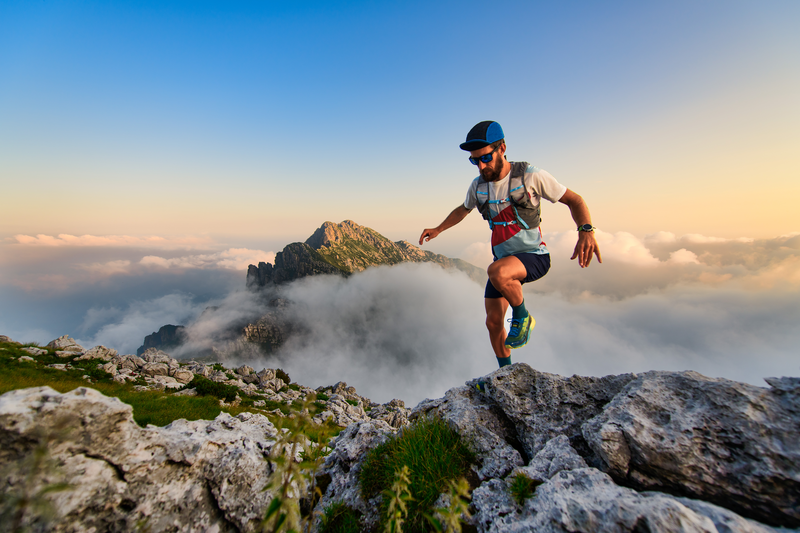
95% of researchers rate our articles as excellent or good
Learn more about the work of our research integrity team to safeguard the quality of each article we publish.
Find out more
ORIGINAL RESEARCH article
Front. Environ. Sci. , 17 March 2022
Sec. Toxicology, Pollution and the Environment
Volume 10 - 2022 | https://doi.org/10.3389/fenvs.2022.843855
This article is part of the Research Topic Novel Insights into Mercury Sources and Behavior in the Surface Earth Environment View all 5 articles
Mercury pollution in the surface ocean has more than doubled over the past century. Within oceanic food webs, sea turtles have life history characteristics that make them especially vulnerable to mercury (Hg) accumulation. In this study we investigated Hg concentrations in the skin and carapace of nesting flatback turtles (Natator depressus) from two rookeries in Western Australia. A total of 50 skin samples and 52 carapace samples were collected from nesting turtles at Thevenard Island, and 23 skin and 28 carapace samples from nesting turtles at Eighty Mile Beach. We tested the influence of turtle size on Hg concentrations, hypothesising that larger and likely older adult turtles would exhibit higher concentrations due to more prolonged exposure to Hg. We compared the rookeries, hypothesising that the turtles from the southern rookery (Thevenard Island) were more likely to forage and reside in the Pilbara region closer to industrial mining activity and loading ports (potential exposure to higher environmental Hg concentrations) with turtles from the northern rookery (Eighty Mile Beach) more likely to reside and feed in the remote Kimberley. Turtles from the Eighty Mile Beach rookery had significantly higher skin Hg concentrations (x̄ = 19.4 ± 4.8 ng/g) than turtles from Thevenard Island (x̄ = 15.2 ± 5.8 ng/g). There was no significant difference in carapace Hg concentrations in turtles between Eighty Mile Beach (x̄ = 48.4 ± 21.8 ng/g) and Thevenard Island (x̄ = 41.3 ± 16.5 ng/g). Turtle size did not explain Hg concentrations in skin samples from Eighty Mile Beach and Thevenard Island, but turtle size explained 43.1% of Hg concentrations in the carapace of turtles from Eighty Mile Beach and 44.2% from Thevenard Island. Mercury concentrations in the flatback turtles sampled in this study are relatively low compared to other sea turtles worldwide, likely a result of the generally low concentrations of Hg in the Australian environment. Although we predicted that mining activities would influence flatback turtle Hg bioaccumulations, our data did not support this effect. This may be a result of foraging ground overlap between the two rookeries, or the predominant wind direction carrying atmospheric Hg inland rather than seaward. This is the first Hg study in skin and carapace of flatback turtles and represents a baseline to compare Hg contamination in Australia’s surrounding oceans.
Mercury (Hg) pollution has a long legacy in the environment. Once emitted to the atmosphere, it can cycle between ecosystems for years or decades before ending up deep in the oceans or deposited on land (Obrist et al., 2018). As a result, 83% of the Hg concentrations in the surface ocean are from anthropogenic enrichment, half of which have occurred since 1950 (Amos et al., 2013). Mercury concentrations in the ocean therefore represent a substantial fraction of the global Hg reservoir (Soerensen et al., 2010) and explain the ever-increasing levels of Hg contamination observed in marine organisms (Alava et al., 2018, 2017).
Once Hg enters the ocean, bacterial activities favour the production of methylmercury (MeHg), allowing subsequent Hg uptake by biota (Paranjape and Hall, 2017). This is of particular concern as, among all the chemical forms of Hg, MeHg is the most efficiently up taken by aquatic organisms. Upon bioaccumulation, it can be distributed into many organs of the body (including the brain), biomagnified through food chains (Lavoie et al., 2013; Finley et al., 2016) and pose a health risk to aquatic organisms, including turtles (Scheuhammer et al., 2015).
Sea turtles are prominent members of the oceanic food web and have life history characteristics that make them vulnerable to Hg accumulation – such as their long life span, high trophic level in the food web, and their aquatic habitat (Sakai et al., 2000; Storelli and Marcotrigiano, 2003; Day et al., 2007; Bezerra et al., 2013; Schneider et al., 2013). The major pathway of Hg exposure and accumulation in marine animals is through diet (Kehrig et al., 2009), which accounts for approximately 80–90% of total Hg bioaccumulation, with the remainder being absorbed from water (Hrenchuk et al., 2012). In addition, turtles can transfer Hg from stored lipids to their offspring via egg production (Perrault et al., 2011; Hopkins et al., 2013; Landler et al., 2017; Sinaei and Bolouki, 2017), increasing the bioaccumulation of Hg in food webs.
The flatback turtle (Natator depressus), endemic to Australia, is the least studied species among all sea turtles in terms of toxic metal bioaccumulation, with only one study available on heavy metals in blood and eggs (Ikonomopoulou et al., 2011) from turtles nesting on Curtis Island, Queensland (QLD). Mercury, however, had concentrations below the detection limit of 0.1 mg/L in blood and 0.05 mg/kg in eggs. The flatback turtle is listed as data deficient by the IUCN Red List of Threatened Species due to a lack of data for population modelling (IUCN, 1996).
Methylmercury biomagnifies through food webs and, consequently, top predatory animals are at greatest risk for increased dietary MeHg exposure and potential Hg-related health effects (Schneider et al., 2013; Scheuhammer et al., 2015). Limited data available on the diet of flatback turtles suggest this species is carnivorous, feeding on soft-bodied invertebrates including soft corals, sea cucumbers, jellyfish, and sea pens (Limpus, 2007). Given its trophic level within the food web, this species could be particularly vulnerable to Hg bioaccumulation and its health effects.
In Western Australia (WA), Thevenard Island and Eighty Mile Beach are important nesting rookeries (nesting sites) for flatback turtles (Fossette et al., 2021). In the last decade, the mining sector has committed to an ongoing structured program of exploration and production activity in parts of WA (Keesing et al., 2018), including the Pilbara region, where Thevenard island is located. Mining activities in this region release Hg via atmospheric particles (Roche and Mudd, 2014), while gas extraction activities emits Hg to the atmosphere during steam cleaning and venting through flares (Young, 2019). Flatback turtles in this region may therefore be exposed to higher-than-background levels of Hg that may pose a health risk to them.
In the present study we sought to determine Hg concentrations in nesting turtles of two flatback turtle rookeries in WA: Thevenard Island and Eighty Mile Beach. We assessed the influence of body size on Hg concentrations in both skin and carapace (keratin layer) of flatback turtles. This is the first study to analyse Hg in skin and carapace of flatback turtles, providing baseline information of Hg bioaccumulation in this endangered species, and key data on the potential for adverse effects on turtle. We compared differences between rookeries over 700 km apart, hypothesising that the turtles from the southern rookery (Thevenard Island) were more likely to forage and reside in the Pilbara region closer to industrial mining activity and loading ports (potential exposure to higher environmental Hg concentrations) with turtles from the northern rookery (Eighty Mile Beach) more likely to reside and feed in the remote Kimberley.
The flatback turtle is unique among the seven species of sea turtles worldwide in that it does not have a pan-oceanic distribution and is reported to be endemic to the Australian continental shelf (Cogger and Lindner, 1969; Limpus et al., 1988). Flatback turtles nest on tropical beaches across northern Australia and use foraging grounds on the Australian continental shelf, southern Papua, Papua New Guinea and coastal waters of Irian Jaya (Limpus, 2007; Roarty, 2010). As adults, flatbacks appear to stay associated with distinct foraging areas that comprise individuals from multiple genetics stocks (distinct rookeries). At breeding season, adults migrate from the resident foraging areas up to 1,300 km to distinct rookeries to breed. Thus the breeding rookery can comprise individuals from both near and far foraging areas (Limpus, 2007). The sampled flatback turtle rockeries are from two genetic was stocks (FitzSimmons et al., 2020)
Western Australia is an important area for both nesting and foraging flatback turtle populations grounds (Fossette et al., 2021. Peel et al. in prep; Tucker et al., 2021). To be representative, samples were collected from a northern and southern rookery with different proximities to industrial development. Collection and sampling of flatback turtles was therefore undertaken at both locations.
Turtle samples were collected in the northern part of WA (Figure 1). The mining and petroleum industry in WA has been expanded significantly in the last decade, accounting for 92% of the state’s income (Australian Bureau of Statistics, 2017). Much of this expansion has occurred in the Pilbara region in the northern part of WA (Figure 1), a mineral rich region that has seen a significant development of mines, solar salt production, natural gas extraction and liquefaction, and the export of these commodities during the past 5 decades (Brocx, 2008). The Pilbara coast is arid (Semeniuk, 2013), making this region particularly prone to dust transport from mining activities.
FIGURE 1. Flatback monitoring sites in Thevenard Island and Eighty Mile Beach; orange circles represent operational mines in Western Australia as of September 2020; the Pilbara region is highlighted. Data obtained as shapefile from MINEDEX (DMIRS-001) database (Department of Mines Industry Regulation and Safety, 2020).
This island is offshore the Pilbara region and known for its large petroleum, natural gas and mineral deposits (Brueckner et al., 2013). Oil extraction began in 1985 with the discovery of the Saladin oil field just off Thevenard Island. Further discoveries in the coastal area of WA led to the development of the North West Shelf Project on the Burrup Peninsula, the Gorgon Project on Barrow Island and the Wheatstone Project on the mainland in Onslow. In 2015, mining activities on Thevenard Island were decommissioned (Wilkinson, 2016), but the North West Shelf Project and Gorgon Project are ongoing. Higher order marine animals could be exposed to higher Hg through particles settling in the surface waters and consumed through seawater or incorporated into the food chain and consumed as food.
Flatback turtles nest on Thevenard Island from November to February. The island represents a medium-size nesting site for flatback turtles (Whittock et al., 2016; Fossette et al., 2021) and has been declared a Nature Reserve. The Department of Biodiversity, Conservation and Attractions (DBCA) in WA started the North West Shelf Flatback Turtle Conservation Program in 2011, which implements population studies through track counts and mark-recapture. Monitoring started in 2016 on Thevenard Island. Monitoring of tracks on nest beaches is required to establish a baseline of turtle numbers and to detect any future changes.
Eighty Mile Beach is a 220 km stretch of uninterrupted sandy coastline in the Kimberley region of WA (Figure 1). It is a Ramsar-listed wetlands site, with the most inland occurring mangroves in WA (Secretariat, 1998). It was declared a Marine Park in 2013, in part due to its importance as a flatback turtle rookery, as well as the significant foraging grounds for flatback turtles (Young et al., 2014). It is approximately 600 km north-east of Thevenard Island and its closest industrial area is Port Hedland, the largest iron ore loading port in Australia 200 km south of Eighty Mile Beach (Figure 1). This is an important nesting site for flatbacks between October and February (Tucker et al., 2021). Although there are exploration permits further offshore Eighty Mile Beach, no current mining activities occur within 100 km of the shoreline (Department of Mines Industry Regulation and Safety, 2020).
Flatback turtle monitoring has been occurring sporadically at Eighty Mile Beach since 2005 (Young et al., 2014). A more regimented monitoring program was introduced in 2012, when the DBCA took responsibility for the Eighty Mile Beach Turtle Monitoring Program.
Carapace and skin samples of the same individual turtles were collected in November and December 2017, during the peak summer nesting season in WA. Sample collection was performed on randomly encountered turtles that were found nesting on the beach. These turtles were approached after oviposition was completed. Standard flipper tagging (Stockbrands titanium tags) was undertaken and morphometrics were taken with a fibreglass measuring tape for standard curved carapace length (CCL). Approximately 1 g of carapace and 1 g of skin samples were collected using a biopsy punch (Kai Medical, diameter: 5 mm). Skin samples were obtained from the trailing edge of the rear flipper, while carapace samples were obtained from the lateral margins of the shell. Only the keratin layer of the carapace was collected, following the protocols of Schneider et al. (2011). Samples were stored in individual plastic bags and frozen immediately at -5°C for transport to the Palaeoworks Lab at the Australian National University for analysis.
Carapace samples were ultrasonicated for 5 minutes in individual beakers holding Milli-Q water to remove any debris. All samples were placed individually in a clean glass vial, covered with parafilm and placed in a FreeZone Plus six freeze-drier (Labconco, Kansas City, MO) and lyophilized at −50°C for 48 h.
Total Hg concentration was determined by thermal decomposition, amalgamation, and atomic absorption spectrometry using a Milestone Direct Mercury Analyser (DMA-80 Tri-cell; Milestone, Bergamo, Italy) using the USEPA method 7,473 (USEPA, 1998). Two blanks and two Standard Reference Materials (SRMs) were analysed for every 36 samples. A replicate sample was run for every 10 samples, with recovery within 10% of the original sample and reported as the mean between the replicates. Dogfish muscle CRM Dorm-3 (National Research Council of Canada) was analysed, and results were in agreement with the certified reference material reports.
Data was analysed using R 3.5.1 (R Development Core Team, 2008) with p < 0.05 as the level of statistical significance. The assumption of normality was checked using the Shapiro-Wilk test and the equality of variances checked using the Bartlett test. As data did not meet the assumptions of normality and homoscedasticity, data were log(x)-transformed to normal distribution in order to use parametric tests, which are typically more powerful than non-parametric tests.
As bioaccumulation of Hg in turtles is known to be influenced by age (Schneider et al., 2013; Schneider and Vogt, 2018), the effects of carapace length (measured as CCL and used as a proxy for age) on Hg concentrations of turtles between the two rookeries was examined using an independent-sample two-tailed t-test. Mercury concentration was used as the dependent variable, and CCL as the independent variable. The same test was used to compare Hg concentrations in carapace and skin samples between the two populations, with Hg concentrations as dependent variable and carapace and/or skin as independent variables. A linear regression model was used to determine the relationship between CCL and Hg concentrations in skin and carapace of each turtle group.
A total of 50 skin and 52 carapace samples (n = 52 individuals) were collected from nesting turtles at Thevenard Island, and 23 skin and 28 carapace samples (n = 28 individuals) from nesting turtles at Eighty Mile Beach. For turtles already departing the beach when encountered, only carapace samples could be collected. The results for turtle size (CCL), skin Hg concentrations and carapace Hg concentrations for both rookeries are reported in Table 1. Turtle size (CCL) ranged from 813 to 990 mm (x̄ = 900 mm) (Figure 2). Mercury concentrations at Thevenard Island ranged from 7.2 to 39.5 ng/g (x̄ = 15.2 ng/g) in skin samples; and 19.1 to 106.9 ng/g (x̄ = 41.3 ng/g) in carapace samples (Figure 3A). In the Eighty Mile Beach samples, Hg concentrations ranged from 9.4 to 33.6 ng/g (x̄ = 19.4 ng/g) in the skin; and 13.7–107.7 ng/g (x̄ = 48.4 ng/g) in the carapace.
TABLE 1. Curved carapace length (CCL, mm), Hg concentrations (ng/g) in skin and carapace of nesting flatback turtles in rookeries from Thevenard Island and Eighty Mile Beach, WA.
FIGURE 2. Boxplot showing CCL (mm) of turtles from Eighty Mile Beach (control site) and Thevenard Island (impact site), WA. Bar whiskers show the 10th and 90th percentiles, box shows the 25th and 75th percentiles and the solid line in the box shows the median metal concentrations for each site. The asterisks indicate values that exceed the 1.5 interquartile range.
FIGURE 3. Boxplot showing natural log(Ln) of Hg concentrations (ng/g) in (A) skin of turtles and (B) carapace of turtles, from Eighty Mile Beach and Thevenard Island, WA.
There was no significant difference in the CCL between turtles nesting at Thevenard Island (x̄ = 902 ± 30.5 mm), and turtles nesting at Eighty Mile Beach (x̄ = 898 ± 34.6 mm) ((t-test results: t (78) = -0.519, p > 0.05) (Figure 2).
Turtles from the Eighty Mile Beach rookery had significantly higher mean skin Hg concentrations (x̄ = 19.4 ± 4.8 ng/g) than turtles from Thevenard Island (x̄ = 15.2 ± 5.8 ng/g), t-test: t (72) = −3.8055, p < 0.001 (Figure 3A).
The results of the t-test show no significant difference in mean Hg concentrations in the carapaces of turtles from Eighty Mile Beach (x̄ = 48.4 ± 21.8 ng/g) and Thevenard Island (x̄ = 41.3 ± 16.5 ng/g), t-test: t (77) = −1.27, p > 0.05 (Figure 3B).
A regression analysis tested if the turtle length (measured CCL) significantly predicted Hg concentrations in turtle skin. The results of the regression indicated that turtle CCL size alone cannot explain Hg concentrations in skin of turtles from either the Eighty Mile Beach rookery (R2 = 0.012, F(1,23) = 1.27, p > 0.05) or the Thevenard Island rookery (R2 = 0.019, F(1,48) = 12.44, p > 0.05) (Figure 4A), although CCL explained 43.1% of the variance in Hg concentrations in the carapace of turtles from Eighty Mile Beach (R2 = 0.431, F (1,25) = 20.7, p < 0.001) and 44.2% from Thevenard Island R2 = 0.442, F (1,50) = 41.35, p < 0.001) (Figure 4B).
FIGURE 4. Scatter plots and linear regression lines of flatback CCL versus Hg concentrations in (A) skin and (B) carapace of turtles from Eighty Mile Beach (pink) and Thevenard Island (blue).
The results of this study showed that flatback nesting females at Thevenard Island (x̄ = 902 mm) and Eighty Mile Beach (x̄ = 898 mm) were of similar mean sizes (CCL) (Table 1). This is an ideal scenario for comparing Hg concentrations between the two nesting sites as there is no effect of size on the sampled populations from different rookeries. The turtle sizes recorded in this study were similar to those previously reported for flatback turtle populations in WA: (x̄ = 887 mm (Limpus, 2007), (x̄ = 900 mm, (Pendoley et al., 2014), from the Northern Territory: x̄ = 869 mm, and slightly smaller than populations from Queensland: x̄ = 937 mm (Limpus, 2007; Pendoley et al., 2014).
In this study, we found a positive relationship between turtle size and Hg concentrations in the carapace of flatback turtles’ carapace, but not between turtle size and Hg concentrations in skin. It follows that carapace Hg concentration is a better predictor of exposure to Hg than Hg concentrations in skin tissue of flatbacks. This is in agreement with previous Hg studies on reptiles, which demonstrated the ability of keratin from both turtle carapace and caiman (Melanosuchus niger and Caiman crocodilus) skin to bioaccumulate Hg (Bezerra et al., 2013; Day et al., 2005; Innis et al., 2008; Komoroske et al., 2011; Schneider et al., 2015, 2009). This is because beta-keratins from caiman and turtle epidermis contain large amounts of amino acids (Alibardi, 2003; Toni et al., 2007) to which Hg is bound in relatively stable Hg complexes (Schneider et al., 2015). Consequently, a positive correlation between Hg concentration and turtle size can be obtained by analysing the keratin layer of carapace.
This is one of the few studies to measure Hg concentrations of a softshell turtle, along with Perrault et al. (2013) and Green et al. (2010), and the first study to measure Hg concentrations in the carapace and skin of the flatback turtle. Softshell turtles (Alibardi, 2002; Alibardi and Toni, 2006) have a soft and deformable carapace epidermis, not forming the hard and inflexible shell found in the loggerhead turtle (Caretta caretta) and the green turtle (Chelonia mydas). This is the first study to find a good correlation between Hg concentrations and body size in a turtle with reduced keratinization of the epidermal carapace, demonstrating that, despite the different keratin arrangement, carapace samples from the outermost keratin layer of soft-shelled turtles can be a good indicator of Hg in soft-shelled turtles (Schneider et al., 2015).
Flatback turtles have lower Hg concentrations compared to the carnivorous loggerhead turtle (Caretta caretta) (Tomas et al., 2006) and the omnivorous Kemp’s ridley Lepidochelys kempii (Schmid and Tucker, 2018), and similar concentrations to the green turtle (Chelonia mydas), which is omnivorous as a juvenile and predominantly herbivorous as an adult (Jimenez Heredia et al., 2017) (Figure 5).
FIGURE 5. Average Mercury (Hg) concentration (ng/g) in muscle/skin and carapace of sea turtle species worldwide (based on Table 2).
In comparison with other sea turtles (Table 2; Figure 5), a higher Hg concentration was expected for the carnivorous flatback turtles as Hg biomagnifies up food chains (Godley et al., 1999; Schneider et al., 2010). There are two possible reasons why Hg concentrations in flatback turtles in this study are relatively low compared to other turtles worldwide: firstly, the few available studies on the diet of this species may not fully capture its entire diet and may have missed potential herbivory or omnivory. The current literature on diet of flatback turtles is mostly limited to the turtle populations from northern Australia (Limpus et al., 1988; Zangerl et al., 1988; Limpus, 2007). Limpus (2007) reported a carnivorous diet in this species, consisting of benthic and pelagic invertebrates. Another study provided evidence of both omnivorous and carnivorous behaviours from stomach samples of stranded individuals (Bjorndal, 1997). Secondly, the lower Hg concentrations found in the flatback turtles might be a result of the naturally low Hg concentrations from the Australian continent (McQueen, 2011), as already hypothesised by Maher et al. (2020). To put this in perspective, for soils to be considered Hg enriched, they have to be >100 ng/g (Connor and Schaklette, 1975; Gustin et al., 2006). Across Australia, Hg concentrations in most soils and sediments are below 50 ng/g (Lintern et al., 2020; Schneider et al., 2021), demonstrating the naturally low Hg concentrations in the Australian environment and therefore low potential for Hg uptake by aquatic organisms.
TABLE 2. Total mean Hg concentration (ng/g) in muscle/skin and carapace of sea turtle species worldwide and in flatbacks from this study.
It is not possible to draw conclusions about the diet of the flatback turtle from our Hg concentrations. Mercury measurements of the entire local food web would be necessary to better establish the trophic level and diet of this species. More studies on the diet of flatback turtles, and analyses of δ13C and δ15N and stomach contents also would reveal the diet of this species to allow a valid conclusion about its tropic level.
In this study, there were no significant differences in Hg concentrations between two sampled turtle rookeries. Despite significant mining activity in the Pilbara region (where turtles nesting on Thevenard island mainly forage), no significant difference in Hg concentrations in carapace was found between turtles nesting at Thevenard Island and Eighty Mile Beach. In contrast, turtles from Eighty Mile Beach had significantly higher Hg concentrations in skin. A plausible explanation for the lack of effect of location on Hg concentrations in carapace could be due individuals from both rookery overlapping in the resident foraging areas. Although these populations have distinct nesting sites, shared foraging areas would inhibit differentiation between rookeries given that likely uptake pathways are through diet and drinking seawater. Foraging grounds that support turtles from multiple rookeries and multiple stocks (including Thevenard Island and Eighty Mile Beach) have been identified from a detailed study of satellite tracking data (Peel et al. in preparation). Other studies have shown that flatbacks from rookeries in the Pilbara share foraging areas (Pendoley et al., 2014; Thums et al., 2017; Waayers et al., 2019). Due to flexibility in feeding behaviour and foraging areas (Whittock et al., 2016), Hg concentrations in this turtle species may not reflect a single region. Future studies could focus on sampling turtles at foraging grounds, but obtaining high enough sample sizes from foraging areas may remain difficult across multiple locations.
A second reason reason for lack of difference in Hg concentrations between the two flatback rookeries is the form of Hg emission by local industry in the Pilbara and the prevailing wind direction. During mining and gas extraction operations, Hg can be released as a gas or salt (Spiric, 2001; Li et al., 2019). In nearby areas of the offshore Pilbara region (Barrow Island), most Hg as a by-product of mining is released into the atmosphere (Young, 2019) and its transport is consequently affected by wind direction. Once in the atmosphere, Hg can attach to fine dust particles and travel significant distances, contaminating sites well away from mining operations (Steinnes et al., 1997; Csavina et al., 2014).
Additional studies on Hg and the diet of flatback turtle would further advance our understanding of the Hg cycle for this species. Below are recommendations on the next research steps to be taken:
a) Diet and food web reconstruction based on the analyses of δ13C and δ15N and stomach contents, considering ontogeny, would provide a detailed diet information and provide insights to the low Hg concentrations measured in this species.
b) Mercury measurements in different environmental matrices (e.g., seawater, sediments, atmospheric aerosols) and biological indicators, would allow a complete overview of the Hg cycle in the coastal WA ecological system and Hg exposure to aquatic organisms.
c) Satellite tracking data would clarify any potential overlap in the foraging grounds for turtles from Thevenard Island and Eighty Mile Beach.
d) Laboratory studies on the metabolism of Hg in turtles would provide an understanding of the distribution, accumulation, and excretion of Hg in this species.
This study is the first to report Hg bioaccumulation in flatback turtles. Our research establishes Hg concentrations for two flatback turtle rookeries in Western Australia, advancing our understanding of Hg concentrations in these marine organisms. Despite limited understanding of environmental Hg concentrations and exposure by flatback turtles, sampled turtles have a much lower Hg concentration than reported for other sea turtle species worldwide. The present exploratory study found low mercury accumulation at rookeries, but a need remains to test for contaminants acquired at widely dispersed foraging grounds. Further studies on the diet and trophic levels of flatback turtles would support the interpretation of Hg exposure to this species.
The original contributions presented in the study are included in the article/supplementary material, further inquiries can be directed to the corresponding author.
The animal study was reviewed and approved by Department of Biodiversity, Conservation and Attractions.
LS conducted the field sampling, performed the laboratory and statistical analysis, and wrote the manuscript. SF, EY conducted field sampling and supported the writing of this manuscript. ADT and SW issued and organised sample collection and transport permits and supported the writing of this manuscript. KV supported the writing of this manuscript. All authors contributed to the article and approved the submitted version.
This work was supported by the North West Shelf Flatback Turtle Conservation Program from The Department of Biodiversity, Conservation and Attractions (DBCA) and the ANU School of Culture, History and Language. Laboratory Analyses Were Funded by The Department of Archaeology And Natural History At The Australian National University And by an Arc Discovery Early Career Researcher Award (De180100573) Awarded To LS.
The authors declare that the research was conducted in the absence of any commercial or financial relationships that could be construed as a potential conflict of interest.
All claims expressed in this article are solely those of the authors and do not necessarily represent those of their affiliated organizations, or those of the publisher, the editors and the reviewers. Any product that may be evaluated in this article, or claim that may be made by its manufacturer, is not guaranteed or endorsed by the publisher.
Field work was done under the auspices of permit authorisation number TFA 2019-0174 and animal ethics approval 2016/01 granted to Scott Whiting, WA DBCA export licence ES002418 and ACT Government import licence LI201868 granted to LS. Laboratory analyses were funded by the Department of Archaeology and Natural History at the Australian National University. We are appreciative of J. Cradock and S. Connor’s insights and comments, which improved this manuscript.
Alava, J. J., Cheung, W. W. L., Ross, P. S., and Sumaila, U. R. (2017). Climate Change-Contaminant Interactions in marine Food Webs: Toward a Conceptual Framework. Glob. Change Biol. 23, 3984–4001. doi:10.1111/gcb.13667
Alava, J. J., Cisneros-Montemayor, A. M., Sumaila, U. R., and Cheung, W. W. L. (2018). Projected Amplification of Food Web Bioaccumulation of MeHg and PCBs under Climate Change in the Northeastern Pacific. Sci. Rep. 8, 13460. doi:10.1038/s41598-018-31824-5
Alibardi, L. (2002). Immunocytochemical Observations on the Cornification of Soft and Hard Epidermis in the Turtle Chrysemys picta. Zoology 105, 31–44. doi:10.1078/0944-2006-00048
Alibardi, L. (2003). Immunocytochemistry and Keratinization in the Epidermis of Crocodilians. Zoolog. Stud. 42.
Alibardi, L., and Toni, M. (2006). Skin Structure and Cornification Proteins in the Soft-Shelled Turtle Trionyx Spiniferus. Zoology 109, 182–195. doi:10.1016/j.zool.2005.11.005
Amos, H. M., Jacob, D. J., Streets, D. G., and Sunderland, E. M. (2013). Legacy Impacts of All-Time Anthropogenic Emissions on the Global Mercury Cycle. Glob. Biogeochem. Cycles 27, 410–421. doi:10.1002/gbc.20040
Australian Bureau of Statistics (2017). Household Income and Wealth, Australia, 2015-2016. Canberra, ACT. Australia. Available at: https://www.abs.gov.au/AUSSTATS/abs@.nsf/Lookup/6523.0Main+Features12015-16?OpenDocument. Accessed November 1, 2021.
Bezerra, M. F., Lacerda, L. D., Lima, E. H. S. M., and Melo, M. T. D. (2013). Monitoring Mercury in green Sea Turtles Using Keratinized Carapace Fragments (Scutes). Mar. Pollut. Bull. 77, 424–427. doi:10.1016/j.marpolbul.2013.09.020
Bjorndal, K. A. (1997). “Foraging Ecology and Nutrition of Sea Turtles,” in The Biology of Sea Turtles. Editors P. Lutz, and J. Musick (Boca Raton, FL: CRC Press), 199–232.
Brocx, M. (2008). Geoheritage: From Global Perspectives to Local Principles for Conservation and Planning | Western Australian Museum. Perth, WA. Australia: Western Australian Museum.
Brueckner, M., Durey, A., Mayes, R., and Pforr, C. (2013). The Mining Boom and Western Australia's Changing Landscape: Towards Sustainability or Business as Usual? Rural Soc. 22, 111–124. doi:10.5172/rsj.2013.22.2.111
Canzanella, S., Danese, A., Mandato, M., Lucifora, G., Riverso, C., Federico, G., et al. (2021). Concentrations Of Trace Elements In Tissues Of Loggerhead Turtles (Caretta Caretta) From The Tyrrhenian And The Ionian Coastlines (Calabria, Italy). Environ. Sci. Pollut. Res. 28 (21), 26545–26557.
Cogger, H. G., and Lindner, D. A. (1969). Marine Turtles in Northern Australia. Australian Zoologist 15, 150–159.
Connor, J. J., and Schaklette, H. T. (1975). Background Geochemistry of Some Rocks, Soils, Plants, and Vegetables in the Conterminous United States.
Csavina, J., Taylor, M. P., Félix, O., Rine, K. P., Eduardo Sáez, A., and Betterton, E. A. (2014). Size-resolved Dust and Aerosol Contaminants Associated with Copper and lead Smelting Emissions: Implications for Emission Management and Human Health. Sci. Total Environ. 493, 750–756. doi:10.1016/j.scitotenv.2014.06.031
Davenport, J., Holland, D. L., Wrench, J., McEvoy, J., East, J., and Camacho-Ibar, V. (1990). “Chemical and Biochemical Analysed of the Tissues of a Beached Leatherback Turtle (Dermochelys coriacea L.),” in Proceedings of the Tenth Annual Workshop on Sea Turtle Biology and Conservation, Hilton Head Island, South Carolina, February 20–24, 1990 NOAA Technical Memorandum NMFS-SEFC-278.
Day, R. D., Christopher, S. J., Becker, P. R., and Whitaker, D. W. (2005). Monitoring Mercury in the Loggerhead Sea Turtle, Caretta caretta. Environ. Sci. Technol. 39, 437–446. doi:10.1021/es049628q
Day, R. D., Segars, A. L., Arendt, M. D., Lee, A. M., and Peden-Adams, M. M. (2007). Relationship of Blood Mercury Levels to Health Parameters in the Loggerhead Sea Turtle (Caretta caretta). Environ. Health Perspect. 115, 1421–1428. doi:10.1289/ehp.9918
Department of Mines Industry Regulation and Safety (2020). Minedex - DMIRS 001 [WWW Document]. Minedex - DMIRS 001. Available at: https://catalogue.data.wa.gov.au/dataset/minedex-dmirs-001 (accessed 9.23.20).
Di Beneditto, A. P., Araújo, B. F., and de Carvalho, C. E. (2019). Hg and δ15N in Juvenile Green Turtles From Southeastern Brazil (∼23°S): Inferences About Contamination Levels And Recruitment To Coastal Waters. Marine pollution bulletin 142, 64–68.
Esposito, P., De Roma, A., Sansone, D., Capozzo, D., Iaccarino, D., di Nocera, F., et al. (2020). Non-Essential Toxic Element (Cd, As, Hg and Pb) Levels In Muscle, Liver And Kidney Of Loggerhead Sea Turtles (Caretta Caretta) Stranded Along The Southwestern Coasts Of Tyrrhenian Sea. Comp. Biochem. Physiol. Part - C: Toxicol. Pharmacol. 231, 108725.
Eggins, S., Schneider, L., Krikowa, F., Vogt, R. C., Silveira, R. D., and Maher, W. (2015). Mercury Concentrations in Different Tissues of Turtle and caiman Species from the Rio Purus, Amazonas, Brazil. Environ. Toxicol. Chem. 34, 2771–2781. doi:10.1002/etc.3151
Febrer-Serra, M., Renga, E., Ferniández, G., Lassnig, N., Tejada, S., Capó, X., et al. (2020). First Report Of Heavy Metal Presence In Muscular Tissue Of Loggerhead Turtles Caretta Caretta (Linnaeus, 1758) From The Balearic Sea (Balearic Islands, Spain). Environ. Sci. Pollution Res. 27 (31), 39651–39656.
Finley, M. L. D., Kidd, K. A., Curry, R. A., Lescord, G. L., Clayden, M. G., and O’Driscoll, N. J. (2016). A Comparison of Mercury Biomagnification through Lacustrine Food Webs Supporting Brook Trout (Salvelinus fontinalis) and Other Salmonid Fishes. Front. Environ. Sci 4, 23. doi:10.3389/fenvs.2016.00023
FitzSimmons, N. N., Pittard, S. D., McIntyre, N., Jensen, M. P., Guinea, M., Hamann, M., et al. (2020). Phylogeography, Genetic Stocks, and Conservation Implications for an Australian Endemic marine Turtle. Aquat. Conserv: Mar Freshw Ecosyst 30, 440–460. doi:10.1002/aqc.3270
Fossette, S., Loewenthal, G., Peel, L. R., Vitenbergs, A., Hamel, M. A., Douglas, C., et al. (2021). Using Aerial Photogrammetry to Assess Stock-wide marine Turtle Nesting Distribution, Abundance and Cumulative Exposure to Industrial Activity. Remote Sensing 13 (6), 1116. doi:10.3390/rs13061116
Godley, B. J., Thompson, D. R., and Furness, R. W. (1999). Do Heavy Metal Concentrations Pose a Threat to Marine Turtles from the Mediterranean Sea? Mar. Pollut. Bull. 38, 497–502. doi:10.1016/s0025-326x(98)00184-2
Gómez-Ramírez, P., Espín, S., Navas, I., Martínez-López, E., Jiménez, P., María-Mojica, P., et al. (2020). Mercury And Organochlorine Pesticides In Tissues Of Loggerhead Sea Turtles (Caretta Caretta) Stranded Along The Southwestern Mediterranean Coastline (Andalusia, Spain). Bulletin Environ. Contam. Toxicol. 104 (5), 559–567.
Green, A. D., Buhlmann, K. A., Hagen, C., Romanek, C., and Gibbons, J. W. (2010). Mercury Contamination in Turtles and Implications for Human Health. J. Environ. Health 72, 14–22.
Gustin, M. S., Engle, M., Ericksen, J., Lyman, S., Stamenkovic, J., and Xin, M. (2006). Mercury Exchange between the Atmosphere and Low Mercury Containing Substrates. Appl. Geochem. 21, 1913–1923. doi:10.1016/j.apgeochem.2006.08.007
Hopkins, B. C., Willson, J. D., and Hopkins, W. A. (2013). Mercury Exposure Is Associated with Negative Effects on Turtle Reproduction. Environ. Sci. Technol. 47, 2416–2422. doi:10.1021/es304261s
Hrenchuk, L. E., Blanchfield, P. J., Paterson, M. J., and Hintelmann, H. H. (2012). Dietary and Waterborne Mercury Accumulation by Yellow Perch: a Field experiment. Environ. Sci. Technol. 46, 509–516. doi:10.1021/es202759q
Ikonomopoulou, M. P., Olszowy, H., Limpus, C., Francis, R., and Whittier, J. (2011). Trace Element Concentrations in Nesting Flatback Turtles (Natator Depressus) from Curtis Island, Queensland, Australia. Mar. Environ. Res. 71, 10–16. doi:10.1016/j.marenvres.2010.09.003
Innis, C., Tlusty, M., Perkins, C., Holladay, S., Merigo, C., and Weber, E. S. (2008). Trace Metal and Organochlorine Pesticide Concentrations in Cold-Stunned Juvenile Kemp's Ridley Turtles (Lepidochelys Kempii) from Cape Cod, Massachusetts. Chelonian Conservation Biol. 7, 230–239. doi:10.2744/CCB-0707.1
Jimenez, A., Pingo, S., Alfaro, J., Mangel, J., and Hooker, Y. (2017). Feeding Ecology of the green Turtle Chelonia mydas in Northern Peru. lajar 45, 585–596. doi:10.3856/vol45-issue3-fulltext-8
Keesing, J. K., Gartner, A., Westera, M., Edgar, G. J., Myers, J., Hardman-Mountford, N. J., et al. (2018). “Impacts and Environmental Risks of Oil Spills on Marine Invertebrates, Algae and Seagrass: A Global Review from an Australian Perspective,” in Oceanography and Marine Biology. Editors S. J. Hawkins, A. J. Evans, A. C. Dale, L. B. Firth, and I. P. Smith (Boca Raton, FL, USA: Taylor & Francis), 311–370. doi:10.1201/9780429454455-5
Kehrig, H. A., Fernandes, K. W. G., Malm, O., Seixas, T. G., Di Beneditto, A. P. M., and Souza, C. M. M. d. (2009). Transferência trófica de mercúrio e selênio na costa norte Do Rio de Janeiro. Quím. Nova 32, 1822–1828. doi:10.1590/S0100-40422009000700026
Komoroske, L. M., Lewison, R. L., Seminoff, J. A., Deheyn, D. D., and Dutton, P. H. (2011). Pollutants and the Health of green Sea Turtles Resident to an Urbanized Estuary in San Diego, CA. Chemosphere 84, 544–552. doi:10.1016/j.chemosphere.2011.04.023
Landler, L., Painter, M. S., Coe, B. H., Youmans, P. W., Hopkins, W. A., and Phillips, J. B. (2017). High Levels of Maternally Transferred Mercury Disrupt Magnetic Responses of Snapping Turtle Hatchlings (Chelydra serpentina). Environ. Pollut. 228, 19–25. doi:10.1016/j.envpol.2017.04.050
Lavoie, R. A., Jardine, T. D., Chumchal, M. M., Kidd, K. A., and Campbell, L. M. (2013). Biomagnification of Mercury in Aquatic Food Webs: A Worldwide Meta-Analysis. Environ. Sci. Technol. 47, 13385–13394. doi:10.1021/es403103t
Li, J., Han, Z., Yan, Q., Wang, S., and Ge, S. (2019). Distribution and Genesis of Mercury in Natural Gas of Large Coal Derived Gas fields in China. Pet. Exploration Develop. 46, 463–470. doi:10.1016/S1876-3804(19)60027-3
Limpus, C. J. (2007). A Biological Review of Australian marine Turtles. Brisbane, QLD: Environmental Protection Agency.
Limpus, C. J., Gyuris, E., and Miller, J. D. (1988). Reassessment of the Taxonomic Status of the Sea Turtle Genus Natator Mcculloch, 1908, with a Redescription of the Genus and Species. Trans. R. Soc. South Aust. 112, 1–10.
Lintern, A., Schneider, L., Beck, K., Mariani, M., Fletcher, M.-S., Gell, P., et al. (2020). Background Concentrations of Mercury in Australian Freshwater Sediments: The Effect of Catchment Characteristics on Mercury Deposition. Elementa: Sci. Anthropocene 8, 019. doi:10.1525/elementa.019
Maher, W., Krikowa, F., and Ellwood, M. (2020). Mercury Cycling in Australian Estuaries and Near Shore Coastal Ecosystems: Triggers for Management. Elementa: Sci. Anthropocene 8. doi:10.1525/elementa.425
Maffucci, F., Caurant, F., Bustamante, P., and Bentivegna, F. (2005). Trace Element (Cd, Cu, Hg, Se, Zn) Accumulation And Tissue Distribution In Loggerhead Turtles (Caretta Caretta) From The Western Mediterranean Sea (Southern Italy). Chemosphere 58 (5), 535–542.
McQueen, K. (2011). Mercury Mining: A Quick History of Quicksilver in Australia. J. Australas. Mining Hist. 9, 74–93.
Obrist, D., Kirk, J. L., Zhang, L., Sunderland, E. M., Jiskra, M., and Selin, N. E. (2018). A Review of Global Environmental Mercury Processes in Response to Human and Natural Perturbations: Changes of Emissions, Climate, and Land Use. Ambio 47, 116–140. doi:10.1007/s13280-017-1004-9
Nicolau, L., Monteiro, S. S., Pereira, A. T., Marçalo, A., Ferreira, M., Torres, T., et al. (2017). Trace Elements In Loggerhead Turtles (Caretta Caretta) Stranded In Mainland Portugal: Bioaccumulation And Tissue Distribution. Chemosphere 179, 120–126.
Novillo, O., Pertusa, J. F., and Tomás, J. (2017). Exploring The Presence Of Pollutants At Sea: Monitoring Heavy Metals And Pesticides In Loggerhead Turtles (Caretta Caretta) From The Western Mediterranean. Sci. Total Environ. 598, 1130–1139.
Paranjape, A. R., and Hall, B. D. (2017). Recent Advances in the Study of Mercury Methylation in Aquatic Systems. FACETS 2, 85–119. doi:10.1139/facets-2016-0027
Pendoley, K. L., Schofield, G., Whittock, P. A., Ierodiaconou, D., and Hays, G. C. (2014). Protected Species Use of a Coastal marine Migratory Corridor Connecting marine Protected Areas. Mar. Biol. 161, 1455–1466. doi:10.1007/s00227-014-2433-7
Perrault, J. R., Miller, D. L., Garner, J., and Wyneken, J. (2013). Mercury and Selenium Concentrations in Leatherback Sea Turtles (Dermochelys Coriacea): Population Comparisons, Implications for Reproductive success, hazard Quotients and Directions for Future Research. Sci. Total Environ. 463-464, 61–71. doi:10.1016/j.scitotenv.2013.05.067
Perrault, J., Wyneken, J., Thompson, L. J., Johnson, C., and Miller, D. L. (2011). Why Are Hatching and Emergence success Low? Mercury and Selenium Concentrations in Nesting Leatherback Sea Turtles (Dermochelys Coriacea) and Their Young in Florida. Mar. Pollut. Bull. 62, 1671–1682. doi:10.1016/j.marpolbul.2011.06.009
R Development Core Team (2008). R: A Language and Environment for Statistical Computing. Vienna, Austria: R Foundation for Statistical Computing. Available at: http://www.R-project.org.
Secretariat, R., 1998. Ramsar Sites Information Service [WWW Document]. Available at: https://rsis.ramsar.org/ris/480 (accessed 8.27.20).
Roarty, M. (2010). The Australian Resources Sector its Contribution to the Nation, and a Brief Review of Issues and Impacts.
Roche, C., and Mudd, G. (2014). “An Overview of Mining and the Environment in Western Australia,” in Resource Curse or Cure ? on the Sustainability of Development in Western Australia, CSR, Sustainability, Ethics & Governance. Editors M. Brueckner, A. Durey, R. Mayes, and C. Pforr (Berlin, Heidelberg: Springer), 179–194. doi:10.1007/978-3-642-53873-5_12
Sakai, H., Saeki, K., Ichihashi, H., Suganuma, H., Tanabe, S., and Tatsukawa, R. (2000). Species-Specific Distribution of Heavy Metals in Tissues and Organs of Loggerhead Turtle (Caretta caretta) and Green Turtle (Chelonia mydas) from Japanese Coastal Waters. Mar. Pollut. Bull. 40, 701–709. doi:10.1016/S0025-326X(00)00008-4
Scheuhammer, A., Braune, B., Chan, H. M., Frouin, H., Krey, A., Letcher, R., et al. (2015). Recent Progress on Our Understanding of the Biological Effects of Mercury in Fish and Wildlife in the Canadian Arctic. Sci. Total Environ. 509-510, 91–103. doi:10.1016/j.scitotenv.2014.05.142
Schmid, J. R., and Tucker, A. D. (2018). Comparing Diets of Kemp's Ridley Sea Turtles (Lepidochelys Kempii) in Mangrove Estuaries of Southwest Florida. J. Herpetology 52, 252–258. doi:10.1670/16-164
Schneider, L., Belger, L., Burger, J., Vogt, R. C., and Ferrara, C. R. (2010). Mercury Levels in Muscle of Six Species of Turtles Eaten by People along the Rio Negro of the Amazon Basin. Arch. Environ. Contam. Toxicol. 58, 444–450. doi:10.1007/s00244-009-9358-z
Schneider, L., Belger, L., Burger, J., Vogt, R. C., Jeitner, C., and Peleja, J. R. P. (2011). Assessment of Non-invasive Techniques for Monitoring Mercury Concentrations in Species of Amazon Turtles. Toxicol. Environ. Chem. 93, 238–250. doi:10.1080/02772248.2010.517627
Schneider, L., Belger, L., Burger, J., and Vogt, R. (2009). Mercury Bioacumulation in Four Tissues of Podocnemis Erythrocephala (Podocnemididae: Testudines) as a Function of Water Parameters. Sci. Total Environ. 407, 1048–1054. doi:10.1016/j.scitotenv.2008.09.049
Schneider, L., Eggins, S., Maher, W., Vogt, R. C., Krikowa, F., Kinsley, L., et al. (2015). An Evaluation of the Use of Reptile Dermal Scutes as a Non-invasive Method to Monitor Mercury Concentrations in the Environment. Chemosphere 119, 163–170. doi:10.1016/j.chemosphere.2014.05.065
Schneider, L., Maher, W., Green, A., and Vogt, R. (2013). “Mercury Contamination in Reptiles: An Emerging Problem with Consequences for Wild Life and Human Health,” in Mercury: Sources, Applications and Health Impacts, 173–232.
Schneider, L., Rose, N. L., Myllyvirta, L., Haberle, S., Lintern, A., Yuan, J., et al. (2021). Mercury Atmospheric Emission, Deposition and Isotopic Fingerprinting from Major Coal-Fired Power Plants in Australia: Insights from Palaeo-Environmental Analysis from Sediment Cores. Environ. Pollut. 287, 117596. doi:10.1016/j.envpol.2021.117596
Schneider, L., and Vogt, R. C. (2018). “Turtles of the Igapó: Their Ecology and Susceptibility to Mercury Uptake,” in Igapó (Black-Water Flooded Forests) of the Amazon Basin. Editor R. W. Myster (Cham: Springer International Publishing), 161–182. doi:10.1007/978-3-319-90122-0_11
Semeniuk, V. (2013). Predicted Response of Coastal Wetlands to Climate Changes: a Western Australian Model. Hydrobiologia 708, 23–43. doi:10.1007/s10750-012-1159-0
Sinaei, M., and Bolouki, M. (2017). Metals in Blood and Eggs of Green Sea Turtles (Chelonia mydas) from Nesting Colonies of the Northern Coast of the Sea of Oman. Arch. Environ. Contam. Toxicol. 73, 552–561. doi:10.1007/s00244-017-0421-x
Soerensen, A. L., Sunderland, E. M., Holmes, C. D., Jacob, D. J., Yantosca, R. M., Skov, H., et al. (2010). An Improved Global Model for Air-Sea Exchange of Mercury: High Concentrations over the North Atlantic. Environ. Sci. Technol. 44, 8574–8580. doi:10.1021/es102032g
Spiric, Z. (2001). “Innovative Approach to the Mercury Control during Natural Gas Processing,” in Engineering Technology Conference on Energy, Houston, Texas, USA, February 5–7, 2001, 8. doi:10.1115/etce2001-17085
Steinnes, E., Allen, R. O., Petersen, H. M., Rambæk, J. P., and Varskog, P. (1997). Evidence of Large Scale Heavy-Metal Contamination of Natural Surface Soils in Norway from Long-Range Atmospheric Transport. Sci. Total Environ. 205, 255–266. doi:10.1016/s0048-9697(97)00209-x
Storelli, M. M, Ceci, E., and Marcotrigiano, G. O. (1998a). Distribution Of Heavy Metal Residues In Some Tissues Of Caretta Caretta (Linnaeus) Specimen Beached Along The Adriatic Sea (Italy). Bulletin Environ. Contamin. Toxicol. 60 (4), 546–552.
Storelli, M. M, Ceci, E., and Marcotrigiano, G. O. (1998b). Comparison Of Total Mercury, Methylmercury, And Selenium In Muscle Tissues And In The Liver Of Stenella Coeruleoalba (Meyen) And Caretta Caretta (Linnaeus). Bulletin Environ. Contamin. Toxicol. 61 (4), 541–547.
Storelli, M. M., and Marcotrigiano, G. O. (2003). Heavy Metal Residues in Tissues of marine Turtles. Mar. Pollut. Bull. 46, 397–400. doi:10.1016/S0025-326X(02)00230-8
Thums, M., Waayers, D., Huang, Z., Pattiaratchi, C., Bernus, J., and Meekan, M. (2017). Environmental Predictors of Foraging and Transit Behaviour in Flatback Turtles Natator Depressus. Endang. Species Res. 32, 333–349. doi:10.3354/esr00818
Tomas, J., Aznar, F. J., and Raga, J. A. (2006). Feeding Ecology of the Loggerhead Turtle Caretta caretta in the Western Mediterranean. J. Zoolog. 255, 525–532. doi:10.1017/S0952836901001613
Toni, M., Dalla Valle, L., and Alibardi, L. (2007). Hard (Beta-)Keratins in the Epidermis of Reptiles: Composition, Sequence, and Molecular Organization. J. Proteome Res. 6, 3377–3392. doi:10.1021/pr0702619
Tucker, A., Pendoley, K., Murray, K., Loewenthal, G., Barber, C., Denda, J., et al. (20212021). Regional Ranking of marine Turtle Nesting in Remote Western Australia by Integrating Traditional Ecological Knowledge and Remote Sensing. Remote Sensing 13, 4696. doi:10.3390/rs13224696
USEPA (1998). Method 7473 - Mercury in Solids and Solutions by thermal Decomposition, Amalgamation, and Atomic Absorption Spectrophotometry (No. SW-846-). Washington, D.C: US Environmental Protection Agency.
Van de Merwe, J. P., Hodge, M., Olszowy, H. A., Whittier, J. A., and Lee, S. Y. (2010). Using Blood Samples To Estimate Persistent Organic Pollutants And Metals In Green Sea Turtles (Chelonia Mydas). Marine pollution bulletin 60 (4), 579–588.
Waayers, D., Tucker, T., Whiting, S., Groom, R., Vanderklift, M., Pillans, R., et al. (2019). Satellite Tracking of Marine Turtles in the South-Eastern Indian Ocean: a Review of Deployments Spanning 1990-2016. Indian Ocean Newsl. 29, 24–37.
Whittock, P. A., Pendoley, K. L., and Hamann, M. (2016). Flexible Foraging: Post-nesting Flatback Turtles on the Australian continental Shelf. J. Exp. Mar. Biol. Ecol. 477, 112–119. doi:10.1016/j.jembe.2016.01.015
Young, E. (2019). Doctors “Very Worried” at Chevron Venting Toxic Chemicals at Gorgon. Perth, Western Australia: WAtoday. (online).
Young, E., Whiting, S., Tucker, T., Bobojcov, A., Williams, C., and Badal, A., and Karrajarri Ranger Group (2014). “Eighty Mile Beach Turtle Monitoring Program: Past, Present and Future,” in Second Australian and Second Western Australian Marine Turtle Symposia (Perth, WA: Department of Parks and Wildlife).
Keywords: Pilbara, Australian continental shelf, keratin, pollution, megafauna
Citation: Schneider L, Tucker AD, Vincent K, Fossette S, Young EJ and Whiting SD (2022) First Assessment of Mercury (Hg) Concentrations in Skin and Carapace of Flatback Turtles (Natator depressus) (Garman) From Western Australia. Front. Environ. Sci. 10:843855. doi: 10.3389/fenvs.2022.843855
Received: 27 December 2021; Accepted: 09 February 2022;
Published: 17 March 2022.
Edited by:
Juan José Alava, University of British Columbia, CanadaReviewed by:
Martin F. Soto-Jimenez, National Autonomous University of Mexico, MexicoCopyright © 2022 Schneider, Tucker, Vincent, Fossette, Young and Whiting. This is an open-access article distributed under the terms of the Creative Commons Attribution License (CC BY). The use, distribution or reproduction in other forums is permitted, provided the original author(s) and the copyright owner(s) are credited and that the original publication in this journal is cited, in accordance with accepted academic practice. No use, distribution or reproduction is permitted which does not comply with these terms.
*Correspondence: Larissa Schneider, TGFyaXNzYS5TY2huZWlkZXJAYW51LmVkdS5hdQ==
Disclaimer: All claims expressed in this article are solely those of the authors and do not necessarily represent those of their affiliated organizations, or those of the publisher, the editors and the reviewers. Any product that may be evaluated in this article or claim that may be made by its manufacturer is not guaranteed or endorsed by the publisher.
Research integrity at Frontiers
Learn more about the work of our research integrity team to safeguard the quality of each article we publish.