- Oil and Gas Production Technology Research Institute, Great Wall Drilling Engineering Technology Research Institute, Panjin, Liaoning, China
Tight sandstone reservoir has the characteristics of low porosity, low permeability and strong reservoir heterogeneity, which leads to a rapid decline in single well production after hydraulic fracturing. Adding nano emulsion to fracturing fluid can not only realize the dual function of fracturing and imbibition oil displacement, but also effectively prolong the stable production time of oil wells. In order to improve the understanding of the interaction between nano emulsion and reservoir, this paper based on the basic physical property test and analysis of nano emulsion, macroscopic and microscopic spontaneous imbibition oil displacement experiments, the mechanism of imbibition displacement of nano emulsion in tight sandstone reservoirs and the influence of related factors is clarified. The results show that: ①The particle size of the three types of nano emulsions ranges from 11 to 16 nm, and they can enter the nanopore throats deep in the reservoir, and can achieve good injectivity in tight reservoirs. The anionic AES nano emulsion is superior to cationic ADT and nonionic AEO-9 nano emulsion in terms of injectability, oil-water interfacial tension and wetting modification effect in tight sandstone cores, and the optimal concentration is 0.30 wt%. ②Spontaneous imbibition oil displacement experiments show that the nano emulsion concentration is not the higher the better. Since the nano emulsion adsorption on the solid surface reaches a dynamic equilibrium, the excessive nano emulsion cannot further change the wettability of the solid surface, and the lower oil-water interfacial tension has the opposite effect on the imbibition displacement. ③The migration and gathering of emulsified oil droplets in solution mainly included five processes: Saturated oil core/Nano-surfactant fluid emulsion reaction, Aqueous/Emulsified oil droplets, Emulsified oil droplet gathering phase, Demulsifying and emulsifying oil droplets, Oil phase after demulsification. The generation of remaining oil in porous media is due to the non-uniform wetting modification in different regions. This study further improves the understanding of the mechanism of spontaneous imbibition of nano emulsion in tight sandstone reservoirs.
1 Introduction
Due to the characteristics of low porosity and low permeability of unconventional tight sandstone reservoirs, it is necessary to drill horizontal wells and hydraulic fracturing to realize the commercial exploitation of tight sandstone reservoirs. A complex fracture network system with high conductivity is formed by hydraulic fracturing in tight reservoir can changes the seepage mode of crude oil in tight reservoir, reduces the seepage resistance of crude oil, and improves oil recovery efficiency (Wang et al., 2020a; Wang et al., 2020b). However, the reservoir matrix is dense, so it is impossible to obtain enough effective energy supplement during the pressure drop around the fracture system, resulting in a rapid decline in oil well production after fracturing (Surguchev et al., 2005; Shoaib and Hoffman, 2009). Domestic and foreign scholars have found that the primary recovery rate of tight oil reservoirs obtained by hydraulic fracturing is generally low, and the oil well production decreases rapidly after 1 year (Baihly et al., 2010; Zhang et al., 2015; Rodriguez and Maldonado, 2019). Most reservoirs need to supplement formation energy by water injection or gas injection, but tight reservoirs often encounter problems such as injection difficulties or insufficient surrounding gas sources (Chen and Reynolds, 2015; Han and Gu, 2015). Therefore, how to effectively replenish formation energy is of great significance for improving recovery efficiency of tight oil reservoirs.
Studies have found that adding surfactants to the fracturing fluid during hydraulic fracturing and performing a period of soaking operation after the fracturing operation can increase reservoir energy and oil well production through the imbibition replacement of the fracturing fluid. The main mechanism of surfactant imbibition displacement includes two aspects, namely, reducing the oil-water interfacial tension and wetting modification. Liu et al. (Liu et al., 2019; Liu et al., 2020; Liu and Sheng, 2020) found that surfactants with lower interfacial tension can significantly improve the oil-water emulsification effect and the recovery of crude oil in rock micro-pore throats. The surfactant changes the wettability of the oil-wet rock surface and peels off the oil film by forming a water-adsorbing film on the rock surface, and the process of demulsification and fusion of the crude oil produced by spontaneous imbibition in solution is observed. Finally, it is concluded that the anionic surfactant has the best effect on the imbibition and oil displacement of shale. With the help of nuclear magnetic resonance (NMR) and microfluidic chip devices, Liang et al. (Liang et al., 2020; Liang et al., 2021a; Liang et al., 2021b) revealed the recovery priority of large and small pore crude oil in the process of spontaneous imbibition displacement of nano emulsion, as well as the microscopic remaining oil distribution in the process of spontaneous imbibition displacement of different chemical agent systems. At the same time, combined with laboratory experiments, the field soaking operation cycle is optimized. Sivabalan et al. developed and evaluated the spontaneous imbibition oil displacement effect of surfactants with salinity resistance in carbonate reservoirs, and found that the wetting modification effect of long-chain surfactants is better (Sivabalan and Mahmoud, 2021; Sivabalan and Mazen, 2021). Morteza et al. studied the microscopic mechanism of surfactant spontaneous imbibition oil displacement from nanometer scale by means of micro-nano CT and other technical means, and concluded that emulsification and wetting modification are the main mechanism of improving oil recovery in spontaneous imbibition process, while the influence of gravity is relatively small (Morteza et al., 2017; Anupong et al., 2022). Hatiboglu et al. studied the influence of core size, solution salinity, temperature, crude oil viscosity, surfactant concentration and other related factors on spontaneous imbibition oil displacement efficiency through experiments, and found that crude oil viscosity, interfacial tension between surfactant solution and crude oil and wetting modification effect on rock have great influence on spontaneous imbibition oil displacement effect (Hatiboglu and Babadagli, 2004; Li, 2006; Peng et al., 2010; Li et al., 2011; Zhu et al., 2022; Wang et al., 2023). The above studies analyze the emulsification effect between the surfactant and the crude oil from the perspective of the interfacial tension between the surfactant and the crude oil, or determine whether the surfactant changes the direction and size of the capillary force by testing the wetting modification effect. Finally, the two main parameters are linked to the spontaneous imbibition oil displacement efficiency. However, the roles of surfactants at different stages in the process of spontaneous imbibition displacement efficiency have not been systematically divided. At the same time, there is no explanation of the emulsification mechanism of surfactant with crude oil in the core pore throat, the wetting modification process of reservoir rock, and the demulsification process of produced crude oil in solution.
In order to fully reveal the mechanism of surfactants in different stages of time and contact medium change during spontaneous imbibition and oil displacement in tight sandstone reservoirs, three kinds of nano emulsions, anionic, cationic and anionic-nonionic mixed nano emulsions, are synthesized by microemulsion method. On the basis of testing the basic physical properties of nano emulsions, the types of nano emulsions suitable for tight sandstone reservoirs are selected. Combined with experiments, the effects of nano emulsion concentration, core type, crude oil type and other factors on spontaneous imbibition oil displacement efficiency are studied, and the mechanism of nano emulsion under different conditions in the imbibition process is analyzed. Through microscopic experiments, the remaining oil distribution under spontaneous imbibition displacement is clarified. Furthermore, combined with the morphology of the core surface and the crude oil in the solution during the spontaneous imbibition oil displacement process, the emulsification and wetting modification of the nano emulsion and the demulsification mechanism of the crude oil are analyzed when the contact medium changed. The innovations of this paper include: 1) the interaction mechanism between nano-emulsion and reservoir rock is clarified; 2) Based on the polyfactor method, the influence of different influencing factors on the imbibition displacement effect of nano-emulsion was clarified; 3) Microfluidic chip technology was used to clarify the microscopic mechanism of imbibition displacement of nano-emulsion. This study further improves the understanding of the mechanism of spontaneous imbibition of nano emulsion in tight sandstone reservoirs.
2 Methodology
2.1 Fluid properties and rock
Nano emulsions commonly used in spontaneous imbibition to enhance oil recovery in tight reservoirs include anionic, cationic and nonionic types. The anionic nano emulsion is coded as AES, and the main component is sodium alcohol ether sulphate. Cationic nano emulsion codenamed ADT, the main component is dodecyl dimethyl tertiary amine; the non-ionic nano emulsion is codenamed AEO-9, and the main component is alcohol ethoxylate. The pH value of the three types of nano emulsions is equal to 7.0, and the purity is more than 99%, produced by Sinopharm Chemical Reagent Co., Ltd.
The natural core types used in the experiment include shale, carbonate rock and tight sandstone. The carbonate rock and shale are taken from the outcrop core of the southwest oil and gas field in Sichuan, China, which are used to study the influence of lithology change on the effect of nano emulsion imbibition displacement. Tight sandstone cores are taken from Shengli Oilfield, China, with a core depth of about 2000 m. The XRD analysis results of the mineral composition of tight sandstone are shown in Table 1. The crude oil includes two types: light crude oil (the content of heavy hydrocarbon components above C20+ is only 5.22%) and heavy oil (the content of heavy hydrocarbon components above C20+ is as high as 42.60%).
2.2 Experimental device and design
In order to reveal the mechanism of surfactant in different stages of time and contact medium change in the process of spontaneous imbibition and oil displacement in tight sandstone reservoirs, the main tests in this paper include: 1) Through the test of nano emulsion particle size, oil-water interfacial tension and wetting modification effect, the injectability of nano emulsion in tight sandstone core is clarified, and the interfacial tension is reduced to promote emulsification effect and change capillary force. 2) The effects of nano emulsion concentration, core type, crude oil type and other factors on the spontaneous imbibition oil displacement efficiency are studied by spontaneous imbibition oil displacement experiments, and the mechanism of nano emulsion in the imbibition process is analyzed. 3) Through microscopic experiments, the remaining oil distribution under the action of spontaneous imbibition is clarified. The name, function and origin of the equipment used in the experiment are shown in Table 2.
2.3 Experimental procedures
2.3.1 Nano emulsion particle size test
The specific dilution method is to slowly add an appropriate amount of nano emulsion (0.3 wt%) to distilled water and fully mix it, and then use a laser nanoparticle size analyzer to test the nanoparticle size distribution of the nano emulsion aqueous solution.
2.3.2 Oil-water interfacial tension test
The test device is JYW-200 A micro-controlled automatic surface and interface tensiometer. The experimental method is as follows: First, the platinum ring is immersed in different concentrations of nano emulsion aqueous solution, and then slowly pulled up. When the platinum ring leaves the liquid surface, a thin film is formed between the platinum ring and the measured liquid due to the tension. The film gradually elongates and the tension gradually increases until the film breaks. The maximum value is the interfacial tension between the two fluids. In order to reduce the error, each experiment is repeated three times and the average value is taken.
2.3.3 Oil-water-rock three-phase contact angle test
Firstly, the natural sandstone core after oil washing is cut into slices by core cutting machine. The diameter of the core slice is about 2.50 cm and the thickness is about 0.3 cm. Then, the surface of the core thin section is polished and smoothed and put into a 60°C oven for drying for 24 h. The thin sections are aged in kerosene containing 15,000 mg/L oleic acid for 24 h to make the core thin sections become oil-wet. When measuring the contact angle, the oil-wet core slice is supported by a stainless steel bracket in a glass container. After the test core slice is immersed in the nano emulsion aqueous solution, the kerosene droplets are slowly injected by using a computer-controlled needle tube to observe the change of contact angle. The experimental device is shown in Figure 1.
2.3.4 Spontaneous imbibition experiment
The spontaneous imbibition oil displacement experiment comprehensively reflects the interaction between nano emulsion wetting modification, interfacial tension reduction and reservoir pore throat. Since spontaneous imbibition is affected by many factors such as nano emulsion type, concentration, rock type and crude oil type, these factors need to be considered comprehensively during the experiment. The imbibition experiment is a volume method, and the Amott bottle is used to study the imbibition and oil displacement effect of nano emulsion in real core. Before the experiment, the basic physical parameters such as porosity and permeability are measured by gas permeability measuring instrument and gas porosity measuring instrument, and the core with similar physical parameters is selected for imbibition experiment. The specific steps include (the experimental device is shown in Figures 2, 3).
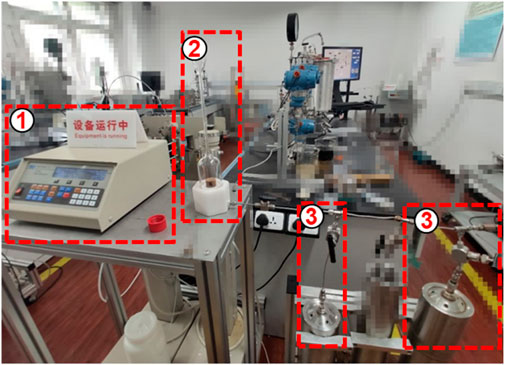
FIGURE 2. Experimental device of saturated fluid in Amot spontaneous imbibition bottle with core. Note: ①- ISCO constant speed constant pressure pump; ②- Amott spontaneous imbibition bottle; ③- Intermediate container with nano emulsion solution.
1) The fully dry core is weighed and put into the vacuum saturation device to record the saturated crude oil volume. 2) The core is put into the imbibition bottle and the solution is injected into the imbibition bottle through the constant pressure and constant speed pump. 3) Due to the density difference between crude oil and water, the crude oil rises, and the amount of imbibition displacement oil in different time periods can be clearly recorded. 4) The curve of imbibition displacement amount and time is drawn, and the related factors affecting imbibition displacement of nano emulsion are explored by changing the concentration and type of nano emulsion, rock and crude oil type.
2.3.5 Microscopic imbibition oil displacement experiment
The main device used in this experiment is a microfluidic chip experimental device (as shown in Figure 4). The main steps include:
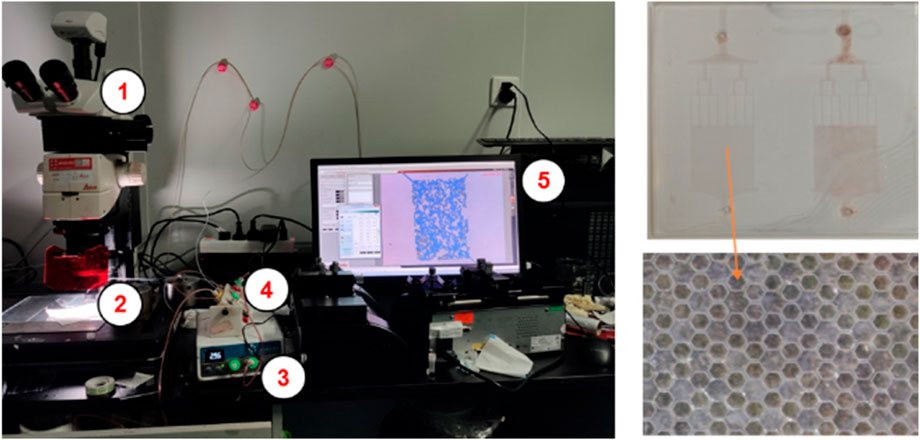
FIGURE 4. Microfluidic chip experimental device and chip model. Note: ①- Leica M165FC optical microscope; ②- objective table and microfluidic chip; ③- neMESYS gas constant-speed constant-pressure micro-flow pump; ④- gas/liquid intermediate buffer tank; ⑤- equipment control and data acquisition system.
1) First, the model is cleaned with a cleanser and completely dried. 2) Then it is immersed in a mixture of sulfuric acid and hydrogen peroxide (volume ratio of 7:3) for 2 h to remove organic pollutants on its surface. 3) Then washed with deionized water and completely dried again, and then immersed in methanol solution of 20,000 mg/L trimethylchlorosilane for 24 h, and finally washed with methane and dried. 4) The microfluidic pump injects Sudan red-stained crude oil into the chip at a flow rate of 0.005 mL/min through the pipeline. 5) After the chip is completely saturated with crude oil, the nano emulsion is injected into the chip at a flow rate of 0.005 mL/min, and the state of the crude oil is observed by a microscope. The low flow rate is used to reduce the viscous force caused by the water flow rate. The effect of oil displacement mainly reflects the effect of imbibition displacement. 6) After each experiment, the microchannel is repeatedly rinsed with 5% hydrochloric acid solution, 2% sodium hydroxide solution, ethanol and deionized water to remove the residual oil in the microfluidic chip, and the microscopic model is dried at 120°C.
3 Results and discussions
3.1 Basic physical properties test results of nano emulsion
The basic physical property test of nano emulsion includes nano emulsion particle size test to evaluate the injectability of chemical agents in tight reservoirs. The interfacial tension test of the nano emulsion is to determine whether the injection pressure of the liquid can be reduced. The wetting modification effect test of nano emulsion is to determine whether the chemical agent can be effectively adsorbed on the solid surface after entering the porous medium, change the free energy of the solid surface, cooperate with the interfacial tension, and change the capillary force between oil and water.
As shown in Figure 5, the particle size test results of the three types of nano emulsions at a concentration of 0.30 wt%. The results shows that the particle size distribution of AES, ADT, and AEO-9 nano emulsion is 15.69 nm, 11.7 nm and 13.54 nm, respectively. The three types of nano emulsions have particle sizes ranging from 11–16 nm and can enter the nanopore throats deep in the reservoir to achieve good injectivity in tight reservoirs. Figure 6 shows the relationship curves of interfacial tension of three kinds of nano emulsions with concentration. When the concentration of nano emulsion is above 0.10 wt%, the interfacial tension between the three nano emulsion aqueous solution and crude oil is below 2 mN/m. The results show that the three nano emulsions can form lower interfacial tension in crude oil, and then achieve the effect of low injection pressure at the initial stage of injection. When the concentration is increased to 0.20 wt%, the interfacial tension between oil and water could be as low as 0.98 mN/m.
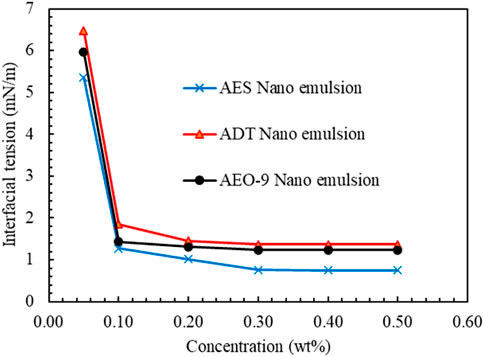
FIGURE 6. The relationship curve of interfacial tension of three kinds of nano emulsions with concentration.
Figure 7 shows the three-phase contact angle of ‘oil-water-rock’ on the core surface treated by three kinds of nano emulsions with different concentrations. When the concentration of nano emulsion is 0.3wt%, the wetting modification effect of three nano emulsion on reservoir rock reaches the inflection point. When the concentration of different types of nano emulsion is the same, the wetting modification effect of AES nano emulsion on tight sandstone reservoir rock is the best (after soaking in 0.30 wt% AES nano emulsion for 24 h, the three-phase contact angle of ‘oil-water-gas’ increases from 51.25° to 148.34°). According to the analysis of the basic physical properties of nano emulsions tested in Figures 5–7, it can be seen that the nano emulsion with a concentration of 0.30 wt% AES exhibits excellent performance in terms of injectability, oil-water interfacial tension and wetting modification effect in tight sandstone cores.
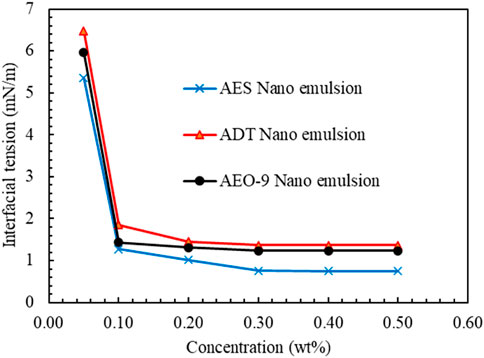
FIGURE 7. Three-phase contact angles of ‘oil-water-rock’ on core surface treated by three types of nano emulsions with different concentrations.
Based on the analysis of the basic physical property test results of nano emulsion and the effect of capillary force in porous media, it can be seen that nano emulsion with nano particle size (11–16 nm) can realize the deep migration effect in dense pore throat, as shown in Figure 8A. Before the nano emulsion interacts with the rock surface, the lower oil-water interfacial tension can reduce the capillary resistance and the injection pressure of the chemical agent. The nano emulsion adsorbed on the rock surface after wetting modification can change the direction of capillary force, as shown in Figure 8B.
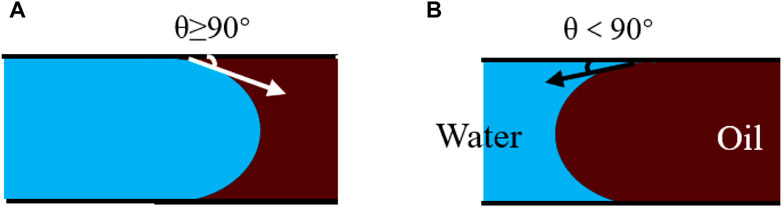
FIGURE 8. The change process of ‘oil-water-rock’ three-phase contact angle in capillary before and after wetting modification.(A) Oil-water-rock contact angle in oil-wet capillary; (B) Oil-water-rock contact angle in water-wet capillary.
3.2 Effect of nano emulsion type and concentration on imbibition displacement efficiency
In order to further clarify the effect of nano emulsion on the imbibition oil displacement of reservoir rock, Amott imbibition bottle is used to carry out imbibition oil displacement experiment. The main changed parameters include nano emulsion type and concentration, rock type and crude oil type.
Three types of nano emulsions with a concentration of 0.30 wt% are prepared, and the imbibition oil displacement efficiency of the three kinds of nano emulsions is tested at the same concentration. The core permeability and porosity are 0.35 mD and 7.53%, respectively. Figure 9 shows that in the initial stage of imbibition (0–50 h), the imbibition oil displacement efficiency of anionic AES and AEO-9 nano emulsions is not much different, and the imbibition oil displacement efficiency of cationic ADT nano emulsion is the lowest. After the imbibition displacement efficiency reaches dynamic equilibrium, the final imbibition displacement efficiencies of AES, AEO-9 and ADT nano emulsions are 32.51, 25.63% and 8.11%, respectively. It shows that among the three nano emulsions with the same concentration, the anionic AES nano emulsion has the best imbibition oil displacement effect. Figure 10 shows the imbibition displacement efficiency of AES nano emulsions with different concentrations. When the concentration of AES nano emulsion is 0.30 wt%, the corresponding imbibition displacement efficiency is the highest (up to 34.26%), while when the AES nano emulsion concentration is increased to 0.50wt%, the imbibition displacement efficiency drops to 27.82%, indicating that the higher the nano emulsion concentration is not the better. Since the adsorption of nano emulsion on the solid surface reaches a dynamic equilibrium, the excessive nano emulsion cannot further change the wettability of the solid surface, and the lower oil-water interfacial tension has the opposite effect on the imbibition displacement.
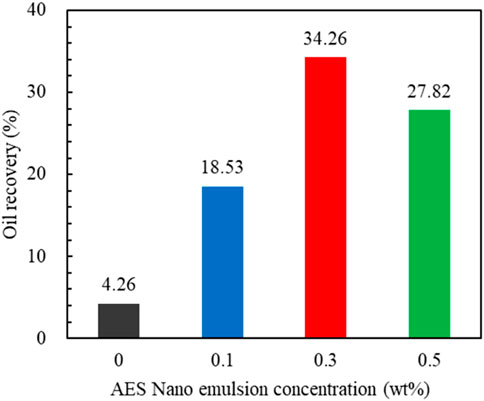
FIGURE 10. Imbibition oil displacement efficiency of AES nano emulsion with different concentrations.
3.3 Effect of rock and crude oil type on imbibition displacement efficiency
In order to compare the effects of lithology and permeability on the imbibition oil displacement efficiency of AES nano emulsion, carbonate rocks (1.22mD, 8.56%), shale (1.08mD, 8.23%) and tight sandstone (1.35mD, 8.54%) with similar permeability and porosity are selected for imbibition oil displacement experiments. At the same time, the imbibition efficiency of tight sandstone with permeability of 0.14, 1.35, 28.97 and 102.34mD is compared. Figure 11 shows the effect of lithology and permeability on the imbibition efficiency of 0.30 wt% AES nano emulsion. When the permeability is similar, the highest imbibition displacement efficiency of AES nano emulsion in tight sandstone is 35.62%, and the worst imbibition displacement efficiency in carbonate rock is 25.94%, with a difference of 9.68%. For tight sandstone, permeability has a significant effect on imbibition displacement efficiency. The imbibition displacement efficiency corresponding to 0.14, 1.35, 28.97 and 102.34 mD is 24.57, 35.62, 42.38% and 57.89%, respectively. The lower the imbibition, the stronger the internal capillary resistance, the more difficult it is for the nano emulsion to enter the interior through spontaneous imbibition for wetting modification, and the lower the corresponding imbibition oil displacement efficiency.
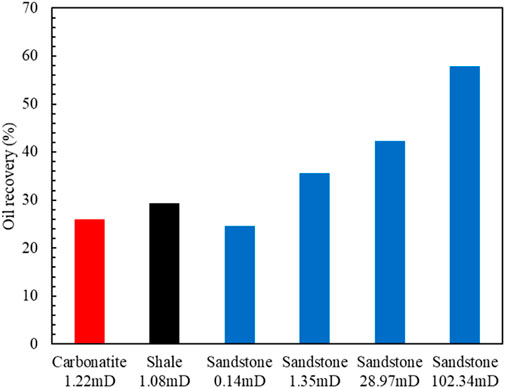
FIGURE 11. Effect of lithology and permeability on imbibition displacement, 0.30 wt% AES nano emulsion.
There are significant differences in crude oil composition in different types of reservoirs. In order to test the effect of AES nano emulsion on the type of crude oil, light crude oil and heavy crude oil saturated cores are selected to test the imbibition displacement efficiency of 0.30 wt% nano emulsion. Table 3 corresponds to the light crude oil C20+ above the molar content of 5.22%, the viscosity of 8 mPas/25°C, heavy crude oil C20+ above the molar content of 42.60%, the viscosity of 3,250 mPas/25°C. Figure 12 shows that the spontaneous imbibition oil displacement efficiency of AES nano emulsion in light and heavy crude oil is 39.59% and 17.28%, respectively. It shows that the emulsification between anionic nano emulsion AES and light crude oil is better, which can produce better imbibition oil displacement effect.
3.4 Analysis of imbibition mechanism of nano emulsion in porous media
The influence of core permeability and lithology on the efficiency of imbibition oil displacement is analyzed. In Figure 13, ① - ③ are the morphologies of crude oil gathered on the surface of the core when the permeability is 0.14, 28.97 and 102.34mD, respectively. When the core permeability is low, the small pore channel occupies the main channel of liquid seepage. When crude oil passes through the small channel from the large channel, the additional resistance of the capillary seriously affects the flow of crude oil, resulting in the crude oil in the low permeability core cannot be effectively converged in the large channel and produced on the ground, as shown in Figure 13A. With the further increase of core permeability, the large channel in the core is the main channel for crude oil seepage, and the crude oil in the small channel converges in the large channel and is driven out of the core by capillary force to achieve higher imbibition oil displacement efficiency. Comparing the morphology and quantity of crude oil formed on the surface of ④ sandstone and ⑤ carbonate rock in Figure 13, it can be seen that AES nano emulsion cannot effectively wet the carbonate rock due to the influence of lithology. The three-phase contact angle of ‘oil-water-rock’ formed by crude oil on the surface of carbonate rock is less than 90°, resulting in weak capillary force of imbibition displacement and low efficiency of imbibition displacement.
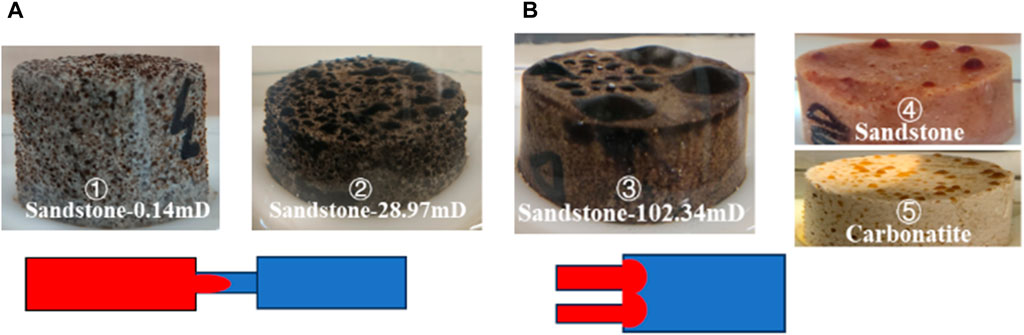
FIGURE 13. The schematic diagram of the influence of lithology and permeability on the effect of imbibition displacement. (A) Crude oil in large pores in lowpermeability core passes throughsmall pores; (B) In high permeability core, smalland medium-sized channels of crudeoil converge in large channels.
Further, the gathering and demulsification process of crude oil in solution after being extracted from the core is analyzed, as shown in Figure 14. Under the action of AES nano emulsion, the crude oil in the core is stripped from the porous medium to form oil-in-water emulsified oil droplets (Figure 14E). After entering the solution, it floats under the action of gravity (Figure 14D), and a large number of oil droplets gathering (Figure 14C). The oil droplets gradually demulsified after gathering (Figure 14B) and converged into a complete oil phase (Figure 14A). The gathering and demulsification process of emulsified oil droplets in solution verified the interaction process between AES nano emulsion and crude oil in porous media.
3.5 Nano emulsion micro-imbibition oil displacement and residual oil distribution
In order to visually observe the imbibition and oil displacement process of AES nano emulsion in porous media, a microfluidic chip device with an inner diameter of 20 mm is used to perform the imbibition and displacement of crude oil with different concentrations of nano emulsion, and ImageJ software is used to calculate the oil saturation in the model. The saturated stained crude oil of the treated oil-wet core is subjected to imbibition displacement experiments using AES nano emulsions with concentrations of 0.05, 0.15, and 0.30 wt%, respectively. The results are shown in Figure 15.
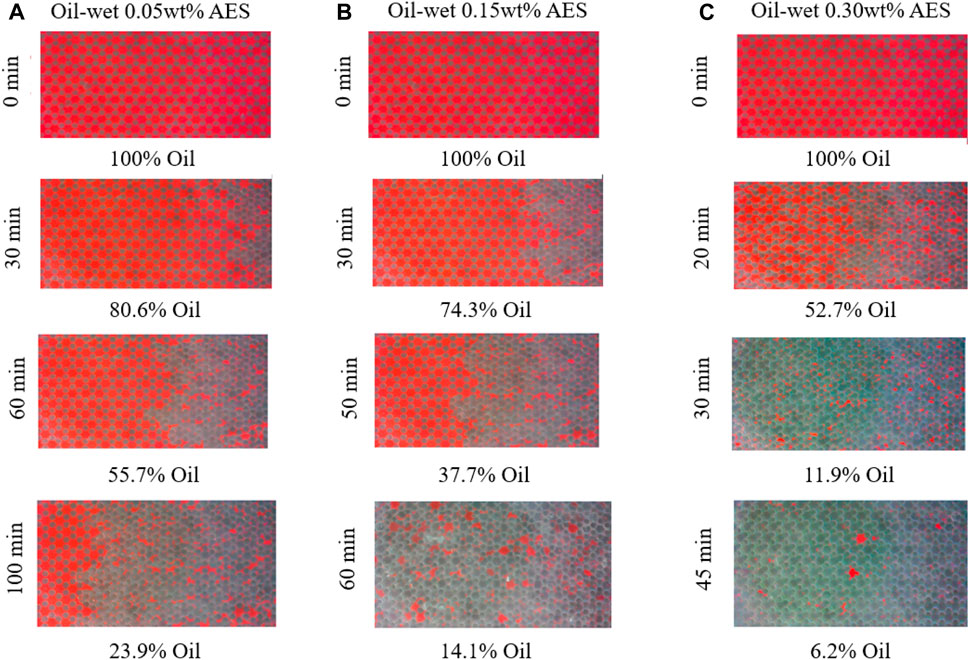
FIGURE 15. Microscopic process of AES nano emulsion imbibition oil displacement with different concentrations.
Figure 15A shows the imbibition displacement process and remaining oil distribution of the oil-wet microfluidic chip model under the action of 0.05 wt% AES nano emulsion. Under the action of AES nano emulsion, the crude oil in the microfluidic chip model is replaced. After 30 min of imbibition, the crude oil at the right end of the model began to be uniformly displaced, and the remaining oil saturation is 80.6%. At 60 min, half of the crude oil in the model is replaced, and the remaining oil saturation is 55.7%. The model imbibition completion time is 100 min, and the final oil saturation is 23.9%. There is still a large amount of crude oil remaining in the pores after imbibition.
Figure 15B shows the imbibition displacement process and remaining oil distribution of the oil-wet microfluidic chip model under the action of 0.15 wt% AES nano emulsion. It can be seen that the remaining oil saturation in the model is 74.3% at 30 min, 37.7% at 50 min, and 14.1% at 60 min, some remaining oil still exists in the swept area after imbibition. The completion time and oil displacement rate of 0.15 wt% AES nano emulsion are higher than those of 0.05 wt% AES nano emulsion. The increase of nano emulsion concentration is beneficial to the imbibition displacement process under the action of capillary force.
Figure 15C shows that when 0.30 wt% AES nano emulsion is used, the imbibition displacement process is completed in about 45 min, the time consumed is much less than 0.05 and 0.15 wt%. At same time, the recovery is the highest, with residual oil saturation of only 6.2%. After the end of imbibition displacement, the model contains only a small amount of crude oil. It is found that the remaining oil is mainly distributed around the pore throat and pore edge of the model, except for the remaining l oil caused by non-uniform imbibition.
Figure 16 shows the regional distribution of crude oil morphology in the model during the imbibition displacement process (0, 15, 40, 60s, respectively). As shown in Figure 16, at the beginning of imbibition (0s), the pores pointed by the black arrow preferentially interact with the nano emulsion, the wetting modification is the fastest, so water driving oil preferentially occurred. The wetting modification of the pore area in the black box is relatively slow, so there is no water driving oil phenomenon in the initial stage. At 40 s, the pores in the black box are gradually affected by the nano emulsion, and the phenomenon of water driving oil begins to form. However, the overall imbibition displacement rate is smaller than that of adjacent pores, and water flows preferentially from adjacent pores. Finally, the oil-water front of spontaneous imbibition flows preferentially from the area with small seepage resistance, and gradually affects on the area with slow wetting modification. The pores in the black box in Figure 16 retain some crude oil that cannot be displaced.
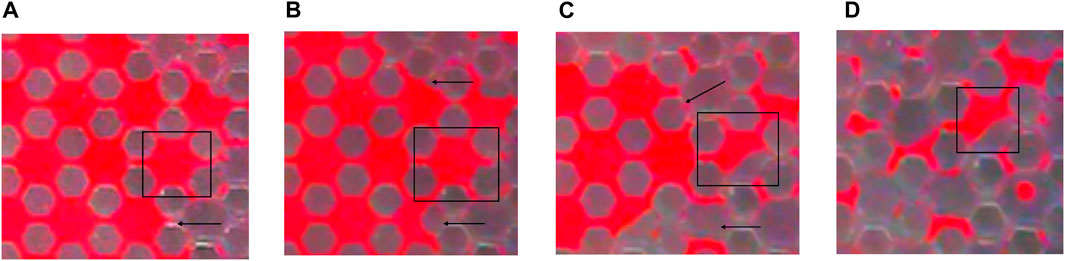
FIGURE 16. Local morphology of remaining oil in the model. (A) t = 0s; (B) t = 15s; (C) t = 40s; (D) t = 60s.
4 Conclusion
This paper based on the test of the basic physical properties of nano emulsions in the spontaneous imbibition displacement process of tight sandstone reservoir, the optimal concentration and type of nano emulsions for the interaction between three kinds of nano emulsions and reservoir rocks and crude oil are determined. Combined with spontaneous imbibition experiments, the effects of nano emulsion concentration, core type, crude oil type on spontaneous imbibition oil displacement efficiency are studied, and the mechanism of nano emulsion under different conditions in the imbibition process is analyzed. The formation process of remaining oil under spontaneous imbibition oil displacement through microscopic experiments is clarified. The main conclusions and recommendations are as follows:
(1) Three kinds of nano emulsions with particle size between 11 and 16 nm have the ability to enter into the deep pore throat of the reservoir, which can achieve good injectivity in the tight reservoir. The oil-water interfacial tension formed by 0.30 wt% AES nano emulsion can be as low as 0.98 mN/m, and the three-phase contact angle of ‘oil-water-gas’ increases from 51.25° to 148.34°. Compared with cationic ADT nano emulsion and nonionic AEO-9 nano emulsion, anionic AES nano emulsion shows excellent performance in terms of injectability, oil-water interfacial tension and wetting modification effect in tight sandstone cores.
(2) Spontaneous imbibition oil displacement experiments show that the higher the nano emulsion concentration is not the better. Since the adsorption of nano emulsion on the solid surface reaches a dynamic equilibrium, the excessive nano emulsion cannot further change the wettability of the solid surface, and the lower oil-water interfacial tension has the opposite effect on the imbibition displacement. The 0.30 wt% AES nano emulsion can improve the efficiency of imbibition and oil displacement in medium-high permeability and sandstone type cores.
(3) Lithology and permeability mainly affect the wetting modification effect of nano emulsion on reservoir rocks and the migration resistance of emulsified oil droplets in porous media. The migration and gathering of emulsified oil droplets in solution mainly include five processes: Saturated oil core/Nano-surfactant fluid emulsion reaction, Aqueous/Emulsified oil droplets, Emulsified oil droplet gathering phase, Demulsifying and emulsifying oil droplets, Oil phase after demulsification. The microscopic experiments show that the formation of remaining oil in porous media is due to the non-uniform wetting modification of nano emulsion in different regions, and the increase of nano emulsion concentration is beneficial to reduce the remaining oil content in the model caused by non-uniformity.
(4) The research results of this paper show that nano-emulsion imbibition displacement can improve the recovery rate of tight sandstone reservoirs, which has certain theoretical significance. All experiments in this paper were carried out in the laboratory. The main shortcomings include core size and reservoir environment, which affect the oil displacement effect of nano-emulsion imbibition displacement, and there are certain differences with the field practice. At the same time, the size of microfluidic chip device can not truly reflect the imbibition displacement effect in dense pore throat.
Data availability statement
The original contributions presented in the study are included in the article/Supplementary Material, further inquiries can be directed to the corresponding author.
Author contributions
ZL: Writing–original draft.
Funding
The author(s) declare that no financial support was received for the research, authorship, and/or publication of this article.
Conflict of interest
ZL was employed by Oil and Gas Production Technology Research Institute, Great Wall Drilling Engineering Technology Research Institute.
Publisher’s note
All claims expressed in this article are solely those of the authors and do not necessarily represent those of their affiliated organizations, or those of the publisher, the editors and the reviewers. Any product that may be evaluated in this article, or claim that may be made by its manufacturer, is not guaranteed or endorsed by the publisher.
References
Anupong, S., Tanakon, N., Maje, A. H., Kalantariasl, A., and Tangparitkul, S. (2022). Influence of sequential changes in the crude oil-water interfacial tension on spontaneous imbibition in oil-wet sandstone. J. Petroleum Sci. Eng. 210, 110032. doi:10.1016/j.petrol.2021.110032
Baihly, J., Altman, R., Malpani, R., and Luo, F. (2010). “Shale gas production decline trend comparison over time and basins,” in Proceedings of the SPE Annual Technical Conference and Exhibition, Florence, Italy, September 19–22, 2010.
Chen, B., and Reynolds, A. C. (2015). “Ensemble-based optimization of the WAG injection process,” in Proceedings of the Society of Petroleum Engineers. SPE Reservoir Simulation Symposium, Houston, Texas, USA, February 2015, 23–25.
Han, L., and Gu, Y. (2015). “Miscible CO2 water-alternating-gas (CO2-WAG) injection in a tight oil formation,” in Proceedings of the SPE Annual Technical Conference and Exhibition, Houston, Texas, USA, September 2015, 28–30.
Hatiboglu, C. U., and Babadagli, T. (2004). “Experimental analysis of primary and secondary oil recovery from matrix by counter-current diffusion and spontaneous imbibition,” in Proceedings of the Paper presented at the SPE Annual Technical Conference and Exhibition, Houston, Texas, September 2004.
Li, A., Fan, T., and Zhao, L. (2011). Experimental study of spontaneous imbibition in low permeability core, fractured reservoir. China Petroleum Geol. Recovery Effic. 18, 67–69. doi:10.7623/syxb200206022
Li, J. (2006). Influence of surfactant system on imbibition process. China: doctoral thesis. Beijing, China: Graduate School of Chinese Academy of Sciences Institute of Percolation Fluid Mechanics.
Liang, X., Liang, T., Zhou, F., Wang, C., Yang, K., Wei, D., et al. (2021b). Impact of shut-in time on production after hydraulic fracturing in fractured shale gas formation: an experimental study. J. Nat. Gas Sci. Eng. 88, 103773. doi:10.1016/j.jngse.2020.103773
Liang, X., Liang, T., Zhou, F., Zhu, J., Wang, R., and Li, Y. (2020). Enhanced fossil hydrogen energy recovery through liquid nanofluid huff-n-puff in low permeability reservoirs. Int. J. Hydrogen Energy 45, 19067–19077. doi:10.1016/j.ijhydene.2020.05.109
Liang, X., Zhou, F., Liang, T., Wang, R., Su, H., and Yuan, S. (2021a). Mechanism of using liquid nanofluid to enhance oil recovery in tight oil reservoirs. J. Mol. Liq. 324, 114682. doi:10.1016/j.molliq.2020.114682
Liu, J., and Sheng, J. (2020). Investigation of countercurrent imbibition in oil-wet tight cores using NMR technology. SPE J. 25, 2601–2614. doi:10.2118/201099-pa
Liu, J., Sheng, J., and Tu, J. (2020). Effect of spontaneous emulsification on oil recovery in tight oil-wet reservoirs. Fuel 279, 118456. doi:10.1016/j.fuel.2020.118456
Liu, J., Sheng, J., Wang, X., Ge, H., and Yao, E. (2019). Experimental study of wettability alteration and spontaneous imbibition in Chinese shale oil reservoirs using anionic and nonionic surfactants. J. Petroleum Sci. Eng. 175, 624–633. doi:10.1016/j.petrol.2019.01.003
Morteza, A., Soheil, S., Mohammad, P., Georgi, D., and Delshad, M. (2017). Nano-scale experimental investigation of in-situ wettability and spontaneous imbibition in ultra-tight reservoir rocks. Adv. Water Resour. 107, 160–179. doi:10.1016/j.advwatres.2017.06.004
Peng, X., Guo, S. P., and Han, D. (2010). Imbibition behavior of brine with and without surfactant in neutral-wet sandstone. China Petroleum Geol. Recovery Effic. 17, 48–51+114. doi:10.13673/j.cnki.cn37-1359/te.2010.04.019
Rodriguez, A., and Maldonado, F. (2019). “Evaluating pressure drawdown strategy for hydraulically fracture shale gas condensate producers,” in Proceedings of the SPE Oklahoma City Oil and Gas Symposium, Oklahoma City, Oklahoma, USA, April 2019.
Shoaib, S., and Hoffman, B. T. (2009). “CO2 flooding the elm coulee field,” in Proceedings of the SPE Rocky Mountain Petroleum Technology Conference, Denver, Colorado, April 2009 (Society of Petroleum Engineers), 14–16.
Sivabalan, S., and Mahmoud, E. (2021). Enhanced oil recovery by spontaneous imbibition of imidazolium based ionic liquids on the carbonate reservoir. J. Mol. Liq. 340, 117301. doi:10.1016/j.molliq.2021.117301
Sivabalan, S., and Mazen, Y. (2021). Spontaneous imbibition characteristics of carbon nanofluids in carbonate reservoirs. Energy Rep. 7, 4235–4248. doi:10.1016/j.egyr.2021.07.002
Surguchev, L., Manrique, E., and Alvarado, V. (2005). “Improved oil recovery: status and opportunities,” in Proceedings of the 18th World Petroleum Congress, Johannesburg, South Africa, September 2005, 25–29.
Wang, J., Huang, Y., Zhang, Y., Zhou, F., Yao, E., and Wang, R. (2020b). Study of fracturing fluid on gel breaking performance and damage to fracture conductivity. J. Petroleum Sci. Eng. 193, 107443. doi:10.1016/j.petrol.2020.107443
Wang, J., Zhou, F., Bai, H., Li, Y., and Yang, H. (2020a). A Comprehensive method to evaluate the viscous slickwater as fracturing fluids for hydraulic fracturing applications. J. Petroleum Sci. Eng. 193, 107359. doi:10.1016/j.petrol.2020.107359
Wang, Y., Zhou, F., Su, H., Li, Y., Yu, F., Dong, R., et al. (2023). Performance evaluation of microemulsion acid for integrated acid fracturing in Middle Eastern carbonate reservoirs. Petroleum Explor. Dev. 50, 1196–1205. doi:10.1016/s1876-3804(23)60458-6
Zhang, K., Sebakhy, K., Wu, K., Jing, G., Chen, N., Chen, Z., et al. (2015). “Future trends for tight oil exploitation,” in Proceedings of the SPE North Africa Technical Conference and Exhibition, Cairo, Egypt, September 14–16, 2015.
Zhu, D., Wang, Y., Cui, M., Zhou, F., Zhang, Y., Liang, C., et al. (2022). Effects of spent viscoelastic-surfactant acid flow on wormholes propagation and diverting performance in heterogeneous carbonate reservoir. Energy Rep. 8, 8321–8332. doi:10.1016/j.egyr.2022.06.056
Nomenclature
Keywords: tight sandstone reservoir, spontaneous imbibition, nano emulsion, action mechanism, microscopic remaining oil
Citation: Li Z (2023) Comprehensive analysis of influencing factors and mechanism of nano emulsion spontaneous imbibition oil displacement in tight sandstone reservoir. Front. Energy Res. 11:1332484. doi: 10.3389/fenrg.2023.1332484
Received: 03 November 2023; Accepted: 27 November 2023;
Published: 06 December 2023.
Edited by:
Yan Peng, China University of Petroleum, Beijing, ChinaReviewed by:
Minghui Li, China University of Petroleum, Beijing, ChinaGuanglong Sheng, Yangtze University, China
Copyright © 2023 Li. This is an open-access article distributed under the terms of the Creative Commons Attribution License (CC BY). The use, distribution or reproduction in other forums is permitted, provided the original author(s) and the copyright owner(s) are credited and that the original publication in this journal is cited, in accordance with accepted academic practice. No use, distribution or reproduction is permitted which does not comply with these terms.
*Correspondence: Zhuang Li, lh_lizhuang@cnpc.com.cn