- 1Civil Engineering Department, College of Engineering, Najran University, Najran, Saudi Arabia
- 2Geology Department, Faculty of Science, Al-Azhar University, Cairo, Egypt
- 3Institute of Physics and Technology, Ural Federal University, Yekaterinburg, Russia
- 4Physics Department, Faculty of Science, Al-Azhar University, Assiut, Egypt
- 5Geology Department, Nuclear Materials Authority, Cairo, Egypt
- 6INPOLDE Research Center, Department of Chemistry, Physics and Environment, Faculty of Sciences and Environment, Dunarea de Jos University of Galati, Galati, Romania
The current research study is aimed at studying the impact of sodium chloride on the performance of semi-arid soils in the Najran area of Saudi Arabia. Experimental work has been undertaken to investigate how adding salt to the semi-arid soil collected in the Najran area affects the boundaries of Atterberg, compaction characteristics, California bearing ratio, and shear strength. All testing was conducted on soil samples from different zones of the Najran area at varying depths of 1.5, 3, and 4.5 m along the soil profiles. The soil samples were analyzed individually and then compared with the same soil samples mixed with NaCl at different percentages of 5, 10, and 20% by weight of the dry soil. Using advanced techniques, such as the scanning electron microscope, energy dispersive x-ray analysis, and X-ray diffraction analysis, the stabilization process was examined. The findings revealed that NaCl significantly impacts the geotechnical characteristics of semi-arid soils. The maximum dry density increased from 1.995, 1.93, and 1.96 to 2.02, 1.99, and 2.03 g/cm3, and the optimal water content decreased from 9.47, 13.7, and 11.29 to 7.01, 9.58%, and 8.09% with 20% NaCl added at various depths, respectively. Shear resistance parameters were improved by adding 20% NaCl, where the soil cohesion increased from 0.1333, 0.0872, and 0.0533 to 0.1843, 0.1034, and 0.0372 kg/cm2, and the angle of internal friction increased from 24°, 25.5°, and 29° to 27.8°, 30°, and 33°, respectively. The liquid and plastic limits and, in turn, the plasticity index reduced as the added percentage of NaCl increased. Furthermore, the California bearing ratio percentages significantly increased and reached more than 50%. As a result, it is established that NaCl is an excellent stabilizer, especially at 20% concentration, and might be used as a sub-base substance in highway construction.
1 Introduction
Salt-affected soils are present on all continents and under virtually all weather conditions. However, their range is comparatively more widespread in the arid and semi-arid areas than in the tropical regions. For economic and environmental reasons, local soils are expected to be used for geotechnical and geo-environmental projects such as subgrades, dikes, dams, and municipal trash barriers. The main point from an engineering perspective is to enhance the mechanical characteristics of the soil in terms of strength, durability, and cost to be used as building materials. Mechanochemical substances may be used to stabilize poor soils, incorporating agents that assist in improving the mechanical characteristics of stabilized soils. Stabilizers which are to be used must fulfill visible behavior, reliability, lower cost, and can be quickly applied (Al-Homidy et al., 2017). The Najran area is an important region in Saudi Arabia, and civilization is currently increasing rapidly due to the flourishing diversity of economic resources (Najran granite, industrial aggregates) and its significant position on the Kingdom of Saudi Arabia’s southern border. Over the last three decades, the area of the town has increased to cover much of the low-lying areas surrounding the old city of Najran. The foundation bed is typically made of clay and sandy silt. In order to obviate future threats in building the new urban governorate around Najran City, which was built on clayey soil, an evaluation of the geotechnical aspects of this bed was necessary (Abd El Aal, Rouaiguia, 2020). The foundation’s shape and depth required will be influenced by the hazards associated with the different ground conditions described (El-Shafie, 2010). Soils usually contain little to no salt in humid areas, and soil water is comparatively poor in dissolved salt-related ions. This is because abundant rainfall leaches any salt present in the soil and transfers salt-related ions downward past the root zone (and, in some places, abundant snowmelt). There is a relatively higher level of chemical reactions and weathering of earth materials, such as soils, minerals, and rocks, resulting in the accumulation of different natural salts.
As for strength characteristics of saline soil, the influence of salt content is different for different salts, soils, or sample preparation methods (Xu et al., 2014; Tabiatnejad et al., 2016; Xu et al., 2017; Abu Zeid and Abd El-Aal, 2017; Yin et al., 2019; Wang et al., 2019b; 2020; Xu et al., 2019a; b). Zhang et al. (2013) performed undrained ring shear tests and concluded that changing the concentration of NaCl in pore water strongly influences the shearing properties of saturated loess; the peak and residual strengths first increase with increasing NaCl concentration and then decrease after reaching a certain value. They also believe that salinization has a reversible influence. Garakani et al. (2018) suggested a constitutive empirical pattern for predicting the stiffness, strength, and yield stress under different salt conditions. Han et al. (2018) discovered a 2.0% critical salt content in the salt crystallization effect on the internal friction angle and therefore developed an empirical mathematical equation describing the influence of the freeze-thaw cycle and salt content on the soil strength.
The speed at which moisture is found in the atmosphere via soil evaporation and plant-process transpiration, collectively called evapotranspiration, is relatively high. In arid and semi-arid zones, the degree of chemical weathering is greater than those for moist areas due to higher air and soil temperatures. In addition, evapotranspiration rates surpass those of humid regions because the solar radiation in the former is higher, and the air is drier there. On SEM pictures, Zhang et al. (2013) and Ying et al. (2020) noticed some salt bonding amongst loess clay particles, which resulted in particle aggregation and increased shear strength. X-ray computed tomography (X-ray CT) was used by Sarkar and Siddiqua (2016) to investigate bentonite-sand products made with salt and distilled water solutions, highlighting the influence of salt on the distribution of pore size features of compacted samples. To avoid the potential hazards connected with saline soils, it is vital to better understand how soil behaves under various environmental situations (Fang and Chaney, 2017). To assess the effect of salinity on clayey soil deformation, it is necessary to combine geotechnical, geochemical, and geo-environmental knowledge (Barbour and Yang, 1993).
Traffic is quickly compacted by snow-fall on roads to create a hard crust. Sodium chloride (salt) is also added to the road by means of an anticompaction measure to prevent layer crust formation. On the planet, many arid areas are close to oceans, rivers, or freshwater saline lakes. Earlier, numerous studies on enhancing the characteristics of susceptible soils were carried out using several other stabilizers such as Ca2CO3, gypsum, cement and cement kiln mud, cement kiln dust CKD, ash flay oil, lime and marble powder, and rice husk powder as done in works by Miller and Azad (2000), Muntohar and Hantoro (2000), López and Castaňo (2001), Al-Malak et al. (2016), Al-Amoudi et al. (2017), Al-Homidy et al. (2017), Rouaiguia and Abd El Aal (2020), Sakr et al. (2021; 2022a), etc.
Several researchers have examined the impact of current salts on the physico-mechanical properties of the soil instead of semi-arid soils such as black cotton (Dubey and Jain, 2015; Guttikonda and Abhilash, 2018), expansive soils (Durotoye et al., 2016), clay soils (Afrin, 2017; Peddaiah and Suresh, 2017), and silty clay soils (Abood et al., 2007; Zi et al., 2021). Both previous studies showed that the addition of different salt additive percentages increased the MDD and decreased the OMC. Furthermore, when the salt concentration grew, Atterberg limits and plasticity index (PI) fell.
Abdullah and Al-Abdul Wahhab (2015), Abdullah and Al-Abdul Wahhab (2018), and Sakr et al. (2022b) have investigated the stabilization of marginal soils (dune sand, sabkha, and marl) covering most areas of Saudi Arabia’s eastern and western parts. Using various stabilizers (sulfur foamed, emulsified sulfur asphalt, and palm leaf powder), they studied the treatment of these soils and concluded that the stabilized soils could be used as the base layer in road construction.
According to the papers previously cited, the effect of salinity on compaction behavior, stiffness, or microstructure appears to have been studied in many soils. This study can be considered the first work to examine all of these varied soil qualities and salinities at different depths. Atterberg limits, shear strength, standard proctor (SP) compaction, and California bearing ratio (CBR) tests were initially performed on soil samples with various salinities (0, 5, 10, and 20%) at different depths in this investigation. The selection of salts’ dosages was based on what was found in the literature review, where most researchers used salts in the percentage of 2, 4, 6, 8, or 10%, and some of them reached 20 or 25%. Furthermore, we decided not to go beyond 20% of salt to avoid high concentrations of salts, which could have bad effects on buried infrastructure.
This study focuses on investigating the effects of salt NaCl on the index and engineering aspects of soils in the arid environment in the Najran area, which has semi-arid soils that have not been researched before.
2 Site investigation and geological setting
Najran City is situated in the south-western part of the Kingdom of Saudi Arabia on the Yemeni border. It occurs between latitudes 17° 41′ and 17° 29′ north and 44° 16′ and 44° 55′ east (Figure 1). The area is estimated at approximately 360,000 km2, with the town rising from 1,173 m to 1,529 m above sea level, and the town is surrounded in the north, south, and west by a cluster of high mountains. Najran is one of the most developed cities, with a population rising from 47,500 in 1974 to 505,562 in 2010. The city comprises a variety of land use activities, including urban, agricultural, and road networks. There is the Najran Valley Dam, considered one of the largest dams constructed in the Kingdom, with a storage capacity of approximately 85 million cubic meters.
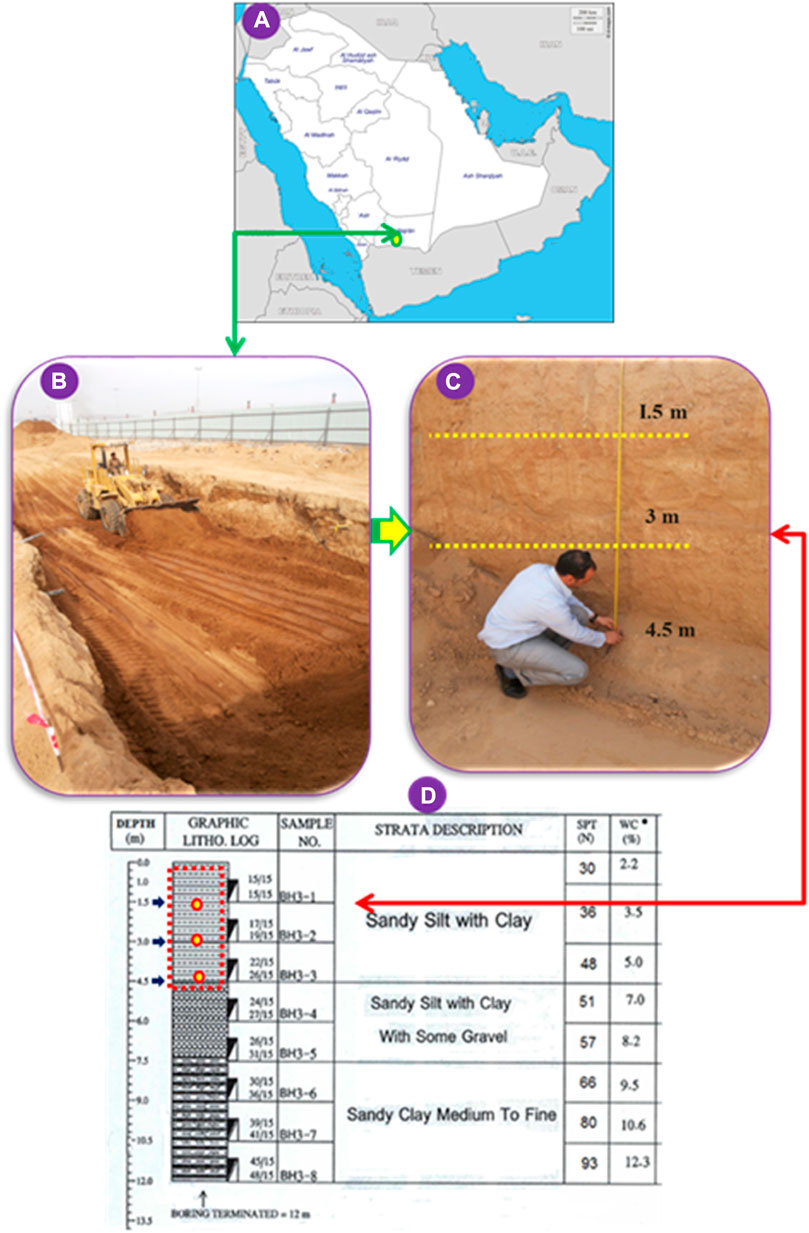
FIGURE 1. (A) Location map for soil sample area examined, (B) and (C) view of drilling and sampling at different depths, and (D) Najran soil’s typical soil profile (borehole log) (see ditched area).
Geomorphologically, there are three distinct geomorphological units, including 1) the high mountain zone in the northwest, 2) areas of floodplains with alluvial deposits along the Najran Valley, and 3) the sand dunes area to the southeast. The stratigraphic sequence of the Najran region (Figure 2) begins from the Precambrian era to the Quaternary, where the Najran region lies in the southern part of the Arabian Shield. The deposits of the Quaternary consist of surface materials, including the placers of Wadi Najran and the sand dunes between Wadi Najran and the Empty Quarter. Precambrian rocks (basement rocks) are a complex series of interlocking and metamorphic igneous and sedimentary assemblages. The composition of igneous rocks varies from gabbro to syenite, while volcanic rocks vary from andesite to rhyolite. These volcanic rocks are closely related to the sediment rock layer, which comprises sandstone, conglomerate, greywacke, shale, and limestone. There is an unconformity between the basement rocks and the sedimentary layer above them, composed of unconsolidated alluvial and aeolian sands and Wajid sandstone, which belong to the Cambro-Ordovician event (Sable, 1985; Al-Shanti, 1993).
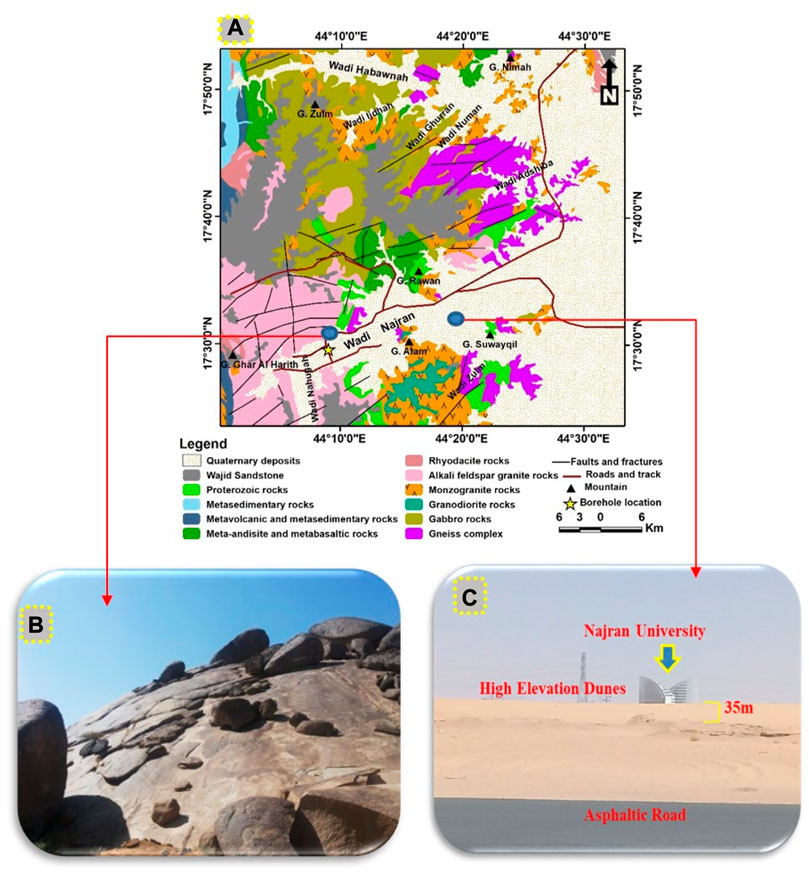
FIGURE 2. (A) Geological map of Najran City, Kingdom of Saudi Arabia , (B) alkali feldspar granites , and (C) quaternary deposits in Wadi Najran.
Najran region is situated in the Great African Rift Valley zone and has simple and complex fault areas with different directions. Faults are oriented northwest to southeast or northeast to southwest (Ahmed et al., 2015).
3 Procedures and methods
3.1 Material collection and characterization
3.1.1 Soils
Soil samples were obtained from the Najran region, Kingdom of Saudi Arabia (Figure 1A) for a project proposal for the completion of Najran interchange, Najran Airport (Bridge No. 2). The field research was conducted using mechanical rotary drilling equipment, and the field work was conducted following ASTM standards. Road holes are ideal ways to analyze the layers within a soil profile or horizons. Figure 1 shows a road cut demonstrating the number of layers that may exist in the soil of the Najran area. The soil section demonstrated in Figures 1B, C presumed that the details provided by most soil profiles were almost identical. At a depth of 12 m, the borehole ended. Figure 1D provides a standard profile (drill log) of the Najran soil. It is noted that the number of blows (N) of the SPT and the moisture content increased with depth, and at the time of the examination, the groundwater table was not found over the entire 12 m depth. Soil samples were transported to the laboratory after processing, and a sieve analysis in accordance with AASHTO T87 (ASTM D 421) was conducted. The specific gravity, density, and water content of Najran soil were determined as per ASTM D- 854 (2014), ASTM D-2937 (2010), and ASTM D-2216 (2010), respectively. Porosity, void ratio, and degree of saturation were calculated as per these equations:
where e is the void ratio, w is the water content, Gs is the specific gravity, γd is the dry unit weight, γ is the bulk unit weight, γw is the unit weight of water, and S is the degree of saturation.
3.1.2 Sodium chloride “NaCl”
This study shows that NaCl can be an efficient alternative to lime as it dissolves rapidly in water and provides sufficient calcium ions for ion exchange reactions with silty sand soil. The chemical (NaCl) function is to form a cluster of fine particulate matter and bind it together. Furthermore, the configuration of sodium chloride is highly stable due to the type of electrovalent or ionic bond it possesses. NaCl may react with soils with a pH of less than or higher than 7. NaCl has properties comparable to those of CaCl. Sodium chloride was applied to each tested soil in increments of 5, 10, and 20% by weight. All soil was carefully mixed with distilled water and left to heal and obtain a homogeneous paste for 2 hours. A significant improvement in CBR, unconfined compressive strength (UCS), and indirect tensile strength of salt-stabilized material has been documented by Singh and Das (1999). Long-term road subgrade is the primary application of NaCl (Singh and Das, 1999).
3.2 Analysis
The global test program took place in two stages. In the first stage, the index and technical properties of the soils were investigated by laboratory experiments. In the second stage, the soils were blended with 5, 10, and 20% NaCl per soil dry weight.
3.2.1 Mineralogical analysis
XRD technique using a Philips PW 1840 diffractometer was applied to analyze 10 powder samples prepared from the collected blocks. Patterns were run with Ni-filtered Cu Ka radiation (k = 1.54056 A°) at 40 kV and 10 mA, with scanning limited from 2Ѳ = 1° to 80°. The SEM supported with energy-dispersive analytical X-ray (EDAX) was applied in the same way Abd El-Aal et al. (2020) described to characterize the tufas under the study’s microstructural features and elemental makeup.
3.2.2 Atterberg limit tests
The soil samples were sieved via sieve No. 40. To establish the liquid limit (LL), a cone penetrometer system (according to BS: 1377 and IS: 2720) was used with a normal mold size with a diameter and a depth of 55 mm and 40 mm, respectively. It allows the LL to be calculated easily and rapidly. Compared to the Casagrande approach, the cone penetrometer process has numerous advantages. It is less reliant on the tool’s setting and is suitable for use in a wide variety of soils. The plastic limit (PL) of soil samples was determined by measuring the moisture content of rolling thread specimens with a uniform diameter of around 3 mm.
3.2.3 Standard proctor test
The SP test was conducted per AASHTO T99 (ASTM D 698) on each soil sample passing through No. 4 sieve, compacting the soil with a 5.5-lb hammer dropping into a filled mold at a distance of one foot to produce compaction energy of 12,400 ft-lbf/ft3. The soil samples are compacted as three layers in the mold, and 25 drops of the hammer are applied to each layer. Water was added to each soil sample to get it to a predetermined percentage of moisture content.
3.2.4 California bearing ratio test
CBR is considered as an important test for the performance evaluation of the materials proposed to be used in pavement structure layers. The test could be conducted either dry or soaked. The soaked test is more realistic and simulates the real field conditions in its worst case (i.e., flood). Therefore, a soaked CBR test was performed in soil specimens prepared with the OMC and at a range of dry density by changing the compaction energy applied to each layer in the compaction CBR mold. This was conducted to produce curves of different dry densities compared to the corresponding CBR ratios. The soaked CBR test was performed in compliance with AASHTO T 193 and (ASTM D698-70) specifications.
3.2.5 Shear strength test
It is well known that soils fail under shearing when subjected to heavy loading. Thus, to assess the shear strength of stabilized soils, a direct shear box test was conducted on the natural semi-arid soil specimens and on the treated soil samples of salt (NaCl) collected from different depths (1.5, 3, and 4.5 m). The test was conducted according to ASTM D3080-98 specification on the treated soil mix that gave higher MDD in the compaction test.
4 Results and discussion
4.1 Physical and mechanical characteristics of Najran soil before being treated with NaCl
The grain size distribution curves for the soils are plotted in Figure 3. The distribution of particle size is normally composed of 87.3% sand, 10.1% silt, and 2.6% of clay fraction at 1.5 m depth; 86.6% sand, 10.6% silt, and 2.8% of clay fraction at 3 m depth; and 86% sand, 10.3% silt, and 3.7% of clay fraction at 4.5 m depth (Table 1). The tested soil is known as silty sands (SM) by the use of the unified soil classification system (USCS).
The average values of physical characteristics of the soil at depths 1.5, 3, and 4.5 m. The highest value of moisture content, specific gravity, bulk density, dry density, void ratio, porosity, and degree of saturation are 5%, 2.68 g/cm3, 1.74 g/cm3, 1.66 g/cm3, 0.73%, 0.41%, and 18.35%, respectively, are listed in Table 2. In contrast, Table 1 presents their description and classification according to USCS and AASHTO specifications. The moisture content and the saturation degree at the site ranged from 2.2% to 5% and from 7.20% to 18.35% for a depth ranging from 0 to 4.5 m, respectively. It should be remembered that even with soil depth, the degree of saturated soil rises.
Table 3 shows the chemical composition of the soils investigated, demonstrating that Najran soils comprise 0.08–0.089% sulfur trioxide, 0.23–0.266% soluble mineral salt, and 0.062–0.08% sodium chloride. The pH range of the soil in Najran is between 7.3 and 7.7, indicating that they may be considered alkaline. If greater than 1% of the dissolved salts are soluble, some form of intervention is required, like blending the base soil with sufficient extracted soil and soaking and compacting the soil if the dissolved salt concentration is higher than 6%. Notably, the proportion from both SO3 and NaCl in Najran soils is lower.
Table 4 provides the test results for the Atterberg limits. The LL values range from 20.7% to 22.85% and PL, from 14.04% to 17.80%. It is noted that the PI is in the range between 4.18% and 6.66%, suggesting low plasticity of the soil. The liquidity index for Najran soil is negative (less than zero), which means semi-solid or solid according to Whitlow (1983), and calculated by this formula: LI = Wc – PL/LL – PL (3).
This is also confirmed by the high consistency index (CI) and SPT-N values. Thus, when sheared, the soil might be treated as a brittle fracture. Consistency index values of studied soils lie in the range of 2.59%–4.06%, which means these soils are incredibly stiff, and calculated by the following formula: CI =(LL-Wc)/LL-PL (4).
The Najran soil quality index is higher than one, indicating the soil is semi-solid and rigid.
4.2 Physical and mechanical characteristics of Najran soil after treated with NaCl
4.2.1 Effect of NaCl on Atterberg limits
The results of Atterberg experiments showed that salt addition influences soil consistency. As seen in Figure 4, an increase in salt addition reduces the LL and PL and contributes to a reduction in the PI, where LL decreased from 22.85%, 20.7%, and 21.98% to 12.19%, 16.20%, and 17.04%; PL decreased from 17.72%, 14.04%, and 17.80% to 11.76%, 10.95%, and 14.81%; and PI, from 5.13%, 6.66%, and 4.18% to 0.43%, 5.25%, and 2.23% by adding NaCl with 5, 10%, and 20% at different depths, respectively (Table 5). They ascribe this behavior to the fact that the LL and the PL control through the shear resistance and the inter-particle level and the thick scattered double layer. The shearing resistance in the case of PL is found to be higher than that in the case of LL, and the diffuse double-layer thickness is much lower.
4.2.2 Effect of NaCl on compaction characteristics
It is worth noticing through the SP compaction tests that changes occurred in results when salt was introduced to the soil. Salt addition resulted in reduced OMC and increased MDD, as shown in Table 6 and Figures 5A–D, 6–8. The reduction in the OMC with the increase in salt content in water added to the soil was ascribed to a higher face-to-face contact between the flocculated soil particles; the lower was the amount of water required for lubrication. These new features of salt-stabilized soils enhance their strength and ability to bear high loads; therefore, they can be used in road structure construction as sub-base layers. The salinity of soil water causes fine particles to stack with large particles in a process known as flocculation. This phenomenon interprets the reasons for the increasing dry density of salt-stabilized soils. Comparison between proctor compaction curves of salt-less soil and saline soils suggested that the salt had more significant Effect on the dry side than on the wet side.
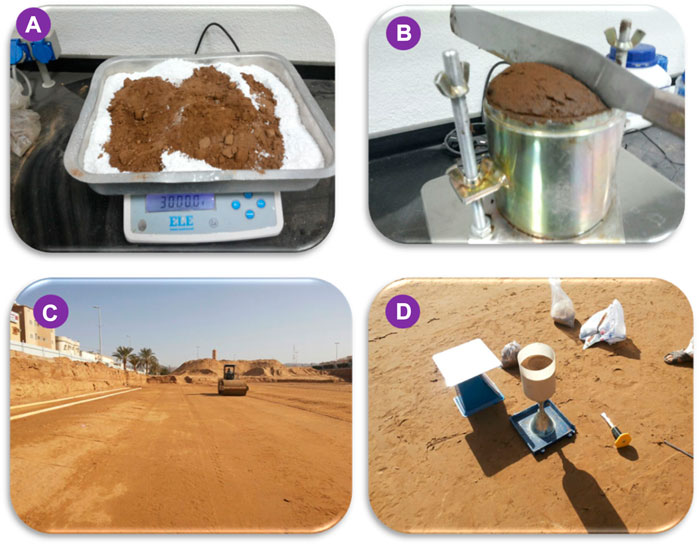
FIGURE 5. (A) and (B) Proctor compaction tests of alluvial soils with chloride in the laboratory. (C) and (D) Field density test.
4.2.3 Effect of NaCl on CBR
For materials to be used in pavement structures (i.e., sub-base layer or subgrade), it is necessary to know their performance in advance. Thus, several CBR tests were conducted for both soil specimens collected from different depths (1.5, 3, and 4.5 m) treated with 20% of NaCl and the untreated specimens (i.e., natural soil). Tests were carried out on soils stabilized with 20% NaCl since they demonstrated a higher MDD in the compaction test. Specimens were prepared with varying densities by applying different compaction energies and then subjected to the soaked CBR test. Figure 9 illustrates the relationship of dry density to CBR. It is clear from the figure that there is a significant effect of the addition of 20% NaCl to the soil in different depths in which the CBR percentages increased significantly compared to the CBR percentages of untreated soil specimens. The ratio of CBR increases sometimes reaches more than 50%. The main reason for these changes has been attributed to an increase of attractive force between soil particles, the establishment of bonding between them, and formation of salt crystals in the pores of soil and the role of the cement. The benefit of these curves is to predict the CBR value corresponding to a specific dry density of stabilized soil according to the purpose of use in road layers with light-, medium-, or high-traffic loading.
4.2.4 Effect of NaCl on shear strength
The results of direct shear box tests on the natural soil samples and soil samples stabilized with 20% NaCl are presented in Figures 10, 11, respectively. For the purpose of comparison, the results of shear strength parameters in both figures are summarized in Table 7. The results presented indicated that the addition of 20% NaCl to the soils at different depths increased the shear strength parameters (C and ϕ), which has increased the shear strength of the treated soils. These results are consistent with the trend obtained in compaction and CBR test results. The increase in shear strength parameters for stabilized soils is due to increased frictional forces between soil particles due to the salts’ adhesion on the soil particles’ surfaces forming rough surfaces. Additionally, the increase in UCS with the increase in salt content in water added to the soil was also attributed to the increase in dry unit weight.
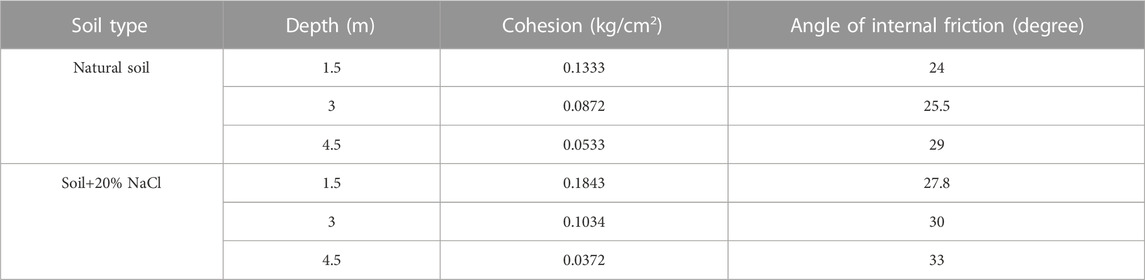
TABLE 7. Shear strength parameters for natural soils and treated soils with 20% NaCl at different depths.
4.3 The interaction between saline soil and infrastructure
The characteristics of soils used as backfill material or in pavement layers around buried pipelines can have an impact on the service life of the structure. Problems related to the backfill material’s quality may consist of pipeline and further infrastructure corrosion. Therefore, the study emphasizes the negative influence of soluble salt accumulation on soil structure stability, starting with utilized salts dosages no higher than 20% to avoid the high concentration of salts that could have harmful effects on infrastructures. Engineers will be able to adequately address design flaws and offer proper mitigation measures and management procedures for the impacted infrastructure by isolating the infrastructures with plastic sheeting or the application of external coating or tapes. Using materials rather than metals in the infrastructures could help avoid this problem.
4.4 Mechanism of stabilization
Since soil samples taken at depth 4.5 m showed higher dry density when compacted with the addition of various percentages of NaCl (i.e., 5, 10%, and 20%), SEM was performed for some representative compacted samples to understand the mechanism of stabilization.
Figure 12 demonstrates the SEM analysis of stabilized soil specimens with 5%, 10%, and 20% of NaCl. The samples were taken from specimens compacted at the OMC corresponding to high MDD, and images are displayed in 400×. After the addition of 5% of NaCl to the normal soil specimens (Figure 12A), salt penetrated voids between the soil grains and a decrease in soil porosity was demonstrated; however, voids between soil particles are still clearly shown. Moreover, the addition of 10% NaCl (Figure 12B) shows a gradual increase in particle densification and flocculation compared to soil structure with 5% NaCl (Figure 12A). Lastly, the addition of 20% NaCl (Figure 12C) resulted in a flocculated and highly dense structure (high dry density), which means high strength, and this is clear from the compaction results trend. Furthermore, crystallized salts act as a skeleton and are adsorbed on the soil grains’ surface, increasing its roughness as seen clearly in Figure 12.
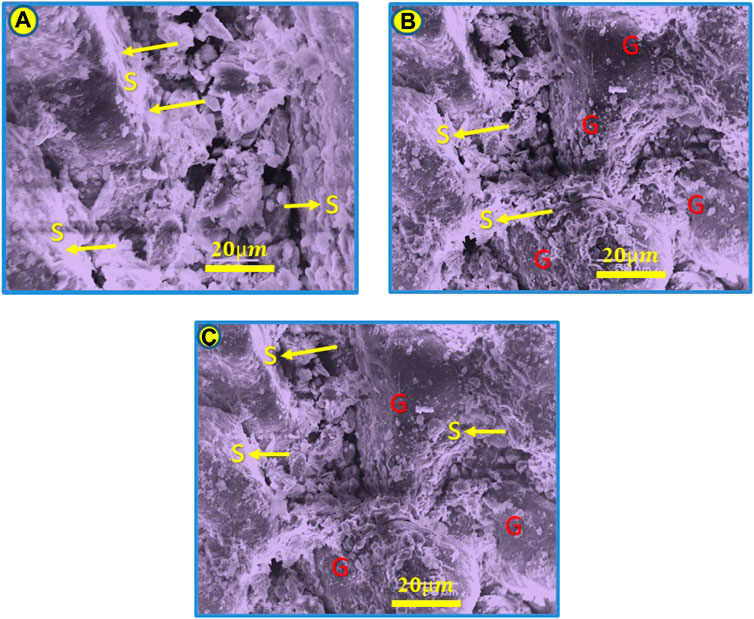
FIGURE 12. SEM images (400x): (A) 5% NaCl, (B) 10% NaCl, and (C) 20% NaCl. (For legend, G = grain of soil, S = salt “NaCl”).
Figure 13 shows SEM of soil treated with 20% NaCl and EDX at spectrum 1. The EDX in Figure 13B shows the existence of O, Na, Mg, Si, Cl, and Ca with about 14.64, 35.17, 2.44, 3.03, 44.04, and 0.67% by weight, respectively. The high percentage of sodium (Na) and chloride (Cl) is associated with the addition of 20% NaCl to standard soil specimens, and it is clearly seen from Figure 12A that the salt crystals are adsorbed on the surface of soil particles.
4.5 Comparing our findings with those of other research studies
With the previous results, the results of this study were compared to those of earlier studies using NaCl to improve soil properties (Figure 14). In this study, NaCl was added to the silty sand soil at rates ranging from 5% to 20%, while in the other articles, NaCl was added at rates varying from 0.5% to 3%. In this document, LL, PL, PI, and OMC of soil have decreased to 22.5%, 16.8%, 46.6%, and 28.3%, respectively, for 20% NaCl, whereas in the other documents, it was up to 8.3%, 7.1%, 10%, and 25% for 2.5% NaCl relative to virgin soil. MDD and CBR increased up to 3.6% and 50%, respectively, for 20% NaCl (Figure 14A), whereas in the other articles, they increased up to 9.02% and 98% for 2.5% NaCl (Figure 14B). A direct shear test may be carried out with various doses of NaCl (Singh et al., 2020). In this study, the shear box was also tested for the effect of NaCl on shear strength coefficients (C and φ). By adding 20% NaCl, soil cohesion increased from 0.1333, 0.0872, and 0.0533 to 0.1843, 0.1034, and 0.0372 kg/cm2, respectively. The soil friction angle increased from 24°, 25.5°, 29° to 27.8°, 30°, and 33°, at 1.5, 3, and 4.5 m depth. The highest reduction percentages of 60.42%, 42.86%, 71.26%, and 28.57% in LL, PL, PI, and OMC, respectively, and maximum percentage increase of 257.67% and 11.38% in CBR and MDD, respectively (Figure 14C) Durotoye et al. (2016). Based on this comparison, we see that the ideal percentage to meet NaCl salt addition targets is 20% in this study and 2.5% in the other study.
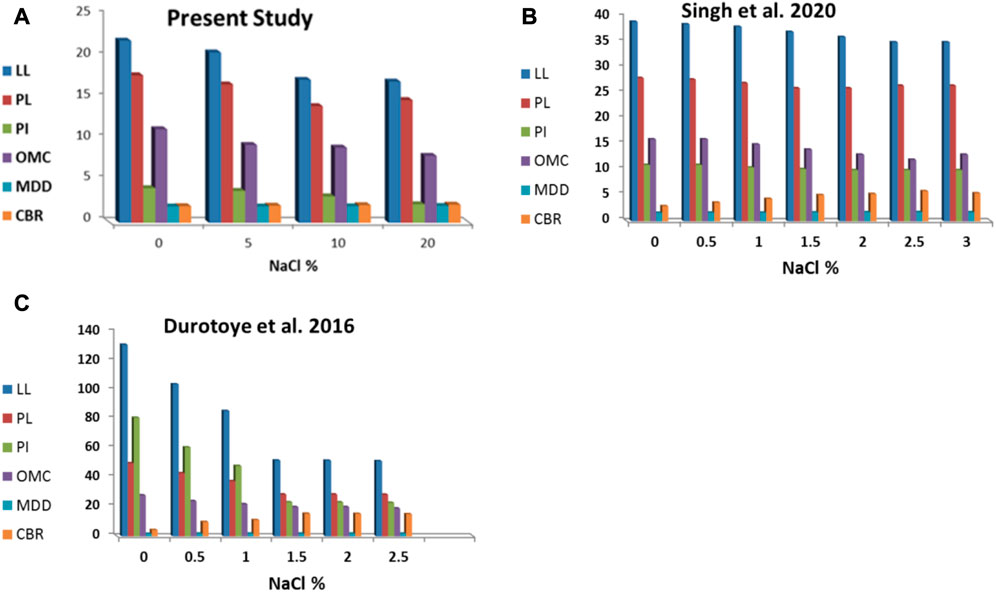
FIGURE 14. Comparison of findings with the existing literature with various percentages of NaCl. (A) Present study, (B) Singh et al. (2020), and (C) Durotoye et al. (2016).
5 Conclusion
In this study, NaCl salt had high effectiveness in enhancing alluvial soil properties. Different percentages of salt (5%–20%) were added to the soil profile from 1.5–4 m depth, where the ideal percentage of salt added to the soil was 20%. The results point to the following conclusions.
With the addition of 20% NaCl, the dry density increased from 1.96 to 2.03 g/cm3, and the OMC decreased from 11.29% to 8.09%.
The LL, PL, and, in turn, PI of soils decreased at the different depths with the increase in added percentage of NaCl.
The addition of NaCl significantly increases the CBR, indicating that soils stabilized by salt could be used in the subgrade or sub-base layer for medium- to high-traffic volume roads. A significant effect of the addition of 20% NaCl to the soil at various depths was noticed in which the CBR percentages increased and sometimes reached more than 50%
Crystallized salts act as a skeleton and are adsorbed on the soil grain surface, increasing its roughness and increasing the soil structure’s strength. For the addition of 20% NaCl at depths of 1.5, 3, and 4.5 m, the soil cohesion increased from 0.1333, 0.0872, and 0.0533 to 0.1843, 0.1034, and 0.0372 kg/cm2, respectively. Similarly, the angle of internal friction increased from 24, 25.5, and 29° to 27.8, 30, and 33°.
From the aforementioned results, the dissolved salt content has a critical value for improving cementation among particles. So, sodium chloride has the potential to be a good stabilizer for semi-arid soils in the Najran region, especially for highway construction.
The stabilization process has proved to be a cost-effective and environmentally effective solution for such major construction projects.
Data availability statement
The raw data supporting the conclusion of this article will be made available by the authors, without undue reservation.
Author contributions
Conceptualization: AEA and AE; data curation: HZ, AO, and GA; formal analysis: HA, MS, and AEA; funding acquisition: AE; investigation, AO and MS; methodology: AEA, HA, and GA; project administration: HA and GA; resources: GA and AO; software: HZ; supervision: HZ; validation: HZ; visualization: AE, AEA, and MS; writing—original draft: AO, HA, and MS; and writing—review and editing: AE, HZ, MS, and AEA. All authors have read and agreed to the published version of the manuscript.
Acknowledgments
The authors are thankful to the Deanship of Scientific Research at Najran University for funding this work under Najran Region Research Program Funding program grant code (NU/NAR/SERC/11/34). The work of author HZ is funding from the Ministry of Science and Higher Education of the Russian Federation (Ural Federal University Program of Development within the Priority-2030 Program). The work of author AE and APC were financed by Dunarea de Jos University of Galati, Romania.
Conflict of interest
The authors declare that the research was conducted in the absence of any commercial or financial relationships that could be construed as a potential conflict of interest.
Publisher’s note
All claims expressed in this article are solely those of the authors and do not necessarily represent those of their affiliated organizations, or those of the publisher, the editors, and the reviewers. Any product that may be evaluated in this article, or claim that may be made by its manufacturer, is not guaranteed or endorsed by the publisher.
References
Abd El Aal, A. K., and Rouaiguia, A. (2020). Determination of the geotechnical parameters of soils behavior for safe future urban development, Najran Area, Saudi Arabia: Implications for Settlements Mitigation. Geotech. Geol. Eng. 38, 695–712. doi:10.1007/s10706-019-01058-x
Abd El-Aal, A. K., Salah, M. K., and Khalifa, M. A. (2020). Acoustic and strength characterization of upper cretaceous dolostones from the bahariya oasis, western desert, Egypt: The impact of porosity and diagenesis. J. Pet. Sci. Eng. 187, 106798. doi:10.1016/j.petrol.2019.106798
Abdullah, G. M. S., and Al-Abdul Wahhab, H. I. (2015). Evaluation of foamed sulfur asphalt stabilized soils for road applications. Constr. Build. Mater 88, 149–158. doi:10.1016/j.conbuildmat.2015.04.013
Abdullah, G. M. S., and Al-Abdul Wahhab, H. I. (2018). Stabilisation of soils with emulsified sulphur asphalt for road applications. Road Mater. Pavement Des. 20 (5), 1228–1242. doi:10.1080/14680629.2018.1436465
Abood, T. T., Kasa, A. B., and Chik, Z. B. (2007). Stabilization of silty clay soil using chloride Compounds. J. Eng. Sci. Technol. 2 (1), 102–110.
Abu Zeid, M. M., and Abd El-Aal, A. (2017). Effect of salinity of groundwater on the geotechnical properties of some Egyptian clay. Egypt. J. Petro 26, 643–648. doi:10.1016/j.ejpe.2016.09.003
Afrin, H. (2017). Stabilization of clayey soils using chloride components. Amer J. Civ. Eng. 5 (6), 365–370. doi:10.11648/j.ajce.20170506.18
Ahmed, A. M., Al Alwadie, A., and Ibrahin, A. M. (2015). Some aspects of groundwater quality in najran town, kigdom of Saudi Arabia. Open Access Libr. J. 2, 1–6. doi:10.4236/oalib.1101833
Al-Amoudi, O. S. B., Al-Homidy, A. A., Maslehuddin, M., and Saleh, T. A. (2017). Method and mechanisms of soil stabilization using electric arc furnace dust. Sci. Rep. 7 (1), 46676. doi:10.1038/srep46676
Al-Homidy, A. A., Dahim, M. H., and Abd El Aal, A. K. (2017). Improvement of geotechnical properties of sabkha soil utilizing cement kiln dust. J. Rock Mech. Geotech. Eng. 9 (4), 749–760. doi:10.1016/j.jrmge.2016.11.012
Al-Malack, M. H., Abdullah, G. M., Al-Amoudi, O. S. B., and Bukhari, A. A. (2016). Stabilization of indigenous Saudi Arabian soils using fuel oil flyash. J. King Saud. Univ. Eng. Sci. 33 (2), 165–173. doi:10.1016/j.jksues.2014.04.005
Astm D- 854, (2014) Standard test methods for specific gravity of soil solids by water pycnometer. West Conshohocken, American Society for Testing Materials; West Conshohocken, USA.
Astm D-2216 (2010), .2010 Standard test methods for laboratory determination of water (moisture) content of soil and rock by mass. West Conshohocken, USA: American Society for Testing Materials.
Astm D-2937 (2010), . Standard test method for density of soil in place by the drive-cylinder method West Conshohocken, USA: American Society for Testing Materials. 2010.
Barbour, S. L., and Yang, N. (1993). A review of the influence of clay–brine interactions on the geotechnical properties of Ca-montmorillonitic clayey soils from Western Canada. Can. Geotech. J. 30 (6), 920–934. doi:10.1139/t93-090
Dubey, P., and Jain, R. (2015). Effect of common salt (NaCl) on index properties of black cotton soil. IJIRST-Int J. Innov. Res. Sci. Technol. 2 (2), 75–79.
Durotoye, T. O., Akinmusuru, J. O., Ogbiye, A. S., and Bamigboye, G. O. (2016). Effect of common salt on the engineering properties of expansive soil. Int. J. Eng. Technol. 6 (7), 233–241.
El-Shafie, M. (2010). Sustainability versus mega urban development projects. Int. J. Civ. Environ. Eng. IJCEE-IJENS 10 (4), 1–7.
Fang, H. Y., and Chaney, R. C. (2017) Introduction to environmental geotechnology. 2nd ed., Boca Raton, Florida, FL, USA.
Garakani, A. A., Haeri, S. M., Cherati, D. Y., Givi, F. A., Tadi, M. K., Hashemi, A. H., et al. (2018). Effect of road salts on the hydromechanical behavior of unsaturated collapsible soils. Transp. Geotech. 17, 77–90. doi:10.1016/j.trgeo.2018.09.005
Guttikonda, R., and Abhilash, N. (2018). Stabilization of black cotton soil using sodium chloride. Int J Adv. Res, Ideas Innov Techno 4 (1).
Han, Y., Wang, Q., Kong, Y. Y., Cheng, S. K., Wang, J. Q., Zhang, X. D., et al. (2018). Experiments on the initial freezing point of dispersive saline soil. Catena 171, 681–690. doi:10.1016/j.catena.2018.07.046
Lopez-lara, T., and Castano, V. M. (2001). Time dependency of CaO treated expansive soils. Electron. J. Geotech. Eng. 7.
Miller, G. A., and Azad, S. (2000). Influence of soil type on stabilization with cement kiln dust. Constr. Build. Mater 14 (2), 89–97. doi:10.1016/S0950-0618(00)00007-6
Muntohar, A. S., and Hantoro, G. (2000). Influence of rice husk ash and lime on engineering properties of a clayey subgrade. Electron. J. Geotechn Eng. 5, 1–13.
Peddaiah, S., and Suresh, K. (2017). Experimental study on Effect of gypsum and NaCl in improvement of engineering properties of clayey soil. Int. J. Eng. Technol. (IJET) 9 (4), 2771–2778. doi:10.21817/ijet/2017/v9i4/170904409
Rouaiguia, A., and El Aal, A. K. A. (2020). Enhancement of the geotechnical properties of soils using marble and lime powders, Guelma City, Algeria. Geotech. Geol. Eng. 38, 5649–5665. doi:10.1007/s10706-020-01368-5
Sable, E. G. (1985). Explanation notes on the geologic map of the Najran quadrangle Sheet 17G. Kingdom ofSaudi Arabi.
Sakr, M. A. H., Omar, A. E., Ene, A., and Hanfi, M. Y. (2022a). Effect of various proportions of rice husk powder on swelling soil from New Cairo City, Egypt. Appl. Sci. 12, 1616. doi:10.3390/app12031616
Sakr, M. A. H., Omar, A. E., Ene, A., Hanfi, M. Y., and Shafaey, O. E. (2022b). Geospatial technology utilization for geotechnical hazard evaluation of sustainable urban development of Buraydah City, Kingdom of Saudi Arabia. Arab. J. Geosci. 15, 1320. doi:10.1007/s12517-022-10546-z
Sakr, M. A. H., Omar, A. E., Saad, A. M., and Moayedi, Hossein (2021). Geotechnical parameters modelling and the radiation safety of expansive clayey soil treated with waste marble powder: A case study at west gulf of suez, Egypt. Environ. Earth Sci. 80, 263. doi:10.1007/s12665-021-09573-y
Sarkar, G., and Siddiqua, S. (2016). Effect of fluid chemistry on the microstructure of light backfill: An X-ray CT investigation. Eng. Geol. 202, 153–162. doi:10.1016/j.enggeo.2016.01.012
Singh, G., and Das, B. M. (1999). Soil stabilization with sodium chloride. Transpor Rese Rec. 1673, 46–54. doi:10.3141/1673-07
Singh, H. P., Chana, J. S., Singh, G., Singh, H., and Singh, M. (2020) Improvement in the engineering properties of clayey soil using sodium chloride. Int. Conf. Adv. Res. Innovation (ICARI-2020):10 127–129. doi:10.2139/ssrn.3569450
Tabiatnejad, B., Siddiqua, S., and Siemens, G. (2016). Impact of pore fluid salinity on the mechanical behavior of unsaturated bentonite–sand mixture. Environ. Earth Sci. 75, 1434.
Wang, Q. Z., Qi, J. L., Wang, S. H., Xu, J., and Yang, Y. G. (2020). Effect of freezethaw on freezing point of a saline loess. Cold Reg. Sci. Technol. 170, 102922. doi:10.1016/j.coldregions.2019.102922
Wang, S. H., Ding, J. L., Xu, J., Ren, J. W., and Yang, Y. G. (2019b). Shear strength behavior of coarse-grained saline soils after freeze-thaw. KSCE J. Civ. Eng. 23 (6), 2437–2452. doi:10.1007/s12205-019-0197-9
Xu, J., Li, Y. F., Lan, W., and Wang, S. H. (2019a). Shear strength and damage mechanism of saline intact loess after freeze-thaw cycling. Cold Reg. Sci. Technol. 164, 102779.
Xu, J., Li, Y. F., Wang, S. H., Wang, Q. Z., and Ding, J. L. (2019b). Shear strength and mesoscopic character of undisturbed loess with sodium sulfate after dry-wet cycling. Bull. Eng. Geol. Environ. 79, 1523–1541. doi:10.1007/s10064-019-01646-4
Xu, J., Liu, H., and Zhao, X. K. (2017). Study on the strength and deformation property of frozen silty sand with NaCl under tri-axial compression condition. Cold Reg. Sci. Technol. 137, 7–16. doi:10.1016/j.coldregions.2017.01.008
Xu, Y. F., Xiang, G. S., Jiang, H., Chen, T., and Chu, F. F. (2014). Role of osmotic suction in volume change of clays in salt solution. Appl. Clay Sci. 101, 354–361. doi:10.1016/j.clay.2014.09.006
Ying, Z., Cui, Y. J., Duc, M., Benahmed, N., Bessaies-Bey, H., and Chen, B. (2020). Salinity effect on the liquid limit of soils. Acta Geotech. 16, 1101–1111. doi:10.1007/s11440-020-01092-7
Yin, J., Hu, M. M., Xu, G. Z., Han, W. X., and Miao, Y. H. (2019). Effect of salinity on rheological and strength properties of cement-stabilized clay minerals. Mar. Georesour Geotecnol 38 (3), 611–620. doi:10.1080/1064119X.2019.1608484
Zhang, F. Y., Wang, G. H., Kamai, T., Chen, W. W., Zhang, D. X., and Yang, J. (2013). Undrained shear behavior of loess saturated with different concentrations of sodium chloride solution. Eng. Geol. 155, 69–79. doi:10.1016/j.enggeo.2012.12.018
Keywords: salts, sodium chloride, semi-arid soils, stabilization, geotechnical aspect
Citation: El Aal AA, Abdullah GMS, Zakaly HMH, Awad HA, Omar AE, Sakr MAH and Ene A (2023) Geotechnical aspects of alluvial soils at different depths under sodium chloride action in Najran region, Saudi Arabia: Field supported by laboratory tests. Front. Environ. Sci. 11:1073718. doi: 10.3389/fenvs.2023.1073718
Received: 18 October 2022; Accepted: 28 February 2023;
Published: 20 March 2023.
Edited by:
Keng Yuen Foo, University of Science Malaysia, MalaysiaReviewed by:
Xiaoming Huang, Southeast University, ChinaHossein Moayedi, Southern Illinois University Edwardsville, United States
Copyright © 2023 El Aal, Abdullah, Zakaly, Awad, Omar, Sakr and Ene. This is an open-access article distributed under the terms of the Creative Commons Attribution License (CC BY). The use, distribution or reproduction in other forums is permitted, provided the original author(s) and the copyright owner(s) are credited and that the original publication in this journal is cited, in accordance with accepted academic practice. No use, distribution or reproduction is permitted which does not comply with these terms.
*Correspondence: Hesham M. H. Zakaly, aC5tLnpha2FseUBnbWFpbC5jb20=; Antoaneta Ene, QW50b2FuZXRhLkVuZUB1Z2FsLnJv