and Wagner, M. (2018). Microplastics are contaminants of emerging concern in freshwater environments: an overview. Springer International Publishing, 1–23.
Lenth, R. (2024). emmeans: estimated marginal means, aka least-squares means. R package version 1.10.4. Available at: https://CRAN.R-project.org/package=emmeans (Accessed October 17, 2024).
Li, Y., Tao, L., Wang, Q., Wang, F., Li, G., and Song, M. (2023). Potential health impact of microplastics: a review of environmental distribution, human exposure, and toxic effects. Environ. and Health 1 (4), 249–257. doi:10.1021/envhealth.3c00052
Lin, W., Luo, H., Wu, J., Liu, X., Cao, B., Liu, Y., et al. (2023). Polystyrene microplastics enhance the microcystin-LR-induced gonadal damage and reproductive endocrine disruption in zebrafish. Sci. Total Environ. 876, 162664. doi:10.1016/j.scitotenv.2023.162664
Lönnstedt, O. M., and Eklöv, P. (2016). RETRACTED: environmentally relevant concentrations of microplastic particles influence larval fish ecology. Science 352 (6290), 1213–1216. doi:10.1126/science.aad8828
Lopez, S., Rousset, F., Shaw, F. H., Shaw, R. G., and Ronce, O. (2009). Joint effects of inbreeding and local adaptation on the evolution of genetic load after fragmentation. Conserv. Biol. 23 (6), 1618–1627. doi:10.1111/j.1523-1739.2009.01326.x
Lowe, R. L., and Hunter, R. D. (1988). Effect of grazing by Physa integra on periphyton community structure. J. North Am. Benthol. Soc. 7 (1), 29–36. doi:10.2307/1467828
Luo, Y., Guo, W., Ngo, H. H., Nghiem, L. D., Hai, F. I., Zhang, J., et al. (2014). A review on the occurrence of micropollutants in the aquatic environment and their fate and removal during wastewater treatment. Sci. total Environ. 473, 619–641. doi:10.1016/j.scitotenv.2013.12.065
Majewsky, M., Bitter, H., Eiche, E., and Horn, H. (2016). Determination of microplastic polyethylene (PE) and polypropylene (PP) in environmental samples using thermal analysis (TGA-DSC). Sci. Total Environ. 568, 507–511. doi:10.1016/j.scitotenv.2016.06.017
Makuch, E., Ossowicz-Rupniewska, P., Klebeko, J., and Janus, E. (2021). Biodegradation of L-valine alkyl ester ibuprofenates by bacterial cultures. Materials 14 (12), 3180. doi:10.3390/ma14123180
Mason, V. G., Skov, M. W., Hiddink, J. G., and Walton, M. (2022). Microplastics alter multiple biological processes of marine benthic fauna. Sci. Total Environ. 845, 157362. doi:10.1016/j.scitotenv.2022.157362
McClain, C. R., Gullett, T., Jackson-Ricketts, J., and Unmack, P. J. (2012). Increased energy promotes size-based niche availability in marine mollusks. Evolution 66 (7), 2204–2215. doi:10.1111/j.1558-5646.2012.01580.x
Meaza, I., Toyoda, J. H., and Wise Sr, J. P. (2021). Microplastics in sea turtles, marine mammals and humans: a one environmental health perspective. Front. Environ. Sci. 8, 575614. doi:10.3389/fenvs.2020.575614
Merbt, S. N., Kroll, A., Sgier, L., Tlili, A., Schirmer, K., and Behra, R. (2024). Fate and effects of microplastic particles in a periphyton-grazer system. Environ. Pollut. 347, 123798. doi:10.1016/j.envpol.2024.123798
Michalaki, A., and Grintzalis, K. (2023). Acute and transgenerational effects of non-steroidal anti-inflammatory drugs on Daphnia magna. Toxics 11 (4), 320. doi:10.3390/toxics11040320
Michler-Kozma, D. N., Neu, T. R., and Gabel, F. (2022). Environmental conditions affect the food quality of plastic associated biofilms for the benthic grazer Physa fontinalis. Sci. Total Environ. 816, 151663. doi:10.1016/j.scitotenv.2021.151663
Miranda, M. O., Cavalcanti, W. E. C., Barbosa, F. F., de Sousa, J. A., da Silva, F. I., Pergher, S. B., et al. (2021). Photocatalytic degradation of ibuprofen using titanium oxide: insights into the mechanism and preferential attack of radicals. RSC Adv. 11 (44), 27720–27733. doi:10.1039/d1ra04340d
Mishra, A. K., Singh, J., and Mishra, P. P. (2021). Microplastics in polar regions: an early warning to the world's pristine ecosystem. Sci. Total Environ. 784, 147149. doi:10.1016/j.scitotenv.2021.147149
Mulholland, P. J., Steinman, A. D., Palumbo, A. V., Elwood, J. W., and Kirschtel, D. B. (1991). Role of nutrient cycling and herbivory in regulating periphyton communities in laboratory streams. Ecology 72 (3), 966–982. doi:10.2307/1940597
Muñiz-González, A. B. (2021). Ibuprofen as an emerging pollutant on non-target aquatic invertebrates: effects on Chironomus riparius. Environ. Toxicol. Pharmacol. 81, 103537. doi:10.1016/j.etap.2020.103537
Naldi, M., Nizzoli, D., Bartoli, M., Viaroli, P., and Viaroli, P. (2020). Effect of filter-feeding mollusks on growth of green macroalgae and nutrient cycling in a heavily exploited coastal lagoon. Estuar. Coast. Shelf Sci. 239, 106679. doi:10.1016/j.ecss.2020.106679
Nandy, G., Paul, P., Tarannum, N., and Aditya, G. (2024). Effect of pollutants on the oviposition and embryo development of the invasive snail Physella acuta (Gastropoda: Physidae). Invertebr. Reproduction and Dev. 68, 36–45. doi:10.1080/07924259.2024.2335923
Negres, S. (2019). Ibuprofen, a drug used in pain, inflammation and fever. Romanian J. Pediatr. 68 (1), 18–21. doi:10.37897/rjp.2019.1.4
Newman, R. M., Kerfoot, W. C., and Hanscom, Z. (1996). Watercress allelochemical defends high-nitrogen foliage against consumption: effects on freshwater invertebrate herbivores. Ecology 77 (8), 2312–2323. doi:10.2307/2265733
Núñez, V. (2010). Differences on allocation of available resources, in growth, reproduction, and survival, in an exotic gastropod of Physidae compared to an endemic one. Iheringia. Série Zool. 100, 275–279. doi:10.1590/s0073-47212010000300014
Oliveira, M., Ribeiro, A., Hylland, K., and Guilhermino, L. (2013). Single and combined effects of microplastics and pyrene on juveniles (0+ group) of the common goby Pomatoschistus microps (Teleostei, Gobiidae). Ecol. Indic. 34, 641–647. doi:10.1016/j.ecolind.2013.06.019
Pantos, O. (2022). Microplastics: impacts on corals and other reef organisms. Emerg. Top. Life Sci. 6 (1), 81–93. doi:10.1042/etls20210236
Pedersen, A. F., Gopalakrishnan, K., Boegehold, A. G., Peraino, N. J., Westrick, J. A., and Kashian, D. R. (2020). Microplastic ingestion by quagga mussels, Dreissena bugensis, and its effects on physiological processes. Environ. Pollut. 260, 113964. doi:10.1016/j.envpol.2020.113964
Perrotta, B. G., Simonin, M., Back, J. A., Anderson, S. M., Avellan, A., Bergemann, C. M., et al. (2020). Copper and gold nanoparticles increase nutrient excretion rates of primary consumers. Environ. Sci. and Technol. 54 (16), 10170–10180. doi:10.1021/acs.est.0c02197
Petrie, B., and Camacho-Muñoz, D. (2021). Analysis, fate and toxicity of chiral non-steroidal anti-inflammatory drugs in wastewaters and the environment: a review. Environ. Chem. Lett. 19 (1), 43–75. doi:10.1007/s10311-020-01065-y
Pharms UBA Umwelt Bundesamt (2024). Database-pharmaceuticals in the environment. Available at: https://www.umweltbundesamt.de (Accessed October 6, 2024).
Prata, J. C., da Costa, J. P., Lopes, I., Duarte, A. C., and Rocha-Santos, T. (2020). Environmental exposure to microplastics: an overview on possible human health effects. Sci. total Environ. 702, 134455. doi:10.1016/j.scitotenv.2019.134455
Prieto-Amador, M., Caballero, P., and Martinez-Guitarte, J. L. (2021). Analysis of the impact of three phthalates on the freshwater gastropod Physella acuta at the transcriptional level. Sci. Rep. 11 (1), 11411. doi:10.1038/s41598-021-90934-9
R Core Team (2024). R: a language and environment for statistical computing. Vienna: R Foundation for Statistical Computing. Available at: https://www.R-project.org/(Accessed October 17, 2024).
Ritchie, H., Samborska, V., and Roser, M. (2023). Plastic pollution. Oxford, United Kingdom: Our World in Data. Available at: https://ourworldindata.org/plastic-pollution (Accessed November 18, 2024).
Rivi, V., Batabyal, A., Juego, K., Kakadiya, M., Benatti, C., Blom, J. M., et al. (2021). To eat or not to eat: a Garcia effect in pond snails (Lymnaea stagnalis). J. Comp. Physiology A 207, 479–495. doi:10.1007/s00359-021-01491-5
Rochman, C. M., Kurobe, T., Flores, I., and Teh, S. J. (2014). Early warning signs of endocrine disruption in adult fish from the ingestion of polyethylene with and without sorbed chemical pollutants from the marine environment. Sci. total Environ. 493, 656–661. doi:10.1016/j.scitotenv.2014.06.051
Sadigov, R. (2022). Rapid growth of the world population and its socioeconomic results. Sci. World J. 2022 (1), 1–8. doi:10.1155/2022/8110229
Saha, C., Pramanik, S., Chakraborty, J., Parveen, S., and Aditya, G. (2019). Life history features of the invasive snail Physa acuta Occurring in Kolkata, India. Natl. Acad. Sci. Lett. 42, 95–98. doi:10.1007/s40009-018-0709-z
Sánchez-Argüello, P., Fernández, C., and Tarazona, J. V. (2009). Assessing the effects of fluoxetine on Physa acuta (Gastropoda, Pulmonata) and Chironomus riparius (Insecta, Diptera) using a two-species water–sediment test. Sci. total Environ. 407 (6), 1937–1946. doi:10.1016/j.scitotenv.2008.12.004
Santillo, D., Miller, K., and Johnston, P. (2017). Microplastics as contaminants in commercially important seafood species. Integr. Environ. Assess. Manag. 13 (3), 516–521. doi:10.1002/ieam.1909
Schmidt, W., and Redshaw, C. H. (2015). Evaluation of biological endpoints in crop plants after exposure to non-steroidal anti-inflammatory drugs (NSAIDs): implications for phytotoxicological assessment of novel contaminants. Ecotoxicol. Environ. Saf. 112, 212–222. doi:10.1016/j.ecoenv.2014.11.008
Shah, A. A., Hasan, F., Hameed, A., and Ahmed, S. (2008). Biological degradation of plastics: a comprehensive review. Biotechnol. Adv. 26 (3), 246–265. doi:10.1016/j.biotechadv.2007.12.005
Shetty, S. S., Deepthi, D., Harshitha, S., Sonkusare, S., Naik, P. B., Madhyastha, H., et al. (2023). Environmental pollutants and their effects on human health. Heliyon 9 (9), e19496. doi:10.1016/j.heliyon.2023.e19496
Sibiya, A., Jeyavani, J., Saravanan, M., Albeshr, M. F., Nicoletti, M., Govindarajan, M., et al. (2023). Response of hepatic biochemical parameters and neurotoxicity to carbamazepine and ibuprofen in Oreochromis mossambicus. Fish Physiology Biochem. 49 (5), 787–799. doi:10.1007/s10695-023-01172-x
Silva, P. V., Van Gestel, C. A., Verweij, R. A., Papadiamantis, A. G., Goncalves, S. F., Lynch, I., et al. (2020). Toxicokinetics of pristine and aged silver nanoparticles in Physa acuta. Environ. Sci. Nano 7 (12), 3849–3868. doi:10.1039/d0en00946f
Srain, H. S., Beazley, K. F., and Walker, T. R. (2021). Pharmaceuticals and personal care products and their sublethal and lethal effects in aquatic organisms. Environ. Rev. 29 (2), 142–181. doi:10.1139/er-2020-0054
Swamikannu, X., and Hoagland, K. D. (1989). Effects of snail grazing on the diversity and structure of a periphyton community in a eutrophic pond. Can. J. Fish. Aquatic Sci. 46 (10), 1698–1704. doi:10.1139/f89-215
Szűcs, M., Melbourne, B. A., Tuff, T., Weiss-Lehman, C., and Hufbauer, R. A. (2017). Genetic and demographic founder effects have long-term fitness consequences for colonising populations. Ecol. Lett. 20 (4), 436–444. doi:10.1111/ele.12743
Tchakonte, S., Nana, P. A., Tamsa, A. A., Tchatcho, N. L. N., Koji, E., Onana, F. M., et al. (2023). Using machine learning models to assess the population dynamic of the freshwater invasive snail Physa acuta Draparnaud, 1805 (Gastropoda: Physidae) in a tropical urban polluted streams-system. Limnologica 99, 126049. doi:10.1016/j.limno.2022.126049
Thammineni, N., Kathi, P. R., Sharma, A., and Jawed, A. (2019). Ibuprofen overuse leading to life-threatening hypokalemia associated with renal tubular acidosis in two patients. Cureus 11 (12), e6404. doi:10.7759/cureus.6404
Vannatta, J. T. (2021). Community and ecosystem level implications of helminth parasitism. West Lafayette, Indiana, USA: Purdue University. Doctoral dissertation.
Wikström, S. A., and Hillebrand, H. (2012). Invasion by mobile aquatic consumers enhances secondary production and increases top-down control of lower trophic levels. Oecologia 168, 175–186. doi:10.1007/s00442-011-2061-6
Yang, X., Xu, X., Wei, X., Wan, J., and Zhang, Y. (2019). Biomarker effects in carassius auratus exposure to ofloxacin, sulfamethoxazole and ibuprofen. Int. J. Environ. Res. public health 16 (9), 1628. doi:10.3390/ijerph16091628
Zhang, J., Gao, D., Li, Q., Zhao, Y., Li, L., Lin, H., et al. (2020). Biodegradation of polyethylene microplastic particles by the fungus Aspergillus flavus from the guts of wax moth Galleria mellonella. Sci. Total Environ. 704, 135931. doi:10.1016/j.scitotenv.2019.135931
Keywords: ibuprofen, microplastics, Physella acuta, ecotoxicology, reproduction, aquatic pollutants
Citation: Elías D, Ramirez Vieytez J, Funoy Sayar M, Loaisiga J, Harper A and Doll J (2025) Effects of ibuprofen and microplastics on movement, growth and reproduction in the freshwater snail Physella acuta. Front. Environ. Sci. 12:1514062. doi: 10.3389/fenvs.2024.1514062
Received: 19 October 2024; Accepted: 13 December 2024;
Published: 06 January 2025.
Edited by:
Ping Li, Shandong University, Weihai, ChinaReviewed by:
Mohamed Ahmed Ibrahim Ahmed, Assiut University, EgyptCopyright © 2025 Elías, Ramirez Vieytez, Funoy Sayar, Loaisiga, Harper and Doll. This is an open-access article distributed under the terms of the Creative Commons Attribution License (CC BY). The use, distribution or reproduction in other forums is permitted, provided the original author(s) and the copyright owner(s) are credited and that the original publication in this journal is cited, in accordance with accepted academic practice. No use, distribution or reproduction is permitted which does not comply with these terms.
*Correspondence: Daniel Elías, ZGVsaWFzQG5jd3UuZWR1
Disclaimer: All claims expressed in this article are solely those of the authors and do not necessarily represent those of their affiliated organizations, or those of the publisher, the editors and the reviewers. Any product that may be evaluated in this article or claim that may be made by its manufacturer is not guaranteed or endorsed by the publisher.
Research integrity at Frontiers
Learn more about the work of our research integrity team to safeguard the quality of each article we publish.