- 1Institute of Chemical Industry of Forest Products, CAF, Nanjing, China
- 2National Engineering Laboratory for Biomass Chemical Utilization, Nanjing, China
- 3Key and Open Laboratory of Forest Chemical Engineering, SFA, Nanjing, China
- 4Key Laboratory of Biomass Energy and Material, Nanjing, China
- 5Co-Innovation Center of Efficient Processing and Utilization of Forest Resources, Nanjing Forestry University, Nanjing, China
Alkali lignin-degrading Bacillus were isolated from forest soils in China and were identified as Bacillus subtilis TR-03 and Bacillus cereus TR-25 by 16S rDNA sequence analysis. Wherein TR-03 displayed optimal 26.72% alkali lignin (2 g/L) degradation at 7 days and 71.23% of Azure-B (0.01%) decolorization at 36 h of cultivation at 37°C. Ligninolytic enzyme analysis revealed that TR-03 was capable of depolymerizing alkali lignin effectively by the producing of lignin peroxidase and laccase, wherein higher laccase activity was cell-associated. At last, the physical and chemical changes of lignin via SEM and FTIR analysis was further observed to prove the lignin degradation by Bacillus subtilis TR-03.
Introduction
Lignin is an aromatic heteropolymer and closely associated with hemicellulose and cellulose to form a complex compound. Owing to its irregular matrix structure and highly branched polymer network, lignin is extremely resistant to microbial breakdown (Muaaz-Us-Salam et al., 2020). The degradation of lignin is critical in the conversion of lignocellulose into second-generation biofuels as well as the lignin valorization strategies (Tonin et al., 2016). Lignin depolymerization by microorganism is one of the significant mechanisms due to its low energy consumption, no chemical addition and environmental friendliness (Niu et al., 2021).
So far, fungi are dominant in the efficiently degradation of lignin and the related enzymes including Manganese peroxidase (MnP), Lignin peroxidase (Lip) and Laccase (Lac) (Wong, 2009) have been extensively studied. LiP is a glycoprotein and could oxidize both non-phenolic and phenolic compounds. MnP is a glycosylated heme-protein and could convert lignin phenolic compounds to phenoxy-radicals. Lac is a multi-copper oxidase and could oxidize the phenolic hydroxyl of lignin to destroy the internal stability of the aromatic ring (Xu Z. et al., 2018; Weng et al., 2021). However, the lignin degradation by fungi failed to make a breakthrough due to the strict growth conditions, long pretreatment cycle, consumption of cellulose and hemicellulose, poor adaptability to the environment (Wang et al., 2013). In contrast, bacteria have been much less clarified as degraders of lignin, even though bacteria are identified as important participants in the lignin depolymerization (Mei et al., 2020). Compared with fungi, bacteria offer advantages for lignocellulosic application, such as rapid propagation, strong environmental adaptability, convenient molecular genetics and protein expression with a smaller genome, etc., (Tonin et al., 2016). Studies have confirmed that bacteria can also secrete enzymes required for lignin degradation, metabolize poplar dioxane lignin and break the lignin biphenyl structure (Navas et al., 2019).
So far, some lignin-degrading bacteria strains such as Thodococcus jostii (Henson et al., 2018), Pseudomonas (Xu R. et al., 2018), Bacillus Sp have been isolated from soil, sediments, animals, insect guts, etc. Bacillus Sp. is a kind of gram-positive bacteria, which can produce spores with special resistance to adverse conditions. Nowadays, Bacillus Sp is widely used in the production of enzymes, antibiotics and exogenous proteins, and has become an important biotechnology tool in the industrial production of medicine, feed and food (Zhu et al., 2020). Bacillus Sp. is known as lignin-degrading bacteria since a few years ago, and several stains, such as Bacillus subtilis, Bacillus licheniformis, Bacillus ligniniphilus have been isolated from compost, soil or other environment all around world (Yang et al., 2021; Lu et al., 2012; Zhu et al., 2017). Bacillus could effectively degrade lignin through the secretion of ligninolytic enzymes including Lip, Lac and MnP (Zhu et al., 2017; Chang et al., 2014). Compared with other lignin degrading bacteria, Bacillus Sp. is secure and can form dormant spores in adverse environment, which is feasible in the industrial application of lignin degradation.
Although some Bacillus strains have been proved to be capable of solubilizing lignin, their lignin-degrading mechanism was still unclear and the lignin-degrading rate was lower than that of fungi. Mei et al. (2020) found the maximum lignin degradation rate of Bacillus amyloliquefaciens SL-7 was 28.55%, which was close to that of 30–36% of fungi. However, the generally degradation rate of lignin in most Bacillus was only 15–18% (Chang et al., 2014; Mishra et al., 2017; Weng et al., 2021). Thus, more efficient ligninolytic Bacillus strains should be explored to expand the knowledge of lignin valorization by bacteria. This paper focuses on the isolation and characterization of Bacillus strains from forest soil samples and their degradation capability of alkali lignin. The isolates obtained are feasible in lignin degradation owing to their excellent growth rate as well as high activity of ligninolytic enzymes.
Materials and Methods
Materials
Soil samples were collected from Zijin Mountain of Nanjing City, China. 2 g samples were suspended in 30 ml LB medium (peptone 10 g/L, yeast extract 5 g/L, NaCl 10 g/L) for enrichment culture at 37°C for 20 h, separately. 1 ml of the clear supernatant was added into 9 ml sterile water and incubated at 75–80°C for 15–20 min. After mixing all the samples, 1 ml of the solution was diluted using 0.9% normal saline and inoculated into the selective medium (alkali lignin medium: MgSO4 7H2O 0.2 g, K2HPO4 1.0 g, KH2PO4 1.0 g, (NH4)2SO4 2.0 g, CaCl2 0.1 FeSO4 7H2O 0.05 g, MnSO4 H2O 0.02 g, CuSO4 5H2O 1.0 mg, ZnSO4 7H2O 1.0 mg, alkali lignin 2.0 g; Azure-B medium: 0.01% azure-B was added in LB medium; 15 g agar was added in 1,000 ml distilled water). The plates were incubated at 29°C, and single colonies were isolated on LB plates.
Identification of Bacterial Isolates
Genomic DNA of the screened was extracted according to the DNA isolation Kit (Sangon Biotech, China). 16S rDNA gene was amplifed using universal primers (27 F: 5′-AGAGTTTGATCCTGGCTCAG-3′ and 1541 R:5′-AAGGAGGTGATCCAGCC-3′). PCR was performed with an initial denaturation step at 94°C for 5 min followed by 30 cycles of denaturation at 94°C for 30 s, annealing at 55°C for 30 s, extension at 72°C for 60 s and a final extension at 72°C for 10 min. Amplified product was run on 0.8% agarose gel, then recovered and sequenced by Sangon Biotech (Shanghai, China). The resulting sequences were compared using BLAST program and MEGA 7.0 software.
Dye Decolorization of Azure-B and Lignin Degradation
The fading rate of Azure-B in liquid medium by lignin-degrading isolates was determined. The isolates were maintained on LB slants at 4°C. Mature spores were harvested with physiological saline and incubated at 37°C and 150 rpm for about 12 h (about OD600 1.0) to make seed culture. 1% seed volume was inoculated into Azure-B (0.01%)-LB fluid medium and cultured in dark at 180 rpm, 37°C. The Azure-B (0.01%)-LB medium with inactivated bacterium as the control. 1 ml fermentation liquor was taken at 6, 12, 18, 24 and 36 h and centrifuged at 1,000 rpm, 8 min. The supernatant was measured for absorbance at 590 nm Microplate reader (Multi-mode Reader, Gene Company Limited, China). The formula of decolorizing rate (Picart et al., 2016) was as follows:
Where λ* is the absorbance of the control sample, which is 0.775; λ is the absorbance of the test samples.
The decrease in absorbance at 280 nm was determined to characterize the lignin degradation (Kumar et al., 2015). 2 ml culture was harvested and centrifuged at 8,000 rpm for 10 min. The absorbance of the supernatant at 280 nm was measured, with uninoculated medium as the control. The degradation ratio of lignin was calculated according to the equation described by Xu Z. et al. (2018). All of the experiments was conducted in triplicates.
Ligninolytic Enzymes Assay
Fermentation was performed with 50 ml alkali lignin medium in a 250 ml shake flask, at 37°C and 150 rpm for 48 h, with 2% (v/v) inoculum. 2 ml cultured fluid was centrifuged at 8,000 rpm for 10 min and the supernatant was used for Lac, Lip and MnP activities measurement according to the procedures of Yang et al. (2011) using ABTS, veratryl and 2,6-dimethoxyphenol as substrates. One unit of laccase activity was defined as the amount of enzyme required to oxidize 1 μmol of substrate per min. All assays were performed in triplicate.
Intracellular Laccase Activity and Characteristics
The intracellular laccase activity was further determined according to the method described by Lu et al. (2012). The bacterial pellets were obtained by the centrifugation at 8,000 rpm for 10 min at 4°C. The cells were broken by lysozyme (0.1 mg/ml) at 37°C for 10 min, washed by 1 M, 0.14 M NaCl and 0.1% (w/v) SDS containing 10 mM EDTA and 0.3 mg/ml PMSF, respectively, then heated at 80°C for 10 min, and washed with sterile deionized water for 3 times. The cell extract was suspended in sterile deionized water to make the crude intracellular enzyme.
The effect of pH on the spore laccase activity was measured under standard assay conditions at 30°C in citrate–phosphate buffer (0.1 M, pH 2.2–7.0) and Tris-HCl buffer (0.1 M, pH7.0–9.0). The intracellular activity was measured based on the oxidation of ABTS, syringaldazine (SGZ) and 2,6-dimethoxyphenol (2,6-DMP) at different pH.
The effect of temperature on the spore laccase activity was investigated under standard assay conditions at temperatures ranging from 20 to 100°C for 5 min. The residual laccase activity was measured periodically with ABTS as the substrate at pH 3.0. The maximum enzyme activity was considered as 100% to calculate the relative activity.
Scanning Electron Microscopy
SEM images were conducted to analyze the microstructural changes and surface characteristics of untreated and pretreated lignin. Control and inoculated samples (50 ml for each) were centrifuged at 8,000 rpm for 10 min. The cell-free supernatant was dried by a freeze drier (LGJ-10, Beijing Songyuanhuaxing Technology Develop Co., Ltd., China). The dried samples were mounted on a stub and sputter-coated with gold prior to imaging with a field emission scanning electron microscope (3400-N, Hitachi, Japan) using 2 kV accelerating voltage.
FTIR Spectroscopy
The structures of untreated and pretreated alkali lignin were analyzed by FTIR spectroscopy. 1 mg dried sample was uniformly mixed with 100 mg KBr and then pressed into a transparent thin film, with a thickness of 10–20 μm. Infrared spectra were conducted by a spectrometer (MAGNA-IR 550, Thermo Nicolet Corporation, United States). Sample spectra were recorded from 4,000 to 400 cm−1 with a spectral resolution of 2 cm−1 using an accumulation of 32 scans.
Results
Screening of Lignin-Degrading Bacillus and Identification
Bacillus sp. strains were proved to be important microorganism in the depolymerization of lignin (Zhou et al., 2017). Several strains of Bacillus sp. have been screened worldwide and their lignin-degrading abilities have been studied. To enrich the diversity of lignin-decomposing bacteria, azure-B and lignin plates were used to screen the lignin-degrading Bacillus. Two strains were isolated from soil samples from Zijin Mountain and named as TR-03 and TR-25. Prominent pale yellow and light brown areas appeared around the colonies on the Azure-B plates (Figure 1A), which illustrates probably LiP, Lac or MnP production of the strains. Furthermore, the 2 strains can effectively grow on the selective medium with lignin as the sole carbon (Figure 1), which also confirms their preliminary lignin-degrading ability.
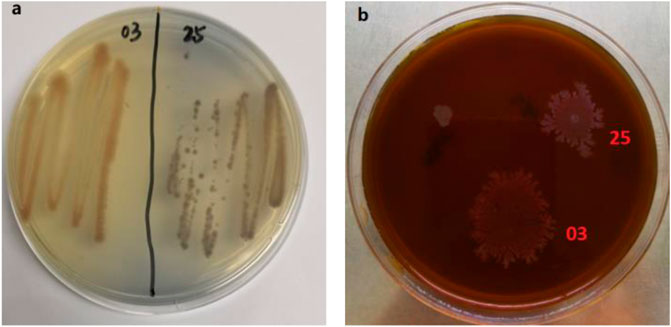
FIGURE 1. Screened strains on the Azure-B and lignin plates.(A) Azure-B plates, with 0.01% azure-B added; (B) alkali lignin plates with alkali lignin as the sole carbon source (2 g/L).
The sequence of 16S rDNA of isolate was determined and submitted to NCBI with accession number of OK559643 and OK559727, respectively. On the basis of 16 S rDNA gene sequencing and taxonomic analyses, TR-03 was closely matched to Bacillus subtilis NR 112116.2 and TR-25 was closely matched to Bacillus cereus NR 115714.1 (Figure 2). Thus, the isolates were identified and named as Bacillus subtilis TR-03 and Bacillus cereus TR-25.
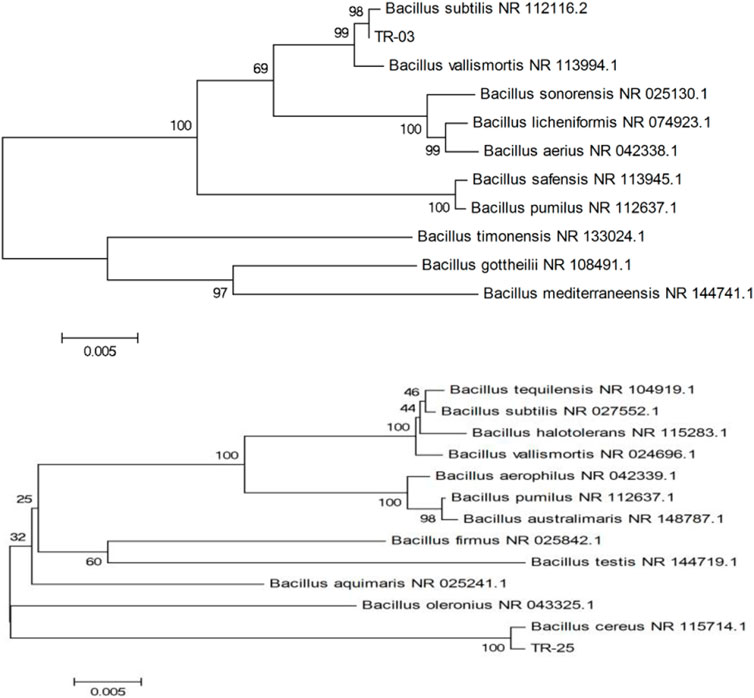
FIGURE 2. Evolutionary relationships and phylogenetic trees based on 16S rDNA sequence of TR-03 and TR-25.
Decolorizing of Azure-B and Lignin Degradation
TR-03 and TR-25 were preliminarily confirmed to decolorize the Azure-B during the plate screening. To quantitatively determine their decoloration ability, fluid medium supplemented with Azure-B was further prepared and the decolorizing rate was determined (Figure 3). The isolates both showed obvious decolorizing of Azure-B. Especially for TR-03, which showed the highest rate of 71.23% Azure-B decolorization at 36 h of cultivation and over 94% of decolorization appeared at about 18 h of cultivation. TR-25 also presented excellent decolorization of Azure-B, with decolorizing rate over 50% at 36 h of cultivation. Azure-B belongs to triarylmethane dyes, with similar structure to that of lignin, and is usually used as the substrate to measure the enzyme activity of lignin-degradation (Bandounas et al., 2013). Tawaf et al. found that Bacillus tequilensis AN-1 only showed 67.6% decolorization of 2.5 mg/L Azure-B after 7 days (Tawaf et al., 2018). Thus, the rapid decolorization of Azure-B suggests the lignin-degrading potentiality of both the isolates.
The intense absorption of benzene ring at 280 nm was characterized to indicate the lignin degradation for the isolates (Chai et al., 2014), and the results are shown in Figure 4. The absorbance of A280 dropped from 0.348 to 0.255 and 0.268 during the 7 days of incubation for TR-03 and TR-25, respectively (data not shown), which means 26.72 and 23% of the lignin degradation. And most of the degradation occurred in the initial 4 days. It indicates that the isolates could break down lignin for their growth and metabolism when lignin is used as sole carbon source. At the same time, both the Azure-B decoloration and lignin degradation tests show more superior lignin-degrading activity for the Bacillus subtilis TR-03. Thus, TR-03 was further investigated for the characterization of lignin depolymerization.
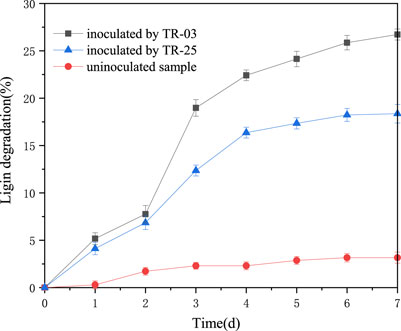
FIGURE 4. Time course of alkali lignin degradation at A280 by TR-03 and TR-25. Initial concentration of alkali lignin was 2 g/L.
Micro-morphology by SEM
The change in the morphology of the alkali lignin by Bacillus subtilis TR-03 was further observed by SEM and the images were presented in Figure 5. The figure showed that the untreated lignin maintained irregular spherical shapes with porous internal structure (Figure 5A). After microbial treatment, the structure of alkali lignin was severely damaged, and smaller-sized particles of lignin were produced instead of irregular spherical particles (Figure 5B).
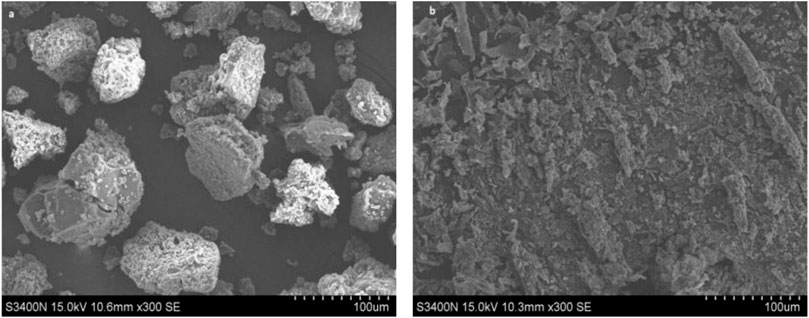
FIGURE 5. SEM images on lignin treated with Bacillus subtilis TR-03. (A) untreated lignin; (B) lignin treated for 5 days with Bacillus subtilis TR-03.
FTIR Spectroscopy
FTIR could demonstrate a visualized and rapid indication of the changes in chemical bonds of lignin (Chen et al., 2015). The absorption peaks in FTIR spectra of alkali lignin samples treated by Bacillus subtilis TR-03 are presented in Figure 6. It can be seen that the FTIR spectra of alkali lignin showed obvious changes from 1,600 to 600 cm−1. The bands representing the aromatic skeleton vibrations appeared at 1,598 cm−1 and 1,410 cm−1 (Chong et al., 2017; Sun et al., 2019). The weaker intensity of these two signals indicated the breakage or replacing of the aromatic ring chain reaction of lignin structure, which suggested that the lignin was degraded to a certain extent with the Bacillus subtilis TR-03 treatment. The band at 1,117 cm−1 was attributed to the C-O stretch of syringyl group and the band at 1,046 cm−1 may be assigned to the deformation of aromatic ring of guaiacyl group in the lignin (Wang et al., 2015). After degradation, the different intensities of 1,117 cm−1 and 1,046 cm−1 suggested the destroyed syringyl and guaiacyl group in the delignification process of lignin, and the degradation of guaiacyl group was faster than that of syringyl group.
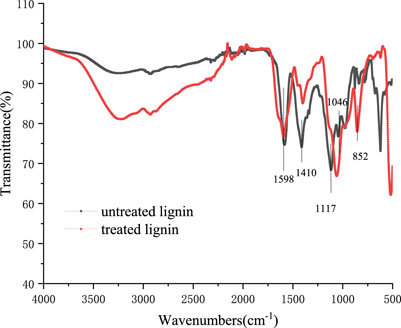
FIGURE 6. FTIR spectrum of alkali lignin before and after biodegradation with Bacillus subtilis TR-03.
Enzyme Activity Assay of Bacillus subtilis TR-03
There are three kinds of enzymes involved in lignin degradation, namely lignin peroxidase (Lip), laccase (Lac) and manganese peroxidase (MnP) (Xu et al., 2017). The enzymes activities determine the ability of bacteria to degrade lignin. Mei et al. (2020) found that Bacillus amyloliquefaciens SL-7 could produce MnP, Lip and Lac with the maximum level of 258.57, 422.68 and 55.95 U/L during 7 days, which could reduce the chemical oxygen demand by 69.35% in lignin. The above-mentioned results all exhibit the considerable lignin degradation by Bacillus subtilis TR-03. The degradation must be related to various lignin degrading enzymes. The activities of three enzymes were determined during 7 days incubation of TR-03. The results show that TR-03 showed considerable Lip and Lac activity, but with no MnP activity observed (Figure 7). The maximum Lip and Lac reached 315.34 U/L and 54.26 U/L on day 4 of incubation, and the Lac activity was lower than that of Lip on the whole level.
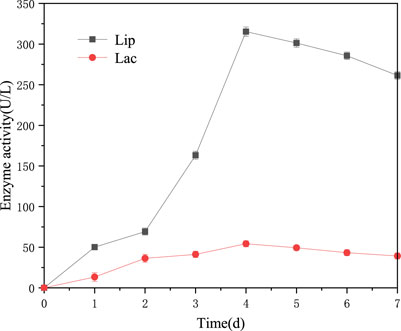
FIGURE 7. Time course of lignin-degrading enzymes in the culture of strain TR-03. Initial concentration of alkali lignin was 2 g/L.
Intracellular laccase activity was 129.44 U/L at the end of the fermentation, which was extremely higher than that of extracellular Lac activity. The results are directly in line with that of Chang et al. (2014), which indicates that more local activity was cell-associated. Intracellular and extracellular Lac activities were found in several Bacillus sp. strains, such as Bacillus atrophaeus and Bacillus pumilus, which could successfully degrade kraft lignin (Huang et al., 2013). The intracellular laccase showed an extensive pH range for catalyzing different substrates with an optimum pH of 3.0, 6.6 and 8.2 for ABTS, SGZ and 2,6-DMP, respectively (Figure 8A). For temperature adaptability, the crude intracellular laccase showed relatively high activity between 40–70°C, with the maximal activity observed at 50°C (Figure 8B). Lac from Bacillus sp. is found to withstand high temperature (80–90°C) and alkaline conditions (pH 7–11) (Xu et al., 2019; Das et al., 2020). The extreme robustness in pH and temperature adaptability of intracellular laccase from TR-03 makes it available in the application of different industrial and environmental remediation processes, such as delignification, pulp bleaching and wastewater treatment.
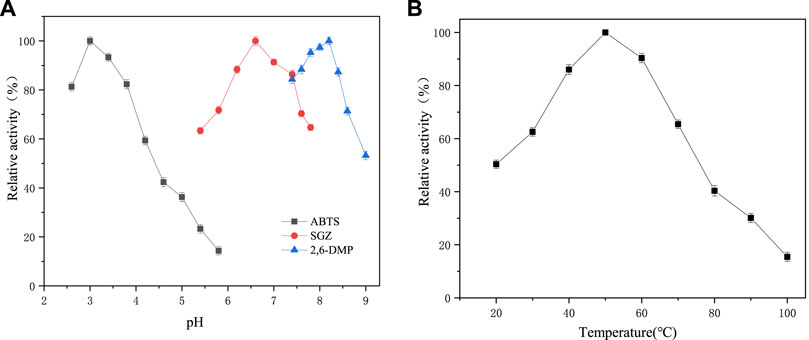
FIGURE 8. Effect of pH and temperature on intracellular laccase activity of Bacillus subtilis TR-03. (A) pH, with ABTS, SGZ and 2,6-DMP as substrate at 30°C; (B) temperature, with ABTS as the substrate at pH 3.0.
Conclusion
Effective ligninolytic Bacillus sp. strains were isolated from forest soils and investigated their capability of alkali lignin. Bacillus subtilis TR-03 show fairly ligninolytic ability by producing enzymes of Lip and Lac. SEM and FTIR results show the physical and chemical changes in the lignin by smaller particle sizes and the breakage or replacing of the aromatic ring chain reaction of lignin structure. These results prove that Bacillus sp. strains were important microorganism in the depolymerization of lignin, and further study should be focus on the degrading mechanism of lignin by Bacillus sp.
Data Availability Statement
The datasets presented in this study can be found in online repositories. The names of the repository/repositories and accession number(s) can be found below: https://www.ncbi.nlm.nih.gov/, OK559643 https://www.ncbi.nlm.nih.gov/, OK559727.
Author Contributions
All authors read and approved the final manuscript. JY, JZ, HX, and NZ carried out the studies and drafted the manuscript, JJ, JX, and MW participated in the project design and manuscript preparation. The work undertaken is not owned by nor has it been conducted for any for-profit entity. The authors have no financial interest in this work.
Funding
This work was financially supported by the Key Lab. of Biomass Energy and Material, Jiangsu Province (JSBEM-S-202011), Natural Science Foundation of Jiangsu Province (BK20200162), National Natural Science Foundation of China (31770636), and Natural Science Foundation of Jiangsu Province (Grant No. K20180153).
Conflict of Interest
The authors declare that the research was conducted in the absence of any commercial or financial relationships that could be construed as a potential conflict of interest.
Publisher’s Note
All claims expressed in this article are solely those of the authors and do not necessarily represent those of their affiliated organizations or those of the publisher, the editors, and the reviewers. Any product that may be evaluated in this article, or claim that may be made by its manufacturer, is not guaranteed or endorsed by the publisher.
References
Bandounas, L., Pinkse, M., de Winde, J. H., and Ruijssenaars, H. J. (2013). Identification of a Quinone Dehydrogenase from a Bacillus Sp. Involved in the Decolourization of the Lignin-Model Dye, Azure B. New Biotechnol. 30 (2), 196–204. doi:10.1016/j.nbt.2012.04.003
Chai, L.-y., Chen, Y.-h., Tang, C.-j., Yang, Z.-h., Zheng, Y., and Shi, Y. (2014). Depolymerization and Decolorization of Kraft Lignin by Bacterium Comamonas Sp. B-9. Appl. Microbiol. Biotechnol. 98, 1907–1912. doi:10.1007/s00253-013-5166-5
Chang, Y.-C., Choi, D., Takamizawa, K., and Kikuchi, S. (2014). Isolation of Bacillus Sp. Strains Capable of Decomposing Alkali Lignin and Their Application in Combination with Lactic Acid Bacteria for Enhancing Cellulase Performance. Bioresour. Techn. 152 (1), 429–436. doi:10.1016/j.biortech.2013.11.032
Chen, Y., Huang, J., Li, Y., Zeng, G., Zhang, J., Huang, A., et al. (2015). Study of the Rice Straw Biodegradation in Mixed Culture of Trichoderma Viride and Aspergillus niger by GC-MS and FTIR. Environ. Sci. Pollut. Res. 22, 9807–9815. doi:10.1007/s11356-015-4149-8
Chong, G.-G., Huang, X.-J., Di, J.-H., Xu, D.-Z., He, Y.-C., Pei, Y.-N., et al. (2017). Biodegradation of Alkali Lignin by a Newly Isolated Rhodococcus Pyridinivorans Cczu-B16. Bioproc. Biosyst. Eng. 41, 501–510. doi:10.1007/s00449-017-1884-x
Das, R., Liang, Z., Li, G., and An, T. (2020). A Non-blue Laccase of Bacillus Sp. GZB Displays Manganese-Oxidase Activity: A Study of Laccase Characterization, Mn(II) Oxidation and Prediction of Mn(II) Oxidation Mechanism. Chemosphere 252, 126619. doi:10.1016/j.chemosphere.2020.126619
Henson, W. R., Hsu, F.-F., Dantas, G., Moon, T. S., and Foston, M. (2018). Lipid Metabolism of Phenol-Tolerant Rhodococcus Opacus Strains for Lignin Bioconversion. Biotechnol. Biofuels 11 (339), 1–15. doi:10.1186/s13068-018-1337-z
Huang, X.-F., Santhanam, N., Badri, D. V., Hunter, W. J., Manter, D. K., Decker, S. R., et al. (2013). Isolation and Characterization of Lignin-Degrading Bacteria from Rainforest Soils. Biotechnol. Bioeng. 110, 1616–1626. doi:10.1002/bit.24833
Kumar, M., Singh, J., Singh, M. K., Singhal, A., and Thakur, I. S. (2015). Investigating the Degradation Process of Kraft Lignin by β-proteobacterium, Pandoraea Sp. ISTKB. Environ. Sci. Pollut. Res. 22, 15690–15702. doi:10.1007/s11356-015-4771-5
Lu, L., Zhao, M., Wang, T.-N., Zhao, L.-Y., Du, M.-H., Li, T.-L., et al. (2012). Characterization and Dye Decolorization Ability of an Alkaline Resistant and Organic Solvents Tolerant Laccase from Bacillus Licheniformis Ls04. Bioresour. Techn. 115, 35–40. doi:10.1016/j.biortech.2011.07.111
Mei, J., Shen, X., Gang, L., Xu, H., Wu, F., and Sheng, L. (2020). A Novel Lignin Degradation Bacteria-Bacillus Amyloliquefaciens SL-7 Used to Degrade Straw Lignin Efficiently. Bioresour. Techn. 310, 123445. doi:10.1016/j.biortech.2020.123445
Mishra, V., Jana, A. K., Jana, M. M., and Gupta, A. (2017). Enhancement in Multiple Lignolytic Enzymes Production for Optimized Lignin Degradation and Selectivity in Fungal Pretreatment of Sweet Sorghum Bagasse. Bioresour. Techn. 236, 49–59. doi:10.1016/j.biortech.2017.03.148
Muaaz-Us-Salam, S., Cleall, P. J., and Harbottle, M. J. (2020). Application of Enzymatic and Bacterial Biodelignification Systems for Enhanced Breakdown of Model Lignocellulosic Wastes. Sci. Total Environ. 728, 138741. doi:10.1016/j.scitotenv.2020.138741
Navas, L. E., Martínez, F. D., Taverna, M. E., Fetherolf, M. M., Eltis, L. D., Nicolau, V., et al. (2019). A Thermostable Laccase from Thermus Sp. 2.9 and its Potential for Delignification of Eucalyptus Biomass. AMB Expr. 9, 24. doi:10.1186/s13568-019-0748-y
Niu, J., Li, X., Qi, X., and Ren, Y. (2021). Pathway Analysis of the Biodegradation of Lignin by Brevibacillus Thermoruber. Bioresour. Techn. 341, 125875. doi:10.1016/j.biortech.2021.125875
Picart, P., Wiermans, L., Pérez-Sánchez, M., Grande, P. M., Schallmey, A., and Domínguez de María, P. (2016). Assessing Lignin Types to Screen Novel Biomass-Degrading Microbial Strains: Synthetic Lignin as Useful Carbon Source. ACS Sustain. Chem. Eng. 4, 651–655. doi:10.1021/acssuschemeng.5b00961
Sun, Y.-C., Liu, X.-N., Wang, T.-T., Xue, B.-L., and Sun, R.-C. (2019). Green Process for Extraction of Lignin by the Microwave-Assisted Ionic Liquid Approach: Toward Biomass Biorefinery and Lignin Characterization. ACS Sustain. Chem. Eng. 7 (15), 13062–13072. doi:10.1021/acssuschemeng.9b02166
Shah, T. A., Ali, S., Afzal, A., and Tabassum, R. (2018). Simultaneous Pretreatment and Biohydrogen Production from Wheat Straw by Newly Isolated Ligninolytic Bacillus Sp. Strains with Two-Stage Batch Fermentation System. Bioenerg. Res. 11 (4), 835–849. doi:10.1007/s12155-018-9936-x
Tonin, F., Vignali, E., Pollegioni, L., D’Arrigo, P., and Rosini, E. (2017). A Novel, Simple Screening Method for Investigating the Properties of Lignin Oxidative Activity. Enzyme Microb. Techn. 96, 143–150. doi:10.1016/j.enzmictec.2016.10.013
Wang, Y., Liu, Q., Yan, L., Gao, Y., Wang, Y., and Wang, W. (2013). A Novel Lignin Degradation Bacterial Consortium for Efficient Pulping. Bioresour. Techn. 139, 113–119. doi:10.1016/j.biortech.2013.04.033
Wang, S., Ru, B., Lin, H., Sun, W., and Luo, Z. (2015). Pyrolysis Behaviors of Four Lignin Polymers Isolated from the Same Pine Wood. Bioresour. Techn. 182, 120–127. doi:10.1016/j.biortech.2015.01.127
Weng, C., Peng, X., and Han, Y. (2021). Depolymerization and Conversion of Lignin to Value-Added Bioproducts by Microbial and Enzymatic Catalysis. Biotechnol. Biofuels 14 (1), 84. doi:10.1186/s13068-021-01934-w
Wong, D. W. S. (2009). Structure and Action Mechanism of Ligninolytic Enzymes. Appl. Biochem. Biotechnol. 157, 174–209. doi:10.1007/s12010-008-8279-z
Xu, H., Guo, M.-Y., Gao, Y.-H., Bai, X.-H., and Zhou, X.-W. (2017). Expression and Characteristics of Manganese Peroxidase from Ganoderma Lucidum in Pichia pastoris and its Application in the Degradation of Four Dyes and Phenol. BMC Biotechnol. 17, 19. doi:10.1186/s12896-017-0338-5
Xu, Z., Qin, L., Cai, M., Hua, W., and Jin, M. (2018a). Biodegradation of Kraft Lignin by Newly Isolated Klebsiella pneumoniae, Pseudomonas Putida, and Ochrobactrum Tritici Strains. Environ. Sci. Pollut. Res. 25 (2), 14171–14181. doi:10.1007/s11356-018-1633-y
Xu, R., Zhang, K., Liu, P., Han, H., Zhao, S., Kakade, A., et al. (2018b). Lignin Depolymerization and Utilization by Bacteria. Bioresour. Techn. 269 (11), 557–566. doi:10.1016/j.biortech.2018.08.118
Xu, K.-Z., Wang, H.-R., Wang, Y.-J., Xia, J., Ma, H., Cai, Y.-J., et al. (2020). Enhancement in Catalytic Activity of Cota-Laccase from bacillus Pumilus W3 via Site-Directed Mutagenesis. J. Biosci. Bioeng. 129 (4), 405–411. doi:10.1016/j.jbiosc.2019.09.020
Yang, Y. S., Zhou, J. T., Lu, H., Yuan, Y. L., and Zhao, L. H. (2011). Isolation and Characterization of a Fungus Aspergillus Sp. Strain F-3 Capable of Degrading Alkali Lignin. Biodegradation 22, 1017–1027. doi:10.1007/s10532-011-9460-6
Yang, G., Yang, D., Wang, X., and Cao, W. (2021). A Novel Thermostable Cellulase-Producing Bacillus Licheniformis A5 Acts Synergistically with Bacillus Subtilis B2 to Improve Degradation of Chinese Distillers' Grains. Bioresour. Techn. 325, 9124729–9124810. doi:10.1016/j.biortech.2021.124729
Zhou, H., Guo, W., Xu, B., Teng, Z., Tao, D., Lou, Y., et al. (2017). Screening and Identification of Lignin-Degrading Bacteria in Termite Gut and the Construction of Lip-Expressing Recombinant Lactococcus Lactis. Microb. Pathogenesis 112, 63–69. doi:10.1016/j.micpath.2017.09.047
Zhu, D., Zhang, P., Xie, C., Zhang, W., Sun, J., Qian, W.-J., et al. (2017). Biodegradation of Alkaline Lignin by Bacillus Ligniniphilus L1. Biotechnol. Biofuels 10 (1), 1–14. doi:10.1186/s13068-017-0735-y
Keywords: Bacillus sp., alkali lignin, biodegradation, isolation, enzyme
Citation: Yang J, Zhao J, Jiang J, Xu H, Zhang N, Xie J and Wei M (2021) Isolation and Characterization of Bacillus Sp. Capable of Degradating Alkali Lignin. Front. Energy Res. 9:807286. doi: 10.3389/fenrg.2021.807286
Received: 02 November 2021; Accepted: 15 November 2021;
Published: 17 December 2021.
Edited by:
Jia-Long Wen, Beijing Forestry University, ChinaCopyright © 2021 Yang, Zhao, Jiang, Xu, Zhang, Xie and Wei. This is an open-access article distributed under the terms of the Creative Commons Attribution License (CC BY). The use, distribution or reproduction in other forums is permitted, provided the original author(s) and the copyright owner(s) are credited and that the original publication in this journal is cited, in accordance with accepted academic practice. No use, distribution or reproduction is permitted which does not comply with these terms.
*Correspondence: Jianchun Jiang, YWV6aW94QDE2My5jb20=; Jingcong Xie, eGllamluZ2NvbmdAaWNpZnAuY24=