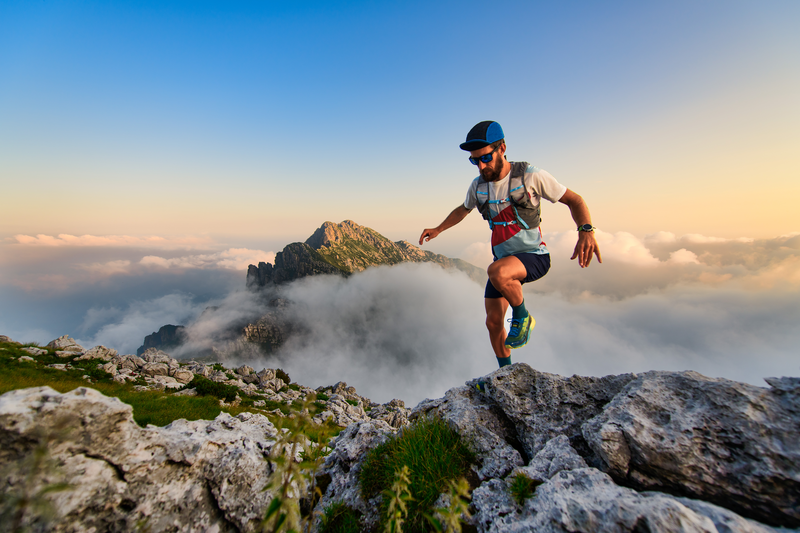
94% of researchers rate our articles as excellent or good
Learn more about the work of our research integrity team to safeguard the quality of each article we publish.
Find out more
REVIEW article
Front. Endocrinol. , 13 January 2025
Sec. Thyroid Endocrinology
Volume 15 - 2024 | https://doi.org/10.3389/fendo.2024.1469268
Thyroid-associated ophthalmopathy (TAO), an autoimmune disease closely related to thyroid dysfunction, remains a challenging ophthalmic condition among adults. Its clinical manifestations are complex and diverse, and disease progression can lead to exophthalmos, diplopia, exposure keratitis, corneal ulceration, and compressive optic neuropathy, resulting in irreversible vision damage or even blindness. Traditional treatment methods for TAO, including glucocorticoids, immunosuppressants, and radiation therapy, often have limitations and side effects, making this disease problematic in ophthalmology. As a result, the development of novel targeted drugs has become a research hotspot for addressing the pathogenesis of TAO. A range of novel targeted drugs, such as teprotumumab and tocilizumab, have been successfully developed and demonstrated remarkable efficacy in relieving inflammation and managing this disease. In addition, some drug candidates and molecular targets identified in the TAO in vitro model have shown promising prospects. This article briefly reviews the potential new strategies for future clinical treatment and the progress of new drug therapies for TAO.
Thyroid-associated ophthalmopathy (TAO), also named Graves’ orbitopathy (GO) or Thyroid-eye disease (TED), is one of the most common orbital diseases in adults and occurs in approximately 20.1% of thyroid dysfunction patients (1). TAO is more frequent in women than men, approximately 4.9: 1 (2). This autoimmune disease is closely associated with thyroid disorders and involves various intraorbital tissues, such as the eyelid, extraocular muscle, and orbital adipose tissue. It is related to genetic factors, stress, radioactive iodine treatment, smoking, and other factors (3, 4). In the early stages of TAO, patients typically present with eyelid retraction, congestion, and edema of the eyelid and conjunctival tissues. Disease progression can lead to exophthalmos, ocular motility disorders, and diplopia. In later stages, exposed keratitis, corneal ulceration, and dysthyroid optic neuropathy (DON) result in irreversible vision loss or even blindness. Currently, we have some specifically designed targeted therapies for TAO, such as teprotumumab. However, these targeted drugs have not yet been widely used clinically. TAO remains a challenging problem in ophthalmology.
The pathogenesis of TAO remains incompletely understood. Current theories suggest that it is a complex organ-specific autoimmune disease arising from immune dysfunction triggered by antigenic stimulation, environmental factors, and genetics. These factors lead to an immune tolerance imbalance, activating autoimmune T cells (including Treg cells, CD8+ cytotoxic T cells, CD4+ T cells, natural killer T cells, Th1 cells, Th2 cells, and Th17 cells) and B cells (including Breg cells), which initiate a cascade of reactions (5–10). In patients with active inflammation, thyroid-stimulating hormone receptor (TSHR) expression in orbital tissues is significantly greater than in patients with quiescent inflammation (11). Additionally, insulin-like growth factor-1 receptor (IGF-1R) is overexpressed in the orbital fibroblast (OF) and lymphocyte of patients with Graves’ ophthalmopathy (12). TSHR and IGF-1R can form functional complexes that mediate downstream signaling via TSHR (13–15), suggesting that they may act as “co-culprits” in the pathogenesis of TAO (16).
OF plays a crucial role as both target and effector cells in the development of TAO (17, 18). OF interacts with infiltrating lymphocytes, monocytes, dendritic cells/macrophages, and mast cells in the orbit, and secrets various chemokines and cytokines, such as IL-1β, IL-2, IL-6, CXCL8, IL-10, and IL-17A (19); IL-35, IL-21, COX2, CCL2, CCL5, and TGF-β (20, 21); and IFN-γ and TNF-α (22). These cytokines amplify inflammation and autoimmune responses, stimulating OF to synthesize and secrete prostaglandin E2 (PGE2). PGE2 modifies B cell behavior, affects their phenotypic switch, and regulates mast cell activation. Furthermore, OFs secrete various glycosaminoglycans, particularly hyaluronic acid (HA), which forms high-molecular-weight polysaccharides with hydrophilic groups that promote orbital soft tissue edema. This leads to increased orbital pressure, exophthalmos, and swelling of the extraocular muscles. The increase in the number of orbital adipocytes, the expansion of soft tissues, and the secretion of adipokines and growth factors by adipose tissue may also contribute to the pathogenesis of TAO (23, 24) (Figure 1).
TAO treatment focuses on reducing orbital inflammation, alleviating exophthalmos, improving appearance, inhibiting the fibrosis of extraocular muscles, orbital fat, and periorbital soft tissues, and preserving visual function. Currently, the clinical management of TAO mainly involves nonspecific glucocorticoids (GCs) therapy, radiotherapy, specific targeted therapies (anti-IGF1R, anti-CD20) and surgical intervention. Current research hotspots include expanding the understanding of TAO pathogenesis, identifying relevant targets, and developing novel pharmacologic treatments. Herein, we comprehensively summarize the potential target strategies for the progress of new drug therapies and future treatment for TAO.
TAO currently includes clinical use of drugs such as immunosuppressants, somatostatin analogs, and monoclonal antibody-targeted biologics, most of which have shown gratifying benefits. Ongoing clinical trials evaluate these drugs’ efficacy and safety, which may soon become integral components of TAO management (Table 1).
Mycophenolate mofetil (MMF) competitively and reversibly inhibits inosine monophosphate dehydrogenase, reducing antibody production by B cells and having dual antiproliferative effects on B and T cells (39). Combined with GCs, MMF has been shown to enhance the treatment response rate among patients with active moderate-to-severe TAO (40, 41). A four-year retrospective cohort study confirmed that MMF is an effective and safe immunosuppressant for treating TAO (42). The clinical efficacy of active moderate-severe TAO patients was 100% (8/8) in non-DON group and 90% (9/10) in DON group at 24 weeks. In a clinical study involving 60 patients with active moderate-to-severe TAO, the efficacy of the MMF combined with GCs pulse therapy group (73.3% and 83.3%) at 12 weeks and 24 weeks, respectively, was significantly higher than that of the GCs pulse therapy alone group (46.7% and 60.0%) (p < 0.05) (43). In another comparative study involving 242 cases of TAO, the combined oral administration of MMF (one 500 mg tablet twice per day) and prednisolone (5 mg per day) was found to be highly beneficial in alleviating exophthalmos and diplopia (44). The latest EUGOGO guidelines also recommend the combination of MMF (0.72 g daily) and methylprednisolone as a first-line treatment for active moderate-to-severe TAO (25). Combined therapy is expected to provide greater benefits to patients.
Cyclosporin reduces T cell secretion of IL-2 by inhibiting the calcineurin pathway. Research suggests that combining it with GCs may be an effective method (56% improvement) for treating moderate to severe TAO, while cyclosporin monotherapy is not superior to GCs (22% vs 61%) (26, 45). Azathioprine is used alone to treat TAO, but its effectiveness is unclear. It may have potential benefits in reducing the recurrence of TAO after the cessation of steroids (27, 46). Methotrexate is an anti-folate antimetabolite that exerts immunosuppressive effects by interfering with the DNA/RNA synthesis of proliferating cells. It can improve periorbital and conjunctival edema, relieve exophthalmos, and reduce intraocular pressure in patients who are unresponsive to GCs (47, 48). Study showed that reduced GCs plus methotrexate therapy is effective and safer in treating active and moderate-to-severe TAO patients than 4.5 g GCs monotherapy (28). These investigations suggest that monotherapy utilizing immunosuppressants for the treatment of TAO has not demonstrated superior efficacy. When used in combination with GCs, they could provide good clinical benefits while reducing the side effects of GCs pulse therapy. Combination therapy represents a viable treatment option that merits consideration within clinical practice, albeit its long-term benefits necessitate further corroboration through extensive and rigorous clinical research endeavors.
Somatostatin is an endogenous cyclic peptide with widespread inhibitory effects on various systems (49). The rationale for using somatostatin analogs for treating TAO is based on their ability to inhibit lymphocyte activation, proliferation, and cytokine production (50, 51). Octreotide and lanreotide are considered first-generation synthetic somatostatin analogs. In a double-masked randomized controlled trial (RCT), octreotide did not demonstrate significant efficacy in patients (n=50) with moderate-to-severe TAO (p=0.043) (52). Chang TC et al. found that compared to placebo, lanreotide only alleviated diplopia when looking downward (p=0.03) and that lanreotide treatment did not significantly impact clinical activity scores (CAS) or proportion requiring orbital surgery (p=0.29) (29). In contrast, compared with placebo, octreotide has been shown to significantly reduce exophthalmos (p=0.027) at the end of the 4-month treatment period (30). Another double-blind RCT revealed that patients (n=25) treated with octreotide had more significant decreases in CAS (p= 0.02) and a significantly improved palpebral fissure height (decreased 1 mm on the right and 0.5 mm on the left, p<0.01) than the placebo group (53). The differences in conclusions between the studies may be attributed to variations in patient inclusion criteria, variations in oral dosage, as well as the small sample and short follow-up duration, leading to biases. Therefore, the clinical application of somatostatin analogs remains an area of ongoing exploration.
Research has indicated that IGF-1R is overexpressed in patients with TAO OFs and lymphocytes (12). Teprotumumab, a monoclonal antibody, binds to IGF-1R with high affinity and competitively inhibits its interaction with endogenous ligands (IGF-1 and IGF-2) (54, 55), demonstrating significant therapeutic potential. The development of this drug represents a milestone in the treatment history of TAO. Two multicenter, randomized, double-blind clinical trials (phase 2 and phase 3) (31, 56) evaluated the efficacy of intravenous teprotumumab compared to placebo (administered every 3 weeks for 8 times; the infusion dose was 10 mg/kg for the first time, followed by 20 mg/kg for subsequent times) in approximately 170 newly diagnosed active TAO patients. The results showed that compared with placebo, teprotumumab had better results with proptosis response (83% vs 10%), CAS of 0 or 1 (59% vs 21%), diplopia response (68% vs 29%), and TAO quality-of-life overall score (13.79 vs 4.43), and these results were maintained in most patients in the long term (p<0.001 for all). Severe adverse events were rare; the typical adverse events after teprotumumab treatment included muscle spasms (25%), nausea (17%), hair loss (13%), diarrhea (13%), fatigue (10%), hearing impairment (10%), and hyperglycemia (8%). Teprotumumab is contraindicated in patients with inflammatory bowel disease and pregnant women (57). The OPTIC-X study showed that TAO patients who did not respond to teprotumumab or whose disease worsened in the first course also benefited from teprotumumab treatment (58). More patients receiving teprotumumab achieved a reduction of at least 2 mm in proptosis at week 24 versus placebo (65 [77%] of 84 patients assigned teprotumumab vs 13 [15%] assigned placebo, p<0·0001) (59). Furthermore, compared to 20 patients in the placebo group, 42 patients in the group receiving teprotumumab exhibited significant improvement in ocular protrusion (-2.41 vs -1.48, p=0.0004) among long-term low-inflammation TAO patients regardless of disease duration/activity (60). Following treatment with teprotumumab, the lacrimal gland volume of TAO patients decreased significantly (768 mm3 to 486 mm3, p < 0.01), while dry eye symptoms decreased markedly (61). Although current dosing regimens have proven effective for treating TAO, further comprehensive research is warranted, including dose-ranging studies involving variable concentrations, infusion frequencies, and maintenance therapy durations.
Rituximab is a monoclonal antibody that targets CD20, which is only expressed by B cells (from pre-B cells to mature memory B cells). It is the first biological therapy applied to treat TAO (62). Mario Salvi et al. (63) reported using rituximab in 43 active GO patients, with 39 patients (91%) showing a tendency toward stability, 3 showing no change, and 1 showing disease progression. Subsequently, a double-blind RCT (64) compared the effects of rituximab and GC shock therapy in active moderate-to-severe patients, with the results showing that 100% of patients in the rituximab group experienced symptom alleviation at 24 weeks and that 38%-62% of patients experienced improvement in quality of life. However, another RCT conducted the same year (65) showed no additional benefit in active moderate-to-severe TAO patients using rituximab compared to those using placebo, accompanied by significant adverse reactions. In a study of rituximab treatment in 17 active moderate-to-severe GO patients, a low-dose (100 mg) single injection was effective (CAS score decreased from 4.56 ± 0.96 to 1.25 ± 1.14 at 24 weeks, p=0.001) (66). In a study of rituximab treatment for active moderate-to-severe TAO with different dosage groups (single dose of 100 mg, single dose of 500 mg, and 1000 mg given in two doses one week apart), considering factors such as patient activity remission, impact on quality of life, and treatment cost, it is recommended that most patients use a single dose of 500 mg (32). Rituximab has shown preliminary efficacy in the clinical treatment of TAO, but some studies report no significant benefits. This may be related to small sample sizes, varying inclusion and exclusion criteria, and differences in population types. Thus, further clarification of the role of rituximab in TAO treatment through long-term, large-sample, multicenter clinical trials is still needed.
Belimumab is a monoclonal antibody targeting B cell stimulating factor (BAFF). Like rituximab, belimumab targets naive and transitional B cells and is currently approved for treating systemic lupus erythematosus (67). Given the reported elevation of serum BAFF concentrations in Graves’ disease patients (68, 69) and the expression of BAFF on thyroid cells in patients with autoimmune diseases or a nodular goiter (70, 71), belimumab potentially exhibits beneficial effects in reducing disease activity in Graves’ disease and TAO. A clinical randomized controlled trial is underway in Italy (72), and the outcomes remain to be evaluated.
Tocilizumab is a recombinant human monoclonal antibody targeting the interleukin-6 (IL-6) receptor (73). IL-6 is a pleiotropic cytokine secreted by various cell types, including T cells, macrophages, fibroblasts, osteoblasts, and endothelial cells. A preliminary study showed that intravenous tocilizumab reduced disease activity in patients unresponsive to GC therapy (mean CAS score reduction 5.89 ± 1.41 points, p< 0.00027) (74). A subsequent double-blind RCT demonstrated a significant decrease in disease activity (CAS score < 3, 86.7% vs 35.2%, p =0.005) and severity in GC-resistant patients treated with tocilizumab compared to those treated with placebo (33). Two longitudinal studies (75, 76) also indicated that at least 4 months of tocilizumab treatment (one dose per month) provided significant benefits to patients with moderate-to-severe GC-resistant TAO. In an observational study of 12 patients with moderate-to-severe GC-resistant TAO, intravenous (tocilizumab 8 mg/kg, once every 28 days for 4 doses) reduced disease activity, with varying degrees of relief of proptosis and diplopia (Hertel score reduced by 2.3 mm on the right eye[p=0.003], 1.6 mm on the left eye [p=0.002]) (77). Tocilizumab appears to be an effective and safe treatment option for refractory TAO, providing a new alternative for patients with GC contraindications or resistance.
Batoclimab, a neonatal fragment crystallizable receptor (FcRn) inhibitor, was evaluated in a double-masked RCT involving 77 patients with moderate-to-severe TAO (34). Subjects received weekly subcutaneous injections of batoclimab (680 mg, 340 mg, or 255 mg) or placebo. At 12 weeks, the batoclimab (680 mg) group showed a significant reduction in TSH-R-Ab levels, and participants tolerated the drug well throughout the study. Furthermore, phase 3 randomized placebo-controlled 24-week trials (NCT. 05524571 and NCT. 05517421) and a 24-week open-label extension (NCT. 05517447) have been designed, and more relevant clinical data are anticipated.
Key tumor necrosis factor α (TNF-α) inhibitors include etanercept (a recombinant human soluble TNF-α receptor fusion protein), adalimumab (a human monoclonal antibody targeting TNF-α), and infliximab (a monoclonal antibody against TNF-α). A preliminary study demonstrated that the subcutaneous etanercept injection improved ocular symptoms in 10 patients with active TAO without serious adverse events (CAS score reduced from 4 to 1.6 at 12 weeks, and the mean ophthalmopathy index reduced from 5.8 to 4.4) (35). Another case report described a female patient with concurrent TAO and rheumatoid arthritis who received etanercept for rheumatoid arthritis treatment, resulting in improved ocular symptoms and reduced exophthalmos (78). A retrospective study suggested that subcutaneous adalimumab might be an effective treatment for patients with active disease and significant inflammation (36), but prospective RCTs are needed to confirm its efficacy. Infliximab has also shown therapeutic effects in case reports of TAO, with a significant reduction in ocular inflammation, improvements in visual function, and no apparent short-term side effects (37, 79). However, large-scale clinical RCTs are still needed to assess the efficacy and safety of anti-TNF-α treatment.
Statins are commonly used as lipid-regulating agents in clinical practice. Previous studies have suggested that statin therapy may be associated with a reduced risk of TAO development in Graves’ disease patients (80). A Swedish study (81) statistically analyzed 34,894 patients newly diagnosed with Graves’ disease and revealed that statin users had a significantly lower likelihood of developing TAO, indicating a potential preventive effect on TAO progression. There is a significant correlation between the occurrence of TAO and total cholesterol and low-density lipoprotein cholesterol levels, suggesting that statin treatment may benefit patients with TAO and provide a new therapeutic direction (82). A phase 2 clinical trial in Italy showed that adding oral atorvastatin to intravenous GC pulse therapy improved TAO outcomes in patients with moderate-to-severe active ophthalmopathy and hypercholesterolemia (p=0·042) (38). This may be due to the ability of stains to shift the primary proinflammatory T-cell response to an anti-inflammatory response by activating TH2 and regulatory T cells, thereby alleviating clinical symptoms. Future phase 3 studies are required to confirm this association.
With continuous exploration by researchers, it has been confirmed that some commonly used clinical drugs and traditional Chinese medicine components have therapeutic effects, such as inhibiting adipogenesis, anti-inflammatory, and anti-fibrotic effects, in the TAO in vitro model. The following section will briefly discuss the new perspectives of old drugs, as well as emerging molecular targets in TAO.
Preventing orbital tissue inflammation and adipose tissue hyperplasia during the early stages of the disease is critical for addressing exophthalmos. Previous studies have reported that several clinically used drugs exhibit therapeutic potential for inhibiting adipogenesis and inflammation in in vitro models of TAO. Diclofenac suppresses COX-2 to inhibit mature adipocyte formation in OFs (83). Salazosulfamide inhibits adipogenesis by downregulating PPARγ expression in OFs (84). Idelalisib, a treatment for lymphocytic leukemia, inhibits lipid droplet formation and decreases the expression of PPARγ and c/EBPα/β in OFs (85). Simvastatin inhibits the expression of adipogenesis-related genes in preadipocytes and OFs stimulated with cigarette smoke extract (86). Metformin, a first-line treatment for type 2 diabetes, inhibits adipogenesis and inflammation in in vitro models of TAO by activating AMPK phosphorylation and suppressing ERK phosphorylation signaling (87). Nintedanib, a treatment for idiopathic pulmonary fibrosis, inhibits fibroblast growth factor-induced adipogenesis in OFs from patients with TAO (88). Disulfiram, a drug used to treat alcohol aversion, significantly inhibits the differentiation of OFs from TAO patients into mature adipocytes, inhibiting ERK phosphorylation by partially inhibiting ALDH1A1 (89). Linsitinib, a novel and highly selective dual inhibitor of IGF-1R and insulin receptors, may reduce local inflammatory responses by promoting Treg differentiation and/or activation and reducing TNF-α levels in a mouse model of TAO (90). Intriguingly, caffeine has also been found to reduce oxidative stress and inhibit adipogenesis in an in vitro model of TAO (91).
Studies have shown elevated levels of platelet-derived growth factor (PDGF)-AA, AB, and BB in the orbital connective tissue of TAO patients, with OFs expressing PDGF receptors (92, 93). PDGFs can induce the proliferation of OFs, promote adipogenesis (94), promote the secretion of hyaluronic acid and IL-6 (95), and increase the expression level of surface TSHR (96). Tyrosine kinase inhibitors, such as imatinib, dasatinib, and nilotinib, may be candidates for targeting PDGF signaling (97). The serum IL-17 concentration and positive detection rate are significantly greater in TAO patients than in healthy controls, and the serum IL-17 concentration is significantly associated with CAS (p < 0.001) (98). The proportion of T cells that secrete IL-17A is significantly greater in TAO patients than in healthy subjects (19, 99). Various IL-17A monoclonal antibodies, including secukinumab (100), ixekizumab (101), and bimekizumab (102), have been successfully developed. They are expected to serve as targets in future treatment approaches.
Traditional Chinese medicines (TCMs) have also been found to inhibit adipogenesis. Quercetin is used as an adjunctive treatment for chronic bronchitis and coronary heart disease, and it inhibits the differentiation of OFs into mature adipocytes by reducing oxidative stress (103). Tanshinone II inhibits adipogenesis in OF in a dose-dependent manner, reducing oil red O staining and decreasing the expression of PPARγ and C/EBPα (104). Icariin, a flavonoid, affects the differentiation of TAO preadipocytes into mature adipocytes by inhibiting AMPK/mTOR-mediated autophagy, reducing orbital adipose tissue expansion and lipid droplet accumulation (105). Chloroquine/hydroxychloroquine, an antimalarial drug, affects the proliferation, adipogenesis, and hyaluronic acid production of TAO OFs by inhibiting autophagy (106). Liensinine, an alkaloid extracted from lotus seed embryos, inhibits IL-13-induced autophagosome formation and the overexpression of autophagy markers, increases the LC3-II/LC3-I ratio, and ultimately downregulates p62 expression in TAO OFs, thereby reducing inflammation and adipogenesis (107). Berberine, a significant component of Coptis chinensis, inhibits lipid droplet formation in OFs and reduces IL-1β-induced inflammation (108).
Suppressing the fibrosis of extraocular muscles and periorbital soft tissues is another urgent clinical issue. Pirfenidone, a treatment for idiopathic pulmonary fibrosis, inhibits IL-1β-induced increases in TIMP-1 and hydroxyproline levels in an in vitro model of TAO, exerting antifibrotic effects (109). Pirfenidone has also been found to regulate TGF-β1-mediated OF differentiation into myofibroblasts and ECM homeostasis (110). Simvastatin has been found to inhibit TGF-β-mediated α-SMA expression in an in vitro model of TAO (111). Metformin exerts anti-inflammatory and antifibrotic effects on OFs by activating autophagy and the AMPK/mTOR signaling pathway (112). Relaxin, a recombinant form of the human hormone relaxin-2, alleviates TGF-β1-induced α-SMA, COL1A1, FN1, and TIMP1 expression in OFs through the Notch pathway, exerting antifibrotic effects (113).
Certain TCMs have also been found to have antifibrotic effects. Curcumin inhibits the TGF-β1 signaling pathway and attenuates TGF-β1-induced CTGF and α-SMA expression (114). Quercetin has been shown to inhibit TGF-β-stimulated FN1 and collagen Iα expression and suppresses MMP2 and MMP9 activity in an in vitro model of TAO, suggesting potential therapeutic and preventive uses for chronic fibrosis (115). Dihydroartemisinin may exert antifibrotic effects on OFs through the MAPK/ERK and STAT3 signaling pathways (116). Lutein reduces TGF-β1-induced α-SMA and COL1A1 expression by inhibiting the ERK/AP-1 pathway (117). Crocin suppresses TGF-β1-induced OF proliferation and migration, exerting antifibrotic effects by inhibiting the ERK and STAT3 signaling pathways (118).
These encouraging findings broaden our clinical therapeutic approaches. The safety of commonly used Western medicine and TCMs active ingredients has been widely validated in clinical practice. However, most of these studies involved in vitro experiments; therefore, whether these drugs can also inhibit adipogenesis and reduce tissue inflammation and fibrosis in TAO animal models remains unverified. Furthermore, there is a lack of an in-depth understanding of drug interactions with systemic conditions (thyroid function indicators, liver and kidney function) and potential systemic adverse effects during TAO treatment. Therefore, there is still a long way to go before the clinical application of TAO therapy. Next, we will outline several new drug treatments and their recent advancements in clinical settings.
With further research, the discovery of emerging molecular targets has revolutionized therapeutic strategies for TAO. Precisely targeted molecules are expected to reduce therapeutic side effects, enhance treatment efficacy, and offer new hope for patients. Human placenta-derived mesenchymal stem cells were shown to inhibit lipid accumulation and ameliorate adipogenesis in the orbital tissues of TAO model mice (119). Fingolimod (a sphingosine-1-phosphate inhibitor) improves the outcome of experimental Graves’ disease and TAO by modulating the autoimmune response to TSHR (120). IL-38 has been shown to exert anti-inflammatory and antifibrotic effects in in vitro models of TAO (121). IL-11 promotes OF fibrosis by increasing the phosphorylation of ERK and STAT3 and upregulating the expression of the fibrosis-related proteins α-SMA and COL1A1 (122). TGF-β has been shown to enhance YAP expression, and YAP silencing or inhibition with cerivastatin, verteporfin, TED-347, and CA3 has been shown to significantly reduce myofibroblast differentiation and collagen formation in TGF-β-induced TAO (123). The expression of BRD4, a member of the bromodomain and extra terminal family, is upregulated in the orbital tissues of TAO patients and in TGF-β1-stimulated TAO OFs. BDR4 may regulate the fibrotic process in the OFs of TAO patients through the FoxM1/Plk1 axis, and targeting the BD2 domain of BRD4 may exert antifibrotic effects (124). TMEM2 has been shown to suppress inflammatory cytokine production, ROS production, lipid droplet formation, and adipogenic factor expression in OFs in TAO mouse models (125). GSDMD-mediated OF cell pyroptosis induces TAO inflammation through the NF-κB/AIM2/caspase-1 pathway (126).
Numerous challenges remain. First, the functions and mechanisms of these targets and small-molecule compounds may not be fully elucidated in laboratory settings, adding uncertainty to subsequent drug development. Second, experimental targets may lack sufficient clinical data, making it difficult to predict their efficacy and safety in humans. Additionally, potential issues such as stability, specificity, and interactions with other biomolecules require further investigation and validation of new targets. Therefore, despite promising new targets for TAO treatment, additional research and overcoming these limitations are necessary. With technological advancements, we anticipate the discovery of more targets and the elucidation of their mechanisms of action, leading to more precise and effective TAO treatment options (Figure 2).
As research on TAO has progressed, the limitations of traditional treatments have spurred the emergence of novel therapeutic approaches, with various new drugs gradually entering clinical trials. Currently, some medications can alleviate the activity and severity of the disease, but there are still few prospective, multicenter, randomized controlled clinical studies. Further research is needed to investigate the effectiveness, safety, optimal dosage, and administration methods of these drugs. The clinical application of these drugs should be guided by specific indications personalized according to the disease grade and activity of each patient. However, the exact mechanisms of these drugs and the risk of complications during treatment remain unclear, thus limiting their clinical use.
Given the complexity and individual differences in the pathogenesis of TAO, personalized treatment plans are crucial. MMF combined with GCs pulse therapy and intravenous teprotumumab can be considered effective methods for current drug therapy. Early intervention can significantly impact the disease course, improve long-term prognosis, and reduce the incidence of severe complications. It is imperative to understand the pathogenesis of TAO further, identify relevant targets, and develop new treatment strategies. Multitarget combinations, new administration methods, and traditional Chinese medicine components may offer promising avenues for improving drug efficacy and reducing risks. However, high-quality RCTs and the elucidation of the exact underlying mechanisms are still needed. There is an urgent need to develop eye drops, ophthalmic gels, and sustained-release formulations for periorbital injection incorporating contemporary polymer-based medical materials.
In conclusion, drug therapy for TAO is evolving from new perspectives. We eagerly await the clinical application of more effective, safer, longer-lasting, and more straightforward drugs or treatment modalities, aiming to maximize the long-term prognosis and quality of life of patients with TAO.
ZL: Conceptualization, Investigation, Supervision, Writing – original draft, Writing – review & editing.
The author(s) declare that no financial support was received for the research, authorship, and/or publication of this article.
I would like to thank all my colleagues who supported my study.
The author declares that the research was conducted in the absence of any commercial or financial relationships that could be construed as a potential conflict of interest.
All claims expressed in this article are solely those of the authors and do not necessarily represent those of their affiliated organizations, or those of the publisher, the editors and the reviewers. Any product that may be evaluated in this article, or claim that may be made by its manufacturer, is not guaranteed or endorsed by the publisher.
1. Bartalena L, Piantanida E, Gallo D, Lai A, Tanda ML. Epidemiology, natural history, risk factors, and prevention of graves’ Orbitopathy. Front Endocrinol (Lausanne). (2020) 11:615993. doi: 10.3389/fendo.2020.615993
2. Laurberg P, Berman DC, Bulow Pedersen I, Andersen S, Carle A. Incidence and clinical presentation of moderate to severe graves’ orbitopathy in a Danish population before and after iodine fortification of salt. J Clin Endocrinol Metab. (2012) 97:2325–32. doi: 10.1210/jc.2012-1275
4. Douglas RS, Gupta S. The pathophysiology of thyroid eye disease: implications for immunotherapy. Curr Opin Ophthalmol. (2011) 22:385–90. doi: 10.1097/ICU.0b013e3283499446
5. de Carli M, D’Elios MM, Mariotti S, Marcocci C, Pinchera A, Ricci M, et al. Cytolytic T cells with Th1-like cytokine profile predominate in retroorbital lymphocytic infiltrates of Graves’ ophthalmopathy. J Clin Endocrinol Metab. (1993) 77:1120–4. doi: 10.1210/jcem.77.5.8077301
6. Gerding MN, van der Meer JW, Broenink M, Bakker O, Wiersinga WM, Prummel MF. Association of thyrotrophin receptor antibodies with the clinical features of Graves’ ophthalmopathy. Clin endocrinology. (2000) 52:267–71. doi: 10.1046/j.1365-2265.2000.00959.x
7. Marique L, Senou M, Craps J, Delaigle A, Van Regemorter E, Wérion A, et al. Oxidative stress and upregulation of antioxidant proteins, including adiponectin, in extraocular muscular cells, orbital adipocytes, and thyrocytes in graves’ Disease associated with orbitopathy. Thyroid: Off J Am Thyroid Assoc. (2015) 25:1033–42. doi: 10.1089/thy.2015.0087
8. Romero-Kusabara IL, Filho JV, Scalissi NM, Melo KC, Demartino G, Longui CA, et al. Distinct inflammatory gene expression in extraocular muscle and fat from patients with Graves’ orbitopathy. Eur J endocrinology. (2017) 176:481–8. doi: 10.1530/EJE-16-0945
9. Yu SH, Kang JG, Kim CS, Ihm SH, Choi MG, Yoo HJ, et al. Clinical implications of immunoglobulin G4 to graves’ Ophthalmopathy. Thyroid: Off J Am Thyroid Assoc. (2017) 27:1185–93. doi: 10.1089/thy.2017.0126
10. Wakelkamp IM, Bakker O, Baldeschi L, Wiersinga WM, Prummel. TSH-R expression MF. and cytokine profile in orbital tissue of active vs. inactive Graves’ ophthalmopathy patients. Clin endocrinology. (2003) 58:280–7. doi: 10.1046/j.1365-2265.2003.01708.x
11. Wakelkamp IM, Bakker O, Baldeschi L, Wiersinga WM, Prummel MF. TSH-R expression and cytokine profile in orbital tissue of active vs. inactive Graves’ ophthalmopathy patients. Clin Endocrinol (Oxf). (2003) 58:280–7. doi: 10.1046/j.1365-2265.2003.01708.x
12. Douglas RS, Naik V, Hwang CJ, Afifiyan NF, Gianoukakis AG, Sand D, et al. B cells from patients with Graves’ disease aberrantly express the IGF-1 receptor: implications for disease pathogenesis. J Immunol. (2008) 181:5768–74. doi: 10.4049/jimmunol.181.8.5768
13. Dik WA, Virakul S, van Steensel L. Current perspectives on the role of orbital fibroblasts in the pathogenesis of Graves’ ophthalmopathy. Exp eye Res. (2016) 142:83–91. doi: 10.1016/j.exer.2015.02.007
14. Krieger CC, Neumann S, Gershengorn MC. TSH/IGF1 receptor crosstalk: Mechanism and clinical implications. Pharmacol Ther. (2020) 209:107502. doi: 10.1016/j.pharmthera.2020.107502
15. Smith TJ. TSH-receptor-expressing fibrocytes and thyroid-associated ophthalmopathy. Nat Rev Endocrinol. (2015) 11:171–81. doi: 10.1038/nrendo.2014.226
16. Paik JS, Kim SE, Kim JH, Lee JY, Yang SW, Lee SB. Insulin-like growth factor-1 enhances the expression of functional TSH receptor in orbital fibroblasts from thyroid-associated ophthalmopathy. Immunobiology. (2020) 225:151902. doi: 10.1016/j.imbio.2019.151902
17. Bahn RS, Heufelder AE. Retroocular fibroblasts: important effector cells in Graves’ ophthalmopathy. Thyroid: Off J Am Thyroid Assoc. (1992) 2:89–94. doi: 10.1089/thy.1992.2.89
18. Koumas L, Smith TJ, Feldon S, Blumberg N, Phipps RP. Thy-1 expression in human fibroblast subsets defines myofibroblastic or lipofibroblastic phenotypes. Am J pathology. (2003) 163:1291–300. doi: 10.1016/S0002-9440(10)63488-8
19. Fang S, Huang Y, Wang S, Zhang Y, Luo X, Liu L, et al. IL-17A exacerbates fibrosis by promoting the proinflammatory and profibrotic function of orbital fibroblasts in TAO. J Clin Endocrinol Metab. (2016) 101:2955–65. doi: 10.1210/jc.2016-1882
20. van Steensel L, Paridaens D, Schrijver B, Dingjan GM, van Daele PL, van Hagen PM, et al. Imatinib mesylate and AMN107 inhibit PDGF-signaling in orbital fibroblasts: a potential treatment for Graves’ ophthalmopathy. Invest Ophthalmol Visual science. (2009) 50:3091–8. doi: 10.1167/iovs.08-2443
21. Tsai CC, Wu SB, Kao SC, Kau HC, Lee FL, Wei YH. The protective effect of antioxidants on orbital fibroblasts from patients with Graves’ ophthalmopathy in response to oxidative stress. Mol vision. (2013) 19:927–34.
22. Pawlowski P, Reszec J, Eckstein A, Johnson K, Grzybowski A, Chyczewski L, et al. Markers of inflammation and fibrosis in the orbital fat/connective tissue of patients with Graves’ orbitopathy: clinical implications. Mediators inflammation. (2014) 2014:412158. doi: 10.1155/2014/412158
23. Nishida Y, Tian S, Isberg B, Hayashi O, Tallstedt L, Lennerstrand G. Significance of orbital fatty tissue for exophthalmos in thyroid-associated ophthalmopathy. Graefe’s Arch Clin Exp Ophthalmol = Albrecht von Graefes Archiv fur klinische und experimentelle Ophthalmologie. (2002) 240:515–20. doi: 10.1007/s00417-002-0498-3
24. Kumar S, Coenen MJ, Scherer PE, Bahn RS. Evidence for enhanced adipogenesis in the orbits of patients with Graves’ ophthalmopathy. J Clin Endocrinol Metab. (2004) 89:930–5. doi: 10.1210/jc.2003-031427
25. Bartalena L, Kahaly GJ, Baldeschi L, Dayan CM, Eckstein A, Marcocci C, et al. The 2021 European Group on Graves’ orbitopathy (EUGOGO) clinical practice guidelines for the medical management of Graves’ orbitopathy. Eur J Endocrinol. (2021) 185:G43–67. doi: 10.1530/EJE-21-0479
26. Prummel MF, Mourits MP, Berghout A, Krenning EP, van der Gaag R, Koornneef L, et al. Prednisone and cyclosporine in the treatment of severe Graves’ ophthalmopathy. N Engl J Med. (1989) 321:1353–9. doi: 10.1056/NEJM198911163212002
27. Rajendram R, Taylor PN, Wilson VJ, Harris N, Morris OC, Tomlinson M, et al. Combined immunosuppression and radiotherapy in thyroid eye disease (CIRTED): a multicentre, 2 x 2 factorial, double-blind, randomised controlled trial. Lancet Diabetes Endocrinol. (2018) 6:299–309. doi: 10.1016/S2213-8587(18)30021-4
28. Shen L, Ye L, Zhu W, Jiao Q, Zhou Y, Wang S, et al. Methotrexate plus reduced or full-dose glucocorticoids for the treatment of active, moderate-to-severe Graves’ orbitopathy. Eur Thyroid J. (2022) 11:e220017. doi: 10.1530/ETJ-22-0017
29. Chang TC, Liao SL. Slow-release lanreotide in Graves’ ophthalmopathy: A double-blind randomized, placebo-controlled clinical trial. J Endocrinol Invest. (2006) 29:413–22. doi: 10.1007/BF03344124
30. Wemeau JL, Caron P, Beckers A, Rohmer V, Orgiazzi J, Borson-Chazot F, et al. Octreotide (long-acting release formulation) treatment in patients with graves’ orbitopathy: clinical results of a four-month, randomized, placebo-controlled, double-blind study. J Clin Endocrinol Metab. (2005) 90:841–8. doi: 10.1210/jc.2004-1334
31. Douglas RS, Kahaly GJ, Patel A, Sile S, Thompson EHZ, Perdok R, et al. Teprotumumab for the treatment of active thyroid eye disease. N Engl J Med. (2020) 382:341–52. doi: 10.1056/NEJMoa1910434
32. Campi I, Vannucchi G, Muller I, Lazzaroni E, Curro N, Dainese M, et al. Therapy with different dose regimens of rituximab in patients with active moderate-to-severe graves’ Orbitopathy. Front Endocrinol (Lausanne). (2021) 12:790246. doi: 10.3389/fendo.2021.790246
33. Perez-Moreiras JV, Gomez-Reino JJ, Maneiro JR, Perez-Pampin E, Romo Lopez A, Rodriguez Alvarez FM, et al. Efficacy of tocilizumab in patients with moderate-to-severe corticosteroid-resistant graves orbitopathy: A randomized clinical trial. Am J Ophthalmol. (2018) 195:181–90. doi: 10.1016/j.ajo.2018.07.038
34. Kahaly GJ, Dolman PJ, Wolf J, Giers BC, Elflein HM, Jain AP, et al. Proof-of-concept and randomized, placebo-controlled trials of an fcRn inhibitor, batoclimab, for thyroid eye disease. J Clin Endocrinol Metab. (2023) 108:3122–34. doi: 10.1210/clinem/dgad381
35. Paridaens D, van den Bosch WA, van der Loos TL, Krenning EP, van Hagen PM. The effect of etanercept on Graves’ ophthalmopathy: a pilot study. Eye (Lond). (2005) 19:1286–9. doi: 10.1038/sj.eye.6701768
36. Ayabe R, Rootman DB, Hwang CJ, Ben-Artzi A, Goldberg R. Adalimumab as steroid-sparing treatment of inflammatory-stage thyroid eye disease. Ophthalmic Plast Reconstr Surg. (2014) 30:415–9. doi: 10.1097/IOP.0000000000000211
37. Komorowski J, Jankiewicz-Wika J, Siejka A, Lawnicka H, Klysik A, Gos R, et al. Monoclonal anti-TNFalpha antibody (infliximab) in the treatment of patient with thyroid associated ophthalmopathy. Klin Oczna. (2007) 109:457–60.
38. Lanzolla G, Sabini E, Leo M, Menconi F, Rocchi R, Sframeli A, et al. Statins for Graves’ orbitopathy (STAGO): a phase 2, open-label, adaptive, single centre, randomised clinical trial. Lancet Diabetes Endocrinol. (2021) 9:733–42. doi: 10.1016/S2213-8587(21)00238-2
39. Allison AC. Mechanisms of action of mycophenolate mofetil. Lupus. (2005) 14 Suppl 1:s2–8. doi: 10.1191/0961203305LU2109OA
40. Ye X, Bo X, Hu X, Cui H, Lu B, Shao J, et al. Efficacy and safety of mycophenolate mofetil in patients with active moderate-to-severe Graves’ orbitopathy. Clin Endocrinol (Oxf). (2017) 86:247–55. doi: 10.1111/cen.2017.86.issue-2
41. Kahaly GJ, Riedl M, Konig J, Pitz S, Ponto K, Diana T, et al. Mycophenolate plus methylprednisolone versus methylprednisolone alone in active, moderate-to-severe Graves’ orbitopathy (MINGO): a randomised, observer-masked, multicentre trial. Lancet Diabetes Endocrinol. (2018) 6:287–98. doi: 10.1016/S2213-8587(18)30020-2
42. Quah Qin Xian N, Alnahrawy A, Akshikar R, Lee V. Real-world efficacy and safety of mycophenolate mofetil in active moderate-to-sight-threatening thyroid eye disease. Clin Ophthalmol. (2021) 15:1921–32. doi: 10.2147/OPTH.S305717
43. Li LF, Xue JL, Guan L, Su FF, Wang H, Zhang DF. Therapeutic outcomes of mycophenolate mofetil and glucocorticoid in thyroid-associated ophthalmopathy patients. Front Endocrinol (Lausanne). (2023) 14:1140196. doi: 10.3389/fendo.2023.1140196
44. Rajabi MT, Rafizadeh SM, Mohammadi A, Eshraghi B, Mohammadi N, Hosseini SS, et al. Mycophenolate mofetil (CellCept(R)) in combination with low dose prednisolone in moderate to severe graves’ Orbitopathy. Front Med (Lausanne). (2022) 9:788228. doi: 10.3389/fmed.2022.788228
45. Kahaly G, Schrezenmeir J, Krause U, Schweikert B, Meuer S, Muller W, et al. Ciclosporin and prednisone v. prednisone in treatment of Graves’ ophthalmopathy: a controlled, randomized and prospective study. Eur J Clin Invest. (1986) 16:415–22. doi: 10.1111/j.1365-2362.1986.tb01016.x
46. Perros P, Weightman DR, Crombie AL, Kendall-Taylor P. Azathioprine in the treatment of thyroid-associated ophthalmopathy. Acta Endocrinol (Copenh). (1990) 122:8–12. doi: 10.1530/acta.0.1220008
47. Sanyal P, Bing-You RG, Braverman LE. Use of methotrexate to treat isolated Graves ophthalmopathy developing years after thyroidectomy and iodine 131 treatment of papillary thyroid cancer. Endocr Pract. (2008) 14:422–5. doi: 10.4158/EP.14.4.422
48. Strianese D, Iuliano A, Ferrara M, Comune C, Baronissi I, Napolitano P, et al. Methotrexate for the treatment of thyroid eye disease. J Ophthalmol. (2014) 2014:128903. doi: 10.1155/2014/128903
49. Gunther T, Tulipano G, Dournaud P, Bousquet C, Csaba Z, Kreienkamp HJ, et al. International union of basic and clinical pharmacology. CV. Somatostatin receptors: structure, function, ligands, and new nomenclature. Pharmacol Rev. (2018) 70:763–835. doi: 10.1124/pr.117.015388
50. Pasquali D, Vassallo P, Esposito D, Bonavolonta G, Bellastella A, Sinisi AA. Somatostatin receptor gene expression and inhibitory effects of octreotide on primary cultures of orbital fibroblasts from Graves’ ophthalmopathy. J Mol Endocrinol. (2000) 25:63–71. doi: 10.1677/jme.0.0250063
51. Pasquali D, Notaro A, Bonavolonta G, Vassallo P, Bellastella A, Sinisi AA. Somatostatin receptor genes are expressed in lymphocytes from retroorbital tissues in Graves’ disease. J Clin Endocrinol Metab. (2002) 87:5125–9. doi: 10.1210/jc.2002-020790
52. Dickinson AJ, Vaidya B, Miller M, Coulthard A, Perros P, Baister E, et al. Double-blind, placebo-controlled trial of octreotide long-acting repeatable (LAR) in thyroid-associated ophthalmopathy. J Clin Endocrinol Metab. (2004) 89:5910–5. doi: 10.1210/jc.2004-0697
53. Stan MN, Garrity JA, Bradley EA, Woog JJ, Bahn MM, Brennan MD, et al. Randomized, double-blind, placebo-controlled trial of long-acting release octreotide for treatment of Graves’ ophthalmopathy. J Clin Endocrinol Metab. (2006) 91:4817–24. doi: 10.1210/jc.2006-1105
54. Smith TJ, Janssen JA. Building the case for insulin-like growth factor receptor-I involvement in thyroid-associated ophthalmopathy. Front Endocrinol (Lausanne). (2016) 7:167. doi: 10.3389/fendo.2016.00167
55. Smith TJ, Jamjl J. Insulin-like growth factor-I receptor and thyroid-associated ophthalmopathy. Endocr Rev. (2019) 40:236–67. doi: 10.1210/er.2018-00066
56. Smith TJ, Kahaly GJ, Ezra DG, Fleming JC, Dailey RA, Tang RA, et al. Teprotumumab for thyroid-associated ophthalmopathy. N Engl J Med. (2017) 376:1748–61. doi: 10.1056/NEJMoa1614949
57. Winn BJ, Kersten RC. Teprotumumab: interpreting the clinical trials in the context of thyroid eye disease pathogenesis and current therapies. Ophthalmology. (2021) 128:1627–51. doi: 10.1016/j.ophtha.2021.04.024
58. Douglas RS, Kahaly GJ, Ugradar S, Elflein H, Ponto KA, Fowler BT, et al. Teprotumumab efficacy, safety, and durability in longer-duration thyroid eye disease and re-treatment: OPTIC-X study. Ophthalmology. (2022) 129:438–49. doi: 10.1016/j.ophtha.2021.10.017
59. Kahaly GJ, Douglas RS, Holt RJ, Sile S, Smith TJ. Teprotumumab for patients with active thyroid eye disease: a pooled data analysis, subgroup analyses, and off-treatment follow-up results from two randomised, double-masked, placebo-controlled, multicentre trials. Lancet Diabetes Endocrinol. (2021) 9:360–72. doi: 10.1016/S2213-8587(21)00056-5
60. Douglas RS, Couch S, Wester ST, Fowler BT, Liu CY, Subramanian PS, et al. Efficacy and safety of teprotumumab in patients with thyroid eye disease of long duration and low disease activity. J Clin Endocrinol Metab. (2023) 109:25–35. doi: 10.1210/clinem/dgad637
61. Ugradar S, Zimmerman E, Parunakian E, Kang J, Cockerham K, Douglas RS. Change in lacrimal gland volume and aqueous tear production following treatment with teprotumumab. Clin Exp Ophthalmol. (2023) 51:339–48. doi: 10.1111/ceo.14208
62. Ristov J, Espie P, Ulrich P, Sickert D, Flandre T, Dimitrova M, et al. Characterization of the in vitro and in vivo properties of CFZ533, a blocking and non-depleting anti-CD40 monoclonal antibody. Am J Transplant. (2018) 18:2895–904. doi: 10.1111/ajt.14872
63. Salvi M, Vannucchi G, Beck-Peccoz P. Potential utility of rituximab for Graves’ orbitopathy. J Clin Endocrinol Metab. (2013) 98:4291–9. doi: 10.1210/jc.2013-1804
64. Salvi M, Vannucchi G, Curro N, Campi I, Covelli D, Dazzi D, et al. Efficacy of B-cell targeted therapy with rituximab in patients with active moderate to severe Graves’ orbitopathy: a randomized controlled study. J Clin Endocrinol Metab. (2015) 100:422–31. doi: 10.1210/jc.2014-3014
65. Stan MN, Garrity JA, Carranza Leon BG, Prabin T, Bradley EA, Bahn RS. Randomized controlled trial of rituximab in patients with Graves’ orbitopathy. J Clin Endocrinol Metab. (2015) 100:432–41. doi: 10.1210/jc.2014-2572
66. Vannucchi G, Campi I, Covelli D, Curro N, Lazzaroni E, Palomba A, et al. Efficacy profile and safety of very low-dose rituximab in patients with graves’ Orbitopathy. Thyroid. (2021) 31:821–8. doi: 10.1089/thy.2020.0269
67. Stohl W. Inhibition of B cell activating factor (BAFF) in the management of systemic lupus erythematosus (SLE). Expert Rev Clin Immunol. (2017) 13:623–33. doi: 10.1080/1744666X.2017.1291343
68. Gilbert JA, Kalled SL, Moorhead J, Hess DM, Rennert P, Li Z, et al. Treatment of autoimmune hyperthyroidism in a murine model of Graves’ disease with tumor necrosis factor-family ligand inhibitors suggests a key role for B cell activating factor in disease pathology. Endocrinology. (2006) 147:4561–8. doi: 10.1210/en.2006-0507
69. Fabris M, Grimaldi F, Villalta D, Picierno A, Fabro C, Bolzan M, et al. BLyS and April serum levels in patients with autoimmune thyroid diseases. Autoimmun Rev. (2010) 9:165–9. doi: 10.1016/j.autrev.2009.07.005
70. Pers JO, Daridon C, Devauchelle V, Jousse S, Saraux A, Jamin C, et al. BAFF overexpression is associated with autoantibody production in autoimmune diseases. Ann N Y Acad Sci. (2005) 1050:34–9. doi: 10.1196/annals.1313.004
71. Campi I, Tosi D, Rossi S, Vannucchi G, Covelli D, Colombo F, et al. B cell activating factor (BAFF) and BAFF receptor expression in autoimmune and nonautoimmune thyroid diseases. Thyroid. (2015) 25:1043–9. doi: 10.1089/thy.2015.0029
72. Salvi M, Covelli D. B cells in Graves’ Orbitopathy: more than just a source of antibodies? Eye (Lond). (2019) 33:230–4. doi: 10.1038/s41433-018-0285-y
73. Hamed Azzam S, Kang S, Salvi M, Ezra DG. Tocilizumab for thyroid eye disease. Cochrane Database Syst Rev. (2018) 11:CD012984. doi: 10.1002/14651858.CD012984.pub2
74. Perez-Moreiras JV, Alvarez-Lopez A, Gomez EC. Treatment of active corticosteroid-resistant graves’ orbitopathy. Ophthalmic Plast Reconstr Surg. (2014) 30:162–7. doi: 10.1097/IOP.0000000000000037
75. Sanchez-Bilbao L, Martinez-Lopez D, Revenga M, Lopez-Vazquez A, Valls-Pascual E, Atienza-Mateo B, et al. Anti-IL-6 receptor tocilizumab in refractory graves’ Orbitopathy: national multicenter observational study of 48 patients. J Clin Med. (2020) 9:2816. doi: 10.3390/jcm9092816
76. Perez-Moreiras JV, Varela-Agra M, Prada-Sanchez MC, Prada-Ramallal G. Steroid-resistant graves’ Orbitopathy treated with tocilizumab in real-world clinical practice: A 9-year single-center experience. J Clin Med. (2021) 10:706. doi: 10.3390/jcm10040706
77. Boutzios G, Chatzi S, Goules AV, Mina A, Charonis GC, Vlachoyiannopoulos PG, et al. Tocilizumab improves clinical outcome in patients with active corticosteroid-resistant moderate-to-severe Graves’ orbitopathy: an observational study. Front Endocrinol (Lausanne). (2023) 14:1186105. doi: 10.3389/fendo.2023.1186105
78. Boskovic O, Medenica S, Radojevic N, Zarkovic M. Etanercept in the treatment of Graves’ ophthalmopathy with primary hypothyroidism and rheumatoid arthritis. Cent Eur J Immunol. (2019) 44:463–5. doi: 10.5114/ceji.2019.92803
79. Durrani OM, Reuser TQ, Murray PI. Infliximab: a novel treatment for sight-threatening thyroid associated ophthalmopathy. Orbit. (2005) 24:117–9. doi: 10.1080/01676830590912562
80. Stein JD, Childers D, Gupta S, Talwar N, Nan B, Lee BJ, et al. Risk factors for developing thyroid-associated ophthalmopathy among individuals with Graves disease. JAMA Ophthalmol. (2015) 133:290–6. doi: 10.1001/jamaophthalmol.2014.5103
81. Nilsson A, Tsoumani K, Planck T. Statins decrease the risk of orbitopathy in newly diagnosed patients with graves disease. J Clin Endocrinol Metab. (2021) 106:1325–32. doi: 10.1210/clinem/dgab070
82. Lanzolla G, Vannucchi G, Ionni I, Campi I, Sileo F, Lazzaroni E, et al. Cholesterol serum levels and use of statins in graves’ Orbitopathy: A new starting point for the therapy. Front Endocrinol (Lausanne). (2019) 10:933. doi: 10.3389/fendo.2019.00933
83. Vondrichova T, de Capretz A, Parikh H, Frenander C, Asman P, Aberg M, et al. COX-2 and SCD, markers of inflammation and adipogenesis, are related to disease activity in Graves’ ophthalmopathy. Thyroid. (2007) 17:511–7. doi: 10.1089/thy.2007.0028
84. Zhang C, Zhang X, Ma L, Peng F, Huang J, Han H. Thalidomide inhibits adipogenesis of orbital fibroblasts in Graves’ ophthalmopathy. Endocrine. (2012) 41:248–55. doi: 10.1007/s12020-012-9600-8
85. Ko J, Kim JY, Lee EJ, Yoon JS. Inhibitory effect of idelalisib, a selective phosphatidylinositol 3-kinase delta inhibitor, on adipogenesis in an in vitro model of graves’ Orbitopathy. Invest Ophthalmol Vis Sci. (2018) 59:4477–85. doi: 10.1167/iovs.18-24509
86. Shahida B, Johnson PS, Jain R, Brorson H, Asman P, Lantz M, et al. Simvastatin downregulates adipogenesis in 3T3-L1 preadipocytes and orbital fibroblasts from Graves’ ophthalmopathy patients. Endocr Connect. (2019) 8:1230–9. doi: 10.1530/EC-19-0319
87. Han YE, Hwang S, Kim JH, Byun JW, Yoon JS, Lee EJ. Biguanides metformin and phenformin generate therapeutic effects via AMP-activated protein kinase/extracellular-regulated kinase pathways in an in vitro model of graves’ Orbitopathy. Thyroid: Off J Am Thyroid Assoc. (2018) 28:528–36. doi: 10.1089/thy.2017.0338
88. Schrijver B, Kooiman MA, Kasteleijn E, van Holten-Neelen C, Virakul S, Paridaens D, et al. Basic fibroblast growth factor induces adipogenesis in orbital fibroblasts: implications for the pathogenesis of graves’ Orbitopathy. Thyroid: Off J Am Thyroid Assoc. (2019) 29:395–404. doi: 10.1089/thy.2018.0544
89. Wang X, Yang S, Ye H, Chen J, Shi L, Feng L, et al. Disulfiram exerts antiadipogenic, anti-inflammatory, and antifibrotic therapeutic effects in an in vitro model of graves’ Orbitopathy. Thyroid. (2022) 32:294–305. doi: 10.1089/thy.2021.0246
90. Gulbins A, Horstmann M, Daser A, Flogel U, Oeverhaus M, Bechrakis NE, et al. Linsitinib, an IGF-1R inhibitor, attenuates disease development and progression in a model of thyroid eye disease. Front Endocrinol (Lausanne). (2023) 14:1211473. doi: 10.3389/fendo.2023.1211473
91. Ko J, Kim JY, Kim JW, Yoon JS. Anti-oxidative and anti-adipogenic effects of caffeine in an in vitro model of Graves’ orbitopathy. Endocr J. (2020) 67:439–47. doi: 10.1507/endocrj.EJ19-0521
92. van Steensel L, Paridaens D, Dingjan GM, van Daele PL, van Hagen PM, Kuijpers RW, et al. Platelet-derived growth factor-BB: a stimulus for cytokine production by orbital fibroblasts in Graves’ ophthalmopathy. Invest Ophthalmol Vis Sci. (2010) 51:1002–7. doi: 10.1167/iovs.09-4338
93. van Steensel L, Paridaens D, van Meurs M, van Hagen PM, van den Bosch WA, Kuijpers RW, et al. Orbit-infiltrating mast cells, monocytes, and macrophages produce PDGF isoforms that orchestrate orbital fibroblast activation in Graves’ ophthalmopathy. J Clin Endocrinol Metab. (2012) 97:E400–8. doi: 10.1210/jc.2011-2697
94. Virakul S, Dalm VA, Paridaens D, van den Bosch WA, Mulder MT, Hirankarn N, et al. Platelet-derived growth factor-BB enhances adipogenesis in orbital fibroblasts. Invest Ophthalmol Vis Sci. (2015) 56:5457–64. doi: 10.1167/iovs.15-17001
95. Virakul S, Heutz JW, Dalm VA, Peeters RP, Paridaens D, van den Bosch WA, et al. Basic FGF and PDGF-BB synergistically stimulate hyaluronan and IL-6 production by orbital fibroblasts. Mol Cell Endocrinol. (2016) 433:94–104. doi: 10.1016/j.mce.2016.05.023
96. van Steensel L, Hooijkaas H, Paridaens D, van den Bosch WA, Kuijpers RW, Drexhage HA, et al. PDGF enhances orbital fibroblast responses to TSHR stimulating autoantibodies in Graves’ ophthalmopathy patients. J Clin Endocrinol Metab. (2012) 97:E944–53. doi: 10.1210/jc.2012-1020
97. Virakul S, van Steensel L, Dalm VA, Paridaens D, van Hagen PM, Dik WA. Platelet-derived growth factor: a key factor in the pathogenesis of graves’ ophthalmopathy and potential target for treatment. Eur Thyroid J. (2014) 3:217–26. doi: 10.1159/000367968
98. Kim SE, Yoon JS, Kim KH, Lee SY. Increased serum interleukin-17 in Graves’ ophthalmopathy. Graefes Arch Clin Exp Ophthalmol. (2012) 250:1521–6. doi: 10.1007/s00417-012-2092-7
99. Fang S, Huang Y, Zhong S, Li Y, Zhang Y, Li Y, et al. Regulation of orbital fibrosis and adipogenesis by pathogenic th17 cells in graves orbitopathy. J Clin Endocrinol Metab. (2017) 102:4273–83. doi: 10.1210/jc.2017-01349
100. Reich K, Warren RB, Lebwohl M, Gooderham M, Strober B, Langley RG, et al. Bimekizumab versus secukinumab in plaque psoriasis. N Engl J Med. (2021) 385:142–52. doi: 10.1056/NEJMoa2102383
101. Gordon KB, Blauvelt A, Papp KA, Langley RG, Luger T, Ohtsuki M, et al. Phase 3 trials of ixekizumab in moderate-to-severe plaque psoriasis. N Engl J Med. (2016) 375:345–56. doi: 10.1056/NEJMoa1512711
102. Warren RB, Blauvelt A, Bagel J, Papp KA, Yamauchi P, Armstrong A, et al. Bimekizumab versus adalimumab in plaque psoriasis. N Engl J Med. (2021) 385:130–41. doi: 10.1056/NEJMoa2102388
103. Yoon JS, Lee HJ, Chae MK, Lee SY, Lee EJ. Cigarette smoke extract-induced adipogenesis in Graves’ orbital fibroblasts is inhibited by quercetin via reduction in oxidative stress. J Endocrinol. (2013) 216:145–56. doi: 10.1530/JOE-12-0257
104. Rhiu S, Chae MK, Lee EJ, Lee JB, Yoon JS. Effect of tanshinone IIA in an in vitro model of Graves’ orbitopathy. Invest Ophthalmol Vis Sci. (2014) 55:5900–10. doi: 10.1167/iovs.14-14008
105. Li H, Yuan Y, Zhang Y, Zhang X, Gao L, Xu R. Icariin inhibits AMPK-dependent autophagy and adipogenesis in adipocytes in vitro and in a model of graves’ orbitopathy in vivo. Front Physiol. (2017) 8:45. doi: 10.3389/fphys.2017.00045
106. Guo Y, Li H, Chen X, Yang H, Guan H, He X, et al. Novel roles of chloroquine and hydroxychloroquine in graves’ Orbitopathy therapy by targeting orbital fibroblasts. J Clin Endocrinol Metab. (2020) 105:1906–17. doi: 10.1210/clinem/dgaa161
107. Li H, Gao L, Min J, Yang Y, Zhang R. Neferine suppresses autophagy-induced inflammation, oxidative stress and adipocyte differentiation in Graves’ orbitopathy. J Cell Mol Med. (2021) 25:1949–57. doi: 10.1111/jcmm.15931
108. Diao J, Chen X, Mou P, Ma X, Wei R. Potential therapeutic activity of berberine in thyroid-associated ophthalmopathy: inhibitory effects on tissue remodeling in orbital fibroblasts. Invest Ophthalmol Vis Sci. (2022) 63:6. doi: 10.1167/iovs.63.10.6
109. Kim H, Choi YH, Park SJ, Lee SY, Kim SJ, Jou I, et al. Antifibrotic effect of Pirfenidone on orbital fibroblasts of patients with thyroid-associated ophthalmopathy by decreasing TIMP-1 and collagen levels. Invest Ophthalmol Vis Sci. (2010) 51:3061–6. doi: 10.1167/iovs.09-4257
110. Wu SB, Hou TY, Kau HC, Tsai CC. Effect of pirfenidone on TGF-beta1-induced myofibroblast differentiation and extracellular matrix homeostasis of human orbital fibroblasts in graves’ Ophthalmopathy. Biomolecules. (2021) 11:1424. doi: 10.3390/biom11101424
111. Wei YH, Liao SL, Wang SH, Wang CC, Yang CH. Simvastatin and ROCK Inhibitor Y-27632 Inhibit Myofibroblast Differentiation of Graves’ Ophthalmopathy-Derived Orbital Fibroblasts via RhoA-Mediated ERK and p38 Signaling Pathways. Front Endocrinol (Lausanne). (2020) 11:607968. doi: 10.3389/fendo.2020.607968
112. Xu Z, Ye H, Xiao W, Sun A, Yang S, Zhang T, et al. Metformin attenuates inflammation and fibrosis in thyroid-associated ophthalmopathy. Int J Mol Sci. (2022) 23:15508. doi: 10.3390/ijms232415508
113. Sun A, Ye H, Xu Z, Chen J, Xiao W, Zhang T, et al. Serelaxin alleviates fibrosis in thyroid-associated ophthalmopathy via the notch pathway. Int J Mol Sci. (2023) 24:8356. doi: 10.3390/ijms24098356
114. Yu WK, Hwang WL, Wang YC, Tsai CC, Wei YH. Curcumin suppresses TGF-beta1-induced myofibroblast differentiation and attenuates angiogenic activity of orbital fibroblasts. Int J Mol Sci. (2021) 22:6829. doi: 10.3390/ijms22136829
115. Yoon JS, Chae MK, Jang SY, Lee SY, Lee EJ. Antifibrotic effects of quercetin in primary orbital fibroblasts and orbital fat tissue cultures of Graves’ orbitopathy. Invest Ophthalmol Vis Sci. (2012) 53:5921–9. doi: 10.1167/iovs.12-9646
116. Yang S, Wang X, Xiao W, Xu Z, Ye H, Sha X, et al. Dihydroartemisinin exerts antifibrotic and anti-inflammatory effects in graves’ Ophthalmopathy by targeting orbital fibroblasts. Front Endocrinol (Lausanne). (2022) 13:891922. doi: 10.3389/fendo.2022.891922
117. Hei X, Lin B, Wu P, Li X, Mao Z, Huang S, et al. Lutein targeting orbital fibroblasts attenuates fibrotic and inflammatory effects in thyroid-associated ophthalmopathy. Exp Eye Res. (2023) 232:109515. doi: 10.1016/j.exer.2023.109515
118. Xu S, Mao H. Crocin inhibits orbital fibroblasts fibrosis in thyroid-associated ophthalmopathy. Curr Eye Res. (2024) 49:330–7. doi: 10.1080/02713683.2023.2280441
119. Park M, Banga JP, Kim GJ, Kim M, Lew H. Human placenta-derived mesenchymal stem cells ameliorate orbital adipogenesis in female mice models of Graves’ ophthalmopathy. Stem Cell Res Ther. (2019) 10:246. doi: 10.1186/s13287-019-1348-0
120. Plohn S, Hose M, Schluter A, Michel L, Diaz-Cano S, Hendgen-Cotta UB, et al. Fingolimod improves the outcome of experimental graves’ Disease and associated orbitopathy by modulating the autoimmune response to the thyroid-stimulating hormone receptor. Thyroid. (2019) 29:1286–301. doi: 10.1089/thy.2018.0754
121. Shi L, Ye H, Huang J, Li Y, Wang X, Xu Z, et al. IL-38 exerts anti-inflammatory and antifibrotic effects in thyroid-associated ophthalmopathy. J Clin Endocrinol Metab. (2021) 106:e3125–e42. doi: 10.1210/clinem/dgab154
122. Wu P, Lin B, Huang S, Meng J, Zhang F, Zhou M, et al. IL-11 is elevated and drives the profibrotic phenotype transition of orbital fibroblasts in thyroid-associated ophthalmopathy. Front Endocrinol (Lausanne). (2022) 13:846106. doi: 10.3389/fendo.2022.846106
123. Ko J, Kim YJ, Choi SH, Lee CS, Yoon JS. Yes-associated protein mediates the transition from inflammation to fibrosis in graves’ Orbitopathy. Thyroid. (2023) 33:1465–75. doi: 10.1089/thy.2023.0309
124. Xie Y, Pan Y, Chen Q, Chen Y, Chen G, Wang M, et al. Selective BD2 inhibitor exerts anti-fibrotic effects via BRD4/foxM1/plk1 axis in orbital fibroblasts from patients with thyroid eye disease. Invest Ophthalmol Vis Sci. (2023) 64:9. doi: 10.1167/iovs.64.7.9
125. Li H, Min J, Yang Y, Suo W, Wang W, Tian J, et al. TMEM2 inhibits the development of Graves’ orbitopathy through the JAK-STAT signaling pathway. J Biol Chem. (2024) 300:105607. doi: 10.1016/j.jbc.2023.105607
Keywords: thyroid-associated ophthalmopathy, drug treatment, research progress, molecular targets, traditional Chinese medicines
Citation: Li Z (2025) Novel perspectives on the pharmacological treatment of thyroid-associated ophthalmopathy. Front. Endocrinol. 15:1469268. doi: 10.3389/fendo.2024.1469268
Received: 23 July 2024; Accepted: 23 December 2024;
Published: 13 January 2025.
Edited by:
Dhiraj Kumar, National Eye Institute (NIH), United StatesReviewed by:
Laiba Arshad, Forman Christian College, PakistanCopyright © 2025 Li. This is an open-access article distributed under the terms of the Creative Commons Attribution License (CC BY). The use, distribution or reproduction in other forums is permitted, provided the original author(s) and the copyright owner(s) are credited and that the original publication in this journal is cited, in accordance with accepted academic practice. No use, distribution or reproduction is permitted which does not comply with these terms.
*Correspondence: Zilin Li, bGl6bDMwMjFAbWFpbHMuamx1LmVkdS5jbg==
Disclaimer: All claims expressed in this article are solely those of the authors and do not necessarily represent those of their affiliated organizations, or those of the publisher, the editors and the reviewers. Any product that may be evaluated in this article or claim that may be made by its manufacturer is not guaranteed or endorsed by the publisher.
Research integrity at Frontiers
Learn more about the work of our research integrity team to safeguard the quality of each article we publish.