- 1Institut de Recherche sur les Forêts (IRF), Université du Québec en Abitibi-Témiscamingue (UQAT), Rouyn-Noranda, QC, Canada
- 2Conservation Research Group, Biodiversity and Global Change, Universidad de Extremadura, Badajoz, Spain
- 3Conservation Biology Group, Landscape Dynamics and Biodiversity Program, Forest Science and Technology Center of Catalonia (CTFC), Solsona, Spain
Citizen science (CS) has gathered an impressive wealth of open biodiversity data over the last decade, with demonstrated significant scientific contributions in biology and conservation science. However, the contribution of CS in botanical research, and more particularly regarding inconspicuous taxonomic groups such as cryptogams remains largely unexplored. Here we assess the current status and contribution of CS in botanical research, with a special focus on non-vascular “cryptogams” (bryophytes, lichens, fungi, and algae). We conducted a literature review for the period 2012 to 2022 to synthesize the use of CS in botanical studies. We found an increasing trend in the use of CS for botanical research (average annual increase of ∼40%), although highly biased towards vascular plants (246 papers). Cryptogams remained strongly underrepresented (58 papers), although receiving slightly growing attention since 2018. The inconspicuousness nature, high diversity, challenges with species identification, and low public perception of cryptogams not only restrict the contribution made by non-experts but raise concerns about the reliability and robustness of generated data. This is fueled by the scarcity of foundational methodological studies in cryptogams, which seems to undermine the scientific confidence in engaging volunteers for their research or using open data from CS platforms and tools. Despite this, our review showed a gradual adoption of CS approaches for cryptogam research, which is particularly led by mycologists. We highlight the versatility and potential of CS approaches for advancing cryptogam knowledge across various research subjects at spatial and temporal scales otherwise unfathomable by researchers, and provide insights on the opportunities of application and possible solutions to the discussed limitations. We hope our work motivates mycologists, phycologists, bryologists, and lichenologists to further embrace CS, and increase public awareness on these highly sensitive and ecologically important taxa.
1 Introduction
The cumulative pressure on biodiversity driven by the increase of anthropogenic activities and climate change has promoted the development of new approaches to efficiently meet biodiversity monitoring and conservation targets. In response, citizen science (hereafter “CS”), which refers to the public involvement in scientific research, has arisen as a powerful approach for generating scientific knowledge from local to global scales across multiple taxa (Dickinson et al., 2010; McKinley et al., 2017; Pocock et al., 2015). This has been favored by the development and rapid spread of new, user-friendly digital technologies and tools, such as online platforms and smartphone applications, which have fostered the participation of volunteers collaborating with the scientific community in generating new data (Howe, 2006; Maund et al., 2020; Wiggins, 2012). This collaborative approach has led to the generation and storage of a large amount of open biodiversity data (Ball-Damerow et al., 2019; Sullivan et al., 2014), which have provided significant contributions in ecological assessments concerning biodiversity monitoring (Chandler et al., 2017), global change (Theobald et al., 2015), species distribution models (Feldman et al., 2021) or invasive species distributions (Crall et al., 2015).
However, CS contributions, regardless of the diverse nature of volunteers’ motivations (Maund et al., 2020), have been taxonomically biased, with an overrepresentation of zoological studies and, more particularly, those focusing on charismatic mammal and bird species, at the expense of most plants (Follett and Strezov, 2015; McKinley et al., 2017). Regarding CS botanical projects, most of them have primarily focused on vascular plants, thus neglecting inconspicuous but highly diverse non-vascular cryptogam taxa, namely, bryophytes, algae, lichens, and fungi (herein referred to as “cryptogams”; Chandler et al., 2017; Feldman et al., 2021). This botanical bias is highlighted within the European CS biodiversity platform by the absence of cryptogams in any of their botanical initiatives (Wagenknecht et al., 2021). This also aligns with the historical lack of recognition that cryptogams have received from conservation authorities, managers, politicians, and civil society (Scheidegger and Goward, 2002) despite their substantial contributions to overall biodiversity and ecosystem functioning globally (Hawksworth and Lücking, 2017; Li and Chang, 2021; Porada et al., 2014; Porada et al., 2016; Porada et al., 2018), as well as their high sensitivity to environmental disturbances and their subsequent utility as bioindicators (Benítez et al., 2019; Bokhorst et al., 2012). Recent significant efforts, such as IUCN Red Lists at European (Hodgetts et al., 2023) or national level (e.g., Callaghan, 2022; Degtjarenko et al., 2024; Mueller and Dahlberg, 2013), are however gradually increasing the recognition of the biological and ecological value of cryptogams along with their representativeness in conservation planning.
As a megadiverse yet poorly known group, cryptogam species may face extinction before they are even documented, and appropriate conservation actions can be implemented (Cornwell et al., 2019; Theobald et al., 2015; Tulloch et al., 2013). In this sense, CS can play a key role in advancing cryptogam research and conservation efforts, not only by acquiring and generating ecological knowledge but also by raising public awareness about these ecologically important species; although volunteers’ initial awareness or interest can also be essential to their recruitment. It is then particularly important to explore how CS can assist in filling knowledge gaps in botanical sciences to effectively address conservation needs. However, to date, our understanding about the extent to which CS data is being used and its impact on botanical research, especially regarding inconspicuous cryptogam taxa, is still limited. Therefore, the objective of this study is to explore the current status and contribution of CS in botanical research, with special emphasis on inconspicuous cryptogam groups, providing insights on the opportunities of application, potential limitations and possible solutions. Henceforth, we refer to “botany” in its broadest sense, thus including lichens and fungi. To achieve our objective, we assessed i) the temporal trend in the use of CS for botanical studies over the last decade according to each taxonomic group (vascular plants, bryophytes, algae, lichens, and fungi), ii) the representation of these taxonomic groups, as well as the research subjects, across the reviewed articles, and iii) the contribution made by CS exclusively in cryptogam research regarding the type of citizen participation, the type of samples or data provided or used and their geographical scale.
2 Methods
We conducted a literature review of peer-reviewed articles that used CS in botanical studies from 2012 to 2022 using the search engine Scopus. Over the past decade, CS has been recognized and widely accepted among academics as a valuable scientific data source (Fraisl et al., 2022; Wagenknecht et al., 2021). Thus, the selected timeframe encompasses the proliferation of open CS platforms and major advancements in collecting, storing and benefiting from CS data (Kullenberg and Kasperowski, 2016; Vohland et al., 2021).
The literature search was carried out by combining keywords related to CS (“citizen science” OR “public participation” OR “community science” OR “community monitoring program” OR “participatory monitoring”) with terms related to the target taxa (“plant” OR “tree” OR “cryptogam” OR “bryophyte” OR “moss” OR “liverwort” OR sphagn* OR “hornwort” OR anthocero* OR “fungus” OR “fungi” OR “lichen” OR “algae”). The search of these terms was performed across articles’ titles, abstracts, and keywords. This resulted in 891 articles matching our search criteria. These articles were individually examined to identify only those relevant for our research focus. Our article selection was therefore restricted to articles using and/or generating any type of CS data to investigate any aspect of the target taxa, excluding reviews. A total of 294 articles were finally retained for analysis (Supplementary Table S1). A PRISMA flow diagram reporting the article selection process for this review can be found in the supplementary material (Supplementary Figure S1).
In order to achieve our objectives, we extracted from each article the year of publication, target taxa, and central research themes of the study. The taxonomic groups considered were vascular plants (tracheophytes), bryophytes, algae, lichens and fungi. The relative proportion represented by each taxonomic group across the reviewed CS-based articles was also assessed as an indicator of the contribution of CS to the scientific research of the different groups. To explore the various applications of CS in botanical research, both in general and across taxonomic groups, we classified each paper according to 13 research subject categories (Table 1), which were defined a posteriori by grouping their central themes. Note that a given article can focus on multiple taxonomic groups and/or address and thus be classified into multiple subject categories.
To further evaluate the contribution of CS to cryptogam research, we initially distinguished between two types of cryptogam studies as follows: i) articles promoting and based on new citizen contributions (hereafter “new citizen contribution articles”), and ii) articles using publicly available CS data from open data sources (“open CS data articles”). Regarding new citizen contribution articles, we identified for each cryptogam taxonomic group, the research steps in which citizens were involved (new data collection, species identification, data analysis, interpretation of results, or manuscript writing or revision), the type of data (e.g., presence/absence, abundance) or samples contributed (specimens vs. substrate), and the geographic scale of the study (global, national, regional or local). For simplicity, we used the term “biodiversity data” to refer jointly to presence-only, presence/absence, abundance, ecology, diversity and functional trait data. Likewise, the term “substrate samples”, which differs from specimen samples, was used to collectively refer to substrate samples that potentially contain the target species, including soil, dust, leaves, water or skin. On the other hand, regarding open CS data articles, we assessed, also by cryptogam taxonomic group, the type of CS data used, their source platforms, the geographic scale of application, as well as the scale covered by the CS platform data (e.g., iNaturalist provides global coverage, allowing the development of studies from local to global scales). This allowed us to investigate not only the role that citizens can play in cryptogam research, but also the current and potential use of the available CS data by the scientific community. Moreover, by identifying the cryptogam CS databases used and the type of data they provide, we were able to assess the tangible contribution of CS to cryptogam research in terms of availability and nature of the data. However, it should be noted that an exhaustive review of all cryptogam CS data sources currently available is beyond the scope of this review.
We did not conduct a similar detailed examination of CS contribution to vascular plant research. This decision was based on the existence of numerous ongoing global to national scale CS projects and open sources dedicated exclusively to vascular plants. Examples of such projects include Pl@ntNet (Joly et al., 2016), Flora incognita (Mäder et al., 2021) Flora Capture (Boho et al., 2020), and Plant Watch (http://www.naturewatch.ca/plantwatch), as well as platforms like iNaturalist (Mesaglio et al., 2023; Wolf et al., 2022). These projects and platforms already provide extensive data on various aspects of vascular plants including distribution, functional traits, animal interactions, invasive species, ecology, and substrate, among others. Although we are aware that some less conspicuous, and thus more difficult to identify vascular plant groups (e.g., ferns and lycophytes, or even grasses, hedges or rushes) can be underrepresented in vascular plant CS projects compared to trees, shrubs, and flowering plants (e.g., Flensted et al., 2016; Zuquim et al., 2022), this assessment is beyond the scope of this review.
3 Results
3.1 Publication trends and spatial distribution of citizen science-based botanical studies
This review revealed a general increasing trend in the use of CS-derived data for botanical research from 2012 to 2022 (Figure 1A). Specifically, we found an average annual increase in the publication of CS botanical studies of approximately 40%, with an average of 27 articles per year, and a maximum of 74 articles in 2022, which vastly outpaces the increase in scientific articles in general, estimated at 9%–10% per year in 2010 (Bornmann and Mutz, 2015). This increasing publication trend was driven by papers targeting vascular plants (n = 246), with an average annual increase of 39% and an average of 22 articles published each year (Figure 1A). In contrast, studies focusing on cryptogams remain rare, with a total of 58 papers and an average of five articles published by year. Specifically, very few articles used CS for cryptogam research during the first half of the study period, with only nine articles published before 2018. From that date on, a gradual but consistent increase is observed (Figure 1A). This slightly increasing trend was mainly supported by studies focusing on fungi (Figure 1B), the most targeted cryptogam group (n = 34), followed by algae, the second most focused group (n = 14). Lichens and bryophytes were less frequently targeted, with a total of eight articles each. Most of the reviewed papers, either for vascular plants or cryptogams, included CS data and projects carried out in the northern hemisphere, mainly in United States, Canada and Europe (Supplementary Figure S2). These are regions where economic resources available for research and conservation allow greater efforts, and more particularly regarding inconspicuous species such as cryptogams (e.g., Canadian Endangered Species Conservation Council, 2016; Garilleti and Albertos, 2012; Hodgetts et al., 2023).
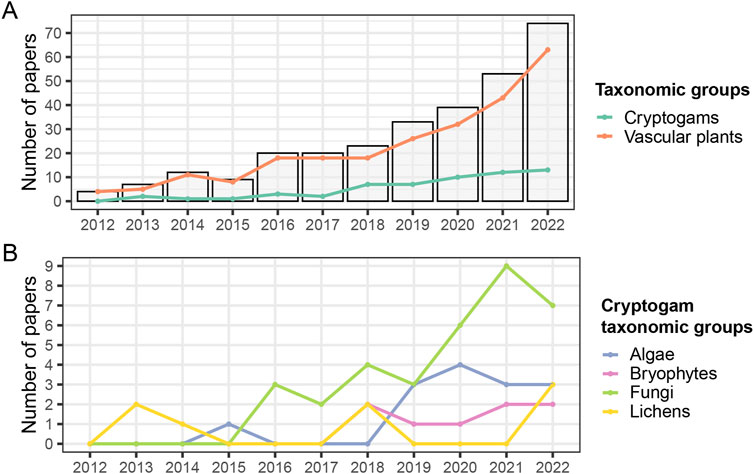
Figure 1. (A) Total number of citizen science-based botanical studies published per year for the period 2012–2022 (bars), as well as split by vascular plant vs. cryptogam taxonomic groups (lines). (B) Number of citizen science-based botanical studies by cryptogam taxonomic group published during the same period.
3.2 Research subjects focused by citizen science-based botanical studies
CS data has focused on a wide variety of research subjects within botanical studies, although they have not been equally represented in the literature. The general research subject patterns across all reviewed articles were driven by vascular plant studies, whereas the patterns identified for cryptograms exhibited more variation (Figure 2). Regarding vascular plants, methodology and application was the top research subject across all reviewed papers, followed by phenology, interspecific interactions, urban ecology, migration and dispersal, species identification, biodiversity monitoring, climate change, new species distribution, and biogeography (Figure 2A). The least studied subjects, were species at risk, land-use changes, and environmental contamination (Figure 2A). For cryptogams, species identification emerged as the main research subject (n = 19), being primarily supported by fungal studies (Figure 2B). Methodology and application was the second most targeted (n = 17), with fungi and algae being the main contributors (Figure 2B), followed by biodiversity monitoring, new species distribution, environmental contamination and urban ecology. Biogeography, species at risk and interspecific interactions were moderately targeted by cryptogam literature, while land-use changes, climate change, migration and dispersal, and phenology were marginally targeted. Environmental contamination was the only subject were cryptogam papers (n = 12) surpassed those focusing on vascular plants (n = 8; Figure 2A).
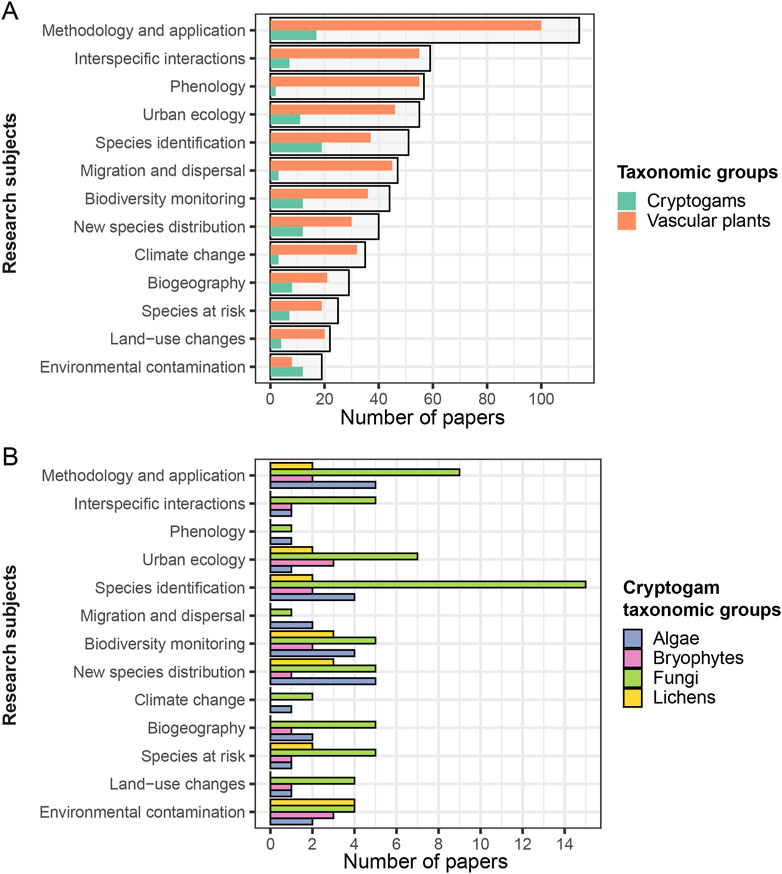
Figure 2. (A) Total number of published citizen science-based botanical articles per research subject category (white bars), as well as by vascular plant (orange bars) and cryptogam taxonomic groups (green bars). (B) Number of published citizen science-based botanical studies per research subject category segmented by cryptogam taxonomic group.
Vascular plants, fungi and algae were represented across all research subject categories (n = 13). Bryophytes and lichens were the least diversified in terms of subjects (n = 10 and 7, respectively), with a lack of papers addressing phenology, migration and dispersal, and climate change for either group, nor on interspecific interactions, biogeography and land-use changes for lichens.
3.3 Citizen science contribution to cryptogam studies
The characterization of both cryptogam new citizen contribution articles and open CS data articles is illustrated in Figure 3. Of the 58 cryptogam papers identified here, 41 consisted in new citizen contribution articles (Figure 3A), which were primarily developed at local (n = 14), regional (n = 9), and national scales (n = 15), with a few at global scale (n = 3). Citizens were always involved in the collection of samples, either of the specimens (n = 9) or their substrate (n = 12), biodiversity data (n = 20), and marginally in algae and bryophyte specimen identification (n = 3). Citizens were not further involved in later research stages such as data analysis, interpretation of results, or manuscript writing or revision. Citizen contributions have mostly been in the form of specimen or substrate samples at local scales, biodiversity data at regional scales, and substrate samples and biodiversity data at both national and global scales. Samples were either of the target specimens when dealing with macroorganisms, or their substrates regarding microorganisms, namely, water for algae (phytoplankton) and soil/dust, leaf and amphibian skin for fungi. Biodiversity data comprised presence-only data for algae, fungi and lichen, presence/absence data exclusively for algae, and presence/absence either plus abundance regarding algae and lichen, or ecology (habitat or substrate) for algae and fungi (Figure 3A). The remaining new citizen contributions were pictures shared across all cryptogam taxa, knowledge and social attitude on algae, diversity and picture-based morphological measurements for bryophytes, and picture-based parataxonomic units for lichens specimen samples.
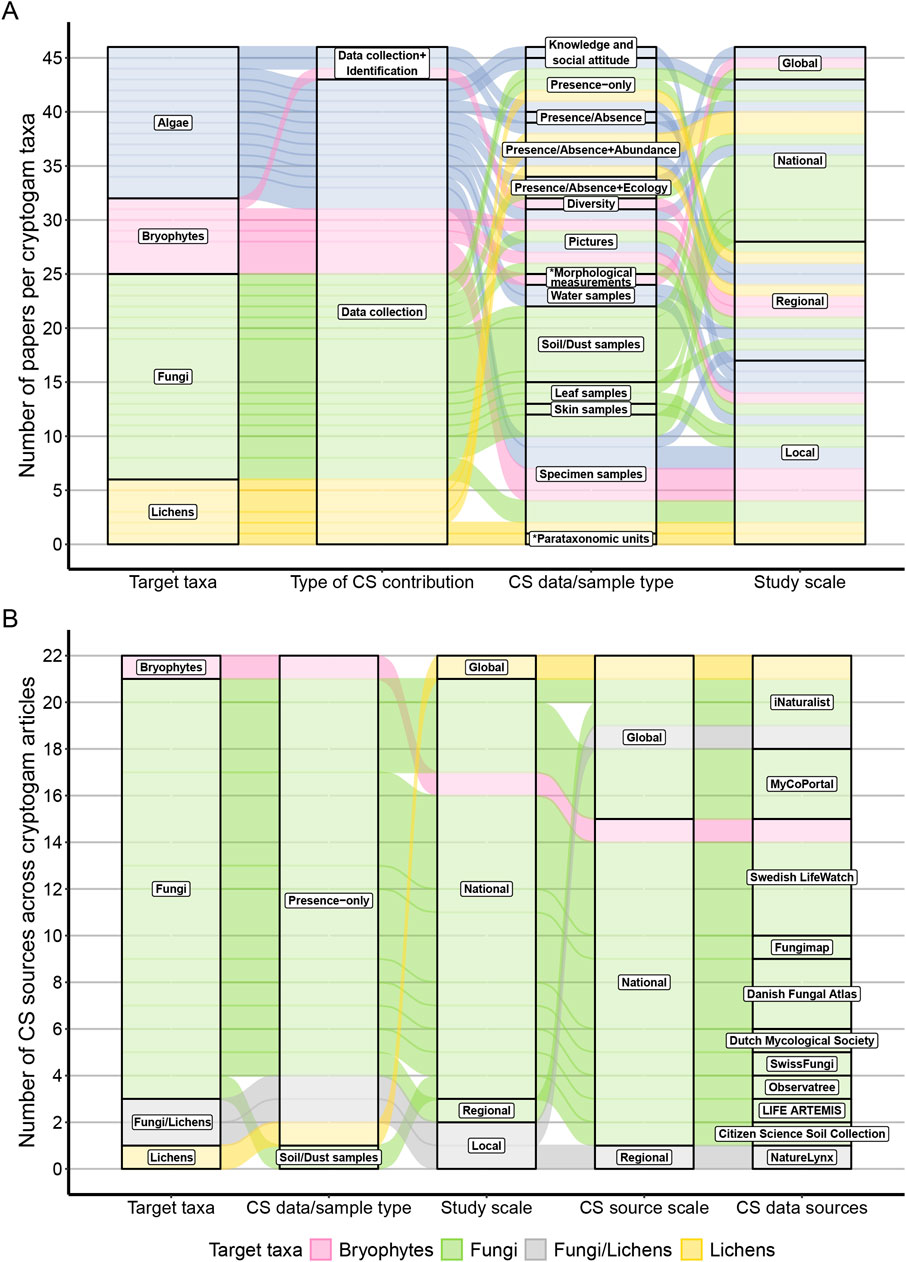
Figure 3. Alluvial diagram relating different aspects of the contribution of citizen science (CS) to cryptogams studies. (A) includes exclusively papers promoting and based on new citizen contributions (i.e., new citizen contribution articles) and relates the cryptogram taxa, the type of contribution made by the participants (or research steps in which they were involved), the type of data or samples they collected, and the scale of the study; note that citizens did not contributed to research steps of data analysis, interpretation of results, and manuscript writing or revision); * in the CS data/sample type column indicates that the data were derived from pictures. (B) includes exclusively the studies where open CS data sources were used, and relates the cryptogam taxa, the type of data or samples used, the scale of the study, the scale covered by the open CS source platforms and the identity of those platforms. Note that the values across the y-axes are sometimes increased due to the inclusion of doubloons, and thus not always correspond with the exact ones presented in the text. Doubloons were included in order to show each cryptogam group independently in (A) by splitting multi-taxa papers, and ídem but splitting multi-CS platform papers in (B). Abbrev.: CS, citizen science.
The number of cryptogam studies using open CS data sources was limited (n = 18), with a predominant focus on fungi (n = 16; Figure 3B). These studies mostly used presence-only data (n = 17), excepting for one article that used soil samples from a pre-existing natural product collection (Anderson et al., 2021). Papers using CS sources targeting lichens, bryophytes and algae have been marginal or inexistent (2, 1 and 0 papers, respectively). A total of 11 different CS open sources were identified across these studies focusing on cryptogams, most of them providing spatial data coverage at national scales (Figure 3B), except for the global platforms iNaturalist and MyCoPortal, as well as the regional platform NatureLynx. Six of the eight national CS platforms were European, excepting for those from Australia and United States. Most studies used national and global platforms to develop their studies at national scales. Only two studies were carried out at either local or regional scale. The most used CS platforms were Swedish LifeWatch (n = 5), iNaturalist (n = 4), MyCoPortal (n = 3) and Danish Fungal Atlas (n = 3).
4 Discussion
The increasing trend in publications on the contribution of CS in botanical research was highly biased towards vascular plants. Cryptogam groups remained strongly underrepresented, despite their substantial contribution to global biodiversity, with approximately 72,500 algae species (Guiry, 2012), 25,000 bryophyte species (Li and Chang, 2021), 20,000 lichen species, and 148,000 fungal species of an estimated total of 2.2–3.8 million species (Hawksworth and Lücking, 2017), compared to around 350,000 vascular plants species (Antonelli et al., 2020). This bias is not unexpected, not only since many vascular plants are more conspicuous and charismatic, but because CS cryptogam research consistently requires a higher level of expertise and/or commitment. Efforts to promote CS vascular plant research are highlighted by the high number of methodology and application studies, offering insights into its applicability and limitations (Follett and Strezov, 2015). This has boosted botanists’ confidence in using CS vascular plant data over the last decade, rising research across various subjects (Supplementary Figure S3). We identified a slight growing trend in CS-based cryptogam research since 2017, which was almost non-existent before then. This reflects a gradual acceptance of CS by the scientific community, which coincides with increased public interest in cryptogams (Munzi and Giovanetti, 2021). However, CS contributions to these inconspicuous species remains poor and skewed towards fungi, which accounts for 59% of the CS cryptogam papers. Public awareness on cryptogams is also negligible compared to conspicuous taxa as vascular plants, birds, or large mammals (Cardoso et al., 2011; Deacon et al., 2023; Scheidegger and Goward, 2002). This is paradoxical, as CS can raise public perception of cryptogams and bridge knowledge gaps on fundamental and crucially informative parameters for their conservations such as diversity, ecology, and distribution (Cerrejón, 2022). As evidenced here, fungi illustrate how this paradox can be addressed. Mycologists have pioneered innovative efforts involving participatory approaches coupled with DNA barcoding to generate microfungal data across scales (Estensmo et al., 2022; Grantham et al., 2020; Hou et al., 2020), or to detect pathogenic fungi (EA et al., 2016; Ellingham et al., 2019; Garbelotto et al., 2020). Furthermore, mycologists have particularly and almost exclusively benefited from using diverse open data sources for their research, which has been infrequent (bryophytes, lichens) or even absent (algae) for other cryptogam groups. This underscores that mycologists have particularly capitalized on the use of CS for advancing fungal knowledge (e.g., Ellingham et al., 2019; Grantham et al., 2020; Heilmann-Clausen et al., 2019).
Cryptogams pose great challenges for scientific research, since they encompass highly diverse groups of small sized organisms that require specialized training and tools for identification (Munzi et al., 2023). This complexity hinders non-experts’ contributions, as highlighted in this review, with public involvement often restricted to early research stages related to data or sample collection, with marginal participation in macro-specimen identification. Public involvement in later research stages (data analysis, interpretation of results, etc.) would require considerable scientific and academic skills and/or knowledge of species taxonomy, ecology, physiology, etc., which makes it even more challenging. These limitations are not unique to cryptogams, but can also apply to other inconspicuous or cryptic taxonomic groups such as micro- or macroinvertebrates, or less conspicuous vascular plants (e.g., ferns, lycophytes, grasses), which face similar issues when implementing participatory approaches for their research (e.g., Ashcroft et al., 2012; Deacon et al., 2023; Flensted et al., 2016; Gąsiorek et al., 2024). Specifically in our review, citizens normally collected biodiversity data such as presence/absence, abundance, etc., for easily recognizable species (e.g., Ellul et al., 2019), or contributed through photographs (e.g., Tucker and La Farge, 2021), or by providing specimens (e.g., McMullin et al., 2018) or substrate samples (e.g., Mascioni et al., 2019). Identification tasks were only feasibly when volunteers were either specialists (Neyens et al., 2019), had extensive experience (Verlaque and Breton, 2019), or received prior identification training (Vye et al., 2020).
Despite such limitations, the wide range of research subjects identified across the reviewed studies underscored the versatility of CS in advancing cryptogam knowledge. CS allows for collection of cryptogam-related data, such as their presence at easily recognizable taxonomic levels, cover or abundance at wide spatial and temporal scales, often exceeding the capacity of traditional research (Pocock et al., 2014). This is particularly useful for studies on new species distributions, biogeography, or climate change (Vye et al., 2020). Using certain cryptogam species as bioindicators, CS supports research on biodiversity monitoring, environmental contamination, urban ecology, and land-use changes (Kondo et al., 2022; Mair et al., 2018; Seed et al., 2013). Alternative CS approaches to species-level data, such as parataxonomic units based on external morphology (Krell, 2004) as proxies for species richness (Casanovas et al., 2014; Oliver and Beattie, 1993), have proven effective for environmental contamination and biodiversity monitoring. Furthermore, CS can enhance research on species at risk by detecting rare events (Pocock et al., 2014), which can help identify new, vulnerable, or threatened cryptogam species (McMullin et al., 2018). This can also benefit studies on species identification regarding the discovery of new species (Giraldo et al., 2019), and interspecific interactions studies focusing on cryptogam-induced diseases (Crow et al., 2020; EA et al., 2016). The usefulness of CS for discovering new species discovery and assessing interspecific interactions is further demonstrated by a very recent Danish CS project called “Mass Experiment, which successfully assessed and mapped the diversity and distribution of tardigrades, along and their bryophyte and lichen hosts across Denmark, and led to the discovery of new tardigrade species (Gąsiorek et al., 2024). Migration and dispersal could also take advantage of CS in monitoring harmful invasions such as algal blooms (Esenkulova et al., 2021) or the spread of invasive bryophytes (Ewald et al., 2020). Furthermore, CS projects using field photographs or digitized natural collections are valuable for characterizing morphological and phenological traits falling into species identification and phenology research subjects (Hedrick et al., 2020; von Konrat et al., 2018). Phenology studies could be further promoted by documenting cryptogam reproductive periods (e.g., appearance of sporophytes in mosses) in areas frequented by citizens. Through its wide range of applications, CS offers invaluable ecological insights into cryptogam biodiversity, with important implications for management and conservation strategies.
Yet the inconspicuous nature and diversity of cryptogams raises concerns about the reliability and robustness of CS data. Methodology and application studies have been scarce and have yielded diverging and sometimes discouraging results. McMullin and Allen (2022) and Munzi et al. (2023) revealed a high rate (41% and 70%, respectively) of misclassified lichen species in iNaturalist. In contrast, users correctly identified 92.82% of macrobasidiomycete observations through the FungiVision tool of the Atlas of Danish Fungi (Picek et al., 2022), with which volunteer mycologists have traditionally shown a high degree of commitment and training for their identification. Detection and taxonomic biases in cryptogams in open CS data sources tend to favor more common or conspicuous organisms, thereby limiting the reliability of specific groups. For instance, within bryophytes, liverworts and hornworts are usually overshadowed by mosses in CS platforms (e.g., iNaturalist; accessed 6 March 2024), while microlichens are also underrepresented compared to macrolichens (Munzi et al., 2023). Likewise, CS contributions to rare and sparsely distributed habitat specialists remain minimal (Lõhmus et al., 2023). Furthermore, opportunistic CS can introduce spatial and temporal biases affecting the quality of inferences, although methods are available to mitigate these issues (Geldmann et al., 2016; Kosmala et al., 2016). While some studies have shown consistency in drawing conclusions from spatially biased CS data (Mair et al., 2017), others emphasized the need to combine opportunistic data with spatially unbiased gold-standard data for reliable results (Neyens et al., 2019). Addressing these biases from the outset is crucial when designing CS projects (Geldmann et al., 2016). All of this indicates that the low use of CS for cryptogam research derives from the skepticism and caution of botanists in trusting this data (Munzi et al., 2023; White et al., 2023).
To address these concerns, further foundational methodology and application studies are critical to mitigate errors and biases (Follett and Strezov, 2015) and establish best practises to ensure that data quality (Lõhmus et al., 2023). As CS accuracy varies with task complexity and data type (Kosmala et al., 2016), additional research involving expert validation would clarify their potential and limitations. New and ongoing CS initiatives and derived research can benefit from i) revisiting research questions according to the known limitations associated to the target organisms ii) the design and standardization of data collection methods (Fraisl et al., 2020; Schacher et al., 2023), iii) reinforcing volunteers’ sampling and identification skills through training (e.g., EA et al., 2016; Tregidgo et al., 2013; Vye et al., 2020), iv) the joint work of citizens and experts during fieldwork (Heilmann-Clausen et al., 2016; Heilmann-Clausen et al., 2019), and v) targeting easily identifiable indicator species (Seed et al., 2013; Welden et al., 2018). On widely used platforms, shared observations of difficult-to-identify taxa should be accompanied by detailed information on relevant structures, key distinctive characteristics, and habitat (McMullin and Allen, 2022). Stricter requirements to validate observations for inconspicuous taxa in CS platforms can enhance scientific confidence. Mandatory fields at various taxonomic levels supported with expert validation are crucial for ensuring data quality and provide continuous feedback to increase usability in science. Since the proposed strategies require greater commitment from volunteers, their implementation should carefully consider the trade-off between the number of volunteers willing to participate under these standards and the quality of the data collected. Nonetheless, these collaborative approaches would be a win-win situation: volunteers can contribute to science and nature conservation while gaining familiarity with poorly known inconspicuous taxa, and the scientific community can benefit from improved data quality for their research endeavors.
5 Conclusion
This review represents a significant effort in understanding and highlighting the important role that CS is playing in botany, and provides the first comprehensive assessment of its contribution to cryptogam research. Vascular plants have increasingly benefited from CS approaches over the past decade and this trend is likely to persist in the future. The high diversity and inconspicuous nature of cryptogams, however, hinder citizen involvement and compromise data quality. The scarcity of methodological studies undermines scientific confidence in engaging volunteers in cryptogam research and using open CS data. Despite this, the adoption of CS for cryptogam research is gradual increasing, particularly led by mycologists. We hope our work will inspire mycologists, phycologists, bryologists, and lichenologists to further embrace CS. Strengthening the collaboration between citizens and researchers and securing financial funding and institutional support are critical for advancing our knowledge of these neglected organisms. Future endeavors should focus on identifying existing cryptogam CS open data sources, and elucidating the nature of the data they provide, which would undoubtedly encourage the representation of these inconspicuous taxa in CS-based botanical research.
Data availability statement
The original contributions presented in the study are included within the article and Supplementary Material. Further inquiries can be directed to the corresponding author.
Author contributions
CC: Conceptualization, Data curation, Formal Analysis, Investigation, Methodology, Project administration, Resources, Software, Supervision, Validation, Visualization, Writing–original draft, Writing–review and editing. MN: Conceptualization, Data curation, Formal Analysis, Investigation, Methodology, Resources, Software, Validation, Visualization, Writing–review and editing. NF: Conceptualization, Funding acquisition, Methodology, Validation, Writing–review and editing. M-FI: Data curation, Writing–review and editing. MF: Conceptualization, Data curation, Formal Analysis, Investigation, Methodology, Project administration, Resources, Software, Supervision, Validation, Visualization, Writing–original draft, Writing–review and editing.
Funding
The author(s) declare that no financial support was received for the research, authorship, and/or publication of this article.
Acknowledgments
We greatly appreciate the comments provided by Osvaldo Valeria and Louis Imbeau on the first versions of this paper. We thank the reviewers for their valuable suggestions, which have greatly improved the quality of this manuscript.
Conflict of interest
The authors declare that the research was conducted in the absence of any commercial or financial relationships that could be construed as a potential conflict of interest.
Publisher’s note
All claims expressed in this article are solely those of the authors and do not necessarily represent those of their affiliated organizations, or those of the publisher, the editors and the reviewers. Any product that may be evaluated in this article, or claim that may be made by its manufacturer, is not guaranteed or endorsed by the publisher.
Supplementary material
The Supplementary Material for this article can be found online at: https://www.frontiersin.org/articles/10.3389/fenvs.2024.1448512/full#supplementary-material
References
Anderson, V. M., Wendt, K. L., Najar, F. Z., McCall, L. I., and Cichewicz, R. H. (2021). Building natural product libraries using quantitative clade-based and chemical clustering strategies. Msystems 6, e00644-21. doi:10.1128/msystems.00644-21
Antonelli, A., Smith, R. J., Fry, C., Simmonds, M. S., Kersey, P. J., Pritchard, H. W., et al. (2020). State of the world’s plants and fungi. Kew (UK): Royal Botanic Gardens. Available at: https://hal.science/hal-02957519/.
Ashcroft, M. B., Gollan, J. R., and Batley, M. (2012). Combining citizen science, bioclimatic envelope models and observed habitat preferences to determine the distribution of an inconspicuous, recently detected introduced bee (Halictus smaragdulus Vachal Hymenoptera: halictidae) in Australia. Biol. Invasions 14, 515–527. doi:10.1007/s10530-011-0092-x
Ball-Damerow, J. E., Brenskelle, L., Barve, N., Soltis, P. S., Sierwald, P., Bieler, R., et al. (2019). Research applications of primary biodiversity databases in the digital age. PloS One 14, e0215794. doi:10.1371/journal.pone.0215794
Benítez, Á., Medina, J., Vásquez, C., Loaiza, T., Luzuriaga, Y., and Calva, J. (2019). Lichens and bromeliads as bioindicators of heavy metal deposition in Ecuador. Diversity 11, 28. doi:10.3390/d11020028
Boho, D., Rzanny, M., Wäldchen, J., Nitsche, F., Deggelmann, A., Wittich, H. C., et al. (2020). Flora Capture: a citizen science application for collecting structured plant observations. BMC bioinf 21, 576. doi:10.1186/s12859-020-03920-9
Bokhorst, S., Bjerke, J. W., Tømmervik, H., Preece, C., and Phoenix, G. K. (2012). Ecosystem response to climatic change: the importance of the cold season. Ambio 41, 246–255. doi:10.1007/s13280-012-0310-5
Bornmann, L., and Mutz, R. (2015). Growth rates of modern science: a bibliometric analysis based on the number of publications and cited references. J. Assoc. Inf. Sci. Technol. 66, 2215–2222. doi:10.1002/asi.23329
Callaghan, D. A. (2022). A new IUCN red list of the bryophytes of britain, 2023. J. Bryol. 44, 271–389. doi:10.1080/03736687.2023.2185393
Canadian Endangered Species Conservation Council (2016). Wild species 2015: the general status of species in Canada. Natl. General Status Work. Group, 128. Available at: https://wildlife-species.canada.ca/species-risk-registry/virtual_sara/files/reports/Wild%20Species%202015.pdf.
Cardoso, P., Erwin, T. L., Borges, P. A., and New, T. R. (2011). The seven impediments in invertebrate conservation and how to overcome them. Biol. Conserv. 144, 2647–2655. doi:10.1016/j.biocon.2011.07.024
Casanovas, P., Lynch, H. J., and Fagan, W. F. (2014). Using citizen science to estimate lichen diversity. Biol. Conserv. 171, 1–8. doi:10.1016/j.biocon.2013.12.020
Cerrejón, C. (2022). Understanding the biodiversity patterns of cryptogams (bryophytes and lichens) in boreal forests through remote sensing. Univ. Québec Abitibi-Témiscamingue. Available at: https://depositum.uqat.ca/id/eprint/1381/.
Chandler, M., See, L., Copas, K., Bonde, A. M., López, B. C., Danielsen, F., et al. (2017). Contribution of citizen science towards international biodiversity monitoring. Biol. Conserv. 213, 280–294. doi:10.1016/j.biocon.2016.09.004
Cornwell, W. K., Pearse, W. D., Dalrymple, R. L., and Zanne, A. E. (2019). What we (don't) know about global plant diversity. Ecography 42, 1819–1831. doi:10.1111/ecog.04481
Crall, A. W., Jarnevich, C. S., Young, N. E., Panke, B. J., Renz, M., and Stohlgren, T. J. (2015). Citizen science contributes to our knowledge of invasive plant species distributions. Biol. Invasions 17, 2415–2427. doi:10.1007/s10530-015-0885-4
Crow, P., Perez-Sierra, A., Kavčič, A., Lewthwaite, K., Kolšek, M., Ogris, N., et al. (2020). Using Citizen Science to monitor the spread of tree pests and diseases: outcomes of two projects in Slovenia and the UK. Manage. Biol. Invasions 11, 703–719. doi:10.3391/mbi.2020.11.4.06
Deacon, C., Govender, S., and Samways, M. J. (2023). Overcoming biases and identifying opportunities for citizen science to contribute more to global macroinvertebrate conservation. Biodiver. Conserv. 32, 1789–1806. doi:10.1007/s10531-023-02595-x
Degtjarenko, P., Kaupuža, R., Motiejūnaitė, J., Randlane, T., and Moisejevs, R. (2024). Toward the first Red List of Latvian lichens according to the IUCN criteria. Plant Biosyst. - Int. J. Deal. Asp. Plant Biosyst. 158, 1244–1252. doi:10.1080/11263504.2024.2399056
Dickinson, J. L., Zuckerberg, B., and Bonter, D. N. (2010). Citizen science as an ecological research tool: challenges and benefits. Annu. Rev. Ecol. Evol. Syst. 41, 149–172. doi:10.1146/annurev-ecolsys-102209-144636
Ea, G., Pope, K. L., Wengert, G. M., Foley, J. E., Ashton, D. T., and Botzler, R. G. (2016). Citizen scientists monitor a deadly fungus threatening amphibian communities in northern coastal California, USA. J. Wildl. Dis. 52, 516–523. doi:10.7589/2015-10-280
Ellingham, O., David, J., and Culham, A. (2019). Enhancing identification accuracy for powdery mildews using previously underexploited DNA loci. Mycologia 111, 798–812. doi:10.1080/00275514.2019.1643644
Ellul, T., Evans, J., and Schembri, P. J. (2019). Invasion alert: rapid range expansion of Caulerpa taxifolia var. distichophylla in Maltese waters (central Mediterranean). BioInvasions Rec. 8, 208–217. doi:10.3391/bir.2019.8.2.02
Esenkulova, S., Suchy, K. D., Pawlowicz, R., Costa, M., and Pearsall, I. A. (2021). Harmful algae and oceanographic conditions in the Strait of Georgia, Canada based on citizen science monitoring. Front. Mar. Sci. 8, 725092. doi:10.3389/fmars.2021.725092
Estensmo, E. L. F., Smebye Botnen, S., Maurice, S., Martin-Sanchez, P. M., Morgado, L., Bjorvand Engh, I., et al. (2022). The indoor mycobiomes of daycare centers are affected by occupancy and climate. Appl. Environ. Microbiol. 88, e0211321. doi:10.1128/aem.02113-21
Ewald, M., Skowronek, S., Aerts, R., Lenoir, J., Feilhauer, H., Van De Kerchove, R., et al. (2020). Assessing the impact of an invasive bryophyte on plant species richness using high resolution imaging spectroscopy. Ecol. Indic. 110, 105882. doi:10.1016/j.ecolind.2019.105882
Feldman, M. J., Imbeau, L., Marchand, P., Mazerolle, M. J., Darveau, M., and Fenton, N. J. (2021). Trends and gaps in the use of citizen science derived data as input for species distribution models: a quantitative review. PloS One 16, e0234587. doi:10.1371/journal.pone.0234587
Flensted, K. K., Bruun, H. H., Ejrnæs, R., Eskildsen, A., Thomsen, P. F., and Heilmann-Clausen, J. (2016). Red-listed species and forest continuity–A multi-taxon approach to conservation in temperate forests. For. Ecol. Manage. 378, 144–159. doi:10.1016/j.foreco.2016.07.029
Follett, R., and Strezov, V. (2015). An analysis of citizen science based research: usage and publication patterns. PloS One 10, e0143687. doi:10.1371/journal.pone.0143687
Fraisl, D., Campbell, J., See, L., Wehn, U., Wardlaw, J., Gold, M., et al. (2020). Mapping citizen science contributions to the UN sustainable development goals. Sustain. Sci. 15, 1735–1751. doi:10.1007/s11625-020-00833-7
Fraisl, D., Hager, G., Bedessem, B., Gold, M., Hsing, P. Y., Danielsen, F., et al. (2022). Citizen science in environmental and ecological sciences. Nat. Rev. Methods Prim. 2, 64. doi:10.1038/s43586-022-00144-4
Garbelotto, M., Popenuck, T., Hall, B., Schweigkofler, W., Dovana, F., Goldstein de Salazar, R., et al. (2020). Citizen science uncovers Phytophthora ramorum as a threat to several rare or endangered California Manzanita species. Plant Dis. 104, 3173–3182. doi:10.1094/PDIS-03-20-0619-RE
Garilleti, R., and Albertos, B. (2012). “Atlas y libro rojo de los briófitos amenazados de España,” in Organismo autónomo parques nacionales. Madrid, 228. Available at: https://www.miteco.gob.es/content/dam/miteco/es/biodiversidad/temas/inventarios-nacionales/briofitos_tcm30-198033.pdf.
Gąsiorek, P., Sørensen, M. V., Lillemark, M. R., Leerhøi, F., and Tøttrup, A. P. (2024). Massive citizen science sampling and integrated taxonomic approach unravel Danish cryptogam-dwelling tardigrade fauna. Front. Zool. 21, 27. doi:10.1186/s12983-024-00547-x
Geldmann, J., Heilmann-Clausen, J., Holm, T. E., Levinsky, I., Markussen, B. O., Olsen, K., et al. (2016). What determines spatial bias in citizen science? Exploring four recording schemes with different proficiency requirements. Divers. Distrib. 22, 1139–1149. doi:10.1111/ddi.12477
Giraldo, A., Hernández-Restrepo, M., and Crous, P. W. (2019). New plectosphaerellaceous species from Dutch garden soil. Mycol. Prog. 18, 1135–1154. doi:10.1007/s11557-019-01511-4
Grantham, N. S., Reich, B. J., Laber, E. B., Pacifici, K., Dunn, R. R., Fierer, N., et al. (2020). Global forensic geolocation with deep neural networks. J. R. Stat. Soc. Ser. C Appl. Stat. 69, 909–929. doi:10.1111/rssc.12427
Guiry, M. D. (2012). How many species of algae are there? J. Phycol. 48, 1057–1063. doi:10.1111/j.1529-8817.2012.01222.x
Hawksworth, D. L., and Lücking, R. (2017). Fungal diversity revisited: 2.2 to 3.8 million species. Microbiol. Spectr. 5, 5–4. doi:10.1128/microbiolspec.funk-0052-2016
Hedrick, B. P., Heberling, J. M., Meineke, E. K., Turner, K. G., Grassa, C. J., Park, D. S., et al. (2020). Digitization and the future of natural history collections. Biosci 70, 243–251. doi:10.1093/biosci/biz163
Heilmann-Clausen, J., Bruun, H. H., Ejrnæs, R., Frøslev, T. G., Læssøe, T., and Petersen, J. H. (2019). How citizen science boosted primary knowledge on fungal biodiversity in Denmark. Biol. Conserv. 237, 366–372. doi:10.1016/j.biocon.2019.07.008
Heilmann-Clausen, J., Maruyama, P. K., Bruun, H. H., Dimitrov, D., Læssøe, T., Frøslev, T. G., et al. (2016). Citizen science data reveal ecological, historical and evolutionary factors shaping interactions between woody hosts and wood-inhabiting fungi. New Phytol. 212, 1072–1082. doi:10.1111/nph.14194
Hodgetts, N., Cálix, M., Englefield, E., Fettes, N., Criado, M. G., Patin, L., et al. (2023). A miniature world in decline: European red list of mosses, liverworts and hornworts. Brussels, Belgium: IUCN. doi:10.2305/IUCN.CH.2019.ERL.2.en
Hou, L., Hernández-Restrepo, M., Groenewald, J. Z., Cai, L., and Crous, P. W. (2020). Citizen science project reveals high diversity in Didymellaceae (Pleosporales, Dothideomycetes). MycoKeys 65, 49–99. doi:10.3897/mycokeys.65.47704
Howe, J. (2006). The rise of crowdsourcing. Wired Mag. 14, 1–4. Available at: https://sistemas-humano-computacionais.wdfiles.com/local--files/capitulo%3Aredes-sociais/Howe_The_Rise_of_Crowdsourcing.pdf.
Joly, A., Bonnet, P., Goëau, H., Barbe, J., Selmi, S., Champ, J., et al. (2016). A look inside the Pl@ ntNet experience: the good, the bias and the hope. Multimed. Syst. 22, 751–766. doi:10.1007/s00530-015-0462-9
Kondo, M. C., Zuidema, C., Moran, H. A., Jovan, S., Derrien, M., Brinkley, W., et al. (2022). Spatial predictors of heavy metal concentrations in epiphytic moss samples in Seattle, WA. Sci. Total Environ. 825, 153801. doi:10.1016/j.scitotenv.2022.153801
Kosmala, M., Wiggins, A., Swanson, A., and Simmons, B. (2016). Assessing data quality in citizen science. Front. Ecol. Environ. 14, 551–560. doi:10.1002/fee.1436
Krell, F.-T. (2004). Parataxonomy vs. taxonomy in biodiversity studies–pitfalls and applicability of ‘morphospecies’ sorting. Biodiv. Conserv. 13, 795–812. doi:10.1023/B:BIOC.0000011727.53780.63
Kullenberg, C., and Kasperowski, D. (2016). What is citizen science? A scientometric meta-analysis. PloS One 11, e0147152. doi:10.1371/journal.pone.0147152
Li, H., and Chang, C. (2021). Evolutionary insight of plant cuticle biosynthesis in bryophytes. Plant Signal. and Behav. 16, 1943921. doi:10.1080/15592324.2021.1943921
Lõhmus, P., Degtjarenko, P., Lotman, S., Copoț, O., Rosenvald, R., and Lõhmus, A. (2023). “Ready! Set! Lichen!”: a citizen-science campaign for lichens, against the odds of success. Biodiv. Conserv. 32, 4753–4765. doi:10.1007/s10531-023-02724-6
Mäder, P., Boho, D., Rzanny, M., Seeland, M., Wittich, H. C., Deggelmann, A., et al. (2021). The flora incognita app—interactive plant species identification. Methods Ecol. Evol. 12, 1335–1342. doi:10.1111/2041-210X.13611
Mair, L., Harrison, P. J., Jönsson, M., Löbel, S., Nordén, J., Siitonen, J., et al. (2017). Evaluating citizen science data for forecasting species responses to national forest management. Ecol. Evol. 7, 368–378. doi:10.1002/ece3.2601
Mair, L., Jönsson, M., Räty, M., Bärring, L., Strandberg, G., Lämås, T., et al. (2018). Land use changes could modify future negative effects of climate change on old-growth forest indicator species. Divers. Distrib. 24, 1416–1425. doi:10.1111/ddi.12771
Mascioni, M., Almandoz, G. O., Cefarelli, A. O., Cusick, A., Ferrario, M. E., and Vernet, M. (2019). Phytoplankton composition and bloom formation in unexplored nearshore waters of the western Antarctic Peninsula. Polar Biol. 42, 1859–1872. doi:10.1007/s00300-019-02564-7
Maund, P. R., Irvine, K. N., Lawson, B., Steadman, J., Risely, K., Cunningham, A. A., et al. (2020). What motivates the masses: understanding why people contribute to conservation citizen science projects. Biol. Conserv. 246, 108587. doi:10.1016/j.biocon.2020.108587
McKinley, D. C., Miller-Rushing, A. J., Ballard, H. L., Bonney, R., Brown, H., Cook-Patton, S. C., et al. (2017). Citizen science can improve conservation science, natural resource management, and environmental protection. Biol. Conserv. 208, 15–28. doi:10.1016/j.biocon.2016.05.015
McMullin, R. T., and Allen, J. L. (2022). An assessment of data accuracy and best practice recommendations for observations of lichens and other taxonomically difficult taxa on iNaturalist. Botany 100, 491–497. doi:10.1139/cjb-2021-0160
McMullin, R. T., Drotos, K., Ireland, D., and Dorval, H. (2018). Diversity and conservation status of lichens and allied fungi in the Greater Toronto Area: results from four years of the Ontario BioBlitz. Can. Field Nat. 132, 394–406. doi:10.22621/cfn.v132i4.1997
Mesaglio, T., Sauquet, H., Coleman, D., Wenk, E., and Cornwell, W. K. (2023). Photographs as an essential biodiversity resource: drivers of gaps in the vascular plant photographic record. New Phytol. 238, 1685–1694. doi:10.1111/nph.18813
Mueller, G. M., and Dahlberg, A. (2013). The global fungal red list initiative. Inoculum 64, e3. Available at: https://www.fao.org/fileadmin/user_upload/GSP/NETSOB/launch-2021/013_Gregory_Mueller.pdf.
Munzi, S., and Giovanetti, M. (2021). Wanted: virtual or live! How lichens are becoming part of mass internet culture. Symbiosis 84, 285–293. doi:10.1007/s13199-021-00780-6
Munzi, S., Isocrono, D., and Ravera, S. (2023). Can we trust iNaturalist in lichenology? Evaluating the effectiveness and reliability of artificial intelligence in lichen identification. Lichenologist 55, 193–201. doi:10.1017/S0024282923000403
Neyens, T., Diggle, P. J., Faes, C., Beenaerts, N., Artois, T., and Giorgi, E. (2019). Mapping species richness using opportunistic samples: a case study on ground-floor bryophyte species richness in the Belgian province of Limburg. Sci. Rep. 9, 19122. doi:10.1038/s41598-019-55593-x
Oliver, I., and Beattie, A. J. (1993). A possible method for the rapid assessment of biodiversity. Conserv. Biol. 7, 562–568. doi:10.1046/j.1523-1739.1993.07030562.x
Picek, L., Šulc, M., Matas, J., Heilmann-Clausen, J., Jeppesen, T. S., and Lind, E. (2022). Automatic fungi recognition: deep learning meets mycology. Sensors 22, 633. doi:10.3390/s22020633
Pocock, M. J., Chapman, D. S., Sheppard, L. J., and Roy, H. E. (2014). Choosing and Using Citizen Science: a guide to when and how to use citizen science to monitor biodiversity and the environment. Wallingford: NERC/Centre for Ecology and Hydrology. Available at: https://nora.nerc.ac.uk/id/eprint/510644/.
Pocock, M. J., Roy, H. E., Preston, C. D., and Roy, D. B. (2015). The Biological Records Centre: a pioneer of citizen science. Biol. J. Linn. Soc. 115, 475–493. doi:10.1111/bij.12548
Porada, P., Weber, B., Elbert, W., Pöschl, U., and Kleidon, A. (2014). Estimating impacts of lichens and bryophytes on global biogeochemical cycles. Global Biogeochem. Cycles 28, 71–85. doi:10.1002/2013GB004705
Porada, P., Ekici, A., and Beer, C. (2016). Effects of bryophyte and lichen cover on permafrost soil temperature at large scale. The Cryosphere 10, 2291–2315. doi:10.5194/tc-10-2291-2016
Porada, P., VanStan, J. T., and Kleidon, A. (2018). Significant contribution of non-vascular vegetation to global rainfall interception. Nat. Geosci. 11, 563–567. doi:10.1038/s41561-018-0176-7
Schacher, A., Roger, E., Williams, K. J., Stenson, M. P., Sparrow, B., and Lacey, J. (2023). Use-specific considerations for optimising data quality trade-offs in citizen science: recommendations from a targeted literature review to improve the usability and utility for the calibration and validation of remotely sensed products. Remote Sens. 15, 1407. doi:10.3390/rs15051407
Scheidegger, C., and Goward, T. (2002). “Monitoring lichens for conservation: red lists and conservation action plans,” in Monitoring with lichens—monitoring lichens (Dordrecht: Springer). doi:10.1007/978-94-010-0423-7_12
Seed, L., Wolseley, P., Gosling, L., Davies, L., and Power, S. A. (2013). Modelling relationships between lichen bioindicators, air quality and climate on a national scale: results from the UK OPAL air survey. Environ. Pollut. 182, 437–447. doi:10.1016/j.envpol.2013.07.045
Sullivan, B. L., Aycrigg, J. L., Barry, J. H., Bonney, R. E., Bruns, N., Cooper, C. B., et al. (2014). The eBird enterprise: an integrated approach to development and application of citizen science. Biol. Conserv. 169, 31–40. doi:10.1016/j.biocon.2013.11.003
Theobald, E. J., Ettinger, A. K., Burgess, H. K., DeBey, L. B., Schmidt, N. R., Froehlich, H. E., et al. (2015). Global change and local solutions: tapping the unrealized potential of citizen science for biodiversity research. Biol. Conserv. 181, 236–244. doi:10.1016/j.biocon.2014.10.021
Tregidgo, D. J., West, S. E., and Ashmore, M. R. (2013). Can citizen science produce good science? Testing the OPAL Air Survey methodology, using lichens as indicators of nitrogenous pollution. Environ. Pollut. 182, 448–451. doi:10.1016/j.envpol.2013.03.034
Tucker, D., and La Farge, C. (2021). Bryophyte communities in quercus garryana ecosystems on south east vancouver island: preliminary mesohabitat assessment. Bryologist 124, 198–217. doi:10.1639/0007-2745-124.2.198
Tulloch, A. I., Possingham, H. P., Joseph, L. N., Szabo, J., and Martin, T. G. (2013). Realising the full potential of citizen science monitoring programs. Biol. Conserv. 165, 128–138. doi:10.1016/j.biocon.2013.05.025
Verlaque, M., and Breton, G. (2019). Biological invasion: long term monitoring of the macroalgal flora of a major European harbor complex. Mar. Pollut. Bull. 143, 228–241. doi:10.1016/j.marpolbul.2019.04.038
Vohland, K., Land-Zandstra, A., Ceccaroni, L., Lemmens, R., Perelló, J., Ponti, M., et al. (2021). The science of citizen science. Springer Nature. doi:10.1007/978-3-030-58278-4
von Konrat, M., Campbell, T., Carter, B., Greif, M., Bryson, M., Larraín, J., et al. (2018). Using citizen science to bridge taxonomic discovery with education and outreach. Appl. Plant Sci. 6, e1023. doi:10.1002/aps3.1023
Vye, S. R., Dickens, S., Adams, L., Bohn, K., Chenery, J., Dobson, N., et al. (2020). Patterns of abundance across geographical ranges as a predictor for responses to climate change: evidence from UK rocky shores. Divers. Distrib. 26, 1357–1365. doi:10.1111/ddi.13118
Wagenknecht, K., Woods, T., Sanz, F. G., Gold, M., Bowser, A., Rüfenacht, S., et al. (2021). EU-Citizen. Science: a platform for mainstreaming citizen science and open science in Europe. Data Intell. 3, 136–149. doi:10.1162/dint_a_00085
Welden, N., Wolseley, P., and Ashmore, M. (2018). Citizen science identifies the effects of nitrogen deposition, climate and tree species on epiphytic lichens across the UK. Environ. Pollut. 232, 80–89. doi:10.1016/j.envpol.2017.09.020
White, E., Soltis, P. S., Soltis, D. E., and Guralnick, R. (2023). Quantifying error in occurrence data: comparing the data quality of iNaturalist and digitized herbarium specimen data in flowering plant families of the southeastern United States. PLoS One 18, e0295298. doi:10.1371/journal.pone.0295298
Wiggins, A. (2012). Crowdsourcing scientific work: a comparative study of technologies, processes, and outcomes in citizen science. Syracuse, NY: Syracuse University. Available at: https://www.proquest.com/openview/17a891562714874135b4756d23c1228c/1?pq-origsite=gscholar&cbl=18750.
Wolf, S., Mahecha, M. D., Sabatini, F. M., Wirth, C., Bruelheide, H., Kattge, J., et al. (2022). Citizen science plant observations encode global trait patterns. Nat. Ecol. Evol. 6, 1850–1859. doi:10.1038/s41559-022-01904-x
Keywords: algae, bryophytes, community science, fungi, lichens, public participation, vascular plants, volunteers
Citation: Cerrejón C, Noualhaguet M, Fenton NJ, Indorf M-F and Feldman MJ (2025) Inconspicuous taxa in citizen science-based botanical research: actual contribution, limitations, and new opportunities for non-vascular cryptogams. Front. Environ. Sci. 12:1448512. doi: 10.3389/fenvs.2024.1448512
Received: 15 June 2024; Accepted: 16 December 2024;
Published: 07 January 2025.
Edited by:
Robert Guralnick, University of Florida, United StatesReviewed by:
Stefano Martellos, University of Trieste, ItalyNatalie Eva Iwanycki Ahlstrand, University of Copenhagen, Denmark
Copyright © 2025 Cerrejón, Noualhaguet, Fenton, Indorf and Feldman. This is an open-access article distributed under the terms of the Creative Commons Attribution License (CC BY). The use, distribution or reproduction in other forums is permitted, provided the original author(s) and the copyright owner(s) are credited and that the original publication in this journal is cited, in accordance with accepted academic practice. No use, distribution or reproduction is permitted which does not comply with these terms.
*Correspondence: Carlos Cerrejón, Y2FybG9zY2xAdW5leC5lcw==
†These authors have contributed equally to this work and share first authorship