- 1Uttar Banga Krishi Viswavidyalaya, Pundibari, Cooch Behar, West Bengal, India
- 2School of Agriculture, Seacom Skills University, Birbhum, West Bengal, India
- 3Texas A&M AgriLife Extension, Killeen, TX, United States
- 4Senior Scientist-Division of Agricultural Physics, ICAR- Indian Agricultural Research Institute, New Delhi, India
- 5Department of Crop, Soil and Environmental Sciences, Auburn University, Auburn, AL, United States
- 6ICAR-National Academy of Agricultural Research Management (NAARM), Rajendranagar, Hyderabad, India
A field experiment (2017–2019) was undertaken to study the short-term effects of tillage [zero tillage (ZT), conventional tillage (CT), and alternate tillage (AT)] and sources of organic and mineral fertilizer N [NS0—control, NS1—recommended doses of fertilizer (160:50:100), NS2—recommended level of fertilizer and crop residue (6 Mg·ha−1), NS3—75% of recommended N as fertilizer (120 kg·ha⁻1) and 25% N (40 kg·ha⁻1) as farm yard manure (FYM), and NS4—75% of recommended N as fertilizer and 25% N as vermicompost] on yield and soil quality under a maize–rice rotation system. Among N sources, NS4 produced the highest maize grain yield (10 Mg·ha⁻1). Residual effects of N sources on mean rice grain yield were evident only in crop residue (NS2)- and vermicompost (NS4)-treated plots. After the harvest of two complete maize–rice crop cycles, higher content of dehydrogenase activity (DHA) and urease activity (UR) were observed in the soil under AT as compared to ZT and CT at 0–10 cm (p < 0.05). Similarly, microbial biomass carbon (MBC) and microbial biomass nitrogen (MBN) also recorded positive changes at 0–10 cm soil depth, especially in NS2 and NS4 treatments. AT resulted in the highest total soil carbon (TOC) (8.10 g·kg−1), followed by CT (6.73 g·kg−1) and ZT (5.98 g·kg−1). Fertilizer N treatments, however, influenced the NO3-N accumulation beyond the root zone, where crop residue-based (NS2) fertilizer N treatment resulted in the highest NO3-N (32.52 kg·ha−1), and the lowest NO3-N (14.48 kg·ha−1) was observed in the FYM-based (NS3) treatment. Therefore, the practice of alternate tillage and integration of vermicompost (40 kg·N·ha−1) and chemical fertilizer (total 120 kg·ha−1) sources should be mostly recommended to farmers in the Terai region of India.
Introduction
Long-term conventional tillage (CT) degrades soil quality through the decomposition of organic matter, thus affecting soil physical, chemical, and biological properties (Liu et al., 2006). Intensive land management results in exposure of soil organic matter (SOM) to high temperature, causing oxidation of profile SOC (Srinivasarao et al., 2020). Furthermore, agriculture associated with improper nutrient management under conventional practices degrades the status of SOC (Rakesh et al., 2021a). To overcome the adverse effects of CT, conservation agriculture (CA) practices like zero tillage (ZT) and alternate tillage (AT) have been important recommended strategies to improve SOC, crop yield, soil quality, and ultimately agricultural sustainability (Omara et al., 2019; Krauss et al., 2020). Crop residue management under the ZT system has a great impact on soil physicochemical and biological properties (Rakesh et al., 2021b). Potential benefits of CA through retention of crop residues on the soil surface have been reported by many researchers throughout the globe including the Indo-Gangetic plains of India (Gathala et al., 2011; Islam et al., 2019; Mitra et al., 2020). However, sustainable tillage systems such as AT and ZT are soil- and crop-specific, and their successful adoption is governed by both bio-physical and socio-economic factors (Prager and Posthums, 2010). Benefits of AT and ZT over CT are significant, especially under long-term cropping practices (Krauss et al., 2020). Rashidi and Keshavarzpour (2007) reported that CT results in loose and finer soil structure as compared to no-tillage and conservation tillage systems and causes decreased water movement into the soil profile, thereby decreasing nitrate leaching.
Continuous over-application of chemical fertilizers with minimum or no use of organic matter for several decades has resulted declined fertility and productivity of soil (Pernes-Debuyser and Tessier, 2004; Dong et al., 2012; Meena et al., 2017), increase in ground water pollution (Chandini et al., 2019; Cooke, 1982), and eutrophication (Feigin and Halevy, 1989). Moreover, intensive agricultural practice without adherence to scientific principles and ecological aspects has led to the loss of soil quality, depletion of freshwater resources, and agrobiodiversity (Kesavan and Swaminathan, 2008). Implementation of suitable fertilizer application programs, which would reduce the chemical fertilizer requirement, with appropriate tillage practices in a sustainable way can enhance soil quality, optimize crop yields, and control the negative impacts on the environment. Reports confirm that integrated fertilizer use which combines organic and inorganic nutrient sources can maintain the soil nutrient status for extended periods toward achieving sustained crop yields across cropping systems and soil types (Srinivasarao et al., 2020). Organic amendments not only increase TOC and its different pools but also accelerate the soil microbial activity and thereby increase the microbial biomass carbon (MBC), microbial biomass nitrogen (MBN), soil enzymatic activities, and in turn soil quality (Melero et al., 2008; Sharma et al., 2020). In deep vertisols of central India, application of cattle dung at 11.2 t·ha−1 on N-equivalent basis to maize crop resulted in increased biological activity of the dehydrogenase enzyme of soil (116.8 μg triphenyl formazan g soil−1 24 h−1) as compared to chemical fertilizers at 100:50:30 kg·ha−1 of N, P2O5, and K2O, respectively (83.2 μg TPF g soil−1 24 h−1) (Ramesh et al., 2008). In a long-term experiment with different nutrient management practices in a maize–onion cropping system, enzymatic activities (dehydrogenase, acid phosphatase, and alkaline phosphatase) in soil were higher with organic nutrient management compared to the chemical fertilizer program (Sridevi et al., 2011).
A study on integrated nutrient management of maize in the Terai region of West Bengal revealed that application of 75% NPK (of the recommended dose) through chemical fertilizers + FYM at 2 t·ha−1 resulted in greater plant height (219.0 cm), leaf area index (3.35 at 45 days after sowing), and dry matter accumulation (872.40 gm−2) at harvest and produced higher crop yield (Haque et al., 2012). Nyamangara et al. (2003) reported that nitrogen fertilizer, especially the high rate (120 kg·N·ha−1), and manure plus N fertilizer combinations resulted in high nitrate concentrations (up to 37 mg·N·L−1) and nitrate losses (up to 56 kg·N·ha−1·yr−1), leading to environmental and economic concerns in tropical sandy soil nitrate leaching from only manure treatments was relatively low (average less than 23 kg·N·ha−1·yr−1). The low-manure (12.5 t·ha−1) plus 60 kg·N·ha−1 fertilizer treatment was the best treatment to maintain dry matter yield and minimize N leaching losses.
Adverse effects of long-term intensive tillage along with non-judicious application of chemical fertilizers on depletion of SOC, microbial activity, and nutrient use efficiency have been reported from tropical and sub-tropical environments (Kai and Tamaki, 2020; Bhatt et al., 2019; Chandini et al., 2019). A study in the subtropical region suggested that ZT along with crop residue significantly enhanced the C sequestration rates (Pathak et al., 2017) and improved the microaggregate-associated C content (Bhattacharya et al., 2020), thus resulting in better conservation of SOM and reducing nutrient loss.
However, research on the impacts of tillage and nitrogen (N) rates on maize (Zea mays L.)–rice (Oryza sativa L.) crop productivity, soil fertility, and nitrate accumulation in soil profile specifically in the Terai zones is limited. Agriculture in Cooch Behar district, located in the northern part of West Bengal and in the foothills of the Himalaya, which comprise the Terai zone, differs from other regions in north-eastern India in terms of soil and climate. The climate of the region is sub-tropical and per humid in nature. Based on soil fertility classification, soils are characterized by low- to moderate-fertility status. It represents rice-based cropping system with high-volume application of inorganic fertilizer. Within this agro-climate, there are dearth of experiments that have compared the ZT and AT in conjugation with sustainable alternatives for fertilizer sources (Blanco-Canqui and Wortmann, 2020), which restrains current knowledge from recommending improvements over the existing management practices.
In the current study, our goal was to evaluate whether the short-term application of organic manures and mineral fertilizers along with ZT and AT could improve the soil quality such as enzyme activity (dehydrogenase, phosphatase, and urease), MBC, MBN, soil chemical properties (TOC and TN), NO3-N accumulation in the soil profile, as well as the yield of maize and rice grown in rotation.
Materials and methods
Rationale to select the experiment
The soil of north Bengal that represents the Terai zone is sandy loam in texture, which is the key reason for leaching losses of soil nutrients. Maize (Zea mays L.) and rice (Oryza sativa L.) are the dominant staple food crops of this region, grown in rotation. Each crop is highly responsive to fertilizer N input. However, due to the leaching of N, plants suffer from low N use efficiency, often leading to N deficiencies and low productivity. Continuous tillage systems are also one of the major factors that cause losses in nitrogen.
Experimental site
A 2-year field experiment from 2017 to 2019 was conducted at the research farm of Uttar Banga Krishi Viswavidyalaya, Pundibari, Cooch Behar, West Bengal, India (26⁰19′ N, 89⁰23′E; 41 m above msl). The experimental site is located within the Terai agro-ecological region of West Bengal, India, which is characterized by a sub-tropical per-humid climate (Figure 1). The area receives an annual rainfall of 3,261 mm, of which 80% or more is received during June to December. The mean maximum and minimum (monthly average) air temperatures were recorded as 30.1 and 19.7°C, respectively (average of 30 years: 1989–2019). Atmospheric temperature recorded during the study period showed minor differences from the corresponding long-term data (Figure 2). The soil of the Terai region is formed from deposition of alluvial sediments transported by the river Tista and are commonly referred as Tista alluvial. Physiographically, this is one of the sub-divided forms of the extensive stretch of the Indo-Gangetic alluvial plains (Debnath et al., 2016).
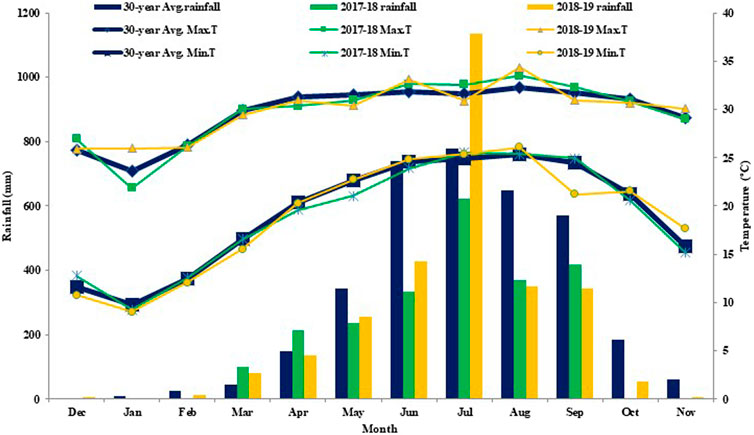
FIGURE 2. Monthly average rainfall (bar) and maximum and minimum temperatures (line) in 2017–18, 2018–19, and 30-year average.
This study site was characterized by a 25-year history of continuous cropping (cereals, pulses, and oilseeds) under CT with exclusively mineral-based fertilizer use. Soils are moderate- to low-fertility (Table 1), recent alluvial soil type (NBSS and LUP Classification, 2001) and physicochemical soil properties of the experimental field at the start of the study are presented in Table 1. The following crop rotation was used in the area: maize in rabi season and rice in kharif season.
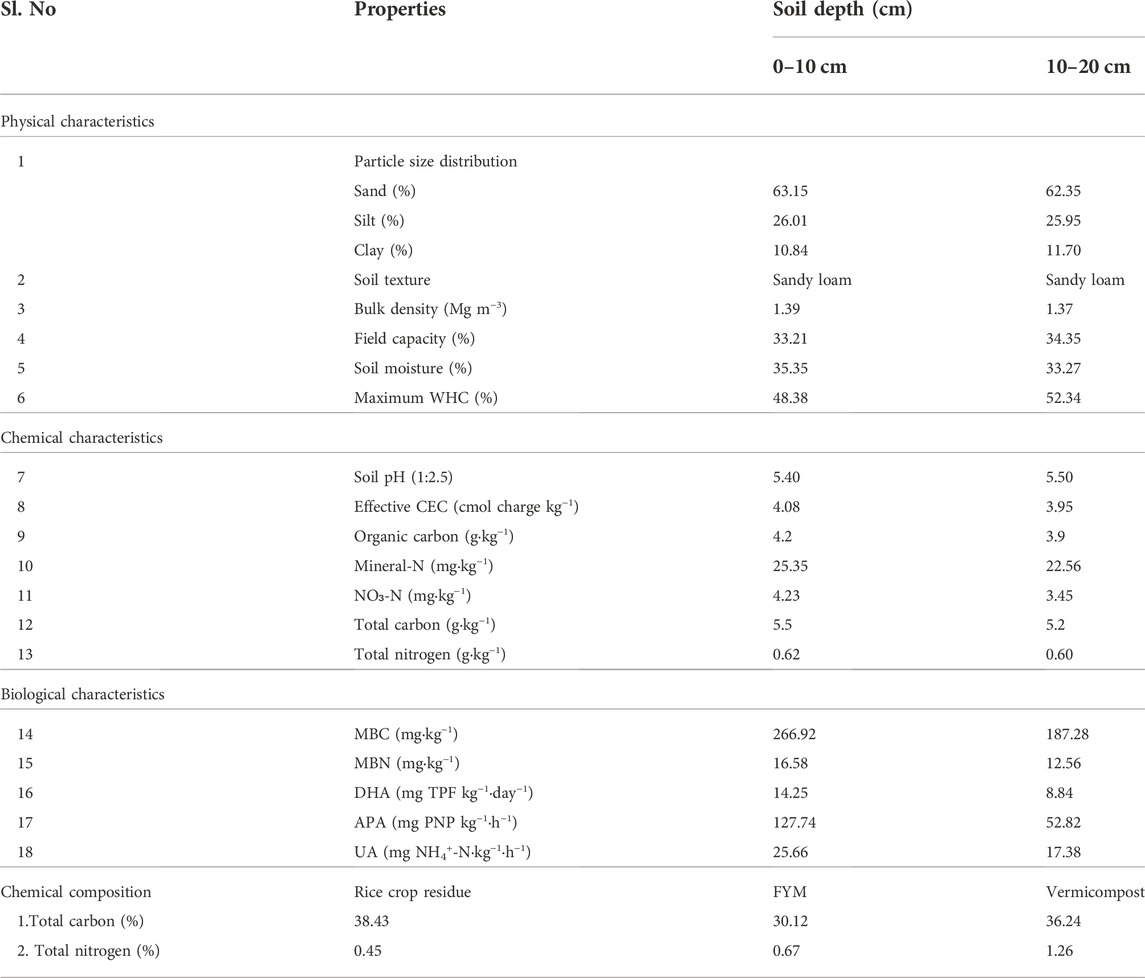
TABLE 1. Initial status of soil physicochemical and biological properties at the experimental site and characterization of organic amendments used in the experimental plot.
Experimental design and treatments
The experiment was established in November 2017, in a split-plot design with three replications, with a plot size of 56 m2 (8 m × 7 m). The main plots were three tillage practices [ZT = zero tillage (without soil disturbance; maize seed sown by using zero-till seed-cum-fertilizer drill and rice transplanted by rice transplanter), CT = conventional tillage; three passes by tractor fitted with cultivator, two passes of power tiller, and two passes by rotavator for final land preparation, AT = alternate tillage (alternate tillage in this field experiment implied as the cultivation of first crop (maize) in zero tillage (ZT) condition and of succeeding crop (rice) in conventional tillage (CT) practice]. The sub-plot treatments consisted of 5 N sources–N control (NS0: N0P50K100), full recommended rate of NPK fertilizer (NS1: N160P50K100), full recommended rate of NPK fertilizer along with crop residue (CR) application (NS2: NS1 + CR@ 6 Mg·ha−1), 75% of fertilizer N along with farmyard manure (FYM: N-0.67%) at 25% N (NS3: N120 P50K100 + FYM at40 kg·N·ha−1), and 75% of fertilizer N plus 25% with vermicompost (VM: N-1.26%) (NS4: N120 P50K100 + VM at 40 kg·N·ha−1).
Crop management
In CT plots, chemical and organic fertilizers were incorporated at the time of tillage operation, while in ZT and AT plots, the fertilizers were spread on the surface. Maize (Var.-DKC 9081) was sown in both the years on 25th November and harvested on 28th April. Three irrigation events were performed in all the treatment plots including control plot at the 7th leaf stage (35 days after sowing), 12th leaf stage (75 days after sowing), and reproductive stages (115 days after sowing) of the maize crop. At 80% maturity, plants were harvested manually from each plot to estimate the grain and straw yield. To assess the residual effects of organic amendments in the succeeding rice crop, a uniform application of NPK (80:40:40) was applied to all plots, except to the control plot. The rice variety “Swarna sub-1” was sown in ZT, CT, and AT plots in both the years on 12th June and harvested on 2nd November. Rice seedlings were sown by a rice transplanter in ZT plots under flooded condition (no soil disturbance). Rice transplanting was done by a rice transplanter in CT and AT plots. Three rounds of irrigation (35, 90, and 125 days after sowing) were given to all treatments including the control plot in rice.
Soil sampling and analysis
After one full rotation of maize–rice crops, soil samples were collected from rhizosphere using 5 × 10 cm core augur from 0–10 and 10–20 cm soil depths from three locations in each plot and made it into one composite sample. After removing all stubble, residues, and root biomass, a composite soil sample of approx. 500 g was obtained. These samples were then air-dried, ground using a wooden mortar and pestle, then sieved through a 2-mm sieve, and preserved in air-tight polythene containers for further analysis. The pH of the soil suspension (1:2) was measured potentiometrically by using a glass electrode-pH meter (Jackson, 1973). Bulk density (BD) was determined for 0–5, 5–10, and 10–20 cm of soil using a steel core of 100 cm3 following the standard method (Blake, 1965). Maximum water holding capacity (WHC) was estimated by the Keen box method (Anderson and Ingram, 1989). Field capacity moisture content was estimated after the soil has drained for 2–3 days following saturation (Anderson and Ingram, 1989). Soil particle size distribution was determined by hydrometer method (Bouyoucos, 1927).
For biological analyses such as microbial biomass carbon (MBC), microbial biomass nitrogen (MBN), dehydrogenase activity (DHA), acid phosphatase activity (APA), and urease activity (UA), soil samples were collected from 0–10 and 10–20 cm soil depths from each plot at the start (0 days after sowing) and end (145 days after sowing for rice and 170 days after sowing for maize) of the experiment. Field-moist soil samples were passed through a 2-mm mesh sieve and stored at 4°C for further analysis. Soil total organic carbon (TOC) was measured by the TOC analyzer (Vario EL, Elementar Analyse Systeme GmBH, Hanau, Germany). The MBC was estimated by chloroform fumigation method (Jenkinson, 1988). Soil MBN was evaluated by the method (Anderson and Ingram, 1993). Dehydrogenase activity was estimated using the procedure outlined by Casida et al. (1964). For determination of acid–phosphatase activity, 1 g (oven-dry equivalent) soil incubated with p-nitrophenyl phosphate, toluene, and modified universal buffer (MUB)-pH-6.5 for 1 h at 37°C. The yellow color intensity was measured at 440 nm wavelength in a visible spectrophotometer (HALO VIS-10; Company Dynamica) (Tabatabai and Bremner, 1969). Urease activity (mg ammonium (NH4+) kg−1 h−1) was measured using the procedure described by Tabatabai and Bremner (1972). NO3-N was determined using the H2SO4–salicylic acid method by a visible spectrophotometer at 410 nm wavelength (Cataldo et al., 1975). Mineral N was estimated by the summation of NH₄⁺-N and NO₃‾-N, where mineral N = (NH₄⁺)-N + (NO₃‾)-N. The NH4+-N was determined from the soil extracts by Indophenol Blue method (Brown, 1973).
Statistical analysis
All the data were checked for normality using the Shapiro–Wilk test in SPSS (SPSS Inc. Released 2007. SPSS for Windows, Version 16.0. Chicago, United States). As in the heterogeneity of the variance test, there were no significant effects of year on the treatments; data of 2 years were pooled and used as replications in subsequent analyses. Analysis of variance (ANOVA) for each parameter was performed using SPSS (Version-16.0) package. Comparison of treatment means was performed by either LSD or Duncan’s multiple range test (DMRT), as indicated, at α = 0.05. For NO3-N accumulation at five depths, linear regression analysis was performed following ANOVA for rate of accumulation through 120 cm as a function of tillage and N-source treatments using the REG procedure in SAS software (SAS, Cary, NC).
Results and discussion
Effects of tillage and nitrogen sources on grain and stover yield of maize crop
The yield of maize was more in the first year and lower in the second year, though the treatment-wise trend followed the same pattern. The lower yield in the second year might be due to low rainfall received during the crop growth season (Figure 2). Two-year pooled (2017–18 and 2018–19) data on grain yield (GY) and stover yield (SY) of maize (Table 2) indicated that tillage had a significant effect on GY. A yield advantage of 4.4% was found both under CT (8.26 Mg·ha−1) and AT (8.25 Mg·ha−1) over ZT (7.91 Mg·ha−1). Higher GY under CT and AT might be due to the temporary improvements in soil aeration, water infiltration, and nutrient uptake necessary for plant growth (Mando et al., 2005).
Maize GY was affected by N sources in a pooled data of two seasons (Table 2 and Figure 3). Fertilizer N treatments with organic matter (crop residue, FYM or vermicompost) produced higher GY than the application of chemical N fertilizer alone. The highest grain yield (10 Mg·ha−1) was recorded under NS4, and was 67.23% higher than the control (NS0; without N). No significant difference in GY was observed between NS1 and NS3 treatments. However, crop residue-amended fertilizer N (NS2) treatment resulted in the second highest GY (9.57 Mg·ha−1). The finding was in agreement with Banik and Sharma (2008), who reported that soil surface covered by residue mulching improves crop yields through soil water conservation, weed control, and increased population of micro-flora which facilitate the decomposition and release of nutrients. Vermicompost with fertilizer N (NS4) treatment under either CT or AT proved to be superior with respect to GY. Similar findings were reported by Ali et al. (2011) who observed that maize grain yields were significantly higher (4.8 Mg·ha−1) under inorganic fertilizers combined with organic N sources. The growth and yield of maize under FYM (N4) combined with N fertilizer treatment was higher by 1.1 Mg·ha−1 than under fertilizer N recommended solely as chemical source (NS1) (Table 2). This could be attributed to greater soil water content, higher nutrient availability due to slow release of nutrients, and reduced nutrient leaching compared to the control (Chiroma et al., 2006).
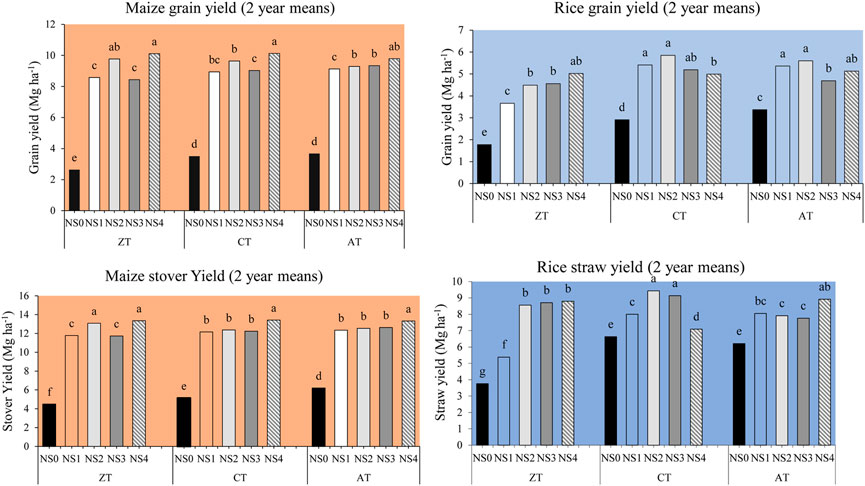
FIGURE 3. Mean yields for maize grain and stover & rice grain and straw over a 2-year cropping sequence. Same letters above the bars indicate no significant difference across the treatment combinations as tested by Fisher’s LSD comparison of means (α = 0.05). ZT, zero tillage; CT, conventional tillage; AT, alternate tillage (maize: zero and rice: conventional tillage), NS0 = no nitrogen (control, 0:50:100), NS1 = recommended dose of NPK fertilizer (N160P50K100), NS2 = NS1+ crop residue at 6 Mg/ha, NS3 = 75% of recommended N as fertilizer along with 25% N as FYM (N120 P50K100 + FYM at 40 kg·N/ha), NS4 = 75% of recommended N as fertilizer along with at 25% N as vermicompost (N120 P50K100 + VM at 40 kg·N/ha).
The SY results followed a similar trend to that of GY under the influence of tillage and N sources in both the seasons (Table 2). The average data of two seasons showed that AT produced higher SY (11.41 Mg·ha−1) as compared to CT and ZT. No-till (NT) reduces soil disturbance, increases soil organic matter accumulation (Rakesh et al., 2020), and also reduces crop yields (Dai et al., 2021). Among N sources, NS4 resulted in higher mean SY (13.37 Mg·ha−1) followed by NS2 (12.67 Mg·ha−1) in both the seasons (Table 3). Like GY, SY obtained from the combined applications of organic and inorganic N sources was greater than that obtained by the solo application of inorganic fertilizer (12.10 Mg·ha−1). From the pooled average of two seasons, the highest SY was achieved under NS4 combined with any of the tillage practices. However, SY also responded well to ZT combined with the NS2 treatment, and it was statistically similar to the ZTNS4 treatment (Table 2). Similarly, ZT with crop residue and inorganic fertilizer application (NS2) had a positive effect on SY of maize crop. Greater SY and GY in NS4 regardless of tillage methods indicated that the N treatment included with vermicompost had a positive effect on crop yields. Inclusion of vermicompost along with inorganic nitrogenous fertilizers enhanced the crop yields (Rathod et al., 2013).
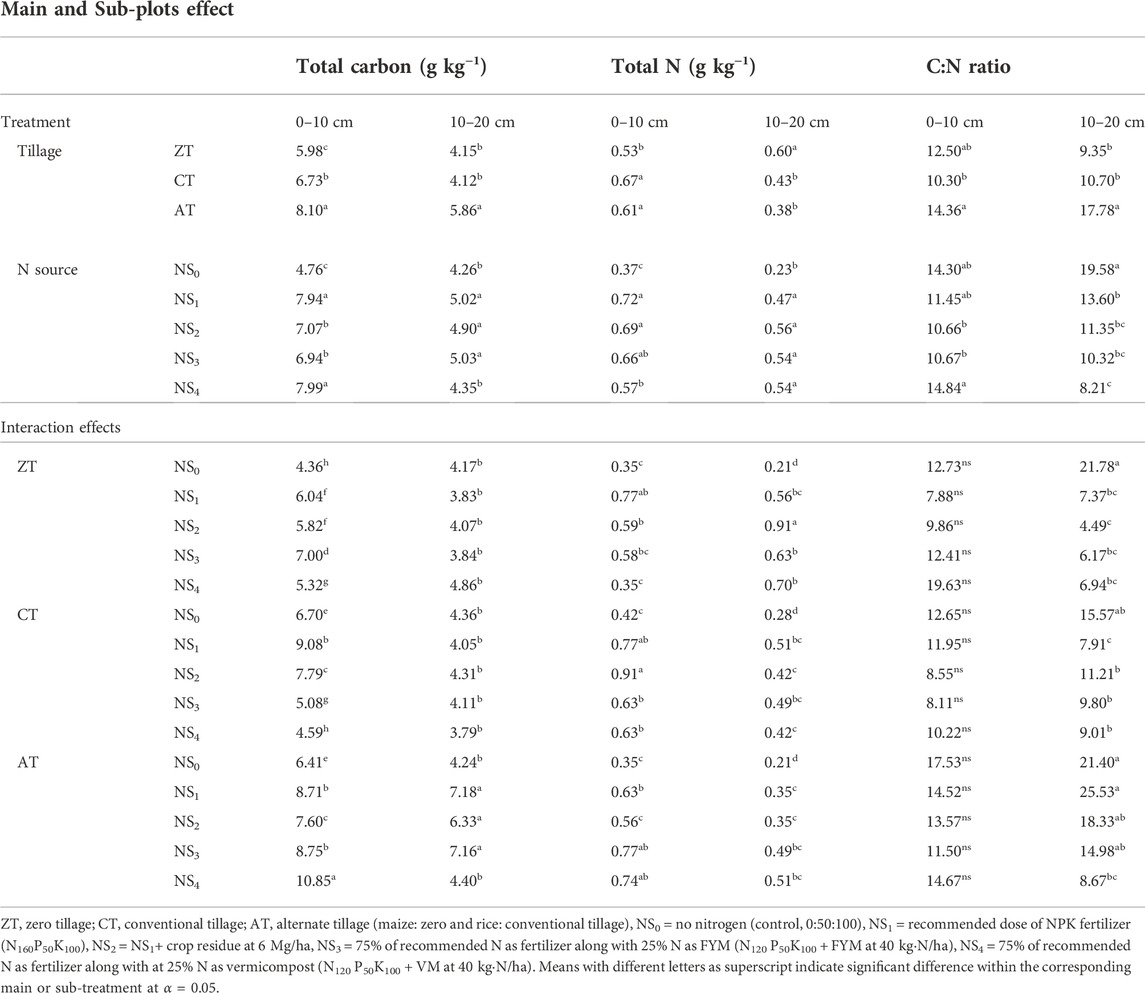
TABLE 3. Effects of tillage practices and N sources on the total carbon (SOC; g·kg−1), total N (TN; g·kg−1), and the C:N ratio in soil at the end of the experiment.
Effects of tillage and nitrogen sources on grain and straw yield of rice crops
The mean rice GY over two seasons under ZT was significantly lower (3.90 Mg·ha−1) compared to CT (4.87 Mg·ha−1) and AT (4.83 Mg·ha−1). Karki et al. (2005) observed an average GY of 3.66 Mg·ha−1 under ZT, and it was statistically significant over CT (2.88 Mg·ha−1) which was in contrast with the findings of our study. In both the seasons, the performance of ZT was poor as compared to those of CT and AT for both rice GY and SY. The rice crop performs well under deep tillage which improves the root length, root proliferation, grain yield, and nitrogen recovery efficiency (NRE) (Motavalli et al., 2003).
Plots under crop residue as organic amendment in the previous maize crop showed a clear residual effect on rice GY. An increase of 10.39 and 5.14% GY over FYM- and vermicompost-based fertilizer N treatments were observed, respectively (Table 2). Budhar et al. (1991) reported that higher rice yield was obtained with organic manures indicating a residual effect of manures on crop yield in the rice–wheat rotation. These findings are in line with those of Ramamurthy and Shivashankar (1996) who reported that organic and inorganic fertilizers applied to preceding crops had a measurable residual effect on yield and yield-contributing components of the succeeding crop. While it is reasonable that all organic materials included in N treatments had some residual impact on rice GY due to continued decomposition and release of nutrients, particularly N. The residual effect of crop residues (over FYM and vermicompost) was likely due to a prolonged release of N (Ramamurthy and Shivashankar, 1996). Crop residues have a greater C:N ratio than manures and composts, resulting in a delay of N release to crops due to microbial immobilization (Power et al., 1998; Marzi et al., 2020). The CT and AT each when combined with crop residue treatment (CTNS2 and ATNS2) had a direct impact on increased rice yield, and it was significantly higher than the sole application of fertilizer N-treated plots in the corresponding tillage practices. The ZT practice showed relatively low response than the rest of two tillage methods. Deep tillage improved the root length, root proliferation, grain yield, and nitrogen recovery efficiency (NRE), that is, lower NRE was recorded in no-till soil treatment than the compacted in sub-soiling treatments (Motavalli et al., 2003) of TOC.
Regardless of the tillage treatments, soil TOC was found to decrease with soil depth after two cycles of maize–rice crops (Table 3). In the soil layer (0–10 cm), plots under AT were found to maintain the maximum amount of TOC (8.10 g·kg−1) compared to CT (6.73 g·kg−1) and ZT (5.98 g·kg−1). Similarly, plots under AT also resulted in higher TOC in the lower soil depth (10–20 cm). Increases in TOC under AT were equivalent to 47.27% and 12.69% at 0–10 and 10–20 cm soil depth, respectively, at the end of the 2nd year in comparison to the initial status. The findings of the present study were in agreement with Cassity-Duffey et al. (2020) who found that the crop residue-treated plots showed consistently higher amounts of TOC at all soil depths than plots where no crop residue was added, though the effect was not significant. Ghimire and Craven (2011) reported no significant change in TOC under short-term practice of NT and also suggested that organic carbon accumulation in soil is considered as a slow process wherein many years might be required to accumulate significant amounts of organic matter in the soil.
TOC varied significantly among different N sources. In our study, both vermicompost-based fertilizer N(NS4) and sole application of fertilizer N (NS1) treatments had similar effects on accumulation of TOC in the uppermost layer (0–10 cm) at the end of the experiment. Malhi et al. (2006) reported a higher content of TOC under organic fertilization as compared to conventional fertilization in silty-loam soil. No such variation was observed between FYM-based and crop residue-based fertilizer N treatment. Application of rice straw in combination with 100% NPK resulted in significant build-up of TOC over control plots after 2 years.
Tillage× N sources had significant implications on the accumulation of TOC in both the soil layers (0–10 cm and 10–20 cm). Results revealed that positive and negative changes occurred due to the influence of treatment combinations and succeeding cropping systems (Table 3). In most of the cases, positive changes occurred in surface (0–10 cm) soil layer and negative changes, in the subsurface one (10–20 cm). The ATNS4 treatment had a profound increasing effect on the accumulation of TOC in the uppermost layer (0–10 cm), followed by CTNS1, ATNS3, and ATNS1 treatment combinations. The AT treatment combined with sole application of fertilizer N- and FYM-based treatment had a residual effect on the accumulation of TOC in lower layer (10–20 cm) at the end of the experiment. Minute positive and negative changes in both the soil layers were noticed because TOC did not appear to be very responsive to differences in management in the short-term period, but its concentration was significantly higher than the sole application of chemical N source due to integrated use. Bhattacharyya et al. (2012) reported that ZT may be a more desirable practice than CT combined with organic-based inorganic N treatment under an irrigated rice–wheat system in the Indian Himalayas from the view point of TOC retention.
Total nitrogen
ZT significantly decreased TN by 16.98% at the 0–10 cm soil depth, while an increase of 8.06% was obtained under CT practice for the corresponding soil depth at the end of two maize–rice crop cycles (Table 3). Non-significant variation in the total N content between CT and AT was noticed in both the soil layers. These findings were in contrast with Melero et al. (2006) who found that N concentration of minimum tillage (MT) was significantly higher than the CT system. Numerous studies also showed higher soil TN under reduced tillage compared to the CT systems (Melero et al., 2006; Perez-Brandán et al., 2012).
Total N content significantly varied among different N sources. In the uppermost soil layer (0–10 cm), higher total N content was observed due to the sole application of chemical N fertilizer and crop residue-based N treatment as compared to organic amendment-based fertilizer N treatments. Plots under 100% NPK treatment significantly increased total N over unfertilized control (NS0). Positive changes of total N were recorded in the 0–10 cm soil layer under NS1, NS2, and NS3 treatments only. In the 10–20 cm depth, negative changes (−0.14 to −0.07 g·kg-1) in total soil N occurred under all N treatments. Crop residue-based N treatment and sole application of fertilizer N improved the soil TN as compared to vermicompost- and FYM-based N treatment at uppermost (0–10 cm) soil layer at the end of maize–rice crop growing seasons. Results revealed that the crop residue-based fertilizer N application had significant effect on soil TN at the uppermost soil layer (0–10 cm). Interaction effect between tillage and N sources was found to be significant in the case of both the soil depths (Table 3). The ZTNS1 and CTNS1 treatment combinations produced similar results with ATNS3 in terms of total N content at 0–10 cm at the end of the experiment.
C/N ratio
Carbon-to-nitrogen ratios (C/N ratio) of 14.4 and 17.8 for the 0–10 and 10–20 cm soil depths, respectively, were obtained in the plots under AT treatment. These were higher than both ZT and CT after completion of two crop cycles (maize-rice). The highest C/N ratios in this study were observed under AT, while several other studies showed higher soil C/N ratios under NT than that of CT (Alijani et al., 2012; Naderi et al., 2016). Blanco-Canqui and Lal (2008) reported that the soil C/N ratio under plough tillage (PT) was greater than that under NT in 0–5 cm depth (p < 0.05), while no significant difference was observed for 5–20 cm soil depth. This difference in results might be due to differences in climate, soil type, residue inputs, and farming management that could affect the C/N ratio (Bhattacharya et al., 2020).
Significant variation in the C/N ratio was observed among different N sources, where vermicompost-based fertilizer N-treated plot and sole application of fertilizer N-treated plot produced the highest C/N ratio at 0–10 cm at the end of the experiment. An increase in the C/N ratio was observed 0–10 cm following all N treatments. DeSa et al. (2001) also reported a similar trend of reduction of the soil C/N ratio with depth (Nascente et al., 2013). This might be attributed to high C/N and leaching of highly soluble organic compounds (e.g., organic acids) into deeper soil layers (Puget and Lal, 2005). In the current study, interaction between tillage and N sources was non-significant for the C/N ratio in the uppermost surface (0–10 cm). However, it was significant in the lower depth (10–20 cm).
Microbial biomass carbon
The highest amount of MBC was obtained under ZT, followed by CT and AT in 0–10 and 10–20 cm soil depths at the end of the 2 years of the experiment. The MBC under ZT was 373.78 mg·kg−1 and 223.19 mg·kg−1 at 0–10 cm and 10–20 cm, respectively. The MBC were 40.03 and 16.08% greater than those of CT plots for the corresponding depths. Negative changes in MBC were recorded in both the layers under AT practice at the end of the experiment.
Among different N sources, the highest MBC was found in the FYM-based fertilizer NS3-treated plots (385.43 mg·kg−1) for 0–10 cm soil depth. The MBC concentration at 10–20 cm was statistically at par with NS1 and NS2 treatments followed by NS4 (Table 4). Increase of 44.39 and 7.6% were observed in 0–10 cm and 10–20 cm soil layers, respectively, in the FYM-based fertilizer N-treated plots. Interestingly, both the control (NS0) and vermicompost-based fertilizer N (NS4)-treated plots showed negative changes in MBC at both soil depths. The sole application of fertilizer N (NS1) and combined application of crop residue with fertilizer N (NS2), resulted in positive changes of MBC in both the soil layers.
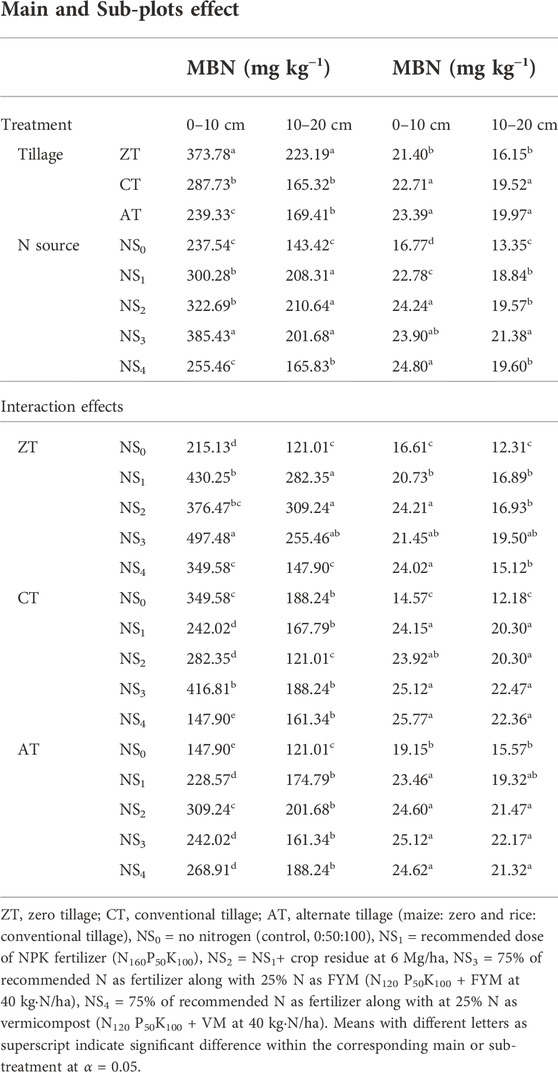
TABLE 4. Effects of tillage practices and N sources on the status of MBC (mg·kg−1) and MBN (mg·kg−1) in soil at the end of the experiment.
Microbial biomass increases with increasing rate of application of FYM in treatments receiving mineral N along with FYM DeSa et al. (2001).
Interaction between tillage and N sources on MBC was found to be significant (Table 4). Although the MBC concentration did not follow any uniform pattern among the treatment combinations, ZT combined with the FYM-based fertilizer N-treated plots accorded the highest value (497.48 mg·kg−1) at 0–10 cm compared to all other treatments. In all the treatments, the 0–10 cm soil layer found to have more MBC than the 10–20 cm soil layer. The present study demonstrated that the interactive effect of tillage and N sources on rhizosphere conditions induced by plant growth played a major role in the improvement of MBC to a greater extent at the end of crop growing seasons. These findings were similar with those reported by Kukreja et al. (1991) who stated that use of organic amendments and reduced tillage can play an important role in increasing SOC and MBC compared to CT without affecting crop yield (Diekow et al., 2005) and microbial biomass nitrogen (MBN).
The CT and AT practices were statistically at par in MBN changes for both 0–10 and 10–20 cm depths (Table 4). In CT, MBN content for 0–10 and 10–20 cm soil depths were 22.71 and 19.52 mg·kg−1, while in AT, MBN were 23.39 and 1.97 mg·kg−1, respectively. The lowest MBN of 21.40 and 16.15 mg·kg−1 was observed in 0–10 cm and 10–20 cm soil depths under ZT. At the 0–10 cm depth, ZT, CT, and AT resulted in increase of 29, 37, and 41%, respectively, as compared to the initial MBN (16.58 mg·kg−1). At 10–20 cm depth, the increase in MBN for ZT, CT, and AT were 29, 55, and 59%, respectively. Lower values of MBC and MBN in lower soil depth could be due to the presence of higher amount of soil organic matter in the upper layer of soil (Kukreja et al., 1991).
The increase of MBN under all tillage practices is in contrast with the negative impacts of CT on MBC and MBN as reported by others (Banerjee et al., 2006; Meena and Biswas, 2014).
Integrated use of organic manures and chemical fertilizer significantly increased the MBN by 37.39, 46.20, 44.14, and 49.57% under NS1, NS2, NS3, and NS4, respectively, over control in the 0–10 cm soil depth. Soil amended with organic manures immediately increases SOC which stimulates microbial growth and activity (Spedding et al., 2004). The plots amended with integrated manures and 75% NPK fertilizers maintained higher MBN than 100% NPK fertilizer alone because manure provided accessible substrate C and N to promote the growth of microorganisms in soil (Gajda et al., 2013). Furthermore, when AT was combined with either vermicompost (NS4)-, or FYM (NS3)- and crop residue-based (NS2) fertilizer N treatments, MBN increase was more in both the soil depths.
Dehydrogenase activity
The DHA content in the initial soil was 14.25 mg TPF kg−1 24 h−1 at 0–10 cm and 8.84 mg TPF kg−1 24 h−1 at 10–20 cm (Table 5). The AT showed higher DHA (34.85%) and the least of 8.98% recorded in ZT plots. The effect of tillage on DHA was mainly confined to the upper (0–10 cm) soil depth. Greater DHA was observed at 0–10 cm than at 10–20 cm by the end of the experiment, which might be due to the greater availability of organic carbon and nutrients closer to the surface, as DHA in soil depends on soluble organic carbon (Kanchikerimath and Singh, 2001). Zaman et al. (2002) observed that DHA activity reduced in the sub-surface soil at all stages of crop growth due to decreased microbial activity with depth.
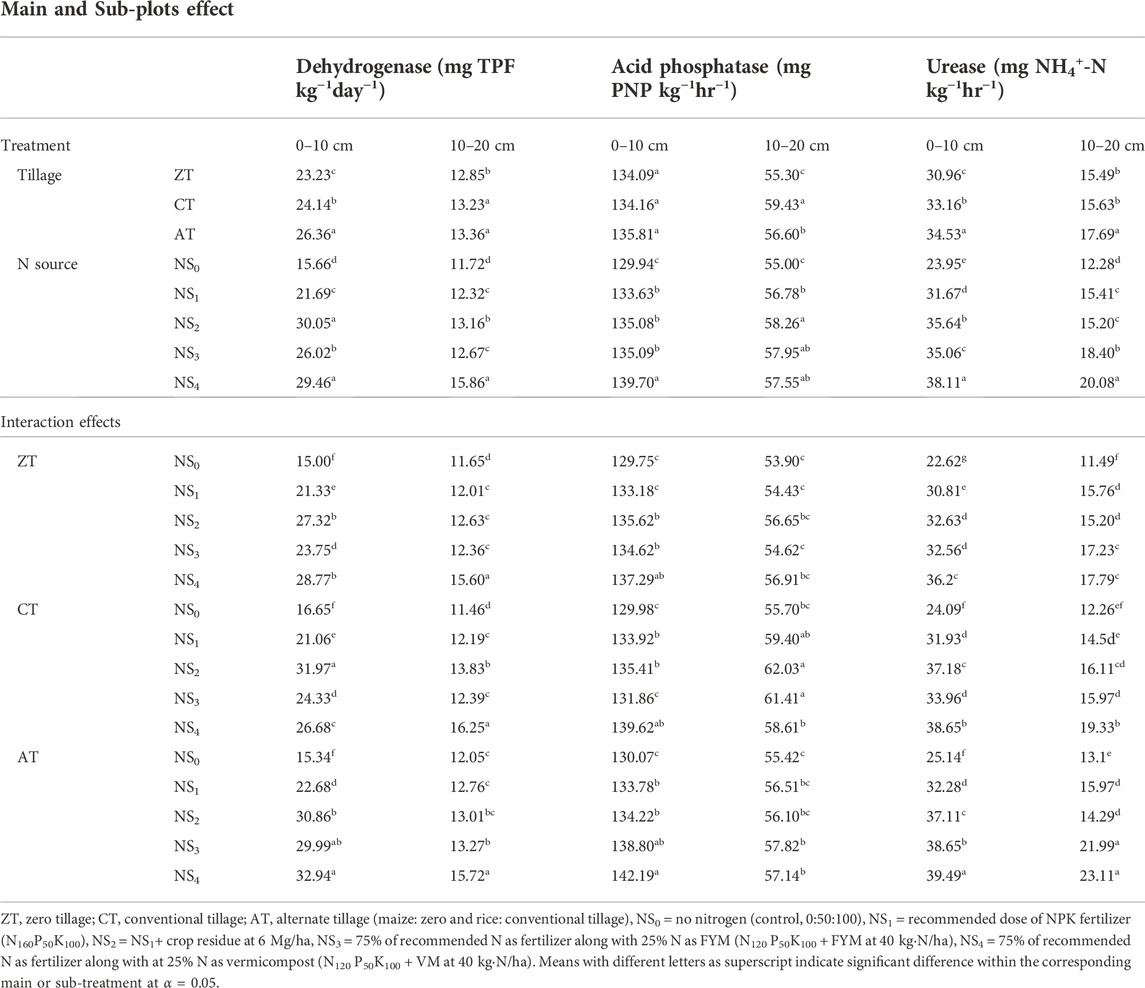
TABLE 5. Effects of tillage practices and N sources on the status of enzymatic activity (viz. dehydrogenase activity, mg TPF kg−1·day−1; acid-phosphatase, mg PNP kg−1·h−1; urease, mg NH4-N kg−1·h−1) in soil at the end of the experiment.
Significant variations of DHA were measured among the different N treatments (Table 5). At 0–10 cm, the vermicompost-based NS4 (15.21 mg TPF kg−1·day−1) and crop residue-based NS2 (15.80 mg TPF kg−1 24 h−1) treatments resulted in similar changes in DHA followed by FYM-based treatment (11.77 mg TPF kg−1 24 h−1). The chemical fertilizer NS1 treatment also resulted in positive but relatively lower changes (7.44 and 3.48 mg TPF kg−1 24 h−1) over the control (NS0;1.41 and 2.88 mg TPF kg−1 24 h−1) at both the 0–10 cm and 10–20 cm depths. At both depths, all integrated N treatment plots resulted in positive changes of DHA over chemical fertilizer N. Typically, composts comprise more stable carbon compounds than those found in manures and crop residues, which would require greater DHA for heterotrophic microorganisms to access C and N (Jat et al., 2020; Furczak and Joniec, 2007; Tremblay et al., 2010). The dehydrogenase activity was found to be low in treatment receiving 100% recommended dose of nitrogenous fertilizer (150 kg·N·ha−1) compared to integrated combinations of organics and fertilizers. The increased enzymatic activity with increase in manure level may be ascribed to the increased population of microbes due to increased availability of substrate (OC) (Parthasarathi et al., 2008). In a recent review on interpretation of microbiological indices of soil function, it was suggested that increased presence of a particular enzyme (e.g., DHA) is an indicator that a particular nutrient or substrate targeted by the enzyme is limiting (Verma and Mathur, 2009).
Acid phosphatase activity
Acid phosphatase activity ranged from 129.94 to 139.70 mg PNP kg−1 soil h−1 at 0–10 cm and 55.00–57.55 mg PNP kg−1 soil h−1 at 10–20 cm depth (Table 5). At 10–20 cm, however, the magnitude of APA from the lowest to highest was ZT (55.30 mg PNP kg−1 soil h−1), AT (56.60 mg PNP kg−1 soil h−1), and CT (59.43 mg PNP kg−1 soil h−1). The greatest mean value amongst N source treatments for APA was reported in the NS4 treatment at 0–10 cm. In the 10–20 cm soil depth, NS2 resulted in the greatest APA value, followed by NS1. Fierer et al. (2021) found that greater APA (722 mg PNP kg−1 soil h−1) was obtained by the incorporation of crop residue from cereal rye and compost (744 mg PNP kg−1 soil h−1). Takeda et al. (2009) reported reduced activity of APA following direct application of animal manures.
In both soil depths, organic integrated N sources increased the APA compared to the sole application of N fertilizer. Like DHA, APA was also greater in surface soil than sub-surface soil. The greater APA in the surface soil could be attributed to the greater demand for P from soil by the crops for its growth and symbiotic functioning (Antonious et al., 2020). Enzymes (acid and alkaline phosphatases) are housed within the root cells of plants and of soil (Mitran et al., 2018). Dakora and Phillips (2002) observed that phosphatase’s activities decreased with increasing soil depth. Both the density of roots and population of microorganisms also decrease with depth, necessarily decreasing the activity of an enzyme that is not released into the extracellular soil solution. Most enzyme activities in the surface soil were higher than those in deep soil. This may be due to the higher population of soil microorganism and plant residues in the surface soil, which were the main parts of soil enzymes.
Results of interaction of tillage × N sources on APA revealed generally positive changes in APA at 0–10 cm over the course of the experiment in most of the treatment combinations. These interactions imply that the use of vermicompost stimulates APA more than other organic amendments used in this study. It is suggested here that the principle put forth by Chaitanya et al. (2011) and presented in the previous section’s discussion on DHA is also applicable to APA. When soil phosphorus is limiting or present only forms that are biologically unavailable, APA is increased at the expense of energy in order to increase microbial access to phosphorus (Chaitanya et al., 2011).
Urease activity
Soil UA activity at 0–10 was the greatest under AT (34.53 mg NH4-N kg−1·h−1), followed by CT (33.16 mg NH4-N kg−1·h−1), and then ZT (30.96 mg NH4-N kg−1·h−1) (Table 5). At 10–20 cm, the same order of tillage treatments was repeated. Positive changes in UA were recorded in both the soil layers under AT practice only. Significant variations in UA due to N source was observed in both soil layers. The NS4 treatment at 0–10 cm resulted in the greatest UA (38.11 mg NH4-N kg−1·h−1) results, whereas the least UA was observed under NS0 at 10–20 cm (12.28 mg NH4-N kg−1·h−1). Positive change in UA over the course of the 2-year study was observed in the NS4 treatment in both soil depths. Organic integrated N sources all resulted in greater UA values than NS0 or NS1 at 0–10 cm. Only NS3 and NS4 resulted in greater UA values than N1 at 10–20 cm. The control treatment NS0 resulted in the lowest UA values at both depths. Meena and Biswas (2014) reported greater urease activity due to the addition of manure in the maize–wheat–cowpea cropping system. Similarly, high urease activity was also reported by Deng and Tabatabai (1997) in no tillage soil under a maize crop. Addition of organic manure was found to improve the microbial activities which in turn preferred the synthesis of various enzymes in soil (Bhattacharya et al., 2020). Significant interactions between different tillage practices and N sources on the status of UA in soil was observed (Table 5). The combined influence of AT with the vermicompost-based fertilizer N treatment had better response and recorded higher UR content followed by the FYM-based fertilizer treatment combined with AT practice in both the soil layers (0–10 cm and 10–20 cm). While negative changes were recorded in the control treatment (NS0) regardless of tillage practices in both the soil layers at the end of the experiment. AT combined with vermicompost-based fertilizer N was shown to increase 53.90% UR in the uppermost layer (0–10 cm) and 32.96% in the lower one (10–20 cm) over the respective initial levels in the corresponding soil layers. Unlike APA and DHA, UA is a function of “accumulated urease” released extracellularly by a wide variety of microorganisms belonging to many taxa (Roscoe et al., 2000). There are many molecular types of urease enzymes found in soil, and their stability is generally very high compared with other enzymes (Dinesh et al., 2000). Therefore, aligned with the outcomes of this study, it is reasonable to associate increased UA with conditions that promote the greatest number of microorganisms.
Accumulation of nitrate nitrogen in soil profile
The NO3-N accumulation ranged from 2.34 to 35.83 kg·ha−1 (Table 6). Tillage practice (p = 0.0437) and soil depths (p < 0.0001) had significant effects on NO3-N results. Though N source was not found to be significant (p = 0.1873), the interaction between tillage and N source was (p = 0.0173) not followed by any uniform pattern all along the depths (Table 6 and Figure 4). Among tillage treatments, CT and AT featured greater NO3-N concentrations than ZT throughout all the soil depths. Regression analysis for accumulated NO3-N for depth up to 120 cm included significant quadratic terms for each tillage treatment as follows: CT (1.74 + 0.1970*depth − 0.0008*depth2), ZT (1.13 + 0.1739*depth − 0.0007*depth2), and AT (NO3-N = 2.03 + 0.1594*depth − 0.0006*depth2). Linear terms decreased in the order CT > ZT > AT, indicating that reduced tillage can reduce downward migration of NO3-N. The maximum amount of NO3-N measured at 80–120 cm was 31.51 kg·ha−1 under ATNS2 and the least amount measured under ZTNS0 (9.61 kg·ha−1). Reduced tillage can therefore be recommended as a potential measure to mitigate N leaching losses (Catt et al., 2000).
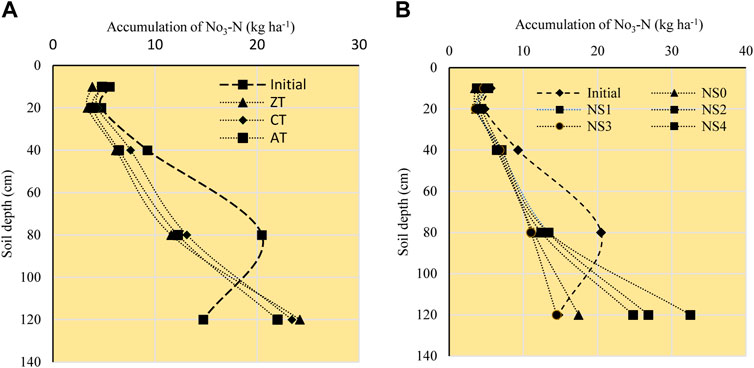
FIGURE 4. Effect of tillage (A) and N sources (B) on accumulation of nitrate nitrogen (kg·ha−1) in different soil depths at the end of experiment. ZT, zero tillage; CT, conventional tillage; AT, alternate tillage (maize: zero and rice: conventional tillage), NS0 = no nitrogen (control, 0:50:100), NS1 = recommended dose of NPK fertilizer (N160P50K100), NS2 = NS1+ crop residue at 6 Mg/ha, NS3 = 75% of recommended N as fertilizer along with 25% N as FYM (N120 P50K100 + FYM at 40 kg·N/ha), NS4 = 75% of recommended N as fertilizer along with at 25% N as vermicompost (N120 P50K100 + VM at 40 kg·N/ha).
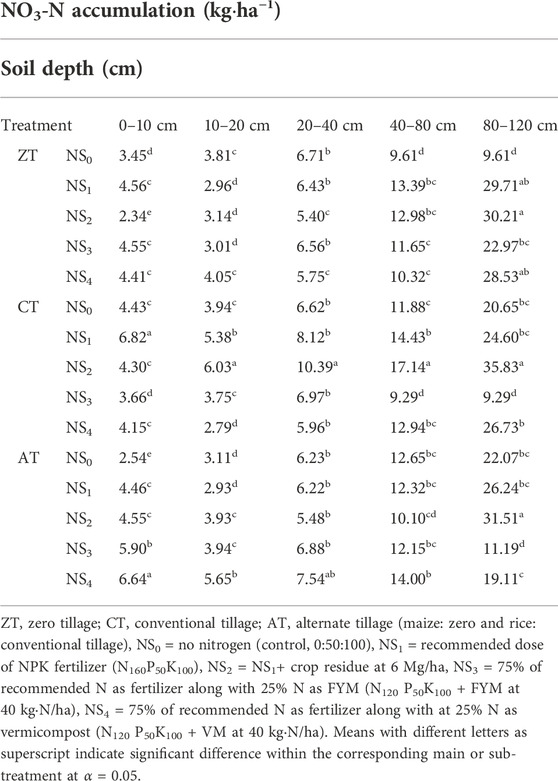
TABLE 6. Combined effects of tillage practices and N sources on nitrate nitrogen (NO3-N) accumulation (kg·ha−1) in different soil depths at the end of the experiment.
Conclusion
In a 2-year maize–rice double-crop sequence, maize GY was found to be influenced by tillage practices. Of these, AT and CT were responsible for greater maize yield than ZT. Among the N sources, integrated use of vermicompost (40 kg·N·ha−1) and chemical fertilizer N (total 120 kg·ha−1) resulted in the greatest maize GY and 12.6% more than the sole application of recommended fertilizer N (160 kg·ha−1). Positive effects on rice GY were observed only with the integrated use of crop residue or vermicompost with fertilizer N. At the end of two maize–rice cycles, AT showed significant positive changes in soil enzyme activity as well as MBC and MBN compared with CT and ZT, but these effects were confined to 0–10 cm soil depth. Increase in TOC was greatest under the AT treatment. Crop residue- and FYM-based fertilizer N treatment, however, resulted in a depletion of TOC as compared to the sole application of recommended fertilizer N. Accumulation of NO3−-N in soil with depth was affected by tillage but not by N source. All treatments were characterized by increasing NO3−-N with depth, though CT promoted more leaching to 80–120 cm than AT and ZT. In this study, maintenance of soil carbon and nitrogen, and soil microbiological activity were found to be enhanced mostly under ATNS2 and ATNS4 treatments. Therefore, the practice of alternate tillage and integration of organic and chemical fertilizer sources should be recommended to farmers in the Terai region of India.
Data availability statement
The original contributions presented in the study are included in the article further inquiries can be directed to the first/corresponding author.
Author contributions
SS, PM, PPM, and JM: conceptualization, conduction of experiment, collection of data, analysis, interpretation of result, writing of the manuscript, and editing. AS, AM, PS, and SR: analysis, interpretation of data, and editing.
Conflict of interest
The authors declare that the research was conducted in the absence of any commercial or financial relationships that could be construed as a potential conflict of interest.
Publisher’s note
All claims expressed in this article are solely those of the authors and do not necessarily represent those of their affiliated organizations, or those of the publisher, the editors, and the reviewers. Any product that may be evaluated in this article, or claim that may be made by its manufacturer, is not guaranteed or endorsed by the publisher.
References
Ali, K., Munsif, F., Zubair, M., Hussain, Z., Shahid, M., Din, I. U., et al. (2011). Management of organic and inorganic nitrogen for different maize varieties. Sarhad J. Agric. 27 (4), 525–529.
Alijani, K., Bahrani, M. J., and Kazemeini, S. A. (2012). Short-term responses of soil and wheat yield to tillage, corn residue management and nitrogen fertilization. Soil Tillage Res. 124, 78–82. doi:10.1016/j.still.2012.05.005
J. M. Anderson, and J. S. I. Ingram (Editors) (1989). Tropical soil biology and fertility (Wallingford: CAB international), 171.
J. M. Anderson, and J. S. I. Ingram (1993). A handbook of methods, 221. Wallingford, Oxfordshire: CAB International, 62–65.
Antonious, G. F., Turley, E. T., and Dawood, M. H. (2020). Monitoring soil enzymes activity before and after animal manure application. Agriculture 10 (5), 166. doi:10.3390/agriculture10050166
Banerjee, B., Aggarwal, P. K., Pathak, H., Singh, A. K., and Chaudhary, A. (2006). Dynamics of organic carbon and microbial biomass in alluvial soil with tillage and amendments in rice-wheat systems. Environ. Monit. Assess. 119 (1), 173–189. doi:10.1007/s10661-005-9021-8
Banik, P., and Sharma, R. C. (2008). Effects of integrated nutrient management with mulching on rice (Oryza sativa)-based cropping systems in rainfed eastern plateau area. Indian J. Agric. Sci. 78, 240.
Bhatt, M. K., Labanya, R., and Joshi, H. C. (2019). Influence of long-term chemical fertilizers and organic manures on soil fertility-A review. Univers. J. Agric. Res. 7 (5), 177–188. doi:10.13189/ujar.2019.070502
Bhattacharyya, P., Roy, K. S., Neogi, S., Adhya, T. K., Rao, K. S., and Manna, M. C. (2012). Effects of rice straw and nitrogen fertilization on greenhouse gas emissions and carbon storage in tropical flooded soil planted with rice. Soil Tillage Res. 124, 119–130. doi:10.1016/j.still.2012.05.015
Bhattacharya, P., Pramanik Maity, P., Mowrer, J., Maity, A., Rao, M., Das, S., et al. (2020). Assessment of soil health parameters and application of the sustainability index to fields under conservation agriculture for 3, 6, and 9 years in India. Heliyon 6 (12), e05640. doi:10.1016/j.heliyon.2020.e05640
Blake, G. R. (1965). “Bulk density,” in Methods of soil analysis: Part 1 physical and mineralogical properties including Statistics of measurement and sampling, 9, 374–390. Madison: American Society of Agronomy.
Blanco-Canqui, H., and Lal, R. (2008). No-tillage and soil-profile carbon sequestration: An on-farm assessment. Soil Sci. Soc. Am. J. 72 (3), 693–701. doi:10.2136/sssaj2007.0233
Blanco-Canqui, H., and Wortmann, C. S. (2020). Does occasional tillage undo the ecosystem services gained with no-till? A review. Soil Tillage Res. 198, 104534. doi:10.1016/j.still.2019.104534
Bouyoucos, G. J. (1927). The hydrometer as a new method for the mechanical analysis of soils. Soil Sci. 23 (5), 343–354. doi:10.1097/00010694-192705000-00002
Brown, M. W. (1973). A highly sensitive automated technique for the determination of ammonium nitrogen. J. Sci. Food Agric. 24 (9), 1119–1123. doi:10.1002/jsfa.2740240915
Budhar, M. N., Palaniappan, S. P., and Rangasamy, A. (1991). Effect of farm waste and green manures on low land rice. Indian J. Agron. 36 (2), 251–252.
Casida, L. E., Klein, D. A., and Santoro, T. (1964). Soil dehydrogenase activity. Soil Sci. 98 (6), 371–376. doi:10.1097/00010694-196412000-00004
Cassity-Duffey, K., Cabrera, M., Gaskin, J., Franklin, D., Kissel, D., and Saha, U. (2020). Nitrogen mineralization from organic materials and fertilizers: Predicting N release. Soil Sci. Soc. Am. J. 84 (2), 522–533. doi:10.1002/saj2.20037
Cataldo, D. A., Maroon, M., Schrader, L. E., and Youngs, V. L. (1975). Rapid colorimetric determination of nitrate in plant tissue by nitration of salicylic acid. Commun. soil Sci. plant analysis 6 (1), 71–80. doi:10.1080/00103627509366547
Catt, J. A., Howse, K. R., Christian, D. G., Lane, P. W., Harris, G. L., and Goss, M. J. (2000). Assessment of tillage strategies to decrease nitrate leaching in the Brimstone Farm Experiment, Oxfordshire, UK. Soil Tillage Res. 53 (3-4), 185–200. doi:10.1016/s0167-1987(99)00105-1
Černý, J., Balík, J., Pavlíková, D., Zitková, M., and Sýkora, K. (2003). The influence of organic and mineral nitrogen fertilizers on microbial biomass nitrogen and extractable organic nitrogen in long-term experiments with maize. Plant Soil Environ. 49 (12), 560–564. doi:10.17221/4194-pse
Chaitanya, T., Padmaja, G., Rao, P. C., and Devi, K. S. (2011). Effect of integrated nutrient management on soil dehydrogenase activity, nutrient uptake and fruit yield of tomato (Lycopersican esculentum L.). The Journal of Research ANGRAU 39 (4), 63–65.
Chandini, R. K., Kumar, R., and Om, P. (2019). The Impact of Chemical Fertilizers on our Environment and Ecosystem. In Research Trends in Environmental Sciences, 2nd Edition, 71–86.
Chiroma, A. M., Folorunso, O. A., and Alhassan, A. B. (2006). Soil water conservation, growth, yield and water use efficiency of sorghum as affected by land configuration and wood-shavings mulch in semi-arid northeast Nigeria. Exp. Agric. 42 (2), 199–216. doi:10.1017/s0014479705003376
Cooke, G. W. (1982). Fertilizing for maximum yield. Third Ed. English Language Book society/Collins, 457.
Corral-Fernández, R., Parras-Alcántara, L., and Lozano-García, B. (2013). Stratification ratio of soil organic C, N and C: N in mediterranean evergreen oak woodland with conventional and organic tillage. Agric. Ecosyst. Environ. 164, 252–259. doi:10.1016/j.agee.2012.11.002
Dai, Z., Hu, J., Fan, J., Fu, W., Wang, H., and Hao, M. (2021). No-tillage with mulching improves maize yield in dryland farming through regulating soil temperature, water and nitrate-N. Agric. Ecosyst. Environ. 309, 107288. doi:10.1016/j.agee.2020.107288
Dakora, F. D., and Phillips, D. A. (2002). Root exudates as mediators of mineral acquisition in low-nutrient environments. Plant Soil 245, 35. Food security in nutrient-stressed environments: exploiting plants’ genetic capabilities. doi:10.1023/A:1020809400075
De, M., Sá, J. C., Cerri, C. C., Dick, W. A., Lal, R., Filho, S. P. V., et al. (2001). Organic matter dynamics and carbon sequestration rates for a tillage chronosequence in a Brazilian Oxisol. Soil Sci. Soc. Am. J. 65 (5), 1486–1499. doi:10.2136/sssaj2001.6551486x
Debnath, M., Ray, S., and Nayak, D. K. (2016). Rural outmigration across agro-climatic regions in West Bengal. Hill Geogr. 32 (2), 59–75.
Deng, S. P., and Tabatabai, M. A. (1997). Effect of tillage and residue management on enzyme activities in soils: III. Phosphatases and arylsulfatase. Biol. Fertil. Soils 24 (2), 141–146. doi:10.1007/s003740050222
Diekow, J., Mielniczuk, J., Knicker, H., Bayer, C., Dick, D. P., and Kögel-Knabner, I. (2005). Soil C and N stocks as affected by cropping systems and nitrogen fertilisation in a southern Brazil Acrisol managed under no-tillage for 17 years. Soil Tillage Res. 81 (1), 87–95. doi:10.1016/j.still.2004.05.003
Dinesh, R., Dubey, R. P., Ganeshamurthy, A. N., and Prasad, G. S. (2000). Organic manuring in rice-based cropping system: effects on soil microbial biomass and selected enzyme activities. Curr. Sci. 79 (12), 1716–1720.
Dong, W., Zhang, X., Wang, H., Dai, X., Sun, X., Qiu, W., et al. (2012). Effect of different fertilizer application on the soil fertility of paddy soils in red soil region of southern China. PloS one 7, e44504. doi:10.1371/journal.pone.0044504
Feigin, A., and Halevy, J. (1989). “Irrigation-fertilisation-cropping management for maximum economic return and minimum pollution of ground water,” in Research report, inst. Soil water, ARO (Bet Dagan: The Volcani Center).
Fierer, N., Wood, S. A., and de Mesquita, C. P. B. (2021). How microbes can, and cannot, be used to assess soil health. Soil Biol. Biochem. 153, 108111. doi:10.1016/j.soilbio.2020.108111
Furczak, J., and Joniec, J. (2007). Changes in biochemical activity of podzolic soil under willow culture in the second year of treatment with municipal-industrial sewage sludge. Int. agrophysics 21 (2).
Gajda, A. M., Przewloka, B., and Gawryjolek, K. (2013). Changes in soil quality associated with tillage system applied. Int. Agrophysics 27 (2), 133–141. doi:10.2478/v10247-012-0078-7
Gathala, M. K., Ladha, J. K., Kumar, V., Saharawat, Y. S., Kumar, V., Sharma, P. K., et al. (2011). Tillage and crop establishment affects sustainability of South Asian rice–wheat system. Agron. J. 103 (4), 961–971. doi:10.2134/agronj2010.0394
Ghimire, S. R., and Craven, K. D. (2011). Enhancement of switchgrass (Panicum virgatum L.) biomass production under drought conditions by the ectomycorrhizal fungus Sebacina vermifera. Appl. Environ. Microbiol. 77 (19), 7063–7067. doi:10.1128/aem.05225-11
Haque, S., Patra, P. S., and Saha, A. (2012). Growth attributes, cob yield and economics of maize (Zea mays L.) cultivars as affected by integrated nutrient management under Terai region of West Bengal. Indian J. Agric. Res. 46 (1), 42–47.
Islam, S., Gathala, M. K., Tiwari, T. P., Timsina, J., Laing, A. M., Maharjan, S., et al. (2019). Conservation agriculture based sustainable intensification: increasing yields and water productivity for smallholders of the eastern gangetic plains. Field Crops Res. 238, 1–17. doi:10.1016/j.fcr.2019.04.005
Jackson, M. L. (1973). Soil chemical analysis, 498. New Delhi, India: pentice hall of India Pvt. Ltd., 151–154.
Jat, H. S., Choudhary, M., Datta, A., Yadav, A. K., Meena, M. D., Devi, R., et al. (2020). Temporal changes in soil microbial properties and nutrient dynamics under climate smart agriculture practices. Soil Tillage Res. 199, 104595. doi:10.1016/j.still.2020.104595
Jenkinson, D. S. (1988). “The Determination of Microbial Biomass Carbon and Nitrogen in Soil,” in Adv. nitrogen Cycl. Editor J. R. Wilson. Wallingford: CAB International, 368–386.
Kai, T., and Tamaki, M. (2020). Effect of organic and chemical fertilizer application on growth, yield, and soil biochemical properties of Landrace <i&gt;Brassica napus&lt;/i&gt; L. Leaf-and-Stem vegetable and Landrace (norabona). J. Agric. Chem. Environ. 9 (4), 314–330. doi:10.4236/jacen.2020.94023
Kanchikerimath, M., and Singh, D. (2001). Soil organic matter and biological properties after 26 years of maize–wheat–cowpea cropping as affected by manure and fertilization in a Cambisol in semiarid region of India. Agric. Ecosyst. Environ. 86 (2), 155–162. doi:10.1016/s0167-8809(00)00280-2
Karki, T. B., Kumar, A., and Gautam, R. C. (2005). Influence of integrated nutrient management on growth, yield, content and uptake of nutrients and soil fertility status in maize (Zea mays). Indian J. Agric. Sci. 75 (10), 682–685.
Kesavan, P. C., and Swaminathan, M. (2008). Strategies and models for agricultural sustainability in developing Asian countries. Phil. Trans. R. Soc. B 363 (1492), 877–891. doi:10.1098/rstb.2007.2189
Kiss, S., Drăgan-Bularda, M., and Rădulescu, D. (1975). Biological significance of enzymes accumulated in soil. Adv. Agron. 27, 25–87. doi:10.1016/s0065-2113(08)70007-5
Krauss, M., Berner, A., Perrochet, F., Frei, R., Niggli, U., and Mäder, P. (2020). Enhanced soil quality with reduced tillage and solid manures in organic farming–a synthesis of 15 years. Sci. Rep. 10 (1), 4403–4412. doi:10.1038/s41598-020-61320-8
Kukreja, K., Mishra, M. M., Dhankar, S. S., Kapoor, K. K., and Gupta, A. P. (1991). Effect of long term manurial application on microbial biomass. J. Indian Soc. soil Sci. 39, 685–688.
Kumar, R., Kumar, R., and Prakash, O. (2019). Chapter-5 the impact of chemical fertilizers on our environment and ecosystem. Chief Ed, 35, 69.
Liu, X., Herbert, S. J., Hashemi, A. M., Zhang, X., and Ding, G. (2006). Effects of agricultural management on soil organic matter and carbon transformation – a review. Plant Soil Environ. 52, 531–543. doi:10.17221/3544-pse
Lou, Y., Xu, M., Chen, X., He, X., and Zhao, K. (2012). Stratification of soil organic C, N and C: N ratio as affected by conservation tillage in two maize fields of China. Catena 95, 124–130. doi:10.1016/j.catena.2012.02.009
Malhi, S. S., Lemke, R., Wang, Z. H., and Chhabra, B. S. (2006). Tillage, nitrogen and crop residue effects on crop yield, nutrient uptake, soil quality, and greenhouse gas emissions. Soil Tillage Res. 90 (1-2), 171–183. doi:10.1016/j.still.2005.09.001
Mando, A., Ouattara, B., Sédogo, M., Stroosnijder, L., Ouattara, K., Brussaard, L., et al. (2005). Long-term effect of tillage and manure application on soil organic fractions and crop performance under Sudano-Sahelian conditions. Soil Tillage Res. 80 (1-2), 95–101. doi:10.1016/j.still.2004.03.002
Marzi, M., Shahbazi, K., Kharazi, N., and Rezaei, M. (2020). The influence of organic amendment source on carbon and nitrogen mineralization in different soils. J. Soil Sci. Plant Nutr. 20 (1), 177–191. doi:10.1007/s42729-019-00116-w
Meena, H. M., Sharma, R. P., Sankhyan, N. K., and Sepehya, S. (2017). Effect of continuous application of fertilizers, farmyard manure and lime on soil fertility and productivity of the maize-wheat system in an acid alfisol. Commun. Soil Sci. Plant Analysis 48 (13), 1552–1563. doi:10.1080/00103624.2017.1373800
Meena, M. D., and Biswas, D. R. (2014). Changes in biological properties in soil amended with rock phosphate and waste mica enriched compost using biological amendments and chemical fertilizers under wheat-soybean rotation. J. Plant Nutr. 37 (12), 2050–2073. doi:10.1080/01904167.2014.920368
Melero, S., Madejón, E., Herencia, J. F., and Ruiz, J. C. (2008). Effect of implementing organic farming on chemical and biochemical properties of an irrigated loam soil. Agron. J. 100 (1), 136–144. doi:10.2134/agrojnl2007.0087
Melero, S., Porras, J. C. R., Herencia, J. F., and Madejon, E. (2006). Chemical and biochemical properties in a silty loam soil under conventional and organic management. Soil Tillage Res. 90 (1-2), 162–170. doi:10.1016/j.still.2005.08.016
Mitra, B., Bhattacharya, P. M., Sinha, A. K., Chatterjee, R., and Chowdhury, A. K. (2020). Zero tillage Technology in Jute cultivation: a successful venture in West Bengal. Int. J. Curr. Microbiol. Appl. Sci. 9 (5), 2068–2075. doi:10.20546/ijcmas.2020.905.237
Mitran, T., Meena, R. S., Lal, R., Layek, J., Kumar, S., and Datta, R. (2018). “Role of soil phosphorus on legume production,” in Legumes for soil health and sustainable management (Singapore: Springer), 487–510.
Motavalli, P. P., Stevens, W. E., and Hartwig, G. (2003). Surface compaction and poultry litter effects on corn growth, nitrogen availability, and physical properties of a claypan soil. Soil and tillage research 71 (12), 121–131.
Motavalli, P. P., Stevens, W. E., and Hartwig, G. (2003). Remediation of subsoil compaction and compaction effects on corn N availability by deep tillage and application of poultry manure in a sandy-textured soil. Soil tillage Res. 71 (2), 121–131. doi:10.1016/s0167-1987(03)00041-2
Naderi, R., Edalat, M., and Kazemeini, S. A. (2016). Short-term responses of soil nutrients and corn yield to tillage and organic amendment. Archives Agron. Soil Sci. 62 (4), 570–579. doi:10.1080/03650340.2015.1066929
Nascente, A. S., Li, Y. C., and Crusciol, C. A. C. (2013). Cover crops and no-till effects on physical fractions of soil organic matter. Soil Tillage Res. 130, 52–57. doi:10.1016/j.still.2013.02.008
NBSS and LUP Classification (2001). Soil Series of West Bengal, 89. West Bengal: NBSS Publ. ISBN: 81-85460-66-3.
Nyamangara, J., Bergström, L. F., Piha, M. I., and Giller, K. E. (2003). Fertilizer use efficiency and nitrate leaching in a tropical sandy soil. J. Environ. Qual. 32 (2), 599–606. doi:10.2134/jeq2003.5990
Omara, P., Aula, L., Eickhoff, E. M., Dhillon, J. S., Lynch, T., Wehmeyer, G. B., et al. (2019). Influence of no-tillage on soil organic carbon, total soil nitrogen, and winter wheat (Triticum aestivum L.) grain yield. Int. J. Agron. 2019, 1–9. doi:10.1155/2019/9632969
Pathak, H., Chakrabarti, B., Mina, U., Pramanik, P., and Sharma, D. K. (2017). Ecosystem services of wheat (Triticum aestivum) production with conventional and conservation agricultural practices in the Indo-Gangetic Plains. Indian Journal of Agricultural Sciences 87 (8), 987–991. doi:10.1155/2019/9632969
Parthasarathi, K., Balamurugan, M., and Ranganathan, L. S. (2008). Influence of vermicompost on the physico-chemical and biological properties in different types of soil along with yield and quality of the pulse crop-blackgram. J. Environ. Health Sci. Eng. 5 (1), 51–58.
Perez-Brandán, C., Arzeno, J. L., Huidobro, J., Grümberg, B., Conforto, C., Hilton, S., et al. (2012). Long-term effect of tillage systems on soil microbiological, chemical and physical parameters and the incidence of charcoal rot by Macrophomina phaseolina (Tassi) Goid in soybean. Crop Prot. 40, 73–82. doi:10.1016/j.cropro.2012.04.018
Pernes-Debuyser, A., and Tessier, D. (2004). Soil physical properties affected by long-term fertilization. Eur. J. Soil Sci. 55 (3), 505–512. doi:10.1111/j.1365-2389.2004.00614.x
Pettit, N. M., Smith, A. R. J., Freedman, R. B., and Burns, R. G. (1976). Soil urease: activity, stability and kinetic properties. Soil Biol. Biochem. 8 (6), 479–484. doi:10.1016/0038-0717(76)90089-4
Power, J. F., Koerner, P. T., Doran, J. W., and Wilhelm, W. W. (1998). Residual effects of crop residues on grain production and selected soil properties. Soil Sci. Soc. Am. J. 62 (5), 1393–1397. doi:10.2136/sssaj1998.03615995006200050035x
Prager, K., and Posthumus, H. (2010). Socio-economic factors influencing farmers’ adoption of soil conservation practices in Europe. Hum. dimensions soil water conservation 12, 1–21.
Puget, P., and Lal, R. (2005). Soil organic carbon and nitrogen in a Mollisol in central Ohio as affected by tillage and land use. Soil tillage Res. 80 (1-2), 201–213. doi:10.1016/j.still.2004.03.018
Rakesh, S., Sarkar, D., Sinha, A. K., Danish, S., Bhattacharya, P. M., Mukhopadhyay, P., et al. (2021b). Soil organic carbon and labile and recalcitrant carbon fractions attributed by contrasting tillage and cropping systems in old and recent alluvial soils of subtropical eastern India. PLoS ONE 16 (12), e0259645. doi:10.1371/journal.pone.0259645
Rakesh, S., Sarkar, D., Sinha, A. K., Mukhopadhyay, P., Danish, S., Fahad, S., et al. (2021a). Carbon mineralization rates and kinetics of surface-applied and incorporated rice and maize residues in Entisol and Inceptisol soil types. Sustainability 13 (13), 7212–7216. doi:10.3390/su13137212
Rakesh, S., Sinha, A. K., and Mukhopadhyay, P. (2020). Vertical distribution of TOC, TN and other important soil attributes and their relationship in Alfisol and Entisol of West Bengal. Int. J. Environ. Clim. Chang. 10, 62–73. doi:10.9734/ijecc/2020/v10i130176
Ramamurthy, V., and Shivashankar, K. (1996). Residual effect of organic matter and phosphorus on growth, yield and quality of maize (Zea mays). Indian J. Agron. 41 (2), 247–251.
Ramesh, P., Panwar, N. R., Singh, A. B., and Ramana, S. (2008). Effect of organic manures on productivity, nutrient uptake and soil fertility of maize (Zea mays)-linseed (Linum usitatissimum) cropping system. Indian J. Agric. Sci. 78 (4), 351–354.
Rashidi, M., and Keshavarzpour, F. (2007). Effect of different tillage methods on soil physical properties and crop yield of watermelon (Citrullus vulgaris). ARPN J. Agric. Biol. Sci. 2 (6), 1–16.
Rathod, D. D., Rathod, P. H., Patel, K. P., and Patel, K. C. (2013). Integrated use of organic and inorganic inputs in wheat-fodder maize cropping sequence to improve crop yields and soil properties. Archives Agron. Soil Sci. 59 (11), 1439–1455. doi:10.1080/03650340.2012.724171
Roscoe, R., Vasconcellos, C. A., Furtini-Neto, A. E., Guedes, G. A., and Fernandes, L. A. (2000). Urease activity and its relation to soil organic matter, microbial biomass nitrogen and urea-nitrogen assimilation by maize in a Brazilian Oxisol under no-tillage and tillage systems. Biol. Fertil. soils 32 (1), 52–59. doi:10.1007/s003740000213
Sharma, S., Singh, P., and Kumar, S. (2020). Responses of soil carbon pools, enzymatic activity, and crop yields to nitrogen and straw incorporation in a rice-wheat cropping system in north-Western India. Front. Sustain. Food Syst. 4, 203. doi:10.3389/fsufs.2020.532704
Spedding, T. A., Hamel, C., Mehuys, G. R., and Madramootoo, C. A. (2004). Soil microbial dynamics in maize-growing soil under different tillage and residue management systems. Soil Biol. Biochem. 36 (3), 499–512. doi:10.1016/j.soilbio.2003.10.026
Sridevi, S., Ramana, M. V., and Rani, S. S. (2011). Soil enzyme activity and nutrient availability as influenced by different nutrient management practices in maize-onion cropping system. J. Res. ANGRAU 39 (3), 32–37.
Srinivasarao, Ch., Kundu, S., Yashavanth, B. S., Rakesh, S., Akbari, K. N., Sutaria, G. S., et al. (2020). Influence of 16 years of fertilization and manuring on carbon sequestration and agronomic productivity of groundnut in vertisol of semi-arid tropics of Western India. Carbon Manag. 12 (1), 1–12. doi:10.1080/17583004.2020.1858681
Tabatabai, M. A., and Bremner, J. M. (1972). Assay of urease activity in soils. Soil Biol. Biochem. 4 (4), 479–487. doi:10.1016/0038-0717(72)90064-8
Tabatabai, M. A., and Bremner, J. M. (1969). Use of p-nitrophenyl phosphate for assay of soil phosphatase activity. Soil Biol. Biochem. 1 (4), 301–307. doi:10.1016/0038-0717(69)90012-1
Takeda, M., Nakamoto, T., Miyazawa, K., Murayama, T., and Okada, H. (2009). Phosphorus availability and soil biological activity in an Andosol under compost application and winter cover cropping. Appl. Soil Ecol. 42 (2), 86–95. doi:10.1016/j.apsoil.2009.02.003
Tremblay, M. E., Nduwamungu, C., Parent, L. E., and Bolinder, M. A. (2010). Biological stability of carbon and nitrogen in organic products and crop residues using Fourier-Transform Near-Infrared reflectance spectroscopy. Commun. soil Sci. plant analysis 41 (8), 917–934. doi:10.1080/00103621003646014
Verma, G., and Mathur, A. K. (2009). Effect of integrated nutrient management on active pools of soil organic matter under maize-wheat system of a Typic Haplustept. J. Indian Soc. Soil Sci. 57 (3), 317–322.
Keywords: alternate tillage, conventional tillage, microbial biomass carbon, microbial biomass nitrogen, zero tillage
Citation: Sahoo S, Mukhopadhyay P, Mowrer J, Maity PP, Maity A, Sinha AK, Sow P and Rakesh S (2022) Tillage and N-source affect soil fertility, enzymatic activity, and crop yield in a maize–rice rotation system in the Indian Terai zone. Front. Environ. Sci. 10:983973. doi: 10.3389/fenvs.2022.983973
Received: 01 July 2022; Accepted: 13 October 2022;
Published: 01 December 2022.
Edited by:
Rahul Mahadev Shelake, Gyeongsang National University, South KoreaReviewed by:
Abhijit Sarkar, Indian Institute of Soil Science (ICAR), IndiaRajesh Ramdas Waghunde, Navsari Agricultural University, India
Copyright © 2022 Sahoo, Mukhopadhyay, Mowrer, Maity, Maity, Sinha, Sow and Rakesh. This is an open-access article distributed under the terms of the Creative Commons Attribution License (CC BY). The use, distribution or reproduction in other forums is permitted, provided the original author(s) and the copyright owner(s) are credited and that the original publication in this journal is cited, in accordance with accepted academic practice. No use, distribution or reproduction is permitted which does not comply with these terms.
*Correspondence: Pragati Pramanik Maity, cHJhZ2F0aS5pYXJpQGdtYWlsLmNvbQ==