- 1Key Laboratory of Energy Thermal Conversion and Control of Ministry of Education, School of Energy and Environment, Southeast University, Nanjing, China
- 2Jiangsu Key Laboratory of Micro and Nano Heat Fluid Flow Technology and Energy Application, School of Physical Science and Technology, Suzhou University of Science and Technology, Suzhou, China
Introduction
The increasing greenhouse gas emissions rates are drawing the attention of the world (Chapman et al., 2022). Carbon neutrality is proposed to guide economical construction within energy conservation and environmental protection. For now, various efforts have been taken to realize a low-carbon economy, for example, the developments of cold chain logistics based on the application of liquefied gas (Dong et al., 2021), thermal environment control of buildings based on energy storage using phase change material (Wang et al., 2012; Zhang et al., 2020), and renewable energy supply of vehicles based on hydrogen fuel cells (Tsuchiya, 2008). With the explosively increasing requirements of data processing, the power density of a data center can reach up to 400–3,000 W/m2, which induces high heat dissipation demand (Zhang et al., 2011; Liu et al., 2013). Thus, the CO2 emission for data center refrigeration is increasing rapidly (Deymi-Dashtebayaz and Valipour-Namanlo, 2019). It is urgent to develop refrigeration and cold-storage technologies based on zero-carbon energy. Since the extensively existed renewable energy (such as solar energy and geothermal energy) provides heat instead of electricity, the refrigeration methods driven by the heat source is optimal. We introduce the thermal-driven refrigeration methods and the renewable energy that can be utilized to provide insights for optimizing of low-carbon refrigeration for data centers.
Thermal-Driven Refrigeration
Absorption Refrigeration
As illustrated in Figure 1A, in an absorption refrigeration cycle, the refrigerant absorbs heat from the heat source in the evaporator and turns from liquid into gas, producing a cooling effect. The gaseous refrigerant is absorbed in the absorber and then pumped to the generator to be pressurized. In the condenser, the gaseous refrigerant condenses into liquid and then expands through the valve and returns to the evaporator for cycling (Best and Rivera, 2015).
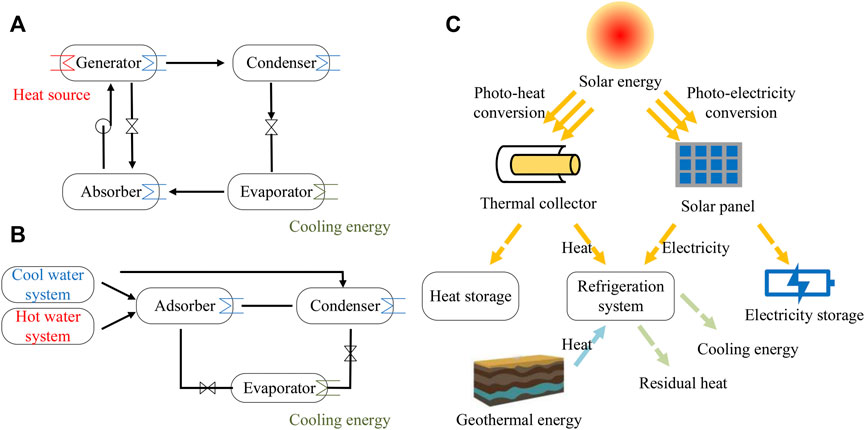
FIGURE 1. Schematic diagram of refrigeration: (A) absorption refrigeration, (B) adsorption refrigeration, and (C) multi-energy complementarity.
The most efficient and common working pairs are water–lithium bromide and ammonia–water. The former is used where moderate temperatures are required while the latter is generally used in large-capacity industrial applications requiring low temperatures. Water–lithium bromide absorption systems are disadvantageous when working at a condensation temperature higher than 40°C due to crystallization (Izquierdo, 2004). The binary ammonia–salt solutions of NH3-NaSCN and NH3-LiNO3 are regarded as a viable alternative for ammonia–water. The Rankine cycle, including single-loop cogeneration cycle, Goswami cycle, and dual-loop cogeneration cycle, is applied in the co- and tri-generation system. The energy efficiency of a trigeneration system can reach more than 80% and meanwhile reduce CO2 emission.
Adsorption Refrigeration
The earliest adsorption refrigeration, single-bed intermittent cycle, is simple and reliable, but the efficiency is very low and the cooling output is discrete, as shown in Figure 1B. So recovery cycles are designed to improve the efficiency and practicability of adsorption refrigeration. Enhancing the adsorbent and optimizing the design of the adsorber are two common ways to improve the heat and mass transfer in adsorption systems. The adsorbent is improved by adding materials with high thermal conductivity. For example, the thermal conductivity of calcium chloride is only 0.1–0.2 W/(m °C) which can be increased by ten times by adding graphite powder (Eltom and Sayigh, 1994). Fins and heat pipes are optimal in increasing the effective heat transfer area of adsorbers (Vasiliev et al., 1996). The effects of fin geometries, spatial positions, and volume fractions of the filled adsorbent in a parallel-flow aluminum flat tube adsorption bed were compared on the heat transfer performance (Chen et al., 2020).
In summary, adsorbent refrigeration has a lower efficiency and is more robust than absorption refrigeration. Some improvements have been made to enhance the efficiencies of adsorbent refrigeration. However, it seems that it does not work for applications below freezing temperature.
Renewable Energy Refrigeration
Among various renewable energy, such as wind energy, biomass energy, solar energy, and geothermal energy (Himri et al., 2009), the last two are the most applicated and easy to access. Hence, refrigeration of data centers based on solar energy and geothermal energy is most feasible.
Solar Energy
The single-effect lithium bromide absorption refrigerators with solar energy as a heat source are superior due to their excellent temperature match and economy. Categories, types, and volumes of solar energy collectors are pivotal in determining energy efficiency of the system (Gao et al., 2021; Yu et al., 2021). The average coefficient of performance (COP) of an absorption air conditioner with solar energy in summer was 0.6, with an average cooling capacity of 40 kW (Ebrahimi and Ahookhosh, 2016). Through TRNSYS software, a solar energy–driven lithium bromide absorption refrigeration system with a capacity of 11 kW was analyzed. The results prove that this system was economically competitive and the effect of global warming was lower than that for conventional refrigeration systems (Balghouthi et al., 2008).
The intensity of solar irradiation meets the duration for refrigeration demand. However, the low power density of solar energy restricts its application. The spotlighting device was proposed to promote the power density of solar energy (Rabl et al., 1978). A composite parabolic concentrator (CPC) combined with a fin-tube adsorber was applied to the adsorption refrigeration, in which the temperature of the adsorption bed rose from 26 to 124°C in 120 min (Wang et al., 2018). Both numerical and experimental studies show that fins enhance the performance of the CPC adsorption bed (Zhao et al., 2019).
Overall, the absorption refrigeration of solar energy is superior to the adsorption refrigeration. However, the inherent instability of solar energy restricts constant and sustainable cooling. Solar-driven refrigeration assisted by energy storage is viable in situations that require unintermittent refrigeration.
Geothermal Energy
A large amount of geothermal energy is contained in the earth’s interior, which offers a stable heat source. It is usually divided into shallow geothermal energy, hydrothermal geothermal energy, and hot dry rock geothermal energy.
The COP of an absorption cycle driven by geothermal energy was expected to reach 0.5654 at the mass flow rate of geothermal energy of 12 kg/s, and the relationship between COP and mass flow rate was positively correlated (Keçeciler et al., 2000). A combination of photovoltaic, solar chimney, and geothermal air tubes was proposed for the refrigeration and ventilation of a room (Rania Elghamry, 2020). It turned out that the room temperature decreased by 3.4°C and the maximum power output of the photovoltaic panel increased by 30%.
Multi-Energy Complementarity
The combination of solar energy and geothermal energy can achieve better performance for refrigeration, as demonstrated in Figure 1C. The geothermal energy with medium and high temperatures is stable and sustainable, which contributes to the stable operation and high cooling efficiency. However, the natural recovery of soil temperature is difficult. Consequently, multi-energy complementarity, which combines the superiorities of geothermal energy and solar energy, is a solution for zero-carbon refrigeration. The solar–geothermal hybrid system showed a 5.5% boost in annual power generation and a 3.4% increment in maximum second efficiency compared to separate systems (Ghasemi et al., 2014). Integrating the CO2-based Enhanced Geothermal Systems (EGS) into the solar power plant can achieve equal or higher efficiency than the sum of the two original systems (Jiang et al., 2017). Moreover, the cost of the system installation and maintenance could be reduced due to the decreased operating pressure and removal of the compressor.
Conclusion
Zero-carbon refrigeration technology based on renewable energy is significant in putting forward the carbon-neutral technical evolution. Multi-energy complementarity is a promising approach to realizing zero-carbon refrigeration for data centers. The high efficiency and sustainable operation of a zero-carbon refrigeration system depends on the efficient utilization of photovoltaic–photothermal energy, energy storage, step utilization of energy, and irreversible losses reduction.
Author Contributions
EH contributed to conception of the study. YL wrote the first draft of the manuscript. All authors contributed to manuscript revision and read and approved the submitted version.
Funding
This work is supported by the National Natural Science Foundation of China (No. 52106023).
Conflict of Interest
The authors declare that the research was conducted in the absence of any commercial or financial relationships that could be construed as a potential conflict of interest.
Publisher’s Note
All claims expressed in this article are solely those of the authors and do not necessarily represent those of their affiliated organizations, or those of the publisher, the editors, and the reviewers. Any product that may be evaluated in this article, or claim that may be made by its manufacturer, is not guaranteed or endorsed by the publisher.
References
Balghouthi, M., Chahbani, M. H., and Guizani, A. (2008). Feasibility of Solar Absorption Air Conditioning in Tunisia. Build. Environ. 43 (9), 1459–1470. doi:10.1016/j.buildenv.2007.08.003
Best, R., and Rivera, W. (2015). A Review of Thermal Cooling Systems. Appl. Therm. Eng. 75, 1162–1175. doi:10.1016/j.applthermaleng.2014.08.018
Chapman, A., Ertekin, E., Kubota, M., Nagao, A., Bertsch, K., Macadre, A., et al. (2022). Achieving a Carbon Neutral Future through Advanced Functional Materials and Technologies. Bull. Chem. Soc. Jpn. 95 (1), 73–103. doi:10.1246/bcsj.20210323
Chen, R., Quan, Z., Zhao, Y., and Tang, S. (2020). Performance Analysis of Adsorption Refrigeration System Based on Parallel Flow Flat Tube. J. Eng. Thermophys. 41 (4), 981–988.
Deymi-Dashtebayaz, M., and Valipour-Namanlo, S. (2019). Thermoeconomic and Environmental Feasibility of Waste Heat Recovery of a Data Center Using Air Source Heat Pump. J. Clean. Prod. 219, 117–126. doi:10.1016/j.jclepro.2019.02.061
Dong, Y., Xu, M., and Miller, S. A. (2021). Overview of Cold Chain Development in China and Methods of Studying its Environmental Impacts. Environ. Res. Commun. 2 (12), 122002. doi:10.1088/2515-7620/abd622
Ebrahimi, M., and Ahookhosh, K. (2016). Integrated Energy-Exergy Optimization of a Novel Micro-CCHP Cycle Based on MGT-ORC and Steam Ejector Refrigerator. Appl. Therm. Eng. 102, 1206–1218. doi:10.1016/j.applthermaleng.2016.04.015
Elghamry, R., and Hassan, H. (2020). An Experimental Work on the Impact of New Combinations of Solar Chimney, Photovoltaic and Geothermal Air Tube on Building Cooling and Ventilation. Sol. Energy 205, 142–153. doi:10.1016/j.solener.2020.05.049
Eltom, O. M. M., and Sayigh, A. A. M. (1994). A Simple Method to Enhance Thermal Conductivity of Charcoal Using Some Additives. Renew. Enerqy 4 (1), 113–118. doi:10.1016/0960-1481(94)90072-8
Gao, W., Lei, Z., Wu, K., and Chen, Y. (2021). Reconfigurable and Renewable Nano‐Micro‐Structured Plastics for Radiative Cooling. Adv. Funct. Mat. 31 (21), 2100535. doi:10.1002/adfm.202100535
Ghasemi, H., Sheu, E., Tizzanini, A., Paci, M., and Mitsos, A. (2014). Hybrid Solar-Geothermal Power Generation: Optimal Retrofitting. Appl. Energy 131, 158–170. doi:10.1016/j.apenergy.2014.06.010
Himri, Y., Malik, A. S., Boudghene Stambouli, A., Himri, S., and Draoui, B. (2009). Review and Use of the Algerian Renewable Energy for Sustainable Development. Renew. Sustain. Energy Rev. 13 (6-7), 1584–1591. doi:10.1016/j.rser.2008.09.007
Izquierdo, M. (2004). Crystallization as a Limit to Develop Solar Air-Cooled LiBr-H2o Absorption Systems Using Low-Grade Heat. Sol. Energy Mater. Sol. Cells 81 (2), 205–216. doi:10.1016/j.solmat.2003.11.002
Jiang, P.-X., Zhang, F.-Z., and Xu, R.-N. (2017). Thermodynamic Analysis of a Solar-Enhanced Geothermal Hybrid Power Plant Using CO 2 as Working Fluid. Appl. Therm. Eng. 116, 463–472. doi:10.1016/j.applthermaleng.2016.12.086
Keçeciler, A., Acar, H. I., and Doğan, A. (2000). Thermodynamic Analysis of the Absorption Refrigeration System with Geothermal Energy: an Experimental Study. Energy Convers. Manag. 41, 37–48. doi:10.1016/S0196-8904(99)00091-6
Liu, X., Chen, Y., and Shi, M. (2013). Dynamic Performance Analysis on Start-Up of Closed-Loop Pulsating Heat Pipes (CLPHPs). Int. J. Therm. Sci. 65, 224–233. doi:10.1016/j.ijthermalsci.2012.10.012
Rabl, A., Goodman, N. B., and Winston, R. (1978). Practal Design Considerations for CPC Solar Collectors. Sol. Energy 22, 373–381. doi:10.1016/0038-092X(79)90192-0
Tsuchiya, H. (2008). Innovative Renewable Energy Solutions for Hydrogen Vehicles. Int. J. Energy Res. 32 (5), 427–435. doi:10.1002/er.1374
Vasiliev, L. L., Kanonchik, L. E., Antuh, A. A., and Kulakov, A. G. (1996). NaX Zeolite, Carbon Fibre and CaCl2 Ammonia Reactors for Heat Pumps and Refrigerators. Adsorption 2, 311–316. doi:10.1007/bf00879546
Wang, Y. J., Tao, H. G., and Zhang, Z. P. (2012). Experimental Study of Binary Composite Phase Change Material for Building. Amm 253-255, 354–357. doi:10.4028/www.scientific.net/AMM.253-255.354
Wang, Y., Li, M., Du, W., Yu, Q., Ji, X., and Ma, X. (2018). Performance Comparative Study of a Solar-Powered Adsorption Refrigerator with a CPC Collector/adsorbent Bed. Energy Convers. Manag. 173, 499–507. doi:10.1016/j.enconman.2018.07.080
Yu, C., Li, H., Chen, J., Qiu, S., Yao, F., and Liu, X. (2021). Investigation of the Thermal Performance Enhancement of a Photovoltaic Thermal (PV/T) Collector with Periodically Grooved Channels. J. Energy Storage 40, 102792. doi:10.1016/j.est.2021.102792
Zhang, C., Chen, Y., Wu, R., and Shi, M. (2011). Flow Boiling in Constructal Tree-Shaped Minichannel Network. Int. J. Heat Mass Transf. 54 (1), 202–209. doi:10.1016/j.ijheatmasstransfer.2010.09.051
Zhang, C., Li, J., and Chen, Y. (2020). Improving the Energy Discharging Performance of a Latent Heat Storage (LHS) Unit Using Fractal-Tree-Shaped Fins. Appl. Energy 259, 114102. doi:10.1016/j.apenergy.2019.114102
Keywords: absorption refrigeration, adsorption refrigeration, solar energy, geothermal energy, multi-energy complementarity
Citation: Lu Y and Huo E (2022) Viewpoints on the Refrigeration by Renewable Energy. Front. Energy Res. 10:928187. doi: 10.3389/fenrg.2022.928187
Received: 25 April 2022; Accepted: 09 May 2022;
Published: 14 June 2022.
Edited by:
Xiangdong Liu, Yangzhou University, ChinaCopyright © 2022 Lu and Huo. This is an open-access article distributed under the terms of the Creative Commons Attribution License (CC BY). The use, distribution or reproduction in other forums is permitted, provided the original author(s) and the copyright owner(s) are credited and that the original publication in this journal is cited, in accordance with accepted academic practice. No use, distribution or reproduction is permitted which does not comply with these terms.
*Correspondence: Erguang Huo, huoerguang@126.com