- 1Anhui Provincial Academy of Eco-Environmental Science Research, Hefei, China
- 2School of Environmental Science and Engineering, Hefei Institute of Technology, Hefei, China
- 3CAS Key Laboratory of Crust-Mantle Materials and the Environment, School of Earth and Space Sciences, University of Science and Technology of China, Hefei, China
Emerging contaminants (ECs), such as polyfluorinated compounds, antibiotics, microplastics, and nonylphenol, continue to challenge environmental management practices due to their persistence and bioaccumulation potential. This review articulates the critical pathways and environmental risks posed by these contaminants, setting the stage for an in-depth exploration of innovative removal technologies. We spotlight groundbreaking methods that are reshaping the landscape of ECs remediation: membrane filtration technology, constructed wetlands, adsorptive materials, algae-based systems, biological treatments, and advanced oxidation processes. Each method is evaluated for its efficacy in removing ECs, with particular emphasis on sustainability and economic viability. Our findings reveal that integrating these technologies can significantly enhance removal efficiency, offering new directions for environmental policy and practical applications. This article positions these advanced removal technologies at the forefront of the fight against ECs, advocating for their broader adoption to safeguard environmental and public health.
1 Introduction
In the past hundred years, the world has experienced great development. The rapid development of industry, agriculture, and urbanization have brought economic benefits and aggravated environmental issues (Mafi et al., 2013). For instance, deleterious solid waste, inadequate sewage treatment, the utilization of non-biodegradable materials, and detrimental greenhouse gases constitute contemporary environmental concerns. Historically, prevalent pollutants encompassed heavy metals, organochlorine pesticides, sulfur dioxide, PM2.5, and other contaminants. Subsequently, there are many kinds of pollutants without relevant laws and regulations, called emerging contaminants. Many classes of drugs are essential to modern society, such as antibiotics, endocrine disruptors, fluorocarbon and others.
A typical example is antibiotics, which have led to the antibiotic revolution since the discovery of penicillin by Alexander Fleming in 1929, as well as the subsequent development of purification of penicillin by Howard Florey and the Bavarian chain company Ernest of Bavaria chain during World War II (Carvalho and Santos, 2016). Antibiotics are present in rivers, lakes, and oceans globally, causing problems such as resistance genes and endocrine disorders. However, for China or some developing countries, these pollutants may not be officially classified as such due to economic, legal, or other considerations. Take antibiotics or perfluorooctane sulfonate (PFOS) for instance, which has been detected in estuarine sediments and water bodies worldwide, as well as in mammalian tissues and human serum, and is widely recognized for its persistence in the environment (Fernandes et al., 2020).
In addition, some endocrine disruptors are the main factors causing pollution today. In 1995, endocrine disruptors became a scientific concept and it was discovered that these pollutants can cause harm to the biological reproductive system and hormone function (Seralini and Jungers, 2021). Artificial sweeteners (ASs) are widely used as sugar substitutes in human diet and animal feed due to their high intensity of sweetness. The use of ASs in all foods was banned by the US Food and Drug Administration (FDA) in 1970, because of its potential carcinogenic effect on experimental animals. However, in Asia, for example, China is the main user of artificial sweeteners (Luo et al., 2019). Although ASs have been found in various environmental media worldwide due to their extensive and sustained use, their ecological toxicity has not received much attention (Buerge et al., 2024). Under the attention of scholars from around the world, the toxicity of some ECs can be estimated using formulas. With the attention of scholars from all over the world, the toxicity of some ECs can be estimated using formulas. However, current research on the mixed toxicity of ECs remains unclear. While acknowledging the role of predictive models in estimating the toxicity of certain ECs, it is imperative to also consider the extensive toxicity data that exists. Additionally, the assertion that research on mixed toxicity is unclear may not fully represent ongoing efforts in the field, as evidenced by recent studies which contribute to our understanding of combined toxic effects (Chen et al., 2022a). While advanced methodologies for the systematic detection and management of ECs have been developed, their practical application is hindered by economic and regulatory challenges, particularly at the real-scale deployment stage. This nuance is essential for a comprehensive discussion on the state of ECs management and research.
In recent years, the study of ECs has covered various aspects, and scholars’ opinions constitute a valuable source of information. As can be seen in Figure 1, research related to emerging contaminants/emerging contaminants removal has been steadily increasing over the past decade. Nevertheless, owing to the extensive reach, myriad origins, and intricate pollution mechanisms of emerging contaminants, effective control and investigation require collaborative efforts across various sectors to mitigate potential and immediate risks to both human health and ecological integrity. In light of these circumstances, the objective of this study is to assemble a comprehensive inventory of emerging pollutants and delve into their toxicity profiles, as well as explore methodologies for their removal and mitigation.
2 Sources and routes of emerging contaminants
Emerging contaminants are known to be toxic, resistant to degradation, prone to bioaccumulation, and often capable of crossing international borders via air, water, and migratory species. Figure 2 illustrates the sources of emerging contaminants. They can deposit far from their emission sites and subsequently accumulate in terrestrial and aquatic ecosystems. Industries such as pharmaceuticals, printing, electroplating, beauty products, chemical manufacturing, antibiotics, and cleaning agents contribute to the release of these pollutants into water, soil, and air. Similar to traditional sources of pollution, they pose significant threats to the environment. The toxicity and physicochemical properties of these substances, regardless of their form or source, play crucial roles in determining how they interact within the biosphere and influence natural transformation processes.
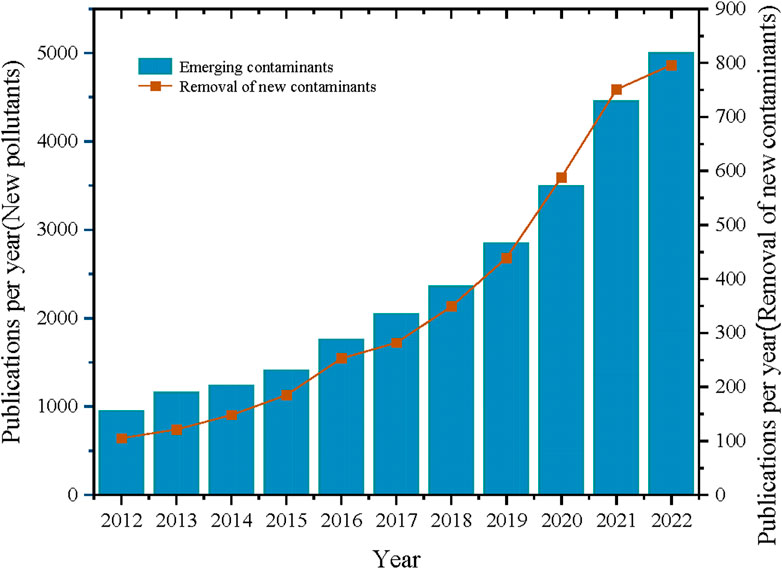
Figure 2. Evolution of the number of ECs and new pollutant removal publications between 2012 and 2022(The data comes from the web of sciense).
It is evident that wastewater pollution sources and the infiltration into groundwater significantly contribute to the presence of ECs in natural water bodies, particularly in areas with poor wastewater treatment oversight and underdeveloped regions. Hospitals, pharmaceutical companies, domestic sewage, industrial wastewater, and aquaculture are major sources of ECs. Furthermore, due to the complex physicochemical properties of ECs, wastewater treatment plants struggle to effectively remove them. For instance, an antibiotic concentration of 13.6 μg/L was detected in a wastewater treatment plant in Wisconsin, USA (Sarmah et al., 2006). Landfill leachate is also a significant pollution pathway and serves as a major reservoir for microplastics, which substantially increase environmental threats (V et al., 2023). Besides posing environmental risks, ECs also facilitate the spread of antibiotic resistance genes. Through the exchange of surface water and groundwater runoff, ECs can cause additive or synergistic toxic effects, necessitating appropriate regulatory oversight or the development of treatment technologies.
3 Known and emerging contaminants
Emerging contaminants, released into the environment, exhibit biotoxicity, environmental persistence, and bioaccumulative properties, thus representing significant risks to ecological health and human wellbeing. This category includes perfluorinated compounds, recognized as persistent organic pollutants under the Stockholm Convention and used predominantly as flame retardants. Additionally, other substances such as bisphenol A (an endocrine disruptor), pharmaceuticals (notably antibiotics), and microplastics, although not listed by the Convention, are of paramount concern. Their widespread prevalence, coupled with the complexities involved in their detection and the necessity for extensive pretreatment processes, underscores the critical importance of continued research and management efforts concerning these contaminants.
3.1 Polyfluorinated compounds
Polyfluorinated Compounds (PFCs) are a new type of persistent organic pollutants. Each hydrogen atom in the carbon chain of a compound molecule is replaced by a fluorine atom (Table 1). The general formula is F(CF2) N-R, where R is the hydrophilic functional group. PFCs have excellent physical and chemical properties and are widely used in industrial production and daily life. They have been detected in the atmosphere, water, soil, sediment, organisms and even polar ice fields. The majority of per- and polyfluoroalkyl substances exhibit extraordinary durability, as they resist hydrolysis, photodegradation, and biodegradation across a spectrum of environmental conditions (Le et al., 2018). It is evident that the likelihood of detecting PFAS in aquatic ecosystems is notably elevated, owing to significant discharges into rivers, lakes, groundwater, and various other aquatic habitats. Beyond these aquatic domains, PFAS compounds are frequently encountered in other environmental matrices such as suspended particulate matter, sediment, and similar substrates (Liu et al., 2021). PFCs are highly bioaccumulative and potentially toxic to reproduction. The accumulation of PFCS in the environment also threatens the microbial community. The effect of long chain PFCs on soil bacterial community composition was greater than that of short chain PFCs. The results showed that PFCS pollution also greatly affected the survival of several major bacterial genera in aquatic ecosystems (Wu et al., 2021). The solubility of PFCs in water plays an important role in its toxicity. In fact, slow dissolution processes may lead to slow accumulation and metabolism, as well as an increase in the half-life of PFC. It is imperative to underscore that, apart from their high affinity for organics dispersed in water and aquatic species, the omnipresence and enduring nature of PFCs in aquatic environments present formidable challenges. For instance, the half-life periods of perfluorooctanoic acid and perfluorooctane sulfonate (PFOS) are approximately 40 and 90 years, respectively, underscoring the formidable persistence of these molecules. Eradicating them poses significant difficulties. Moreover, in aquatic organisms, half-lives can vary depending on metabolic pathways and are particularly influenced by the functional groups present (Savoca and Pace, 2021). Furthermore, the half-life of these compounds may also vary based on the size and species of the organism, owing to cumulative effects within the food chain. For instance, the half-life of PFOS is reported to be 5 months in dolphins and 29–31 days in marbled flounder (Chisaki, 2005; Sakurai et al., 2013).
3.2 Antibiotic
The development of modern antibiotics dates back to pivotal moments in the late 1920s and 1930s. In 1929, Fleming’s discovery of penicillin marked a significant milestone, followed by the revelation of sulfonamide (SAs) as the inaugural broad-spectrum antibiotic in clinical practice in 1935. The industrial-scale production and commercialization of penicillin began in 1946, marking the beginning of the era widely recognized as the antibiotic age. Subsequently, a plethora of antibiotics emerged, revolutionizing the field of medicine (Carvalho and Santos, 2016). The antibiotics that are currently the most widely used and abundant in the environment include: tetracyclines, sulfonamides, quinolones, and macrolide. The presence of these antibiotics in the environment is mainly affected by factors such as human activities, animal husbandry and medical wastewater.
The aquatic presence of many antibiotics manifests as amphoteric ions, attributable to their distinct pka values, which intricately influence adsorption mechanisms and consequent environmental distribution. For example, tetracycline has three different pka values and is readily adsorbed on different solid particles and sediments. Compared to other antibiotics, sulfonamides have fewer functional groups, exhibit weaker interactions with soil and sediment, and possess lower biodegradability, making them more frequently detected in water (Fan et al., 2010). Notably, the solubility of antibiotics in water constitutes another pivotal determinant. For sulfonamide, the solubility of sulfadimidine in water is significantly higher than that of Sulfamethoxazole (1,500 and 610 mg/L, respectively). In contrast, fluoroquinolones and Macrolide are less soluble in water and can’t be permanently present in surface water. At all events, these antibiotics can be strongly adsorbed on sediment particles, which means that sediment eventually becomes a sink for antibiotics. For example, this is observed in mangrove forests in Takahashi, China (Li Y. et al., 2016).
Much literature has emphasized the influence of temperature on antibiotics, However, in natural settings, a variety of factors can affect the presence of antibiotics, even unstable β-lactam antibiotics, the longest half-life under natural conditions can also reach 27 days. The breakdown of antibiotics is highly dependent on pH, temperature, and the presence of hydrolysis-sensitive functional groups in the antibiotic structure (Mitchell et al., 2013). pH affects the adsorption and desorption of antibiotics mainly by affecting the existing form of ions. For example, norfloxacin may repel Ca2+ and Mg2+ ions in water, leading to its detection in large quantities in aquatic environments. In natural water samples, tetracycline and oxytetracycline inhibited photodegradation at pH 7.3, but the presence of Ca2+ and Mg2+ made tetracycline and oxytetracycline form metal ion complexes. The kinetics of antibiotics in water are related to their adsorptive solubility, and the degradation rate is largely dependent on the contribution of possible hydroxyl radicals and other unidentified reactive substances (Bahnmueller et al., 2014). Complex adsorption mechanisms, including hydrogen bonding and ion exchange, also contribute to antibiotic migration (Chen and Zhou, 2014). However, the specific mechanisms and reasons for the degradation or adsorption of different antibiotics need to be further studied.
3.3 Microplastics
Plastics with a diameter of less than 5 mm are classified as microplastics (MPs). Microplastics may originate from the degradation of personal care products and larger plastic items. Concurrently, owing to inadequate management, plastic waste undergoes photolysis, fragmentation, and microbial decomposition, generating secondary microplastics (Laskar and Kumar, 2019). Plastic fragments smaller than 100 nm are classified as nanoplastics. Despite the relatively large specific surface area of MPs, which facilitates adsorption across diverse environmental media and their migration, nanoplastics are not readily removed by standard water purification processes (Chen et al., 2022b). Figure 3 illustrates the path of microplastics production and the associated management practices. Physical separation in wastewater treatment plants remains the primary method for removing polyethylene (Yu et al., 2023). Regrettably, sludge from physical separation is often recycled for landfill or agricultural applications, leading to the re-introduction of MPs into water systems (Chen et al., 2022c). According to Sharma, nanoplastics are considered more harmful than microplastics. The aquatic environment may be subject to more complex contamination due to the possible adsorption of heavy metals or organic pollutants on the surfaces of microplastics and nanomaterials. More seriously, nanoplastic particles are small in size and can act as adherents to microbial surfaces, or enter microorganisms, affecting local microbial communities or generating cascading toxicity (Sharma et al., 2022). Owing to the absence of efficient microplastic removal techniques in sewage treatment, numerous microplastics continue to enter aquatic environments through this process. In addition, the MP removed from wastewater treatment is mainly retained in bottom sludge, which is mostly directly buried or further processed as farmland fertilizer. Consequently, these microplastics may reenter natural water bodies via soil erosion or surface runoff.
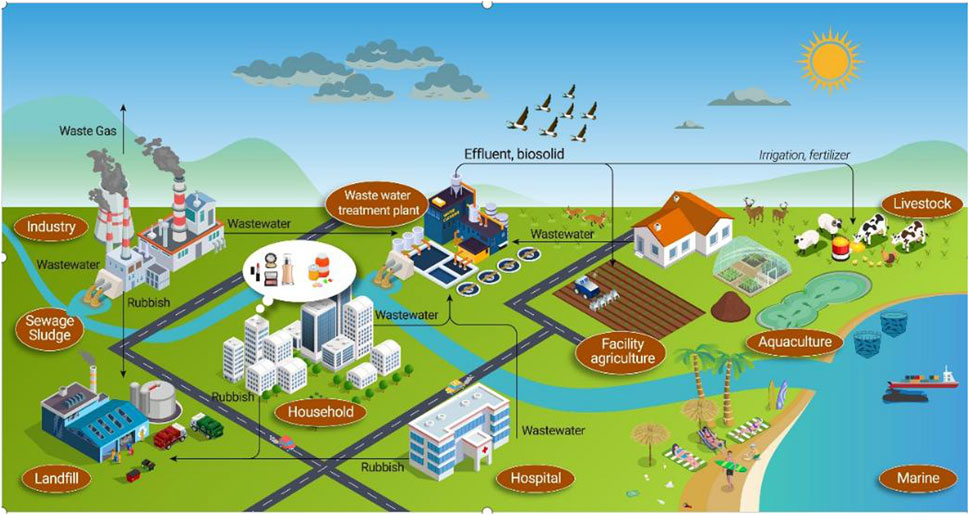
Figure 3. Sources and transmission pathways of emerging contaminants (Wang et al., 2024).
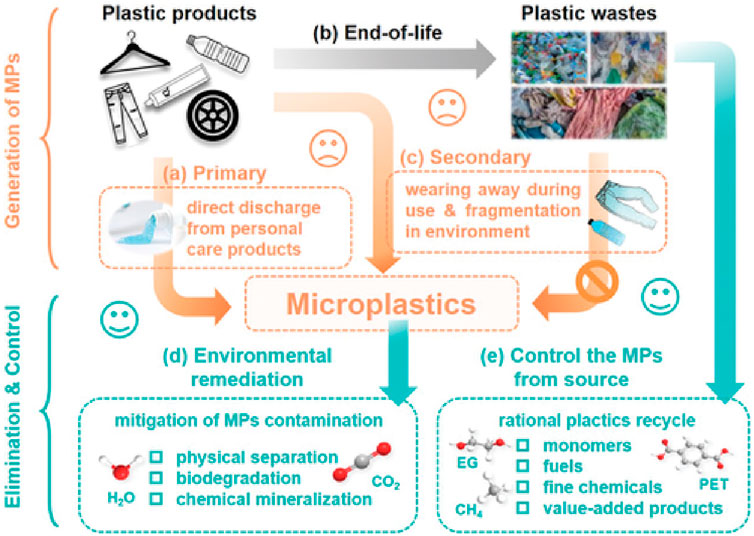
Figure 4. Microplastics production way, explain the process and management (Chen et al., 2022b).
3.4 Nonylphenol
Nonylphenol (NP) represents a broad class of isomeric compounds, each characterized by a nine-carbon alkyl chain bonded to a phenol ring, with the chemical formula C15H25O. The primary derivative of NP is nonylphenol ethoxylates (NPE). Organic compounds with a phenol group attached to a nine-carbon tail. The general formula is: C15H24O+ (OCH2CH2) nOH, where n may range from 1 to 100, but most NPE contains 6 to 12 ethoxy groups. NP is recognized as a potential endocrine-disrupting chemical impacting both human health and the environment. Nonylphenol was first synthesized in 1940 and its production and application continue to this day. Annually, the United States produces approximately 1,54,200 tons of NP, compared to 73,500 tons in Europe, 16,500 tons in Japan, and 16,000 tons in China. Current research indicates that China is a major producer of nonylphenol (Bhandari et al., 2021) (Figure 5). The most prevalent form of NP in the environment is branched-chain 4-nonylphenol. As a critical component in the production of nonionic surfactants, nonylphenol is commonly detected in water. However, water samples frequently underestimate the quantities of hydrophobic organic matter transported to sediments by particles. In addition, one-time or infrequent surface water sampling does not explain the temporal variation in potential toxic concentrations (Crane, 2021). Unlike some countries, in Japan, nonylphenol is designated as a criterion within the environmental quality standards for water pollution (Hong et al., 2020).
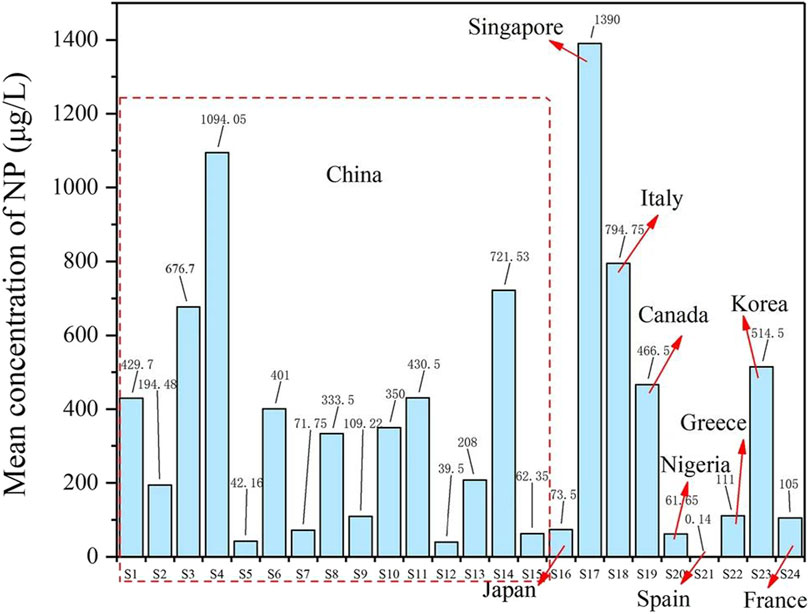
Figure 5. Distribution of nonylphenol in water environment of China and other countries. NP: nonylphenol. (S1: Yangtze River (Nanjing Section), S2: Yangtze River, S3: Yellow River, S4: Liao River–River, S5: Liao River-Reservoir, S6: Pearl River–River, S7: Pearl River-Reservoir, S8: Haihe-River, S9: Haihe-Reservoir, S10: Daliao River Estuary-Seawater, S11: Daliao River Estuary-Freshwater, S12: Sishili Bay and Taozi Bay-Seawater, S13: Sishili Bay and Taozi Bay-Freshwater, S14: Taihu Lake, S15: Chaohu Lake, S16: Japan, S17: Singapore, S18: Italy, S19: Canada, S20: Nigeria, S21: Spain, S22: Greece, S23: Korea, S24: France) (Hong et al., 2020).
4 Risk assessment of ECs
The chemical structure of a substance dictates its molecular and reactive properties, which in turn affect its toxicity and toxicological effects on humans, animals, and the environment. Specifically, the factors that affect the toxic properties of toxins include the following (Parida et al., 2021): 1. The functional groups and types of chemical bonds; 2. The spatial structure of the chemical substance; 3. The water-solubility and fat-solubility of the chemical substance; 4. Chemical substances with electrical properties, such as strong acids and bases, are prone to stimulation, while strong oxidants can cause oxidative stress reactions.
4.1 Ecological and environmental risks
The toxic substances that are excreted and left in the water body will become one of the main sources of antibiotics in the aquatic ecosystem. Research has shown that chemical compounds in water impact a variety of fungi, aquatic animals, and plants to varying degrees, including algae, fish, and nitrifying bacteria. In general, Predicted no-effect concentration (PNEC) values for different classes of antibiotics can be calculated using ECOSAR models or literature values to derive the extent to which antibiotics affect aquatic organisms (Formula 1).
Where MEC is the measured environmental concentration and PNEC is the predicted unaffected concentration, PENC can be expressed as follows:
This was determined using the ECOSAR model (Formula 2) (United States Environmental Protection Agency, 2011), which is widely used by researchers to assess the toxicity of organic compounds to aquatic organisms. General risk can be classified into three levels: high risk (RQ > 1), moderate risk (0.1 < RQ < 1), and low risk (0.01 < RQ < 0.1) (Krkstrm et al., 2020).
Enrofloxacin and Ciprofloxacin have been identified as posing significant risks to aquatic organisms in Laizhou Bay (Zhang et al., 2012). In fact, singular models are inadequate for predicting actual harm, as multiple compounds coexist in water and certain antibiotic combinations exhibit pronounced synergies. Liu et al. (2011) found that the coexistence of erythromycin, Ciprofloxacin and Sulfamethoxazole significantly reduced the growth rate, chlorophyll content, and photosynthetic rate of the freshwater algae Selenastrum capricornutum. A recent study indicates thatsildenafil (RQ = 3,048), lovastatin (RQ = 320), and trimethoprim (RQ = 74) in hospital wastewater pose high risks to animals and plants in the water body; However, the elevated risk associated with some compounds is attributed to their high concentrations, particularly those with significant ecotoxicological potential (Rodriguez-Rodriguez et al., 2023). In general, algae are the most sensitive species to antibiotics in water due to their high PNEC.
4.2 Hazards to human health
For certain ECs, the adjusted concentration can be calculated based on the Toxicity Equivalence Factor (TEF), and the lifetime lung cancer risk can be calculated using the WHO method.
The calculation of noncarcinogenic risk is as follows, divided into inhalation (Formula 3), nondietary intake (Formula 4), and skin contact (Formula 5). Common carcinogenic risks include substances such as polycyclic aromatic hydrocarbons, pesticides, and endocrine disruptors, among others.
5 Interaction of new pollutants and reactions with metals
The complexation of antibiotics with metals is influenced by numerous factors, resulting in diverse coordination outcomes. The main influencing factors include pH value, temperature, natural organic matter, salinity, properties of microplastics and coordination metal ions, among others. Different types of β-lactamases can render new-generation β-lactam antibiotics ineffective, among which metallo-β-lactamases (MBLs) are one of the main causes of widespread antibacterial resistance to carbapenem antibiotics. These metallohydrolases require at least one metal ion at the active site to coordinate with the essential nucleophile for hydrolysis (Möhler et al., 2017). Macrolide antibiotics typically function as monodentate ligands, binding to metal ions via hydroxyl groups on their smaller rings, in contrast to most other antibiotics, which are multidentate ligands (Wang et al., 2022). Tetracycline possesses multiple metal-binding sites, and its pharmacological activity largely depends on metal coordination. Berton found that the proportion of antibiotics that do not bind to proteins in plasma occurs almost exclusively in the form of calcium and magnesium complexes. Within cells, however, these drugs primarily coordinate with magnesium (II) ions, while calcium ions influence the absorption and bioavailability of tetracycline (Wendell et al., 2016).
Microplastic-mediated effects have also been reported. For example, it may play a mediating role between polycyclic hydrocarbons and antibiotics under multiple adsorption interactions. The surfaces of microplastics are capable of adsorbing polycyclic aromatic hydrocarbons and antibiotics, thereby facilitating their physical and chemical interactions. This medium may alter the distribution, transportation, and bioavailability of these substances, thereby affecting the ecosystem in the environment. Tong found that in the presence of Cu, there was a significant change in the adsorption of tetracycline and ciprofloxacin on microplastics, due to Cu-induced strong complexation between tetracycline and ciprofloxacin (Tong et al., 2023). Zhang employed ultraviolet light to simulate solar aging of microplastics, discovering that this aging significantly enhanced their capacity to adsorb levofloxacin hydrochloride. When the concentration of heavy metals in the environment is higher than that of antibiotics, heavy metals act as cation bridges and can form levofloxacin chromium complexes with levofloxacin, thereby promoting the adsorption process (Zhang et al., 2023; Zhou et al., 2022). Research indicates a significant positive correlation between microplastics and antibiotics resistance genes (ARGs), with microplastics increasing the frequency of bacterial gene transfer by 1.4–1.7 times, suggesting they may exacerbate the spread of antibiotic resistance genes in the environment (Yu et al., 2023). However, given the complexity of natural environments, the interactions among ECs warrant further investigation.
6 Removal methods for ECs
With the increasing maturity of technological means, there are various technologies for the treatment of new pollutants, such as metal-organic frameworks and biochar adsorption of organics, among others. The following chapters summarize and analyze various methods for the removal of pollutants.
6.1 Membrane filtration technology
A typical case provides comprehensive data on the occurrence of 19 antibiotics, 10 antibiotic-resistant bacteria, and 15 ARGs in the initial influent and different treatment stages of conventional activated sludge (CAS) and membrane Bioreactor (MBR) systems. Compared with CAS, MBR systems demonstrate superior removal efficiency. Specifically, Amoxicillin, Ciprofloxacin, Chloramphenicol, Meropenem, Minocycline, Azithromycin, Oxytetracycline, Sulfadimidine, and Vancomycin exhibited the highest removal rates in both CAS and MBR systems, with a median removal efficiency of 70%. No antibiotic-resistant bacteria were detected in the microfiltration permeate of the MBR system (Le et al., 2018). Compared with conventional membrane treatment technologies, electrochemical membrane filtration (EMF), a combination of electrochemical advanced oxidation process and low-pressure membrane filtration—has proven effective in degrading organic pollutants in wastewater, particularly persistent organic and micro-pollutants. This technology effectively removes pollutants in wastewater. The conventional membrane merely block the flow, while the electrochemical membrane can produce free radicals to degrade the pollutants and mineralize the pollutants into small molecules (Table 2). For instance, the high conductivity of carbon nanotubes facilitates their integration into hollow fiber membranes for combined membrane filtration and electrochemical processes (Yang et al., 2019). Compared with membrane filtration alone, the permeation flux of electrochemically assisted Carbon Nanotubes (CNTs)/AL2O3 membrane filtration was increased by 1.6 times, and the natural organic matter removal efficiency improved by 300% (Yang et al., 2019).
6.2 Constructed wetland
Constructed wetland (CW) are a sustainable treatment technology used for removing various metal and organic pollutants from wastewater. This technology requires without the need for additional energy sources and features low operating costs. Figures 5, 6 illustrates the principles of constructed wetlands for pollutant removal. It has been employed in landfill leachate treatment, hospital wastewater, municipal wastewater, among applications. For example, Hanwell investigated the impact of active aeration on the removal of selected drugs in a simulated wetland. It was found that continuous aeration can remove about 99% of the two-meta duality and Sagastan, and at the same time, Atenolol and Pesor were still effectively removed (75% and 50%, respectively) among the high-concentration drugs detected at the hospital site (Auvinen et al., 2017). Sabri employed the CW for Sewage Treatment wastewater and has found significant treatment with antibiotics, with an overall removal rate of 28%–100%, depending on the type of antibiotic (Sabri et al., 2021). The CWs are multifunctional systems that improve water quality, act as hydrological buffers, and support natural habitats and recreational areas.By simulating natural wetland systems, such as wetland plants, soil, and soil microorganisms, continuous water treatment can remove various pollutants from different wastewater sources. Initially, constructed wetlands were primarily utilized for secondary and tertiary treatment of domestic and urban sewage; however, further research has expanded their capability to effectively address a broader spectrum of pollutants, with efficiency varying by substrate material. Considering various objectives, such as hydraulic penetration, pollutant types, and treatment needs, substrates of varying diameters are utilized in CWs. Biological processes (biodegradation, plant absorption, etc.) and physicochemical processes (adsorption, oxidation, chemical degradation, optical degradation, volatilization, and hydrolysis, etc.) also influence pollutant removal in CWs (Ji et al., 2022).
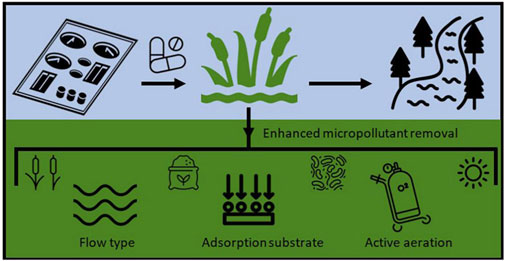
Figure 6. Constructed wetlands remove pollutants (Wagner et al., 2023).
6.3 Adsorption material
Biochar is a kind of material with multi-layer and fibrous porous structure, which is usually pyrolyzed under oxygen-limited conditions. Based on temperature, three primary pyrolysis processes are identified: fast pyrolysis (temperature >500°C), moderate pyrolysis (temperature 300°C–500°C) and slow pyrolysis (temperature <300°C) (Luo et al., 2022). In the process of adsorption, both physical and chemical adsorptions take place, with the dominant mechanism varying according to the type of pollutant involved (Figure 7). Significant electron donor-acceptor (EDA) interactions typically occur between the aromatic ring of the π donor and the π protonated adsorbent on the surface of the modified biochar. Additionally, electrostatic interactions, hydrogen bonding, surface complexation, cation exchange, and non-specific van der Waals forces may all contribute to the adsorption process (Peiris et al., 2017). For example, a coke made from olive oil waste from the biochar-thermal carbonization process, which removes triclosan, ibuprofen, and diclofenac from wastewater. Similar to activated carbon, the efficiency of biochar adsorption is influenced by the physical and chemical properties of the pollutants, the pKa of the adsorbent, and the temperature. Under the influence of oxygen-containing functional groups and solution pH, triclosan removal efficiency can reach up to 98% (Delgado-Moreno et al., 2021). Owing to the limitations in the application of original biochar for environmental remediation, an increasing number of researchers are turning to the production of biochar-based composites. These composites incorporate techniques such as liquid precipitation, nano-composite materials, and magnetic fields. However, magnetic biochar composites are predominantly employed for metal adsorption (Liang et al., 2021). Additionally, biochar derived from animal manure, chicken bones, bamboo and other raw materials is also popular because of its environmental characteristics (Patel et al., 2022). The pyrolytic calcium-rich biochar made from crab shells shows great advantages in the removal of chlortetracycline. At low concentrations, adsorption predominates; however, as concentrations increase, the adsorption capacity escalates impressively to 5,048 mg/g, combining both adsorption and flocculation mechanisms. Remarkably, the system maintained enhanced removal efficiency even after five operational cycles (Xu et al., 2020).In terms of Polycyclic aromatic hydrocarbon, pyrolysis of biochar using sludge from a groundwater treatment plant can be very effective, with a degradation efficiency of 87%. Biochar produced at 700°C predominantly undergoes degradation through Fenton oxidation, where Fe3 +/Fe2+ and Mn3+/Mn2+ redox pairs catalyze the formation of O2−• and HO• radical (Hung et al., 2020). In recent years, the research progress of biochar modification to remove organic pollutants has been recorded. Further research is necessary to develop targeted biocarbons with clear interactions that enable precise on-off regulation of cyclic remediation processes (Patel et al., 2022).
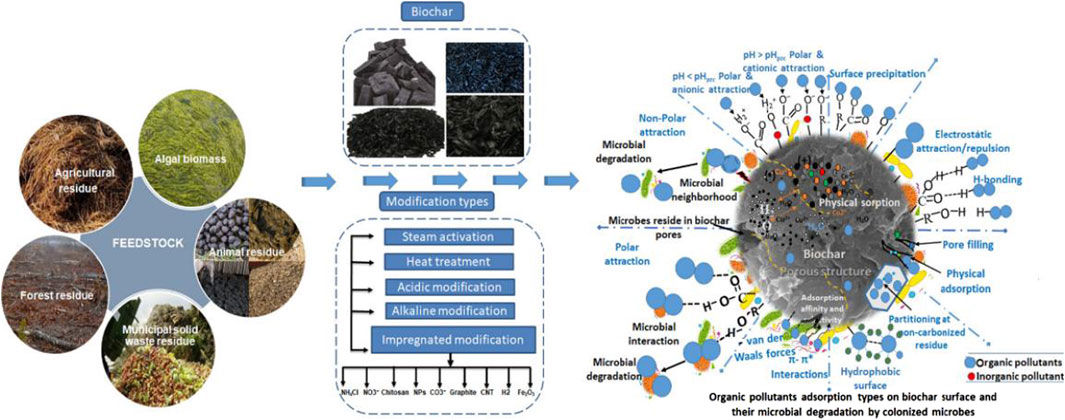
Figure 7. Biochar removes organic matter (Patel et al., 2022).
In fact, an increasing number of adsorbent materials, such as metal-organic frameworks (MOFs), nanomaterials, and metal oxide nanocomposites, are receiving significant attention from scholars. Several cases of MOFs removing emerging contaminants (ECs) have been reported, including MIL-101 adsorbing tetracycline (Hu et al., 2017), and MOF-5 adsorbing ciprofloxacin (Gadipelly et al., 2018). Due to their large surface area, customizable pore structure, diverse topologies, and ease of regeneration, MOFs are key to achieving complex applications in environmentally-friendly adsorption. However, the instability of MOFs in aqueous solutions is a crucial factor to consider, as studies have shown that currently no MOFs can effectively adsorb multiple pollutants simultaneously (Jeong et al., 2023). Experiments have found that the principles of adsorption are often electrostatic adsorption, hydrogen bonding, or π-π interactions. This is consistent with the study by Zhang et al., who investigated the adsorption of sulfamethoxazole using carbon nanotubes (Zhang et al., 2011). Lv developed high-performance coal gasification slag-based adsorbents from waste coal gasification slag through optimized preparation processes to adsorb microplastics, finding a maximum adsorption capacity exceeding 1,400 mg/g and an adsorption removal efficiency of up to 99.2% (Lv et al., 2025). Bele investigated the production of graphene oxide from hydrazine hydrate; at optimal reduction levels, the enhanced π-π interactions between the adsorbent and adsorbate led to an adsorption capacity for bisphenol A of 94.06 mg/L (Bele et al., 2016). Overall, providing surface functional groups and adsorption sites on adsorbents remains key to improving adsorption efficiency. Since adsorption can be combined with other processes to enhance efficiency, it is considered one of the green processes for removing ECs from wastewater resources (Sophia and Lima, 2018).
6.4 Algae repair
The most important processes in algal reactions are biodegradation, photodegradation, volatilization and adsorption (Gondi et al., 2022). Generally, biodegradation and photodegradation are the primary methods for removing organic micropollutants in urban wastewater (Matamoros et al., 2015). For instance, algal-mediated removal of five pharmaceutical and personal care products species (Trimethoprim, Sulfamethoxazole, carbamazepine, Ciprofloxacin and triclosan) from lake water has been documented. Significant removal efficiency was demonstrated under light conditions, with Ciprofloxacin and Triclosan being highly sensitive to light resulting in a 100% removal efficiency (Bai and Acharya, 2017). Zhang et al. (2021) found that intertidal algal blooms could remove bisphenol A and NP at ambient concentrations, with Ulva pertusa proving most efficient. The removal mechanism involves initial rapid biosorption, followed by slow bioaccumulation and biodegradation (Zhang et al., 2021). In addition, biosorption and bioaccumulation are also important ways to remove the concentration of organic pollutants in the environment. The difference lies in whether pollutants accumulate on the surface or are assimilated into the cytoplasm (Figure 8). Microalgae can continue to grow even after absorbing pollutants. Clearly, bioaccumulation necessitates that microalgae remain viable, as opposed to merely living, which is more suitable for removing dyes and heavy metals (Ratnasari et al., 2022). Consequently, owing to the metabolic activity of the cell wall and physicochemical processes on its surface, various organic compounds in wastewater and algal culture ingredients may facilitate distinct organic removal mechanisms.
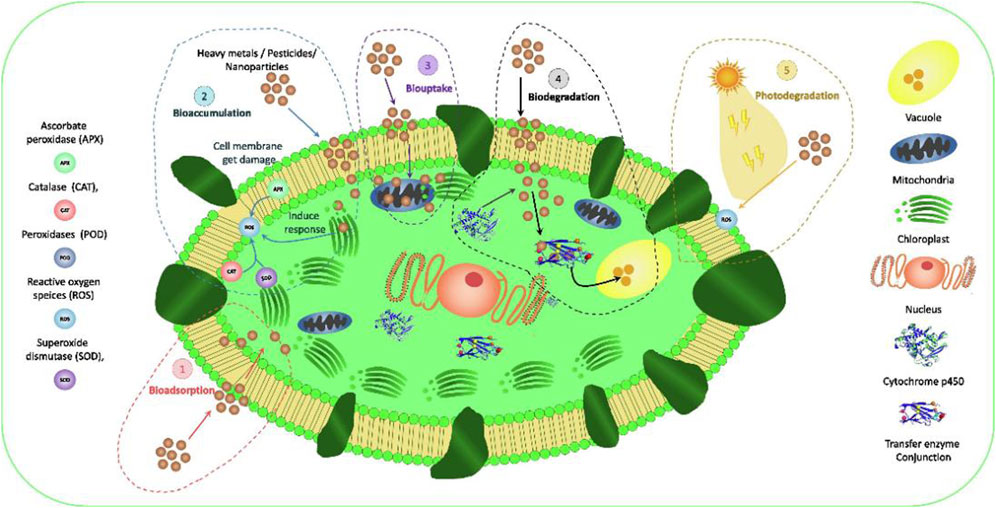
Figure 8. Mechanisms of bioremediation of new pollutants through microalgae-based treatment systems: (1) EP crosses the algal cell wall and binds to intracellular proteins in living cells during biouptake; And (2) in inactive cells during bioaccumulation (3) EP is adsorbed to algal cell wall or extra polymer(EPS) during bioadsorption (4) complex EP is decomposed into simpler and less toxic compounds during biodegradation (5) EP is exposed to UV light during direct or photodegradation (Gondi et al.,2022).
6.5 Biological treatment technologies
Conventional activated sludge treatment technology is commonly employed in municipal wastewater treatment plants, featuring three main degradation pathways: microbial processes (biodegradation, metabolism, or co-metabolism), sludge flocculation adsorption, and volatilization (primarily during aeration). Generally, pollutants exist in different ionic forms under varying pH conditions, and since log D is pH-dependent, the log D value is a critical factor to consider for adsorptive removal of pollutants (Grandclement et al., 2017). Some studies have proposed using activated sludge technology to remove new pollutants (Coccia and Bontempi, 2023; Fan et al., 2022; Verlicchi et al., 2013), however, emerging contaminants (ECs) often exist in trace amounts and their complex physicochemical properties restrict degradation capabilities. Research has shown that activated sludge can remove acetaminophen, fluconazole, and ibuprofen with efficiencies of 99.9%, 97%, and 99.9% respectively (Dos Santos et al., 2022), but shows poorer removal for ofloxacin, penicillin V, sulfamethoxazole, and methoxybenzamine (Blair et al., 2015). Thus, developing integrated technologies is the future direction for treating ECs, such as a wastewater treatment system combining aquatic foxtail algae with activated sludge, where forming a biofilm post-inoculation significantly enhanced the removal efficiency of pollutants in the wastewater (Guo et al., 2019). Another study applied a static magnetic field (SMF) to the activated sludge process to enhance tetracycline removal from swine wastewater. The SMF increased electrostatic interactions between activated sludge and tetracycline, providing more binding sites and promoting the growth of ammonia-oxidizing bacteria, tetracycline-degrading bacteria, and aromatic compound-degrading bacteria. Additionally, SMF mitigated the enrichment and spread of antibiotic resistance genes by reducing the potential host abundance and suppressing the upregulation of genes encoding ABC transporters and hypothetical transposases (Zhu et al., 2024). Therefore, the application of magnetic fields (MF) in biological wastewater treatment has garnered significant attention due to its sustainability, cost-effectiveness, and eco-friendliness (Li et al., 2024).
Generally, pollutants are adsorbed onto the lipid structures of bacterial communities and the fatty parts of wastewater sludge through hydrophobic interactions (e.g., aromatic and aliphatic). Moreover, they can also be adsorbed onto the negatively charged polysaccharide structures outside bacterial cells through electrostatic interactions (e.g., amino groups). Given the ubiquity of bacteria, protozoa, and fungi in aquatic environments, microbial degradation often represents the most critical process in the mass balance of environmental transformation.
Additionally, the Membrane Bioreactor (MBR) represents another prevalent technology for biowastewater treatment. According to previous reports, MBR has demonstrated effective removal of nonylphenol and certain hormones (Gonzalez et al., 2006), yet it appears to be less effective for antibiotics (Kim et al., 2007). Although MBR has well-recognized advantages, the accumulation of fouling on the membrane surface reduces membrane flux, necessitating the use of corrosive chemicals for cleaning and thus posing a significant challenge due to secondary pollution. Ashish suggests that pharmaceuticals can alter the secretion patterns of various contaminants, such as disrupting cellular structures, thereby forcing the release of intracellular polymers. All these factors contribute to the accelerated fouling propensity in MBR systems (Sengar and Vijayanandan, 2022).
6.6 Advanced oxidation technology
The electrochemical treatment process involves the mineralization of pollutants through two primary pathways: direct anodic oxidation at the main electrodes (anode and cathode) or indirect oxidation via reactive oxygen species in the solution and reduction reactions at the cathode. For example, Tang employed a silver-plated titanium (Ti/Ag) anode for the electrochemical degradation of perfluorinated compounds (PFCs) in the effluent from a municipal wastewater treatment plant. Their study demonstrated excellent PFCs removal efficiencies, with maximum removal efficiencies of approximately 70.8% for short-chain PFCs (CF < 7), 91.5% for long-chain PFCs (CF ≥ 7), and 92.0% for chemical oxygen demand (COD) (Tang et al., 2021). Generally, the removal rate increases with the increase in current density. This rule applies not only to perfluorinated compounds but also to the removal of pharmaceutical residues, such as ciprofloxacin, in a pilot-scale pulsed corona discharge system, where the removal efficiency increased from 29% to 100% as the nominal power increased from 30 W to 250 W (Ajo et al., 2018). Due to the oxygen release reaction potential in electrochemical systems, the environmental friendliness of electrode materials is crucial. The electrochemical oxidation efficiency of the anode largely depends on the electrode material itself, where weak interactions between the electrode and reactive oxygen species result in high chemical reactivity, leading to the complete mineralization or oxidation of organic pollutants. Electrochemical oxidation systems are considered a viable option for the treatment of pharmaceutical pollutants (Ganthavee and Trzcinski, 2023; Li et al., 2016a).
Another effective and environmentally friendly removal method is photocatalysis. As an emerging green technology, it primarily accelerates reactions by generating electrons and holes upon light irradiation. Efficient photocatalysis involves a broad light absorption spectrum and high separation efficiency of photogenerated charge carriers. Under ideal conditions, molecular oxygen can be excited by electrons to produce superoxide anion radicals and hydrogen peroxide, which can completely decompose organic pollutants into non-toxic CO₂ and H₂O without generating harmful by-products (Rajaitha et al., 2022). Photocatalysis has been applied to various materials, such as carbon nanotubes and titanium dioxide. Baran et al. found that TiO₂ photocatalysis can effectively remove sulfamethoxazole and its by-products, with the resulting by-products being less toxic to Chlorella vulgaris and easier to degrade (Baran et al., 2006). In the context of microplastic photocatalysis, Nabi et al. discovered that after 12 h of UV irradiation at 254 nm, the degradation rate of 5 mm polystyrene microplastics reached 44.6% under liquid phase conditions and increased to 99% in the solid phase (Nabi et al., 2020).Xu suggested that lower pH and lower temperatures are more conducive to photocatalysis during degradation, as low pH can introduce ions into the reaction process, and low temperatures can facilitate the fragmentation of microplastics (Xu et al., 2021).
Despite the availability of numerous advanced oxidation-reduction methods for the removal of emerging pollutants, we must consider the discrepancies between laboratory-simulated experiments and actual complex water bodies. Additionally, whether the experimental process incurs additional costs remains uncertain. More importantly, the stability and recyclability of materials are critical factors that need to be considered.
7 Conclusion
The scope of new pollutants has been expanding over time, and in addition to those mentioned in the article, it also includes a large number of compounds such as polychlorobenzenes, polycyclic aromatic hydrocarbons, etc. With large-scale production and use, they are ubiquitous in the environment. Although there are many reports of their presence, the relative concentrations are higher in Asia and Europe. There are many studies in treatment technology, lack of corresponding innovation is also a huge problem. It is worth noting that the current research on the deprivation of new pollutants is mainly concentrated in the simulation of the laboratory. And despite the fruitful results, the effects of de-priming trace pollutants in the actual aqueous environment as well as coping with complex environmental matrices are yet to be investigated, and intermediates produced by the degradation of parent neopollutants, for example, are likely to be more toxic. Considering the current situation, several factors need to be considered:
1. Regulation: As understanding of neo-pollutants deepens and monitoring technologies advance, regulatory systems will become more comprehensive and refined. Future regulations will increasingly focus on real-time monitoring and data sharing to promptly detect and address the emergence of new pollutants. Simultaneously, there will be a strengthened emphasis on international cooperation and information sharing to effectively tackle transboundary pollution.
2. Removal technologies: Future research directions for removal technologies will focus on enhancing efficiency, environmental compatibility, and economic feasibility. With technological advancements, it is anticipated that more sophisticated methods for pollutant elimination will be developed. Furthermore, there will be a heightened emphasis on the practical deployment and sustainability of these technologies. While the primary findings of this research highlight promising approaches for contaminant removal, certain limitations exist, such as the synergistic effects of various technologies and the yet unclear implications of certain byproducts. Indeed, enhancing energy efficiency at the source to mitigate environmental impacts represents a vital strategy for sustainable development, encompassing the innovation of new materials and the formulation of less toxic pharmaceuticals.
3. Environmental hazards: Future research will be more in-depth and comprehensive in response to the potential environmental hazards of new pollutants. The focus of research may include studies on the ecotoxicity, bioaccumulation and potential long-term effects of pollutants to comprehensively assess their impacts on the ecosystem and develop corresponding countermeasures.
Author contributions
QZ: Investigation, Writing–original draft, Writing–review and editing. HC: Funding acquisition, Writing–review and editing. GL: Writing–review and editing. XW: Data curation, Funding acquisition, Writing–review and editing.
Funding
The author(s) declare that financial support was received for the research, authorship, and/or publication of this article. This work was supported by the Key R&D projects of Department of Science and Technology of Anhui Province, China (2023t07020002).
Conflict of interest
The authors declare that the research was conducted in the absence of any commercial or financial relationships that could be construed as a potential conflict of interest.
Publisher’s note
All claims expressed in this article are solely those of the authors and do not necessarily represent those of their affiliated organizations, or those of the publisher, the editors and the reviewers. Any product that may be evaluated in this article, or claim that may be made by its manufacturer, is not guaranteed or endorsed by the publisher.
References
Ajo, P., Preis, S., Vornamo, T., Mänttäri, M., Kallioinen, M., and Louhi-Kultanen, M. (2018). Hospital wastewater treatment with pilot-scale pulsed corona discharge for removal of pharmaceutical residues. J. Environ. Chem. Eng. 6 (2), 1569–1577. doi:10.1016/j.jece.2018.02.007
Auvinen, H., Havran, I., Hubau, L., Vanseveren, L., Gebhardt, W., Linnemann, V., et al. (2017). Removal of pharmaceuticals by a pilot aerated sub-surface flow constructed wetland treating municipal and hospital wastewater. Ecol. Eng. 100, 157–164. doi:10.1016/j.ecoleng.2016.12.031
Bahnmueller, S., Gunten, U. V., and Canonica, S. (2014). Sunlight-induced transformation of sulfadiazine and sulfamethoxazole in surface waters and wastewater effluents. Water Res. 57, 183–192. doi:10.1016/j.watres.2014.03.019
Bai, X., and Acharya, K. (2017). Algae-mediated removal of selected pharmaceutical and personal care products (PPCPs) from Lake Mead water. Sci. Total Environ. 581-582, 734–740. doi:10.1016/j.scitotenv.2016.12.192
Baran, W., Sochacka, J., and Wardas, W. (2006). Toxicity and biodegradability of sulfonamides and products of their photocatalytic degradation in aqueous solutions. Chemosphere 65 (8), 1295–1299. doi:10.1016/j.chemosphere.2006.04.040
Bele, S., Samanidou, V., and Deliyanni, E. (2016). Effect of the reduction degree of graphene oxide on the adsorption of Bisphenol A. Chem. Eng. Res. Des. 109, 573–585. doi:10.1016/j.cherd.2016.03.002
Bhandari, G., Bagheri, A. R., Bhatt, P., and Bilal, M. (2021). Occurrence, potential ecological risks, and degradation of endocrine disrupter, nonylphenol, from the aqueous environment. Chemosphere 275, 130013. doi:10.1016/j.chemosphere.2021.130013
Blair, B., Nikolaus, A., Hedman, C., Klaper, R., and Grundl, T. (2015). Evaluating the degradation, sorption, and negative mass balances of pharmaceuticals and personal care products during wastewater treatment. Chemosphere 134, 395–401. doi:10.1016/j.chemosphere.2015.04.078
Buerge, I. J., Kasteel, R., and Poiger, T. (2024). Leaching of herbicides and their metabolites in lysimeters filled with soils from railway tracks. Sci. Total Environ. 909, 168396. doi:10.1016/j.scitotenv.2023.168396
Carvalho, I. T., and Santos, L. (2016). Antibiotics in the aquatic environments: a review of the European scenario. Environ. Int. 94, 736–757. doi:10.1016/j.envint.2016.06.025
Chen, J., Wang, B., Huang, J., Deng, S., Wang, Y., Blaney, L., et al. (2022a). A machine-learning approach clarifies interactions between contaminants of emerging concern. One Earth 5 (11), 1239–1249. doi:10.1016/j.oneear.2022.10.006
Chen, J., Wu, J., Sherrell, P. C., Chen, J., Wang, H., Zhang, W., et al. (2022b). How to build a microplastics-free environment: strategies for microplastics degradation and plastics recycling. Adv. Sci. 9 (6), e2103764. doi:10.1002/advs.202103764
Chen, J., Wu, J., Sherrell, P. C., Chen, J., Wang, H., Zhang, W. X., et al. (2022c). How to build a microplastics-free environment: strategies for microplastics degradation and plastics recycling. Adv. Sci. (Weinheim, Baden-Wurttemberg, Ger.) 9 (6), e2103764. doi:10.1002/advs.202103764
Chen, K., and Zhou, J. L. (2014). Occurrence and behavior of antibiotics in water and sediments from the Huangpu River, Shanghai, China. Chemosphere 95, 604–612. doi:10.1016/j.chemosphere.2013.09.119
Chisaki, O. (2005). 1S4-PM1 bioindustry and biotechnology innovation in 21st century. ence Total Environ. 349 (1-3), 106–119. doi:10.1016/j.scitotenv.2005.01.010
Coccia, M., and Bontempi, E. (2023). New trajectories of technologies for the removal of pollutants and emerging contaminants in the environment. Environ. Res. 229, 115938. doi:10.1016/j.envres.2023.115938
Crane, J. L. (2021). Distribution and toxic potential of alkylphenols, nonylphenol ethoxylates, and pyrethroids in Minnesota, USA lake sediments. Sci. Total Environ., 776. doi:10.1016/j.scitotenv.2021.145974
Delgado-Moreno, L., Bazhari, S., Gasco, G., Mendez, A., El Azzouzi, M., and Romero, E. (2021). New insights into the efficient removal of emerging contaminants by biochars and hydrochars derived from olive oil wastes. Sci. Total Environ. 752, 141838. doi:10.1016/j.scitotenv.2020.141838
Dos Santos, C. R., Lebron, Y. A. R., Moreira, V. R., Koch, K., and Amaral, M. C. S. (2022). Biodegradability, environmental risk assessment and ecological footprint in wastewater technologies for pharmaceutically active compounds removal. Bioresour. Technol. 343, 126150. doi:10.1016/j.biortech.2021.126150
Fan, Z., Casey, F. X. M., Hakk, H., Larsen, G. L., and Khan, E. (2010). Sorption, fate, and mobility of sulfonamides in soils. Water, Air, and Soil Pollut. 218 (1-4), 49–61. doi:10.1007/s11270-010-0623-6
Fan, Z., Yang, S., Zhu, Q., and Zhu, X. (2022). Effects of different oxygen conditions on pollutants removal and the abundances of tetracycline resistance genes in activated sludge systems. Chemosphere 291 (Pt 1), 132681. doi:10.1016/j.chemosphere.2021.132681
Fernandes, M. J., Paíga, P., Silva, A., Llaguno, C. P., Carvalho, M., Vázquez, F. M., et al. (2020). Antibiotics and antidepressants occurrence in surface waters and sediments collected in the north of Portugal. Chemosphere 239, 124729. doi:10.1016/j.chemosphere.2019.124729
Gadipelly, C. R., Marathe, K. V., and Rathod, V. K. (2018). Effective adsorption of ciprofloxacin hydrochloride from aqueous solutions using metal-organic framework. Sep. Sci. and Technol. 53, 2826–2832. doi:10.1080/01496395.2018.1474225
Ganthavee, V., and Trzcinski, A. P. (2023). Removal of pharmaceutically active compounds from wastewater using adsorption coupled with electrochemical oxidation technology: a critical review. J. Industrial Eng. Chem. 126, 20–35. doi:10.1016/j.jiec.2023.06.003
Gondi, R., Kavitha, S., Yukesh Kannah, R., Parthiba Karthikeyan, O., Kumar, G., Kumar Tyagi, V., et al. (2022). Algal-based system for removal of emerging pollutants from wastewater: a review. Bioresour. Technol. 344 (Pt B), 126245. doi:10.1016/j.biortech.2021.126245
Gonzalez, S., Muller, J., Petrovic, M., Barcelo, D., and Knepper, T. P. (2006). Biodegradation studies of selected priority acidic pesticides and diclofenac in different bioreactors. Environ. Pollut. 144 (3), 926–932. doi:10.1016/j.envpol.2006.02.021
Grandclement, C., Seyssiecq, I., Piram, A., Wong-Wah-Chung, P., Vanot, G., Tiliacos, N., et al. (2017). From the conventional biological wastewater treatment to hybrid processes, the evaluation of organic micropollutant removal: a review. Water Res. 111, 297–317. doi:10.1016/j.watres.2017.01.005
Guo, X., Li, Y., Zhong, H., Li, P., Zhao, T., and Zhang, C. (2019). A wastewater treatment system combining Myriophyllum aquaticum and activated sludge: optimization of construction conditions and evaluation of wastewater treatment performance. J. Environ. Manage 235, 105–111. doi:10.1016/j.jenvman.2019.01.032
Hong, Y., Feng, C., Yan, Z., Wang, Y., Liu, D., Liao, W., et al. (2020). Nonylphenol occurrence, distribution, toxicity and analytical methods in freshwater. Environ. Chem. Lett. 18 (6), 2095–2106. doi:10.1007/s10311-020-01060-3
Hu, T., Jia, Q., He, S., Shan, S., Su, H., Zhi, Y., et al. (2017). Novel functionalized metal-organic framework MIL-101 adsorbent for capturing oxytetracycline. J. Alloys Compd. 727, 114–122. doi:10.1016/j.jallcom.2017.08.116
Hung, C. M., Huang, C. P., Chen, C. W., Wu, C. H., Lin, Y. L., and Dong, C. D. (2020). Activation of percarbonate by water treatment sludge-derived biochar for the remediation of PAH-contaminated sediments. Environ. Pollut. 265 (Pt B), 114914. doi:10.1016/j.envpol.2020.114914
Jeong, C., Ansari, M. Z., Hakeem Anwer, A., Kim, S.-H., Nasar, A., Shoeb, M., et al. (2023). A review on metal-organic frameworks for the removal of hazardous environmental contaminants. Sep. Purif. Technol. 305, 122416. doi:10.1016/j.seppur.2022.122416
Ji, Z., Tang, W., and Pei, Y. (2022). Constructed wetland substrates: a review on development, function mechanisms, and application in contaminants removal. Chemosphere 286 (Pt 1), 131564. doi:10.1016/j.chemosphere.2021.131564
Kim, S. D., Cho, J., Kim, I. S., Vanderford, B. J., and Snyder, S. A. (2007). Occurrence and removal of pharmaceuticals and endocrine disruptors in South Korean surface, drinking, and waste waters. Water Res. 41 (5), 1013–1021. doi:10.1016/j.watres.2006.06.034
Krkstrm, M., Saeid, S., Tolvanen, P., Salmi, T., and Kronberg, L. (2020). Catalytic ozonation of the antibiotic sulfadiazine: reaction kinetics and transformation mechanisms. Chemosphere 247, 125853. doi:10.1016/j.chemosphere.2020.125853
Laskar, N., and Kumar, U. (2019). Plastics and microplastics: a threat to environment. Environ. Technol. and Innovation 14, 100352. doi:10.1016/j.eti.2019.100352
Le, T. H., Ng, C., Tran, N. H., Chen, H., and Gin, K. Y. (2018). Removal of antibiotic residues, antibiotic resistant bacteria and antibiotic resistance genes in municipal wastewater by membrane bioreactor systems. Water Res. 145, 498–508. doi:10.1016/j.watres.2018.08.060
Li, B., Zheng, M., Xue, H., and Pang, H. (2016a). High performance electrochemical capacitor materials focusing on nickel based materials. Inorg. Chem. Front. 3 (2), 175–202. doi:10.1039/c5qi00187k
Li, J., Li, D., Liang, D., Wu, Y., Zhu, Y., Du, L., et al. (2024). Static magnetic fields enhance microbial aggregation and adhesion to promote aerobic granulation. Chem. Eng. J. 489, 151392. doi:10.1016/j.cej.2024.151392
Li, Y., Li, Q., Zhou, K., Sun, X. L., and Zhang, Y. B. (2016b). Occurrence and distribution of the environmental pollutant antibiotics in Gaoqiao mangrove area, China. Chemosphere 147, 25–35. doi:10.1016/j.chemosphere.2015.12.107
Liang, L., Xi, F., Tan, W., Meng, X., Hu, B., and Wang, X. (2021). Review of organic and inorganic pollutants removal by biochar and biochar-based composites. Biochar 3 (3), 255–281. doi:10.1007/s42773-021-00101-6
Liu, B., Liu, W., Nie, X., Guan, C., Yang, Y., Wang, Z., et al. (2011). Growth response and toxic effects of three antibiotics on Selenastrum capricornutum evaluated by photosynthetic rate and chlorophyll biosynthesis. J. Environ. Sci. 23 (9), 1558–1563. doi:10.1016/s1001-0742(10)60608-0
Liu, X., Li, L., Gu, L., Hua, Z., Zhang, Y., and Xue, H. (2021). Distribution and release of perfluorinated compounds (PFCs) in water-sediment systems: the effect of confluence channels. Sci. Total Environ. 775, 145720. doi:10.1016/j.scitotenv.2021.145720
Luo, J., Zhang, Q., Cao, M., Wu, L., Cao, J., Fang, F., et al. (2019). Ecotoxicity and environmental fates of newly recognized contaminants-artificial sweeteners: a review. Sci. Total Environ. 653, 1149–1160. doi:10.1016/j.scitotenv.2018.10.445
Luo, Z., Yao, B., Yang, X., Wang, L., Xu, Z., Yan, X., et al. (2022). Novel insights into the adsorption of organic contaminants by biochar: a review. Chemosphere 287 (Pt 2), 132113. doi:10.1016/j.chemosphere.2021.132113
Lv, B., Jiao, Y., Deng, X., Fan, W., and Xing, B. (2025). Adsorptive removal of microplastics from aquatic environments using coal gasification slag-based adsorbent in a liquid–solid fluidized bed. Sep. Purif. Technol. 354, 128935. doi:10.1016/j.seppur.2024.128935
Ma, Q., Chu, Y., Ni, X., Zhang, J., Chen, H., Xu, F., et al. (2023). CeO(2) modified carbon nanotube electrified membrane for the removal of antibiotics. Chemosphere 310, 136771. doi:10.1016/j.chemosphere.2022.136771
Mafi, A., Nejad, E. G., Ashouri, A., and Nia, M. V. (2013). “Dinoflagellate cysts from the upper bajocian-lower oxfordian of the dalichai formation,” in Binalud Mountains (NE Iran): their biostratigraphical and biogeographical significance.
Matamoros, V., Gutierrez, R., Ferrer, I., Garcia, J., and Bayona, J. M. (2015). Capability of microalgae-based wastewater treatment systems to remove emerging organic contaminants: a pilot-scale study. J. Hazard Mater 288, 34–42. doi:10.1016/j.jhazmat.2015.02.002
Mitchell, S. M., Ullman, J. L., Teel, A. L., and Watts, R. J. (2013). PH and temperature effects on the hydrolysis of three ?? lactam antibiotics: ampicillin, cefalotin and cefoxitin. Sci. Total Environ. 466-467C, 547–555. doi:10.1016/j.scitotenv.2013.06.027
Möhler, J. S., Kolmar, T., Synnatschke, K., Hergert, M., Wilson, L. A., Ramu, S., et al. (2017). Enhancement of antibiotic-activity through complexation with metal ions - combined ITC, NMR, enzymatic and biological studies. J. Inorg. Biochem. 167, 134–141. doi:10.1016/j.jinorgbio.2016.11.028
Nabi, I., Bacha, A. U., Li, K., Cheng, H., Wang, T., Liu, Y., et al. (2020). Complete photocatalytic mineralization of microplastic on TiO(2) nanoparticle film. iScience 23 (7), 101326. doi:10.1016/j.isci.2020.101326
Ni, X., Hou, X., Ma, D., Li, Q., Li, L., Gao, B., et al. (2023). Simultaneous removal of antibiotics and antibiotic resistant genes using a CeO(2)@CNT electrochemical membrane-NaClO system. Chemosphere 338, 139457. doi:10.1016/j.chemosphere.2023.139457
Pan, Z., Yu, F., Li, L., Song, C., Yang, J., Wang, C., et al. (2019). Electrochemical microfiltration treatment of bisphenol A wastewater using coal-based carbon membrane. Sep. Purif. Technol. 227, 115695. doi:10.1016/j.seppur.2019.115695
Parida, V. K., Saidulu, D., Majumder, A., Srivastava, A., Gupta, B., and Gupta, A. K. (2021). Emerging contaminants in wastewater: a critical review on occurrence, existing legislations, risk assessment, and sustainable treatment alternatives. J. Environ. Chem. Eng. 9 (5), 105966. doi:10.1016/j.jece.2021.105966
Patel, A. K., Singhania, R. R., Pal, A., Chen, C. W., Pandey, A., and Dong, C. D. (2022). Advances on tailored biochar for bioremediation of antibiotics, pesticides and polycyclic aromatic hydrocarbon pollutants from aqueous and solid phases. Sci. Total Environ. 817, 153054. doi:10.1016/j.scitotenv.2022.153054
Pei, S., Shi, H., Zhang, J., Wang, S., Ren, N., and You, S. (2021). Electrochemical removal of tetrabromobisphenol A by fluorine-doped titanium suboxide electrochemically reactive membrane. J. Hazard Mater 419, 126434. doi:10.1016/j.jhazmat.2021.126434
Peiris, C., Gunatilake, S. R., Mlsna, T. E., Mohan, D., and Vithanage, M. (2017). Biochar based removal of antibiotic sulfonamides and tetracyclines in aquatic environments: a critical review. Bioresour. Technol. 246, 150–159. doi:10.1016/j.biortech.2017.07.150
Rajaitha, P. M., Hajra, S., Sahu, M., Mistewicz, K., Toroń, B., Abolhassani, R., et al. (2022). Unraveling highly efficient nanomaterial photocatalyst for pollutant removal: a comprehensive review and future progress. Mater. Today Chem. 23, 100692. doi:10.1016/j.mtchem.2021.100692
Ratnasari, A., Syafiuddin, A., Zaidi, N. S., Hong Kueh, A. B., Hadibarata, T., Prastyo, D. D., et al. (2022). Bioremediation of micropollutants using living and non-living algae - current perspectives and challenges. Environ. Pollut. 292 (Pt B), 118474. doi:10.1016/j.envpol.2021.118474
Ren, L., Chen, M., Zheng, J., Li, Z., Tian, C., Wang, Q., et al. (2021). Efficacy of a novel electrochemical membrane-aerated biofilm reactor for removal of antibiotics from micro-polluted surface water and suppression of antibiotic resistance genes. Bioresour. Technol. 338, 125527. doi:10.1016/j.biortech.2021.125527
Rodriguez-Rodriguez, C. E., Ramirez-Morales, D., Masis-Mora, M., Montiel-Mora, J. R., Soto-Garita, C., Araya-Valverde, E., et al. (2023). Occurrence and risk assessment of pharmaceuticals in hospital wastewater in Costa Rica. Chemosphere, 339. doi:10.1016/j.chemosphere.2023.139746
Sabri, N. A., Schmitt, H., van der Zaan, B. M., Gerritsen, H. W., Rijnaarts, H. H. M., and Langenhoff, A. A. M. (2021). Performance of full scale constructed wetlands in removing antibiotics and antibiotic resistance genes. Sci. Total Environ. 786, 147368. doi:10.1016/j.scitotenv.2021.147368
Sakurai, T., Kobayashi, J., Kinoshita, K., Ito, N., Serizawa, S., Shiraishi, H., et al. (2013). Transfer kinetics of perfluorooctane sulfonate from water and sediment to a marine benthic fish, the marbled flounder (Pseudopleuronectes yokohamae). Environ. Toxicol. Chem. 32 (9), 2009–2017. doi:10.1002/etc.2270
Sarmah, A. K., Meyer, M. T., and Boxall, A. (2006). A global perspective on the use, sales, exposure pathways, occurrence, fate and effects of veterinary antibiotics (VAs) in the environment. Chemosphere 65 (5), 725–759. doi:10.1016/j.chemosphere.2006.03.026
Savoca, D., and Pace, A. (2021). Bioaccumulation, biodistribution, toxicology and biomonitoring of organofluorine compounds in aquatic organisms. Int. J. Mol. Sci. 22 (12), 6276. doi:10.3390/ijms22126276
Sengar, A., and Vijayanandan, A. (2022). Effects of pharmaceuticals on membrane bioreactor: review on membrane fouling mechanisms and fouling control strategies. Sci. Total Environ. 808, 152132. doi:10.1016/j.scitotenv.2021.152132
Seralini, G. E., and Jungers, G. (2021). Endocrine disruptors also function as nervous disruptors and can be renamed endocrine and nervous disruptors (ENDs). Toxicol. Rep. 8, 1538–1557. doi:10.1016/j.toxrep.2021.07.014
Sharma, V. K., Ma, X., Lichtfouse, E., and Robert, D. (2022). Nanoplastics are potentially more dangerous than microplastics. Environ. Chem. Lett. 21 (4), 1933–1936. doi:10.1007/s10311-022-01539-1
Sophia, A. C., and Lima, E. C. (2018). Removal of emerging contaminants from the environment by adsorption. Ecotoxicol. Environ. Saf. 150, 1–17. doi:10.1016/j.ecoenv.2017.12.026
Tang, J., Liu, Z., Lu, W., Wang, L., Zhang, C., and Su, P. (2021). Electrochemical degradation of perfluorinated compounds by Ag coated Ti (Ti/Ag) anode: electrode preparation, characterization and application. Environ. Sci. Water Res. and Technol. 7, 455–467. doi:10.1039/d0ew00785d
Tong, F., Liu, D., Zhang, Z., Chen, W., Fan, G., Gao, Y., et al. (2023). Heavy metal-mediated adsorption of antibiotic tetracycline and ciprofloxacin on two microplastics: insights into the role of complexation. Environ. Res. 216 (Pt 3), 114716. doi:10.1016/j.envres.2022.114716
Verlicchi, P., Galletti, A., Petrovic, M., Barcelo, D., Al Aukidy, M., and Zambello, E. (2013). Removal of selected pharmaceuticals from domestic wastewater in an activated sludge system followed by a horizontal subsurface flow bed - analysis of their respective contributions. Sci. Total Environ. 454-455, 411–425. doi:10.1016/j.scitotenv.2013.03.044
V, G., Shanmugavel, S. P., Tyagi, V. K., and Rajesh Banu, J. (2023). Microplastics as emergent contaminants in landfill leachate: source, potential impact and remediation technologies. J. Environ. Manage 343, 118240. doi:10.1016/j.jenvman.2023.118240
Wagner, T. V., Rempe, F., Hoek, M., Schuman, E., and Langenhoff, A. (2023). Key constructed wetland design features for maximized micropollutant removal from treated municipal wastewater: a literature study based on 16 indicator micropollutants. Water Res. 244, 120534. doi:10.1016/j.watres.2023.120534
Wang, F., Xiang, L., Leung, K., Elsner, M., Zhang, Y., Guo, Y., et al. (2024). Emerging contaminants: a one health perspective. Innov. J. 5, 100612. doi:10.1016/j.xinn.2024.100612
Wang, Q., He, X., Xiong, H., Chen, Y., and Huang, L. (2022). Structure, mechanism, and toxicity in antibiotics metal complexation: recent advances and perspectives. Sci. Total Environ. 848, 157778. doi:10.1016/j.scitotenv.2022.157778
Wendell, G., Priscila, P., Silva, C., Hernán, T., Elene, C., and Pereira-Maia, (2016). Impact of metal coordination on the antibiotic and non-antibiotic activities of tetracycline-based drugs. Coord. Chem. Rev. 327-328, 188–199. doi:10.1016/j.ccr.2016.04.009
Wu, J. Y., Hua, Z. L., and Gu, L. (2021). Planktonic microbial responses to perfluorinated compound (PFC) pollution: integrating PFC distributions with community coalescence and metabolism. Sci. Total Environ. 788, 147743. doi:10.1016/j.scitotenv.2021.147743
Xu, Q., Huang, Q.-S., Luo, T.-Y., Wu, R.-L., Wei, W., and Ni, B.-J. (2021). Coagulation removal and photocatalytic degradation of microplastics in urban waters. Chem. Eng. J. 416, 129123. doi:10.1016/j.cej.2021.129123
Xu, Q., Zhou, Q., Pan, M., and Dai, L. (2020). Interaction between chlortetracycline and calcium-rich biochar: enhanced removal by adsorption coupled with flocculation. Chem. Eng. J. 382, 122705. doi:10.1016/j.cej.2019.122705
Yang, Y., Qiao, S., Zheng, M., Zhou, J., and Quan, X. (2019). Enhanced permeability, contaminants removal and antifouling ability of CNTs-based hollow fiber membranes under electrochemical assistance. J. Membr. Sci. 582, 335–341. doi:10.1016/j.memsci.2019.04.026
Yu, X., Zhou, Z. C., Shuai, X. Y., Lin, Z. J., Liu, Z., Zhou, J. Y., et al. (2023). Microplastics exacerbate co-occurrence and horizontal transfer of antibiotic resistance genes. J. Hazard Mater 451, 131130. doi:10.1016/j.jhazmat.2023.131130
Zhang, C., Lu, J., and Wu, J. (2021). Enhanced removal of phenolic endocrine disrupting chemicals from coastal waters by intertidal macroalgae. J. Hazard Mater 411, 125105. doi:10.1016/j.jhazmat.2021.125105
Zhang, D., Pan, B., Wu, M., Wang, B., Zhang, H., Peng, H., et al. (2011). Adsorption of sulfamethoxazole on functionalized carbon nanotubes as affected by cations and anions. Environ. Pollut. 159 (10), 2616–2621. doi:10.1016/j.envpol.2011.05.036
Zhang, R., Zhang, G., Zheng, Q., Tang, J., Chen, Y., Xu, W., et al. (2012). Occurrence and risks of antibiotics in the Laizhou Bay, China: impacts of river discharge. Ecotoxicol. Environ. Saf. 80, 208–215. doi:10.1016/j.ecoenv.2012.03.002
Zhang, Y., Li, Y., Wang, Y., Su, F., Qian, J., and Liu, S. (2023). Adsorption of levofloxacin by ultraviolet aging microplastics. Chemosphere 343, 140196. doi:10.1016/j.chemosphere.2023.140196
Zhou, Z., Sun, Y., Wang, Y., Yu, F., and Ma, J. (2022). Adsorption behavior of Cu(II) and Cr(VI) on aged microplastics in antibiotics-heavy metals coexisting system. Chemosphere 291 (Pt 1), 132794. doi:10.1016/j.chemosphere.2021.132794
Keywords: emerging contaminants, risk assessment, removal technologies, environmental pollution, sustainable development
Citation: Zhou Q, Chen H, Liu G and Wang X (2024) Occurrence, sustainable treatment technologies, potential sources, and future prospects of emerging pollutants in aquatic environments: a review. Front. Environ. Sci. 12:1455377. doi: 10.3389/fenvs.2024.1455377
Received: 26 June 2024; Accepted: 27 August 2024;
Published: 13 September 2024.
Edited by:
Ping Li, Shandong University, Weihai, ChinaReviewed by:
Giovanni Cagnetta, Tsinghua University, ChinaNnanake-Abasi O. Offiong, Topfaith University, Nigeria
Copyright © 2024 Zhou, Chen, Liu and Wang. This is an open-access article distributed under the terms of the Creative Commons Attribution License (CC BY). The use, distribution or reproduction in other forums is permitted, provided the original author(s) and the copyright owner(s) are credited and that the original publication in this journal is cited, in accordance with accepted academic practice. No use, distribution or reproduction is permitted which does not comply with these terms.
*Correspondence: Qiqi Zhou, zhouq523@ustc.edu.cn; Guijian Liu, lgj@ustc.edu.cn; Hongfeng Chen, hongfeng.chen71@163.com