- 1Key Laboratory of Wetland Ecology and Environment, Northeast Institute of Geography and Agroecology, Chinese Academy of Sciences, Changchun, China
- 2Key Laboratory of Songliao Aquatic Environment, Ministry of Education, Jilin Jianzhu University, Changchun, China
- 3School of Municipal and Environmental Engineering, Jilin Jianzhu University, Changchun, China
Introduction: Microplastics are characterized by their small size, widespread distribution, and durability, present a significant environmental risk. Despite their omnipresence in terrestrial and aquatic systems, the potential consequences on nutrient cycling remain under-investigated. Microplastics have emerged as a focal point of current research, presenting both a challenge and a frontier in environmental science.
Methods: This study explores the effects of microplastics on the high-resolution, in situ distribution and exchange dynamics of key nutrients, nitrogen (N) and phosphorus (P), at the soil-water interface in rice paddies, utilizing the Diffusive Gradients in Thin-films (DGT) technique.
Results: Our results reveal distinct spatial distribution patterns for N and P across the soil-water interface. Labile phosphorus (P) concentrations were significantly higher in the soil than in the overlying water, whereas DGT-NO3− concentrations exhibited the inverse trend. Different microplastic concentrations notably impacted DGT-NO3− (P = 0.022) and DGT-NH4+ (P = 0.033), with an increase between 27.79% and 150.68%. Moreover, different particle sizes significantly influenced NH4+. Interestingly, paddy soil acted as a “source” for labile P and a “sink” for NH4+ and NO3−.
Discussion: These insights provide valuable insights into the interactions between microplastics and nutrient cycles at the soil-water interface, and assess the effects on nutrient migration and transformation. The outcomes of this study will contribute to an improved understanding of the broader ecological implications of microplastic pollution in agricultural settings. It will also provide a foundation for the development of strategies to manage and mitigate the impacts of microplastic pollution in agricultural soils, particularly in rice dominated agroecosystems.
1 Introduction
Microplastics (MPs), initially termed by Professor Richard Thompson in 2004 (Thompson et al., 2004), are heterogeneous plastic particles less than 5 mm in diameter. Owing to their low density, diminutive size, and lightweight nature (Thompson et al., 2004), MPs have proliferated extensively, permeating diverse environments such as soils, water bodies, and the atmosphere (Fuller and Gautam, 2016). Recent research has detected the presence of microplastics in human tissues, including the lungs, placenta, and blood (Cavaliere and Baulch, 2018; Amato-Lourenço et al., 2021; Leslie et al., 2022), suggesting their widespread human exposure. Additionally, traces of MPs have been detected in seemingly pristine environments like Antarctic snowfall, the abyssal regions of the North Atlantic, and even the summit of Mount Everest (Pabortsava and Lampitt, 2020), revealing their global pervasiveness. Microplastics in the environment are susceptible to self-induced physical adsorption, with potential long-term persistence and accumulation in soil matrices. As accumulated microplastic concentrations escalate to specific thresholds, they could impede the growth, development, and reproductive processes of various organisms (Qiguo et al., 2006), presenting a potential hazard to human health and safety (Lombardi et al., 2022). Consequently, microplastics have emerged as a focal point of current research, presenting both a challenge and a frontier in environmental science. (Tirkey and Upadhyay, 2021; Davtalab et al., 2023).
China, in 2020, accounted for approximately 70% of the world’s mulch usage, the largest global share, equating to around 135.7 × 104t (Jing et al., 2023). Agricultural mulch is resistant to natural degradation processes, leading to widespread residual deposits in the soil, and persisting for decades, or even centuries (Qiguo et al., 2006). Microplastic density in soil has been reported to be between 4 and 23 times higher than in marine environments, yet soil microplastic pollution reports represent less than one-third of those related to the ocean (Yang and He, 2021). The infiltration of microplastics into soil significantly impedes the activities of soil microorganisms, disrupting key ecological processes like nitrogen and phosphorus nutrient cycling, thereby affecting seed germination and plant growth (De Silva et al., 2022). Microplastics impact the nitrogen and phosphorus cycling flora function, mainly through the activity of soil microorganisms and associated enzymes, thereby affecting soil nitrogen transformation, as well as the broader environmental nitrogen transformation via effects on nitrification and denitrification processes (Seeley et al., 2020). The influence of microplastics on soil is multifaceted and associated with various factors, such as microplastic particle size and concentration (Zhang et al., 2014).
The soil-water interface in paddy fields is an active ecological junction where nitrogen and phosphorus cycling frequently occurs and is influenced by environmental factors like pH, redox potential, and dissolved oxygen (Fan et al., 2004). It has been found that microplastic addition in soils disrupts the nitrogen and phosphorus cycles (Hu et al., 2021; Yin et al., 2023). Contrary to the prevailing notion that microplastics negatively impact the environment, some suggest that microplastics could contribute to soil carbon stocks, thus influencing the biogeochemical cycling of nitrogen (Lu et al., 2021). Yet, the addition of microplastics disrupts the ecological health of paddy soils, reduces soil microbial diversity, and may negatively impact the migration and release of nitrogen and phosphorus (Wang et al., 2022). Therefore, a more thorough examination of the effects of microplastics on nitrogen and phosphorus migration and release at the soil-water interface in rice fields is necessary.
Previous studies have found that microplastic addition reduced microbial activity and altered enzymatic activities associated with the nitrogen cycle with increased Ammonia monooxygenase activity and decreased nitrate reductase activity (Huang et al., 2020), reducing the release of nitrogen and phosphorus from the sediment to the overlying water (Yu et al., 2022). Microplastics can alter the dispersion and surface properties of sedimental minerals (Lu et al., 2023), thereby may influence nutrient accumulation and transportation at the sediment–water interface. Moreover, microplastic addition restrained worm bioturbation activities and thus decreased the contribution of tubificid worms on organic matter mineralization and nutrient fluxes at the sediment–water interface (Wazne et al., 2023). However, current research primarily focuses on the impact of microplastics on nitrogen and phosphorus migration and transformation in laboratory experimentations, with limited in situ studies evaluating the effect of microplastics on nitrogen and phosphorus concentration and distribution in the soil-water interface in rice fields.
Thin Film Diffusion Gradient Technology (DGT), an in situ passive sampling technique, is based on the Fick’s first law for measuring non-stable components that can pass the diffusion layer and be accumulated by the binding layer, namely the labile concentrations (Zhang et al., 2014). It has been effectively used for in situ quantification of labile concentrations of elements in the water-sediment and soil interface zones (Li et al., 2020). Recently developed multi-phase DGTs (e.g., ZrO-Chelex and ZrO-AgI) enhance simultaneous acquisition of multiple target analytes, making it a powerful tool for understanding crucial elemental biogeochemical processes (Han et al., 2015; Wang et al., 2017).
Northeast China, known as an important commercial grain base and one of four major black soil areas in the world, has been reported to be increasing in the usage of agricultural mulch, leading to issues of land quality degradation and microplastic pollution (Yan et al., 2022). In this study, we propose to utilize DGT technology to investigate the concentration and distribution of labile nitrogen and phosphorus at the soil-water interface in rice fields. We will consider black soil regions with different sizes of microplastic particles (10 um and 80 um) and varying concentrations, and aim to elucidate the distribution pattern and source-sink characteristics of microplastics in the soil-water interface of these rice fields.
The goals of this study were to provide valuable insights into the interactions between microplastics and nutrient cycles at the soil-water interface, and assess the effects on nutrient migration and transformation. The outcomes of this study will contribute to an improved understanding of the broader ecological implications of microplastic pollution in agricultural settings. It will also provide a foundation for the development of strategies to manage and mitigate the impacts of microplastic pollution in agricultural soils, particularly in rice-dominated agroecosystems. Given the scale of rice farming in China and the increasing reliance on agricultural mulch, our findings will hold significant importance, not only for the ecological health of our farmlands, but also for the sustainability of our food production systems.
2 Materials and methods
2.1 Experimental design and sample analysis
The experiments were conducted in the experimental greenhouse base from April to October 2021. Dry soil was thoroughly mixed and placed in flowerpots of 25 cm in length, 20 cm in width, and 28 cm in height with 8 kg for each pot. Five full-height seedlings of the Longjing 31 rice variety were planted in each pot. The experiment included four microplastics (MPs; polyvinyl chloride, PVC) treatments and a control group (CK), with each group consisting of four replicates. Microplastics of differing concentrations and particle sizes were added, as follows: 5 g/kg of 10 μm (high concentration addition with small size, HS), 0.5 g/kg of 10 μm (low concentration addition with small size, LS), 0.5 g/kg of 80 μm (low concentration addition with large size, LL), and 5 g/kg of 80 μm (high concentration addition with large size, HL). The pots were randomly arranged in the greenhouse, and the rice plants were watered following standard management practices.
All the ZrO-AT DGT devices were provided by EasySensor Ltd (Nanjing, China). After rice maturation in October, the ZrO-AT binding gel devices were carefully inserted vertically into the soil-water interface within each treatment, allowing for a 72-h deployment period. During this deployment period, water temperature measurements were collected in triplicate. Subsequently, all the DGT probes were retrieved from the soil and rinsed meticulously with deionized water. The ZrO-AT binding gel pieces were then precisely sliced into 2 mm intervals using a ceramic cutter, following the established protocol as described in previous studies (Menzies et al., 2005; Ding et al., 2015). Each slice was subjected to elution using 1.0M NaOH, facilitating the subsequent analysis of phosphorus (P) concentrations through the application of the phenanthroline colorimetric method. Similarly, the concentrations of DGT-ammonium ions (NH4+) and nitrate ions (NO3−) were determined using a segmented flow analyzer.
2.2 Data analysis
The concentrations of labile nitrogen and phosphorus at the soil-water interface of the paddy field were calculated according to the following formula CDGT (mg/L) (Heidari et al., 2017).
where M represents the total amount of target analyte adsorbed by DGT (mg); Δg is the total thickness between the diffusion and the filter membrane; D is the diffusion coefficient of the target substance within the diffusion film (cm2·s-1); A is the opening area of DGT device (cm2); and T represents the placement time (s) of the DGT device.
Exchange fluxes (F) at the sediment-water interface were estimated according to Fick’s first Law, as follows (Xu et al., 2012):
where F is the material exchange flux at the interface (mg/(m2·d)); Fs represents the exchange flux in the sediment; Fw is the exchange flux in the overlying water; ∂cs/∂xs denotes the concentration gradient in the sediment; ∂cw/∂xw denotes the concentration gradient in the overlying water; xs is the sediment depth (cm); cs is the ion concentration in the sediment (mg/L); xw is the depth of overlying water (cm); cw is the ion concentration in the overlying water (mg/L); and Ds is the diffusion coefficient in the sediment (cm2/s), which can be calculated from the molecular diffusion coefficient in the water (Dw) and the porosity of the surface sediment (φ).
The Origin software (Origin 2021) was employed to construct the distribution profiles of labile nitrogen and phosphorus concentrations at the soil-water interface. Two-way ANOVA was performed using SPSS Statistics 26 software (IBM Corporation, USA) to assess the effects of different concentrations and particle sizes of microplastics, and their combined effects on the labile nitrogen and phosphorus concentrations. The effects of microplastic addition were further analyzed by the Duncan’s multiple comparison (p < 0.05).
3 Results
3.1 Distribution patterns of labile nitrogen and phosphorus at the soil-water interface under different microplastic treatments
The distribution patterns of nitrogen (N) and phosphorus (P) concentrations at the soil-water interface in rice fields under different microplastic treatments are shown in Figure 1. The concentrations of labile P ranged from 0.03 to 0.79 mg/L, NH4+ from 0.01 to 0.24 mg/L, and NO3− from 0.13 to 2.37 mg/L (Figure 1). The overall trend of labile P remained consistent across different microplastic addition treatments, showing low concentrations in the overlying water but increasing with soil depth with a peak at a soil depth of 75–85 mm. The concentration of NH4+ at the soil-water interface exhibited relative stability, while the concentration of NO3− in the overlying water decreased with depth, reaching a minimum value at the soil-water interface, and then exhibited an increasing trend with further soil depth.
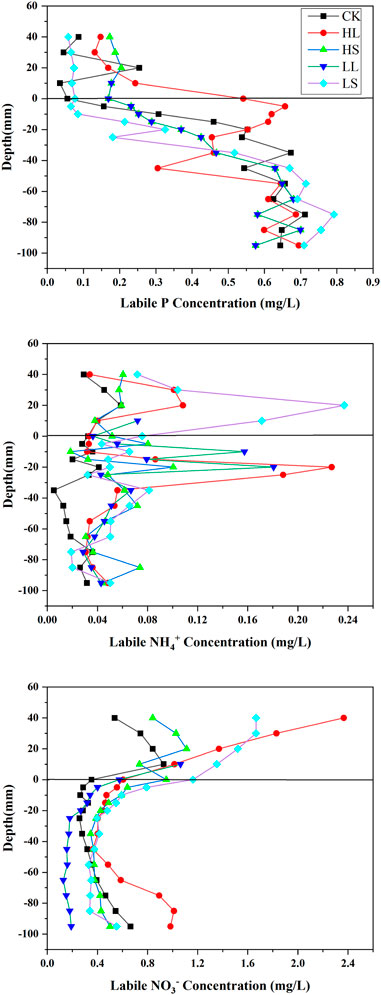
FIGURE 1. Trends in labile N and P distributions at the soil-water interface in rice paddy across under different microplastic treatments. The x-axis represents the depth of overlying water (positive values) and the depth of sediments (negative values). CK, control group; HS, high concentration of microplastics, 5 g/kg, small size–10 μm; LS, low concentration of microplastics, 0.5 g/kg, small size–10 μm; LL, low concentration of microplastics, 0.5 g/kg, large size–80 μm; and HL, high concentration of microplastics, 5 g/kg, large size–80 μm. Note: The depth for treatment LL was shorter 25 mm due to accidently broken.
Our results reveal complex relationships between the presence of microplastics and the labile N and P concentrations in our experimental conditions (Figure 2). The addition of different concentrations and particle sizes of microplastics had significant effects on NH4+ and NO3− concentrations (Table 1), with no significant effect on the labile P (p > 0.05) (Table 1). In comparison with CK, the labile NH4+ concentration increased from 84.83% to 150.68%, and NO3− increased from 27.79% to 81.80% under different treatments (Figure 2). Furthermore, the interaction effects of microplastic concentration and particle sizes had a significant effect on the labile NO3− concentration (p < 0.001).
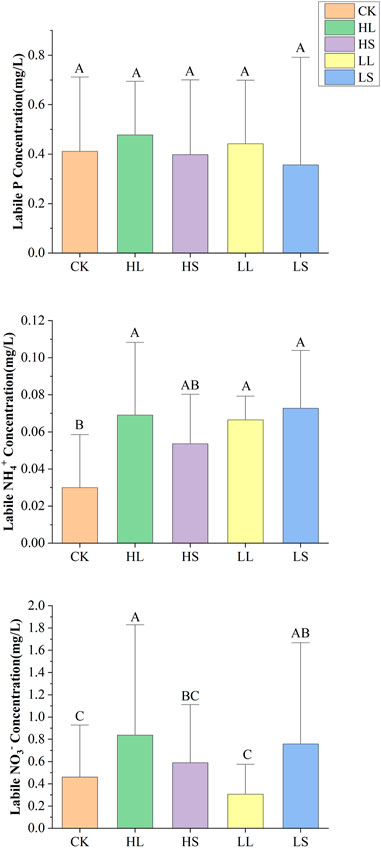
FIGURE 2. Concentrations of labile N and P at the soil-water interface in rice fields under microplastic treatment. Different uppercase letters represent significant differences.

TABLE 1. Two-way ANOVA for labile N and P at the soil-water interface in rice fields under microplastic addition treatments. *: p < 0.05; **: p < 0.01; ***: p < 0.001.
3.2 Sink-source dynamics of labile N and P fluxes under microplastic treatments
The NH4+, NO3− and P fluxes were determined based on Fick’s first law (Figure 3). Positive flux values imply that the labile N and P are being released from the soil to the overlying water, hence acting as a source. Conversely, negative values suggest that nutrients migrate from the overlying water into the soil, acting as a sink. The diffusion flux of labile P varied from −0.09–0.64 mg/d·m2 in all treatments, and the mean value for different treatments was 0.32 mg/d·m2, indicating that the labile P source came from soil. The diffusion fluxes of labile NH4+ ranged from −9.52 to 0.75 mg/d·m2, and the mean value for different treatments was −2.17 mg/d·m2. The diffusion fluxes of labile NO3− ranged from −2.82 to 1.22 mg/d·m2, and the mean value for different treatments was −0.85 mg/d·m2. Thus, both of NH4+ and NO3− exhibited the characteristics of “sink” in soil.
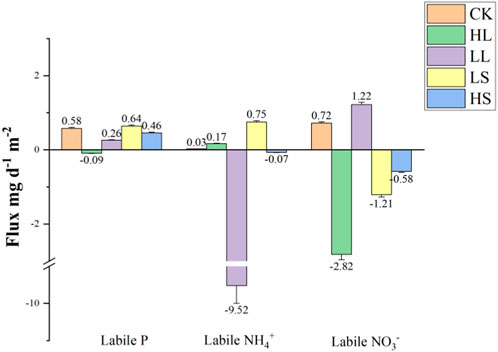
FIGURE 3. Diffusion fluxes of labile N and P at the soil-water interface under different treatments.
The mean values of diffusion fluxes of labile N and P fluxes in the CK treatment were positive, while the addition treatments of different concentrations and particle sizes of microplastics changed the direction of fluxes (Figure 3). Especially, the diffusion flux of NH4+ with a low concentration of 80 μm (LL group) changed from 0.03 to −9.52 mg/d·m2, indicating a shift from source to sink. The addition of microplastics also enlarged the NO3− flux from 0.72 to 1.22 mg/d·m2 in the LL treatment.
4 Discussion
4.1 Distribution patterns of vertical gradient of nutrients in overlying water and soil
Distinct spatial heterogeneity in the concentrations of labile P, NH4+-N, and NO3−-N at the soil-water interface were found via the DGT technique. The fact that concentrations of labile P increased with soil depth is primarily attributed to the process of leaching in the soil profile and the uptake of rice. Our results indicated there were no significant effects of microplastic addition on labile P concentrations, which is consistent with precious study that PVC MPs have no significant short-term effect on phosphorus cycling in soil-water systems (Yin et al., 2023).
The concentration trend of NH4+ at the soil-water interface remained relatively stable, while NO3− concentration in the overlying water was significantly higher than those in the soil. Because the surface of soil particles is capable of absorbing NH4+, this adsorption can limit NH4+ migration and leaching in the soil, so as to keep NH4+ concentration at the interface relatively stable (Liu et al., 2008). In addition, negatively charged ion-exchange complexes such as clay minerals and organic matter were present in the soil. These negative charges are capable of binding NH4+. The addition of microplastics increased soil porosity, increased oxygen flux, and increased nitrification (Lu et al., 2021). Besides, oxygen is usually sufficient in the overlying water, which is conducive to the nitrification process and increases the concentration of NO3− in the overlying water (Wang et al., 2002). Microplastics also lead to increased nitrate reductase (NR) activity in soil, which affects nitrogen utilization and promotes labile NO3− to labile NH4+ conversion (Yu et al., 2022). Moreover, MPs used in our study can promote the abundance of microbial denitrification genes in the soil (Yin et al., 2023), leading to low NO3− concentrations in soil.
The penetration depth of dissolved oxygen in soil is usually limited to a few centimeters of the sediment depth, and the environment becomes more reduced as depth increased and oxygen is depleted. Under anoxic conditions in deep soil, the need for an electron acceptor for the decomposition of organic matter by the obligate anaerobic bacteria and anaerobic bacteria may lead to the reduction of labile NO3− as an alternative electron acceptor (Pabortsava and Lampitt, 2020). The concentration of labile NO3− in overlying water was significantly higher than that in the soil.
The addition of microplastic significantly increased the concentrations of NH4+ and NO3− at the soil-water interface (Figure 2), because microplastics can act as organic substrates and release of additives in microplastics (Seeley et al., 2020). The surfaces on the microplastic particles provide many available biofilms that are ideal environments for the attachment and growth of nitrifying bacteria. This process of attachment and growth increases the number of nitrifying bacteria and further catalyzes the nitrification reaction, this leads to an increase in labile NO3− concentration (Chen et al., 2020). PVC microplastics can reduce the abundance amoA, nirS and nirK, which leads to an increase in labile NH4+ concentrations (Seeley et al., 2020). It was reported that microplastics affect microbial community composition, enzyme activity and gene abundance associated with nitrogen cycling, which in turn affects nitrogen cycling processes and increases NH4+ concentrations (Zhang et al., 2014; Liu et al., 2017; Ren et al., 2018). PVC microplastics reduce the concentration of denitrifying bacteria and inhibit the removal of NO3− (Ding et al., 2019).
4.2 Exchange fluxes of labile nitrogen and phosphorus under different treatments
The average phosphorus exchange flux at the soil-water interface presented a positive value, implying that phosphorus diffused from soil to water. Notably, the presence of microplastics did not significantly influence the flux of phosphorus, regardless of the differences in microplastic concentration and particle size. Conversely, the average exchange fluxes of NH4+ and NO3− were negative, demonstrating a “sink” characteristic, where these substances migrated from water to soil. The translocation of labile nitrogen from the water to the soil was dictated not only by the concentration gradient at the soil-water interface in the rice fields, but also by microplastic adsorption (Sun et al., 2022). PVC microplastic adsorption in the soil obstructed the transfer of labile nitrogen from the soil to the water surface (Sun et al., 2022), and thus promoted the soil as a sink of nitrogen.
Smaller microplastic particles are more likely to impact soil porosity and aeration (Kumar et al., 2022). This not only accelerates the flux rate of labile nitrogen, but also enhances oxygen diffusion, thereby promoting nitrification and reducing NH4+ concentrations. High concentrations of microplastics can alter enzymatic activities related to nitrification and denitrification, facilitating the conversion of NO3− to N2. Besides, compared with low MPs concentration treatments, high MPs concentrations can strongly affect NH4+ metabolism via altering microbial denitrification genes abundance, and influence NO3− metabolism via regulating the abundance of nirS and nirK genes and Nitrospirae related to nitrogen cycle (Sun et al., 2022). Moreover, the migration of active nitrogen from the water column to the soil is driven by both oxygen enrichment and concentration gradients in the water (Zhu et al., 2022). Overall, microplastic particle size and concentrations strongly impacted nutrient cycles at the soil-water interface, particular in nitrogen.
5 Conclusion
In this study, the thin-film diffusion gradient technique was employed to obtain in situ, high-resolution information about key nutrients at the soil-water interface in the rice paddy. There were distinct spatial distribution patterns for labile N and labile P across the soil-water interface under different treatments. The addition of microplastic significantly increased the concentrations of NO3− and NH4+ by affecting the nitrification and denitrification processes. Additionally, the presence of microplastics significantly changed the source and sink characteristics of NO3− and NH4+ fluxes. Our study offers a theoretical foundation for understanding the in situ distribution patterns and source-sink characteristics of nutrients impacted by microplastics at the soil-water interface in rice fields.
Data availability statement
The raw data supporting the conclusion of this article will be made available by the authors, without undue reservation.
Author contributions
YJ and XZ wrote the manuscript. XZ proposed and structured the study. YJ, SZ, JY, and YW performed the experiments. SZ, JY, and YW made the figures. XZ and YL reviewed and improved the language of the manuscript and secured funding acquisition. All authors contributed to the article and approved the submitted version.
Funding
This research was financially supported by the Natural Science Foundation of Jilin Province (YDZJ202201ZYTS480).
Acknowledgments
The authors would like to thank QC Wang, Z Wu and XQ Dong for their preparation for the data, and YQ Wang for the field work in the greenhouse.
Conflict of interest
The authors declare that the research was conducted in the absence of any commercial or financial relationships that could be construed as a potential conflict of interest.
Publisher’s note
All claims expressed in this article are solely those of the authors and do not necessarily represent those of their affiliated organizations, or those of the publisher, the editors and the reviewers. Any product that may be evaluated in this article, or claim that may be made by its manufacturer, is not guaranteed or endorsed by the publisher.
References
Amato-Lourenço, L. F., Carvalho-Oliveira, R., Júnior, G. R., dos Santos Galvão, L., Ando, R. A., and Mauad, T. (2021). Presence of airborne microplastics in human lung tissue. J. Hazard. Mater. 416, 126124. doi:10.1016/j.jhazmat.2021.126124
Cavaliere, E., and Baulch, H. M. (2018). Denitrification under lake ice. Biogeochemistry 137 (3), 285–295. doi:10.1007/s10533-018-0419-0
Chen, X., Chen, X., Zhao, Y., Zhou, H., Xiong, X., and Wu, C. (2020). Effects of microplastic biofilms on nutrient cycling in simulated freshwater systems. Sci. Total Environ. 719, 137276. doi:10.1016/j.scitotenv.2020.137276
Davtalab, M., Byčenkienė, S., and Uogintė, I. (2023). Global research hotspots and trends on microplastics: A bibliometric analysis. Environ. Sci. Pollut. Res. 2023, 1–16. doi:10.1007/s11356-023-27647-1
De Silva, Y. S. K., Rajagopalan, U. M., Kadono, H., and Li, D. (2022). Effects of microplastics on lentil (Lens culinaris) seed germination and seedling growth. Chemosphere 303, 135162. doi:10.1016/j.chemosphere.2022.135162
Ding, J., Jiang, F., Li, J., Wang, Z., Sun, C., Wang, Z., et al. (2019). Microplastics in the coral reef systems from xisha islands of south China sea. Environ. Sci. Technol. 53 (14), 8036–8046. doi:10.1021/acs.est.9b01452
Ding, S., Han, C., Wang, Y., Yao, L., Wang, Y., Xu, D., et al. (2015). In situ, high-resolution imaging of labile phosphorus in sediments of a large eutrophic lake. Water Res. 74, 100–109. doi:10.1016/j.watres.2015.02.008
Fan, C. X., Zhang, L., Qin, B. Q., Hu, W. P., Gao, G., and Wang, J. J. (2004). Migration mechanism of biogenic elements and their quantification on the sediment-water interface of lake taihu: I. Spatial variation of the ammonium release rates and its source and sink fluxes. J. Lake Sci. 16 (1), 11–21. doi:10.18307/2004.0102
Fuller, S., and Gautam, A. (2016). A procedure for measuring microplastics using pressurized fluid extraction. Environ. Sci. Technol. 50 (11), 5774–5780. doi:10.1021/acs.est.6b00816
Han, C., Ding, S., Yao, L., Shen, Q., Zhu, C., Wang, Y., et al. (2015). Dynamics of phosphorus–iron–sulfur at the sediment–water interface influenced by algae blooms decomposition. J. Hazard. Mater. 300, 329–337. doi:10.1016/j.jhazmat.2015.07.009
Heidari, S., Reyhanitabar, A., and Oustan, S. (2017). Kinetics of phosphorus desorption from calcareous soils using DGT technique. Geoderma 305, 275–280. doi:10.1016/j.geoderma.2017.06.012
Hu, X. J., Liu, J. J., Wang, H., and Wang, G. H. (2021). Impacts of microplastics on soil environmental quality and microbial ecological characteristics. Chin. J. Soil Sci. 52 (6), 1479–1485. doi:10.19336/j.cnki.trtb.2021022501
Huang, Y., Liu, Q., Jia, W., Yan, C., and Wang, J. (2020). Agricultural plastic mulching as a source of microplastics in the terrestrial environment. Environ. Pollut. 260, 114096. doi:10.1016/j.envpol.2020.114096
Jing, W., Chen, T., Jie, Y., Ying, L., Shuopei, H., Yuanqing, B., et al. (2023). Effects of microplastics on soil physicochemical properties, soil microbial community structure and function in farmland. J. Ecol. Rural Environ. 39 (05), 644–652. doi:10.19741/j.issn.1673-4831.2023.0112
Kumar, A., Mishra, S., Pandey, R., Yu, Z. G., Kumar, M., Khoo, K. S., et al. (2022). Microplastics in terrestrial ecosystems: Un-ignorable impacts on soil characterises, nutrient storage and its cycling. TrAC Trends Anal. Chem. 158, 116869. doi:10.1016/j.trac.2022.116869
Leslie, H. A., van Velzen, M. J. M., Brandsma, S. H., Vethaak, A. D., Garcia-Vallejo, J. J., and Lamoree, M. H. (2022). Discovery and quantification of plastic particle pollution in human blood. Environ. Int. 163, 107199. doi:10.1016/j.envint.2022.107199
Li, X. Y., Teng, H., and Zhao, Y. J. (2020). Application of diffusive gradients in thin-films (DGT) technique in studying solute transport processes across environmental micro-interfaces. Journal of Agro-Environment Science 39 (8), 1649–1660. doi:10.11654/jaes.2020-009
Liu, H., Yang, X., Liu, G., Liang, C., Xue, S., Chen, H., et al. (2017). Response of soil dissolved organic matter to microplastic addition in Chinese loess soil. Chemosphere 185, 907–917. doi:10.1016/j.chemosphere.2017.07.064
Liu, Y. L., Zhang, B., Li, C. L., Hu, F., and Velde, B. (2008). Long-term fertilization influences on clay mineral composition and ammonium adsorption in a rice paddy soil. Soil Sci. Soc. Am. J. 72 (6), 1580–1590. doi:10.2136/sssaj2007.0040
Lombardi, G., Di Russo, M., Zjalic, D., Lanza, T., Simmons, M., Moscato, U., et al. (2022). Microplastics inhalation and their effects on human health: A systematic review. Eur. J. Public Health 32 (03), 131–152. doi:10.1093/eurpub/ckac131.152
Lu, X., Hu, H., Li, J., Li, J., Wang, L., Liu, L., et al. (2023). Microplastics existence affected heavy metal affinity to ferrihydrite as a representative sediment mineral. Sci. Total Environ. 859, 160227. doi:10.1016/j.scitotenv.2022.160227
Lu, P., Yan, Z. H., and Lu, G. H. (2021). Influence of microplastics on nitrogen cycle in different environments. Research of Environmental Sciences 34 (11), 2563–2570. doi:10.13198/j.issn.1001-6929.2021.08.08
Menzies, N. W., Kusumo, B., and Moody, P. W. (2005). Assessment of P availability in heavily fertilized soils using the diffusive gradient in thin films (DGT) technique. Plant soil 269, 1–9. doi:10.1007/s11104-004-1725-y
Pabortsava, K., and Lampitt, R. S. (2020). High concentrations of plastic hidden beneath the surface of the Atlantic Ocean. Nat. Commun. 11 (1), 4073. doi:10.1038/s41467-020-17932-9
Qiguo, Z., Shenglu, Z., Shaohua, W., and ku, R. (2006). Changes of cultivated land resources in China and its sustainable utilization and protection countermeasures. Acta Pedol. sin. 43 (4), 662–672. doi:10.11766/200511020420
Ren, X., Tang, J., Yu, C., and He, J. (2018). Advances in research on the ecological effects of microplastic pollution on soil ecosystems. J. Agro-Environment Sci. 37 (6), 1045–1058. doi:10.11654/jaes.2017-1409
Seeley, M. E., Song, B., Passie, R., and Hale, R. C. (2020). Microplastics affect sedimentary microbial communities and nitrogen cycling. Nat. Commun. 11 (1), 2372. doi:10.1038/s41467-020-16235-3
Sun, X., Zhang, X., Xia, Y., Tao, R., Zhang, M., Mei, Y., et al. (2022a). Simulation of the effects of microplastics on the microbial community structure and nitrogen cycle of paddy soil. Sci. Total Environ. 818, 151768. doi:10.1016/j.scitotenv.2021.151768
Sun, Y., Duan, C., Cao, N., Ding, C., Huang, Y., and Wang, J. (2022b). Biodegradable and conventional microplastics exhibit distinct microbiome, functionality, and metabolome changes in soil. J. Hazard. Mater. 424, 127282. doi:10.1016/j.jhazmat.2021.127282
Thompson, R. C., Olsen, Y., Mitchell, R. P., Davis, A., Rowland, S. J., John, A. W., et al. (2004). Lost at sea: Where is all the plastic? Science 304 (5672), 838. doi:10.1126/science.1094559
Tirkey, A., and Upadhyay, L. S. B. (2021). Microplastics: An overview on separation, identification and characterization of microplastics. Mar. Pollut. Bull. 170, 112604. doi:10.1016/j.marpolbul.2021.112604
Wang, X., Xing, Y., Lv, M., Zhang, T., Ya, H., and Jiang, B. (2022). Recent advances on the effects of microplastics on elements cycling in the environment. Sci. Total Environ. 849, 157884. doi:10.1016/j.scitotenv.2022.157884
Wang, Y., Ding, S., Shi, L., Gong, M., Xu, S., and Zhang, C. (2017). Simultaneous measurements of cations and anions using diffusive gradients in thin films with a ZrO-Chelex mixed binding layer. Anal. Chim. acta 972, 1–11. doi:10.1016/j.aca.2017.04.007
Wang, Y., Wan, G., Yin, C., and Huang, R. (2002). Distribution of total, exchangeable and fixed nitrogen in the sediments of two lakes in Guizhou Province. J. Lake Sci. 14 (4), 289–309. doi:10.18307/2002.0402
Wazne, M., Mermillod-Blondin, F., Vallier, M., Hervant, F., Dumet, A., Nel, H. A., et al. (2023). Microplastics in freshwater sediments impact the role of a main bioturbator in ecosystem functioning. Environ. Sci. Technol. 57 (8), 3042–3052. doi:10.1021/acs.est.2c05662
Xu, D., Wu, W., Ding, S., Sun, Q., and Zhang, C. (2012). A high-resolution dialysis technique for rapid determination of dissolved reactive phosphate and ferrous iron in pore water of sediments. Sci. Total Environ. 421, 245–252. doi:10.1016/j.scitotenv.2012.01.062
Yan, P., Zhang, S., Wang, J., Wang, W., Xu, B., Hao, X., et al. (2022). Field management changes the distribution of mesoplastic and macroplastic in Mollisols of Northeast China. Chemosphere 308, 136282. doi:10.1016/j.chemosphere.2022.136282
Yang, Y., and He, W. Q. (2021). Status and progress of soil microplastic pollution in farmland. J. Environ. Eng. 39 (5), 5022. doi:10.13205/j.hjgc.202105022
Yin, M., Yan, B., Wang, H., Wu, Y., Wang, X., Wang, J., et al. (2023). Effects of microplastics on nitrogen and phosphorus cycles and microbial communities in sediments. Environ. Pollut. 318, 120852. doi:10.1016/j.envpol.2022.120852
Yu, H., Liu, M., Gang, D., Peng, J., Hu, C., and Qu, J. (2022). Polyethylene microplastics interfere with the nutrient cycle in water-plant-sediment systems. Water Res. 214, 118191. doi:10.1016/j.watres.2022.118191
Zhang, C., Ding, S., Xu, D., Tang, Y., and Wong, M. H. (2014). Bioavailability assessment of phosphorus and metals in soils and sediments: A review of diffusive gradients in thin films (DGT). Environ. Monit. Assess. 186, 7367–7378. doi:10.1007/s10661-014-3933-0
Keywords: soil-water interface, diffusive gradients in thin-films, exchange fluxes, microplastics, source-sink characteristic
Citation: Jiang Y, Zhu X, Zhang S, Yang J, Lu Y and Wang Y (2023) Impact of microplastics on the in situ, high-resolution of key nutrient dynamics at the soil-water interface in rice fields. Front. Environ. Sci. 11:1239282. doi: 10.3389/fenvs.2023.1239282
Received: 16 June 2023; Accepted: 29 August 2023;
Published: 18 September 2023.
Edited by:
Alaa El-Din Hamid Sayed, Assiut University, EgyptReviewed by:
Jie Wang, China Agricultural University, ChinaLei Ren, Guangdong Ocean University, China
Copyright © 2023 Jiang, Zhu, Zhang, Yang, Lu and Wang. This is an open-access article distributed under the terms of the Creative Commons Attribution License (CC BY). The use, distribution or reproduction in other forums is permitted, provided the original author(s) and the copyright owner(s) are credited and that the original publication in this journal is cited, in accordance with accepted academic practice. No use, distribution or reproduction is permitted which does not comply with these terms.
*Correspondence: Yongzheng Lu, luyz@iga.ac.cn