- Basic Department of Power Engineering, Engineering academy, Peoples’ Friendship University of Russia, Moscow, Russia
This study examines the effects of methanol–diesel blends on the emissions of a diesel engine, concentrating on carbon monoxide (CO), carbon dioxide (CO2), nitrogen oxides (NOx), hydrocarbons (HCs), and particulate matter (PM). Using a single-cylinder four-stroke diesel engine at varying torque settings (2 N m–6 N m), significant reductions in CO, CO2, HC, and PM emissions were observed with increasing methanol content. CO emissions reduced by up to 81.8%, CO2 by up to 64.2%, HC by up to 80.4%, and PM by up to 23.5% with the MD11 blend. NOx emissions initially increased but decreased by up to 20% at higher torques with the same blend. These results highlight the environmental benefits of methanol–diesel blends and the need for effective NOx reduction strategies.
1 Introduction
Diesel fuel plays a key role in various areas like industries, transportation, and agriculture due to its widespread use and high efficiency. However, the growing consumption of diesel also increases the pollution level in the environment, which increases global warming due to the extensive emission of different gasses (Alalwan et al., 2023; Hassan et al., 2023). To reduce these environmental impacts, widespread efforts have been directed toward discovering alternative fuels such as biofuels. Yet, challenges related to the ease of use and scalability of biofuels persevere, prompting ongoing enhancements to predictable fossil fuels through the incorporation of essences. These additives aim to reduce emissions of particulate matter (PM), unburned hydrocarbons (HCs), nitrogen oxides (NOx), and carbon oxides (COx) (Alalwan and Alminshid, 2021), improving fuel quality, enhancing engine performance, and optimizing productivity (Joshi, 2020).
Among these essences, alcohol blends, particularly ethanol, have been comprehensively studied for their potential to improve diesel fuel properties. Research has shown that blending diesel with ethanol in varying relations, typically ranging from 3% to 13%, improves combustion efficiency due to the enriched oxygen content of ethanol (Johnson and Joshi, 2018; Vellandi et al., 2021). Despite the advantages of ethanol, its adoption in some situations remains limited due to factors such as its poor cetane number and blending compatibility with diesel.
In contrast, methanol presents an absorbing alternative as an additive due to its distinct advantages such as cost -effectiveness, high oxygen content, superior octane ratings, minimal soot formation, and reduced pollutant emissions related to conventional fossil fuels (Gülcan et al., 2022; Bhagat et al., 2023; Suresh and Porpatham, 2024). Methanol can be produced economically from various feedstocks such as biomass, natural gas, or coal, further improving its appeal as a sustainable fuel additive (Biswal et al., 2023; Zhou et al., 2023). Recently, the methanol blend with diesel has been studied by different engine configurations, which provide enough explanations proposing improvements in engine performance and decreases in fuel consumption and emissions (Xu and Cho, 2021).
Previous studies mostly focus on the implication of methanol–diesel blends with a focus of engine efficiency and fuel consumption. However, there is a broad gap in understanding the specific environmental effects of these methanol blends with diesel fuels, particularly in the environment of Baghdad, Iraq. To achieve this gap in various aspects, Iraq’s unique environmental and economic challenges are considered among its reliance on diesel fuel (Alalwan et al., 2021; Palani et al., 2022).
Building upon previous research on methanol–diesel blends, this study aims to develop and evaluate progressive catalytic converters and after-treatment systems to lessen the increased NOx emissions resulting from methanol–diesel blends while enhancing methanol blending ratios for enhanced engine performance and fuel efficiency (Ramachandra et al., 2020). The objectives include creating tailored NOx reduction technologies, performing comprehensive lifecycle and cost-benefit analyses, investigating necessary engine modifications for higher methanol blends, conducting long-term performance studies, comparing the efficacy of methanol–diesel blends with other alternative fuels, and providing practical insights for broader adoption through real-world application studies and policy analysis (Jamshaid et al., 2022).
2 Experimental procedure
2.1 Machine test and measurement unit
The experimental work was conducted using a Kohler K321 single-cylinder four-stroke diesel engine, as shown in Figure 1, at Mustansiriyah University, Baghdad, Iraq. This engine, with a displacement of 512 cc and a maximum torque of 6 N m (this maximum torque setting represents typical engine loads to ensure accurate and relevant emission data), was connected to a DYNOMite hydraulic dynamometer to apply the desired torque and measure the engine efficiency. Exhaust emissions were analyzed using an AIRREX HG-540 gas analyzer, and particulate matter was collected using a fiberglass filter. Further specifications of the test unit provided in Table 1.
2.2 Fuel preparation
Four types of fuels were used in this study. The first type was pure diesel, referred to as type D. The other three types were blends of methanol (ACS grade >99.8%) and diesel at volume ratios of 3%, 7%, and 11%, designated as MD3, MD7, and MD11, respectively. These specific ratios were chosen based on previous studies that have shown effective emission reductions and engine performance improvements at these levels. The diesel fuel was sourced from Kirkuk Refinery Station (Iraq), and the blends were prepared in the laboratory of the Mechanical Department. To prevent phase separation, 1% of 1-dodecanol (ACS grade >98%) was added to each blend. Methanol and 1-dodecanol were procured from Sigma-Aldrich. Further details on the fuel types and their properties are available in previous publications (Tutak et al., 2020; Hassan et al., 2023).
2.3 Experimental procedure
The experimental procedure was carefully designed to ensure accurate and reliable results. Initially, the engine fuel tank was emptied and refilled with the designated fuel type as shown in Figure 2. The engine was then operated at constant speed (3,600 rpm) and different torque settings (2, 4, and 6 N m). The torque settings (2, 4, and 6 N m) were chosen to reflect typical operating conditions of the engine. These settings allow for the analysis of engine emissions across a range of loads, ensuring comprehensive assessment of the effects of the methanol–diesel blends on emissions. After starting the engine, it was allowed to warm up for 10 min before the desired load was applied using the dynamometer control.
Exhaust emissions were measured to assess the environmental impact of each fuel type. The emissions included CO and CO2, HC, NOx, and PM. An AIRREX HG-540 emission analyzer was utilized for this purpose, as shown in Figure 3, connected to the engine exhaust via a gas line equipped with a gas meter to measure the flue gas volume. To capture the particulate matter, a fiberglass filter (Grade 934-AH, Whatman) was installed, which prevented PM from entering the analyzer (Zhang et al., 2022). The weight of the particulate matter was determined by weighing the filter before and after the experiment.
Each experimental setup was run twice to ensure the repeatability and accuracy of the results, and the average values were recorded. This methodology provides a robust framework for evaluating the emissions and performance of methanol–diesel blends compared to pure diesel.
3 Results and discussion
This section explains the results obtained during the experiment under different load conditions, and also, the legends explain the following data:
• D for diesel
• MD3 shows 3% methanol blend
• MD7 shows 7% methanol blend
• MD11 shows 11% methanol blend
3.1 Carbon monoxide emission
The study measured CO emissions for pure diesel (D) and methanol–diesel blends (MD3, MD7, and MD11) at different torque settings (2, 4, and 6 N m). The results indicate a clear reduction in CO emissions as the methanol content in the blend increases, as shown in Figure 4.
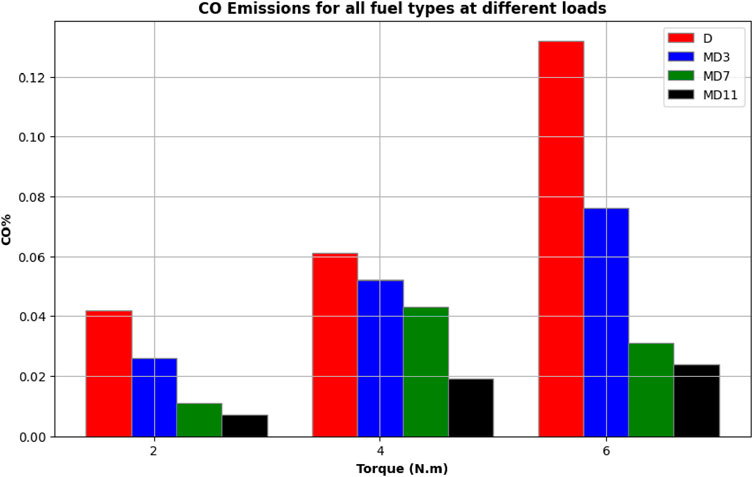
Figure 4. Variation in CO emissions with different methanol–diesel blends at various torque settings.
For pure diesel, CO emissions increased significantly with increasing torque, showing values of 0.042%, 0.061%, and 0.132% at 2, 4, and 6 N m, respectively. In contrast, the MD3 blend showed a moderate reduction in CO emissions, with values of 0.026%, 0.052%, and 0.076%. This trend continued with the MD7 blend, where emissions were further reduced to 0.011%, 0.043%, and 0.031%, and the lowest CO emissions were recorded for the MD11 blend, with values of 0.007%, 0.019%, and 0.024%. This reduction can be attributed to the higher oxygen content in methanol, which promotes more complete combustion than pure diesel. These findings align with those of previous research, suggesting that alcohol blends improve combustion efficiency and reduce CO emissions by 81.8%.
3.2 CO2 emission
The CO2 emissions for different fuel blends were analyzed under varying torque settings, as shown in Figure 5. Pure diesel showed the highest CO2 emissions, with values of 2.5%, 3.8%, and 8.1% at 2, 4, and 6 N m, respectively.
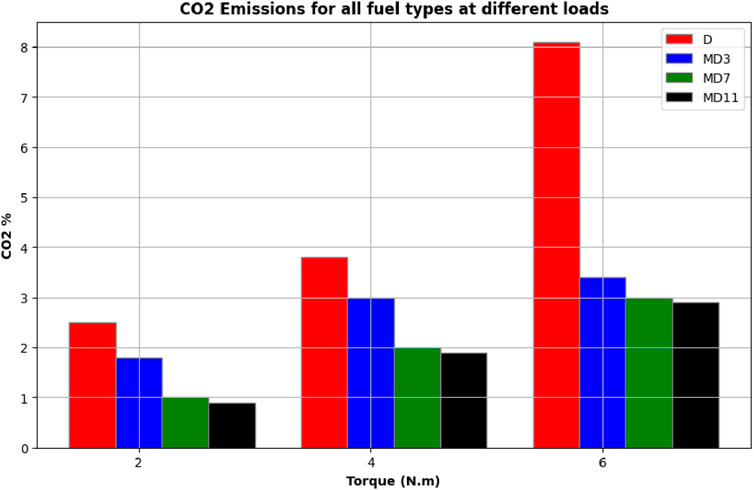
Figure 5. Variation in CO2 emissions with different methanol–diesel blends at various torque settings.
As when methanol was added to the diesel, there was a notable reduction in CO2 emissions. The MD3 blend showed lower emissions, with values of 1.8%, 3.0%, and 3.4%. This reduction continued with the MD7 blend, which recorded values of 1%, 2%, and 3%, and the lowest CO2 emissions were observed for the MD11 blend, with values of 0.9%, 1.9%, and 2.9%. The decrease in CO2 emissions with increasing methanol content is due to the lower carbon-to-hydrogen ratio of methanol, which results in lower carbon emissions per unit of fuel burned, and the emission of CO2 was decreased by 64.2%.
3.3 NOx emissions
The NOx emissions were measured for pure diesel and methanol–diesel blends at different torque settings. For pure diesel, NOx emissions were relatively stable, showing values of 95, 93.4, and 93 ppm at 2, 4, and 6 N m, respectively, as shown in Figure 6.
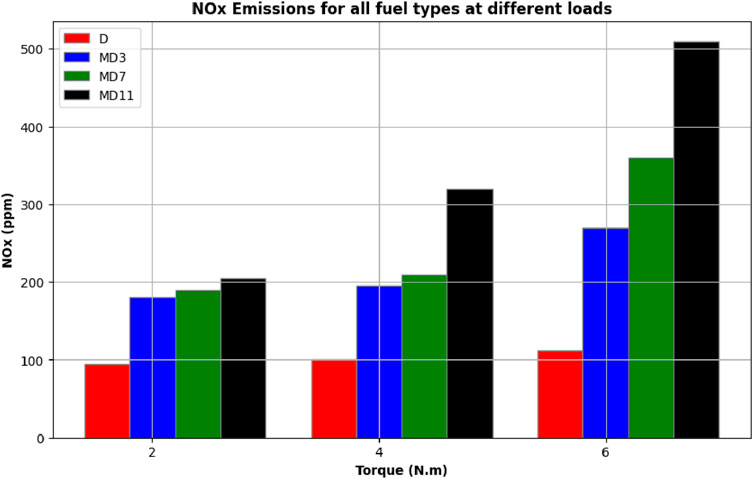
Figure 6. Variation in NOx emissions with different methanol–diesel blends at various torque settings.
With the addition of methanol, NOx emissions initially increased but then showed a slight decrease at the highest torque settings. The MD3 blend showed emissions of 180, 179.1, and 177.3 ppm, while the MD7 blend recorded values of 190, 188.3, and 167.9 ppm. The highest NOx emissions were observed with the MD11 blend, with values of 205, 200.2, and 199.2 ppm. The higher NOx emissions at lower torques can be attributed to the increased combustion temperatures associated with the higher oxygen content of methanol, which facilitates NOx formation. However, at higher torques, the slight reduction in NOx emissions was observed due to more stable combustion and better mixing of air and fuel.
3.4 HC emissions
HC emissions decreased with the addition of methanol to diesel. Pure diesel emitted 14, 19, and 25% HC units at 2, 4, and 6 N m, respectively, as shown in Figure 7.
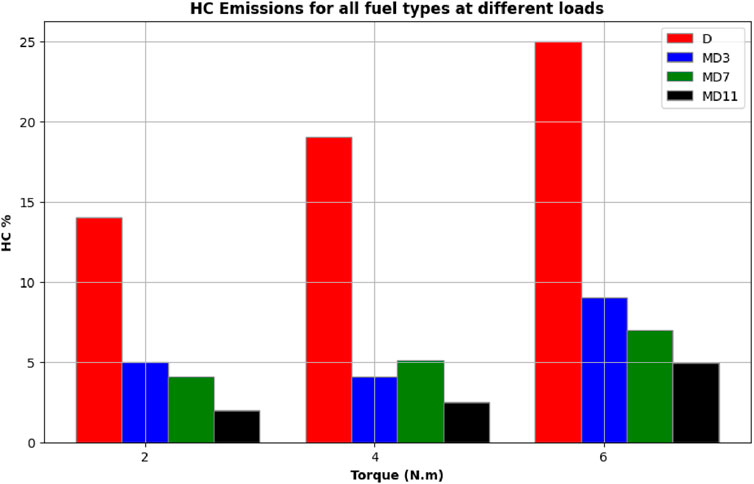
Figure 7. Variation in hydrocarbon (HC) emissions with different methanol–diesel blends at various torque settings.
The MD3 blend showed a reduction in HC emissions, with values of 5, 4.1, and 9%. This trend of reduction continued with the MD7 blend, which recorded 4.1, 5.1, and 7%, and the lowest HC emissions were noted for the MD11 blend, with values of 2, 2.5, and 4.9%. The reduction in HC emissions is likely due to the more complete combustion facilitated by the higher oxygen content in methanol. These findings support those of earlier research, indicating that alcohol blends can effectively reduce HC emissions.
3.5 PM emission
PM emissions also showed a decreasing trend with increasing methanol content in the blends. For pure diesel, PM emissions were 1.3, 1.4, and 1.7 g/hr at 2, 4, and 6 N m, respectively, as shown in Figure 8. The MD3 blend showed a slight reduction in PM emissions, with values of 1.2, 1.27, and 1.46 g/hr. This decrease continued with the MD7 blend, which recorded values of 1, 1.32, and 1.4 g/hr. The lowest particulate matter emissions were observed with the MD11 blend, with values of 0.7, 1.1, and 1.3 g/hr, respectively.
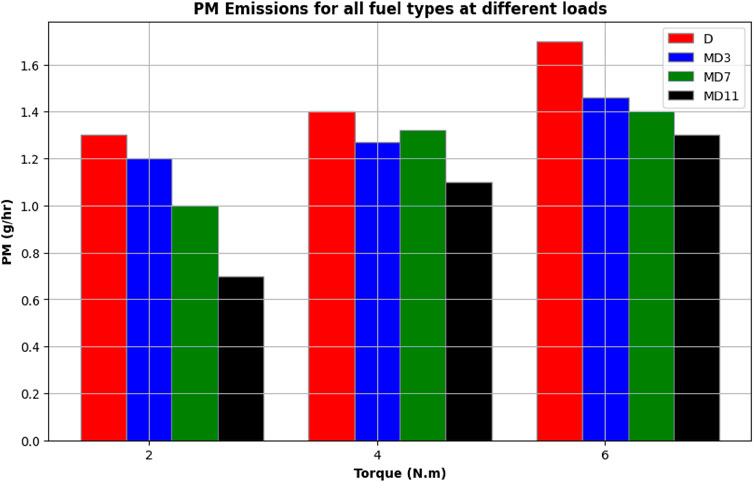
Figure 8. Variation in particulate matter (PM) emissions with different methanol–diesel blends at various torque settings.
The reduction in PM emissions can be attributed to the ability of methanol to reduce soot formation as the methanol blend burns more cleanly than diesel due to its simpler molecular structure. This result aligns with that of preceding studies, demonstrating lower PM emissions from methanol–diesel blends.
The reduction in CO and HC emissions with higher methanol content can be explained by the increased oxygen content in methanol, which promotes more complete combustion. Similarly, the initial increase in NOx emissions may be attributed to the higher combustion temperatures associated with methanol blends. However, at higher torque settings, the NOx emissions decrease slightly, possibly due to the cooling effect of the higher latent heat of vaporization of methanol.
4 Conclusion
The study demonstrates that methanol–diesel blends significantly impact the emissions of COx, HC, and PM while also affecting NOx emissions. These findings indicate the potential environmental benefits of methanol–diesel blends, although the increase in NOx emissions suggests the need for further NOx reduction technologies.
• CO emissions reduced by up to 81.8% with the MD11 blend at a torque of 6 N m.
• CO2 emissions reduced by up to 64.2% with the MD11 blend at a torque of 6 N m.
• NOx emissions initially increased, but after achieving a high-torque setting, the emission reduced by 20% with the MD11 blend at a torque of 6 N m.
• HC emissions reduced by up to 80.4% with the MD11 blend at a torque of 6 N m.
• PM emissions reduced by up to 23.5% with the MD11 blend at a torque of 6 N m.
5 Recommendation
The addition of blends reduces the emission of gasses and also NOx, but there is still a knowledge gap that can be reduced more by studying different blends with a combination of diesel fuels.
Data availability statement
The raw data supporting the conclusion of this article will be made available by the authors, without undue reservation.
Author contributions
MK: conceptualization, formal analysis, funding acquisition, investigation, methodology, project administration, software, and writing–original draft. PO: data curation, resources, supervision, validation, and writing–review and editing.
Funding
The author(s) declare that no financial support was received for the research, authorship, and/or publication of this article.
Conflict of interest
The authors declare that the research was conducted in the absence of any commercial or financial relationships that could be construed as a potential conflict of interest.
Publisher’s note
All claims expressed in this article are solely those of the authors and do not necessarily represent those of their affiliated organizations, or those of the publisher, the editors, and the reviewers. Any product that may be evaluated in this article, or claim that may be made by its manufacturer, is not guaranteed or endorsed by the publisher.
References
Alalwan, H. A., and Alminshid, A. H. (2021). CO2 capturing methods: chemical looping combustion (CLC) as a promising technique. Sci. Total Environ. 788, 147850. doi:10.1016/j.scitotenv.2021.147850
Alalwan, H. A., Alminshid, A. H., Mohammed, M. M., and Mohammed, M. F. (2023). Methane activation on metal oxide nanoparticles: spectroscopic identification of reaction mechanism. Part. Sci. Technol. 41 (5), 653–660. doi:10.1080/02726351.2022.2129533
Alalwan, H. A., Augustine, L. J., Hudson, B. G., Abeysinghe, J. P., Gillan, E. G., Mason, S. E., et al. (2021). Linking solid-state reduction mechanisms to size-dependent reactivity of metal oxide oxygen carriers for chemical looping combustion. ACS Appl. energy Mater. 4 (2), 1163–1172. doi:10.1021/acsaem.0c02029
Bhagat, R. N., Sahu, K. B., Ghadai, S. K., and Kumar, C. B. (2023). A review of performance and emissions of diesel engine operating on dual fuel mode with hydrogen as gaseous fuel. Int. J. Hydrogen Energy 48, 27394–27407. doi:10.1016/j.ijhydene.2023.03.251
Biswal, S., Das, S. R., Saha, N., and Mishra, P. C. (2023). Environmental sustainability assessment of gasoline and methanol blended smart fuel for reduced emission formation. Environ. Dev. Sustain., 1–32. doi:10.1007/s10668-023-03752-6
Gülcan, H. E., Gültekin, N., and Ciniviz, M. (2022). The effect of methanol-Dodecanol addition on performance and smoke emission in a CI engine with diesel fuel. Int. J. Automot. Sci. Technol. 6 (2), 207–213. doi:10.30939/ijastech.1074513
Hassan, Q. H., Alalwan, H., Mohammed, M., and Mohammed, M. F. (2023). Identifying the impact of methanol-diesel fuel on the environment using a four-stroke CI engine. J. Appl. Eng. Sci. 21 (1), 188–193. doi:10.5937/jaes0-39809
Jamshaid, M., Masjuki, H. H., Kalam, M. A., Zulkifli, N. W. M., Arslan, A., and Qureshi, A. A. (2022). Experimental investigation of performance, emissions and tribological characteristics of B20 blend from cottonseed and palm oil biodiesels. Energy 239, 121894. doi:10.1016/j.energy.2021.121894
Johnson, T., and Joshi, A. (2018). Review of vehicle engine efficiency and emissions. SAE Int. J. Engines 11 (6), 1307–1330. doi:10.4271/2018-01-0329
Joshi, A. (2020). Review of vehicle engine efficiency and emissions. SAE Int. J. Adv. Curr. Pract. Mobil. 2 (2020-01-0352), 2479–2507. doi:10.4271/2020-01-0352
Palani, Y., Devarajan, C., Manickam, D., and Thanikodi, S. (2022). Performance and emission characteristics of biodiesel-blend in diesel engine: a review. Environ. Eng. Res. 27 (1), 200338–200340. doi:10.4491/eer.2020.338
Ramachandra, R., Pandurangadu, V., and Raju, K. A. K. (2020). Performance and emission characteristics of four stroke diesel engine with neem and cotton seed biodiesel blended with diesel. Int. J. Sci. Res. (IJSR), 282–285. doi:10.21275/v5i4.nov162446
Suresh, D., and Porpatham, E. (2024). Influence of high compression ratio on the performance of ethanol-gasoline fuelled lean burn spark ignition engine at part throttle condition. Case Stud. Therm. Eng. 53, 103832. doi:10.1016/j.csite.2023.103832
Tutak, W., Jamrozik, A., and Grab-Rogaliński, K. (2020). Effect of natural gas enrichment with hydrogen on combustion process and emission characteristic of a dual fuel diesel engine. Int. J. hydrogen energy 45 (15), 9088–9097. doi:10.1016/j.ijhydene.2020.01.080
Vellandi, V., Krishnasamy, A., and Ramesh, A. (2021). Fuel injection strategies for improving performance and reducing emissions of a low compression ratio diesel engine. SAE Technical Paper.
Xu, C., and Cho, H. (2021). Effect of methanol/water mixed fuel compound injection on engine combustion and emissions. Energies 14 (15), 4491. doi:10.3390/en14154491
Zhang, Z., Lv, J., Li, W., Long, J., Wang, S., Tan, D., et al. (2022). Performance and emission evaluation of a marine diesel engine fueled with natural gas ignited by biodiesel-diesel blended fuel. Energy 256, 124662. doi:10.1016/j.energy.2022.124662
Zhou, F., Yu, J., Wu, C., Fu, J., Liu, J., and Duan, X. (2023). The application prospect and challenge of the alternative methanol fuel in the internal combustion engine. Sci. Total Environ. 913, 169708. doi:10.1016/j.scitotenv.2023.169708
Nomenclature
Keywords: methanol–diesel blend, gas emission, internal combustion, alternative fuels, engine performance
Citation: Kadhim MQ and Oshchepkov PP (2024) Experimental investigation of emissions from a single-cylinder diesel engine using methanol–diesel blends. Front. Energy Res. 12:1449652. doi: 10.3389/fenrg.2024.1449652
Received: 15 June 2024; Accepted: 09 August 2024;
Published: 23 September 2024.
Edited by:
Xiongbo Duan, Central South University, ChinaReviewed by:
Feng Zhou, Central South University Forestry and Technology, ChinaRenhua Feng, Chongqing University of Technology, China
Copyright © 2024 Kadhim and Oshchepkov. This is an open-access article distributed under the terms of the Creative Commons Attribution License (CC BY). The use, distribution or reproduction in other forums is permitted, provided the original author(s) and the copyright owner(s) are credited and that the original publication in this journal is cited, in accordance with accepted academic practice. No use, distribution or reproduction is permitted which does not comply with these terms.
*Correspondence: Petr Platonovich Oshchepkov, oshchepkov-pp@rudn.ru
†ORCID: Mohammed Qays Kadhim, orcid.org/0000-0002-5251-9264; Petr Platonovich Oshchepkov, orcid.org/0000-0001-5504-106X