- 1Food Functionality Research Division, Korea Food Research Institute, Wanju-gun, Republic of Korea
- 2Department of Pharmacology, Asan Medical Center, University of Ulsan College of Medicine, Seoul, Republic of Korea
- 3Bio-medical Institute of Technology, University of Ulsan College of Medicine, Seoul, Republic of Korea
- 4Department of Food Biotechnology, Korea University of Science and Technology, Daejeon, Republic of Korea
Introduction: The status of an impaired gut microbial community, known as dysbiosis, is associated with metabolic diseases such as obesity and insulin resistance. The use of probiotics has been considered an effective approach for the treatment and prevention of obesity and related gut microbial dysbiosis. The anti-obesity effect of Lacticaseibacillus paracasei AO356 was recently reported. However, the effect of L. paracasei AO356 on the gut microbiota has not yet been identified. This study aimed to elucidate the effect of L. paracasei AO356 on gut microbiota and ensure its safety for use as a probiotic.
Methods: Oral administration of L. paracasei AO356 (107 colony-forming units [CFU]/mg per day, 5 days a week, for 10 weeks) to mice fed a high-fat diet significantly suppressed weight gain and fat mass. We investigated the composition of gut microbiota and explored its association with obesity-related markers.
Results: Oral administration of L. paracasei AO356 significantly changed the gut microbiota and modified the relative abundance of Lactobacillus, Bacteroides, and Oscillospira. Bacteroides and Oscillospira were significantly related to the lipid metabolism pathway and obesity-related markers. We also confirmed the safety of L. paracasei AO356 using antibiotics resistance, hemolysis activity, bile salt hydrolase activity, lactate production, and toxicity tests following the safety assessment guidelines of the Ministry of Food and Drug Safety (MFDS).
Discussion: This study demonstrated that L. paracasei AO356 is not only associated with an anti-obesity effect but also with changes in the gut microbiota and metabolic pathways related to obesity. Furthermore, the overall safety assessment seen in this study could increase the potential use of new probiotic materials with anti-obesity effects.
1 Introduction
Obesity is a global public health concern associated with a wide range of health problems, including diabetes, cardiovascular disease, and cancer. Obesity, characterized by an excessive accumulation of body fat, is a complex and multifactorial condition resulting from a combination of factors such as genetics, environment, and behavior (1). While traditional interventions such as diet and exercise are effective in obesity management, emerging evidence suggests that gut microbiota may also play a role in regulating body weight and inflammation related to obesity (2, 3). Gut microbiota influences nutrient absorption, energy balance, and the immunologic system, which contribute to the modulation of body homeostasis and metabolism (4–6).
Probiotics are microorganisms that confer a health benefit on the host when administered in adequate amounts (7). Probiotics are thought to exert their beneficial effects through various mechanisms, including modulating the gut microbiota, strengthening the gut barrier, and interacting with the host immune system (8). Therefore, the use of probiotics may serve as an effective approach to the treatment and prevention of obesity via modulation of the gut microbiota. Our previous study suggested that Lacticaseibacillus paracasei AO356 exerts its anti-obesity effect by regulating adipogenesis and thermogenesis (9). However, the effect of L. paracasei AO356 on gut microbiota was not confirmed. Lactic acid bacteria including Lactobacillus have been reported to have functions related to the inhibition of pathogen colonization, cholesterol metabolism, liver function, and fat accumulation (10–14). Moreover, the anti-obesity effect of L. paracasei strains are reported in several animal and human studies (15–17). Of note, L. paracasei K56 exerts its anti-obesity effect by modulating the gut microbiota in high-fat diet (HFD)-induced obesity mice (18). Therefore, we expect the changes in the gut microbiota to be related to the anti-obesity effect of L. paracasei AO356.
As many beneficial effects of probiotics have been reported, safety concerns such as antibiotic resistance and toxicity of strains have been raised as important issues for probiotics selection. In 2021, the Korea Ministry of Food and Drug Safety (MFDS) presented guidelines regarding the safety of probiotics (19). Thus, in this study, we investigated the effect of L.paracasei AO356 on gut microbiota and its association with obesity and evaluated its safety following the guidelines presented by MFDS. Our findings suggest that L.paracasei AO356 may be a promising strategy for the management of obesity and its associated health problems.
2 Materials and methods
2.1 Preparation for L. paracasei AO356
L. paracasei AO356 (KCCM12145P), isolated from fecal samples of healthy Koreans in general dietary pattern, was cultured in the MRS broth medium (BD Difco co. USA) at 37°C for 24 h aerobically, collected by centrifugation (1000 × g for 20 min), and washed twice with sterilized PBS. Freeze-dried cells were then stored at -80°C until they were administered to the mice. The colony forming unit (CFU) with freeze-dried cells was determined before they were used.
2.2 An animal experiment
C57BL/6 mice (thirty 6-week-old males) purchased from Central Lab Animal, Inc. (Seoul, Korea) were housed under standard laboratory conditions (22 ± 2°C, 12 h light/dark cycle) and acclimatized to the lab environment for 1 week. The animals were randomly allocated into three groups. One group was fed a normal chow diet (ND, Teklad 2018S; Envigo Research Indianapolis, Indiana, USA). The other two groups were fed a high-fat diet (HFD) comprising 55% fats (D12492 formula of Research Diet, Saeron Bio, Gyeonggi, Korea) with or without L. paracasei AO356. The diets were provided ad libitum, and L. paracasei AO356 was administered at a dose of 5 × 107 CFU/mg in 100 µL of PBS by oral gavage 5 days a week for 10 weeks. Two mice were housed per cage and their body weights were measured once a week (n=9 for ND, n=8 for HFD and n=9 for HFD + AO356). At the end of the experiment, fresh feces were collected and stored at −80°C for gut microbiome analysis. All animals were then subjected to overnight fasting and sacrificed. Tissues, including epididymal white fat tissue (EFT), retroperitoneal white fat tissue (RFT), inguinal white fat tissue (IFT), and intrascapular brown adipose tissue (BAT), were immediately excised and weighed. The concentrations of glucose, triglycerides, LDL cholesterol, and HDL cholesterol in serum were determined by appropriate enzyme kinetic or colorimetric assays (Roche, Mannheim, Germany) on a Hitachi automatic analyzer Modular Analytics (Hitachi, Japan). Serum insulin was quantified using ELISA assay kits (ALPCO, Diagnostics, NH, USA). Homeostasis model assessment for insulin resistance (HOMA-IR) was calculated via the following equation (20): fasting glucose (mg/dL) × fasting insulin (µU/mL) ÷ 2430. This experiment was approved by the Korea Food Research Institutional Animal Care and Use Committee (approval # KFRI-M-16047) following their guidelines and regulations on the care and use of laboratory animals.
2.3 Gut microbiota analysis
Metagenomic DNA from feces was extracted using the QIAamp DNA Stool Mini Kit (Qiagen) with an additional bead-beating step as previously described (21). The V1-V2 hypervariable region of the bacterial 16S rRNA genes was amplified by PCR using universal primers (8F and 338R) with barcode sequences for multiplexing reads of each sample. Amplicon sequencing was performed on an Ion Torrent PGM system (Thermo Scientific, DE, USA) with a 318D sequencing chip. Raw sequence reads were quality-filtered and quality-controlled reads were processed for diversity analysis and taxonomy assignment using the Quantitative Insights into Microbial Ecology 1 (QIIME1) software package (22). The molecular functions of each sample were predicted based on 16S rRNA data in the Phylogenetic Investigation of Communities by Reconstruction of Unobserved States (PICRUSt) software (23).
2.4 Determination of minimum inhibitory concentration (MIC) of antibiotics
The MIC of AO356 was determined with an ETEST® strip (BioMérieux, SA, France). AO356 was diluted in 0.9% saline (0.5~1.0 McFarland standard) and streaked on MRS agar plates. After the plates were dried, ETEST® strips for ampicillin (AM), chloramphenicol (CL), clindamycin (CM), erythromycin (EM), gentamicin (GM), kanamycin (KM), streptomycin (SM), tetracycline (TC), and vancomycin (VA) were applied to the plates. The antibiotics concentration scale of the strips was from 0.016 μg/mL to 256 μg/mL. The agar plates were incubated anaerobically at 37°C for 48 h.
2.5 Hemolysis activity test
Hemolysis activity was evaluated by the plate assay described by the Ministry of Food and Drug Safety (MFDS) for microbiology guidelines. A loop of AO356 was streaked on a sheep blood agar plate and incubated at 37°C for 24 h. Escherichia coli (ACTC 1682) was cultured and used as a positive control. Hemolysis activity was determined by the form of hemolytic cells formed around colony on the plate (24, 25). Beta-hemolysis causes a clear zone with the transparency of the base medium surrounds the colony when observed under the light. Alpha hemolysis causes a green or brown discoloration in the medium. Gamma-hymolysis indicates the lack of hemolysis.
2.6 Bile salt hydrolase (BSH) activity test
The bile salt hydrolase activity was tested using MRS agar plates including 0.5% taurodeoxycholic acid (MRS-TDCA). AO356 was then streaked on MRS-TDCA and incubated anaerobically at 37°C for 48 h. The BSH activity was determined by the visible halo surrounding colonies (26).
2.7 Toxicity test with Lactate dehydrogenase (LDH) assay
The Caco-2 cell line (human epithelial colorectal adenocarcinoma cell line; ATCC HTB-37) was seeded into a 24-well plate at a density of 1×105 cells/well with a minimum essential medium (MEM) containing 10% fetal bovine serum and 1% penicillin-streptomycin and incubated at 37°C and 5% CO2 for 2 days. AO356 was treated on a Caco-2 cell with 1×107, 1×108, and 1×109 CFU/well and incubated at 37°C and 5% CO2 for 24 h. Escherichia coli (ACTC 1682) was used as a positive control. The test wa perfomed in triplicate. The supernatant was centrifuged at 5,000 g for 15 min and used as a sample. The toxicity of AO356 was determined using an LDH assay kit following the manufacturer’s protocol (ab65393, Abcam, Cambridge, UK). The non-treated culture medium was used as a low control, the cell lysis buffer was used as a high control, and Escherichia coli (ACTC1682), known to be toxic, was used as a positive control.
2.8 D-lactate production test
The D-lactate production was determined using a D-/L-lactic acid assay kit following the manufacturer’s protocol (K-DLATE, Megazyme Ltd., Wicklow, Ireland). AO356 was inoculated on MRS broth and incubated at 37°C for 24 h. The culture medium was centrifuged at 5,000 g for 15 min and the supernatant was used for measurement of lactate production. Lactobacillus rhamnosus GG was used as a positive control.
2.9 Statistical analysis
Statistical significance was evaluated using a one-way analysis of variance (ANOVA) followed by Tukey’s a post-hoc test or the Kruskal-Wallis test followed by Dunn’s test. Permutational multivariate analysis of variance (PERMANOVA) statistical analyses were conducted on the Bray-Curtis dissimilarity with 999 permutations using the adonis2 function in the vegan package in R (27). Statistical significance was set at p<0.05. To identify taxa with differing relative abundances among groups, linear discriminant analysis effect size (LEfSe) analyses were conducted using the web-based program Galaxy (28). The strength of relationships between parameters was assessed using the Spearman correlation test.
3 Results
3.1 Effects of L. paracasei AO356 on HFD-induced obesity mice
To examine the effects of L. paracasei AO356 on obesity, we established an HFD-induced obesity mice model. Food and water intake was monitored every 2–3 days, and daily food intake and calories consumed were calculated. HFD feeding led to a significant decrease in water intake and an increase in calorie intake, body weight, and fat accumulation compared with the normal diet (NOR) group (Figure 1). There was no significant difference in water and calorie intake between the HFD group (HFD) and the HFD group treated with L. paracasei AO356 (HFD+AO356) (Figures 1A, B). Notably, 10 weeks of L. paracasei AO356 intervention affected body weight and fat accumulation upon HFD feeding. L. paracasei AO356 intervention significantly decreased body weight by 34.21% compared with that in the HFD group (Figure 1C). Additionally, L. paracasei AO356 intervention significantly decreased the weights of EFT (34.83%), RFT (36.51%), IFT (35.40%) and BAT (26.56%) (Figure 1D).
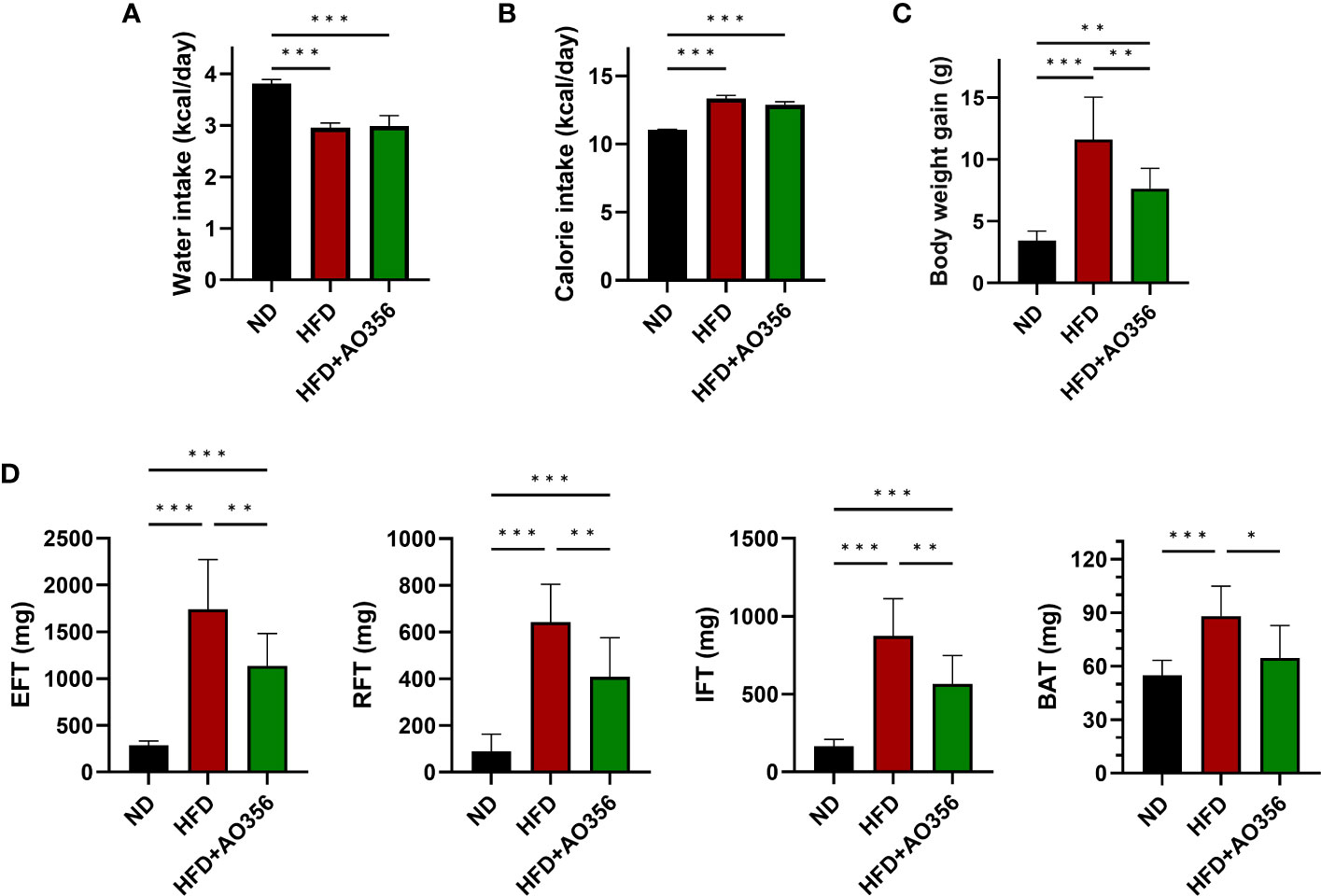
Figure 1 Effect of L. paracasei AO356 on weight and fat accumulation in a high-fat diet (HFD)-induced obese model after intervention for 10 weeks. (A) Water intake (B) Calorie intake (C) Body weight (D) Fat weight. Statistical significance was assessed using one-way ANOVA and Tukey’s multiple comparison test. The significance is indicated by *P < 0.05, **P < 0.01, ***P < 0.001. ND, normal diet mice; HFD, HFD mice; HFD+AO356, HFD mice with Lacticaseibacillus paracasei AO356 intervention; EFT, epididymal white fat tissue; RFT, retroperitoneal white fat tissue; IFT, inguinal white fat tissue; BAT, brown fat tissue.
The HFD led to significant increases in insulin resistance and serum lipid profiles (Supplementary Figure 1). L. paracasei AO356 intervention significantly decreased the levels of glucose (16.07%), insulin (36.60%), and HOMA-IR (15.82%) which were increased by the HFD. Although microbial intake improved blood markers that were increased by the HFD, only glucose levels showed a significant difference between the L. paracasei AO356 intervention group and the HFD group (Supplementary Figures 1A-C). L. paracasei AO356 intervention also significantly decreased the TG levels by 11.42%. In addition, HDL and LDL cholesterol were decreased by 5.81% and 21.69%, respectively, but this decrease was not statistically significant when comparing the L. paracasei AO356 intervention group to the HFD group (Supplementary Figures 1D-F). These results indicate that L. paracasei AO356 has anti-obesity effects such as preventing weight gain and fat accumulation.
3.2 Gut microbial change after administration of L. paracasei AO356
The alpha diversity indices such as Chao1 and Shannon were similar among all groups (Figures 2A, B). However, the results of PCoA on the Bray-Curtis distance matrix showed differences among groups (Figure 2C). The ND group and the other two groups were clustered into two areas. The HFD and the L. paracasei AO356 intervention groups did not overlap and could be distinguished. The composition and the relative abundance of bacteria at the phylum level are shown in Figure 2D. In the ND group, the gut microbiota was dominated by Bacteroidetes followed by Firmicutes. Conversely, in the other two groups, Firmicutes was predominant, followed by Bacteroidetes. The HFD significantly decreased the relative abundance of Bacteroidetes and increased the relative abundance of Firmicutes in the HFD group compared to the ND groups. However, there was no significant difference in the relative abundance of Bacteroidetes and Firmicutes between the HFD and HFD+AO356 groups. Figure 2E presents the composition and relative abundance of the gut microbiota at the family level. In the ND group, the gut was dominated by the S24-7 family, followed by an unclassified family of Clostridiales. In contrast, in the HFD and HFD+AO356 groups, the S24-7 family, and unclassified families of Clostridiales, Ruminococcaceae, Lachnospiraceae, and Desulfovibrionaceae shared the predominance. Of note, the L. paracasei AO356 intervention significantly restored the relative abundance of Bacteroidaceae, which was significantly increased by the HFD when compared to the ND group.
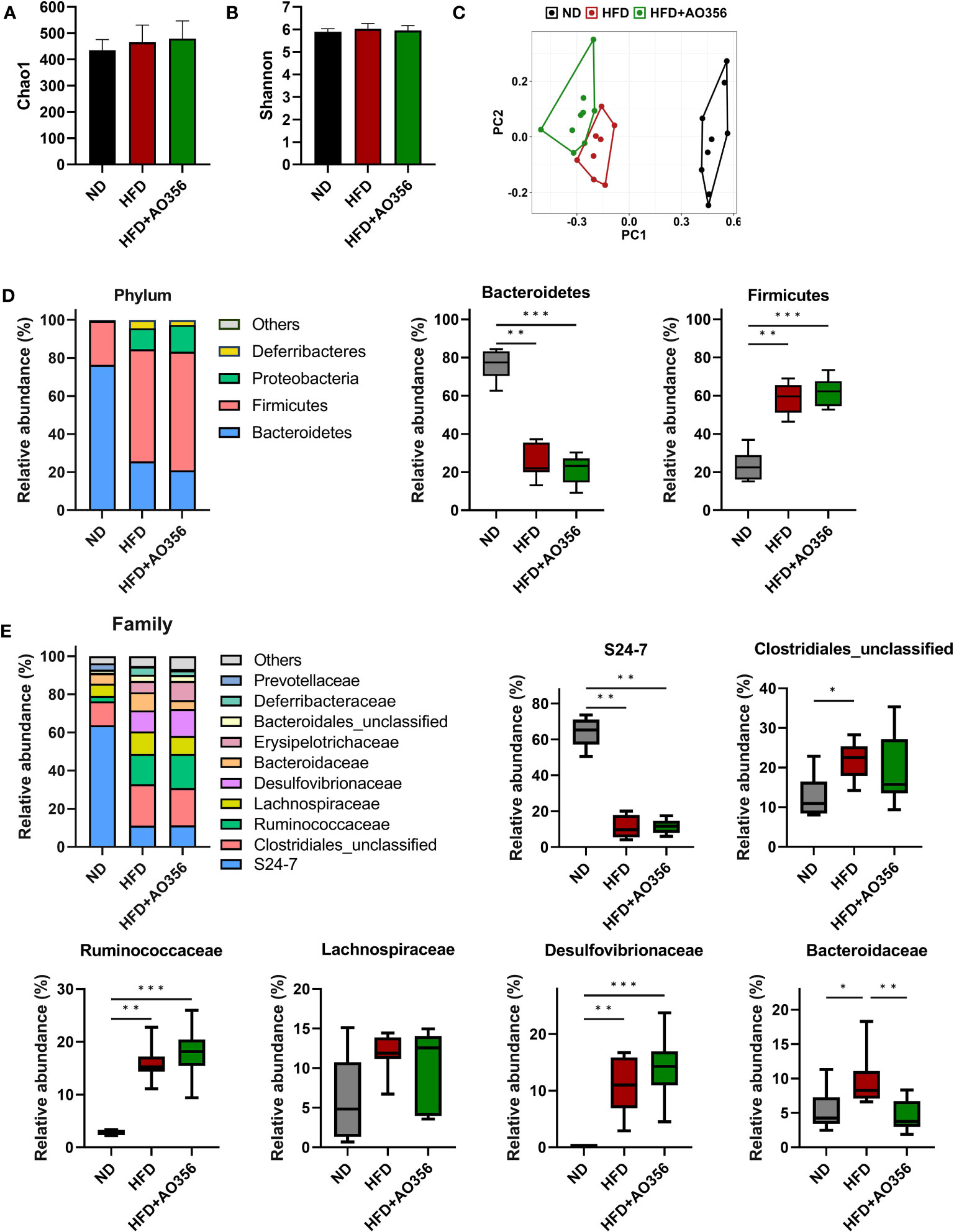
Figure 2 Effect of Lacticaseibacillus paracasei AO356 on the structure of gut microbiota in a high-fat diet (HFD)-induced obese model after intervention for 10 weeks. (A) Chao1 alpha diversity index. (B) Shannon alpha diversity index. (C) principal coordinate analysis (PCoA) plot based on Bray-Curtis dissimilarity. (D) Stacked bar chart of gut microbiota at phylum level and the relative abundance of phyla in each group. (E) Stacked bar chart of gut microbiota at phylum level and the relative abundance of families in each group. ND, normal diet mice; HFD, HFD mice; HFD+AO356, HFD mice with Lacticaseibacillus paracasei AO356 intervention; Data in the box plots are presented as the mean ± SD. Statistical significance was assessed using the Kruskal–Wallis test and Dunn’s multiple comparison test. The significance is indicated by *P < 0.05, **P < 0.01, ***P < 0.001.
3.3 Association of gut microbiota with body weight and fat accumulation
LEfSe analysis was performed to identify significantly differential genera among groups (Figure 3A). The ND group had a higher relative abundance of Prevotella, Sutterella, and Lactobacillus. The HFD group had a higher relative abundance of Bacteroides and Mucispirillum. The HFD+AO356 group had a higher relative abundance of nine genera including Allobaculum, Oscillospira, and Ruminococcus. Moreover, L. paracasei AO356 intervention significantly increased the relative abundance of Lactobacillus and decreased the relative abundance of Bacteroides in the HFD+AO356 group compared to the HFD group (Figure 3B). A Spearman correlation analysis was performed to identify the association of gut microbial genera with obesity-related markers (Figure 3C). Six genera were found to be significantly correlated with one or more obesity-related markers. In particular, Bacteroides showed a positive correlation with body weight, fat accumulation (RFT, IFT, and EFT), insulin resistance (Glucose, insulin, and HOMA-IR), and triglyceride levels. In contrast, Oscillospira showed a negative correlation with body weight, fat accumulation (RFT and IFT, insulin resistance (Glucose, insulin, and HOMA-IR), and lipid profiles (LDL and HDL cholesterol). These results suggested that the L. paracasei AO356 intervention-induced changes in gut microbiota are linked to its anti-obesity effects such as preventing weight gain and fat accumulation.
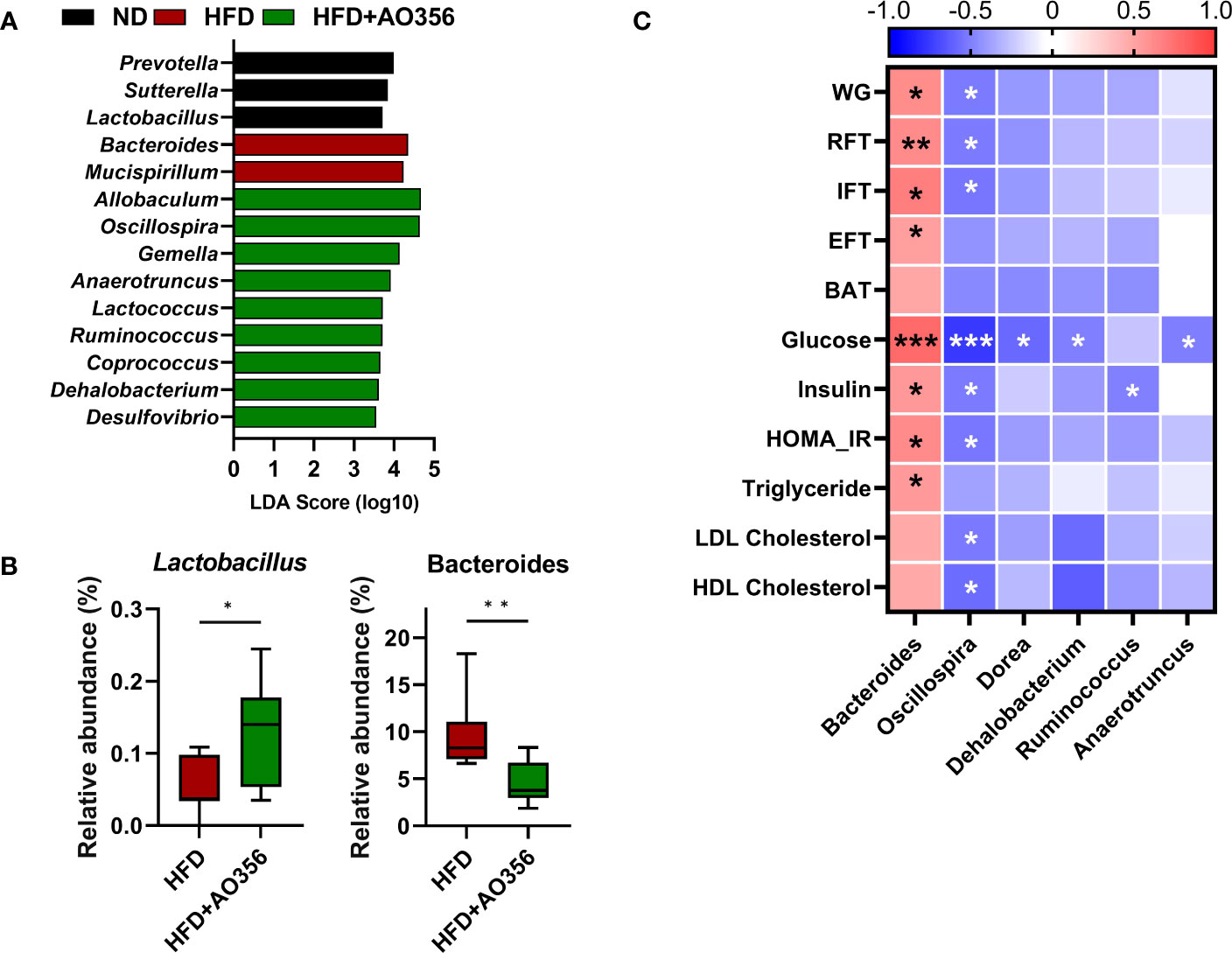
Figure 3 Association of microbiota Lacticaseibacillus paracasei AO356 and obesity-related markers. (A) LEfSe analysis identifies the significantly differently abundant taxa with a cutoff value of log10(LDA score) above 2.0. (B) Box plot of the relative abundance of Lactobacillus and Bacteroides. (C) A heatmap generated using Spearman correlation shows the association of genera with obesity-related markers. HFD, HFD mice; HFD+AO356, HFD mice with Lacticaseibacillus paracasei AO356 intervention; Data in the box plots are presented as the mean ± SD. Statistical significance was assessed using the Mann–Whitney test. The significance is indicated by *P < 0.05, **P < 0.01, ***P < 0.001. The color scale of the heatmap indicates the value of the correlation coefficient.
3.4 Functional metagenome prediction analysis
To evaluate differences in functional attributes of gut microbiota in response to L. paracasei AO356 intervention, predicted functional metagenomic profiles based on the Kyoto Encyclopedia of Genes and Genomes (KEGG) pathways were generated using PICRUSt. Twenty-five pathways were found to be associated with L. paracasei AO356 intervention (Supplementary Table 1). The L. paracasei AO356 intervention resulted in the enrichment of the biosynthesis of other secondary metabolites (isoflavonoid biosynthesis), carbohydrate metabolism-related pathways (butanoate metabolism, propanoate metabolism, and pentose phosphate metabolism), and lipid metabolism-related pathways (biosynthesis of unsaturated fatty acids, fatty acid metabolism, and ether lipid metabolism). Spearman’s correlation analysis was performed to identify the association of enriched pathways with obesity-related markers and genera (Figure 4). Ten pathways were found to be significantly correlated with one or more obesity-related markers. Of note, lipid metabolism-related pathways (Ether lipid metabolism, biosynthesis of unsaturated fatty acids, fatty acid biosynthesis) are strongly negatively correlated with obesity-related markers. In particular, biosynthesis of unsaturated fatty acids showed a negative correlation with RFT, IFT, glucose, and Bacteroides, and a positive correlation with Oscillospira, Dorea, and Anaerotruncus. Additionally, Fatty acid biosynthesis showed a negative correlation with body weight, RFT, HOMA-IR, TG, and HDL-C, and a positive correlation with Oscillospira. These results suggested that the L. paracasei AO356 intervention induced the modulation of lipid metabolism such as ether lipid metabolism, biosynthesis of unsaturated fatty acids, and fatty acid biosynthesis linked to the changes in gut microbiota such as Bacteroides, Oscillospira, and Dorea, resulting in an anti-obesity effect.
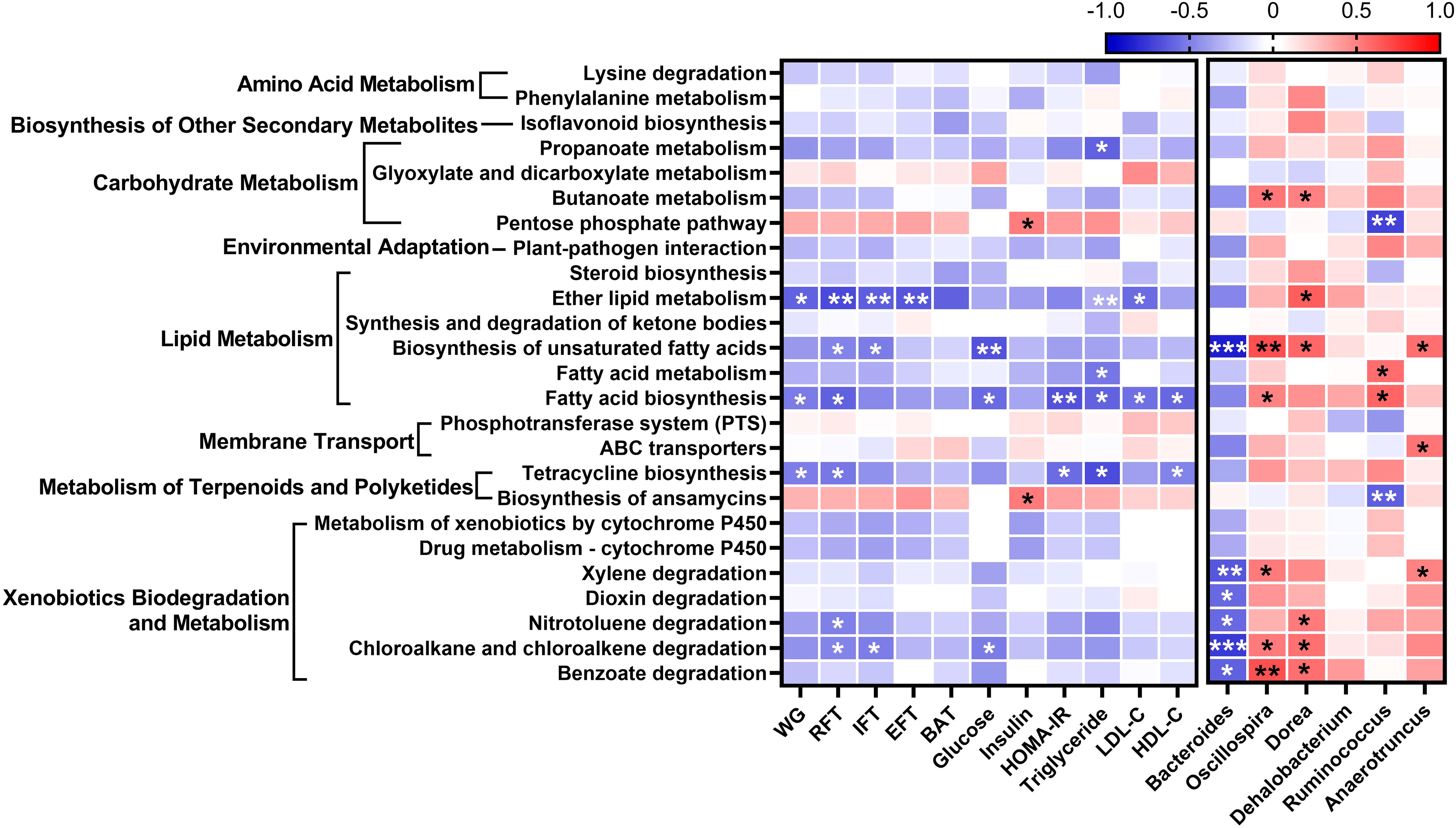
Figure 4 Association of predicted functional metagenome with gut microbiota and obesity-related markers. A heatmap generated using Spearman correlation shows the association of predicted functional metagenome at level 3 with obesity-related markers and genera that correlate with obesity-related markers. The significant correlations are indicated by *P < 0.05, **P < 0.01, ***P < 0.001. The color scale indicates the value of the correlation coefficient.
3.5 Safety evaluation
To evaluate the safety of L. paracasei AO356, we examined the antibiotics resistance, hemolysis activity, bile salt hydrolase (BSH) activity, measurements of D-/L-lactate production, and toxicity using an LDH assay which included safety assessment items presented in the food safety guidelines (29). The antibiotic resistance of L. paracasei AO356 for ampicillin, chloramphenicol, clindamycin, erythromycin, gentamicin, kanamycin, streptomycin, tetracycline, and vancomycin was determined using an E-test strip. The MIC values of L. paracasei AO356 were lower than the cut-off in the the European Safety Food Authority (ESFA) guidelines, suggesting that L. paracasei AO356 is susceptible to 9 antibiotics (Supplementary Table 2). In the hemolysis activity test, L. paracasei AO356 exhibited non-hemolytic activity (γ-hemolysis). Conversely, Escherichia coli, which is known to be toxic, showed clear hemolytic activity (β-hemolysis) (Supplementary Figure 2A). We examined the BSH activity of L. paracasei AO356 with taurodeoxycholic acid (TDCA), which is one of the bile salts, and the result was negative (Supplementary Figure 2B). To verify the safety of L. paracasei AO356 for virulence or toxin-related propertes, we performed an LDH assay (Supplementary Figure 2C). The cytotoxicity of caco-2 cells co-cultured with E. coli was 95–100%, whereas the cytotoxicity of caco-2 cells co-cultured with L. paracasei AO356 was 17–22%. The cytotoxicity of L. paracasei AO356 was similar to that of the low control (21%) treated only media. In measurement of D-lactate, L. paracasei AO356 produced 2.18 g/L (94.30%) of L-lactate and 0.14 g/L (5.70%) of D-lactate. The level of D-lactate production was lower than that of Lactobacillus rhamnosus GG which is one of the most commonly used probiotic strain (Supplementary Figure 2E). These results suggested that L. paracasei AO356 can be considered a safe strain and used as a healthy functional food ingredient.
4 Discussion
In this study, the L. paracasei AO356 intervention prevented weight gain and fat accumulation without changes in energy intake. The L. paracasei AO356 intervention also modulated the overall composition of gut microbiota and changed the gut microbiota at the genus level. Additionally, the L. paracasei AO356 intervention also modulated the predicted functional metagenomic profiles and changed the pathways correlated with weight gain and fat accumulation. Furthermore, we have secured the safety of L. paracasei AO356 for antibiotics resistance, hemolysis activity, bile salt hydrolase activity, toxicity, and D-lactate production. These results indicate that L. paracasei AO356 has an anti-obesity effect, can modulate gut microbiota, and is guaranteed to be safe as a probiotic and healthy functional food.
Our previous study investigated the effects of L. paracasei AO356 on an obesity mice model concerning aspects of regulating adipogenesis and thermogenesis (9). The study revealed that the anti-obesity effect of L. paracasei AO356 may be associated with the downregulation of adipogenesis transcription factor and lipid metabolism-related genes, such as Srebp1c, Pparγ, Fas, C/ebpα, and Fabp4 and upregulation of thermogenesis-related genes, such as Ucp1, Cpt1, Pgc1α, Cidea, and Prdm16 in epididymal and subcutaneous fat pads. The study also compared the anti-obesity effects of L. paracasei AO356 at two concentrations of 1× 107 CFU/head or 1 × 108 CFU/head. There was no significant difference in the anti-obesity effect of L. paracasei between the two concentrations, which suggested that L. paracasei AO356 showed anti-obesity activity at doses > 107 CFU/head. However, the previous study did not explore the effect of L. paracasei AO356 on gut microbiota. Therefore, in this study, we investigated the influence of L. paracasei AO356 and its anti-obesity effects on the composition of gut microbiota and predicted metagenomic profiles at an intermediate concentration of 5 × 107 CFU/head.
In this study, Prevotella and Bacteroides were the most characteristic genera in the ND and HFD groups, respectively. The association of Prevotella and Bacteroides with diet and obesity has been reported. Industrialized populations consuming Western diets, low in fiber and high in fat and processed sugar, have a gut microbiota dominated by Bacteroidaceae (whose representative genus is Bacteroides), whereas traditional populations have a gut microbiota dominated by Prevotellaceae (whose representative genus is Prevotella) (30). Individuals whose guts are enriched with Bacteroides have a higher incidence of diseases associated with a Western diet than individuals whose guts are enriched with Prevotella (31). Bacteroides were prominent among obese individuals and positively correlated with BMI (32). Additionally, an abundance of Prevotella and Bacteroides are associated with anti-obesity susceptibility. Individuals with high Prevotella/Bacteroides ratios are more susceptible to loss of body weight and body fat after a high-fiber diet (33, 34). Anti-obesity probiotics, including complexes of Lactobacillus plantarum CBT LP3 and Bifidobacterium breve CBT BR3, were not effective in individuals whose guts were enriched with Bacteroides (35). The rate of decrease in BMI in individuals whose guts were enriched with Prevotella was higher than in individuals whose guts were enriched with Bacteroides after 3 weeks of a calorie-restriction diet (36). Our results showed that the L. paracasei AO356 intervention restored the relative abundance of Bacteroides which was increased by the HFD feeding. Our results, in combination with previous literature, suggest that decreased relative abundance of Bacteroides after L. paracasei AO356 intervention may have contributed to increased susceptibility to body weight and body fat loss in HFD-induced obese mice.
We also discovered that the L. paracasei AO356 intervention significantly increased the relative abundance of Oscillospira. Oscillospira showed a negative correlation with obesity-related markers and a negative correlation with metabolic pathways such as unsaturated fatty acid biosynthesis, fatty acid biosynthesis, and butanoate metabolism. Several clinical studies found that individuals with lower BMIs had guts that were more enriched Oscillospira compared to those with a higher BMI and that Oscillospira was negatively correlated with BMI (37–41). Recently, Oscillospira was proposed as a candidate for next-generation probiotics (42). However, the mechanism by which Oscillospira acts on obesity is still unclear. Chen et al. suggested that the glycan degradation properties of Oscillospira were a possible mechanism for the reason why Oscillospira is related to leanness (43). Hosts need to consume energy to regenerate the degraded glycan that constitutes gut mucins degraded by Oscillospira. In our study, the anti-obesity effect of L. paracasei AO356 and enriched Oscillospira was correlated with the biosynthesis of unsaturated fatty acids. Unsaturated fatty acids are a type of fatty acid that have one or more double bonds in their chemical structure. Omega-3 fatty acids are well known unsaturated fatty acids and have health beneficial properties such as anti-inflammatory effects and hypotriglyceridemia. They also improve body composition and obesity-related metabolic changes including lipid metabolism (44, 45). Conjugated linoleic acid (CLA), one of the unsaturated fatty acids, has positive health effects on body composition and blood lipid concentrations (46). Diets rich in unsaturated fatty acids improve blood glucose control (47). Given that Oscillospira, obesity-related markers and metabolic pathways are correlated, and in light of previous literature, further research should be conducted to determine whether the anti-obesity effect of L. paracasei AO356 is caused by gut microbial changes.
Butanoate, also known as butyrate, and propanoate, also known as propionate, are short-chain fatty acids that are produced by bacteria in the gut during the fermentation of dietary fiber (48). Butanoate metabolism involves the breakdown of butanoate into acetyl-CoA, which can then be used as a source of energy by the body. Propanoate metabolism involves the conversion of succinate to methylmalonyl-CoA, which can then enter the citric acid cycle to produce energy. Butanoate and propanoate metabolism are important for maintaining gut health by reducing inflammation and improving gut function and energy metabolism (49–51). In this study, we found a positive correlation between enriched carbohydrate metabolism-related pathways (butanoate metabolism and propanoate metabolism) and Oscillospira after the L. paracasei AO356 intervention. Similar to our result, a study showed that Pleurotus ostreatus had a preventive effect on obesity and beneficially modulated gut microbiota, including increased relative abundance of Oscillospira and the butanoate and propanoate metabolism pathway (52). Other studies also showed that propanoate metabolism was enriched after Lactobacillus plantarum HNU082 intervention in an HFD-induced hyperlipidemia rat model. Propanoate metabolism was also linked to improvements in the gut microbiota and the prevention of hyperlipidemia (53). Our results showed that an enriched propanoate metabolism pathway after L. paracasei AO356 intervention was negatively correlated with serum triglycerides. Further studies to determine whether Oscillospira has an anti-obesity effect via the butanoate and propanoate metabolism pathways will help elucidate the mechanism of the anti-obesity effect of L. paracasei AO356.
Additionally, we found that the isoflavonoid biosynthesis pathway was enriched after the L. paracasei AO356 intervention. Isoflavonoids are a class of phytoestrogens that are found in soybeans and other legumes and have been associated with various health benefits, including anti-inflammatory and anti-cancer effects (54). Recent research has also highlighted the potential role of isoflavonoids in the regulation of body weight and metabolism. For example, a meta-analysis study with 17 trials found that isoflavonoids tended to decrease body mass index (55). An animal study found that isoflavonoids decreased body weight and adipose tissue weight (56). The mechanism of action of isoflavonoids on obesity was found to be the inhibition of fat production and increased fatty acid β-oxidation, which leads to reduced body fat (57, 58). Another suggested mechanism is that isoflavonoids interact with intracellular estrogen receptors, which results in reductions in the accumulation of lipids and the distribution of adipose tissue (59). Additionally, the isoflavonoid biosynthesis pathway includes the metabolism of isoflavonoids, such as daidzein and genistein, into more bioactive compounds, such as equol, which has been shown to have anti-obesity effects (60–62). Conversion of isoflavonoids into bioactive compounds mediated by gut microbiota is also proposed as one of the mechanisms of action of isoflavonoids on obesity. Overall, our findings suggest that the anti-obesity effects of L. paracasei AO356 may be mediated, at least in part, by changes in gut microbiota function, including the enrichment of the isoflavonoid biosynthesis pathway. However, further studies are needed to elucidate the role of the isoflavonoid biosynthesis pathway in the anti-obesity effect of L. paracasei AO356.
Recently, as side effects from taking probiotics or migration of antibiotic resistance genes through probiotic strains have become a problem, each country presented guidelines for the safety of probiotics (19). In previous studies, the safety of L. paracasei AO356 was evaluated before the presentation of the guideline from the Korea Ministry of Food and Drug Safety (MFDS) (24). To evaluate the safety, the study conducted including antibiotics resistance tests, a hemolytic test, and enzyme (gelatinase and β-glucuronidase) activity tests in previous study. In 2021, MFDS presented guidelines that included the evaluation of antibiotics resistance, hemolysis activity, bile salt hydrolase (BSH) activity, toxicity, and D-lactate production (19). Therefore, we additionally evaluated the safety of L. paracasei AO356 according to the MFDS guideline. Some strains of lactic acid bacteria (LAB) isolated from fermented foods have been reported to have resistance to antibiotics including erythromycin and tetracycline (63, 64). In addition, LAB play role as a storage for antibiotic-resistant genes and can transfer them to other bacteria (65). Antibiotic resistance is an important for health issue because it can increase morbidity and mortality in patients. For this reason, there is an increasing concern in antibiotic-resistant bacteria in the field of foods and medicine, and antibiotic resistance must be confirmed for the safety of probiotics. In our study, the assessment of antibiotic resistance with E-test demonstrated that L. paparacasei AO356 is a safe strain due to its sensitivity accorded with the EFSA-presented antibiotics guidance for L. paracasei species. Hemolysis, which is virulence factor, is a lysis of red blood cells by hemolysins secreted by bacteria. In particular, the absence of hemolytic activity is a critical factor in probiotics selection because beta-hemolysis could lead hemolytic anemia by destroying red blood cells (66). Also, hemolysis affects the innate and adaptive immune system and people with chronic inflammatory diseases are particularly vulnerable to infection (67). As with the results that most LAB strains had no hemolytic activity, it was observed that L. paracasei AO356 also did not have a hemolytic activity (68). BSH produced by intestinal bacteria is involved in the first steps of bile acid transformation in catalyzing hydrolysis of conjugated bile slats (69). The BSH activity has been reported to be related to functional activity such as serum cholesterol reduction and anti-obesity activity (70); However, there are some negative concerns regarding BSH activity, such as the potential production of cytotoxic and carcinogenic secondary bile salts (71). The enriched deconjugated bile acids by BSH can lead to diarrhea, promoted intestinal inflammation, cholestasis (72). Furthermore, deconjugated bile acids are subsequently transformed into secondary bile acids such as deoxycholic acid (DCA) and lithocholic acid (LCA), by 7-α-dehydroxylase (69). These secondary bile acids have been reported to be cytotoxic and co-carconogenic in vitro, and related to colorectal cancer (71, 73). In this study, we confirmed that L. paracasei AO356 have no BSH activity and it indicated that L. paracasei AO356 with minimal stability concerns in toxic substances produced by BSH. Other toxin and virulence properties were confirmed through the cytotoxicity of the L. paracasei AO356 for Caco-2 cell line. The Cytotoxicity was verified by measurement of LDH concentration released from damaged cells (74). E.coli (ACTC1682) is known to be toxic and induced cell damage similar to treated cell lysis buffer, but our finding demonstrated athat L. paracasei AO356 showed no toxicity to Caco-2 cell line. Finally, we evaluated the capacity to produce D-lactate of L. paracasei AO356. Some Lactobacillus spp. can produce D-lactate as well as L-lactate. In human, while L-lactate is rapidly metabolized to pyruvate, D-lactate is restrictively metabolized by D-α-hydroxy acid dehydrogenase, an enzyme produced by intestinal microbes (75). The excessive accumulation of D-lacatate in blood (≥3 mmol/L in healthy people or 2.5-3.0 mmol/L in patient with short bowel syndrome) can lead to D-lactic acidosis accompanied by various neurologic manifestations (75). Particularly, it could be severe problems in infants an in patients with short bowel syndrome. Thus, the FAO/WHO requires information about D-lactate production to assess the safety of probiotics, and recommended that the product label should specify the information about strains that produce D-lacate (76, 77). In this study, we confirmed that L. paracasei AO356 mainly produced L-lactate and rarely produced D-lactate, which is similar to L. rhamnosus GG, commercial probiotics. L. paracasei AO356 has secured safety for all indicators presented by the guideline. These findings indicated that L. paracasei AO356 meets the safety criteria for probiotics recommended by EFSA and MFDA, thereby suggesting its potential as safe probiotics.
In summary, we showed that the L. paracasei AO356 intervention attenuated the development of HFD-induced obesity in mice. The L. paracasei AO356 intervention also modulated gut microbiota, which may be associated with anti-obesity related markers, biosynthesis of other secondary metabolites-related pathways (isoflavonoid biosynthesis), carbohydrate metabolism-related pathways (butanoate metabolism, propanoate metabolism, and pentose phosphate metabolism) and lipid metabolism-related pathways (biosynthesis of unsaturated fatty acids, fatty acid metabolism, and ether lipid metabolism). L. paracasei AO356 with anti-obesity effects can modulate gut microbiota, however more accurate mechanisms should be studied to evaluate whether the anti-obesity effect is caused by changes in gut microbiota induced by L. paracasei AO356. Moreover, L. paracasei AO356 conformed to the safety assessment guides presented by the MFDS and is therefore suitable for the development of probiotics as a healthy functional food and especially as an alternative therapeutic target for obesity prevention and treatment.
Data availability statement
The datasets presented in this study can be found in online repositories. The names of the repository/repositories and accession number(s) can be found below: https://www.ddbj.nig.ac.jp/, DRA006554.
Author contributions
E-JS and E-SL analyzed and interpreted the data, and drafted the manuscript. YK acquired and analyzed the data, D-US and J-EE acquired the data, and HS conceptualized and supervised the research. S-YL and Y-DM conceptualized and supervised the research and performed a critical revision of the manuscript. All authors contributed to the article and approved the submitted version.
Funding
This research was supported by the Research Project (E0170600-07) of the Korea Food Research Institute (KFRI).
Conflict of interest
The authors declare that the research was conducted in the absence of any commercial or financial relationships that could be construed as a potential conflict of interest.
Publisher’s note
All claims expressed in this article are solely those of the authors and do not necessarily represent those of their affiliated organizations, or those of the publisher, the editors and the reviewers. Any product that may be evaluated in this article, or claim that may be made by its manufacturer, is not guaranteed or endorsed by the publisher.
Supplementary material
The Supplementary Material for this article can be found online at: https://www.frontiersin.org/articles/10.3389/fendo.2023.1224636/full#supplementary-material
References
1. Romieu I, Dossus L, Barquera S, Blottière HM, Franks PW, Gunter M, et al. Energy balance and obesity: what are the main drivers? Cancer Causes Control (2017) 28(3):247–58. doi: 10.1007/s10552-017-0869-z
2. Zhao L. The gut microbiota and obesity: from correlation to causality. Nat Rev Microbiol (2013) 11(9):639–47. doi: 10.1038/nrmicro3089
3. Muscogiuri G, Cantone E, Cassarano S, Tuccinardi D, Barrea L, Savastano S, et al. Gut microbiota: a new path to treat obesity. Int J Obes Suppl (2019) 9(1):10–9. doi: 10.1038/s41367-019-0011-7
4. Turnbaugh PJ, Ley RE, Mahowald MA, Magrini V, Mardis ER, Gordon JI. An obesity-associated gut microbiome with increased capacity for energy harvest. Nature (2006) 444(7122):1027–31. doi: 10.1038/nature05414
5. Turnbaugh PJ, Gordon JI. The core gut microbiome, energy balance and obesity. J Physiol (2009) 587(Pt 17):4153–8. doi: 10.1113/jphysiol.2009.174136
6. Thaiss CA, Zmora N, Levy M, Elinav E. The microbiome and innate immunity. Nature (2016) 535:65. doi: 10.1038/nature18847
7. FAO/WHO. Evaluation of health and nutritional properties of powder milk and live lactic acid bacteria. FAO food and nutrition paper (2001) 85, 1–4.
8. Liu Y, Wang J, Wu C. Modulation of gut microbiota and immune system by probiotics, pre-biotics, and post-biotics. Front Nutr (2021) 8:634897. doi: 10.3389/fnut.2021.634897
9. Kim YI, Lee E-S, Song E-J, Shin D-U, Eom J-E, Shin HS, et al. Lacticaseibacillus paracasei AO356 ameliorates obesity by regulating adipogenesis and thermogenesis in C57BL/6J male mice. J Funct Foods (2023) 101:105404. doi: 10.1016/j.jff.2023.105404
10. Bubnov RV, Babenko LP, Lazarenko LM, Mokrozub VV, Demchenko OA, Nechypurenko OV, et al. Comparative study of probiotic effects of Lactobacillus and Bifidobacteria strains on cholesterol levels, liver morphology and the gut microbiota in obese mice. EPMA J (2017) 8(4):357–76. doi: 10.1007/s13167-017-0117-3
11. Park D-Y, Ahn Y-T, Park S-H, Huh C-S, Yoo S-R, Yu R, et al. Supplementation of Lactobacillus curvatus HY7601 and Lactobacillus plantarum KY1032 in Diet-Induced Obese Mice Is Associated with Gut Microbial Changes and Reduction in Obesity. PloS One (2013) 8(3):e59470. doi: 10.1371/journal.pone.0059470
12. Huang Y, Wang J, Quan G, Wang X, Yang L, Zhong L. Lactobacillus acidophilus ATCC 4356 prevents atherosclerosis via inhibition of intestinal cholesterol absorption in apolipoprotein E-knockout mice. Appl Environ Microbiol (2014) 80(24):7496. doi: 10.1128/AEM.02926-14
13. Aronsson L, Huang Y, Parini P, Korach-André M, Håkansson J, Gustafsson J-Å, et al. Decreased fat storage by lactobacillus paracasei is associated with increased levels of angiopoietin-like 4 protein (ANGPTL4). PloS One (2010) 5(9):e13087. doi: 10.1371/journal.pone.0013087
14. Ma X, Hua J, Li Z. Probiotics improve high fat diet-induced hepatic steatosis and insulin resistance by increasing hepatic NKT cells. J Hepatology (2008) 49(5):821–30. doi: 10.1016/j.jhep.2008.05.025
15. Castañeda-Márquez AC, Díaz-Benítez CE, Bahena-ROman M, Campuzano-Benítez GE, Galván-Portillo M, Campuzano-Rincón JC, et al. Lactobacillus paracasei as a protective factor of obesity induced by an unhealthy diet in children. Obes Res Clin Pract (2020) 14(3):271–8. doi: 10.1016/j.orcp.2020.04.005
16. Cheng MC, Tsai TY, Pan TM. Anti-obesity activity of the water extract of Lactobacillus paracasei subsp. paracasei NTU 101 fermented soy milk products Food Funct (2015) 6(11):3522–30. doi: 10.1039/c5fo00531k
17. Tanida M, Shen J, Maeda K, Horii Y, Yamano T, Fukushima Y, et al. High-fat diet-induced obesity is attenuated by probiotic strain Lactobacillus paracasei ST11 (NCC2461) in rats. Obes Res Clin Pract (2008) 2(3):159–69. doi: 10.1016/j.orcp.2008.04.003
18. Miao Z, Zheng H, Liu W-H, Cheng R, Lan H, Sun T, et al. Lacticaseibacillus paracasei K56 attenuates high-fat diet-induced obesity by modulating the gut microbiota in mice. Probiotics Antimicrobial Proteins (2022) 15(4):844–55. doi: 10.1007/s12602-022-09911-x
19. MFDS. Health functional food functional ingredient probiotics safety evaluation guide. Cheongju-si, Chungcheongbuk-do, Republic of Korea: Safety MoFaD (2021).
20. Cacho J, Sevillano J, de Castro J, Herrera E, Ramos MP. Validation of simple indexes to assess insulin sensitivity during pregnancy in Wistar and Sprague-Dawley rats. Am J Physiol - Endocrinol And Metab (2008) 295(5):E1269–E76. doi: 10.1152/ajpendo.90207.2008
21. Lim MY, Song EJ, Kim SH, Lee J, Nam YD. Comparison of DNA extraction methods for human gut microbial community profiling. Systematic Appl Microbiol (2017) 41(2):151–7. doi: 10.1016/j.syapm.2017.11.008
22. Caporaso JG, Kuczynski J, Stombaugh J, Bittinger K, Bushman FD, Costello EK, et al. QIIME allows analysis of high-throughput community sequencing data. Nat Methods (2010) 7(5):335–6. doi: 10.1038/nmeth.f.303
23. Langille MG, Zaneveld J, Caporaso JG, McDonald D, Knights D, Reyes JA, et al. Predictive functional profiling of microbial communities using 16S rRNA marker gene sequences. Nat Biotechnol (2013) 31(9):814–21. doi: 10.1038/nbt.2676
24. Lee S-Y, Shin D-U, Kim SY, Eom J-E, Nam Y-D, Shin H-S, et al. Assessment the of anti-obesity effects and safety of Lactobacillus paracasei AO356. J Korean Society of Food Science and Nutrition (2021) 50(9):904–11. doi: 10.3746/jkfn.2021.50.9.904
26. Begley M, Hill C, Gahan CG. Bile salt hydrolase activity in probiotics. Appl Environ Microbiol (2006) 72(3):1729–38. doi: 10.1128/AEM.72.3.1729-1738.2006
27. Dixon P. VEGAN, a package of R functions for community ecology. J Vegetation Sci (2003) 14(6):927–30. doi: 10.1111/j.1654-1103.2003.tb02228.x
28. Segata N, Izard J, Waldron L, Gevers D, Miropolsky L, Garrett WS, et al. Metagenomic biomarker discovery and explanation. Genome Biol (2011) 12(6):1. doi: 10.1186/gb-2011-12-6-r60
29. Safety MoFaD. Guidelines for the Evaluation of Probiotics in Food. Cheongju-si, Chungcheongbuk-do, Republic of Korea: Safety MoFaD (2021). Available at: https://www.mfds.go.kr/2021.
30. Smits SA, Leach J, Sonnenburg ED, Gonzalez CG, Lichtman JS, Reid G, et al. Seasonal cycling in the gut microbiome of the Hadza hunter-gatherers of Tanzania. Science (2017) 357(6353):802–6. doi: 10.1126/science.aan4834
31. Wu GD, Chen J, Hoffmann C, Bittinger K, Chen Y-Y, Keilbaugh SA, et al. Linking long-term dietary patterns with gut microbial enterotypes. Science (2011) 334(6052):105–8. doi: 10.1126/science.1208344
32. Patil DP, Dhotre DP, Chavan SG, Sultan A, Jain DS, Lanjekar VB, et al. Molecular analysis of gut microbiota in obesity among Indian individuals. J Biosci (2012) 37(4):647–57. doi: 10.1007/s12038-012-9244-0
33. Hjorth MF, Blædel T, Bendtsen LQ, Lorenzen JK, Holm JB, Kiilerich P, et al. Prevotella-to-Bacteroides ratio predicts body weight and fat loss success on 24-week diets varying in macronutrient composition and dietary fiber: results from a post-hoc analysis. Int J Obes (2019) 43(1):149–57. doi: 10.1038/s41366-018-0093-2
34. Hjorth MF, Roager HM, Larsen TM, Poulsen SK, Licht TR, Bahl MI, et al. Pre-treatment microbial Prevotella-to-Bacteroides ratio, determines body fat loss success during a 6-month randomized controlled diet intervention. Int J Obes (Lond) (2018) 42(3):580–3. doi: 10.1038/ijo.2017.220
35. Song EJ, Han K, Lim TJ, Lim S, Chung MJ, Nam MH, et al. Effect of probiotics on obesity-related markers per enterotype: a double-blind, placebo-controlled, randomized clinical trial. Epma J (2020) 11(1):31–51. doi: 10.1007/s13167-020-00198-y
36. Zou H, Wang D, Ren H, Cai K, Chen P, Fang C, et al. Effect of caloric restriction on BMI, gut microbiota, and blood amino acid levels in non-obese adults. Nutrients (2020) 12(3):631. doi: 10.3390/nu12030631
37. Goodrich Julia K, Waters Jillian L, Poole Angela C, Sutter Jessica L, Koren O, Blekhman R, et al. Human genetics shape the gut microbiome. Cell (2014) 159(4):789–99. doi: 10.1016/j.cell.2014.09.053
38. Verdam FJ, Fuentes S, de Jonge C, Zoetendal EG, Erbil R, Greve JW, et al. Human intestinal microbiota composition is associated with local and systemic inflammation in obesity. Obesity (2013) 21(12):E607–E15. doi: 10.1002/oby.20466
39. Tims S, Derom C, Jonkers DM, Vlietinck R, Saris WH, Kleerebezem M, et al. Microbiota conservation and BMI signatures in adult monozygotic twins. ISME J (2013) 7(4):707–17. doi: 10.1038/ismej.2012.146
40. Escobar JS, Klotz B, Valdes BE, Agudelo GM. The gut microbiota of Colombians differs from that of Americans, Europeans and Asians. BMC Microbiol (2014) 14(1):311. doi: 10.1186/s12866-014-0311-6
41. de la Cuesta-Zuluaga J, Corrales-Agudelo V, Carmona JA, Abad JM, Escobar JS. Body size phenotypes comprehensively assess cardiometabolic risk and refine the association between obesity and gut microbiota. Int J Obes (2018) 42(3):424–32. doi: 10.1038/ijo.2017.281
42. Yang J, Li Y, Wen Z, Liu W, Meng L, Huang H. Oscillospira - a candidate for the next-generation probiotics. Gut Microbes (2021) 13(1):1987783. doi: 10.1080/19490976.2021.1987783
43. Chen Y-R, Zheng H-M, Zhang G-X, Chen F-L, Chen L-D, Yang Z-C. High Oscillospira abundance indicates constipation and low BMI in the Guangdong Gut Microbiome Project. Sci Rep (2020) 10(1):9364. doi: 10.1038/s41598-020-66369-z
44. Albracht-Schulte K, Kalupahana NS, RaMalingam L, Wang S, Rahman SM, Robert-McComb J, et al. Omega-3 fatty acids in obesity and metabolic syndrome: a mechanistic update. J Nutr Biochem (2018) 58:1–16. doi: 10.1016/j.jnutbio.2018.02.012
45. Swanson D, Block R, Mousa SA. Omega-3 fatty acids EPA and DHA: health benefits throughout life. Adv Nutr (2012) 3(1):1–7. doi: 10.3945/an.111.000893
46. Roche HM, Noone E, Gibney ANMJ. Conjugated linoleic acid: a novel therapeutic nutrient? Nutr Res Rev (2001) 14(1):173–88. doi: 10.1079/095442201108729187
47. Imamura F, Micha R, Wu JH, de Oliveira Otto MC, Otite FO, Abioye AI, et al. Effects of saturated fat, polyunsaturated fat, monounsaturated fat, and carbohydrate on glucose-insulin homeostasis: A systematic review and meta-analysis of randomised controlled feeding trials. PloS Med (2016) 13(7):e1002087. doi: 10.1371/journal.pmed.1002087
48. Koh A, De Vadder F, Kovatcheva-Datchary P, Bäckhed F. From dietary fiber to host physiology: short-chain fatty acids as key bacterial metabolites. Cell (2016) 165(6):1332–45. doi: 10.1016/j.cell.2016.05.041
49. Parada Venegas D, de la Fuente MK, Landskron G, González MJ, Quera R, Dijkstra G, et al. Short chain fatty acids (SCFAs)-mediated gut epithelial and immune regulation and its relevance for inflammatory bowel diseases. Front Immunol (2019) 10:277. doi: 10.3389/fimmu.2019.00277
50. Ríos-Covián D, Ruas-Madiedo P, Margolles A, Gueimonde M, de Los Reyes-Gavilán CG, Salazar N. Intestinal short chain fatty acids and their link with diet and human health. Front Microbiol (2016) 7:185. doi: 10.3389/fmicb.2016.00185
51. Morrison DJ, Preston T. Formation of short chain fatty acids by the gut microbiota and their impact on human metabolism. Gut Microbes (2016) 7(3):189–200. doi: 10.1080/19490976.2015.1134082
52. Hu Y, Xu J, Sheng Y, Liu J, Li H, Guo M, et al. Pleurotus ostreatus ameliorates obesity by modulating the gut microbiota in obese mice induced by high-fat diet. Nutrients (2022) 14(9):1868. doi: 10.3390/nu14091868
53. Shao Y, Huo D, Peng Q, Pan Y, Jiang S, Liu B, et al. Lactobacillus plantarum HNU082-derived improvements in the intestinal microbiome prevent the development of hyperlipidaemia. Food Funct (2017) 8(12):4508–16. doi: 10.1039/C7FO00902J
54. Messina M. Soy and health update: evaluation of the clinical and epidemiologic literature. Nutrients (2016) 8(12):754. doi: 10.3390/nu8120754
55. Akhlaghi M, Zare M, Nouripour F. Effect of soy and soy isoflavones on obesity-related anthropometric measures: A systematic review and meta-analysis of randomized controlled clinical trials. Adv Nutr (2017) 8(5):705–17. doi: 10.3945/an.117.015370
56. Yao Y, Li X-B, Zhao W, Zeng Y-Y, Shen H, Xiang H, et al. Anti-obesity effect of an isoflavone fatty acid ester on obese mice induced by high fat diet and its potential mechanism. Lipids Health Dis (2010) 9(1):49. doi: 10.1186/1476-511X-9-49
57. Ørgaard A, Jensen L. The effects of soy isoflavones on obesity. Exp Biol Med (Maywood NJ) (2008) 233(9):1066–80. doi: 10.3181/0712-MR-347
58. Akhlaghi M. Non-alcoholic fatty liver disease: beneficial effects of flavonoids. Phytother Res (2016) 30(10):1559–71. doi: 10.1002/ptr.5667
59. Wang S, Wang Y, Pan M-H, Ho C-T. Anti-obesity molecular mechanism of soy isoflavones: weaving the way to new therapeutic routes. Food Funct (2017) 8(11):3831–46. doi: 10.1039/C7FO01094J
60. Mayo B, Vázquez L, Flórez AB. Equol: A bacterial metabolite from the daidzein isoflavone and its presumed beneficial health effects. Nutrients (2019), 11(9):2231. doi: 10.3390/nu11092231
61. Usui T, Tochiya M, Sasaki Y, Muranaka K, Yamakage H, Himeno A, et al. Effects of natural S-equol supplements on overweight or obesity and metabolic syndrome in the Japanese, based on sex and equol status. Clin Endocrinol (Oxf) (2013) 78(3):365–72. doi: 10.1111/j.1365-2265.2012.04400.x
62. Charles C, Yuskavage J, Carlson O, John M, Tagalicud AS, Maggio M, et al. Effects of high-dose isoflavones on metabolic and inflammatory markers in healthy postmenopausal women. Menopause (2009) 16(2):395–400. doi: 10.1097/gme.0b013e3181857979
63. Coppola R, Succi M, Tremonte P, Reale A, Salzano G, Sorrentino E. Antibiotic susceptibility of Lactobacillus rhamnosus strains isolated from Parmigiano Reggiano cheese. Le Lait (2005) 85(3):193–204. doi: 10.1051/lait:2005007
64. Dec M, Urban-Chmiel R, Stępień-Pyśniak D, Wernicki A. Assessment of antibiotic susceptibility in Lactobacillus isolates from chickens. Gut pathogens (2017) 9(1):1–16. doi: 10.1186/s13099-017-0203-z
65. Nawaz M, Wang J, Zhou A, Ma C, Wu X, Moore JE, et al. Characterization and transfer of antibiotic resistance in lactic acid bacteria from fermented food products. Curr Microbiol (2011) 62:1081–9. doi: 10.1007/s00284-010-9856-2
66. Sohail M. Effects of different types of microbes on blood cells, current perspectives and future directions. Saudi J Med Pharm Sci (2021) 7(1):1–6. doi: 10.36348/sjmps.2021.v07i01.001
67. Zhong H, Yazdanbakhsh K. Hemolysis and immune regulation. Curr Opin hematology (2018) 25(3):177. doi: 10.1097/MOH.0000000000000423
68. Casarotti SN, Carneiro BM, Todorov SD, Nero LA, Rahal P, Penna ALB. In vitro assessment of safety and probiotic potential characteristics of Lactobacillus strains isolated from water buffalo mozzarella cheese. Ann Microbiol (2017) 67:289–301. doi: 10.1007/s13213-017-1258-2
69. Huang C-H, Ho C-Y, Chen C-T, Hsu H-F, Lin Y-H. Probiotic BSH activity and anti-obesity potential of Lactobacillus plantarum strain TCI378 isolated from Korean Kimchi. Prev Nutr Food science (2019) 24(4):434. doi: 10.3746/pnf.2019.24.4.434
70. Liong M, Shah N. Bile salt deconjugation ability, bile salt hydrolase activity and cholesterol co-precipitation ability of lactobacilli strains. Int Dairy J (2005) 15(4):391–8. doi: 10.1016/j.idairyj.2004.08.007
71. Marteau P, Gerhardt M, Myara A, Bouvier E, Trivin F, Rambaud J. Metabolism of bile salts by alimentary bacteria during transit in the human small intestine. Microbial Ecol Health disease (1995) 8(4):151–7. doi: 10.3109/08910609509140093
72. King CE, Toskes PP. Small intestine bacterial overgrowth. Gastroenterology (1979) 76(5):1035–55. doi: 10.1016/S0016-5085(79)91337-4
73. Carpenedo R, Mannaioni G, Moroni F. Oxindole, a sedative tryptophan metabolite, accumulates in blood and brain of rats with acute hepatic failure. J neurochemistry (1998) 70(5):1998–2003. doi: 10.1046/j.1471-4159.1998.70051998.x
74. Aslantürk ÖS. In vitro cytotoxicity and cell viability assays: principles, advantages, and disadvantages. Genotoxicity-A predictable Risk to our actual World (2018) 2:64–80. doi: 10.5772/intechopen.71923
75. Ewaschuk JB, Naylor JM, Zello GA. D-lactate in human and ruminant metabolism. J Nutr (2005) 135(7):1619–25. doi: 10.1093/jn/135.7.1619
76. Ban OH, Bang WY, Jeon HJ, Jung YH, Yang J, Kim DH. Potential of Bifidobacterium lactis IDCC 4301 isolated from breast milk-fed infant feces as a probiotic and functional ingredient. Food Sci Nutr (2023) 11(4):1952–64. doi: 10.1002/fsn3.3230
Keywords: lacticaseibacillus paracasei, lab, probiotics, gut microbiota, obesity
Citation: Song E-J, Lee E-S, Kim YI, Shin D-U, Eom J-E, Shin HS, Lee S-Y and Nam Y-D (2023) Gut microbial change after administration of Lacticaseibacillus paracasei AO356 is associated with anti-obesity in a mouse model. Front. Endocrinol. 14:1224636. doi: 10.3389/fendo.2023.1224636
Received: 18 May 2023; Accepted: 14 August 2023;
Published: 29 August 2023.
Edited by:
Xu Zhang, Health Canada, CanadaReviewed by:
Siti Wulan, University of Brawijaya, IndonesiaCarina Shianya Alvarez Villagomez, Universidad Juárez Autónoma de Tabasco, Mexico
Copyright © 2023 Song, Lee, Kim, Shin, Eom, Shin, Lee and Nam. This is an open-access article distributed under the terms of the Creative Commons Attribution License (CC BY). The use, distribution or reproduction in other forums is permitted, provided the original author(s) and the copyright owner(s) are credited and that the original publication in this journal is cited, in accordance with accepted academic practice. No use, distribution or reproduction is permitted which does not comply with these terms.
*Correspondence: Young-Do Nam, youngdo98@kfri.re.kr; So-Young Lee, sylee09@kfri.re.kr
†These authors have contributed equally to this work