- 1State Environmental Protection Key Laboratory of Food Chain Pollution Control, Beijing Technology and Business University, Beijing, China
- 2Key Laboratory of Cleaner Production and Integrated Resource Utilization of China National Light Industry, Beijing Technology and Business University, Beijing, China
- 3School of Ecology and Environment, Beijing Technology and Business University, Beijing, China
1 Introduction
Polycyclic aromatic hydrocarbons (PAHs) are carcinogenic and/or mutagenic chemicals found in the environment (Yang et al., 2017; Cheng et al., 2019). They can be produced from a variety of emission sources, including natural sources such as volcanic eruptions, forestfires, and diagenesis (Ali, 2019; Bao et al., 2019; Deelaman et al., 2021); anthropogenic sources, for example, traffic sources, domestic heating, industrial processes, and biomass burning; and some unique sources such as shipping emissions and firework display (Tobiszewski and Namiesnik, 2012; Pongpiachan et al., 2015; Abdel-Shafy and Mansour, 2016; Pongpiachan et al., 2017), in which the incomplete combustion of residential solid fuel is the main source of contribution (Shen et al., 2012; Škrbić et al., 2018; Li Z. et al., 2019). More than 40% of households worldwide were reported to rely on solid fuels for cooking and heating, and the proportions were higher in rural areas of developing countries (Zhang and Tao, 2009; Bonjour et al., 2013; Duan et al., 2014; Sun et al., 2018; Du et al., 2021). In rural areas without pollution control measurements, higher PAH emission factors (EFs) have been achieved in domestic cooking and heating systems than in industrial combustion processes with pollution control systems (Oanh et al., 1999; Sun et al., 2018a). Residential solid fuel combustion has been found to contribute to almost half of the total global PAH emissions (Shen et al., 2013). Low-efficiency solid fuel combustion in residential areas strongly pollutes both the ambient air and indoor environment (Gustafson et al., 2008; Lv et al., 2009), inducing many adverse health problems for people inhaling polluted indoor and outdoor air (Lin et al., 2016; Shen, 2017; Janta et al., 2020; Li et al., 2020). Numerous studies have indicated that exposure to PAHs might lead to metabolic disorders and gastrointestinal cancers (Yang et al., 2015; Bhardwaj et al., 2019; Apiratikul et al., 2020). Kong et al. (2018) conducted field tests in Jiangsu, China, pointing out that inhalation of PAHs emitted from domestic coal and biomass combustion is associated with high potential cancer risk. The study by Du et al. (2018a) also showed that the incremental lifetime cancer risk of indoor PAH inhalation using residential solid fuels in northern China might be as high as 2.3 × 10−4, far exceeding the acceptable level of 10−6. Growing attention is therefore being paid to PAH emissions caused by residential solid fuel combustion in rural areas (Secrest et al., 2016; Li et al., 2017; Li et al., 2018; Marchetti et al., 2019).
The main solid fuels burned in residential areas are bituminous coal, charcoal, crop residues, and wood (Sun et al., 2018a; Kalisa et al., 2019). Bonjour et al. (2013) found that crop residues are the most commonly used solid fuels for cooking and heating in rural households around the world. As the largest developing country and a leading agricultural country, China produced 803.2 × 106 t of crop residues in 2009 and possibly larger amounts in recent years (Song et al., 2014; Yang et al., 2016). Crop residues are important solid fuels in residences in China. Many studies of PAH emissions from coal and biomass combustion have been published in recent decades (Tao et al., 2006; Xu et al., 2006; Yang et al., 2010; Singh et al., 2013; Chen et al., 2015; Wang et al., 2018). Some studies were focused on PAH emissions during crop residue burning (Shen et al., 2011, 2012, 2013a, 2013b; Yang et al., 2017). At present, most combustion experiments are being carried out in laboratory test and field measurement (Wei et al., 2014; Shen et al., 2016; Du et al., 2018b, 2020). Due to many uncontrollable factors, the EFs of PAHs measured in the field measurements are usually higher and have great variations (Shen et al., 2011,; Yang et al., 2017; Sun et al., 2018b). Shen et al. (2011) determined PAH emissions when nine types of crop residues commonly used were burned in a typical cooking stove in rural areas in China. The mean total PAH EFs were 63 ± 37 mg kg−1 in the range of 27–142 mg kg−1. Shen et al. (2013b) tested that the EF of 28 PAHs was 13 ± 4.8 mg kg−1 for poplar branch combustion in a typical brick cooking stove. Zhang et al. (2021) observed much higher PAH EFs (ranged from 736 to 1033 mg/kg) in typical solid fuel combustions in rural Guanzhong Plain, China. Nowadays, there are huge gaps in data availability, as well as regional differences between different fuel types and stoves (Du et al., 2020). Therefore, it is necessary to fully obtain the EFs of PAHs in different real-world fuel-stove combinations and spatial differences.
In this study, PAH emissions were investigated when crop residues were burned in domestic stoves in rural China. The objectives were 1) to measure the PAH emissions when different crop residues are burned in traditional and improved stoves and 2) to evaluate the carcinogenic risks induced by PAH emissions from traditional and improved stoves burning different crop residues. The study was expected to improve the data available on crop residue combustion in rural areas and decrease uncertainties in PAH emission inventories. The data were expected to enable PAH emissions from domestic stoves in rural China to be estimated and carcinogenic risk assessments to be performed.
2 Materials and Methods
2.1 Field Site, Fuels, and Stoves
The study was performed in two rural areas of northern China (see Supplementary Figure S1). One location was in Huairou, Beijing (40.45°N, 116.70°E), and the other was in Cangzhou, Hebei Province (37.95°N, 116.86°E). These two areas are similar to most rural areas in China, where crops and trees are widely planted, resulting in a large amount of crop residues. Due to the undeveloped infrastructure and lack of central heating, local residents of these areas mainly use biomass stoves for their daily cooking and heating requirements. Thus, the resultant air pollution and health problems cannot be ignored.
Six commonly used crop residues (apricot branches, chestnut branches, corn cobs, corn straw, cotton straw, and poplar branches) were used in the emission tests. The C, H, N, O, and S contents were determined by using an element analyzer (vario EL cube, Elementar, Germany). In the CHNS mode, the temperatures of furnaces ranging from 1 to 3 are 1,150, 850, and 0°C, respectively. In O mode, the temperature of furnace 1 is 1,150°C, and the temperatures of furnaces 2 and 3 are 0°C. Each crop residue was tested three times.
Two types of typically self-built adobe stoves (a traditional stove and an improved stove), which are widely used for domestic cooking and heating in rural China, were used. The traditional stove was 40 cm high with one chamber, a 1.1 m tall chimney with a column diameter of 0.1 m without grates. The improved stove was 60 cm high with a chamber, a 2.3 m tall chimney with a column diameter of 0.3 m and a grate. The main difference between the improved and traditional stove is the grate, which can improve the ventilation, leading to complete combustion. A total of 20 stoves were measured (five traditional stoves and five improved stoves in Huairou, Beijing; four traditional stoves and six improved stoves in Cangzhou, Hebei Province). The two types of stoves are shown in Supplementary Figure S2. Sample information and the fuel properties are shown in Table 1.
2.2 Combustion Experiments and the Sampling System
The samples were collected during normal cooking, and the local residents were required to conduct fuel combustion activities as usual. Sampling covered the entire combustion process from ignition to burnout. Each test lasted for about 20–30 min. The crop residues were weighed to calculate the weight loss by combustion before each combustion test and the ash remaining in the stove after a combustion process. All samplings were repeated twice.
The sampling system is shown in Figure 1; there are five parts, a sampling pipe to collect flue gases from the stove chimney, a hot-box for heating the flue gas to avoid condensation, a mixing room to dilute the flue gases with dry and filtered air, a PM2.5 cyclone to remove the particles with diameters >2.5 μm from the flue gases (at a flow rate of 16.7 L min−1), and a sample-collection unit with three channels. One channel is to collect the gaseous and particulate-bound PAHs using quartz filters (QF, 47 mm in diameters, 2500QAT-UP, PALL Corp., United States) and three polyurethane foam (PUF, 22 mm diameter × 7.6 cm, Restek Technology Co., Ltd., China) cartridges in series, respectively. The flue gases were passed through the QF and then the three PUF cartridges (to avoid the breakthrough phenomena). There was a flow meter and pump at the end of the sampling train, after the PUF cartridges. The flow rate was ∼7 L min−1. The second channel is to collect PM2.5 used for elemental and organic carbon (EC and OC) analyses using a teflon filter (47 mm in diameters, PALL Corp., United States) and a QF, and the flow rate was ∼5 L min−1. The last one is parallel sampling of channel 2 using two QFs with the flow rate of approximately 5 L min−1. The dilution ratio was approximately 10 during the tests. Another probe placed at the center of the chimney was connected to a flue gas analyzer (Ratnoze 3, Mountain Air Engineering, United States), which can quantify the carbon dioxide (CO2) and carbon monoxide (CO) concentrations. The flue gas analyzer was calibrated before each test using a gas standard. The average flue gas velocity was at the level of 1.6 ± 0.7 m s−1 for traditional stoves and 1.9 ± 0.3 m s−1 for improved stoves. There was no obvious difference between the temperature of flue gas for the traditional and improved stoves, and the average temperature at the sampling points was 9.0 ± 3.4°C.
2.3 Extraction and Analysis
Gravimetric filtration was used to determine the PM mass measurement. The masses of PM2.5 were measured by the particles on teflon filters (TFs) that were weighed using a microbalance (MT5, Mettler Toledo, Switzerland). The TFs were preconditioned at 650°C for 8 h to remove the organic contaminants. Each TF was cut a 1.5 cm2 circle to determine the OC and EC using an OCEC analyzer (Model 5 L, Sunset Laboratory) with IMPROVE A method.
PAH sample extraction and analysis methods referred to previous studies (Cao et al., 2017). QFs and PUFs were purified, concentrated, and then analyzed using an Agilent 1290 high-performance liquid chromatography system (Agilent Technologies, Santa Clara, CA, United States) with an Agilent G1314A UV detector. A total of 16 U.S. EPA priority PAHs were quantified (the substances and abbreviations are shown in Table 2). All the samples were added with a mixed standard (naphthalene-D8, acenaphthene-D10, phenanthrene-D10, fluoranthene-D10, and chrysene-D12, J&W Chemical, United States) and were quantitatively analyzed by the internal standard method. Detailed information can be found in Supplementary Material S1.
2.4 Data Analysis
2.4.1 Calculation of EFs
The carbon mass balance method was used to calculate the emission factors (EFs). For this method, it was assumed that all of the carbon in a fuel was released as gases [CO2, CO, and total hydrocarbons (THC)] and particles [organic carbon (OC) and elemental carbon (EC)] during combustion (Dhammapala et al., 2007). The details on the calculation process can be found in Supplementary Material S2.
2.4.2 Carcinogenic Risk Assessment
The carcinogenic risks induced by PAHs are generally expressed as benzo[a]pyrene (BaP) toxic equivalents (TEQBaP) (Nisbet and LaGoy 1992; Apiratikul et al., 2020, 2021; Deelaman et al., 2020a, 2020b, 2021). The toxic equivalents of each PAH (TEQi) were equal to the concentration of each PAH in the sample (Ci), multiplied by its toxic equivalency factor (TEFi). The equation used to calculate the TEQBaP is shown in Eq. 1:
The values of TEFi are based on the study by Nisbet and LaGoy. (1992). More details were described in Hao et al. (2018). TEF values are listed in Supplementary Table S1.
2.5 Statistical Analysis
Statistical analysis was performed by Statistical Package for the Social Sciences version 19.0 software (IBM, Armonk, NY, United States). Statistically significant differences were identified by performing the analysis of variance (ANOVA) and t-test using a significance level of 0.05.
2.6 QA/QC
PUFs were extracted three times with dichloromethane by ultrasonication for 30 min before use. QFs were baked at 550°C in a muffle furnace for 12 h before use. The field blanks were analyzed and used to correct the concentration of samples. The standard reference material, “Urban-Dust, SRM 1649b” [U.S. National Institute of Standards and Technology (NIST), MD, United States] was determined to verify the recovery of the extraction method and the accuracy and precision of the analysis method. The determined concentrations were within acceptable value ranges according to SRM 1649b. The recovery percentages ranged from 76.0 ± 15.7 (Ant) to 107.4 ± 18.2 (DBahA). The relative standard deviations (n = 5) for all the target compounds were <5%. The detection and quantification limits were calculated as the concentrations correspond to 3- and 10-times the standard deviation of the blank noise, respectively. The PAH detection limits ranged from 0.4 ng/ml (for Phe) to 9.1 ng/ml (for Ant). The quantification limits for the 16 PAHs ranged from 3.2 (for Phe) to 30.3 ng/ml (for Ant). Relevant method validation data are shown in Supplementary Tables S2, S3.
3 Results and Discussion
3.1 PAH EFs
The EFs of PAH isomers from six crop residues and two stove types are listed in Supplementary Table S4. Figure 2 shows the EFs of16 PAHs (gas + PM2.5 bound) and the fraction of particles of 16 PAHs in PM2.5 and OC in six fuels and two stoves. The EFs of total 16 PAHs ranged from 49.4 mg kg−1 (for TAB) to 160.5 mg kg−1 (for TPB) for traditional stoves and 26.8 mg kg−1 (for IAB) to 104.9 mg kg−1 (for IPB) for improved stoves, respectively. The EFs of total 16 PAHs in improved stoves were significantly reduced compared with those of traditional stoves, resulting in an average decrease of 45.1%. The good air supply in the improved stoves decreased incomplete combustion, decreasing the amounts of PAHs formed (Sun et al., 2018a). The PAH EFs for apricot branches, chestnut branches, corn cobs, and corn straw were similar. Cotton straw and poplar branches produced larger amounts of PAHs than the other crop residues. Singh et al. (2013) also found a high total PAH EF for cotton straw. However, Sun et al. (2018b) found much lower organic carbon EFs for ligneous plants (e.g., pine and poplar) than herbaceous plants (e.g., corn cobs and cotton straw). The high PAH EF for poplar branches was probably caused by the low combustion temperature used, the moisture content of the poplar branches, and the air supply used (Lu et al., 2009).
As shown in Figure 2, higher PAH EFs were found for the particulate phase than the gas phase. In addition to TCT and ICT, the particulate phase PAHs accounted for 89.3% of the total PAHs averagely, which may be caused by the high content of high molecular weight (HMW) PAHs in the particles. The combustion process in rural households can increase the content and composition of HMW PAHs, which is a common indicator of solid fuel combustion (Yan et al., 2006; Li Y. et al., 2019). High particulate phase PAH contributions to total PAH emissions were also achieved by Yang et al. (2017) and Singh et al. (2013), and particulate phase PAHs were found to respectively contribute to ∼75.5% and ∼87.6% of the total PAH emissions from various crop residues. Figure 2 also shows the mass fractions of the EFs of particulate phase PAHs to PM2.5 and OC, which ranged from 0.5% to 8.2% (for PM2.5) and from 1.1% to 13.6% (for OC), respectively. The results were comparable with those of Sun et al. (2018a).
A negative correlation was observed between the PAH EFs and MCE, as previously widely reported (p < 0.05, shown in Figure 3) (Shen et al., 2013; Du et al., 2021). The PAH EFs were positively correlated with the EFs of CO (p < 0.05) since both were the products of incomplete combustions. Similarly, the EFs of particulate phase PAHs were also positively correlated with the fraction of particles of PAHs in PM2.5 and OC, respectively (p < 0.05). Increased PAH emissions from stove combustions are often accompanied by higher PM2.5 and OC emissions, indicating the higher PAHs emissions and the higher potential toxicity of particles (Du et al., 2020, 2021).
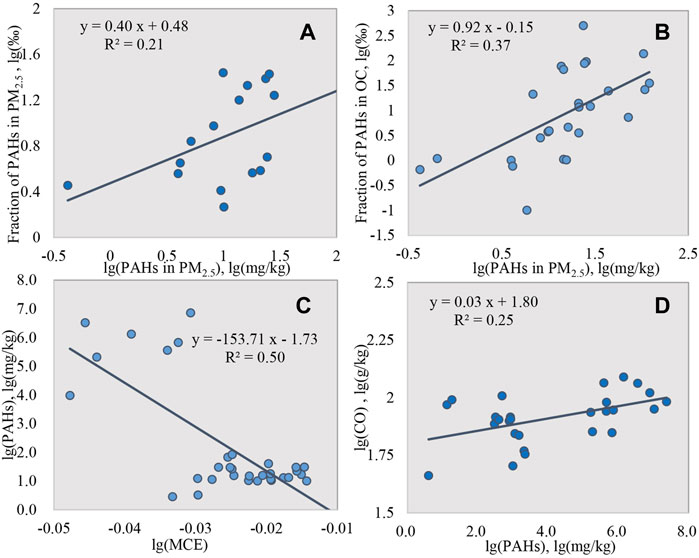
FIGURE 3. Correlations between PAH EFs and other EFs (data were log transformed). (A) Correlations between EFs of particulate phase PAHs (EFparticulate PAHs) and the fraction of EFparticulate PAHs in PM2.5; (B) Correlations between EFparticulate PAHs and the fraction of EFparticulate PAHs in OC; (C) Correlations between PAH EFs and MCE; (D) Correlations between EFs of PAHs and CO.
In this study, the mean gas phase, particulate phase, and total PAH EFs were 18.9 ± 24.2, 63.2 ± 43.3, and 82.1 ± 38.5 mg kg−1, respectively, for the traditional stoves and 9.6 ± 15.9, 38.0 ± 29.1, and 47.6 ± 29.8 mg kg−1, respectively, for the improved stoves. The PAH EFs between the traditional stoves and improved stoves were significantly different according to the t-test (p < 0.05). However, the EFs of PAHs have large variations in previous studies (as shown in Figure 4). For example, our results agreed with the investigation from Yang et al. (2017), in which the gas phase, particulate phase, and total PAH EFs for peanut hulls were 10.77, 33.1, and 43.87 mg kg−1, respectively, for an improved stove. Wiriya et al. (2016) found the EF of the total PAH from the burning of maize residue was only 0.5 ± 0.1 mg/kg in a laboratory chamber, which was three orders of magnitude lower than our results. However, Zhang et al. (2021) observed much higher PAH EFs (ranged from 736 to 1033 mg/kg) in typical solid fuel combustions in rural Guanzhong Plain, China, which were one order of magnitude higher than our results and four orders of magnitude higher than the results by Wiriya et al. (2016). It has been reported that the results of the laboratory test are different from those in real field measurements (Oanh et al., 2005; Shen et al., 2013; Du et al., 2018b) because the laboratory tests are usually performed under well-controlled conditions and the field measurements are subjected to many uncontrollable factors. In addition, there are also large variations among different field measurements (Shen et al., 2013c; Sun et al., 2017; Zhang Y. et al., 2021; Zhang et al., 2021). Shen et al. (2011) found the gas phase, particulate phase, and total PAH EFs of 27 ± 13, 35 ± 23, and 62 ± 35 mg kg−1, respectively, for nine commonly used crop residues burned in a cooking stove typically used in rural areas in China. Singh et al. (2013) found the gas phase, particulate phase, and total PAH EFs of 5.09, 35.84, and 40.93 mg kg−1, respectively, for the crop residue burning in rural households in India. Our results were higher than the EFs from Shen et al. (2011), Singh et al. (2013), and Shen et al. (2013b) but lower than the total PAH EFs of 141–276 mg kg−1 for a traditional stove found by Shen et al. (2013a). These differences are presumably affected by many factors, including sampling methods, type of fuels, the stove’s architecture, and user operation (Wielgosiński et al., 2017; Zhao et al., 2020). The sampling systems were different although they had the same field measurement in rural household as previous studies. Shen et al. (2011, 2013b) used the direct sampling method, in which the flue gas in the chimney is directly obtained through the sampling pipe without dilution, whereas Singh et al. (2013) and this study used a dilution unit to cool down the diluted flue gas before sampling. In order to avoid the influence of high temperature flue gas on sampling, the direct sampling method needs to keep the sampling system including the filter membrane at a specific temperature, while this problem can be addressed by diluting and cooling the high temperature flue gas. However, the dilution rate, dilution ratio, and temperature of dilution gas will directly influence the quality and particle size distribution of particulate matter; the distribution variation of volatile organic compounds induced by dilution will also have a direct impact on the ratio of OC to EC in particles. Therefore, it is important to control the dilution rate and ratios and monitor the temperature and humidity of dilution gas (Lipsky and Robinson, 2006; Rode et al., 2006). Furthermore, various stoves with different architecture were determined in these studies. It was found that chimneys play an important role in stove performance by influencing the draft, while the errors in the design impact the ideal air/fuel ratio (Praoas et al., 2014). In addition, these differences may also have been affected by many factors, including the type of fuels (with different properties, e.g., fuel moisture, volatile matter content, and heating values), combustion conditions (e.g., temperature and air flow), and user operation (Wielgosiński et al., 2017; Zhao et al., 2020).
3.2 PAH Profiles
The PAH profiles (the gas and particulate phase) from six crop residues and two stove types are shown in Supplementary Table S5. The results indicated that the type of stove affected the PAH profiles little. The PAH profiles for the apricot branch, chestnut branch, corn cob, corn straw, and poplar branch combustion were similar but being different for the profiles for cotton straw combustion.
The percentages of PAH isomers for all the samples are presented in Figure 5. Phe and Flu were the dominant gas phase PAHs, contributing to, respectively, 22.9% and 13.9% of the total PAH concentrations averagely. BbF and BaP were the major contributors to the particulate phase and total emissions, together contributing to 28.7%–31.8% and 24.8%–26.7% of the total PAH concentrations in the particulate phase and total emissions, respectively. Organic compounds with low molecular weight are usually found at higher concentrations in the gas phase than the particulate phase; organic compounds with high molecular weight are usually found under higher concentrations in the particulate phase than in the gas phase. The actual proportions of a compound found in the gas and particulate phases are related to the polarity and vapor pressure of the compound (Shen et al., 2016). Similar to the results for the PAH isomers, no significant differences were achieved between the distributions separated by the number of rings for different stoves and crop residues (p > 0.05) (Figure 6). The three- and four-ring PAHs were the dominant gas phase PAHs, together accounting for 54.6%–77.8% of the total PAH concentrations. The three-ring PAHs were the most abundant, contributing 44.1% of the total PAH concentrations. These results agreed with the previous study from Yang et al. (2017). Four- and five-ring PAHs were dominant in both the particulate phase and total emissions, contributing to 72.8%–95.5% together, of both the particulate phase and total emissions except for cotton straw. Five-ring PAHs were the most abundant, which contributed to 71.7% of the particulate phase and total emissions. Similar results were found by Lai et al. (2009), which revealed that four-ring PAHs strongly contributed to the total PAH concentrations in the gas and particulate phases but that PAHs in the particulate phase were dominated by PAHs with more than five rings.
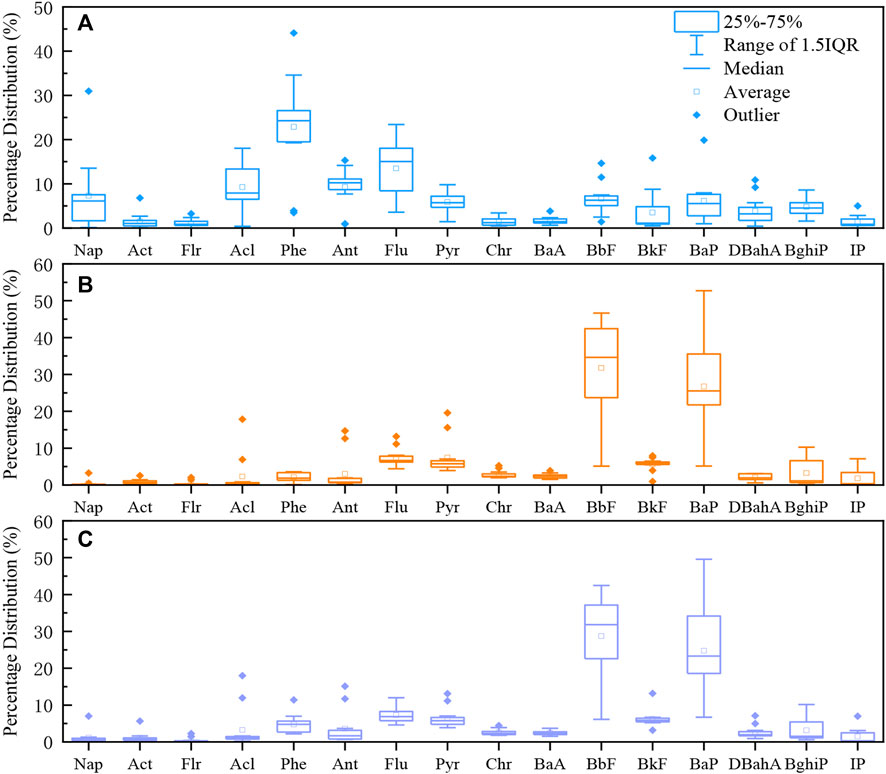
FIGURE 5. Percentages of PAH isomers for all the samples. (A) gas phase samples; (B) particulate phase samples; (C) total emission samples.
The ratios between different PAH concentrations are often used to identify sources of PAHs in environmental media (Yunker et al., 2002; Katsoyiannis et al., 2007; Galarneau, 2008; Ladji et al., 2009). The diagnostic ratios Ant/(Ant + Phe), Flu/(Flu + Pyr), BaA/(BaA + Chr), BbF/(BbF + BkF), BaP/(BaP + Chr), and IP/(IP + BghiP) calculated in this study and previous studies are summarized in Table 3. Hays et al. (2005) found distinct variation of PAH isomer ratios from crop residue burning in clean and traditional stoves. However, no significant differences in the ratios of the improved and traditional stoves are achieved in this study. Except cotton straw, the PAH isomer ratios of other five crop residues are mostly similar. It is suggested that Ant/(Ant + Phe) larger than 0.1 originates from combustion (Škrbić et al., 2017); the ratio of Flu/(Flu + Pyr) greater than 0.5 arises from biomass and coal combustion (Yunker et al., 2002). In this study, the values of these ratios fell in the range for biomass and coal combustion. The ratios derived in this study are generally comparable to those of crop residue burning in stoves, chambers, or open fire and wood in stoves reported by other studies (Table 3). It could be demonstrated that there were significant differences in the calculated ratios between crop residue burning in this study and coal combustion. The measured ratios were also remarkably different with those from diesel or gasoline engines. However, it is worth noting that there are some ratios with conflicting results. The IP/(IP + BghiP) ratio is employed to distinguish coal (> 0.5), biomass burning (0.2–0.5), and traffic and petrogenic (< 0.2) (Yunker et al., 2002). In this study, the ratios of IP/(IP + BghiP) for the traditional stove and improved stove were 0.25 ± 0.08 and 0.15 ± 0.11, respectively. Contradictory results were also obtained by Chen et al. (2005) and Zhang et al. (2008b), in which IP/(IP + BghiP) for coal combustion were 0.33 and 0.35, respectively. Moreover, the ratio of BbF/(BbF + BkF) less than 0.4 indicated biomass burning (Tobiszewski and Namiesnik, 2012), while the value in this study was much higher than 0.4. Large variations of PAH isomeric ratios may be related to the widely varied fuel properties and combustion conditions (Fang et al., 2004; Yang et al., 2017). In addition, the aging and degradation of PAHs in aerosols emitted from primary sources during the transmission process from the source to sampling medium will cause the change in the diagnostic ratio (Zhang et al., 2015). On the other hand, it is confirmed that the PAH diagnostic ratios have not only intrasource variability but also have intersource similarity (Galarneau, 2008). For example, the ratio of Flu/(Flu + Pyr) for diesel emissions was in the range of 0.6–0.7 (Schauer et al., 1999; Yang et al., 2010), while that for coal combustion has a similar range of 0.32–0.7 (Chen et al., 2005; Oanh et al., 2005; Zhang et al., 2008a). The BaA/(BaA + Chr) ratio of gasoline emissions was 0.2–0.6 (Khalili et al., 1995) similar to coal emissions with the ratio of 0.27–0.56 (Chen et al., 2005; Oanh et al., 2005; Zhang et al., 2008a). Hence, although the diagnostic ratios have been widely used in source apportionment, they should be used with caution (Tobiszewski and Namiesnik, 2012; Pongpiachan et al., 2017), and multiple diagnostic ratios should be combined to characterize source apportionment.
3.3 Carcinogenic Risk Assessment for the PAHs
The estimated emissions of carcinogenic risks posed by PAH are shown in Table 4. The toxic equivalents (TEQ) for the sums of seven carcinogenic PAHs (BaA, Chr, BbF, BkF, BaP, IP, and DBahA) (∑PAH7), BaP (TEQBaP), and PAHs (TEQPAHs) were calculated.
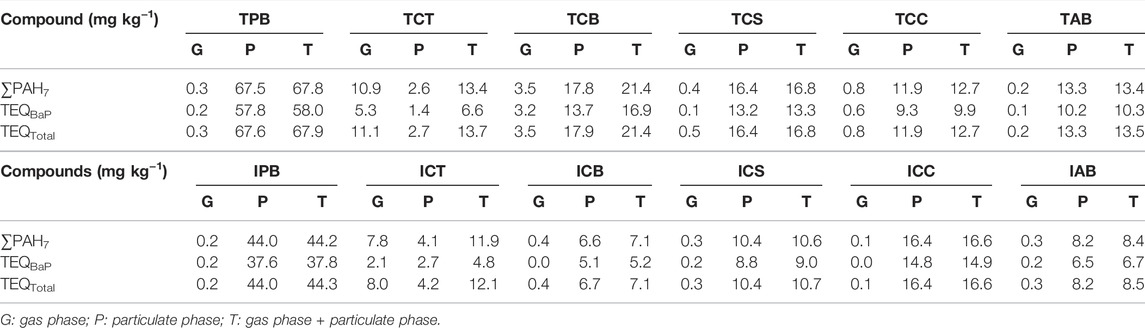
TABLE 4. Carcinogenic risks posed by PAHs emitted from crop residues burning in two types of stoves.
∑PAH7, TEQBaP, and TEQPAHs for the gas phase, particulate phase, and total emissions generally followed similar trends. The ∑PAH7, TEQBaP, and TEQPAHs were higher for cotton straw than the other crop residues in the gas phase, but these were much higher for the polar branch than the other crop residues in the particulate phase and total emissions. The ∑PAH7, TEQBaP, and TEQPAHs were much higher for the particulate phase than the gas phase, and the particulate phase TEQPAHs accounted for ∼91.2% of the total emission TEQPAHs. Most of the high-molecular weight PAHs (containing four rings or more), which are more toxic than the low-molecular weight PAHs, were emitted in the particulate phase. The mean ∑PAH7, TEQBaP, and total emission TEQPAHs were 24.3, 19.2, and 24.4 mg kg−1, respectively, for the traditional stoves and 16.5, 13.1, and 16.5 mg kg−1, respectively, for the improved stoves. The mean PAH carcinogenic risk EFs were >30% lower for the improved stoves than the traditional stoves. The ∑PAH7 was the main contributor to the TEQPAHs; in particular, TEQBaP dominated a mean of ∼78.1% to the TEQPAHs.
4 Conclusion
Emissions of 16 PAHs were measured when six types of crop residues were burned in the traditional and improved stoves used in rural China. Compared with the traditional stoves, the EFs of PAHs in the improved stoves reduced significantly, with an average reduction of 45.1%. Good air supply in the improved stoves decreases incomplete combustion, decreasing the amounts of PAHs emitted. Higher PAH EFs were generally observed in the particulate phase than the gas phase. Negative correlation was observed between PAH EFs and MCE, and the EFs of CO were positively correlated with the PAH EFs. Similarly, the EFs of PAHs in PM2.5 were also positively correlated with the fraction of particles of PAHs in PM2.5 and OC. The study was expected for the supplement of the data available on crop residue combustion in domestic stoves in rural areas, decreasing the uncertainties in PAH emission inventories.
The carcinogenic risks induced by PAH emissions were calculated when crop residues were burned in the stoves. The mean PAH carcinogenic risk EFs were >30%, which was lower for the improved stoves than the traditional stoves. The TEQPAHs were dominated by the ∑PAH7, particularly BaP (which contributed ∼78.1% of the TEQPAHs averagely). These data are expected to provide more basic foundation for the PAH carcinogenic risk assessment of rural household stoves in China.
In fact, there are still several limitations to this study. First, some influencing factors have not been deeply considered and discussed, such as, the impact of cooking oil fumes was not assessed, which may lead to the overestimation of PAH emissions. Second, the dynamic changes of PAH emissions during the combustion process are complex, and the dynamic evolution mechanism of PAH formation has not been well studied. Finally, only two sites are covered in this study, and there is limited discussion on PAH spatiotemporal distributions. Larger sample sizes and more field measurements are needed in the future to improve our understanding of PAH formation mechanisms and actual emissions during residential fuel combustions.
Data Availability Statement
The original contributions presented in the study are included in the article/Supplementary Material, further inquiries can be directed to the corresponding author.
Author Contributions
XS, XY, and ZY contributed to the design of the study. QZ, SF, and PW implemented the experiments and data analysis. XH drafted the article. XC and XL revised the original draft.
Funding
Funding for this study was provided through the School Level Cultivation Fund of Beijing Technology and Business University for Distinguished and Excellent Young Scholars (No. BTBUYP 2020) and the Young Teachers Scientific Research Ability Promotion Program of the Beijing Technology and Business University (grant no. PXM 2019_014213_000007).
Conflict of Interest
The authors declare that the research was conducted in the absence of any commercial or financial relationships that could be construed as a potential conflict of interest.
Publisher’s Note
All claims expressed in this article are solely those of the authors and do not necessarily represent those of their affiliated organizations, or those of the publisher, the editors, and the reviewers. Any product that may be evaluated in this article, or claim that may be made by its manufacturer, is not guaranteed or endorsed by the publisher.
Supplementary Material
The Supplementary Material for this article can be found online at: https://www.frontiersin.org/articles/10.3389/fenvs.2022.883576/full#supplementary-material
References
Abdel-Shafy, H. I., and Mansour, M. S. M. (2016). A Review on Polycyclic Aromatic Hydrocarbons: Source, Environmental Impact, Effect on Human Health and Remediation. Egypt. J. Pet. 25, 107–123. doi:10.1016/j.ejpe.2015.03.011
Ali, N. (2019). Polycyclic Aromatic Hydrocarbons (PAHs) in Indoor Air and Dust Samples of Different Saudi Microenvironments; Health and Carcinogenic Risk Assessment for the General Population. Sci. Total Environ. 696, 133995. doi:10.1016/j.scitotenv.2019.133995
Apiratikul, R., Pongpiachan, S., and Deelaman, W. (2021). Spatial Distribution, Sources and Quantitative Human Health Risk Assessments of Polycyclic Aromatic Hydrocarbons in Urban and Suburban Soils of Chile. Environ. Geochem. Health 43, 2851–2870. doi:10.1007/s10653-020-00798-7
Apiratikul, R., Pongpiachan, S., and Hashmi, M. Z. (2020). Health Risk Assessment of Polycyclic Aromatic Hydrocarbons in Coastal Soils of Koh Samed Island (Thailand) after the Oil Spill Incident in 2013. Mar. Pollut. Bull. 150, 110736. doi:10.1016/j.marpolbul.2019.110736
Bao, K., Zaccone, C., Tao, Y., Wang, J., Shen, J., and Zhang, Y. (2020). Source Apportionment of Priority PAHs in 11 lake Sediment Cores from Songnen Plain, Northeast China. Water Res. 168, 115158. doi:10.1016/j.watres.2019.115158
Bonjour, S., Adair-Rohani, H., Wolf, J., Bruce, N. G., Mehta, S., Prüss-Ustün, A., et al. (2013). Solid Fuel Use for Household Cooking: Country and Regional Estimates for 1980-2010. Environ. Health Perspect. 121, 784–790. doi:10.1289/ehp.1205987
Cao, X., Hao, X., Shen, X., Jiang, X., Wu, B., and Yao, Z. (2017). Emission Characteristics of Polycyclic Aromatic Hydrocarbons and nitro-polycyclic Aromatic Hydrocarbons from Diesel Trucks Based on On-Road Measurements. Atmos. Environ. 148, 190–196. doi:10.1016/j.atmosenv.2016.10.040
Chen, Y., Sheng, G., Bi, X., Feng, Y., Mai, B., and Fu, J. (2005). Emission Factors for Carbonaceous Particles and Polycyclic Aromatic Hydrocarbons from Residential Coal Combustion in China. Environ. Sci. Technol. 39, 1861–1867. doi:10.1021/es0493650
Chen, Y., Zhi, G., Feng, Y., Chongguo Tian, C. G., Bi, X., Li, J., et al. (2015). Increase in Polycyclic Aromatic Hydrocarbon (PAH) Emissions Due to Briquetting: A challenge to the Coal Briquetting Policy. Environ. Pollut. 204, 58–63. doi:10.1016/j.envpol.2015.04.012
Cheng, Y., Kong, S., Yan, Q., Liu, H., Wang, W., Chen, K., et al. (2019). Size-segregated Emission Factors and Health Risks of PAHs from Residential Coal Flaming/smoldering Combustion. Environ. Sci. Pollut. Res. 26, 31793–31803. doi:10.1007/s11356-019-06340-2
Deelaman, W., Pongpiachan, S., Tipmanee, D., Choochuay, C., Iadtem, N., Suttinun, O., et al. (2020a). Source Identification of Polycyclic Aromatic Hydrocarbons in Terrestrial Soils in Chile. J. South Am. Earth Sci. 99, 102514. doi:10.1016/j.jsames.2020.102514
Deelaman, W., Pongpiachan, S., Tipmanee, D., Choochuay, C., Suttinun, O., Charoenkalunyuta, T., et al. (2021). Ecotoxicological Risk and Health Risk Characterization of Polycyclic Aromatic Hydrocarbons (PAHs) in Terrestrial Soils of King George Island, Antarctica. Polar Sci. 29, 100715. doi:10.1016/j.polar.2021.100715
Deelaman, W., Pongpiachan, S., Tipmanee, D., Suttinun, O., Choochuay, C., Iadtem, N., et al. (2020b). Source Apportionment of Polycyclic Aromatic Hydrocarbons in the Terrestrial Soils of King George Island, Antarctica. J. South Am. Earth Sci. 104, 102832. doi:10.1016/j.jsames.2020.102832
Dhammapala, R., Claiborn, C., Simpson, C., and Jimenez, J. (2007). Emission Factors from Wheat and Kentucky Bluegrass Stubble Burning: Comparison of Field and Simulated Burn Experiments. Atmos. Environ. 41, 1512–1520. doi:10.1016/j.atmosenv.2006.10.008
Du, W., Chen, Y., Zhu, X., Zhong, Q., Zhuo, S., Liu, W., et al. (2018a). Wintertime Air Pollution and Health Risk Assessment of Inhalation Exposure to Polycyclic Aromatic Hydrocarbons in Rural China. Atmos. Environ. 191, 1–8. doi:10.1016/j.atmosenv.2018.07.054
Du, W., Wang, J., Zhuo, S., Zhong, Q., Wang, W., Chen, Y., et al. (2021). Emissions of Particulate PAHs from Solid Fuel Combustion in Indoor Cookstoves. Sci. Total Environ. 771, 145411. doi:10.1016/j.scitotenv.2021.145411
Du, W., Yun, X., Chen, Y., Zhong, Q., Wang, W., Wang, L., et al. (2020). PAHs Emissions from Residential Biomass Burning in Real-World Cooking Stoves in Rural China. Environ. Pollut. 267, 115592. doi:10.1016/j.envpol.2020.115592
Du, W., Zhu, X., Chen, Y., Liu, W., Wang, W., Shen, G., et al. (2018b). Field-based Emission Measurements of Biomass Burning in Typical Chinese Built-In-Place Stoves. Environ. Pollut. 242, 1587–1597. doi:10.1016/j.envpol.2018.07.121
Fang, G., Wu, Y. S., Chen, M. H., Ho, T. T., Huang, S. H., and Rau, J. Y. (2004). Polycyclic Aromatic Hydrocarbons Study in Taichung, Taiwan, during 2002?2003. Atmos. Environ. 38, 3385–3391. doi:10.1016/j.atmosenv.2004.03.036
Galarneau, E. (2008). Source Specificity and Atmospheric Processing of Airborne PAHs: Implications for Source Apportionment. Atmos. Environ. 42, 8139–8149. doi:10.1016/j.atmosenv.2008.07.025
Guerrero, F., Yáñez, K., Vidal, V., and Cereceda-Balic, F. (2019). Effects of wood Moisture on Emission Factors for PM2.5, Particle Numbers and Particulate-phase PAHs from Eucalyptus Globulus Combustion Using a Controlled Combustion Chamber for Emissions. Sci. Total Environ. 648, 737–744. doi:10.1016/j.scitotenv.2018.08.057
Gustafson, P., Östman, C., and Sällsten, G. (2008). Indoor Levels of Polycyclic Aromatic Hydrocarbons in Homes with or without wood Burning for Heating. Environ. Sci. Technol. 42, 5074–5080. doi:10.1021/es800304y
Hays, M. D., Fine, P. M., Geron, C. D., Kleeman, M. J., and Gullett, B. K. (2005). Open Burning of Agricultural Biomass: Physical and Chemical Properties of Particle-phase Emissions. Atmos. Environ. 39, 6747–6764. doi:10.1016/j.atmosenv.2005.07.072
Janta, R., Sekiguchi, K., Yamaguchi, R., Sopajaree, K., Pongpiachan, S., and Chetiyanukornkul, T. (2020). Ambient PM2.5, Polycyclic Aromatic Hydrocarbons and Biomass Burning Tracer in Mae Sot District, Western Thailand. Atmos. Pollut. Res. 11, 27–39. doi:10.1016/j.apr.2019.09.003
Jenkins, B. M., Daniel Jones, A., Turn, S. Q., and Williams, R. B. (1996b). Particle Concentrations, Gas-Particle Partitioning, and Species Intercorrelations for Polycyclic Aromatic Hydrocarbons (PAH) Emitted during Biomass Burning. Atmos. Environ. 30, 3825–3835. doi:10.1016/1352-2310(96)00084-2
Jenkins, B. M., Jones, A. D., Turn, S. Q., and Williams, R. B. (1996a). Emission Factors for Polycyclic Aromatic Hydrocarbons from Biomass Burning. Environ. Sci. Technol. 30, 2462–2469. doi:10.1021/es950699m
Kalisa, E., Nagato, E., Bizuru, E., Lee, K., Tang, N., Pointing, S., et al. (2019). Pollution Characteristics and Risk Assessment of Ambient PM2.5-bound PAHs and NPAHs in Typical Japanese and New Zealand Cities and Rural Sites. Atmos. Pollut. Res. 10, 1396–1403. doi:10.1016/j.apr.2019.03.009
Katsoyiannis, A., Terzi, E., and Cai, Q.-Y. (2007). On the Use of PAH Molecular Diagnostic Ratios in Sewage Sludge for the Understanding of the PAH Sources. Is This Use Appropriate? Chemosphere 69, 1337–1339. doi:10.1016/j.chemosphere.2007.05.084
Keshtkar, H., and Ashbaugh, L. L. (2007). Size Distribution of Polycyclic Aromatic Hydrocarbon Particulate Emission Factors from Agricultural Burning. Atmos. Environ. 41, 2729–2739. doi:10.1016/j.atmosenv.2006.11.043
Khalili, N. R., Scheff, P. A., and Holsen, T. M. (1995). PAH Source Fingerprints for Coke Ovens, Diesel and, Gasoline Engines, Highway Tunnels, and wood Combustion Emissionsfingerprints for Coke Ovens, Diesel and Gasoline Engines, Highway Tunnels, and wood Combustion Emissions. Atmos. Environ. 29, 533–542. doi:10.1016/1352-2310(94)00275-P
Kim Oanh, N. T., Albina, D. O., Ping, L., and Wang, X. (2005). Emission of Particulate Matter and Polycyclic Aromatic Hydrocarbons from Select Cookstove-Fuel Systems in Asia. Biomass and Bioenergy 28, 579–590. doi:10.1016/j.biombioe.2005.01.003
Kim Oanh, N. T., Bætz Reutergårdh, L., and Dung, N. T. (1999). Emission of Polycyclic Aromatic Hydrocarbons and Particulate Matter from Domestic Combustion of Selected Fuels. Environ. Sci. Technol. 33, 2703–2709. doi:10.1021/es980853f
Kong, S., Yan, Q., Zheng, H., Liu, H., Wang, W., Zheng, S., et al. (2018). Substantial Reductions in Ambient PAHs Pollution and Lives Saved as a Co-benefit of Effective Long-Term PM2.5 Pollution Controls. Environ. Int. 114, 266–279. doi:10.1016/j.envint.2018.03.002
Ladji, R., Yassaa, N., Balducci, C., Cecinato, A., and Meklati, B. Y. (2009). Annual Variation of Particulate Organic Compounds in PM10 in the Urban Atmosphere of Algiers. Atmos. Res. 92, 258–269. doi:10.1016/j.atmosres.2008.12.002
Lai, C.-H., Chen, K.-S., and Wang, H.-K. (2009). Influence of rice Straw Burning on the Levels of Polycyclic Aromatic Hydrocarbons in Agricultural County of Taiwan. J. Environ. Sci. 21, 1200–1207. doi:10.1016/S1001-0742(08)62404-3
Li, Q., Jiang, J., Wang, S., Rumchev, K., Mead-Hunter, R., Morawska, L., et al. (2017). Impacts of Household Coal and Biomass Combustion on Indoor and Ambient Air Quality in China: Current Status and Implication. Sci. Total Environ. 576, 347–361. doi:10.1016/j.scitotenv.2016.10.080
Li, Y., Xu, H., Wang, J., Ho, S. S. H., He, K., Shen, Z., et al. (2019a). Personal Exposure to PM2.5-bound Organic Species from Domestic Solid Fuel Combustion in Rural Guanzhong Basin, China: Characteristics and Health Implication. Chemosphere 227, 53–62. doi:10.1016/j.chemosphere.2019.04.010
Li, Z., Fan, L., Wang, L., Ma, H., Hu, Y., Jiang, Y., et al. (2018). PAH Profiles of Emitted Ashes from Indoor Biomass Burning across the Beijing-Tianjin-Hebei Region and Implications on Source Identification. Aerosol Air Qual. Res. 18, 749–761. doi:10.4209/aaqr.2017.12.0588
Li, Z., Wang, Y., Guo, S., Li, Z., Xing, Y., Liu, G., et al. (2019b). PM2.5 Associated PAHs and Inorganic Elements from Combustion of Biomass, cable Wrapping, Domestic Waste, and Garbage for Power Generation. Aerosol Air Qual. Res. 19, 2502–2517. doi:10.4209/aaqr.2019.10.0495
Li, Z., Wang, Y., Li, Z., Guo, S., and Hu, Y. (2020). Levels and Sources of PM2.5-associated PAHs during and after the Wheat Harvest in a central Rural Area of the Beijing-Tianjin-Hebei (BTH) Region. Aerosol Air Qual. Res. 20, 1070–1082. doi:10.4209/aaqr.2020.03.0083
Lin, N., Chen, Y., Du, W., Shen, G., Zhu, X., Huang, T., et al. (2016). Inhalation Exposure and Risk of Polycyclic Aromatic Hydrocarbons (PAHs) Among the Rural Population Adopting wood Gasifier Stoves Compared to Different Fuel-Stove Users. Atmos. Environ. 147, 485–491. doi:10.1016/j.atmosenv.2016.10.033
Lipsky, E. M., and Robinson, A. L. (2006). Effects of Dilution on fine Particle Mass and Partitioning of Semivolatile Organics in Diesel Exhaust and wood Smoke. Environ. Sci. Technol. 40, 155–162. doi:10.1021/es050319p
Lu, H., Zhu, L., and Zhu, N. (2009). Polycyclic Aromatic Hydrocarbon Emission from Straw Burning and the Influence of Combustion Parameters. Atmos. Environ. 43, 978–983. doi:10.1016/j.atmosenv.2008.10.022
Lv, J., Xu, R., Wu, G., Zhang, Q., Li, Y., Wang, P., et al. (2009). Indoor and Outdoor Air Pollution of Polycyclic Aromatic Hydrocarbons (PAHs) in Xuanwei and Fuyuan, China. J. Environ. Monit. 11, 1368–1374. doi:10.1039/b900382g
Marchetti, S., Longhin, E., Bengalli, R., Avino, P., Stabile, L., Buonanno, G., et al. (2019). In Vitro lung Toxicity of Indoor PM10 from a Stove Fueled with Different Biomasses. Sci. Total Environ. 649, 1422–1433. doi:10.1016/j.scitotenv.2018.08.249
Nisbet, I. C. T., and LaGoy, P. K. (1992). Toxic Equivalency Factors (TEFs) for Polycyclic Aromatic Hydrocarbons (PAHs). Regul. Toxicol. Pharmacol. 16 (3), 290–300. doi:10.1016/0273-2300(92)90009-X
Pongpiachan, S., Hattayanone, M., Choochuay, C., Mekmok, R., Wuttijak, N., and Ketratanakul, A. (2015). Enhanced PM10 Bounded PAHs from Shipping Emissions. Atmos. Environ. 108, 13–19. doi:10.1016/j.atmosenv.2015.02.072
Pongpiachan, S., Hattayanone, M., Suttinun, O., Khumsup, C., Kittikoon, I., Hirunyatrakul, P., et al. (2017). Assessing Human Exposure to PM 10 -bound Polycyclic Aromatic Hydrocarbons during Fireworks Displays. Atmos. Pollut. Res. 8 (5), 816–827. doi:10.1016/j.apr.2017.01.014
Prapas, J., Baumgardner, M. E., Marchese, A. J., Willson, B., and Defoort, M. (2014). Influence of Chimneys on Combustion Characteristics of Buoyantly Driven Biomass Stovesfluence of Chimneys on Combustion Characteristics of Buoyantly Driven Biomass Stoves. Energ. Sust. Dev. 23, 286–293. doi:10.1016/j.esd.2014.08.007
Roden, C. A., Bond, T. C., Conway, S., and Pinel, A. B. O. (2006). Emission Factors and Real-Time Optical Properties of Particles Emitted from Traditional Wood Burning Cookstoves. Environ. Sci. Technol. 40, 6750–6757. doi:10.1021/es052080i
Schauer, J. J., Kleeman, M. J., Cass, G. R., and Simoneit, B. R. T. (1999). Measurement of Emissions from Air Pollution Sources. 2. C1 through C30 Organic Compounds from Medium Duty Diesel Trucks. Environ. Sci. Technol. 33, 1578–1587. doi:10.1021/es980081n
Secrest, M. H., Schauer, J. J., Carter, E. M., Lai, A. M., Wang, Y., Shan, M., et al. (2016). The Oxidative Potential of PM2.5 Exposures from Indoor and Outdoor Sources in Rural China. Sci. Total Environ. 571, 1477–1489. doi:10.1016/j.scitotenv.2016.06.231
Sheesley, R. J., Schauer, J. J., Chowdhury, Z., Cass, G. R., and Simoneit, B. R. T. (2003). Characterization of Organic Aerosols Emitted from the Combustion of Biomass Indigenous to South Asia. J. Geophys. Res. 108, a–n. doi:10.1029/2002JD002981
Shen, G., Chen, Y., Du, W., Lin, N., Wang, X., Cheng, H., et al. (2016). Exposure and Size Distribution of Nitrated and Oxygenated Polycyclic Aromatic Hydrocarbons Among the Population Using Different Household Fuels. Environ. Pollut. 216, 935–942. doi:10.1016/j.envpol.2016.07.002
Shen, G. (2017). Mutagenicity of Particle Emissions from Solid Fuel Cookstoves: A Literature Review and Research Perspective. Environ. Res. 156, 761–769. doi:10.1016/j.envres.2017.05.001
Shen, G., Tao, S., Wei, S., Chen, Y., Zhang, Y., Shen, H., et al. (2013a). Field Measurement of Emission Factors of PM, EC, OC, Parent, Nitro-, and Oxy- Polycyclic Aromatic Hydrocarbons for Residential Briquette, Coal Cake, and Wood in Rural Shanxi, China. Environ. Sci. Technol. 47 (6), 2998–3005. doi:10.1021/es304599g
Shen, G., Tao, S., Wei, S., Zhang, Y., Wang, R., Wang, B., et al. (2012). Emissions of Parent, nitro, and Oxygenated Polycyclic Aromatic Hydrocarbons from Residential wood Combustion in Rural China. Environ. Sci. Technol. 46 (15), 8123–8130. doi:10.1021/es301146v
Shen, G., Wang, W., Yang, Y., Ding, J., Xue, M., Min, Y., et al. (2011). Emissions of PAHs from Indoor Crop Residue Burning in a Typical Rural Stove: Emission Factors, Size Distributions, and Gas−Particle Partitioning. Environ. Sci. Technol. 45 (4), 1206–1212. doi:10.1021/es102151w
Shen, G., Wei, S., Zhang, Y., Wang, B., Wang, R., Shen, H., et al. (2013c).Emission and Size Distribution of Particle-Bound Polycyclic Aromatic Hydrocarbons from Residential wood Combustion in Rural ChinaPollutant Emissions from Improved Coal- and wood-fuelled Cookstoves in Rural Households. Biomass and Bioenergy, 5549, 1416590–1476598. doi:10.1016/j.biombioe.2013.01.031Shen10.1021/es506343z
Shen, G., Xue, M., Wei, S., Chen, Y., Zhao, Q., Li, B., et al. (2013b). Influence of Fuel Moisture, Charge Size, Feeding Rate and Air Ventilation Conditions on the Emissions of PM, OC, EC, Parent PAHs, and Their Derivatives from Residential wood Combustion. J. Environ. Sci. 25 (9), 1808–1816. doi:10.1016/S1001-0742(12)60258-7
Shen, H., Huang, Y., Wang, R., Zhu, D., Li, W., Shen, G., et al. (2013). Global Atmospheric Emissions of Polycyclic Aromatic Hydrocarbons from 1960 to 2008 and Future Predictions. Environ. Sci. Technol. 47, 6415–6424. doi:10.1021/es400857z
Singh, D. P., Gadi, R., Mandal, T. K., Saud, T., Saxena, M., and Sharma, S. K. (2013). Emissions Estimates of PAH from Biomass Fuels Used in Rural Sector of Indo-Gangetic Plains of India. Atmos. Environ. 68, 120–126. doi:10.1016/j.atmosenv.2012.11.042
Škrbić, B. D., Đurišić-Mladenović, N., Tadić, Đ. J., and Cvejanov, J. Đ. (2017). Polycyclic Aromatic Hydrocarbons in Urban Soil of Novi Sad, Serbia: Occurrence and Cancer Risk Assessment. Environ. Sci. Pollut. Res. 24, 16148–16159. doi:10.1007/s11356-017-9194-z
Škrbić, B., Marinković, V., Spaić, S., Milanko, V., and Branovački, S. (2018). Profiles of Polycyclic Aromatic Hydrocarbons in Smoke from Combustion and thermal Decomposition of poplar wood Pellets and Sawdust. Microchemical J. 139, 9–17. doi:10.1016/j.microc.2018.02.007
Song, G., Che, L., Yang, Y., Lyakurwa, F., and Zhang, S. (2014). Estimation of Crop Residue in China Based on a Monte Carlo Analysis. Chin. J. Popul. Resour. Environ. 12 (1), 88–94. doi:10.1080/10042857.2014.886759
Sun, J., Shen, Z., Cao, J., Zhang, L., Wu, T., Zhang, Q., et al. (2017). Particulate Matters Emitted from maize Straw Burning for winter Heating in Rural Areas in Guanzhong Plain, China: Current Emission and Future Reduction. Atmos. Res. 184, 66–76. doi:10.1016/j.atmosres.2016.10.006
Sun, J., Shen, Z., Zeng, Y., Niu, X., Wang, J., Cao, J., et al. (2018a). Characterization and Cytotoxicity of PAHs in PM2.5 Emitted from Residential Solid Fuel Burning in the Guanzhong Plain, China. Environ. Pollut. 241, 359–368. doi:10.1016/j.envpol.2018.05.076
Sun, J., Zhi, G., Jin, W., Chen, Y., Shen, G., Tian, C., et al. (2018b). Emission Factors of Organic Carbon and Elemental Carbon for Residential Coal and Biomass Fuels in China- A New Database for 39 Fuel-Stove Combinations. Atmos. Environ. 190, 241–248. doi:10.1016/j.atmosenv.2018.07.032
Tao, S., Li, X., Yang, Y., Coveney, R. M., Lu, X., Chen, H., et al. (2006). Dispersion Modeling of Polycyclic Aromatic Hydrocarbons from Combustion of Biomass and Fossil Fuels and Production of Coke in Tianjin, China. Environ. Sci. Technol. 40, 4586–4591. doi:10.1021/es060220y
Tobiszewski, M., and Namieśnik, J. (2012). PAH Diagnostic Ratios for the Identification of Pollution Emission Sources. Environ. Pollut. 162, 110–119. doi:10.1016/j.envpol.2011.10.025
Wang, R., Liu, G., Sun, R., Yousaf, B., Wang, J., Liu, R., et al. (2018). Emission Characteristics for Gaseous- and Size-Segregated Particulate PAHs in Coal Combustion Flue Gas from Circulating Fluidized Bed (CFB) Boiler. Environ. Pollut. 238, 581–589. doi:10.1016/j.envpol.2018.03.051
Wei, S., Shen, G., Zhang, Y., Xue, M., Xie, H., Lin, P., et al. (2014). Field Measurement on the Emissions of PM, OC, EC and PAHs from Indoor Crop Straw Burning in Rural China. Environ. Pollut. 184, 18–24. doi:10.1016/j.envpol.2013.07.036
Wielgosiński, G., Łechtańska, P., and Namiecińska, O. (2017). Emission of Some Pollutants from Biomass Combustion in Comparison to Hard Coal Combustion. J. Energ. Inst. 90, 787–796. doi:10.1016/j.joei.2016.06.005
Wiriya, W., Sillapapiromsuk, S., Lin, N.-H., and Chantara, S. (2016). Emission Profiles of PM10-Bound Polycyclic Aromatic Hydrocarbons from Biomass Burning Determined in Chamber for Assessment of Air Pollutants from Open Burning. Aerosol Air Qual. Res. 16, 2716–2727. doi:10.4209/aaqr.2015.04.0278
Xu, S., Liu, W., and Tao, S. (2006). Emission of Polycyclic Aromatic Hydrocarbons in China. Environ. Sci. Technol. 40, 702–708. doi:10.1021/es0517062
Yang, X.-Y., Igarashi, K., Tang, N., Lin, J.-M., Wang, W., Kameda, T., et al. (2010). Indirect- and Direct-Acting Mutagenicity of Diesel, Coal and wood Burning-Derived Particulates and Contribution of Polycyclic Aromatic Hydrocarbons and Nitropolycyclic Aromatic Hydrocarbons. Mutat. Research/Genetic Toxicol. Environ. Mutagenesis 695, 29–34. doi:10.1016/j.mrgentox.2009.10.010
Yang, X., Geng, C., Sun, X., Yang, W., Wang, X., and Chen, J. (2016). Characteristics of Particulate-Bound Polycyclic Aromatic Hydrocarbons Emitted from Industrial Grade Biomass Boilers. J. Environ. Sci. 40, 28–34. doi:10.1016/j.jes.2015.09.010
Yang, X., Liu, S., Xu, Y., Liu, Y., Chen, L., Tang, N., et al. (2017). Emission Factors of Polycyclic and nitro-polycyclic Aromatic Hydrocarbons from Residential Combustion of Coal and Crop Residue Pellets. Environ. Pollut. 231 (2), 1265–1273. doi:10.1016/j.envpol.2017.08.087
Yunker, M. B., Macdonald, R. W., Vingarzan, R., Mitchell, R. H., Goyette, D., and Sylvestre, S. (2002). PAHs in the Fraser River basin: a Critical Appraisal of PAH Ratios as Indicators of PAH Source and Composition. Org. Geochem. 33, 489–515. doi:10.1016/S0146-6380(02)00002-5
Zhang, B., Sun, J., Jiang, N., Zeng, Y., Zhang, Y., He, K., et al. (2021). Emission Factors, Characteristics, and Gas-Particle Partitioning of Polycyclic Aromatic Hydrocarbons in PM2.5 Emitted for the Typical Solid Fuel Combustions in Rural Guanzhong Plain, China. Environ. Pollut. 286, 117573. doi:10.1016/j.envpol.2021.117573
Zhang, Y., Dou, H., Chang, B., Wei, Z., Qiu, W., Liu, S., et al. (2008a). Emission of Polycyclic Aromatic Hydrocarbons from Indoor Straw Burning and Emission Inventory Updating in China. Ann. NY Acad. Sci. 1140, 218–227. doi:10.1196/annals.1454.006
Zhang, Y., Schauer, J. J., Zhang, Y., Zeng, L., Wei, Y., Liu, Y., et al. (2008b). Characteristics of Particulate Carbon Emissions from Real-World Chinese Coal Combustion. Environ. Sci. Technol. 42, 5068–5073. doi:10.1021/es7022576
Zhang, Y., Shen, Z., Sun, J., Zhang, L., Zhang, B., Zhang, T., et al. (2020). Parent, Alkylated, Oxygenated and nitro Polycyclic Aromatic Hydrocarbons from Raw Coal Chunks and Clean Coal Combustion: Emission Factors, Source Profiles, and Health Risks. Sci. Total Environ. 721, 137696. doi:10.1016/j.scitotenv.2020.137696
Zhang, Y., Sun, J., Zou, H., Zhang, B., Yang, D., Wang, Q., et al. (2021a). Photochemical Aging Process on PM2.5 Bound PAHs Emission from Solid Fuel Combustion in Traditional and Improved Stoves. Atmos. Res. 263, 105807. doi:10.1016/j.atmosres.2021.105807
Zhang, Y., and Tao, S. (2009). Global Atmospheric Emission Inventory of Polycyclic Aromatic Hydrocarbons (PAHs) for 2004. Atmos. Environ. 43, 812–819. doi:10.1016/j.atmosenv.2008.10.050
Zhang, Y., Zhang, H., Luan, S., Zeng, L., He, L., Huang, X., et al. (2015). Emission Characteristics of PAHs from Open and Residential Biomass Burning. China Environ. Sci. 35, 387–395. (in Chinese).
Keywords: PAHs, domestic stoves, emission factors, carcinogenic risk, rural China
Citation: Hao X, Zhao Q, Shen X, Cao X, Feng S, Li X, Yao X, Wang P and Yao Z (2022) Emissions of PAHs From Crop Residues Burning in Domestic Stoves in Rural China. Front. Environ. Sci. 10:883576. doi: 10.3389/fenvs.2022.883576
Received: 25 February 2022; Accepted: 11 April 2022;
Published: 18 May 2022.
Edited by:
Peter S. Hooda, Kingston University, United KingdomReviewed by:
Lav Kumar Kaushik, Gujarat Power Engineering & Research Institute (GPERI), IndiaSiwatt Pongpiachan, National Institute of Development Administration, Thailand
Copyright © 2022 Hao, Zhao, Shen, Cao, Feng, Li, Yao, Wang and Yao. This is an open-access article distributed under the terms of the Creative Commons Attribution License (CC BY). The use, distribution or reproduction in other forums is permitted, provided the original author(s) and the copyright owner(s) are credited and that the original publication in this journal is cited, in accordance with accepted academic practice. No use, distribution or reproduction is permitted which does not comply with these terms.
*Correspondence: Zhiliang Yao, eWFvemhsQHRoLmJ0YnUuZWR1LmNu