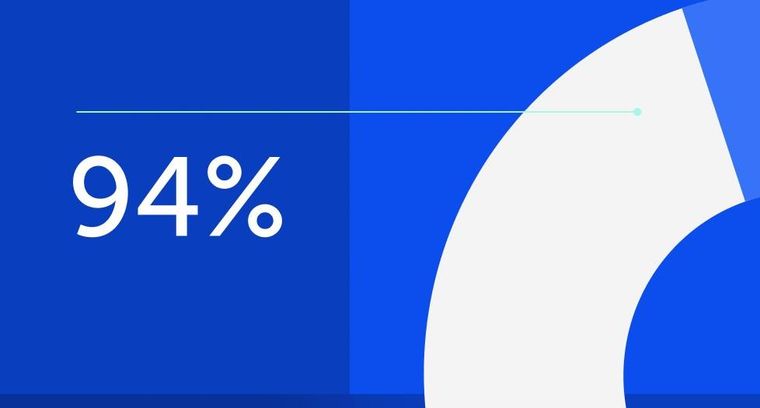
94% of researchers rate our articles as excellent or good
Learn more about the work of our research integrity team to safeguard the quality of each article we publish.
Find out more
ORIGINAL RESEARCH article
Front. Endocrinol., 27 February 2023
Sec. Diabetes: Molecular Mechanisms
Volume 14 - 2023 | https://doi.org/10.3389/fendo.2023.1115619
This article is part of the Research TopicA Year in Review: Discussions in Molecular and Structural EndocrinologyView all 14 articles
A retraction of this article was approved in:
Retraction: High Glucose Induced HIF-1α/TREK1 Expression and Myometrium Relaxation During Pregnancy
RETRACTED: Erratum: High glucose induced HIF-1α/TREK1 expression and myometrium relaxation during pregnancy
Citation: Li T, Fei J, Yu H, Wang X, Bai J, Chen F, Li D and Yin Z (2023) High glucose induced HIF-1α/TREK1 expression and myometrium relaxation during pregnancy. Front. Endocrinol. 14:1115619. doi: 10.3389/fendo.2023.1115619
Received: 04 December 2022; Accepted: 13 February 2023;
Published: 27 February 2023; Retracted: 14 March 2025.
Edited by:
Katsumi Iizuka, Fujita Health University, JapanReviewed by:
Ken Takao, Gifu University Hospital, JapanDisclaimer: All claims expressed in this article are solely those of the authors and do not necessarily represent those of their affiliated organizations, or those of the publisher, the editors and the reviewers. Any product that may be evaluated in this article or claim that may be made by its manufacturer is not guaranteed or endorsed by the publisher.
Research integrity at Frontiers
Learn more about the work of our research integrity team to safeguard the quality of each article we publish.