- Faculty of Chemical and Food Engineering, Bahir Dar Institute of Technology, Bahir Dar University, Bahir Dar, Ethiopia
Dyes, especially azo dyes contained in wastewaters released from textile, pigment, and leather industries, are entering into natural waterbodies. This results in environmental deterioration and serious health damages (for example carcinogenicity and mutagenesis) through food chains. Physiochemical, membrane processes, electrochemical technology, advanced oxidation processes, reverse osmosis, ion exchange, electrodialysis, electrolysis, and adsorption techniques are commonly used conventional treatment technologies. However, the limitations of most of these methods include the generation of toxic sludge, high operational and maintenance costs. Thus, technological advancements are in use to remediate dyes from effluents. Adsorption using the nonconventional biomass-based sorbents is the greatest attractive alternatives because of their low cost, sustainability, availability, and eco-friendly. We present and reviewed up-to-date publications on biomass-based sorbents used for dye removal. Conceptualization and synthesizing their state-of-the-art knowledge on their characteristics, experimental conditions used were also discussed. The merits and limitations of various biosorbents were also reflected. The maximum dye adsorption capacities of various biosorbents were reviewed and synthesized in the order of the biomass type (algae, agricultural, fungal, bacterial, activated carbon, yeast, and others). Surface chemistry, pH, initial dye concentration, temperature, contact time, and adsorbent dose as well as the ways of the preparations of materials affect the biosorption process. Based on the average dye adsorption capacity, those sorbents were arranged and prioritized. The best fit of the adsorption isotherms (for example Freundlich and Langmuir models) and basic operating parameters on the removal dyes were retrieved. Which biomass-based adsorbents have greater potential for dye removal based on their uptake nature, cost-effectiveness, bulk availability, and mono to multilayer adsorption behavior was discussed. The basic limitations including the desorption cycles of biomass-based adsorbent preparation and operation for the implementation of this technology were forwarded.
Highlights
• Dye removal from wastewater using bio-based adsorbents is a promising alternative.
• Algae, yeast, bacterial, fungal, agro-waste, and plant debris are potential bioadsorbent sources.
• Simulated wastewater studies need to switch into pollutants in the actual wastewater.
• Knowing the surface charge nature of the biosorbents is important.
• Suitable modification of biosorbents for enhanced removal is required
1 Introduction
Availability of accepted quality of water is one of the major problems faced in the 21st century (Zhou et al., 2019; Al-Amshawee et al., 2019). The quality of water resources is declining daily due to various anthropogenic activities, unplanned urbanization, and increasing industrialization. Various types of dyes and metal ions are the major pollutants encountered in wastewater effluent, which disturb the aquatic environment (Varghese et al., 2019; Saravanan et al., 2021).
Dyes are organic compounds that are released from several industrial sources including textile, paper, leather, rubber, cosmetic, and printing industries (Piaskowski et al., 2018; Katheresan et al., 2018). To satisfy the modern need, it is assessed that 0.7–1.6 million tons of dyes are delivered yearly and 10–15% of this volume is disposed of as wastewater, making it major water pollutants (Bhatia et al., 2017; Varghese et al., 2019; Syafiuddin and Fulazzaky, 2020). Excessive exposure to dye causes skin irritation, respiratory problems and some dyes even increase the risk of cancer in humans (Amirza et al., 2017). Consequently, it is of most extreme significance to eliminate colors from wastewater viably to guarantee the protected release of treated effluent into streams.
Many technologies have been emerging for treating and handling pollutant-laden wastewater. Some commonly used treatment technologies consist of biological treatments, membrane process, chemical, and electrochemical technology, reverse osmosis, ion exchange, electrodialysis, electrolysis, and adsorption techniques (Varghese et al., 2019; Guo et al., 2019; Ahmad et al., 2015). However, the limitations of most of these methods include the generation of toxic sludge, high operational and maintenance costs, and the intricate technique involved in the treatment (Yahiaoui et al., 2021). Comparatively, the adsorption method is considered a better treatment process in wastewater treatment technologies due to ease of operation, convenience, and simplicity of design (Aragaw and Angerasa, 2020; Aragaw, 2020), but it has a limitation of sludge generation (spent adsorbents after use) as other removal processes (Patel, 2021; Vakili et al., 2019). Activated carbon is undoubtedly considered a universal adsorbent for effluent treatment and is commonly used for the remo//val of several pollutants (Rahimian and Zarinabadi, 2020). However, its extensive use in wastewater treatment is sometimes limited due to its higher cost. Different varieties of nonconventional sorbents have been investigated for their capacity to remove various types of contaminants from the wastewater (Sivaranjanee and Kumar, 2021), but a report of various low-cost adsorbents shows limited sorption potential than commercial activated carbon in the removal of various pollutants (Ahmad et al., 2011a; Varghese et al., 2019). In contrast to these investigations, Hassan and Carr (2021) reviewed studies that revealed the dye-adsorption capacity of some carbonaceous adsorbents derived from biomasses and their composites is noticeably higher than the commercial activated carbon adsorbents (Hassan and Carr, 2021). Therefore, the search for the development of low-cost materials as adsorbents along with the precursor for the preparation of activated carbon is ongoing. Bio adsorbents are the recent materials employed in various sectors to remove dyes and other pollutants. Charcoal, peat, chitin, microbial biomass (fungi, bacteria, yeast, etc.), wood bark, and other agricultural and industrial wastes are frequently used bio-adsorbents (Kumar et al., 2021).
Owing to eco-friendly, low cost, and good surface characteristics, biomass-based adsorbents are attracting attention and are widely studied. The publication trends (Figure 1) confirmed that recently the number of publications increased. The publication trends with a search query “biosorbents for the removal of dyes” were conducted from PubMed (https://pubmed.ncbi.nlm.nih.gov/). The journal article publications were filtered and found a total of 209 papers with a publication date accustomed from 2002 to 2020. Furthermore, the specific search query of the biobased adsorbents for the dyes was retrieved. As it can be observed, publication counts from 2017 to 2020 are highly consistently increased suggested that the nonconventional adsorbents from biomass are recently attracting attention for dye removal. In addition to PubMed, the journal article publications were retrieved from Google Scholar (https://scholar.google.com/) because it is not a comprehensive database. The reviewing, filtration, and prioritization criteria on the retrieved publications were employed based on the contents contained in the articles and fitted to the scope of the present review. The retrieved publications were found as adsorbents prepared from different biomasses activated and/or modified with various chemicals or techniques. For example, the bioadsorbents may be activated with acid/base (such as HCl, NaOH) and thermal activation, and also some are composited with inorganic and/or organic nanomaterials. The number of publications from 2017 to 2020 was found as a consistent increase (Figure 1) suggested that wastewater treatments using bioadsorbent are recent and attracting scholars.
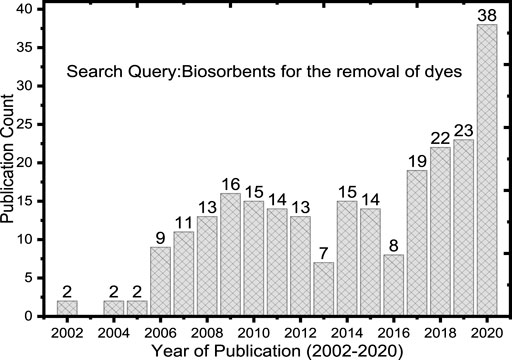
FIGURE 1. Publication trends from the years 2002–2020 in the Biosorbents application for the removal of dyes.
However, biomass-based adsorbents need to be researched and a sufficient number of comprehensive review papers are required because of their being ecofriendly, cost-effective, and widely available. The present review evaluates the adsorption of dyes using bioadsorbents prepared from various biomasses, detailed adsorptive applications, and removal capacities of the prepared adsorbents for different types of dyes. Also, different wastewater treatment techniques for dye removal are highlighted. Moreover, the limitation of bioadsorbents for the adsorptive removal of contaminants is discussed. At the end, the cost analysis, regeneration capacity, and prospects of the bioadsorbents to the area are pointed out. Thus, this review mainly aimed at assessing the potential of several biomass-based adsorbents utilized so far for the removal of various dyes. Several up-to-date biomass-based adsorbents including fungal, bacterial, algal, yeast, and agricultural and forest biomasses used for a variety of dyes removal are discussed. Furthermore, this review could discourse a literature review in one referenced paper that can draw up the separated publications to the area resulting in suggestion of new/modified research tips by synthesizing at-hand studies.
2 Wastewater Treatment Techniques for Dyes Removal
Dyes are colored compounds, used for coloring textiles, wools, and fiber from various industries such as textile, food processing, ink, cosmetics, pharmaceutics, printer inks, leather, and plastics production (Piaskowski et al., 2018; Rodríguez Couto, 2009), which need to be removed after usage not to pollute the marine environment and ecosystem, and not to pose health effect to humans. It also blocks sunlight penetration thereby inhibiting photosynthesis (Bello et al., 2015; Dey et al., 2017). Dyes are usually classified based on their chemical structure, such as anionic, cationic, and nonionic; reactive dyes are stable and anionic, and display resistance toward light (Heidari et al., 2019; Dai et al., 2018). As shown in Figure 2, several types of dye removal technologies are accessible with varying degrees of success, such as chemical precipitation, reduction, oxidation, coagulation, ion-exchange, reverse osmosis, solvent extraction, flocculation, membrane separation, filtration, evaporation, electrolysis, and adsorption, which have been used to remove and recover toxic contaminants from industrial effluent (Afroze and Sen, 2018; Ahmad et al., 2011b). All the aforementioned methods have their own merits and demerits (Table 1), which need vast considerations to use and investigate during dye removal processes.
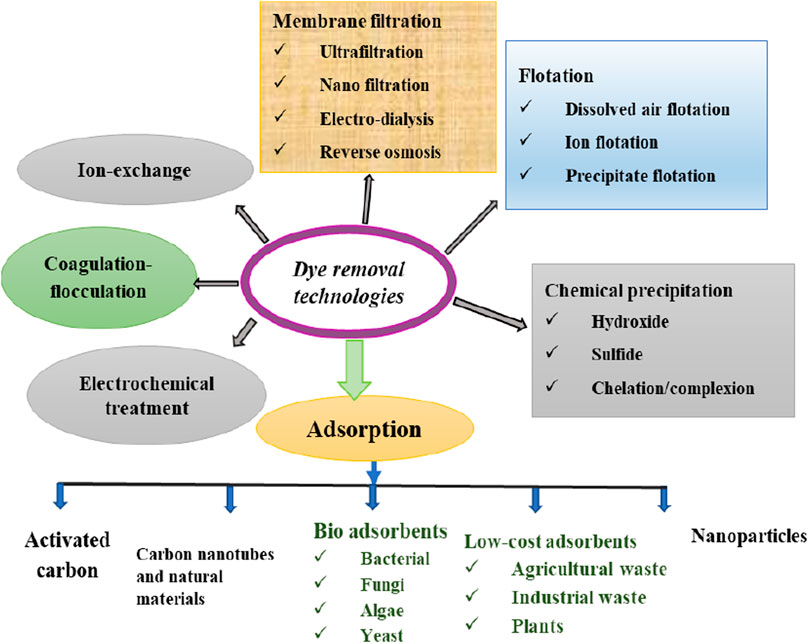
FIGURE 2. Schematic representations of available dye removal technologies: adopted with modification from Roy and Saha (2021).
2.1 Chemical Precipitation
This wastewater treatment method involves chemicals such as hydroxides, carbonates, and sulfides to react with impurities present in the solution to form precipitates that can settle easily. Chemicals introduced in the coagulation tank interact with molecules of dye to form easily removable precipitates (Mohan et al., 2004). The common procedures comprise: 1) the addition of appropriate chemicals into targeted pollutant-containing solutions, 2) precipitate will be formed after interaction of molecule of dye, 3) allow the suspension to settle (i.e. insoluble particles), and 4) separation of the sludge. The most common chemical precipitation method for dye removal is hydroxide precipitation (Ahmad et al., 2015). Vimonses et al. (2010) studied the mechanisms involved in the decolorization of Congo red dye. The removal was governed by combined physiochemical reactions of adsorption, ion-exchange, and precipitation. The precipitation contributed over 70% of total dye removal, followed by adsorption and ion-exchange (Vimonses et al., 2010). Sludge generation, the extra operational cost for sludge disposal, and high maintenance costs are major challenges in applying this process.
2.2 Complexion
Complex formation in the treatment process has been considered as a highly effective method for utilization to improve economical adsorbents for wastewater treatment. The hydrophobic dye can be removed from the aqueous solution through inclusion complex formation (Saifi et al., 2021). The potential of β-cyclodextrin (β-CD) was studied to remove highly toxic oil orange SS (OOSS) dye by complexion. This process needs the synthesis of complexes that can interact with the particular contaminant. The complexion mechanism may occur simultaneously with the ion-exchange mechanism (Kubra et al., 2021). Hisada et al. (2019) reported the occurrence of simultaneous mechanisms in the removal of Basic Orange 2 (BO2) by poly-γ-glutamic acid (PGA) in different pH ranges. Adsorption and complexation/precipitation have occurred in the pH range of 4-5 and hydrogen-bonded organic ammonium carboxylate salt complexes were formed (Hisada et al., 2019). When the pH was greater than 6, complexation/precipitation was significantly suppressed due to the neutral nature of the dye and van der Waals adsorption and hydrophobic interaction’s domination. Moreover, 99.5% removal was reported at pH = 5.
2.3 Ion-Exchange
The ion-exchange technique of wastewater treatment is categorized under widely used techniques. The process is based on the presence of ion-exchange resins that interact strongly with functional groups and charged dyes so that various dyes can be effectively removed from the aqueous solutions. The ion-exchange resins can be anion exchangers or cation exchangers (Ahmad et al., 2015). In the ion-exchange process, strong bonds are formed between solutes (dye molecules) and resins through an exchange of positive and negative ions. Conceptually, anionic (e.g., acid, mordant, reactive, direct, metal complexes) as well as cationic (basic) dyes, if treated with ion-exchange resins, should form complexes in the form of large flocs, able to be separated by filtration. Among different resins, quarternized cellulose and quarternized sugarcane bagasse were reported as viable ion-exchange resins capable of binding hydrolyzed reactive dyes (Singh and Arora, 2011). Several studies are reported in this area, for example, Acid orange 10 was removed from the environment by an Amberlite IRA 400 anion-exchange resin with up to 96.8% removal efficiency (Marin et al., 2019). Ion exchange dominates the mechanism of Congo red adsorption on the magnetic ion-exchange (MIEX) resin (Jia et al., 2020). In ion-exchange processes, there is no loss of adsorbent on regeneration, reclamation of solvent after use is possible and can remove soluble dyes effectively, but technology and organic solvent are expensive (Gnanadoss, 2013).
2.4 Membrane Technologies
Membrane filtration is an alternative physical treatment that can be used to remove the color from wastewater. It has some advantages such as appreciable resistance to temperature, good resistance to adverse chemical environment, and excellent performance of color removal (Roy et al., 2018). Also worthy of note are disadvantages, among which the one linked to the high cost of disposal of the remaining concentrated residue following the separation, the high management costs and the inability to deal with high flow rates, and frequent clogging of membrane pores by the dye molecules (Collivignarelli et al., 2019). Reverse osmosis and nanofiltration membrane systems effectively removed dyes such as acid red, reactive black, and reactive blue dyes as an alternative treatment method of wastewater discharged from Iraqi textile mills (Abid et al., 2012). However, Zhou et al. (2019) pointed out the limitation of using membrane technologies for treating dye-containing wastewater due to the short service life of the membrane and the ease to be pollution (Zhou et al., 2019).
2.4.1 Ultrafiltration
Ultrafiltration requires less pressure than nano-filtration and reverse osmosis (Shindhal et al., 2021). The success of ultrafiltration has been studied in the discoloration of reactive dyes with a removal efficiency of up to 90% (Erkanlı et al., 2017). In the other study, 98 and 100% color removal efficiencies have been obtained from real wastewater using ultrafiltration and Nanofiltration respectively (Collivignarelli et al., 2019). Ultrafiltration ceramic membrane was effectively decolorized Reactive Black dye solutions at different dye concentrations (Alventosa deLara et al., 2012).
Micellar-enhanced ultrafiltration employs surfactant micelles to solubilize inorganic and organic pollutants from the effluent stream and is subsequently filtered using an open membrane to restrict the micelle-pollutant complex in the permeate stream. Micelles being large can be removed together with the organic pollutants by a porous membrane. More than 90% removal efficiency, along with high throughput, can be attained by using pollutant-specific surfactant (or mixed surfactant system) and high permeability membrane, depending on the charge and other physical properties of the contaminants (Mondal et al., 2018).
2.4.2 Nanofiltration
The less energy consumption in NF (compared to RO) makes its use more frequent for the removal of various industrial effluents. The advantages of NF over other conventional separation processes include being less energy-intensive, can be operable under ambient temperature, having no phase change, and usually causing no damage to the species under processing (Mondal et al., 2018). Considering these advantages, the process can play a major role in replacing many of the conventional separation processes. The NF method was employed for the removal of dyes from solutions containing different dyes. The percentage removal of 93.77%, 95.67%, and 97% was reported for red, black, and blue dyes, respectively. The removal process was directly related to operating pressure, pH, TDS, and initial dye concentration, but not to the feed temperature (Abid et al., 2012).
The removal of different classes of dyes such as reactive, acidic, disperse, and direct dyes was studied by spiral-wound NF membrane in the thin composite film. The result reveals that color removal increased up to 98% as dye concentration increased for acidic and reactive blue dyes. Moreover, COD removal efficiencies were also found to be approximately 100% for reactive blue, disperse blue, direct, and disperse red dyes (Hassani et al., 2008).
2.4.3 Reverse Osmosis
Reverse osmosis is equally widely used in the textile industry as NF. This process has the potential to remove the dyes and allow the reuse of auxiliary chemicals for dyeing (Mondal et al., 2018). Dye concentration, solution pH, dissolved salts, temperature, and operating pressure on permeate flux and dye rejection affect the RO process. For example, the performance of RO membrane was reported with dye removal percentage of 97.2%, 99.58%, and 99.9% for acid red, reactive black, and reactive blue dyes, respectively, at an initial dye concentration of 65 mg/L, 39°C dye temperature, and 8 bar pressure (Abid et al., 2012).
2.4.4 Electrodialysis
The electrodialysis process is electrically driven, comprising appropriate ion-exchange membranes that are placed between the anode and cathode electrodes. This process provides high recovery of water and does not involve phase change and reaction as well as with no need for pressure and chemicals (Al-Amshawee et al., 2019). The ED technique was studied to remove methylene blue dye from simulated saline solutions. The effect of applied voltage, pH, initial dye concentration, and ionic strength on the removal performance was studied. Electrostatic interaction between ions of dye and the fixed charged groups of the ion-exchange membrane, and affinity toward interactions are the main factors in fouling (Lafi et al., 2019).
This technique can also be used in the treatment of textile or tannery dye to remove salts, COD, and color with the integration of ultrafiltration process (Lafi et al., 2018), electrocoagulation process (Deghles and Kurt, 2016), and photodegradation (Sindelar et al., 2015). Moreover, 97% of Indigo Carmine dye was removed by the ED process at various current intensities, conductivity, and pH of solutions (Caprarescu et al., 2016). Although the ED process has attractive advantages in contaminant removal, the following problems are reported such as scaling, membrane fouling, permselectivity, and high cost of electrodes (Peng and Guo, 2020; Xue et al., 2015).
2.5 Flotation Techniques
Flotation is a solid-liquid separation process, applicable to particles having a lower density than the solution, then the impurities floated on the top are removed by collectors. It involves the introduction of the transport medium (gas bubbles). The flotation technique was originally applicable in the mineral processing practices to extract minerals and particulate solids from water. The application of these techniques mainly comprises the treatment of heavy metals and dyes containing water and wastewater (Deliyanni et al., 2017; Gross et al., 2017). The efficiency of floatation techniques are dependent on the surface properties of different particles, pH value, temperature, current density, initial dye concentration, ionic strength, and stirring speed (El-Hosiny et al., 2017). This process can achieve effective treatment of dyes when combining with conventional coagulation, flotation, and electrochemistry in wastewater treatment (El-Hosiny et al., 2018; Hu et al., 2020; Tara et al., 2019). The flotation techniques can be divided into three processes: 1) dissolved air flotation (DAF) process, 2) ionic flotation, and 3) precipitate flotation. The generation of a bubble could be applied for techniques (Kyzas and Matis, 2018). The dissolved air flotation method has been commonly used in wastewater treatment (Al-Zoubi et al., 2015). Ion flotation removes surface-inactive ions from the solution by adding oppositely charged surfactants or collectors followed by introducing gas bubbles through the solutions (Deliyanni et al., 2017).
2.6 Advanced Oxidation Processes
Recently, advanced oxidation processes (AOPs) have been effectively investigated in the removal of a variety of contaminants from water and wastewater. Advanced oxidation processes (AOPs) involve the generation of oxidizing species such as hydroxyl radicals and sulfate radicals in sufficient quantity to interact with the organic compounds of the medium (Wang and Wang, 2020; Duan et al., 2020). AOPs comprise all the catalytic and noncatalytic processes that take advantage of the high oxidizing capacity of the hydroxyl radical (OH), and they differ from each other in the way in which this radical is generated (Cuerda-Correa et al., 2020). These processes are mainly based on the in-situ generation of the hydroxyl radical that reacts rapidly with most organic compounds. Thus, such a radical is generated in sufficient quantity to interact with organic compounds (Babu et al., 2019; Mokif, 2019).
Photolysis, O3-based processes (ozonation and catalytic ozonation), H2O2-based processes (Fenton, photo-Fenton), sonochemical oxidation, electrochemical oxidation, persulfate-based oxidation, wet air oxidation, and heterogeneous photocatalysis are the major advanced oxidation processes (Cuerda-Correa et al., 2020; Sharma and Feng, 2019; Miklos et al., 2018).
Several studies used advanced oxidation processes (AOPs) to treat different dyes in the wastewater stream. Zero-valent aluminum (ZVAl)-based AOPs were utilized to treat textile wastewater treatment. A color removal efficiency of 94.4% was achieved after the oxidation process (Khatri et al., 2018). Some of the other studies include: Fenton and photo-Fenton processes for the degradation of methylene blue with an efficiency of 65 and 83% respectively at pH of 3 and 30 min contact time (Gowtham and Pauline, 2021), combined sono-photo-electro-Fenton (SPEF) process for the removal of Acid Black 172 and Disperse Blue 56 with a removal efficiency of 97.4 and 95.5% respectively (Mahmoudi et al., 2021a), dark-Fenton process conditions for the removal of methylene blue and acid blue 29 with the removal efficiency of 82 and 95% respectively (Nasuha et al., 2021), electro-Fenton (EF) process and Ozone oxidation for the removal of Basic Blue 9 (BB9) dye with more than 97% removal efficiency (Bustos-Terrones et al., 2021), and electro-Fenton processes for the complete degradation of reactive red 195 (Elbatea et al., 2021) and more than 94% of neutral red (NR) dye (Ebratkhahan et al., 2021). Temperature, pH, the concentration of the oxidant, the initial concentration of dyes, current density, irradiation time, and reaction time affect the catalytic decomposition of dyes (Javaid and Qazi, 2019; Titchou et al., 2021; Mahmoudi et al., 2021b). Sludge production in the case of Fenton reagent, formation of toxic byproduct in the case of photocatalyst, and high cost due to the use of expensive reagents (for example, H2O2) and energy consumption (generation of O3 or UV radiation) are the major drawback of AOPs for dye removal (Cuerda-Correa et al., 2020; Moosavi et al., 2020).
2.7 Electrochemical Techniques
This treatment process is a comparatively novel technique, and usually does not require the consumption of chemicals (Roy and Saha, 2021). The anode continuously disintegrates as a result of electrical current flowing through it and produces in-situ cations, which acts as electro coagulants that attract the contaminants in the solution. The dyes attached to the electro coagulant either precipitate or flocculate, and thereby are separated. The principle of EC is based on three parameters: 1) the ionic charge, 2) particulate size, and 3) droplet (or vapor) spatial density. The generation of the charged agglomeration involves three stages: 1) coagulant formation by electrolytic disintegration of the anode, 2) destabilization of the suspension, and 3) aggregation to form the floating material (flocs). The EC process is successful in the removal of organic dyes, fine suspended particles, heavy metals, and oil and grease (or other heavier hydrocarbons) from diverse industrial effluents (Mondal et al., 2018). A report revealed that up to 94% of indigo dye could be decolorized at a pH of 7.5 using a continuous electrocoagulation process (Hendaoui et al., 2021). The limitation of this process includes the necessity of electrolytic apparatus, high-cost electricity, and less color removal for some dyes due to the higher flow rate.
2.8 Coagulation-Flocculation
Coagulation-flocculation is among the most widespread physio-chemical processes for the removal and distraction of coloring substances in wastewater. The removal of color by coagulation is strongly dependent on the structure of dye molecules (chromophores and auxochromes), so structural considerations of dyes are very important when choosing the most appropriate coagulant for dye removal (Mcyotto et al., 2021). The method can be separated into coagulation (destabilization of the colloidal suspensions by charge neutralization), flocculation, and sedimentation (physical processes that allow the smaller particles to be grouped so that they can be easily separated by sedimentation). The removal efficiency of color up to 99% has been reported for treating simulated water containing Brilliant green and Congo red (Collivignarelli et al., 2019). Moreover, many reports are accessible on dye removal by coagulation and flocculation from simulated and real wastewater. Magnesium chloride, lime, alum have been widely used coagulants in the process (Roy et al., 2018).
2.9 Adsorption
Adsorption is found to be a very effective, cheap, and commonly used method among all available dye removal techniques (Kandisa and Saibaba KV, 2016). Adsorption technique in wastewater treatment comprises removals of contaminants using commercial activated carbon and various available potentially low-cost adsorbents including biomass-based adsorbents. Several potential low-cost adsorbents have been studied in the application of wastewater treatment containing dyes (Figure 3) and widespread studies on dyes, their classification, and harmfulness in conjunction with various dye-containing wastewater treatment approaches, and adsorption characteristics of various nonconventional and cost-effective sustainable adsorbents were reported (Dawood and Sen, 2014; Mondal et al., 2018). The application of waste organic materials (e.g., compounds extracted from peels, leaves, barks) and microbial biomasses (fungus bio-sorbent, bacterial, and green algal biomasses) is gaining an increasing interest being effective, low-cost, and ecologically friendly sorbents. Moreover, newly discovered carbon nanomaterials (carbon nanotubes, graphene, and their derivatives) have been used for dye removal processes (Piaskowski et al., 2018).
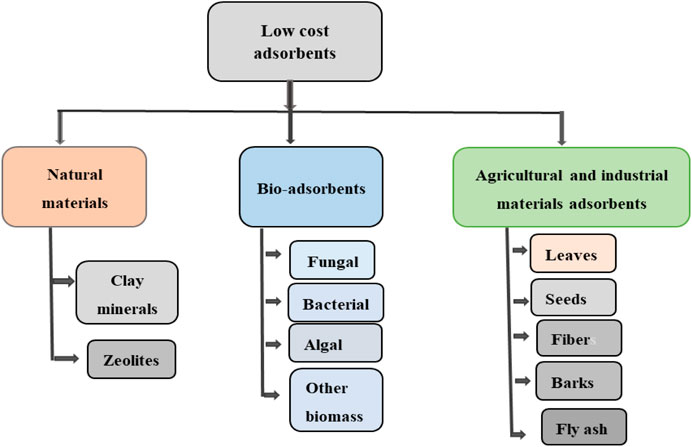
FIGURE 3. Various low-cost adsorbents potential for dye removal: adopted with modification from Siddiqui et al. (2018).
Activated carbon (AC) is a commonly used adsorbent that refers to a group of carbon materials with high oscillation and high internal surface which are unique because of their remarkable interior area, pore structure, high adsorption capacity, surface reactivity, and low cost compared to inorganic adsorbents such as zeolite (Hasanzadeh et al., 2020). Special processes for the production of activated carbons in powdered, granular, and spherical forms have so far been developed. Activated carbon can be commercially produced from pyrolysis of carbon or carbon-containing plant materials, such as coal, bamboo wood, charcoal, kernels, or fruit shells, such as coconut shells, and is subsequently activated (Heidarinejad et al., 2020; Yeow et al., 2021; Hassan and Carr, 2021).
There are three main processes for the activation of carbon which are steam activation, activation with carbon dioxide, chemical activation. Among the above three methods, steam activation is the best environmentally and economically viable option, while chemical activation results in the highest surface area and porosity (Rahimian and Zarinabadi, 2020). In the chemical activation process, the chemically active agents (such as phosphoric acid, KOH, H2SO4) are utilized initially to prepare the precursor followed by heat treatment up to 450–700°C. The charcoal is then washed with water to remove the acid from the carbon and dry.
The adsorption method is attractive and preferable, and carbonaceous adsorbents fit that purpose due to their high dye-binding capacity (Hassan and Carr, 2021). The performance of some commercial activated carbons (CAC) was evaluated for the removal of the Reactive Black dye from synthetic wastewaters (Table 2) (Giannakoudakis et al., 2016). The activated carbon samples were Norit Darco 12 × 20 (DARCO), Norit R008 (R008), and Norit PK 1–3 (PK13) with the maximum theoretical adsorption capacities 348, 527, 394 mg/g respectively at 25°C for all carbon samples. Moreover, Shu et al. (2017) modified the commercial activated carbon by loading copper metal and used it in the removal of dyes containing Rhodamine B, MB, Amaranth, Congo red, and Eosin-Y from the wastewater; they found that the adsorption capacity is better in MB than in Rhodamine B (Shu et al., 2017). Although the removal of dye using CAC is efficient, its cost is high for industrial applications. And some studies reported that the adsorption capacities of some biomass-based activated carbons prepared in the laboratory scale showed superior adsorption capacity than CAC; however, limited information is available on the application of biomass-based activated carbon at the industrial level. Coal-derived activated carbon was supposed to be the most efficient type of activated carbon in adsorption. The efficiency of treatment is 99.8%, and it can treat various types of dyes (Yeow et al., 2021). The capacity of removal of Reactive Red 120 was compared using Spirulina platensis (microalgae) and CAC. The batch adsorption system revealed that the maximum adsorption capacities of microalgae and CAC to dye were 482.2 and 267.2 mg/g respectively at pH of 2 and 25°C (Cardoso et al., 2012). The percentage removal of the dye using AC was 93.6–97.7%, while it was 94.4–99.0% using microalgae. In another study, the adsorption capacity for Remazol Red on CAC has higher than activated carbon prepared from olive stone (Uǧurlu et al., 2008). The substitution of CAC by emerging alternatives needs a detailed study like a method of activation and other desirable properties of adsorbents.
Several factors that affect dye adsorption capacity include initial dye concentration, pH, temperature, the dosage of adsorbents and their type, contact time, etc. For certain dye adsorption, the optimum parameters of each sorbent vary significantly (Yadav et al., 2021), the optimization of each factor is helpful to large-scale industrial applications and the understanding of the adsorption mechanism. For an efficient treatment process, adsorbent should have enough mechanical strength and can tolerate various conditions of wastewater, high adsorption amount, and rapid adsorption rate, effective to a variety of dyes or have selectivity for a certain pollutant, and easy to be regenerated and reused (Zhou et al., 2019).
Dyes from the industrial wastewater effluents are effectively removed by using activated carbon; however, it has some constraints such as capital cost, energy consumption, and loss of its ability after sorption-desorption cycles. To have effective removal of dyes, several economically available nonconventional adsorbents are required (Kandisa and Saibaba KV, 2016). Therefore, bioadsorbents obtained from fungal, bacterial, algal, and agricultural, or forest biomass are promising environmentally friendly adsorbents utilized for dye and heavy metal removal.
3 Bioadsorbents
Different technologies can be used for the treatment of wastewater containing dyes. Among them, biological methods are the most promising due to environmentally safe treatment capability (Przystaś et al., 2018; Batool and Valiyaveettil, 2021). A biological treatment especially biosorption using nonliving biomass is a relatively inexpensive way to remove dyes from the wastewater. The effective removal of dyes from the effluent depends on the unique surface chemistry with the presence of different functional groups in the cell wall of microorganisms (Karthik et al., 2016) such as alcohol, aldehydes, ketones, carboxylic, ether, phenolic, which make the bio-sorbents having a high affinity toward dye and attractive material for dye removal (Siddiqui et al., 2018). Biological materials including chitin, peat, chitosan, yeast, and fungi biomass are frequently used in the sorption of dye from the solution through the mechanism of chelation and complexion (Almeida and Corso, 2019).
A good adsorbent used in the removal of dye must have several desirable properties including large surface area, high adsorption capacity, large porosity, easy availability, stability, feasibility, compatibility, eco-friendly, ease of regeneration, and highly selective to remove a different variety of dyes (Nasar and Mashkoor, 2019). The pore volume of the bioadsorbents and functional groups of dyes are the deciding factors in achieving high dye adsorption. The presence of a large pore volume allows the binding of the highest number of dye molecules to the adsorbent (Hassan and Carr, 2021; Moosavi et al., 2020). Higher surface area, higher porosity, and low ash content lead to high adsorption capacity. Functional groups (hydroxyl, carboxyl, etc.) on the surface of biomass-based adsorbents are important properties determining the hydrophobicity or hydrophilicity of biochar as well as their adsorptive mechanism (Law et al., 2021).
The diversity of microbes consisting of different species of bacteria, fungi, yeast, and algae has been studied to remove dye molecules (Karthik et al., 2016). Besides the high sorption capacity toward dye, the dye removal performance can be improved by combining the biosorption process with the bio-degradation processes by living cells (Singh and Singh, 2017; Roy et al., 2018). Many factors affect the biomass biosorption capacities including pH, bio-sorbent dose, initial dye concentration, temperature, contact time (Pearce et al., 2003). Recently, the potential of bio-sorbents prepared from bacterial, fungal, algal, yeasts, forest biomasses, and agricultural and industrial wastes has been studied for treating both dye-containing simulated and field wastewater.
3.1 Adsorbents From Fungal Biomass
Fungal biomasses are composed of sugars, proteins, and lipids, and different functional groups (alcohols, carboxyl, and alkanes) which provide it certain properties and applications as a biosorption in treatments of wastewater (Isaza-Pérez et al., 2020; Ahmed and Ebrahim, 2020). Biotreatment of dye-containing wastewater effluent by fungal cell was provided a cost-effective, easily applicable, eco-friendly (Salem et al., 2019; (Argumedo-Delira et al., 2021), and absence of nutrient needs (Ghany et al., 2019). Different species of fungus have been used as an effective candidate for the removal of a variety of dyes from effluents such as Trichoderma sp. (Argumedo-Delira et al., 2021), Sarocladium sp. (Nouri et al., 2021), growing Rhizopus arrhizus (Gül, 2013), different varieties of white-rot fungi (Gnanadoss, 2013; Dai et al., 2018; Forgacs et al., 2004), Aspergillus niger (Salem et al., 2019; Asses et al., 2018), Aspergillus flavus (Mahmooda Takey, 2014), Lentinus concinnus (Bayramoglu and Yilmaz, 2018), Penicillium simplicissimum (Chen et al., 2020), Pycnoporus cinnabarinus, Pleurotus ostreatus and Trametes hirsute (Pecková et al., 2020), macro fungi spent mushroom waste (SMW) (Agaricus bisporus) (Ahmed and Ebrahim, 2020), Trichoderma harzianum (Karthik et al., 2020), and many others.
Bayramoglu and Yilmaz (2018) studied the removal of azo dye (Reactive Yellow 86 dye (RY-86)) using free (Lentinus concinnus) and immobilized fungal biomasses with polyvinyl alcohol/polyethylene oxide hydrogels (PVA/PEO) along with isotherms, kinetics, and thermodynamic studies (Bayramoglu and Yilmaz, 2018). They reported, maximum RY-86 dye adsorption for the free fungal and composite fungal biomasses was 190.2 and 87.6, respectively, using 200 mg/L initial dye concentration at pH 5.0 with 2.0 h contact time (Table 3). Moreover, the equilibrium data were well described with the Freundlich and Temkin isotherm models. The adsorption of RY-86 dye was fitted best by the pseudo-second-order kinetic model. The adsorption performance of fungal biomass is dependent on the chemical structure and surface charge of several dyes associated with the electrostatic interaction. From FTIR studies, it was observed that the carboxylate, hydroxyl, and amine groups were involved in the adsorption of the RY-86 dye. Similarly, Bayramoglu and Arica (2018) reported that the amine, carboxyl, and hydroxyl groups are involved in the adsorption of the Congo Red by iminodiacetic acid and triethylenetetramine modified fungal biomasses of Funalia trogii (Bayramoglu and Arica, 2018).
Fungal biomass (Trichoderma harzianum) was used to remove Reactive Red-3 (RR3) from an aqueous solution (Table 3). The biosorption mechanism revealed that the fitted kinetics (pseudo-second-order) suggested the adsorption mode of chemisorption in which the adsorbate (RR3 dye) is monopolized over the external layer of the biomass by electrostatic interaction (Karthik et al., 2020). After all the vacant spots are monopolized, the RR3 dye starts propagating into the adsorbent opening for further interactions.
Table 3 summarizes the application of fungal immobilization to dye removal. Most species of fungi potentially achieved more than 90% of a specific type of dye except Pleurotus ostreatus, Aspergillus flavus, and Diaporthe schini which had a removal efficiency of 53%, 53.62, and 87%, respectively. This variation might be due to the poor monitoring of experimental conditions, the processing of bioadsorbents, and the availability of functional groups that have a limited binding capacity with the dye molecule.
It has been observed that (Table 3) in low pH solutions, the removal rate of anionic dyes is increased while the removal rate of cationic dyes is lower. Conversely, a high pH solution increases the removal of the cationic dyes and causes a low removal percentage for anionic dyes (Ahmed and Ebrahim, 2020). The point of zero charges (pHpzc) is an essential parameter for understanding the mechanism and the favorability of the adsorption process. The pHpzc value indicates the type of active sites and the adsorption ability of adsorbents. When pH is greater than pHpzc, it is favorable for cationic dye adsorption due to the existence of functional groups (OH−, COO−), whereas anionic dye adsorption is favorable at pH less than pHpzc because the surfaces of adsorbents become positively charged (Zhou et al., 2019). In general, the use of fungal biomass is a promising substitute to the current technologies for dye decoloration and adsorption. In addition to optimizing the environmental parameters, considering the genotype and the preparation of the biomass are important for effective dye adsorption performance.
3.2 Adsorbents From Algae Biomass
Algae are considered one of the sources of the most favorable types of bio-sorbents because they have high biosorption capacity and are easily available in large amounts (Singh and Singh, 2017; Azam et al., 2020). The biosorption depends on the composition and structure of the algal cell wall, which is made up of several polysaccharides: xylan, mannan, alginic acid, chitin. These components, along with the proteins present, can provide acid-binding sites such as amino, amine, hydroxyl, imidazole, phosphate, and sulfate groups (Singh et al., 2018). Encapsulation and surface modification can be used as pretreatment methods to improve the adsorption capacity of algae. Citric acid-functionalized brown algae were reported to improve the adsorption capacity for textile dye (crystal violet) removal in aqueous solutions (Table 4). An optimum monolayer uptake capacity of 279.14 mg/g was reported for the modified algal adsorbent (Essekri et al., 2021). Moreover, electrostatic attractions and π-π interactions were responsible for the dye adsorption toward the algal biomass surface. Magnetically responsive brown algae (Sargassum horneri) was utilized for the adsorption of five water-soluble dyes. The magnetic modification leads to rapid and selective separation through an external magnetic field by using microwave-synthesized iron oxide nano and microparticles (Angelova et al., 2016). The sorbent had maximum sorption capacity for acridine orange (193.8 mg/g) but lower for malachite green (110.4 mg/g) at 2 h contact time.
Table 4 revealed that Spirulina and Chlorella species are common types of microalgae studied and achieved up to 99% removal efficiency. Similarly, Sargassum species of macro algae are commonly studied and can remove more than 90% of dyes. MB is among the common dyes removed by various dye species. The type of dye to be removed significantly depends on the pH of the solution. Anionic dyes are removed in acidic conditions and cationic dyes (e.g. MB) in alkaline conditions. This is due to the involvement of H+ ions in the biomass-pollutant interaction process. If the surface of the adsorbent possesses a positive charge, the hydrogen ions (H+) may compete productively with the cations present in the dye solution, resulting in a decrease in the amount of adsorbed dye. The carboxyl groups have a negative charge at a higher pH, which results in electrostatic binding of the cationic dyes. Moreover, the biosorption performance is strongly influenced by other parameters including the processing of biomass into adsorbent, initial contaminant concentration, biomass dosage, temperature, and contact time.
3.3 Adsorbents From Bacterial Biomass
The role of bacteria in bioremediation applications can be based on the adsorption of contaminants from aqueous media (Sarvajith et al., 2018) through different mechanisms using dead biomasses (Roy et al., 2018). Their small size, ubiquity, and capability to grow under varying environmental conditions make them good adsorbents (Singh et al., 2018). Bacterial species have been proved to be highly effective to treat wastewater containing reactive dyes by adsorption under optimized environmental conditions (San Keskin et al., 2015; Mishra and Maiti, 2018; Zabłocka-Godlewska et al., 2018). The bacterial dye decolorization rates vary with the type of bacteria, the reactivity of dye, and operational parameters such as temperature, pH, co-substrate, electron donor, and dissolved oxygen concentration. As shown in Table 5 the literature revealed that Pseudomonas spp. are relatively more successful to treat reactive dyes-containing wastewater (Mishra and Maiti, 2018; Eslami et al., 2016). Treatment of textile dyes using extremophiles can effectively be achieved. For example, 87% of Reactive Black and 85% of Reactive Red dyes were removed by haloalkaliphilic bacteria grown from textile wastewater (Seyedi et al., 2020).
Kim et al. (2015) isolated the bacterial strains (Bacillus catenulatus JB-022) from polluted ponds and soils for the biosorption of cationic dye. The isolate achieved 58% of cationic basic blue dye removal at the initial concentration of 2000 mg/L. The maximum sorption capacity for basic blue dye was found to be 139.74 mg/g from the Langmuir adsorption isotherm. The presence of carboxyl and phosphonate groups at the adsorbent surfaces can act as a potential surface functional groups capable of binding to cationic pollutants (Kim et al., 2015). Several functional groups on the Penaeus indicus biomass surface were likely involved in Acid Blue 25 dye binding, but the amino groups, alpha-chitin were by far the most important ones (Kousha et al., 2015). Bacillus subtilis had been immobilized in calcium alginate bead and utilized in batch and continuous reactor for the removal of MB. The kinetic study in the batch and continuous contactor gave greater than 90% removal (Upendar et al., 2016).
Different biocomposites have been reported for improved performance of dye removal (Chen et al., 2019; Hu et al., 2019). A bacterial strain (Clavibacter michiganensis) was studied for the removal of a reactive dye by joining with electrospun polycaprolactone and polylactic acid nanofibrous polymeric webs (Sarioglu et al., 2017b). The biocomposite was a promising material for the treatment of textile dyes with reusable and improvable properties. Moreover, electrospun nanofibrous-encapsulated bacterial cells were utilized for MB dye treatment (Sarioglu et al., 2017a). In another study, a design of a paper-like multifunctional purifier that has a biomass-based structure by embedding polyethyleneimine derived from ammonium compounds onto the substrate of bacterial cellulose was reported with higher adsorption capacities toward anionic dyes (including methyl orange, congo red, and methyl red) (Hu et al., 2019). Kang et al. (2020) used polysulfone and Escherichia coil biomass to prepare polysulfone Escherichia coil biomass composite fiber (PSBF) and they combined poly(acrylic acid) (PAA) at the surface of the composite. The adsorption performance of the prepared material on MB was studied by considering several parameters; they found an adsorption capacity of 225.2 mg/g at a pH of 7 fitted by Langmuir isotherm (Kang et al., 2020). Moreover, the regeneration ability of the composite was tested by desorption using an HCl-acidified solution at a pH of 2 as an eluent and the material can be reused at least 3 times.
Table 4 revealed that Langmuir isotherm was fitted by most biosorption studies, indicating that the monolayer coverage adsorption was the dominant process. Similarly, the pseudo-2nd -order kinetic model showed the best model fit. The adsorption involves valency forces through the sharing or exchange of electrons between the adsorbent and dye molecules by covalent forces and ion exchange (Mohd Nasir et al., 2021). At acidic pH maximum biosorption has been occurred in the case of metallic cations due to the involvement of the carboxyl functional group present in the bacterial cell walls, which is responsible for binding metal ions via different mechanisms. The fidelity of biosorption depends not only on the kind of ions but also on the type of bacterial species due to variation in their cell wall compositions (Roy et al., 2018). The presence of different anionic functional groups, gram-negative bacteria containing phospholipids, peptidoglycan, and lipopolysaccharides, and in the gram-positive bacteria including peptidoglycan, teichoic acids were responsible for the metal-binding capacity of the bacterial cell wall. Extracellular polysaccharides have also the ability to bind ions.
3.4 Adsorbents From Yeasts
Yeast is a single-celled organism, a member of the kingdom of fungi that have many favorable applications in the treatment of dye-containing solutions due to their high capacity to adsorb and accumulate pollutants, fast growth, faster decolorization than filamentous fungi, and the ability to survive unfavorable environments (Sen et al., 2016). The yeast surface consists of several functional groups including carboxyl hydroxide, polymer, amino, and phosphate, etc. that affect the desired pH of the solution to be analyzed (Singh et al., 2020).
Several studies revealed the bio-adsorption of different dyes using yeast biomass. Different species of yeast that could potentially remove different types of dyes are summarized in Table 6. Several factors including pH of the solution, the concentration of pollutants, and mass of yeast, temperature contact time influence the bio-sorption method (Al-Najar et al., 2021). For example, in the optimal condition of pH = 2, biomass dose = 2.0 g, and temperature = 30°C, complete decolorization of indigo dye was achieved by yeast (Diutina rugosa) biomass (Bankole et al., 2017). NADH-DCIP reductase and lignin peroxidase played a major role in the asymmetric cleavage, initial reduction, and deamination of indigo dye. Ramazole blue was also decolorized effectively by Baker’s yeast cells, but pH of the solution, bio-sorbent dosage, initial dye concentration, and contact times significantly affect the removal process (Mahmoud, 2016; Dil et al., 2017).
Ruscasso et al. (2021) investigated the biosorption of two reactive dyes (Reactive Blue 19 (RB 19) and Reactive Red 141 (RR 141)) using the Antarctic yeast (Debaryomyces hansenii F39A) with considering several factors. They found that 90% of RR 141 and 50% of RB 19 was adsorbed at pH 6.0, initial dye concentration of 100 mg/L, and 2 g/L biomass dose, but when the dosage of biomass was increased to 6 g/L the adsorption of RB 19 increased to 90%. Moreover, the adsorption process followed pseudo-second-order kinetics for each dye system and the Langmuir isotherm was the best fitted model (Ruscasso et al., 2021).
Semião et al. (2020) studied the adsorption performance of residual yeast and diatomaceous earth for the removal of Reactive Blue 160 dye (RB 160). They found that the two biosorbents had a dye removal capacity of 8.66 mg/g and 7.96 mg/g respectively at a pH of 2 (Semião et al., 2020). The positive charge in the biomass functional group is responsible for the chemical interaction with the anionic dye. Biosorption of yeast isotherm data was better fitted by the Freundlich isotherm model, but for diatomaceous earth, Langmuir isotherm was fitted indicating that the active sites of residual yeasts are more heterogeneous. In another research, the brewer’s yeast biomass was assessed as a biosorbent for removal of basic dyes (safranin O (SO), MB, and malachite green (MG)) from aqueous solutions within 1 h (Table 6). The adsorption kinetics of MB and MG followed pseudo-second-order, while SO followed pseudo-first-order. The maximum monolayer adsorption capacities of BYB for MB, MG, and SO were 212.05, 212.05, and 76.77 mg/g, respectively. Hydroxyl group, cyano group, and other functional groups are the major sources of adsorption by yeast (Lin et al., 2019). Table 6 revealed that most studies showed the best fit for Langmuir isotherm and pseudo-second-order kinetic models, suggesting the dominance of monolayer chemo-adsorption except some yeast species in which the Freundlich and Temkin isotherm models showed the best fit model.
3.5 Adsorbents From Forest or Agricultural Debris Wastes
The utilization of agricultural wastes and plants for the adsorptions of organic pollutants and inorganic pollutants is treated as an alternative to conventional wastewater treatment methods (Mo et al., 2018). Several studies have been conducted in the use of nonconventional, naturally occurring, low-cost biomass as adsorbents such as seeds, straw, fruit peels, leaves, bark, sawdust, ash, sludge, and others that are easily available. Different studies show that the dye adsorption abilities of those biomasses mainly depend on the types of dyes as well as the techniques of processing (Deniz and Kepekci, 2017); (Kharat, 2015).
Rice husk, sawdust, and bark are commonly used abundant, cheap, and low-value by-products for the preparation of adsorbent materials that are used in the removal of dye (Chuah et al., 2005); (Amirza et al., 2017). Sewu et al. (2017) prepared biochar from Korean cabbage (KC), rice straw, and wood chip and can be used as an alternative to activated carbon to remove congo red and CV (Sewu et al., 2017), but KC had better absorption performance than others. Potato peel biochar was considered a promising adsorbent for removing Cibacron Blue dye. The sorption ability of this waste follows the order: calcined > activated > native materials (Bouhadjra et al., 2021).
Activated carbon provided from Persea Americana was suggested for basic yellow dye removal from wastewater with 98% removal. This activated carbon can substitute the commercially available adsorbents (Regti et al., 2017). Pathania et al. (2013) synthesized Ficus carica bast (FCBAC) AC that showed an encouraging adsorption capacity for MB with a low amount of operating parameters such as an initial dye concentration of 0.5 g/L, sorbent dosage (5 g/L), contact time 1.3 h, and temperature (30°C) at a pH of 7.8 (Pathania et al., 2013). The isotherm models confirmed that the sorption is heterogeneous and occurred through physicochemical interactions.
Watermelon peels were used in the preparation of modified biochar with ozone initially and further modification with ammonium hydroxide and triethylenetetramine for the removal of Acid Yellow 11 (AY11) dye (El Nemr et al., 2020). The sorption study revealed that the capacity of prepared watermelon biochars was ranged between 76.94 and 462.18 mg/g at pH of 1.0, contact time of 3 h, and room temperature, and more than 96% removal of AY11 was achieved. Moreover, the isotherm model fitted Freundlich and Langmuir isotherm and the kinetics followed the pseudo-second-order model. Electrostatic interaction was responsible for the primary mechanism controlling the adsorption of AY11 dye onto the biochars. In another study, pea (Pisum sativum) peels were used to prepare biochar for the removal of Acid Orange 7 (AO7) dye with a similar modification process stated in the utilization of watermelon peels for AY11 removal. The adsorption capacity of the modified biochar was significantly enhanced from 78.18 to 523.12 mg/g at equilibrium. Up to 98% of AO7 was removed by triethylenetetramine-modified biochar, while 96% removal was achieved using unmodified biochar (El-Nemr et al., 2020).
Mandarin (Citrus reticulata) peel (MP) was utilized in the removal of SO from aqueous solution with the maximum adsorption capacity of 464 mg/g, 0.4 g/L adsorbent dose, 2 h contact time, and 84.75% removal. Hydrogen bonds, π-interactions, and electrostatic interactions were the possible adsorptive mechanisms of SO removal (Januário et al., 2021). Moreover, the desorption study revealed that the adsorption capacity was maintained more than 50% after four cycles.
Agricultural and forestry wastes are generally rich in cellulose, hemicellulose, and lignin. Its surface comprises several active groups including hydroxyl, carboxyl, amino, carboxyl, methyl, and so on (Zhou et al., 2019). These functional groups can adsorb dyes through mechanisms of complexation, hydrogen bonding, ion exchange, etc (Dai et al., 2018). Various agricultural and forest waste materials are summarized in Table 7 along with the biosorption capacity and the required reagents to activate them. Different acids have been used to activate the bio-sorbents to enhance the binding sites, the chemistry of the aqueous solution, to improve porosity and specific surface area. For example, phosphoric acid stimulates bond cleavage in agricultural waste biomass to increase the carbon yield (Bello et al., 2019). Phosphoric acid promoted dye biosorption by grafting phosphate functions onto the biomass and enhancing the acid functions involved in dye fixation (Benabbas et al., 2021). Moreover, H2SO4, NaOH, and KOH are the common activating agents during the preparation of agricultural waste-based bioadsorbents (Table 7).
Several environmental conditions including adsorbent dosage, temperature, contact time, pH of the solution, the particle size of the plant-based adsorbent, agitation, and initial dye concentration significantly affect the biosorption process. The pH of the solution affects both the chemistry of the aqueous solution and the binding sites present on the surface of adsorbents (Kumar et al., 2021).
4 Cost Analysis of Biomass-Based Adsorbents
Several authors reported that the cost of bioadsorbents prepared from microbes and forest and agricultural waste is low as compared with conventional treatment technologies, although they did not incorporate cost-benefit analysis in their study. The advantages of biosorption are related to the nature of adsorbent material used in the adsorption processes of dyes. To be economical, the availability of a large quantity of adsorbent material and the ease of preparation or processing, and the requirements of activation are significantly important along with the concepts of green chemistry (Bulgariu et al., 2019). The cost of forest wastes is only associated with the transport cost from the storage place to the site where they will be utilized (Adegoke and Bello, 2015). Some authors argue that the naming of “low-cost adsorbents” actually only indicates their original prices so that their local availability, transportation, treatment process, and both recycle and lifetime issues, and the processes of regeneration and treatment should be critically investigated (Zhou et al., 2019). Unfortunately, most studies on biomass-based adsorption have been conducted on the laboratory scale using simulated wastewater, so that the cost analysis of the biomass-based adsorbents is limited, particularly for microbial biomass.
A comprehensive cost analysis was carried out for the biochars made from agro-waste (Coconut shell, Groundnut Shell, and Rice husk) for their adsorption performance of the Basic Red 09 dye removal from wastewater. It was estimated that the cost of 1 g of adsorbent is ₹4.54, ₹0.91, and ₹0.97 for Coconut shell, Groundnut shell, and Rice husk, respectively, by considering operational costs including manufacturing costs, maintenance costs, feedstock costs, transportation costs, labor costs, and the distribution costs (Praveen et al., 2021). Groundnut shell-based biochar had maximum adsorption capacity (46.3 mg/g) as well as the least cost (₹0.91) per unit gram of Basic Red 09 dye removal. Bello et al. (2019) prepared phosphoric acid-functionalized locust bean pod AC for the removal of RhB dye and studied the preliminary cost analysis of this adsorbent. They concluded that the AC prepared from this plant material was approximately six times cheaper than conventional AC. Phosphoric acid and deionized water contribute most of the cost (Bello et al., 2019).
5 Regeneration of Bioadsorbents
After the adsorption process is accomplished, the desorption process is of great importance to regenerate and reuse those used adsorbents and to reduce the generation of waste as well as to keep the process price down (Zhou et al., 2019). The common desorption methods include thermal treatment, acid (H2SO4, HNO3, HCl, H3PO4, NaOH) treatment, organic solvents (methanol and ethanol), biological methods, and vacuum methods (Hassan and Carr, 2021). In the case of the solvent desorption process, the eluent (dye-saturated substrate) is mixed with the appropriate solvent to extract the dye and then separate the adsorbent by filtration. The dye-solvent mixture is dried to evaporate the solvent (Zhou et al., 2019).
The regeneration process should be cost-effective and should have potentially low energy consumption. Some authors stated that the recovery step of powder-activated carbons is very costly, time-consuming, and not very efficient so that they are not suitable for industrial applications (Moosavi et al., 2020), and more particularly reusability is almost impossible for low-cost products (Saha et al., 2020). However, some studies report the regeneration of biomass-based adsorbents (Essekri et al., 2021; Elgarahy et al., 2019; Kang et al., 2020; Pathania et al., 2013; Yang et al., 2021). Saha et al. (2020) studied Congo red loaded java citronella (Cymbopogon winterianus) based bio-adsorbent and commercial AC regeneration using ethanol. They found 90.3 and 83.7% desorption for biomass and commercial AC, respectively, from dye-loaded adsorbents (Saha et al., 2020). Moreover, the adsorbents were effective up to two adsorption-desorption cycles. In another study, the adsorption capacity of biosorbent prepared from mandarin (Citrus reticulata) peels was maintained more than 50% after four cycles (from 77.90 to 41.55 mg/g) during the removal of Safranin orange (SO) dye. HCl was the best chamical that had a good performance during desorption (Januário et al., 2021). Dahiru et al. (2018) reported the efficiency of the banana peel-based adsorbent for the removal of Malachite green (MG) and Methylene blue (MB) decreased from 100 to 64% and 76% after 5 consecutive usages respectively (Dahiru et al., 2018).
6 Biosorbent Prospects for Dye Adsorption
Various adsorbents can be prepared, modified, and applied to different contaminants in the environment. The preparation process for the activation and modification of adsorbents determines their characteristics. In general, the most critical characteristics of a good adsorbent are surface area, void active sites, and reproducibility in the activation processes (Leng et al., 2021). Among all the adsorbents, the biomass-derived adsorbent is preferred comparatively having the highest surface area, regardless of the activation techniques, depending on source types resulted in increasing the adsorption of the contaminants.
Among the adsorbents, bioadsorbents are the most popular for the adsorption of dyes, contaminants in general, from wastewater due to their versatility, cost-effectiveness. Also, their byproducts are not hazardous, and in addition to easy operation. Moreover, the biomass-derived adsorbents are commonly cellulose-based structures and can produce a good porous carbon that can adsorb various types of contaminants in the natural environment. One of the important features for the use of biomass-derived adsorbent for removal of dyes is that it can be used on a large scale because of its huge availability. Also, biomass-derived adsorbents have high affinity/binding capacity for various types of contaminants, and also have good regeneration capacities. In addition, their good surface chemistry such as pore distribution, specific surface area, and functional groups helps for the removal of dyes in the wastewater (Sherugar et al., 2022).
However, environmental factors such as temperature, pH, ionic strength, nutrient availability, photodegradation through natural light, which can arise in some seasonal and temporal variations, may affect structural characteristics and chemical constituents of the biomass. Thus, this can strongly affect the real adsorption capacity of biomass-based adsorbents. As a result, the biomass-derived adsorbents should be well characterized to understand the adsorption mechanisms for certain contaminants. In addition, studies should be conducted in a multi-pollutant system to meet the requirements of real wastewater treatment containing diversified contaminants that can assure stability resulted in the suitability of biomass for the practical biosorption process (Zhou et al., 2019).
The other most significant constraint for utilizing biomass-derived adsorbents for dye removal is the scaling up and cost analysis. Although there are several available low-cost adsorbents, their processing and improvements (activation, cross-linking, drying, autoclaving, etc.), modification, and transportation cost should be considered to improve the selectivity of a certain pollutant with the corresponding sorption capacity. In addition, the recovery or regeneration procedure may also need a significant amount of energy and solvents depending on the activation processes used. After the dye-removal from wastewater, the effective utilization, and handling of the adsorbent and the eluent containing the contaminants more concentrate is important. The major solution for this management is the reuse and regeneration of the adsorbent several times using efficient regenerating agents (Patel, 2021). After complete exhaustion of the adsorbents, dumping, storing, or disposing of are options but safe disposal is necessary (Vakili et al., 2019). The potential solutions for safely disposing of the final effluent include stabilization and immobilization, for example, using a cement-based system as a binding agent (Dey et al., 2017). Consequently, difficulties are faced in the comparison among them. This indicates the necessity for more researches that can compare the superiority of an effective dye removal technique over the other.
7 Conclusion and Recommendation
The review gave a general overview on the removal and degradation of dyes from wastewater using conventional removal technologies and biomass-based adsorbents with particular emphasis on the various bio-adsorbents such as biomasses from fungi, bacteria, algae, yeast, forest, and agricultural wastes. The review reveals that the conventional dye treatment methods have some particular limitations related to operation efficiency, total cost, energy, and generation of toxic by-products, although they have good performances to a particular pollutant. The adsorption method is a good water and wastewater treatment process due to its suitability, ease of operation, and simplicity of design. Among different available adsorbents, biomass-based treatments are found to be the recent materials employed at various sectors to remove dyes and other pollutants. Distinctive surface chemistry with the incorporation of several functional groups (alcohol, ketones, aldehydes, carboxylic, ether, phenol) makes those bio-sorbents with a high attraction toward dye and makes the biomass-based adsorbents effective material for dye removal. The bio-sorption process is affected by various environmental parameters such as pH, initial dye concentration, temperature, contact time, and adsorbent dose as well as the ways of the preparations of materials. Although most biomasses achieved a good removal efficiency, each study reviewed has considered different parameters for efficiency determination. Thus, making the comparison among them difficult, most parts of each biomass reviewed show a remarkable removal efficiency of up to 99%. More studies are important that can compare and define the superiority of biomass over the other. Basic dyes (particularly MB and CV) are among frequently studied types of dye by most biomass-based adsorbents reviewed, of which 83–99% removal efficiency is reported.
Most of the studies on biomass-based adsorption concentrated on the removal of a single pollutant, however, in real dye-containing wastewater, like textile industries that usually comprise a mixture of many dyes. For practical applications, further studies should be conducted in the mixed and multi-pollutants system to satisfy the requirements of wastewater treatment. The adsorbent’s stability and the cost of adsorbents are also essential parameters that can determine the suitability of the adsorbent for practical applications. Most of the reported researches are limited to laboratory assessments of adsorption capacity, while the cost analyses are neglected, all factors are needed to be considered such as the local availability, transportation, treatment process, and both recycle and lifetime issues. Desorption study is also an essential activity to keep the process price down, recover the adsorbed compounds, and minimize the amount of waste.
Author Contributions
TA: Review contents construction, Supervising, Writing—review and editing, Proofreading. FB: Writing—First draft, Formal analysis, Graphics, and synthesis.
Conflict of Interest
The authors declare that the research was conducted in the absence of any commercial or financial relationships that could be construed as a potential conflict of interest.
Publisher’s Note
All claims expressed in this article are solely those of the authors and do not necessarily represent those of their affiliated organizations, or those of the publisher, the editors and the reviewers. Any product that may be evaluated in this article, or claim that may be made by its manufacturer, is not guaranteed or endorsed by the publisher.
Acknowledgments
The authors thank their expertise for their constructive comments and proofreads. Also, the authors thank the researchers and/or scientists, and organizations for their contribution in the field, and apologize if any fault on the scientific papers or do not adequately acknowledge the scientific papers.
References
Abid, M. F., Zablouk, M. A., and Abid-Alameer, A. M. (2012). Experimental Study of Dye Removal from Industrial Wastewater by Membrane Technologies of Reverse Osmosis and Nanofiltration. J. Environ. Health Sci. Engineer 9, 1. doi:10.1186/1735-2746-9-17
Adegoke, K. A., and Bello, O. S. (2015). Dye Sequestration Using Agricultural Wastes as Adsorbents. Water Resour. Industry 12, 8–24. doi:10.1016/j.wri.2015.09.002
Afroze, S., and Sen, T. K. (2018). A Review on Heavy Metal Ions and Dye Adsorption from Water by Agricultural Solid Waste Adsorbents. Water Air Soil Pollut. 229, 1–50. doi:10.1007/s11270-018-3869-z
Ahmad, A., Mohd-Setapar, S. H., Chuong, C. S., Khatoon, A., Wani, W. A., Kumar, R., et al. (2015). Recent Advances in New Generation Dye Removal Technologies: Novel Search for Approaches to Reprocess Wastewater. RSC Adv. 5, 30801–30818. doi:10.1039/c4ra16959j
Ahmad, M. A., Afandi, N. S., and Bello, O. S. (2017). Optimization of Process Variables by Response Surface Methodology for Malachite green Dye Removal Using Lime Peel Activated Carbon. Appl. Water Sci. 7, 717–727. doi:10.1007/s13201-015-0284-0
Ahmad, T., Rafatullah, M., Ghazali, A., Sulaiman, O., and Hashim, R. (2011a). Oil Palm Biomass-Based Adsorbents for the Removal of Water Pollutants-A Review. J. Environ. Sci. Health C 29, 177–222. doi:10.1080/10590501.2011.601847
Ahmad, T., Rafatullah, M., Ghazali, A., Sulaiman, O., and Hashim, R. (2011b). Oil Palm Biomass-Based Adsorbents for the Removal of Water Pollutants-A Review. J. Environ. Sci. Health Part C 29, 177–222. doi:10.1080/10590501.2011.601847
Ahmed, H. A. B., and Ebrahim, S. E. (2020). Removal of Methylene Blue and congo Red Dyes by Pretreated Fungus Biomass-Equilibrium and Kinetic Studies. J. Adv. Res. Fluid Mech. Therm. Sci. 66, 84–100.
Al-Amshawee, S., Yunus, M. Y. B. M., Azoddein, A. A. M., Hassell, D. G., Dakhil, I. H., and Hasan, H. A. (2020). Electrodialysis Desalination for Water and Wastewater: A Review. Chem. Eng. J. 380, 122231–122254. doi:10.1016/j.cej.2019.122231
Al-Najar, J. A., Lutfee, T., and Alwan, N. F. (2021). The Action of Yeast as an Adsorbent in Wastewater Treatment: A Brief Review. IOP Conf. Ser. Earth Environ. Sci. 779, 012054. doi:10.1088/1755-1315/779/1/012054
Al-Zoubi, H., Ibrahim, K. A., and Abu-Sbeih, K. A. (2015). Removal of Heavy Metals from Wastewater by Economical Polymeric Collectors Using Dissolved Air Flotation Process. J. Water Process Eng. 8, 19–27. doi:10.1016/j.jwpe.2015.08.002
Almeida, E. J. R., and Corso, C. R. (2019). Decolorization and Removal of Toxicity of Textile Azo Dyes Using Fungal Biomass Pelletized. Int. J. Environ. Sci. Technol. 16, 1319–1328. doi:10.1007/s13762-018-1728-5
Alventosa-deLara, E., Barredo-Damas, S., Alcaina-Miranda, M. I., and Iborra-Clar, M. I. (2012). Ultrafiltration Technology with a Ceramic Membrane for Reactive Dye Removal: Optimization of Membrane Performance. J. Hazard. Mater. 209-210, 492–500. doi:10.1016/j.jhazmat.2012.01.065
Amirza, M. A. R., Adib, M. M. R., and Hamdan, R. (2017). Application of Agricultural Wastes Activated Carbon for Dye Removal - an Overview. MATEC Web Conf. 103, 06013–06034. doi:10.1051/matecconf/201710306013
Amreen, S., Dash, S. K., Mohanty, L., and Sahoo, S. (2020). Fast and Efficient Elimination of Malachite Green Dye by Activated Carbon Derived from Fresh Water Micro Algae : Kinetics and Thermodynamic Studies. Bhubaneshwar, Odisha: Test engineering management, 2581–2593.
Angelova, R., Baldikova, E., Pospiskova, K., Maderova, Z., Safarikova, M., and Safarik, I. (2016). Magnetically Modified Sargassum Horneri Biomass as an Adsorbent for Organic Dye Removal. J. Clean. Prod. 137, 189–194. doi:10.1016/j.jclepro.2016.07.068
Aragaw, T. A., and Angerasa, F. T. (2020). Synthesis and Characterization of Ethiopian Kaolin for the Removal of Basic Yellow (BY 28) Dye from Aqueous Solution as a Potential Adsorbent. Heliyon 6, e04975. doi:10.1016/j.heliyon.2020.e04975
Aragaw, T. A. (2020). Recovery of Iron Hydroxides from Electro-Coagulated Sludge for Adsorption Removals of Dye Wastewater: Adsorption Capacity and Adsorbent Characteristics. Surf. Inter. 18, 100439. doi:10.1016/j.surfin.2020.100439
Argumedo-Delira, R., Gómez-Martínez, M. J., and Uribe-Kaffure, R. (2021). Trichoderma Biomass as an Alternative for Removal of congo Red and Malachite green Industrial Dyes. Appl. Sci. 11, 448–515. doi:10.3390/app11010448
Asses, N., Ayed, L., Hkiri, N., and Hamdi, M. (20182018). Congo Red Decolorization and Detoxification by Aspergillus Niger: Removal Mechanisms and Dye Degradation Pathway. Biomed. Res. Int. 2018, 1–9. doi:10.1155/2018/3049686
Astuti, W., Sulistyaningsih, T., Kusumastuti, E., Thomas, G. Y. R. S., and Kusnadi, R. Y. (2019). Thermal Conversion of Pineapple crown Leaf Waste to Magnetized Activated Carbon for Dye Removal. Bioresour. Tech. 287, 121426. doi:10.1016/j.biortech.2019.121426
Azam, R., Kothari, R., Singh, H. M., Ahmad, S., Ashokkumar, V., and Tyagi, V. V. (2020). Production of Algal Biomass for its Biochemical Profile Using Slaughterhouse Wastewater for Treatment under Axenic Conditions. Bioresour. Tech. 306, 123116. doi:10.1016/j.biortech.2020.123116
Babu, D. S., Srivastava, V., Nidheesh, P. V., and Kumar, M. S. (2019). Detoxification of Water and Wastewater by Advanced Oxidation Processes. Sci. Total Environ. 696, 133961. doi:10.1016/j.scitotenv.2019.133961
Bankole, P. O., Adekunle, A. A., Obidi, O. F., Olukanni, O. D., and Govindwar, S. P. (2017). Degradation of Indigo Dye by a Newly Isolated Yeast, Diutina Rugosa from Dye Wastewater Polluted Soil. J. Environ. Chem. Eng. 5, 4639–4648. doi:10.1016/j.jece.2017.08.050
Batool, A., and Valiyaveettil, S. (2021). Chemical Transformation of Soya Waste into Stable Adsorbent for Enhanced Removal of Methylene Blue and Neutral Red from Water. J. Environ. Chem. Eng. 9, 104902. doi:10.1016/J.JECE.2020.104902
Bayazıt, G., Tastan, B. E., and Ülküye, D. G. (2019). Biosorption, Isotherm and Kinetic Properties of Common Textile Dye by Phormidium Animale. Glob. NEST Journa 21, 1–7.
Bayramoglu, G., and Arica, M. Y. (2018). Adsorption of Congo Red Dye by Native Amine and Carboxyl Modified Biomass of Funalia Trogii: Isotherms, Kinetics and Thermodynamics Mechanisms. Korean J. Chem. Eng. 35, 1303–1311. doi:10.1007/s11814-018-0033-9
Bayramoglu, G., and Yilmaz, M. (2018). Azo Dye Removal Using Free and Immobilized Fungal Biomasses: Isotherms, Kinetics and Thermodynamic Studies. Fibers Polym. 19, 877–886. doi:10.1007/s12221-018-7875-y
Bello, O. S., Adegoke, K. A., Olaniyan, A. A., and Abdulazeez, H. (2015). Dye Adsorption Using Biomass Wastes and Natural Adsorbents: Overview and Future Prospects. Desalination Water Treat. 53, 1–24. doi:10.1080/19443994.2013.862028
Bello, O. S., Adegoke, K. A., Sarumi, O. O., and Lameed, O. S. (2019). Functionalized Locust Bean Pod (Parkia Biglobosa) Activated Carbon for Rhodamine B Dye Removal. Heliyon 5, e02323. doi:10.1016/j.heliyon.2019.e02323
Benabbas, K., Zabat, N., and Hocini, I. (2021). Study of the Chemical Pretreatment of a Nonconventional Low-Cost Biosorbent (Callitriche Obtusangula) for Removing an Anionic Dye from Aqueous Solution. Euro-mediterr J. Environ. Integr. 6, 1–17. doi:10.1007/s41207-021-00265-4
Bhatia, D., Sharma, N. R., Singh, J., and Kanwar, R. S. (2017). Biological Methods for Textile Dye Removal from Wastewater: A Review. Crit. Rev. Environ. Sci. Tech. 47, 1836–1876. doi:10.1080/10643389.2017.1393263
Bouhadjra, K., Lemlikchi, W., Ferhati, A., and Mignard, S. (2021). Enhancing Removal Efficiency of Anionic Dye (Cibacron Blue) Using Waste Potato Peels Powder. Sci. Rep. 11, 1–10. doi:10.1038/s41598-020-79069-5
Bulgariu, L., Escudero, L. B., Bello, O. S., Iqbal, M., Nisar, J., Adegoke, K. A., et al. (2019). The Utilization of Leaf-Based Adsorbents for Dyes Removal: A Review. J. Mol. Liquids 276, 728–747. doi:10.1016/j.molliq.2018.12.001
Bustos-Terrones, Y. A., Hermosillo-Nevárez, J. J., Ramírez-Pereda, B., Vaca, M., Rangel-Peraza, J. G., Bustos-Terrones, V., et al. (2021). Removal of BB9 Textile Dye by Biological, Physical, Chemical, and Electrochemical Treatments. J. Taiwan Inst. Chem. Eng. 121, 29–37. doi:10.1016/j.jtice.2021.03.041
Canizo, B. V., Agostini, E., Wevar Oller, A. L., Dotto, G. L., Vega, I. A., and Escudero, L. B. (2019). Removal of Crystal Violet from Natural Water and Effluents through Biosorption on Bacterial Biomass Isolated from Rhizospheric Soil. Water Air Soil Pollut. 230, 1–14. doi:10.1007/s11270-019-4235-5
Caprarescu, S., Miron, A. R., Purcar, V., Radu, A.-L., Sarbu, A., Ion-Ebrasu, D., et al. (2016). Efficient Removal of Indigo Carmine Dye by a Separation Process. Water Sci. Technol. 74, 2462–2473. doi:10.2166/wst.2016.388
Cardoso, N. F., Lima, E. C., Royer, B., Bach, M. V., Dotto, G. L., Pinto, L. A. A., et al. (2012). Comparison of Spirulina Platensis Microalgae and Commercial Activated Carbon as Adsorbents for the Removal of Reactive Red 120 Dye from Aqueous Effluents. J. Hazard. Mater. 241-242, 146–153. doi:10.1016/j.jhazmat.2012.09.026
Castro, K. C. d., Cossolin, A. S., Reis, H. C. O. d., and Morais, E. B. d. (2017). Biosorption of Anionic Textile Dyes from Aqueous Solution by Yeast Slurry from Brewery. Braz. Arch. Biol. Technol. 60, 1–13. doi:10.1590/1678-4324-2017160101
Chen, S. H., Cheow, Y. L., Ng, S. L., and Ting, A. S. Y. (2020). Removal of Triphenylmethane Dyes in Single-Dye and Dye-Metal Mixtures by Live and Dead Cells of Metal-Tolerant Penicillium simplicissimum. Sep. Sci. Tech. 55, 2410–2420. doi:10.1080/01496395.2019.1626422
Chen, X., Cui, J., Xu, X., Sun, B., Zhang, L., Dong, W., et al. (2020). Bacterial Cellulose/attapulgite Magnetic Composites as an Efficient Adsorbent for Heavy Metal Ions and Dye Treatment. Carbohydr. Polym. 229, 115512–115525. doi:10.1016/j.carbpol.2019.115512
Chin, J. Y., Chng, L. M., Leong, S. S., Yeap, S. P., Yasin, N. H. M., and Toh, P. Y. (2020). Removal of Synthetic Dye by Chlorella Vulgaris Microalgae as Natural Adsorbent. Arab. J. Sci. Eng. 45, 7385–7395. doi:10.1007/s13369-020-04557-9
Chuah, T. G., Jumasiah, A., Azni, I., Katayon, S., and Thomas Choong, S. Y. (2005). Rice Husk as a Potentially Low-Cost Biosorbent for Heavy Metal and Dye Removal: An Overview. Desalination 175, 305–316. doi:10.1016/j.desal.2004.10.014
Collivignarelli, M. C., Abbà, A., Carnevale Miino, M., and Damiani, S. (2019). Treatments for Color Removal from Wastewater: State of the Art. J. Environ. Manage. 236, 727–745. doi:10.1016/j.jenvman.2018.11.094
Cuerda-Correa, E. M., Alexandre-Franco, M. F., and Fernández-González, C. (2020). Advanced Oxidation Processes for the Removal of Antibiotics from Water. An Overview. An overviewWater 12, 102–157. doi:10.3390/w12010102
da Rosa, A. L. D., Carissimi, E., Dotto, G. L., Sander, H., and Feris, L. A. (2018). Biosorption of Rhodamine B Dye from Dyeing Stones Effluents Using the green Microalgae Chlorella Pyrenoidosa. J. Clean. Prod. 198, 1302–1310. doi:10.1016/j.jclepro.2018.07.128
Dahiru, M., Zango, Z. U., and Haruna, M. A. (2018). Cationic Dyes Removal Using Low-Cost Banana Peel Biosorbent. Am. J. Mater. Sci. 8, 32–38. doi:10.5923/j.materials.20180802.02
Dai, Y., Sun, Q., Wang, W., Lu, L., Liu, M., Li, J., et al. (2018). Utilizations of Agricultural Waste as Adsorbent for the Removal of Contaminants: A Review. Chemosphere 211, 235–253. doi:10.1016/j.chemosphere.2018.06.179
Dawood, S., and Sen, T. K. (2014). Review on Dye Removal from its Aqueous Solution into Alternative Cost Ef-Fective and Non-conventional Adsorbents. J. Chem. Proc. Eng. 1, 1–11.
Deghles, A., and Kurt, U. (2016). Treatment of Tannery Wastewater by a Hybrid Electrocoagulation/electrodialysis Process. Chem. Eng. Process. Process Intensification 104, 43–50. doi:10.1016/j.cep.2016.02.009
Deliyanni, E. A., Kyzas, G. Z., and Matis, K. A. (2017). Various Flotation Techniques for Metal Ions Removal. J. Mol. Liquids 225, 260–264. doi:10.1016/j.molliq.2016.11.069
Deniz, F., and Kepekci, R. A. (2017). Bioremoval of Malachite green from Water Sample by Forestry Waste Mixture as Potential Biosorbent. Microchemical J. 132, 172–178. doi:10.1016/j.microc.2017.01.015
Dey, M. D., Das, S., Kumar, R., Doley, R., Bhattacharya, S. S., and Mukhopadhyay, R. (2017). Vermiremoval of Methylene Blue Using Eisenia fetida : A Potential Strategy for Bioremediation of Synthetic Dye-Containing Effluents. Ecol. Eng. 106, 200–208. doi:10.1016/j.ecoleng.2017.05.034
Dil, E. A., Ghaedi, M., Ghezelbash, G. R., and Asfaram, A. (2017). Multi-responses Optimization of Simultaneous Biosorption of Cationic Dyes by Live Yeast Yarrowia Lipolytica 70562 from Binary Solution: Application of First Order Derivative Spectrophotometry. Ecotoxicology Environ. Saf. 139, 158–164. doi:10.1016/j.ecoenv.2017.01.030
Drumm, F. C., Grassi, P., Georgin, J., Tonato, D., Pfingsten Franco, D. S., Chaves Neto, J. R., et al. (2019). Potentiality of the Phoma Sp. Inactive Fungal Biomass, a Waste from the Bioherbicide Production, for the Treatment of Colored Effluents. Chemosphere 235, 596–605. doi:10.1016/j.chemosphere.2019.06.169
Duan, X., Yang, S., Wacławek, S., Fang, G., Xiao, R., and Dionysiou, D. D. (2020). Limitations and Prospects of Sulfate-Radical Based Advanced Oxidation Processes. J. Environ. Chem. Eng. 8, 103849–103916. doi:10.1016/j.jece.2020.103849
Ebratkhahan, M., Naghash Hamed, S., Zarei, M., Jafarizad, A., and Rostamizadeh, M. (2021). Removal of Neutral Red Dye via Electro-Fenton Process: A Response Surface Methodology Modeling. Electrocatalysis 12, 579–594. doi:10.1007/s12678-021-00640-3
El-Hosiny, F., Abdeldayem AbdelKhalek, M., Selim, K., Osama, I., Abdelkhalek, M. A., Selim, K., et al. (2017). A Designed Electro-Flotation Cell for Dye Removal from Wastewater. J. Appl. Res. Ind. Eng. 4, 133–147. doi:10.22105/jarie.2017.100801.1021
El-Hosiny, F. I., Abdel-Khalek, M. A., Selim, K. A., and Osama, I. (2018). Physicochemical Study of Dye Removal Using Electro-Coagulation-Flotation Process. Physicochem. Probl. Miner. Process. 54, 321–333. doi:10.5277/ppmp1825
El-Nemr, M. A., Abdelmonem, N. M., Ismail, I. M. A., Ragab, S., and El Nemr, A. (2020). The Efficient Removal of the Hazardous Azo Dye Acid Orange 7 from Water Using Modified Biochar from Pea Peels. Dwt 203, 327–355. doi:10.5004/dwt.2020.26190
El-Nemr, M. A., Abdelmonem, N. M., Ismail, I. M. A., Ragab, S., and El-Nemr, A. (2020). Removal of Acid Yellow 11 Dye Using a Novel Modified Biochar Derived from Watermelon Peels. Dwt 203, 403–431. doi:10.5004/dwt.2020.26207
Elbatea, A. A., Nosier, S. A., Zatout, A. A., Hassan, I., Sedahmed, G. H., Abdel-Aziz, M. H., et al. (2021). Removal of Reactive Red 195 from Dyeing Wastewater Using Electro-Fenton Process in a Cell with Oxygen Sparged Fixed Bed Electrodes. J. Water Process Eng. 41, 102042. doi:10.1016/j.jwpe.2021.102042
Elgarahy, A. M., Elwakeel, K. Z., Elshoubaky, G. A., and Mohammad, S. H. (2019). Microwave-accelerated Sorption of Cationic Dyes onto green marine Algal Biomass. Environ. Sci. Pollut. Res. 26, 22704–22722. doi:10.1007/s11356-019-05417-2
Erkanlı, M., Yilmaz, L., Çulfaz-Emecen, P. Z., and Yetis, U. (2017). Brackish Water Recovery from Reactive Dyeing Wastewater via Ultrafiltration. J. Clean. Prod. 165, 1204–1214. doi:10.1016/j.jclepro.2017.07.195
Eslami, H., Sedighi Khavidak, S., Salehi, F., Khosravi, R., Fallahzadeh, R. A., Peirovi, R., et al. (2016). Biodegradation of Methylene Blue from Aqueous Solution by Bacteria Isolated from Contaminated Soil. J. Adv. Environ. Heal. Res. 5, 10–15. Available at: http://jaehr.muk.ac.ir/article_46690.html. doi:10.22102/JAEHR.2017.46690
Essekri, A., Hsini, A., Naciri, Y., Laabd, M., Ajmal, Z., El Ouardi, M., et al. (2021). Novel Citric Acid-Functionalized Brown Algae with a High Removal Efficiency of crystal Violet Dye from Colored Wastewaters: Insights into Equilibrium, Adsorption Mechanism, and Reusability. Int. J. Phytoremediation 23, 336–346. doi:10.1080/15226514.2020.1813686
Forgacs, E., Cserháti, T., and Oros, G. (2004). Removal of Synthetic Dyes from Wastewaters: A Review. Environ. Int. 30, 953–971. doi:10.1016/j.envint.2004.02.001
Ghany, T., Abboud, M., Alawlaqi, M., and Shater, A. (2019). Dead Biomass of Thermophilic Aspergillus fumigatus for Congo Red Biosorption. Egypt. J. Exp. Biol. (Bot.) 15, 1–6. doi:10.5455/egyjebb.20181206084342
Ghariani, B., Hadrich, B., Louati, I., Mtibaà, R., Daâssi, D., Rodriguez-Couto, S., et al. (2019). Porous Heat-Treated Fungal Biomass: Preparation, Characterization and Application for Removal of Textile Dyes from Aqueous Solutions. J. Porous Mater. 26, 1475–1488. doi:10.1007/s10934-019-00746-6
Giannakoudakis, D. A., Kyzas, G. Z., Avranas, A., and Lazaridis, N. K. (2016). Multi-parametric Adsorption Effects of the Reactive Dye Removal with Commercial Activated Carbons. J. Mol. Liquids 213, 381–389. doi:10.1016/j.molliq.2015.07.010
Gnanadoss, J. J. (2013). Bioremediation of Textile Dye Using White Rot Fungi: a Review. Rev. Int. J. Cur Res. Rev. 05, 1–13.
Gowtham, B., and Pauline, S. (2021). Experimental Study on Performance Assessment of Fenton and Photo- Fenton Oxidation Process for Methylene Blue. Proc. Int. Acad. Ecol. Environ. Sci. 11, 43–51.
Grassi, P., Reis, C., Drumm, F. C., Georgin, J., Tonato, D., Escudero, L. B., et al. (2019). Biosorption of crystal Violet Dye Using Inactive Biomass of the Fungus Diaporthe Schini. Water Sci. Technol. 79, 709–717. doi:10.2166/wst.2019.091
Gross, M., Tupinamba Lima, M., Uhlig, M., Ebraheme, A., Roeber, O., Olschewski, B., et al. (2017). Biopolymers for Dye Removal via Foam Separation. Sep. Purif. Tech. 188, 451–457. doi:10.1016/j.seppur.2017.07.025
Guarín, J. R., Moreno-Pirajan, J. C., and Giraldo, L. (20182018). Kinetic Study of the Bioadsorption of Methylene Blue on the Surface of the Biomass Obtained from the Algae D. antarctica. J. Chem. 2018, 1–12. doi:10.1155/2018/2124845
Gül, Ü. D., Taştan, B. E., and Bayazıt, G. (2019). Assessment of Algal Biomasses Having Different Cell Structures for Biosorption Properties of Acid Red P-2BX Dye. South Afr. J. Bot. 127, 147–152. doi:10.1016/j.sajb.2019.08.047
Gül, Ü. (2013). Treatment of Dyeing Wastewater Including Reactive Dyes (Reactive Red RB, Reactive Black B, Remazol Blue) and Methylene Blue by Fungal Biomass. Wsa 39, 593–598. doi:10.4314/wsa.v39i5.2
Gunasundari, E., Senthil Kumar, P., Rajamohan, N., and Vellaichamy, P. (2020). Feasibility of Naphthol green-b Dye Adsorption Using Microalgae: Thermodynamic and Kinetic Analysis. Dwt 192, 358–370. doi:10.5004/dwt.2020.25777
Guo, G., Li, X., Tian, F., Liu, T., Yang, F., Ding, K., et al. (2020). Azo Dye Decolorization by a Halotolerant Consortium under Microaerophilic Conditions. Chemosphere 244, 125510–125537. doi:10.1016/j.chemosphere.2019.125510
Hannachi, Y., and Hafidh, A. (2020). Biosorption Potential of Sargassum Muticum Algal Biomass for Methylene Blue and lead Removal from Aqueous Medium. Int. J. Environ. Sci. Technol. 17, 3875–3890. doi:10.1007/s13762-020-02742-9
Hasanzadeh, M., Simchi, A., and Shahriyari Far, H. (2020). Nanoporous Composites of Activated Carbon-Metal Organic Frameworks for Organic Dye Adsorption: Synthesis, Adsorption Mechanism and Kinetics Studies. J. Ind. Eng. Chem. 81, 405–414. doi:10.1016/j.jiec.2019.09.031
Hassan, M. M., and Carr, C. M. (2021). Biomass-derived Porous Carbonaceous Materials and Their Composites as Adsorbents for Cationic and Anionic Dyes: A Review. Chemosphere 265, 129087–7. doi:10.1016/j.chemosphere.2020.129087
Hassani, A. H., Mirzayee, R., Nasseri, S., Borghei, M., Gholami, M., and Torabifar, B. (2008). Nanofiltration Process on Dye Removal from Simulated Textile Wastewater. Int. J. Environ. Sci. Technol. 5, 401–408. doi:10.1007/BF03326035
Hasyimah, N. A. R., Furusawa, G., and Amirul, A. A. (2020). Biosorption of a Dye and Heavy Metals Using Dead Cells of Filamentous Bacterium, Aureispira Sp. CCB-QB1. Int. J. Environ. Sci. Technol. 18, 1627–1636. doi:10.1007/s13762-020-02918-3
Heidari, M., Varma, R., Ahmadian, M., Pourkhosravani, M., Asadzadeh, S., Karimi, P., et al. (2019). Photo-Fenton like Catalyst System: Activated Carbon/CoFe2O4 Nanocomposite for Reactive Dye Removal from Textile Wastewater. Appl. Sci. 9, 963–1015. doi:10.3390/app9050963
Heidarinejad, Z., Dehghani, M. H., Heidari, M., Javedan, G., Ali, I., and Sillanpää, M. (2020). Methods for Preparation and Activation of Activated Carbon: a Review. Environ. Chem. Lett. 18, 393–415. doi:10.1007/s10311-019-00955-0
Hendaoui, K., Trabelsi-Ayadi, M., and Ayari, F. (2021). Optimization and Mechanisms Analysis of Indigo Dye Removal Using Continuous Electrocoagulation. Chin. J. Chem. Eng. 29, 242–252. doi:10.1016/j.cjche.2020.07.065
Hisada, M., Tomizawa, Y., and Kawase, Y. (2019). Removal Kinetics of Cationic Azo-Dye from Aqueous Solution by Poly-γ-Glutamic Acid Biosorbent: Contributions of Adsorption and Complexation/precipitation to Basic Orange 2 Removal. J. Environ. Chem. Eng. 7, 103157. doi:10.1016/j.jece.2019.103157
Horciu, I. L., Blaga, A. C., Rusu, L., Zaharia, C., and Suteu, D. (2020). Biosorption of Reactive Dyes from Aqueous media Using the bacillus Sp. Residual Biomass. Dwt 195, 353–360. doi:10.5004/dwt.2020.25901
Hu, N., Zhang, K., Zhao, Y., Zhang, Z., and Li, H. (2020). Flotation-based Dye Removal System: Sweet Potato Protein Fabricated from Agro-Industrial Waste as a Collector and Frother. J. Clean. Prod. 269, 122121. doi:10.1016/j.jclepro.2020.122121
Hu, Y., Liu, F., Sun, Y., Xu, X., Chen, X., Pan, B., et al. (2019). Bacterial Cellulose Derived Paper-like Purifier with Multifunctionality for Water Decontamination. Chem. Eng. J. 371, 730–737. doi:10.1016/j.cej.2019.04.091
Isaza-Pérez, F., Ramírez-Carmona, M., Rendón-Castrillón, L., and Ocampo-López, C. (2020). Potential of Residual Fungal Biomass: a Review. Environ. Sci. Pollut. Res. 27, 13019–13031. doi:10.1007/s11356-020-08193-6
Januário, E. F. D., Vidovix, T. B., Araújo, L. A. d., Bergamasco Beltran, L., Bergamasco, R., and Vieira, A. M. S. (2021). Investigation of Citrus Reticulata Peels as an Efficient and Low-Cost Adsorbent for the Removal of Safranin orange Dye. Environ. Tech. 1, 1–15. doi:10.1080/09593330.2021.1946601
Javaid, R., and Qazi, U. Y. (2019). Catalytic Oxidation Process for the Degradation of Synthetic Dyes: An Overview. Ijerph 16, 2066–2127. doi:10.3390/ijerph16112066
Jawad, A. H., Mohd Firdaus Hum, N. N., Abdulhameed, A. S., and Mohd Ishak, M. A. (2020). Mesoporous Activated Carbon from Grass Waste via H3PO4-Activation for Methylene Blue Dye Removal: Modelling, Optimisation, and Mechanism Study. Int. J. Environ. Anal. Chem., 1–17. doi:10.1080/03067319.2020.1807529
Jia, Y., Ding, L., Ren, P., Zhong, M., Ma, J., and Fan, X. (2020). Performances and Mechanism of Methyl Orange and Congo Red Adsorbed on the Magnetic Ion-Exchange Resin. J. Chem. Eng. Data 65, 725–736. doi:10.1021/acs.jced.9b00951
Joseph, J., Radhakrishnan, R. C., Johnson, J. K., Joy, S. P., and Thomas, J. (2020). Ion-exchange Mediated Removal of Cationic Dye-Stuffs from Water Using Ammonium Phosphomolybdate. Mater. Chem. Phys. 242, 122488. doi:10.1016/j.matchemphys.2019.122488
Kabbout, R., and Taha, S. (2014). Biodecolorization of Textile Dye Effluent by Biosorption on Fungal Biomass Materials. Phys. Proced. 55, 437–444. doi:10.1016/j.phpro.2014.07.063
Kandisa, R. V., and Saibaba Kv, N. (2016). Dye Removal by Adsorption: A Review. J. Bioremediat Biodegrad 07, 371. doi:10.4172/2155-6199.1000371
Kang, S. Bin., Wang, Z., and Won, S. W. (2020). Adsorption Characteristics of Methylene Blue on PAA-PSBF Adsorbent. Chem. Eng. Trans. 78, 205–210. doi:10.3303/CET2078035
Karthik, V., Kumar, P. S., Harsha Vardhan, K., Saravanan, K., and Nithyakala, N. (2020). Adsorptive Behaviour of Surface Tailored Fungal Biomass for the Elimination of Toxic Dye from Wastewater. Int. J. Environ. Anal. Chem., 1–16. doi:10.1080/03067319.2020.1787400
Karthik, V., Saravanan, K., Sivarajasekar, N., and Suriyanarayanan, N. (2016). Bioremediation of Dye Bearing Effluents Using Microbial Biomass. Ecol. Environ. Conserv 22, S423–S434.
Katheresan, V., Kansedo, J., and Lau, S. Y. (2018). Efficiency of Various Recent Wastewater Dye Removal Methods: A Review. J. Environ. Chem. Eng. 6, 4676–4697. doi:10.1016/j.jece.2018.06.060
Kausar, R. A., Buhani, , and Suharso, (2020). Methylene Blue Adsorption Isotherm on Spirulina Sp. Microalgae Biomass Coated by Silica-Magnetite. IOP Conf. Ser. Mater. Sci. Eng. 857, 012019–12028. doi:10.1088/1757-899X/857/1/012019
Kavci, E. (2020). Malachite green Adsorption onto Modified pine Cone: Isotherms, Kinetics and Thermodynamics Mechanism. Chem. Eng. Commun. 208, 318–327. doi:10.1080/00986445.2020.1715961
Kharat, D. S. (2015). Preparing Agricultural Residue Based Adsorbents for Removal of Dyes from Effluents - A Review. Braz. J. Chem. Eng. 32, 1–12. doi:10.1590/0104-6632.20150321s00003020
Khatri, J., Nidheesh, P. V., Anantha Singh, T. S., and Suresh Kumar, M. (2018). Advanced Oxidation Processes Based on Zero-Valent Aluminium for Treating Textile Wastewater. Chem. Eng. J. 348, 67–73. doi:10.1016/j.cej.2018.04.074
Kim, S. Y., Jin, M. R., Chung, C. H., Yun, Y.-S., Jahng, K. Y., and Yu, K.-Y. (2015). Biosorption of Cationic Basic Dye and Cadmium by the Novel Biosorbent Bacillus Catenulatus JB-022 Strain. J. Biosci. Bioeng. 119, 433–439. doi:10.1016/j.jbiosc.2014.09.022
Kousha, M., Tavakoli, S., Daneshvar, E., Vazirzadeh, A., and Bhatnagar, A. (2015). Central Composite Design Optimization of Acid Blue 25 Dye Biosorption Using Shrimp Shell Biomass. J. Mol. Liquids 207, 266–273. doi:10.1016/j.molliq.2015.03.046
Kubra, K. T., Salman, M. S., and Hasan, M. N. (2021). Enhanced Toxic Dye Removal from Wastewater Using Biodegradable Polymeric Natural Adsorbent. J. Mol. Liquids 328, 115468. doi:10.1016/J.MOLLIQ.2021.115468
Kumar, A., Singh, R., Kumar Upadhyay Sanjay Kumar, S., Charaya, M. U., and Charaya, M. U. (2021). Biosorption: the Removal of Toxic Dyes from Industrial Effluent Using Phytobiomass- a Review. Plant Arch. 21, 1320–1325. doi:10.51470/plantarchives.2021.v21.s1.207
Kyzas, G., and Matis, K. (2018). Flotation in Water and Wastewater Treatment. Processes 6, 116. doi:10.3390/pr6080116
Lafi, R., Gzara, L., Lajimi, R. H., and Hafiane, A. (2018). Treatment of Textile Wastewater by a Hybrid Ultrafiltration/electrodialysis Process. Chem. Eng. Process. - Process Intensification 132, 105–113. doi:10.1016/j.cep.2018.08.010
Lafi, R., Mabrouk, W., and Hafiane, A. (2019). Removal of Methylene Blue from saline Solutions by Adsorption and Electrodialysis. Membr. Water Treat. 10, 139–148. doi:10.12989/mwt.2019.10.2.139
Law, X. N., Cheah, W. Y., Chew, K. W., Ibrahim, M. F., Park, Y.-K., Ho, S.-H., et al. (2022). Microalgal-based Biochar in Wastewater Remediation: Its Synthesis, Characterization and Applications. Environ. Res. 204, 111966. doi:10.1016/j.envres.2021.111966
Leal, A. N. R., De Lima, A. D. C. A., Azevedo, M. G. F. d. A., Santos, D. K. D. d. N., Zaidan, L. E. M. C., De Lima, V. F., et al. (2021). Removal of Remazol Black B Dye Using Bacterial Cellulose as an Adsorbent. Sci. Plena 17, 1–21. doi:10.14808/sci.plena.2021.034201
Leng, L., Xiong, Q., Yang, L., Li, H., Zhou, Y., Zhang, W., et al. (2021). An Overview on Engineering the Surface Area and Porosity of Biochar. Sci. Total Environ. 763, 144204. doi:10.1016/j.scitotenv.2020.144204
Lin, H.-H., Stephen Inbaraj, B., and Kao, T.-H. (2019). Removal Potential of Basic Dyes and Lead from Water by Brewer's Yeast Biomass. J. Am. Soc. Brewing Chemists 77, 30–39. doi:10.1080/03610470.2018.1561794
Mahmooda, T. (2014). Bioremediation of Xenobiotics: Use of Dead Fungal Biomass as Biosorbent. Ijret 03, 565–570. doi:10.15623/ijret.2014.0301094
Mahmoud, M. S. (2016). Decolorization of Certain Reactive Dye from Aqueous Solution Using Baker's Yeast (Saccharomyces cerevisiae) Strain. HBRC J. 12, 88–98. doi:10.1016/j.hbrcj.2014.07.005
Mahmoud, M. S., Mostafa, M. K., Mohamed, S. A., Sobhy, N. A., and Nasr, M. (2017). Bioremediation of Red Azo Dye from Aqueous Solutions by A Spergillus niger Strain Isolated from Textile Wastewater. J. Environ. Chem. Eng. 5, 547–554. doi:10.1016/j.jece.2016.12.030
Mahmoudi, N., Farhadian, M., Solaimany Nazar, A. R., Eskandari, P., and Esfahani, K. N. (2021a). Investigation and Optimization of the Performance of Sono-Photo-Electro-Fenton Process for Removal of Acid Black 172 and Disperse Blue 56 from Polluted Water: Comparison of the Degradation Activity with electro-Fenton-based Processes. Int. J. Environ. Sci. Technol., 1–12. doi:10.1007/s13762-021-03296-0
Mahmoudi, N., Farhadian, M., Solaimany Nazar, A. R., Eskandari, P., and Esfahani, K. N. (2021b). Investigation and Optimization of the Performance of Sono-Photo-Electro-Fenton Process for Removal of Acid Black 172 and Disperse Blue 56 from Polluted Water: Comparison of the Degradation Activity with electro-Fenton-based Processes. Int. J. Environ. Sci. Technol., 1–12. doi:10.1007/s13762-021-03296-0
Marin, N. M., Pascu, L. F., Demba, A., Nita-Lazar, M., Badea, I. A., and Aboul-Enein, H. Y. (2019). Removal of the Acid Orange 10 by Ion Exchange and Microbiological Methods. Int. J. Environ. Sci. Technol. 16, 6357–6366. doi:10.1007/s13762-018-2164-2
Mcyotto, F., Wei, Q., Macharia, D. K., Huang, M., Shen, C., and Chow, C. W. K. (2021). Effect of Dye Structure on Color Removal Efficiency by Coagulation. Chem. Eng. J. 405, 126674. doi:10.1016/j.cej.2020.126674
Miklos, D. B., Remy, C., Jekel, M., Linden, K. G., Drewes, J. E., and Hübner, U. (2018). Evaluation of Advanced Oxidation Processes for Water and Wastewater Treatment - A Critical Review. Water Res. 139, 118–131. doi:10.1016/j.watres.2018.03.042
Mishra, S., and Maiti, A. (2018). The Efficacy of Bacterial Species to Decolourise Reactive Azo, Anthroquinone and Triphenylmethane Dyes from Wastewater: a Review. Environ. Sci. Pollut. Res. 25, 8286–8314. doi:10.1007/s11356-018-1273-2
Mo, J., Yang, Q., Zhang, N., Zhang, W., Zheng, Y., and Zhang, Z. (2018). A Review on Agro-Industrial Waste (AIW) Derived Adsorbents for Water and Wastewater Treatment. J. Environ. Manage. 227, 395–405. doi:10.1016/j.jenvman.2018.08.069
Moghazy, R. M., Labena, A., Husien, S., Mansor, E. S., and Abdelhamid, A. E. (2020). Neoteric Approach for Efficient Eco-Friendly Dye Removal and Recovery Using Algal-Polymer Biosorbent Sheets: Characterization, Factorial Design, Equilibrium and Kinetics. Int. J. Biol. Macromolecules 157, 494–509. doi:10.1016/j.ijbiomac.2020.04.165
Mohan, S. V., Bhaskar, Y. V., and Karthikeyan, J. (2004). Biological Decolourisation of Simulated Azo Dye in Aqueous Phase by Algae Spirogyra Species. Ijep 21, 211–222. doi:10.1504/IJEP.2004.004190
Mohd Nasir, M. Z., Indiran, G., and Ahmad Zaini, M. A. (2021). Assessment of thermal Regeneration of Spent Commercial Activated Carbon for Methylene Blue Dye Removal. Particulate Sci. Tech. 39, 504–510. doi:10.1080/02726351.2020.1775738
Mokif, L. A. (2019). Removal Methods of Synthetic Dyes from Industrial Wastewater: A Review. Mesopotamia Environ. J. 5, 23–40. Available at: http//:www.bumej.com. doi:10.31759/mej.2019.5.1.0040
Mondal, S., Purkait, M. K., and De, S. (2018). Advances in Dye Removal Technologies. Springer Nature. doi:10.1007/978-981-10-6293-3
Moosavi, S., Lai, C. W., Gan, S., Zamiri, G., Akbarzadeh Pivehzhani, O., and Johan, M. R. (2020). Application of Efficient Magnetic Particles and Activated Carbon for Dye Removal from Wastewater. ACS Omega 5, 20684–20697. doi:10.1021/acsomega.0c01905
Nasar, A., and Mashkoor, F. (2019). Application of Polyaniline-Based Adsorbents for Dye Removal from Water and Wastewater-A Review. Environ. Sci. Pollut. Res. 26, 5333–5356. doi:10.1007/s11356-018-3990-y
Nasuha, N., Hameed, B. H., and Okoye, P. U. (2021). Dark-Fenton Oxidative Degradation of Methylene Blue and Acid Blue 29 Dyes Using Sulfuric Acid-Activated Slag of the Steel-Making Process. J. Environ. Chem. Eng. 9, 104831–104833. doi:10.1016/j.jece.2020.104831
Nguyen, T. A., Fu, C.-C., and Juang, R.-S. (2016). Biosorption and Biodegradation of a Sulfur Dye in High-Strength Dyeing Wastewater by Acidithiobacillus Thiooxidans. J. Environ. Manage. 182, 265–271. doi:10.1016/j.jenvman.2016.07.083
Nouri, H., Azin, E., Kamyabi, A., and Moghimi, H. (2021). Biosorption Performance and Cell Surface Properties of a Fungal-Based Sorbent in Azo Dye Removal Coupled with Textile Wastewater. Int. J. Environ. Sci. Technol. 18, 2545–2558. doi:10.1007/s13762-020-03011-5
Öztürk, A., Bayol, E., and Abdullah, M. I. (2020). Characterization of the Biosorption of Fast Black Azo Dye K Salt by the Bacterium Rhodopseudomonas Palustris 51ATA Strain. Electron. J. Biotechnol. 46, 22–29. doi:10.1016/j.ejbt.2020.05.002
Patel, H. (2021). Review on Solvent Desorption Study from Exhausted Adsorbent. J. Saudi Chem. Soc. 25, 101302. doi:10.1016/j.jscs.2021.101302
Pathania, D., Sharma, S., and Singh, P. (2017). Removal of Methylene Blue by Adsorption onto Activated Carbon Developed from Ficus Carica Bast. Arabian J. Chem. 10, S1445–S1451. doi:10.1016/j.arabjc.2013.04.021
Pearce, C., Lloyd, J. R., and Guthrie, J. T. (2003). The Removal of Colour from Textile Wastewater Using Whole Bacterial Cells: A Review. Dyes Pigm. 58, 179–196. doi:10.1016/S0143-7208(03)00064-0
Pecková, V., Legerská, B., Chmelová, D., Horník, M., and Ondrejovič, M. (2020). Comparison of Efficiency for Monoazo Dye Removal by Different Species of white-rot Fungi. Int. J. Environ. Sci. Technol. 18, 21–32. doi:10.1007/s13762-020-02806-w
Peng, H., and Guo, J. (2020). Removal of Chromium from Wastewater by Membrane Filtration, Chemical Precipitation, Ion Exchange, Adsorption Electrocoagulation, Electrochemical Reduction, Electrodialysis, Electrodeionization, Photocatalysis and Nanotechnology: a Review. Environ. Chem. Lett. 18, 2055–2068. doi:10.1007/s10311-020-01058-x
Piaskowski, K., Świderska-Dąbrowska, R., and Zarzycki, P. K. (2018). Dye Removal from Water and Wastewater Using Various Physical, Chemical, and Biological Processes. J. AOAC Int. 101, 1371–1384. doi:10.5740/jaoacint.18-0051
Praveen, S., Gokulan, R., Pushpa, T. B., and Jegan, J. (2021). Techno-economic Feasibility of Biochar as Biosorbent for Basic Dye Sequestration. J. Indian Chem. Soc. 98, 100107. doi:10.1016/j.jics.2021.100107
Przystaś, W., Zabłocka-Godlewska, E., and Grabińska-Sota, E. (2018). Efficiency of Decolorization of Different Dyes Using Fungal Biomass Immobilized on Different Solid Supports. Braz. J. Microbiol. 49, 285–295. doi:10.1016/j.bjm.2017.06.010
Puchana-Rosero, M. J., Lima, E. C., Ortiz-Monsalve, S., Mella, B., da Costa, D., Poll, E., et al. (2017). Fungal Biomass as Biosorbent for the Removal of Acid Blue 161 Dye in Aqueous Solution. Environ. Sci. Pollut. Res. 24, 4200–4209. doi:10.1007/s11356-016-8153-4
Rahimian, R., and Zarinabadi, S. (2020). A Review of Studies on the Removal of Methylene Blue Dye from Industrial Wastewater Using Activated Carbon Adsorbents Made from Almond Bark. Prog. Chem. Biochem. Res. J. Homepage 3, 251–268. Available at: www.pcbiochemres.com. doi:10.33945/SAMI/PCBR.2020.3.8
Regti, A., Laamari, M. R., Stiriba, S.-E., and El Haddad, M. (2017). Potential Use of Activated Carbon Derived from Persea Species under Alkaline Conditions for Removing Cationic Dye from Wastewaters. J. Assoc. Arab Universities Basic Appl. Sci. 24, 10–18. doi:10.1016/j.jaubas.2017.01.003
Ribas, M. C., Adebayo, M. A., Prola, L. D. T., Lima, E. C., Cataluña, R., Feris, L. A., et al. (2014). Comparison of a Homemade cocoa Shell Activated Carbon with Commercial Activated Carbon for the Removal of Reactive Violet 5 Dye from Aqueous Solutions. Chem. Eng. J. 248, 315–326. doi:10.1016/j.cej.2014.03.054
Rodríguez Couto, S. (2009). Dye Removal by Immobilised Fungi. Biotechnol. Adv. 27, 227–235. doi:10.1016/j.biotechadv.2008.12.001
Roy, M., and Saha, R. (2021). Dyes and Their Removal Technologies from Wastewater: A Critical Review. Intell. Environ. Data Monit. Pollut. Manag., 127–160. doi:10.1016/b978-0-12-819671-7.00006-3
Roy, U., Manna, S., Sengupta, S., Das, P., Datta, S., Mukhopadhyay, A., et al. (2018). Dye Removal Using Microbial Biosorbents. Environ. Chem. A Sustain. World, 253–280. doi:10.1007/978-3-319-92162-4_8
Ruscasso, F., Bezus, B., Garmendia, G., Vero, S., Curutchet, G., Cavello, I., et al. (2021). Debaryomyces Hansenii F39A as Biosorbent for Textile Dye Removal. Revista Argentina de Microbiología 53, 257–265. doi:10.1016/j.ram.2020.10.004
Saha, A., Basak, B. B., and Ponnuchamy, M. (2020). Performance of Activated Carbon Derived from Cymbopogon Winterianus Distillation Waste for Scavenging of Aqueous Toxic Anionic Dye Congo Red: Comparison with Commercial Activated Carbon. Sep. Sci. Tech. 55, 1970–1983. doi:10.1080/01496395.2019.1620277
Saifi, A., Joseph, J. P., Singh, A. P., Pal, A., and Kumar, K. (2021). Complexation of an Azo Dye by Cyclodextrins: A Potential Strategy for Water Purification. ACS Omega 6, 4776–4782. doi:10.1021/acsomega.0c05684
Salem, S., Fouda, A., Mohamed, A., El-Gamal, M., and Talat, M. (2019). Biological Decolorization of Azo Dyes from Textile Wastewater Effluent by Aspergillus niger. Egypt. J. Chem. 62, 1799–1813. doi:10.21608/EJCHEM.2019.11720.1747
San Keskin, N. O., Celebioglu, A., Sarioglu, O. F., Ozkan, A. D., Uyar, T., and Tekinay, T. (2015). Removal of a Reactive Dye and Hexavalent Chromium by a Reusable Bacteria Attached Electrospun Nanofibrous Web. RSC Adv. 5, 86867–86874. doi:10.1039/c5ra15601g
Saravanan, A., Kumar, P. S., Govarthanan, M., George, C. S., Vaishnavi, S., Moulishwaran, B., et al. (2021). Adsorption Characteristics of Magnetic Nanoparticles Coated Mixed Fungal Biomass for Toxic Cr(VI) Ions in Aquatic Environment. Chemosphere 267, 129226. doi:10.1016/j.chemosphere.2020.129226
Sarioglu, O. F., Keskin, N. O. S., Celebioglu, A., Tekinay, T., and Uyar, T. (2017a). Bacteria Encapsulated Electrospun Nanofibrous Webs for Remediation of Methylene Blue Dye in Water. Colloids Surf. B: Biointerfaces 152, 245–251. doi:10.1016/j.colsurfb.2017.01.034
Sarioglu, O. F., San Keskin, N. O., Celebioglu, A., Tekinay, T., and Uyar, T. (2017b). Bacteria Immobilized Electrospun Polycaprolactone and Polylactic Acid Fibrous Webs for Remediation of Textile Dyes in Water. Chemosphere 184, 393–399. doi:10.1016/j.chemosphere.2017.06.020
Sarvajith, M., Reddy, G. K. K., and Nancharaiah, Y. V. (2018). Textile Dye Biodecolourization and Ammonium Removal over Nitrite in Aerobic Granular Sludge Sequencing Batch Reactors. J. Hazard. Mater. 342, 536–543. doi:10.1016/j.jhazmat.2017.08.064
Schimmel, D., Fagnani, K. C., Santos, J. B. O. d., Barros, M. A. S. D., and Silva, E. A. d. (2010). Adsorption of Turquoise Blue QG Reactive Bye Commercial Activated Carbon in Batch Reactor: Kinetic and Equilibrium Studies. Braz. J. Chem. Eng. 27, 289–298. doi:10.1590/S0104-66322010000200007
Semião, M. A., Haminiuk, C. W. I., and Maciel, G. M. (2020). Residual Diatomaceous Earth as a Potential and Cost Effective Biosorbent of the Azo Textile Dye Reactive Blue 160. J. Environ. Chem. Eng. 8, 103617–103627. doi:10.1016/j.jece.2019.103617
Sen, S. K., Raut, S., Bandyopadhyay, P., and Raut, S. (2016). Fungal Decolouration and Degradation of Azo Dyes: A Review. Fungal Biol. Rev. 30, 112–133. doi:10.1016/j.fbr.2016.06.003
Sewu, D. D., Boakye, P., and Woo, S. H. (2017). Highly Efficient Adsorption of Cationic Dye by Biochar Produced with Korean Cabbage Waste. Bioresour. Tech. 224, 206–213. doi:10.1016/j.biortech.2016.11.009
Seyedi, Z. S., Zahraei, Z., and Jookar Kashi, F. (2020). Decolorization of Reactive Black 5 and Reactive Red 152 Azo Dyes by New Haloalkaliphilic Bacteria Isolated from the Textile Wastewater. Curr. Microbiol. 77, 2084–2092. doi:10.1007/s00284-020-02039-7
Sharma, V. K., and Feng, M. (2019). Water Depollution Using Metal-Organic Frameworks-Catalyzed Advanced Oxidation Processes: A Review. J. Hazard. Mater. 372, 3–16. doi:10.1016/j.jhazmat.2017.09.043
Shen, C., Pan, Y., Wu, D., Liu, Y., Ma, C., Li, F., et al. (2019). A Crosslinking-Induced Precipitation Process for the Simultaneous Removal of Poly(vinyl Alcohol) and Reactive Dye: The Importance of Covalent Bond Forming and Magnesium Coagulation. Chem. Eng. J. 374, 904–913. doi:10.1016/j.cej.2019.05.203
Sherugar, P., Padaki, M., Naik, N. S., George, S. D., and Murthy, D. H. K. (2022). Biomass-derived Versatile Activated Carbon Removes Both Heavy Metals and Dye Molecules from Wastewater with Near-unity Efficiency: Mechanism and Kinetics. Chemosphere 287, 132085. doi:10.1016/j.chemosphere.2021.132085
Shindhal, T., Rakholiya, P., Varjani, S., Pandey, A., Ngo, H. H., Guo, W., et al. (2021). A Critical Review on Advances in the Practices and Perspectives for the Treatment of Dye Industry Wastewater. Bioengineered 12, 70–87. doi:10.1080/21655979.2020.1863034
Shokry, H., Elkady, M., and Hamad, H. (2019). Nano Activated Carbon from Industrial Mine Coal as Adsorbents for Removal of Dye from Simulated Textile Wastewater: Operational Parameters and Mechanism Study. J. Mater. Res. Tech. 8, 4477–4488. doi:10.1016/j.jmrt.2019.07.061
Shu, J., Cheng, S., Xia, H., Zhang, L., Peng, J., Li, C., et al. (2017). Copper Loaded on Activated Carbon as an Efficient Adsorbent for Removal of Methylene Blue. RSC Adv. 7, 14395–14405. doi:10.1039/c7ra00287d
Siddiqui, S. I., Fatima, B., Tara, N., Rathi, G., and Chaudhry, S. A. (2019). Recent Advances in Remediation of Synthetic Dyes from Wastewaters Using Sustainable and Low-Cost Adsorbents. Impact Prospects Green. Chem. Textile Tech., 471–507. doi:10.1016/B978-0-08-102491-1.00015-0
Simi, A., and Azeeza, V. (2010). Removal of Methylene Blue Dye Using Low Cost Adsorbent. Asian J. Chem. 22, 4371–4376.
Sindelar, F. W., Silva, L. F. O., Machado, V. R., dos Santos, L. C. M., and Stülp, S. (2015). Treatment of Effluent from the Agate Dyeing Industry Using Photodegradation and Electrodialysis Processes. Sep. Sci. Tech. 50, 142–147. doi:10.1080/01496395.2014.947519
Singh, K., and Arora, S. (2011). Removal of Synthetic Textile Dyes from Wastewaters: A Critical Review on Present Treatment Technologies. Crit. Rev. Environ. Sci. Tech. 41, 807–878. doi:10.1080/10643380903218376
Singh, N. B., Nagpal, G., Agrawal, S., and Rachna, (2018). Water Purification by Using Adsorbents: A Review. Environ. Tech. Innovation 11, 187–240. doi:10.1016/j.eti.2018.05.006
Singh, P. K., and Singh, R. L. (2017). Bio-removal of Azo Dyes: A Review. Int. J. Appl. Sci. Biotechnol. 5, 108–126. doi:10.3126/ijasbt.v5i2.16881
Singh, S., Kumar, V., Datta, S., Dhanjal, D. S., Sharma, K., Samuel, J., et al. (2020). Current Advancement and Future prospect of Biosorbents for Bioremediation. Sci. Total Environ. 709, 135895. doi:10.1016/j.scitotenv.2019.135895
Sivaranjanee, R., and Kumar, P. S. (2021). Treatment of Textile Wastewater Using Biochar Produced from Agricultural Waste. Sustainable Technologies for Textile Wastewater Treatments. Elsevier, 187–208. doi:10.1016/b978-0-323-85829-8.00004-3
Streit, A. F. M., Côrtes, L. N., Druzian, S. P., Godinho, M., Collazzo, G. C., Perondi, D., et al. (2019). Development of High Quality Activated Carbon from Biological Sludge and its Application for Dyes Removal from Aqueous Solutions. Sci. Total Environ. 660, 277–287. doi:10.1016/j.scitotenv.2019.01.027
Sulyman, M., and Gierak, A. (2020). Green Environmental Approach for Adsorption of Hazardous Dye from Water Using Tree and Sea Plant Leaves (Dead L.), green-environmental-approach-for-adsorption-of-hazardous-dye-from-water-using-tree-and-sea-plant-leaves-dead-l. Act Scie Agri 4, 01–10. doi:10.31080/asag.2020.04
Sun, Z., Qu, K., Cheng, Y., You, Y., Huang, Z., Umar, A., et al. (2021). Corncob-derived Activated Carbon for Efficiently Adsorption Dye in Sewage. ES Food Agrofor 4, 61–73. doi:10.30919/esfaf473
Syafiuddin, A., and Fulazzaky, M. A. (2021). Decolorization Kinetics and Mass Transfer Mechanisms of Remazol Brilliant Blue R Dye Mediated by Different Fungi. Biotechnol. Rep. 29, e00573. doi:10.1016/j.btre.2020.e00573
Tan, L., He, M., Song, L., Fu, X., and Shi, S. (2016). Aerobic Decolorization, Degradation and Detoxification of Azo Dyes by a Newly Isolated Salt-Tolerant Yeast Scheffersomyces Spartinae TLHS-SF1. Bioresour. Tech. 203, 287–294. doi:10.1016/j.biortech.2015.12.058
Tara, N., Iqbal, M., Mahmood Khan, Q., and Afzal, M. (2019). Bioaugmentation of Floating Treatment Wetlands for the Remediation of Textile Effluent. Water Environ. J. 33, 124–134. doi:10.1111/wej.12383
Titchou, F. E., Zazou, H., Afanga, H., El Gaayda, J., Ait Akbour, R., Hamdani, M., et al. (2021). Electro-Fenton Process for the Removal of Direct Red 23 Using BDD Anode in Chloride and Sulfate media. J. Electroanalytical Chem. 897, 115560. doi:10.1016/J.JELECHEM.2021.115560
Uğurlu, M., Gürses, A., and Açıkyıldız, M. (2008). Comparison of Textile Dyeing Effluent Adsorption on Commercial Activated Carbon and Activated Carbon Prepared from Olive Stone by ZnCl2 Activation. Microporous Mesoporous Mater. 111, 228–235. doi:10.1016/j.micromeso.2007.07.034
Upendar, G., Dutta, S., Chakraborty, J., and Bhattacharyya, P. (2016). Removal of Methylene Blue Dye Using Immobilized bacillus Subtilis in Batch & Column Reactor. Mater. Today Proc. 3, 3467–3472. doi:10.1016/j.matpr.2016.10.029
Vakili, M., Deng, S., Shen, L., Shan, D., Liu, D., and Yu, G. (2019). Regeneration of Chitosan-Based Adsorbents for Eliminating Dyes from Aqueous Solutions. Sep. Purif. Rev. 48, 1–13. doi:10.1080/15422119.2017.1406860
Varghese, A. G., Paul, S. A., and Latha, M. S. (2019). Remediation of Heavy Metals and Dyes from Wastewater Using Cellulose-Based Adsorbents. Environ. Chem. Lett. 17, 867–877. doi:10.1007/s10311-018-00843-z
Vimonses, V., Jin, B., and Chow, C. W. K. (2010). Insight into Removal Kinetic and Mechanisms of Anionic Dye by Calcined clay Materials and Lime. J. Hazard. Mater. 177, 420–427. doi:10.1016/j.jhazmat.2009.12.049
Wang, J., and Wang, S. (2020). Reactive Species in Advanced Oxidation Processes: Formation, Identification and Reaction Mechanism. Chem. Eng. J. 401, 126158. doi:10.1016/j.cej.2020.126158
Xue, C., Chen, Q., Liu, Y.-Y., Yang, Y.-L., Xu, D., Xue, L., et al. (2015). Acid Blue 9 Desalting Using Electrodialysis. J. Membr. Sci. 493, 28–36. doi:10.1016/j.memsci.2015.06.027
Yadav, S., Yadav, A., Bagotia, N., Sharma, A. K., and Kumar, S. (2021). Adsorptive Potential of Modified Plant-Based Adsorbents for Sequestration of Dyes and Heavy Metals from Wastewater - A Review. J. Water Process. Eng. 42. doi:10.1016/j.jwpe.2021.102148
Yahiaoui, C., Kameche, M., Innocent, C., and Khenifi, A. (2021). Conception of Yeast Microbial Desalination Cell: Applications to Dye Wastewater Treatment and lead Removal. Chem. Eng. Commun. 208, 364–375. doi:10.1080/00986445.2020.1721479
Yang, Z., Hou, J., Miao, L., and Wu, J. (2021). Comparison of Adsorption Behavior Studies of Methylene Blue by Microalga Residue and its Biochars Produced at Different Pyrolytic Temperatures. Environ. Sci. Pollut. Res. 28, 14028–14040. doi:10.1007/s11356-020-11470-z
Yeow, P. K., Wong, S. W., and Hadibarata, T. (2021). Removal of Azo and Anthraquinone Dye by Plant Biomass as Adsorbent - A Review. Biointerface Res. Appl. Chem. 11, 8218–8232. doi:10.33263/BRIAC111.82188232
Yu, K. L., Lee, X. J., Ong, H. C., Chen, W.-H., Chang, J.-S., Lin, C.-S., et al. (2021). Adsorptive Removal of Cationic Methylene Blue and Anionic Congo Red Dyes Using Wet-Torrefied Microalgal Biochar: Equilibrium, Kinetic and Mechanism Modeling. Environ. Pollut. 272, 115986. doi:10.1016/j.envpol.2020.115986
Zabłocka-Godlewska, E., Przystaś, W., and Grabińska-Sota, E. (2018). Possibilities of Obtaining from Highly Polluted Environments: New Bacterial Strains with a Significant Decolorization Potential of Different Synthetic Dyes. Water Air Soil Pollut. 229, 1–13. doi:10.1007/s11270-018-3829-7
Keywords: biomass, bio-adsorbent, conventional treatment techniques, dye removal, optimal conditions, removal efficiency
Citation: Aragaw TA and Bogale FM (2021) Biomass-Based Adsorbents for Removal of Dyes From Wastewater: A Review. Front. Environ. Sci. 9:764958. doi: 10.3389/fenvs.2021.764958
Received: 26 August 2021; Accepted: 04 November 2021;
Published: 09 December 2021.
Edited by:
Paula Oulego, University of Oviedo, SpainReviewed by:
Shaukat Ali Mazari, Dawood University of Engineering and Technology, PakistanMateus Torres Nazari, The University of Passo Fundo, Brazil
Flávia Melara, The University of Passo Fundo, Brazil
Copyright © 2021 Aragaw and Bogale. This is an open-access article distributed under the terms of the Creative Commons Attribution License (CC BY). The use, distribution or reproduction in other forums is permitted, provided the original author(s) and the copyright owner(s) are credited and that the original publication in this journal is cited, in accordance with accepted academic practice. No use, distribution or reproduction is permitted which does not comply with these terms.
*Correspondence: Tadele Assefa Aragaw, taaaad82@gmail.com