- 1Departamento de Sistemas Acuáticos, Facultad de Ciencias Ambientales y Centro EULA Chile, Universidad de Concepción, Concepción, Chile
- 2Institute for Environmental Science, University of Koblenz-Landau, Landau, Germany
- 3Instituto Milenio en Socio-Ecología Costera (SECOS), Santiago, Chile
Increased human activity on the Antarctic Peninsula has generated microplastic contamination in marine systems; however, less attention has been paid to soils so far. We investigated the occurrence of microplastics in 11 surface soils and intertidal sediments collected from Fildes Bay, King George Island. A transect of soils at Antarctic stations until Fildes Bay was made (i.e., S1–S5). Intertidal sediments along the shore (i.e., IS1–IS5) and a reference sample from Ardley Island (i.e., IS6) were also collected. All samples were stored at 4°C and analyzed for the organic matter content, particle size, and pH. Plastic particles were counted and classified by shape using metal dissecting forceps and a stereomicroscope and further analyzed by Fourier-transform infrared spectroscopy (FT-IR). They were classified by length as fibers (length: 500–2,000 μm) and fragments (length: 20–500 μm). In soil, fragments reached an average of 13.6 particles/50 ml sample, while in intertidal sediments, no fragments were found, but a fiber abundance of 1.5 particles/50 ml sample was observed. The principal component analysis shows a relationship between fibers and intertidal sediments, whereas fragments present a relationship with soils. There were differences between the numbers of fragments found in soils and intertidal sediments (p = 0.003), with a high abundance of fragments at site S5, but no significant differences were observed for fibers. The physicochemical soil analysis revealed that larger particle sizes were observed in intertidal sediments (average = 706.94 ± 230.51 μm) than in soils (p = 0.0007). The organic matter content was higher in soil than in intertidal sediments (p = 0.006) reaching an average of 6.0%. Plastic fragments and organic matter were significantly correlated (r = 0.779, p = 0.005), while fibers were positively correlated with particle size (r = 0.713, p = 0.014). The fragments were composed of phenoxy resin with the same appearance, shape, and bright orange color as the coatings of the facilities. According to the FT-IR analysis, the fibers had different colors and were composed of polyethylene terephthalate (PET). Cotton was also present at the sites surrounding the sampling site close to the base effluent. The presence of fiber on Ardley Island (i.e., control) may indicate that microplastic contamination has reached protected areas. This is the first study to confirm the presence of plastic debris in Antarctic soils. Further studies should focus on the identification of plastic sources and on the management of human activities and their eventual effects on biota.
Introduction
Since the first expeditions, and especially since the 1950s, scientific interest in the Antarctic continent has increased; there are currently more than 80 facilities distributed mainly in coastal areas and on the Antarctic Peninsula (Bruni et al., 1997; Gröndahl et al., 2009; Lu et al., 2012; Morales Calvo, 2013). These facilities host more than 5,000 people a year; however, this number is much lower than the 74,000 tourists who arrived in the 2019/2020 season (Council of Managers of National Antarctic Program, 2020; International Association of Antarctica Tour Operators, 2020). Using the data of human density and accessibility features of the Antarctic continent along with land uses, Pertierra et al. (2017) concluded that the Antarctic Peninsula is one of the three regions with the greatest human footprint pressure. On coasts worldwide, a high human density has resulted in contamination by microplastics, spread over six continents from the poles to the equator, sustained by an annual worldwide production of 368 million tons of plastic (Browne et al., 2011; Plastics Europe, 2020). In Antarctica, Barnes et al. (2010) reported the presence of macroplastics, such as fishing buoys and packing material in the Durmont D’Urville, Davis, and Amundsen seas. Nevertheless, at present, the international scientific community does not regularly sample microplastics or record at-sea observations of macroplastics in the Southern Ocean region, and there are few peer-reviewed scientific publications that quantify plastics in Antarctic waters (Waller et al., 2017). In studies carried out in seawater, a high abundance of microplastics has been reported in the vicinity of the Antarctic continent, in contrast to the low abundances that they reach in the Southern Ocean, which probably derive from long-distance transport (Isobe et al., 2017). Also, microplastics reached higher concentrations along the Antarctic Peninsula than at open ocean sub-Antarctic stations (Jones-Williams et al., 2020). Cunningham et al. (2020) reported high levels of microplastic contamination in marine sediment cores from three regions in Antarctica and the Southern Ocean. This is consistent with the findings of Onink et al. (2021), who, using a Lagrange particle transport model, verified that coastlines and coastal waters are an important reservoir of plastic debris and that there is limited transport of the marine plastic debris with positive buoyancy between the coastal zone and the open ocean. In general, high microplastic abundance has been reported in seawater and marine sediments collected in the areas closest to scientific stations, with wastewater effluents identified as being among their main sources (Cincinelli et al., 2017; Waller et al., 2017; Reed et al., 2018).
At present, there are no studies on Antarctic soils. Li et al. (2016) concluded that land-based sources account for 80% of the plastic waste in the marine environment, with high human density and industrial activities playing a key role. While evidence of the ecological impacts of microplastics has increased worldwide, evidence of the potential consequences of microplastics in soil ecosystems is still relatively scarce (Huang et al., 2019). This lack of information is of an ecotoxicological concern, given that once in the soil, these particles decompose slowly and accumulate as relatively persistent pollutants (Rillig, 2018). Decades ago, harm to fur seals, gulls, and penguin species caused by the presence of macroplastics was reported; however, the situation has resulted in sustained increases in their accumulation rates on Antarctic islands (Torres and Gajardo, 1985; Torres and Jorquera, 1992, 1994). There is no information on the presence of microplastics in terrestrial Antarctic environments, although they were recently reported in a freshwater stream, with their presence attributed likely to air transport (González-Pleiter et al., 2020).
Since 1968, various countries have established facilities on the Fildes Peninsula, generating great environmental pressure resulting from the scientific and logistical activities that take place in the area (Lu et al., 2012; Amaro et al., 2015). The high density of the facilities and the varied human activities in the region often clash with the environmental standards laid down in the Protocol on Environmental Protection to the Antarctic Treaty (Peter et al., 2013). Since human activities in the Antarctic are the main source of microplastic contamination, the objective of this study was to evaluate its occurrence and distribution on the adjacent soil and intertidal sediments of Fildes Bay, Antarctica.
Materials and Methods
Study Area and Sampling Site
The study area is the Fildes Peninsula, King George Island (Antarctic Specially Protected Area No. 125), one of the areas in Antarctica with the greatest paleontological interest and an area with a great diversity of organisms, including vertebrates, invertebrates, and flora (Secretariat of the Antarctic Treaty [ATS], 2009). The area contains six permanent Antarctic stations belonging to different countries (i.e., Chile, Russia, Uruguay, and China), built between 1968 and 1994. In the 1980s, the construction of the airport turned the area into a major logistical hub for the Antarctic Peninsula (Braun et al., 2012). A part of the Fildes Peninsula is formed by Ardley Peninsula (62°13′S, 58°54′W), Antarctic Specially Protected Area No. 150, located on the southwest coast of King George Island (Fildes Bay). Ardley Island was designated as a protected area on account of the diverse assemblage of bird species that breed on it and to allow the study of their ecology and the factors that affect their populations. Ardley Island also has developed an outstanding flora, with species of lichens, mosses, and vascular plants (Secretariat of the Antarctic Treaty [ATS], 2009) (Figure 1).
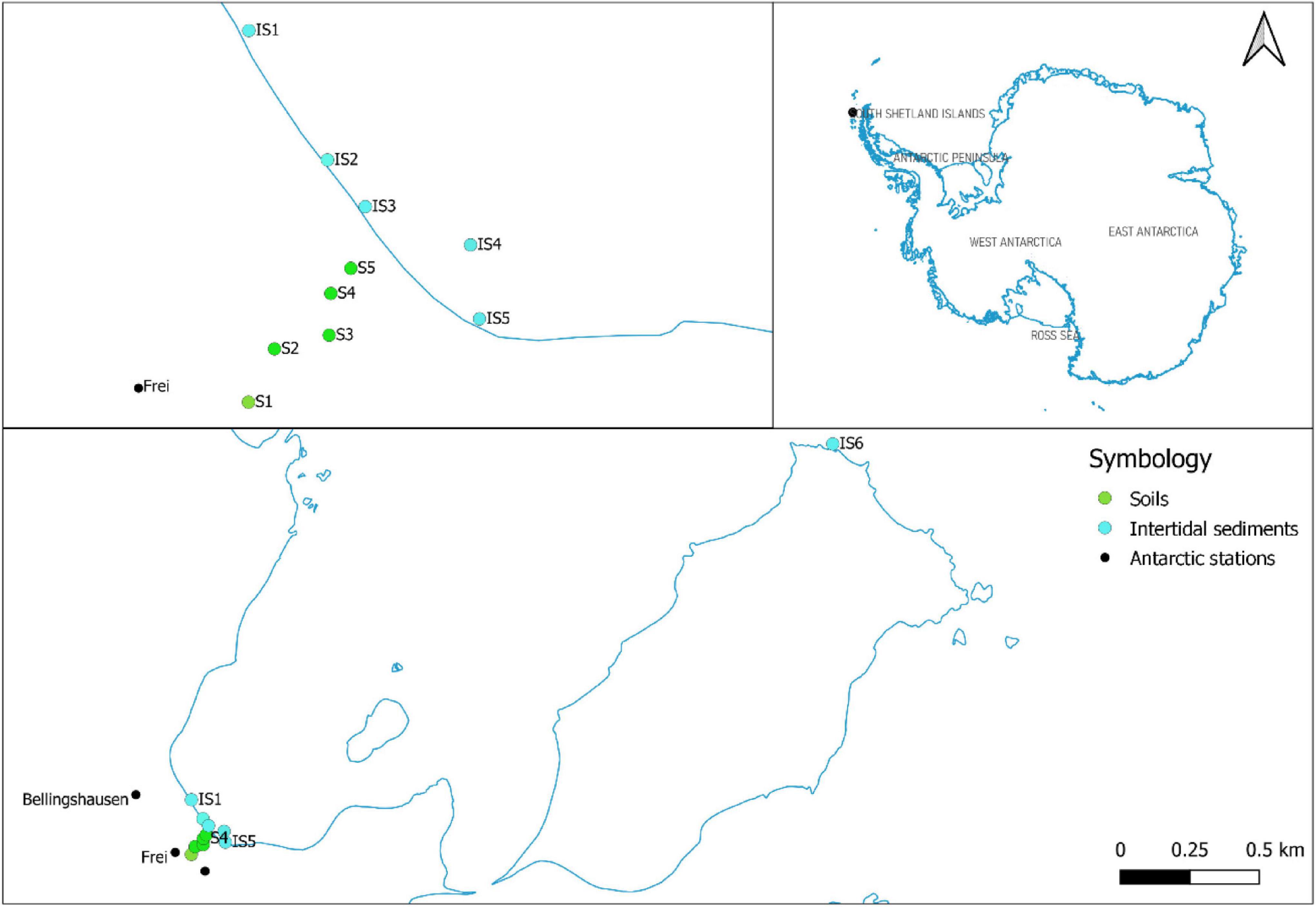
Figure 1. Sampling areas on Fildes Bay and Ardley Island, Antarctica. Green points indicate soil samples (i.e., S1–S5), and light blue points represent intertidal sediments (i.e., IS1–IS6).
The study area was divided into two sampling sites (Figure 1), namely, Antarctic stations and intertidal zones.
Sample Collection and Analysis
Sampling was performed in December 2018. In areas corresponding to Antarctic stations, five soil samples were collected along a transect stretching from the Frei Antarctic station to Fildes Bay (i.e., S1–S5) (Figure 1). In intertidal zones, five sediment intertidal samples were collected along the shore zone (i.e., IS1–IS5). In addition, an intertidal sediment sample (i.e., IS6) was collected from the shore of Ardley Island as a reference from an area free of human activities. An amount of 200 ml of soil and 500 ml of intertidal sediment was extracted at a depth of 0–1 cm using a metal spatula that had been previously washed with acetone. The soil samples were stored in aluminum foil, and the intertidal sediments were collected in glass bottles; they were then refrigerated at 4°C.
To extract the microplastics, the method described by Thompson et al. (2004) and modified by Browne et al. (2010) was used. In brief, 50 ml of the sample was suspended in 100 ml of supersaturated solution of NaCl (1.2 kg NaCl/L), previously filtered by filter paper with a pore size of 1 μm (Advantec Grade NO.5C size 11 cm). This solution was stirred for 30 s, and after 2 min, the particles in the supernatant were separated from the solution using a glass filter under a vacuum with a pore size of 1.6 μm (glass fiber prefilters; Merck Milipore Ltd.). This step was repeated three times for each sample. To minimize contamination by airborne microplastics during drying at room temperature, the filters were placed in Petri dishes and kept inside a glass box.
The plastic particles were separated from non-plastic material and counted using metal dissecting forceps and a stereomicroscope (Olympus SZ61 40X, Japan). Particles with a length of less than 5 mm and greater than 1 μm were considered microplastics GESAMP, 2019 [Group of Experts on the Scientific Aspects of Marine Environmental Protection (GESAMP) (United States)]. Fourier-transform infrared spectroscopy (FT-IR) (Spotlight 400 Perkin Elmer) was used to identify the nature of the polymer in the microplastics from the sampling sites. The attenuated total reflectance (ATR) micro-imaging technique with a germanium crystal was used. A spectral range between 750 and 4,000 cm–1 and a pixel of size 6.25 μm was used. The spectra carry the data with an interval of 3 μm and a resolution of 6 cm–1. Samples from soil and sediments are expressed in volumes to enable comparisons between sample types. All spectra were compared with fused deposition modeling (FDM) FT-IR and Raman spectral libraries and libraries created by the laboratory that analyzed the samples.
Analysis of Physicochemical Parameters in the Collected Samples
Organic matter: The organic matter content in the soil and intertidal sediment samples was determined based on the method described by Heiri et al. (2001). Soil and intertidal sediment were dried at 60°C for 72 h. One g of soil/sediment was homogenized in a mortar and combusted in a muffle furnace at 550°C for 4 h. Finally, the sample was weighed to determine the organic matter content (%) by loss ignition. The results are expressed in dry matter.
Particle size: Samples were dried at room temperature and sieved using a 2-mm sieve. Subsequently, organic matter was removed from each sample using 30% hydrogen peroxide. The sample was deposited in a funnel with a filter paper with a pore size of 1 μm (Advantec Grade NO.5C size 11 cm) and cleaned with milli-Q water. Then, the sample was introduced into a dispersion unit until 10–20% laser obscuration was reached (Malvern Mastersizer 3000S with Hydro EV dispersion unit, United Kingdom). Finally, the results were analyzed using Gradistat v.8 to obtain the average particle size (Blott, 2010).
pH: Soil and intertidal sediment samples were dried at room temperature and homogenized in a mortar. Subsequently, each sample was sieved using a 2-mm sieve. Subsequently, 1 g of sample was placed in 50 ml of Milli-Q water (20°C). To homogenize the sample, a magnetic stirrer was used for 5 min at 225 rpm. The sample was left to stand for 2 h, at which point, the pH in the supernatant was determined using a pH meter (Hanna Edge, United States).
Maps
The sampling area map was prepared using the QGIS 3.14 software (QGIS.org, 2020) and the layers were available in Quantarctica (2019).
Statistical Analyses
To explore the variance of the environmental variables (e.g., fibers, fragments, organic matter, particle size, and pH) in the data set, a Euclidean distance matrix was built, and then a principal component analysis (PCA) was performed. Then, a Shapiro-Wilk test was carried out to test the normal data distribution. A Kruskal-Wallis test and ANOVA were carried out to verify the existence of significant differences between soils and intertidal sediments. A Kendall correlation analysis between particles—whether fibers or fragments—and organic matter, particle size, and pH were performed. The level of significance was set at p = 0.05. All statistical analyses were performed with the R Studio software version 4.0.2.
Results
This study revealed the presence of microplastics in soils and intertidal sediments at 81% of the sites analyzed at Fildes Bay, Antarctica. Five topsoil samples (i.e., S1–S5), five intertidal sediments (i.e., IS1–IS5), and a reference sample (i.e., IS6) were analyzed. Plastic debris after treatment was classified as fibers or fragments based on size, and the nature of the polymer was characterized using FT-IR. Microplastic occurrence (i.e., fibers and fragments), organic matter, particle size, and pH in soils and sediments, respectively, are shown in Figure 2. Plastic fragments (length < 500 μm) were detected in all soil samples, while the presence of fiber was only observed in sample S1 (1 particle/50 ml). The distribution of the fragments in the samples varied from 4 to 37 particles/50 ml sample. The highest occurrence was observed in S5, which is the sample from closest to the shore. Samples taken far from the shore (i.e., S1–S4) presented an occurrence of fragments between 4 and 11 particles/50 ml. The soil organic matter content varied from 4.4 to 8.2%. Similarly, the soil particle size in the samples ranged from 40.6 to 323.6 μm. The soil was considered neutral, with a pH ranging from 6.9 to 7.5. Regarding the evaluated physicochemical variables, no trends were identified in terms of proximity to the coast.
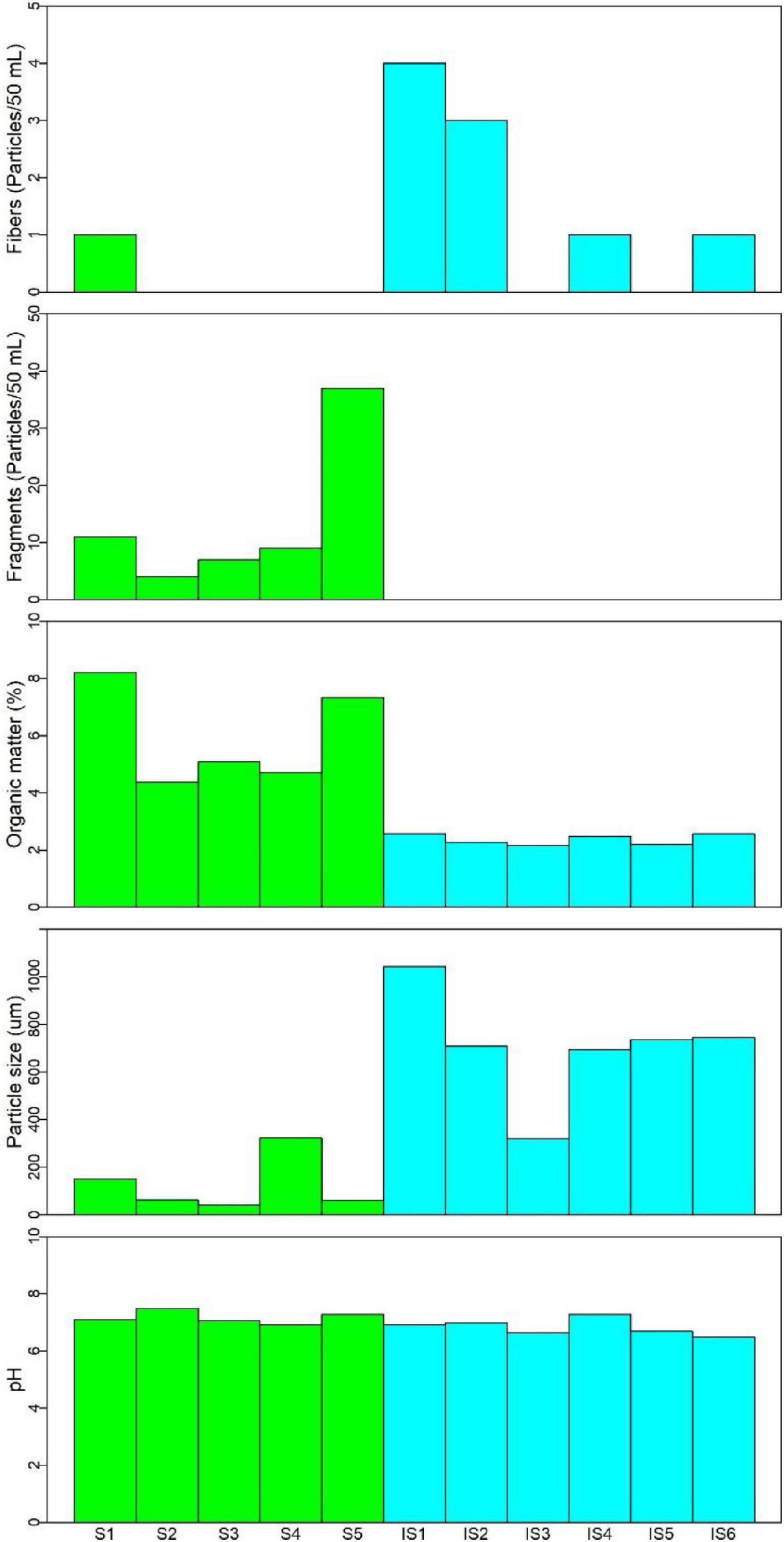
Figure 2. The abundance of microplastics (i.e., fibers and fragments) and determination of an organic matter, particle size, and pH in soils (i.e., S1–S5) and intertidal sediments (i.e., IS1–IS6) of Fildes Bay, Antarctica.
In the intertidal sediments, only fibers (length < 2,000 μm) were observed, ranging from 1 to 4 particles/50 ml. Samples IS1 (4 particles/50 ml) and IS2 (3 particles/50 ml) presented the highest detected abundances; these samples were taken at sites close to the effluent. Abundances in the other intertidal samples (i.e., IS3–IS5) ranged from 0 to 1 particle/50 ml. The inspection of sample IS6 (i.e., protected area, control) revealed a transparent, brilliant, and hard fiber (Figure 3D). We recognized its similarity to the ropes used on boats; however, during sample preparation for the FT-IR analysis, the sample was lost and could not be further identified. The physicochemical values are indicative of homogeneous organic matter in the intertidal sediments (2.2–2.6%). The particle size ranged from 318.9 to 1,042.8 μm. Similar to the soils, the intertidal sediments presented a neutral range (6.5–7.3).
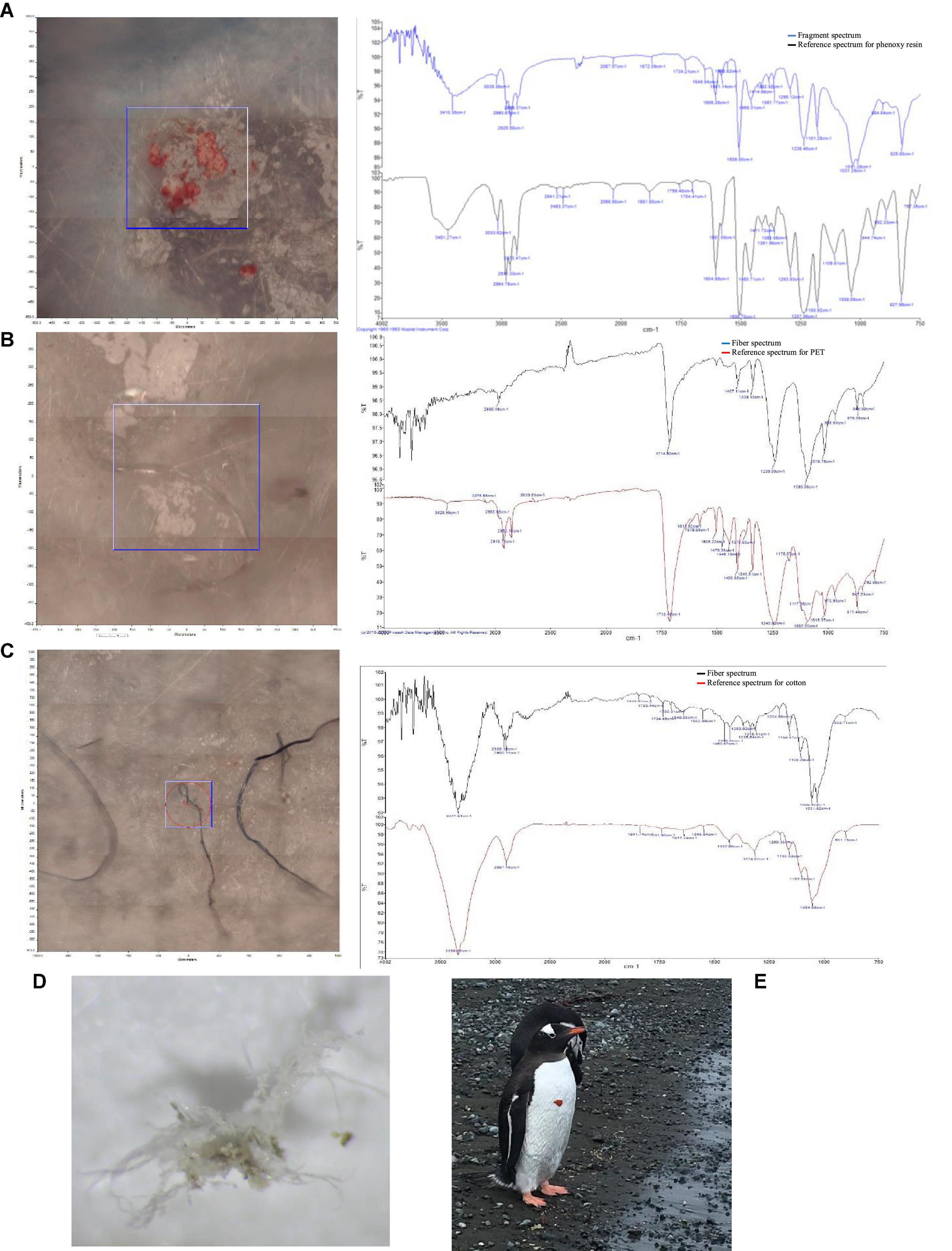
Figure 3. Photograph and spectrum of an orange tree fragment of phenoxy resin (A) collected in soils, PET fiber (B), cotton fiber (C) and a fiber found at the site IS6 (D) from intertidal sediments and a mesoplastic piece on P. papua (E) in Fildes Bay, Antarctica.
Fragments and particle size presented the greatest variability along the PC1 axis, which captured 83.67% of the total variance (Figure 4). The abundance of the type of plastic debris (i.e., fibers and fragments) in soils and intertidal sediments was correlated with physicochemical variables. Specifically, intertidal sediments were characterized by the largest occurrence of fibers, whereas fragments were highly predominant in soil samples. In addition, intertidal sediment sites were influenced by particle size, although soils do not seem to show a significant relationship with other soil variables (i.e., pH and organic matter).
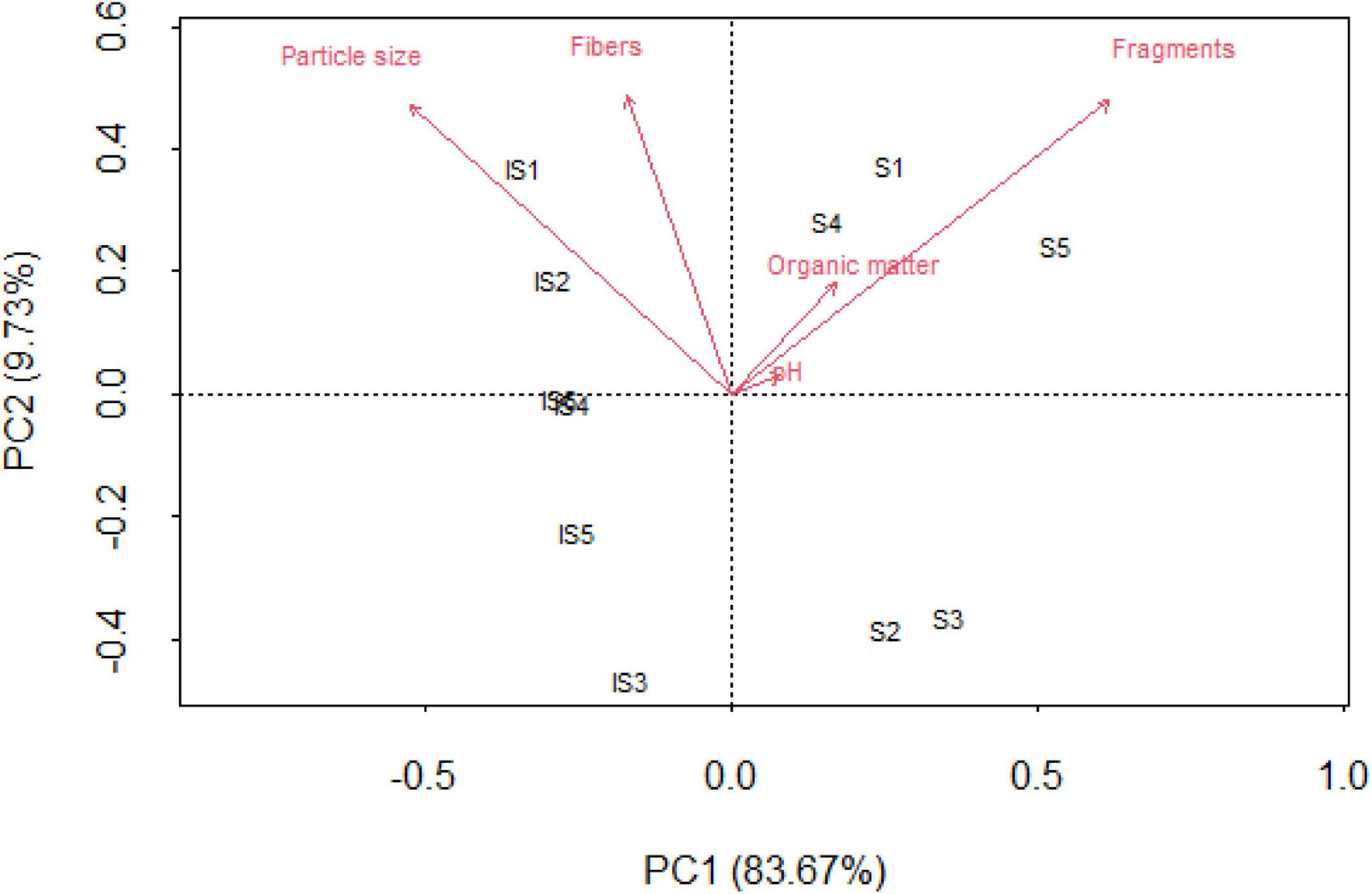
Figure 4. Principal component analysis (PCA) between fibers, fragments, organic matter, particle size, and pH in soils and intertidal sites.
The number of fragments differed significantly between the investigated sample types, i.e., soils and sediments (p = 0.003). In terms of soil physicochemical parameters, organic matter differed significantly between the matrices (p = 0.006), with higher concentrations in soils (S5 = 7.3% and S1 = 8.2%) compared with sediments. Particle size differed significantly between sample types (p = 0.0007), with higher sizes in the intertidal matrix (S1 = 1,042 μm) than in soils. pH did not present significant differences between matrices. Positive correlations between fragments and organic matter (r = 0.7786, p = 0.005) were observed, as well as between fibers and particle size (r = 0.7128, p = 0.014).
All the fragments collected in the soils presented uniformity in color, which was classified as bright orange. The presence of mesoplastics with the same characteristics was also observed in the area, with evident secondary fragmentation in situ. The orange fragment had a high similarity to phenoxy resin (Figure 3A). In contrast, the intertidal plastic fibers presented different colors (i.e., black, blue, red, and transparent). The FT-IR analysis determined that 67% and 50% of all fibers recorded at sites IS1 and IS2, respectively, were cotton fibers (Figure 3C). In addition, for all sites, the analysis indicated that the remaining fibers had a high similarity to polyethylene terephthalate (PET) (Figure 3B).
Discussion
Microplastics in Antarctic Soils
The largest amount of microplastics was detected in soils (1–37 particles/50 ml soil), with fragments predominating, which were associated with high concentrations of organic matter. A comparison with other reports is difficult due to the lack of similar studies on microplastics in Antarctic soils. According to Bläsing and Amelung (2018), our poor understanding of microplastics in soils may be due to the lack of standardized methods. In general, abundances of plastics are highly variable depending on land use and population density; so far plastic debris have been reported in agricultural soils in Shanghai, China (78 ± 12.91 particles/kg) (Liu et al., 2018); roadside soils (1,108 particles/kg) and agricultural soils (3,440 particles/kg) in Yeoju, Republic of Korea (Choi et al., 2021); soils of cultivated areas and the riparian forest zone of Dian Lake, China (7,100–42,960 particles/kg) (Zhang and Liu, 2018); agricultural soils in Nanjing and Wuxi, China (420–1,290particles/kg) (Li et al., 2019); and agricultural soils (2,200–6,875 particles/kg), parks (6,250 ± 3,776 particles/kg), industrial areas (5,780 ± 3,251 particles/kg), and dumps (2,429 ± 1,817 particles/kg) in Lahore, Pakistan (Rafique et al., 2020). The values found in the present study are far lower than those listed above, suggesting that the intensity of the land use and human activities clearly determine the occurrence of plastic debris in soils.
Fragments were observed in all investigated soils, while only one fiber, at site S1, was identified (Figure 2). The fragments had the same appearance, shape, and orange color as those observed in the coatings of the neighboring facilities, which present deterioration associated with local environmental and climatic conditions. This suggests that local land use is the main driver of soil fragments on Fildes Bay. Land use may in part explain the presence of a certain type of microplastic, such as in agricultural soils, which reach high fiber abundances due to the use of sewage sludge as fertilizer (Liu et al., 2018; Corradini et al., 2019; Jiang et al., 2020). Wind also plays an important role in the investigated area, as it reaches speeds of over 100 km/h (Cerda, 2006). In the province of Sakarya, Turkey, Kaya et al. (2018) reported that fibers were more abundant than fragments in the air, with microplastics having a presence of up to four orders of magnitude higher in the air than in soils. This is consistent with the findings of Wright et al. (2020) who recorded atmospheric deposition rates ranging from 575 to 1,008 microplastics/m2/day in the city of London, with the representativeness of 92% of fibers. This finding could explain the low abundance of fibers in soils, which might have been constantly resuspended from the soils or transported to other areas. Furthermore, it has been shown that particle density is decisive in their deposition in coastal systems, since less dense particles tend to settle downwind, in contrast to denser particles, whose settling is not determined by wind, with deposition along the coast (Browne et al., 2010). Finally, once in the soil, the behavior of microplastics varies; according to Waldschläger and Schüttrumpf (2020), fibers that have a smaller diameter reach greater infiltration depths, in contrast to the fragments, which infiltrate less due to the entanglement of the angular particles in the pores.
The highest concentration of fragments was recorded at site S5, the closest to the sea and, therefore, the lowest, which could also suggest a certain degree of runoff as a result of melting snow. Site S5 is also an area with a high-degree of human activity, the use of which is mainly associated with the transit of towing machinery for small boats and motorized vehicles to assist in logistics and maintenance tasks for scientific and tourism purposes. Based on a visual inspection, the fragments even show a degree of secondary fragmentation in situ. The determination of their composition by FT-IR shows a high coincidence with phenoxy resin (Figure 3A), which has a high density and is used as a flexibilizer for cross-linked phenolic and epoxy formulations in adhesives, coatings, and compounds and to make compatible mixtures of various plastic materials (Song et al., 2015; Jones-Williams et al., 2020). Previous studies have not frequently reported the detection of synthetic resins in the environment; however, the detection of this material is very likely since these resins, especially phenoxy resins, are often blended with plastics (Paler et al., 2021). For example, microplastics derived from thermoplastic road-surface marking paints (Horton et al., 2017) and ship paints (Song et al., 2014) have been described. In terms of abundance, paint resins are comparable to microplastics, together reaching 75% of total particles in the surface waters of Jinhae Bay, South Korea (Song et al., 2015). In surface waters between Adelaide Island, Antarctica, and the mid Scotia Sea, it has been reported that, although phenoxy resin has local sources, it has a long range and, together with polyethylene, is one of the most common resins (41%) (Jones-Williams et al., 2020). Therefore, although we did not verify its presence in the intertidal sediments, it is highly probable that it is present not only in the soils of Fildes Bay but also offshore. In addition, transport through biovectors from terrestrial to aquatic environments, such as that which took place via the adhesion of a piece of mesoplastic to the chest of an adult individual of the species Pygoscelis papua (Forster, 1781), should not be ruled out (Figure 3E).
Our study showed a relationship between the fragments and organic matter of the soils. This contrasts with the results reported by Watteau et al. (2018), who analyzed soils enriched with municipal compost in France and concluded that microplastics in soils do not present an association with organic matter. In experimental soils with different microplastic sizes, Dong et al. (2021) reported that the presence of polystyrene and polytetrafluoroethylene microplastics caused a reduction in the soil organic matter. In our research, organic matter (%) was determined using the method of Heiri et al. (2001), who indicate that weight loss is proportional to the amount of organic carbon contained in the sample. However, Rillig (2018) emphasized that the methods used for quantitation of organic carbon in soil can cover “invisible” microplastics because plastics are composed mainly of carbon. This could explain the relationship that we verified between the increase in organic matter in sites and a high abundance of fragments. As organic matter (%) does not seem to be a precise variable, it is suggested that future studies employ more specific analyses regarding the composition of the type of carbon present in soils.
The information available on exposure and effects of microplastics on Antarctic organisms is similarly scarce. Plastic particles can persist, accumulate, and eventually affect the functioning and biodiversity of terrestrial ecosystems (Rillig, 2012). Habib et al. (2020) isolated bacteria Pseudomonas sp. ADL15 and Rhodococcus sp. ADL35 from soil samples collected in Victoria Land, Ross Sea, Antarctica. They corroborated positive growth in a medium containing fragments of polypropylene (Habib et al., 2020). In addition, bacterial assemblages with distinct community structures colonized the PE microplastics (Huang et al., 2019). In general, soil fauna has an active intake of microplastics, with a consequent alteration of its intestinal microbiome and adverse effects on motility, growth, metabolism, reproduction, and mortality in various combinations (Büks et al., 2020), especially at high concentrations and with small particle sizes. The small size and large surface area of microplastics allow the adsorption of pollutants on their surfaces, increasing the local concentration in soils and generating potential ecological risks (Moore, 2008; Ashton et al., 2010; Rillig, 2012; Liu et al., 2018). Finally, although it was not found in the intertidal zone, phenoxy resin could be transported offshore; according to European Chemicals Agency (2021), this substance is toxic to aquatic life, with long-lasting effects.
Microplastics in Antarctic Intertidal Sediments
The intertidal sediments were dominated by fibers (up to 4 fibers/50 ml sediment) (Figure 2), which were associated with large particle sizes. Similar abundances have been reported by Reed et al. (2018), who recorded up to 3 fibers/10 ml sediment in North Cove, Adelaide Island, Antarctic Peninsula. A previous study carried out by Rebolledo and Franeker (2015) on Cape Shirreff did not record microplastics in intertidal sediments, despite the presence of macroplastics in the area. Although the information on microplastics in Antarctic intertidal sediments is limited (Table 1), these data could suggest a low abundance compared with those of deep sediments. Close to the study area, Waller et al. (2017) carried out research in semi-deep sediments (6–60 m) in Mackellar Inlet, Almirantazgo Bay. They reported between 16 and 766 particles/m2; however, they did not find a clear pattern of abundance or distribution, and the proportion of fibers and fragments found was not reported. Consistent with our findings, the predominance of fibers (42.8%) was verified in semi-deep sediments (25–140 m) in Terra Nova Bay (Munari et al., 2017). Fibers also predominated for all recorded microplastics, with the exception of one particle, in shallow sediments (0–20 m) on Adelaide Island (Reed et al., 2018). This pattern of fiber prevalence has also been reported in Singapore mangroves (Nor and Obbard, 2014). In addition, Browne et al. (2010) analyzed the sediments in the high tide line of the Tamar Estuary, United Kingdom, finding values of up to 1 fiber/50 ml sediment, similar to those of Fildes Bay. A low abundance in the intertidal sediments of coastal systems in Plymouth, United Kingdom, was also described by Thompson et al. (2004), who indicated that fibers increased in subtidal sediments. In marine environments of the Alboran Sea (42 m deep), an abundance of 45 fibers/50 ml sediment has been described (Sanchez-Vidal et al., 2018). Although Sanchez-Vidal et al. (2018) did not include other forms of particles in deep sediments of southern European seas in their study, they estimated that around 20% of the fibers found had accumulated in the open sea beyond 2,000 m of water depth. This could be explained by the fibers having an abundance up to four orders of magnitude higher in deep sediments than in the surface waters of the Atlantic Ocean, Mediterranean Sea, and the Indian Ocean (Woodall et al., 2014). In contrast, very deep sediments (136–3,633 m) analyzed on the Antarctic Peninsula, South Sandwich Islands, and South Georgia were shown to be dominated by fragments, which accounted for 56% of the total (Cunningham et al., 2020). Density could partly explain the distribution of the fibers, once in the water, in the water column, and in the deep sediments (Thompson et al., 2004; Sanchez-Vidal et al., 2018). In general, depth seems to be a determining factor in the abundance of certain forms of microplastics, with fibers predominating in intertidal environments and shallow areas.
Regarding the values at each site, the highest abundances were recorded at the sites closest to the Frei base effluent (i.e., IS1 and IS2), and are consistent with those reported in marine sediments near wastewater from the Rothera station west of the Antarctica Peninsula (Reed et al., 2018). In addition, 67% and 50% of all fibers recorded at sites IS1 and IS2, respectively, were cotton fibers, confirming the contribution of wastewater effluents. Although sludge acts as a microplastic retention agent, with estimated retention rates of 75.7% to 90% (Corradini et al., 2019; Jiang et al., 2020), fibers have also been found in the effluents (37.7–60.8%) of secondary treatment systems (Jiang et al., 2020), and up to 14 fibers/L have been observed in primary treatment systems (Talvitie et al., 2015). Given this scenario, a negative situation is projected for some facilities, as their treatment systems are prone to failure due to both the low temperatures that impede biological processes and the lack of maintenance and sludge evacuation, resulting in continuous emission of waste into the environment (Hughes and Blenkharn, 2003; Gröndahl et al., 2009; Morales Calvo, 2013). In addition, 37% of permanent stations and 69% of non-permanent stations do not have any type of wastewater treatment (Morales Calvo, 2013). Due to the high population density in the north of the Antarctic Peninsula, microplastics derived from personal care products and laundry are likely to be concentrated there (Waller et al., 2017). Microfibres from laundry released in wastewater may be a more substantial source of microplastic pollution compared with other sources as personal care products (Waller et al., 2017). However, the contribution of fibers from sources related to other types of local human activities should not be ruled out.
Regarding sediment characteristics, we found that a greater fiber abundance is related to large particle sizes. In other regions, studies on intertidal sediments have not identified a pattern in terms of microplastic abundance and sediment particle size, with high abundances found in both sediments larger than 2 mm in Canada (Cluzard et al., 2015) and fine sediments in Singapore (Nor and Obbard, 2014). The physical interactions between microplastics and sediments are not yet fully understood due to the diversity of shapes, diameters, lengths, surface areas, and densities, among other characteristics, which makes it difficult to know where they will settle and at what sedimentation rate (Browne et al., 2010; Cluzard et al., 2015).
All the fibers found in the intertidal zone were made of PET, also called polyester (Figure 3B). This material is widely used in the manufacturing of cold-weather clothing (Gonzalez et al., 1998; Das and Gersak, 2014; Gnanauthayan et al., 2017). Polyethylene terephthalate has a density of 1.34–1.39 g/cm3, and according to Browne et al. (2010), almost 80% of the microplastics recorded on the coastline are denser, which, as they state, maybe due to its slow degradation, longer contact time with abrasive particles in the sediment, or transport by the wind of less dense plastics toward the coasts and inland. This could explain the presence of this type of polymer composition at all sites and in other regions, as reported by Naji et al. (2017) in a study on intertidal sediments of Hormozgan, Persian Gulf, in which fibers predominated by 88%, PET was identified as the most abundant polymer (i.e., 41%), and high concentrations in wastewater release zones were found. In Portugal and Morocco, 29% of the fibers found in intertidal sediments were made of PET (Velez et al., 2019). Similarly, a large-scale study conducted at high tide in intertidal sediments (0–5 cm) in Auckland, New Zealand, reported that fibers predominated (i.e., 88%) and PET accounted for 22% of all microplastics analyzed (Bridson et al., 2020). This abundance of PET in relatively shallow sediments was also described by Woodall et al. (2014) in the Atlantic Ocean, the Mediterranean Sea, and the Indian Ocean. Similarly, it was reported that 12.9% of the fibers found in the deep sea were polyester (Sanchez-Vidal et al., 2018). It has been observed that many marine microplastics have this composition and that their distribution and sinking rate presumably differ from those of other high-density microplastics (Cunningham et al., 2020).
In marine environments of Victoria Land, Antarctica, Sfriso et al. (2020) collected benthic invertebrates, reporting that species contained between 0.01 and 3.29 items/mg–1, and that bivalves and gastropods displayed the highest microplastic contamination. Despite these findings, no evident accumulation through the food web was detected. In other regions, the dominance of PET fibers in coastal ecosystems on the coast of Pará, Brazil, was described by Morais et al. (2020), who reported that plastic fibers accounted for 84% of plastics ingested by individuals of Bunodosoma cangicum (Belém and Preslercravo, 1973), with PET being the main polymer (44.7%), and that this organism presented a higher amount of plastic debris in the more populated sampling sites.
It is still not clear if the role of microplastics is that of pollutant or merely contaminant; however, it is necessary to deepen our knowledge on the distribution and effects of microplastics and additives at all levels of the food web to evaluate their effects on marine organisms and ecosystems from a broader perspective (Sfriso et al., 2020). Aragaw and Mekonnen (2021) have recommended that microplastic contamination investigation guidelines emphasize experimental ecotoxicological studies and risk assessments for aquatic organisms. Vighi et al. (2021) stressed that key aspects in the production of an adequate risk assessment are frequently overlooked and that the impacts of environmental variables on additive leaching must be included.
Pollution of Protected Areas
Ardley Island was selected to assess the base level of contamination in an area with less human activity and no residential, scientific, or military settlements, only two sporadically used shelters. However, it was possible to detect the presence of a fiber particle in the intertidal sediment, which indicates that Antarctic environments with restricted entry and protected for their biological value are not exempt from contamination by microplastics. Thus, the inspection of King George Island has shown clear deficiencies with respect to waste management at some stations in the form of waste storage and the existence of more than 40 waste dumps (Peter et al., 2013). Although in other locations of the continent, there has been an effort regarding the recovery of waste and mitigation of old landfills (Eriksen et al., 2020), the Protocol to the Antarctic Treaty on Environmental Protection has recognized the lack of data on plastics that would allow adequate decision-making and reduction of pollution and has recently made recommendations regarding the use of plastic on the continent (Secretariat of the Antarctic Treaty, 2019a). Among the recommendations are halting the use of personal care products that contain plastic microbeads and considering the use of filtration technologies to reduce the amount of microplastic particles that enter the Antarctic marine environment (Secretariat of the Antarctic Treaty, 2019a,b); however, there are no recommendations focused on soils. This is worrying as our study has shown that the soil-intertidal sediment interface is not necessarily a continuum and that there is a difference in the composition of plastic particles, suggesting specific sources of microplastics in each matrix at Fildes Bay. Therefore, an understanding of microplastic pollution in Antarctica requires an increased focus on identifying sources and sinks.
Methods Used in Microplastic Research in Antarctica
In general, few studies are available on marine sediments in Antarctica, with most focusing on subtidal sediments and none so far on soils (Table 1). Research efforts are made mainly on the Antarctic Peninsula, which makes it difficult to understand what occurs inside the Antarctic Continent. Antarctica is characterized by difficult-to-access sites, low temperatures, and strong winds; this last factor can generate a high degree of contamination during sample collection. Given this scenario, sample collection techniques, microplastic selection criteria, and sample treatment vary among studies. Although all authors have used FT-IR to determine the composition of the particles, the results are expressed in different units of abundance (e.g., particles/ml and particles/m2). In these cases, we suggest the incorporation of a standardized expression of units whenever possible, such as particles per surface or per volume. In our study, for comparative purposes, we decided to use the same expression for both matrices, which allowed us to identify a greater abundance in soils (average = 13.8 particles/50 ml) than in intertidal sediments (average = 1.5 particles/50 ml). This expression of the results permitted us to corroborate that even though the studied environments are contiguous, their abundances were independent and that contrary to what we expected, surface intertidal sediments did not behave as a sink for land-based sources. For instance, at site IS5, the presence of microplastics was not recorded, even though the adjacent soils were visibly affected by high abundances of microplastics as a result of the infrastructure that had been affected by a fire. In addition, despite the continuous release of fibers from the effluents, a low abundance was found at all intertidal sediment sites, which could be due to environmental dynamics such as tidal waves that affect intertidal sediments. In this regard, Cluzard et al. (2015) observed that a greater influence of tides and waves caused a lower accumulation of microplastics. Thus, although the abundances alone did not allow us to explain the distribution of microplastics in the study area, improving their comparability facilitates understanding of the complex intrinsic processes associated with Antarctic matrices.
Conclusion
The results of this study revealed the occurrence of microplastics in soils and intertidal sediments at 81% of the analyzed sites at Fildes Bay, Antarctica. This is the first study to report the presence of microplastics in Antarctic soils, with a high abundance of phenoxy resin fragments in Antarctic soils near stations, probably associated with deterioration of infrastructure coatings. Compared with terrestrial abundances, the abundance of microplastics in intertidal sediments was lower and consistent with results previously reported in shallow Antarctic sediments. Polyethylene terephthalate fibers predominated in the intertidal sediments, especially at the sites near the effluent from the Frei base, where the presence of cotton fibers was confirmed. Although this base has a treatment system, the situation of the bases affected by logistical limitations or without treatment systems is worrying. In addition, microplastics were found on Ardley Island, corroborating that there is contamination in protected areas. Regarding the influence of environmental variables, we observed a relationship between fragments and organic matter; however, this relationship seems to be a result of the composition of the polymers. In addition, the observed relationship between fibers and particle size was not conclusive, and to date, there are no studies that confirm a clear relationship. This investigation shows the importance of the early identification and management of local sources of microplastics and the study of each matrix, as the behavior of microplastics seems to differ depending on the substrate into which they are released. Paint resins should be considered in future studies, as they have demonstrated their persistence in the environment despite local climatic conditions. It is suggested that the Fildes Bay area be monitored to evaluate the behavior of these microplastics in other matrices and their eventual interactions with local fauna. This will make it possible to strengthen efforts to reduce the presence of microplastics in areas of major human activity such as Fildes Bay.
Data Availability Statement
The original contributions presented in the study are included in the article/supplementary material, further inquiries can be directed to the corresponding author.
Author Contributions
AP-B and ROB conceptualized the study and provided funding for the study. AP-B performed the fieldwork and laboratory analysis. AP-B, AA, KM, and ROB wrote the manuscript. All authors contributed to the article and approved the submitted version.
Funding
This project was funded by ANID–Millennium Science Initiative Program–Code ICN2019_015 and ANID-PFCHA/Doctorado Nacional/2017-21170746.
Conflict of Interest
The authors declare that the research was conducted in the absence of any commercial or financial relationships that could be construed as a potential conflict of interest.
Publisher’s Note
All claims expressed in this article are solely those of the authors and do not necessarily represent those of their affiliated organizations, or those of the publisher, the editors and the reviewers. Any product that may be evaluated in this article, or claim that may be made by its manufacturer, is not guaranteed or endorsed by the publisher.
Acknowledgments
This article is part of the doctoral thesis of AP-B, supervised by ROB and AA. AP-B thanks ANID-PFCHA/Doctorado Nacional/2017-21170746 for the financing of their postgraduate studies. ROB is grateful for the financial support of the ANID–Millennium Science Initiative Program–Code ICN2019_015. We would like to thank the Instituto Antártico Chileno (INACH) and Correos de Chile for their support in carrying out this project (PR_01-18) during ECA 55.
References
Amaro, E., Padeiro, A., de Ferro, A. M., Mota, A. M., Leppe, M., Verkulich, S., et al. (2015). Assessing trace element contamination in Fildes peninsula (king George Island) and Ardley Island, Antarctic. Mar. Pollut. Bull. 97, 523–527. doi: 10.1016/j.marpolbul.2015.05.018
Aragaw, T. A., and Mekonnen, B. A. (2021). Distribution and Impact of Microplastics in the Aquatic Systems: A Review of Ecotoxicological Effects on Biota. Microplastic pollution. Sustainable Textiles: Production, Processing, Manufacturing & Chemistry. Singapore: Springer, 65–104.
Ashton, K., Holmes, L., and Turner, A. (2010). Association of metals with plastic production pellets in the marine environment. Mar. Pollut. Bull. 60, 2050–2055. doi: 10.1016/j.marpolbul.2010.07.014
Barnes, D. K., Walters, A., and Gonçalves, L. (2010). Macroplastics at sea around Antarctica. Mar. Environ. Res. 70, 250–252. doi: 10.1016/j.marenvres.2010.05.006
Belém, M. J., and Preslercravo, J. C. (1973). Contribuições ao conhecimento da fauna de Cnidarios do Espírito Santo, Brasil. l. considerações sobre Actiniaria do Município de Aracruz, ES. Bol. Mus. Biol. Prof. Mello Leitão, S. Zoologia, 80, 1–14.
Bläsing, M., and Amelung, W. (2018). Plastics in soil: analytical methods and possible sources. Sci. Total Environ. 612, 422–435. doi: 10.1016/j.scitotenv.2017.08.086
Blott, J. (2010). Gradistat Version 8.0: A Grain Size Distribution and Statistics Package for the Analysis of Unconsolidated Sediments by Sieving or Laser Granulometer. Berkshire: Kenneth Pye Associates Ltd.
Braun, C., Mustafa, O., Nordt, A., Pfeiffer, S., and Peter, H.-U. (2012). Environmental monitoring and management proposals for the Fildes region, King George Island, Antarctica. Polar Res. 31:18206. doi: 10.3402/polar.v31i0.18206
Bridson, J. H., Patel, M., Lewis, A., Gaw, S., and Parker, K. (2020). Microplastic contamination in Auckland (New Zealand) beach sediments. Mar. Pollut. Bull. 151:110867. doi: 10.1016/j.marpolbul.2019.110867
Browne, M. A., Crump, P., Niven, S. J., Teuten, E., Tonkin, A., Galloway, T., et al. (2011). Accumulation of microplastic on shorelines woldwide: sources and sinks. Environ. Sci. Technol. 45, 9175–9179. doi: 10.1021/es201811s
Browne, M. A., Galloway, T. S., and Thompson, R. C. (2010). Spatial patterns of plastic debris along estuarine shorelines. Environ. Sci. Technol. 44, 3404–3409. doi: 10.1021/es903784e
Bruni, V., Maugeri, T. L., and Monticelli, L. (1997). Faecal pollution indicators in the Terra Nova Bay (Ross Sea, Antarctica). Mar. Pollut. Bull. 34, 908–912. doi: 10.1016/S0025-326X(97)00050-7
Büks, F., Loes, van Schaik, N., and Kaupenjohann, M. (2020). What do we know about how the terrestrial multicellular soil fauna reacts to microplastic? Soil 6, 245–267. doi: 10.5194/soil-6-245-2020
Cerda, J. C. (2006). Climatología de la Península Antártica y de la Base Presidente Eduardo Frei Montalva. Available online at: http://www2.dgf.uchile.cl/ACT19/COMUNICACIONES/OtrosTextos/Climatologia%20Edo%20Frei_ABSTRACT.pdf [Accessed June 02, 2021]
Choi, Y. R., Kim, Y. N., Yoon, J. H., Dickinson, N., and Kim, K. H. (2021). Plastic contamination of forest, urban, and agricultural soils: a case study of Yeoju City in the Republic of Korea. J. Soils Sediments 21, 1962–1973. doi: 10.1007/s11368-020-02759-0
Cincinelli, A., Scopetani, C., Chelazzi, D., Lombardini, E., Martellini, T., Katsoyiannis, A., et al. (2017). Microplastic in the surface waters of the Ross Sea (Antarctica): occurrence, distribution and characterization by FTIR. Chemosphere 175, 391–400. doi: 10.1016/j.chemosphere.2017.02.024
Cluzard, M., Kazmiruk, T. N., Kazmiruk, V. D., and Bendell, L. I. (2015). Intertidal concentrations of microplastics and their influence on ammonium cycling as related to the shellfish industry. Arch. Environ. Contam. Toxicol. 69, 310–319. doi: 10.1007/s00244-015-0156-5
Corradini, F., Meza, P., Eguiluz, R., Casado, F., Huerta-Lwanga, E., and Geissen, V. (2019). Evidence of microplastic accumulation in agricultural soils from sewage sludge disposal. Sci. Total Environ. 671, 411–420. doi: 10.1016/j.scitotenv.2019.03.368
Council of Managers of National Antarctic Program [CONMAP] (2020). COMNAP Antarctic Facilities. Available online at: https://github.com/PolarGeospatialCenter/comnap-antarctic-facilities/tree/master/dist (01 june, 2020). [Accessed June 15, 2021].
Cunningham, E. M., Ehlers, S. M., Dick, J. T., Sigwart, J. D., Linse, K., Dick, J. J., et al. (2020). High abundances of microplastic pollution in deep-sea sediments: evidence from Antarctica and the Southern Ocean. Environ. Sci. Technol. 54, 13661–13671. doi: 10.1021/acs.est.0c03441
Das, A., and Gersak, J. (2014). Physiological responses of different types clothing in cold weather condition–a wear trial study. Indian J. Fibre Text Res. 39, 33–42.
Dong, Y., Gao, M., Qiu, W., and Song, Z. (2021). Effect of microplastics and arsenic on nutrients and microorganisms in rice rhizosphere soil. Ecotoxicol. Environ. Saf. 211:111899. doi: 10.1016/j.ecoenv.2021.111899
Eriksen, M., Borgogno, F., Villarrubia-Gómez, P., Anderson, E., Box, C., and Trenholm, N. (2020). Mitigation strategies to reverse the rising trend of plastics in Polar Regions. Environ. Int. 139:105704. doi: 10.1016/j.envint.2020.105704
European Chemicals Agency [ECHA] (2021). Substance Infocard 4,4’-Isopropylidenediphenol, Oligomeric Reaction Products with 1-chloro-2,3-epoxypropane. Available online at: https://echa.europa.eu/es/substance-information/-/substanceinfo/100.105.541#CAS_NAMEScontainer [Accessed June 20, 2021].
GESAMP (2019). Guidelines for the Monitoring and Assessment of Plastic Litter and Microplastics in the Ocean (Kershaw P.J., Turra A. and Galgani F. editors), (IMO/FAO/UNESCO-IOC/UNIDO/WMO/IAEA/UN/UNEP/UNDP/ISA Joint Group of Experts on the Scientific Aspects of Marine Environmental Protection). Rep. Stud. GESAMP No. 99. 130. Available online at: http://www.gesamp.org/publications/guidelines-for-the-monitoring-and-assessment-of-plastic-litter-in-the-ocean [Accessed May 08, 2021]
Gnanauthayan, G., Rengasamy, R. S., and Kothari, V. K. (2017). Heat insulation characteristics of high bulk nonwovens. J. Text. Inst. 108, 2173–2179. doi: 10.1080/00405000.2017.1316697
Gonzalez, R. R., Endrusick, T. L., and Santee, W. R. (1998). Thermoregulatory responses to cold: effects of handwear with multi-layered clothing. Aviat. Space Environ. Med. 69, 1076–1082.
González-Pleiter, M., Edo, C., Velázquez, D., Casero-Chamorro, M. C., Leganés, F., Quesada, A., et al. (2020). First detection of microplastics in the freshwater of an Antarctic Specially Protected Area. Mar. Pollut. Bull. 161:111811. doi: 10.1016/j.marpolbul.2020.111811
Gröndahl, F., Sidenmark, J., and Thomsen, A. (2009). Survey of waste water disposal practices at Antarctic research stations. Polar Res. 28, 298–306. doi: 10.1111/j.1751-8369.2008.00056.x
Habib, S., Iruthayam, A., Abd Shukor, M. Y., Alias, S. A., Smykla, J., and Yasid, N. A. (2020). Biodeterioration of untreated polypropylene microplastic particles by Antarctic bacteria. Polymers 12:2616. doi: 10.3390/polym12112616
Heiri, O., Lotter, A., and Lemcke, G. (2001). Loss on ignition as a method for estimating organic and carbonate content in sediments: reproducibility and comparability of results. J. Paleolimnol. 25, 101–110. doi: 10.1023/A:1008119611481
Horton, A. A., Svendsen, C., Williams, R. J., Spurgeon, D. J., and Lahive, E. (2017). Large microplastic particles in sediments of tributaries of the River Thames, UK–Abundance, sources and methods for effective quantification. Mar. Pollut. Bull. 114, 218–226. doi: 10.1016/j.marpolbul.2016.09.004
Huang, Y., Zhao, Y., Wang, J., Zhang, M., Jia, W., and Qin, X. (2019). LDPE microplastic films alter microbial community composition and enzymatic activities in soil. Environ. Pollut. 254:112983. doi: 10.1016/j.envpol.2019.112983
Hughes, K. A., and Blenkharn, N. (2003). A simple method to reduce discharge of sewage microorganisms from an Antarctic research station. Mar. Pollut. Bull. 46, 353–357. doi: 10.1016/S0025-326X(02)00224-2
International Association of Antarctica Tour Operators [IAATO] (2020). IAATO Antarctic Visitor Figures 2019-2020. Available online at: https://iaato.org/wp-content/uploads/2020/07/IAATO-on-Antarctic-visitor-figures-2019-20-FINAL.pdf [Accessed March 27, 2021].
Isobe, A., Uchiyama-Matsumoto, K., Uchida, K., and Tokai, T. (2017). Microplastics in the Southern Ocean. Mar. Pollut. Bull. 114, 623–626. doi: 10.1016/j.marpolbul.2016.09.037
Jiang, J., Wang, X., Ren, H., Cao, G., Xie, G., Xing, D., et al. (2020). Investigation and fate of microplastics in wastewater and sludge filter cake from a wastewater treatment plant in China. Sci. Total Environ. 746:141378. doi: 10.1016/j.scitotenv.2020.141378
Jones-Williams, K., Galloway, T., Cole, M., Stowasser, G., Waluda, C., and Manno, C. (2020). Close encounters-microplastic availability to pelagic amphipods in sub-antarctic and antarctic surface waters. Environ. Int. 140:105792. doi: 10.1016/j.envint.2020.105792
Kaya, A. T., Yurtsever, M., and Bayraktar, S. Ç (2018). Ubiquitous exposure to microfiber pollution in the air. Eur. Phys. J. Plus 133:488. doi: 10.1140/epjp/i2018-12372-7
Li, Q., Wu, J., Zhao, X., Gu, X., and Ji, R. (2019). Separation and identification of microplastics from soil and sewage sludge. Environ. Pollut. 254:113076. doi: 10.1016/j.envpol.2019.113076
Li, W. C., Tse, H. F., and Fok, L. (2016). Plastic waste in the marine environment: a review of sources, occurrence and effects. Sci. Total Environ. 566, 333–349. doi: 10.1016/j.scitotenv.2016.05.084
Liu, M., Lu, S., Song, Y., Lei, L., Hu, J., Lv, W., et al. (2018). Microplastic and mesoplastic pollution in farmland soils in suburbs of Shanghai. China. Environ. Pollut. 242, 855–862. doi: 10.1016/j.envpol.2018.07.051
Lu, Z., Cai, M., Wang, J., Yang, H., and He, J. (2012). Baseline values for metals in soils on Fildes Peninsula, King George Island, Antarctica: the extent of anthropogenic pollution. Environ. Monit. Assess. 184, 7013–7021. doi: 10.1007/s10661-011-2476-x
Moore, C. J. (2008). Synthetic polymers in the marine environment: a rapidly increasing, long-term threat. Environ. Res. 108, 131–139. doi: 10.1016/j.envres.2008.07.025
Morais, L. M. S., Sarti, F., Chelazzi, D., Cincinelli, A., Giarrizzo, T., and Martinelli Filho, J. E. (2020). The sea anemone Bunodosoma cangicum as a potential biomonitor for microplastics contamination on the Brazilian Amazon coast. Environ. Pollut. 265:114817. doi: 10.1016/j.envpol.2020.114817
Morales Calvo, G. (2013). Tratamiento De Aguas Residuales En Áreas Medioambientalmente Protegidas. El caso de la Antártida. Revista Científica Monfragüe Resiliente. Available online at: https://www.eweb.unex.es/eweb/monfragueresilente/paginas/numerosanteriores.html [Accessed January 10, 2021]
Munari, C., Infantini, V., Scoponi, M., Rastelli, E., Corinaldesi, C., and Mistri, M. (2017). Microplastics in the sediments of Terra Nova Bay (Ross Sea, Antarctica). Mar. Pollut. Bull. 122, 161–165. doi: 10.1016/j.marpolbul.2017.06.039
Naji, A., Esmaili, Z., Mason, S. A., and Vethaak, A. D. (2017). The occurrence of microplastic contamination in littoral sediments of the Persian Gulf. Iran. Environ. Sci. Pollut. Res. 24, 20459–20468. doi: 10.1007/s11356-017-9587-z
Nor, N. H. M., and Obbard, J. P. (2014). Microplastics in Singapore’s coastal mangrove ecosystems. Mar. Pollut. Bull. 79, 278–283. doi: 10.1016/j.marpolbul.2013.11.025
Onink, V., Jongedijk, C., Hoffman, M., van Sebille, E., and Laufkötter, C. (2021). Global simulations of marine plastic transport show plastic trapping in coastal zones. Environ. Res. Lett. 16:064053. doi: 10.1088/1748-9326/abecbd
Paler, M. K. O., Leistenschneider, C., Migo, V., and Burkhardt-Holm, P. (2021). Low microplastic abundance in Siganus spp. from the Tañon Strait, Central Philippines. Environ. Pollut. 284, 117166. doi: 10.1016/j.envpol.2021.117166
Pertierra, L. R., Hughes, K. A., Vega, G. C., and Olalla-Tárraga, M. Á (2017). High resolution spatial mapping of human footprint across Antarctica and its implications for the strategic conservation of avifauna. PLoS One 12:e0168280. doi: 10.1371/journal.pone.0168280
Peter, H.-U. P., Braun, C., Janowski, S., Ruß, A., Anke, N., and Stelter, M. (2013). The Current Environmental Situation and Proposals for the Management of the Fildes Peninsula Region. Available online at: https://www.umweltbundesamt.de/sites/default/files/medien/461/publikationen/4424.ppd [Accessed February 10, 2021]
Plastics Europe (2020). Plastics - the Facts 2020. An Analysis of European Plastics Production, Demand and Waste Data. Available online at: https://www.plasticseurope.org/application/files/5716/0752/4286/AF_Plastics_the_facts-WEB-2020-ING_FINAL.pdf [Accessed January 29, 2021]
QGIS.org (2020). QGIS Geographic Information System. QGIS Association. Available online at: http://www.qgis.org [Accessed August 09, 2020]
Quantarctica (2019). Downloads. Available online at: https://www.npolar.no/quantarctica/ [Accessed September 18, 2020]
Rafique, A., Irfan, M., Mumtaz, M., and Qadir, A. (2020). Spatial distribution of microplastics in soil with context to human activities: a case study from the urban center. Environ. Monit. Assess. 192, 1–13. doi: 10.1007/s10661-020-08641-3
Rebolledo, E. L. B., and Franeker, J. V. (2015). Impact of Marine Debris on Antarctic fur Seals Arctocephalus gazella at Cape Shirreff: Diet Dependent Ingestion and Entanglement. Den Helder: Institute for Marine Resources & Ecosystem Studies.
Reed, S., Clark, M., Thompson, R., and Hughes, K. A. (2018). Microplastics in marine sediments near Rothera research station. Antarctica. Mar. Pollut. Bull. 133, 460–463. doi: 10.1016/j.marpolbul.2018.05.068
Rillig, M. C. (2012). Microplastic in terrestrial ecosystems and the soil? Environ. Sci. Technol. 46, 6453–6454. doi: 10.1021/es302011r
Rillig, M. C. (2018). Microplastic disguising as soil carbon storage. Environ. Sci. Technol. 52, 6079–6080. doi: 10.1021/acs.est.8b02338
Sanchez-Vidal, A., Thompson, R. C., Canals, M., and de Haan, W. P. (2018). The imprint of microfibres in southern European deep seas. PLoS One 13:e0207033. doi: 10.1371/journal.pone.0207033
Secretariat of the Antarctic Treaty [ATS] (2009). Final Report of the Thirty-Second Antarctic Treaty Consultative Meeting. Management Plan for Antarctic Specially Protected Area No. 125. Fildes Peninsula, King George Island (25 de mayo). (Fossil Hill, Holz Stream (Madera Stream), Glacier Dome Bellingshausen (Collins Glacier), Halfthree Point, Suffield Point, Fossil Point, Gradzinski Cove and Skua Cove): revised Management Plan. 705. Available online at: https://documents.ats.aq/ATCM32/fr/ATCM32_fr002_e.pdf [Accessed August 09, 2021].
Secretariat of the Antarctic Treaty [ATS] (2019a). Resolution 5 (2019) - ATCM XLII - CEP XXII, Prague. Reducing Plastic Pollution in Antarctica and the Southern Ocean. Available online at: https://www.ats.aq/devAS/Meetings/Measure/705 [Accessed August 09, 2021]
Secretariat of the Antarctic Treaty [ATS] (2019b). XLII Antarctic Treaty Consultative Meeting 2019. Mitigating Microplastic Pollution in Antarctica. Prague: Czech Republic.
Sfriso, A. A., Tomio, Y., Rosso, B., Gambaro, A., Sfriso, A., Corami, F., et al. (2020). Microplastic accumulation in benthic invertebrates in Terra Nova Bay (Ross Sea, Antarctica). Environ. Int. 137:105587. doi: 10.1016/j.envint.2020.105587
Song, Y. K., Hong, S. H., Jang, M., Han, G. M., and Shim, W. J. (2015). Occurrence and distribution of microplastics in the sea surface microlayer in Jinhae Bay, South Korea. Arch. Environ. Contam. Toxicol. 69, 279–287. doi: 10.1007/s00244-015-0209-9
Song, Y. K., Hong, S. H., Jang, M., Kang, J. H., Kwon, O. Y., Han, G. M., et al. (2014). Large accumulation of micro-sized synthetic polymer particles in the sea surface microlayer. Environ. Sci. Technol. 48, 9014–9021. doi: 10.1021/es501757s
Talvitie, J., Heinonen, M., Pääkkönen, J. P., Vahtera, E., Mikola, A., Setälä, O., et al. (2015). Do wastewater treatment plants act as a potential point source of microplastics? Preliminary study in the coastal Gulf of Finland, Baltic Sea. Water Sci. Technol. 72, 1495–1504. doi: 10.2166/wst.2015.360
Thompson, R. C., Olsen, Y., Mitchell, R. P., Davis, A., Rowland, S. J., John, A. W., et al. (2004). Lost at sea: where is all the plastic? Science 304, 838. doi: 10.1126/science.1094559
Torres, D., and Gajardo, M. (1985). Información preliminar sobre desechos plásticos hallados en cabo Shirreff, isla Livingston, Shetland del Sur, Chile. Bol. Antártico Chil. 5, 12–13.
Torres, D., and Jorquera, D. (1992). Analysis of Marine Debris found at Cape Shirreff, Livingston Island, South Shetlands, Antarctica. SC-CAMLR/BG/7. Hobart, TAS: CCAMLR, 12.
Torres, D., and Jorquera, D. (1994). Marine Debris Collected at Cape Shirreff, Livinston Island, During the Antarctic Season 1993/94. CCMALR-XIII/BG/17. CCAMLR: Hobart, TAS, 10.
Velez, N., Zardi, G. I., Savio, R. L., McQuaid, C. D., Valbusa, U., Sabour, B., et al. (2019). A baseline assessment of beach macrolitter and microplastics along northeastern Atlantic shores. Mar. Pollut. Bull. 149:110649. doi: 10.1016/j.marpolbul.2019.110649
Vighi, M., Bayo, J., Fernández-Piñas, F., Gago, J., Gómez, M., Hernández-Borges, J., et al. (2021). Micro and nanoplastics in the environment: research priorities for the near future. Rev. Environ. Contam. Toxicol. 257, 163–218. doi: 10.1007/398_2021_69
Waldschläger, K., and Schüttrumpf, H. (2020). Infiltration behavior of microplastic particles with different densities, sizes, and shapes—from glass spheres to natural sediments. Environ. Sci. Technol. 54, 9366–9373. doi: 10.1021/acs.est.0c01722
Waller, C. L., Griffiths, H. J., Waluda, C. M., Thorpe, S. E., Loaiza, I., Moreno, B., et al. (2017). Microplastics in the Antarctic marine system: an emerging area of research. Sci. Total Environ. 598, 220–227. doi: 10.1016/j.scitotenv.2017.03.283
Watteau, F., Dignac, M. F., Bouchard, A., Revallier, A., and Houot, S. (2018). Microplastic detection in soil amended with municipal solid waste composts as revealed by transmission electronic microscopy and pyrolysis/GC/MS. Front. Sustain. Food Syst. 2:81. doi: 10.3389/fsufs.2018.00081
Woodall, L. C., Sanchez-Vidal, A., Canals, M., Paterson, G. L., Coppock, R., Sleight, V., et al. (2014). The deep sea is a major sink for microplastic debris. R. Soc. Open Sci. 1:140317. doi: 10.1098/rsos.140317
Wright, S. L., Ulke, J., Font, A., Chan, K. L. A., and Kelly, F. J. (2020). Atmospheric microplastic deposition in an urban environment and an evaluation of transport. Environ. Int. 136:105411. doi: 10.1016/j.envint.2019.105411
Keywords: fibers, fragments, PET, phenoxy resin, pollution, soil, intermareal sediment, Antarctica
Citation: Perfetti-Bolaño A, Araneda A, Muñoz K and Barra RO (2022) Occurrence and Distribution of Microplastics in Soils and Intertidal Sediments at Fildes Bay, Maritime Antarctica. Front. Mar. Sci. 8:774055. doi: 10.3389/fmars.2021.774055
Received: 10 September 2021; Accepted: 10 November 2021;
Published: 02 February 2022.
Edited by:
Stefania Ancora, University of Siena, ItalyReviewed by:
Agnieszka Da̧browska, University of Warsaw, PolandTadele Assefa Aragaw, Bahir Dar University, Ethiopia
Copyright © 2022 Perfetti-Bolaño, Araneda, Muñoz and Barra. This is an open-access article distributed under the terms of the Creative Commons Attribution License (CC BY). The use, distribution or reproduction in other forums is permitted, provided the original author(s) and the copyright owner(s) are credited and that the original publication in this journal is cited, in accordance with accepted academic practice. No use, distribution or reproduction is permitted which does not comply with these terms.
*Correspondence: Alessandra Perfetti-Bolaño, aleperfetti@udec.cl