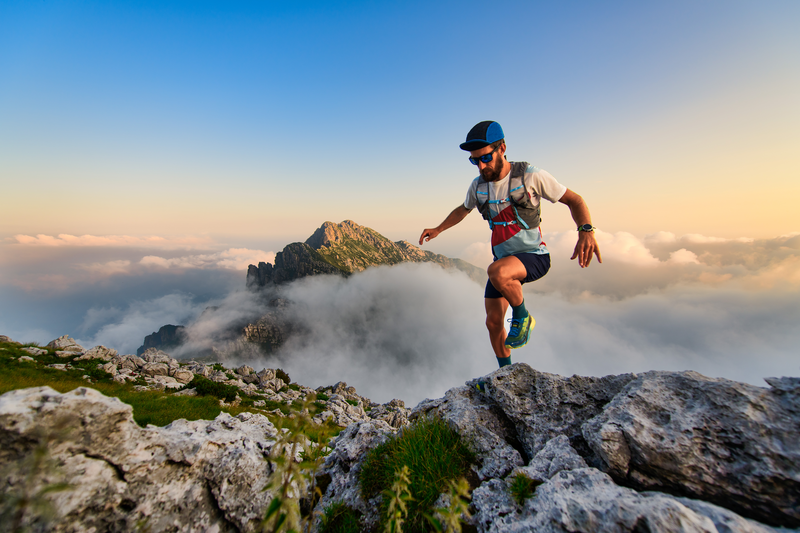
94% of researchers rate our articles as excellent or good
Learn more about the work of our research integrity team to safeguard the quality of each article we publish.
Find out more
REVIEW article
Front. Environ. Eng.
Sec. Environmental Catalysis
Volume 4 - 2025 | doi: 10.3389/fenve.2025.1532795
The final, formatted version of the article will be published soon.
You have multiple emails registered with Frontiers:
Please enter your email address:
If you already have an account, please login
You don't have a Frontiers account ? You can register here
Chemical oxidation remediation is a technique that involves the addition of chemical oxidants to contaminated soil to degrade total petroleum hydrocarbons (TPHs), with the goal of reducing soil organic matter content or eliminating organic contamination. This paper reviews the research progress of several major chemical oxidation remediation technologies, including hydrogen peroxide (H₂O₂)-based Fenton and Fenton-like systems, calcium peroxide (CaO₂)-based Fenton and Fenton-like systems, and persulfate-activated oxidation systems (e.g., Na₂S₂O₈). Among these, the persulfate-activated oxidation system has recently emerged as a research hotspot due to its potential in eliminating TPHs from soil. The efficiency of TPHs degradation depends significantly on the activation method employed and the oxidative capacity of the system.Consequently, future research should focus on two critical directions: (1) the development of highly efficient, cost-effective, and environmentally sustainable activation methods; and (2) the enhancement of oxidative performance in existing systems, such as Na₂S₂O₈/CaO₂ and Na₂S₂O₈/H₂O₂. In discussing the advancements in these major chemical oxidation remediation technologies, this paper specifically examines various persulfate activation methods and their corresponding treatment efficiencies. The aim is to provide insights and references for the development of efficient, cost-effective, and environmentally friendly persulfate-activated oxidation systems, thereby promoting the application of chemical oxidation remediation technologies in the treatment of petroleum hydrocarbon-contaminated soils.
Keywords: Total petroleum hydrocarbons, Chemical oxidative remediation technologies, Fentonbased reaction, Activated persulfate, Advanced Oxidation
Received: 22 Nov 2024; Accepted: 03 Mar 2025.
Copyright: © 2025 Liu and Dai. This is an open-access article distributed under the terms of the Creative Commons Attribution License (CC BY). The use, distribution or reproduction in other forums is permitted, provided the original author(s) or licensor are credited and that the original publication in this journal is cited, in accordance with accepted academic practice. No use, distribution or reproduction is permitted which does not comply with these terms.
* Correspondence:
Zhihao Liu, Chongqing University, Chongqing, China
Disclaimer: All claims expressed in this article are solely those of the authors and do not necessarily represent those of their affiliated organizations, or those of the publisher, the editors and the reviewers. Any product that may be evaluated in this article or claim that may be made by its manufacturer is not guaranteed or endorsed by the publisher.
Research integrity at Frontiers
Learn more about the work of our research integrity team to safeguard the quality of each article we publish.